- 1Department of Endocrinology and Metabolism, Affiliated Hospital of Weifang Medical University, Weifang, China
- 2Clinical Research Center, Affiliated Hospital of Weifang Medical University, Weifang, China
- 3Department of Pathology, Affiliated Hospital of Weifang Medical University, Weifang, China
- 4School of Pharmacy, Weifang Medical University, Weifang, China
Calycosin, one of small molecules derived from astragalus, has anti-tumor effects in various tumors. However, the effects of calycosin on papillary thyroid cancer (PTC) remain unclear. This study aimed to explore the anti-tumor ability of calycosin on human PTC and its potential mechanisms. The B-CPAP cells were treated with calycosin, then cell proliferation, apoptosis and invasiveness were measured by CCK8 assay, flow cytometry, wound healing and transwell invasion assay, respectively. The cells were also performed by whole transcriptome microarray bioinformatics analysis. Apoptosis and autophagy-related markers or proteins were measured by qRT-PCR or western blot. Sestrin2-mediated AMPK/mTOR pathways were determined by western blot. We found that calycosin inhibited migration and invasion of B-CPAP cells and induced apoptosis (Bax/Bcl-2) and autophagy (LC3II/I, Beclin1) of B-CPAP cells. Differential expressed genes were screened between the calycosin-treated cells and control (524 genes upregulated and 328 genes downregulated). The pathway enrichment suggested that the role of calycosin in B-CPAP cells is closely related to apoptosis-related genes and p70S6 Kinase. Transmission electron microscopy found an increase in autophagosomes in calycosin-treated cells. Sestrin2 in human PTC tissues and B-CPAP cells was lower than in normal thyroid tissues and cells. And the pharmacological effects of calycosin in PTC cells were related to Sestrin2 activation, increased p-AMPK and inhibited p-mTOR and p-p70S6Kinase; these alterations were reversed when silencing Sestrin2. In conclusion, calycosin has an inhibitory effect on PTC via promoting apoptosis and autophagy through the Sestrin2/AMPK/mTOR pathway.
Introduction
Thyroid cancer is the most common endocrine tumor and head and neck cancer with increasing incidence (Siegel et al., 2021). Papillary thyroid cancer (PTC) is the type with the largest proportion, accounting for about 85%–90% of all thyroid cancers (Wells Jr, 2016). PTC is a pathological type of thyroid cancer with good differentiation and high survival rates and is usually inert and curable with a 5-year survival rate >95% (Schlumberger, 1998). However, in some cases, about 30% of PTCs still dedifferentiate and become aggressive, leading to tumor recurrence and distant metastases (Beierwaltes et al., 1982). Surgical resection remains the primary treatment for PTC, supplemented by radioiodine therapy, thyroid-stimulating hormone suppression therapy, local radiotherapy, and targeted drug therapy (Filetti et al., 2019). Selecting potentially useful drugs is undoubtedly feasible for patients with advanced thyroid cancer or who lose the chance of operation (Ancker et al., 2019). Thus, developing clinically effective drugs based on pathogenesis is essential.
Autophagy, the intracellular degradation process of damaged proteins and organelles, is an alternative way of programmed cell death different from apoptosis (Gudipaty et al., 2018). Autophagy maintains cellular homeostasis and regulates cell growth and death (Li et al., 2021). Autophagy plays a dual role in tumors, promoting or suppressing via regulating apoptosis (Levy et al., 2017). Studies have reported that activating autophagy could inhibit the progression of PTC by inducing apoptosis (Zhang et al., 2015). Moreover, autophagy activators could enhance the chemosensitivity and therapeutic response during PTC treatment (Lin et al., 2010).
Sestrin (SESN) is a highly conserved protein encoded by genes whose cell expression is up-regulated when exposed to DNA damage, oxidative stress and hypoxia (Qu et al., 2021). It includes three isoforms: SESN1, SESN2, and SESN3(Lee et al., 2013). Among them, SESN2 is a downstream target gene of p53, encoded by hypoxia-inducible gene 95 (Maiuri et al., 2009). Mainly expressed in mammals, SESN2 can resist oxidative stress damage, regulate cellular metabolism, and regulate autophagy and apoptosis (Sun et al., 2020). Current studies have shown that Sestrin2 initiates cellular autophagy through the AMP-activated protein kinase (AMPK)/mammalian target of rapamycin complex 1 (mTORC1) pathway, i.e.; SESN2 activates the AMPK pathway and inhibits downstream mTORC1-P70S6K to promote autophagy (Peng et al., 2014; Wolfson et al., 2016). Similar to autophagy, the role of SESN2 in tumors is paradoxical (Qu et al., 2021). Some studies have found that SESN2 is a tumor suppressor gene, such as neuroblastoma (Ambrosio et al., 2017), bladder cancer (Liang et al., 2016), colorectal cancer (Wei et al., 2015), and endometrial cancer (Shin et al., 2020). However, other studies confirm that it is a cancer-promoting gene, such as lung cancer (Byun et al., 2017), osteosarcoma (Tang et al., 2021), and melanoma cells (Zhao et al., 2014). However, the research of Sestrin2 in thyroid cancer is still not fully elucidated.
Calycosin (C16H12O5), one of the main components of astragalus (Gong et al., 2016), has been proven to have anti-inflammatory (Dong et al., 2018), antioxidant (Zhai et al., 2020), neuroprotective (Yang et al., 2019), antitumor (Tian et al., 2017) and vascular protective effects (Han et al., 2018). Its biological effects may be related to its flavonoids. Current research has confirmed that calycosin has anti-tumor properties and can exert its anti-tumor effects through multiple pathways and targets (Wang et al., 2019). Calycosin induced apoptosis via PI3K/AKT/mTOR signaling pathway in osteosarcoma cells (Sun et al., 2018). Moreover, calycosin can induce colorectal cancer cells (HT29) through the SIRT1/AMPK pathway-mediated autophagy (El-Kott et al., 2019). However, the effect of calycosin on autophagy and apoptosis of thyroid cancer is unknown. Thus, this study examined whether calycosin could have antitumor effects and the molecular mechanisms underlying SESN2-mediated autophagy in PTC.
Materials and methods
Cell culture
The human papillary thyroid (B-CPAP) cancer cells and the human normal thyroid cells (Nthy-ori 3-1) were from the National Collection of Authenticated Cell Cultures (Shanghai, China). The medium was fresh RPMI1640 (Gibco, China) with 10% fetal bovine serum and 1% penicillin and streptomycin (Invitrogen, Carlsbad, CA, United States).
Proliferation assay
Calycosin powder dissolved in dimethyl sulfoxide was diluted with RPMI1640 to the appropriate concentration. The cancer cells with 5 × 103 cells/well and cultured with calycosin at various concentrations (0, 25, 50, and 100 μM) for 24 h. Absorbance was measured at 450 nm after using the CCK8 Assay Kit (Sparkjade, China).
Colony formation
800 cells/well of B-CPAP were plated on a six-well plate with calycosin (100 μM) or control (0 μM) for 12 days; cell clones were fixed with methanol for 15 min. Subsequently, the cell clones were stained for 30 min by adding giemsa stain solution (Solarbio, Beijing, China) and photographed.
Wound healing assay
B-CPAP cells were cultured in six-well plates for 24 h to allow cells to confluence; wounds were created through the center line using a pipette tip. Photographs were taken to measure the distance between the wound edges. The culture was then continued for 24 h with calycosin, photographs were taken, and the distance between wound edges was measured by ImageJ software.
Transwell assay
The B-CPAP cells were added into transwell membranes (Corning, NY, United States) at 1 × 104 cells/well in medium and calycosin. The lower chamber of the 24-well plate contains conventional cell culture medium. After 24 h, the upper chambers were fixed with methanol, stained for 45 min by giemsa stain solution, and photographed with a microscope (Olympus, Tokyo, Japan).
Flow cytometry analysis
After being treated with calycosin (100 μM) or control (0 μM) for 24 h, the cells were fixed with 70% ethanol, stained by Annexin V-FITC Apoptosis Detection Kit (BD, United States), and analyzed in FACS with CellQuest software version 3.3.
Transmission electron microscopy
The B-CPAP cells were inoculated to a density of about 80%, treated with calycosin or control group for 24 h, then washed with PBS, fixed with 2% glutaraldehyde, and then photographed and imaged by transmission electron microscopy (HT7800/HT7700, Hitachi, Japan).
Cell transfection
Cell transfection was performed using lipofectamine 3,000 (Invitrogen, United States). siRNA directed against SESN2 (si-SESN2: sense (5′-3′)CCGAAGAAUGUACAACCUCUU, antisense (5′-3′) AAGAGGUUGUACAUUCUUCGG) and negative control siRNA (siNC) were from Qingke Co., Ltd. (Beijing, China). Antibiotic-free medium in six-well plates was used to seed B-CPAP cells as 2.5 × 105 cells/well. si-SESN2 or si-NC (9 μL) and lipofectamine 3,000 reagent was diluted in the same volume of Opti-MEM. Then mix two liquids, incubate for 10 min, and add dropwise to the plate. And the culture was continued until 48 h. Cells were then harvested 24 h after treatment with calycosin (100 μM) or control (0 μM).
qRT-PCR
Total RNA from clinical sample tissues (triturated with a homogenizer on ice)/cell lysates were extracted using RNA TRIzol reagent (Invitrogen, United States) and reverse transcribed using the PrimeScript™ RT Kit (TaKaRa Dalian, China) as instructed and amplified using TB Green ® Premix Ex Taq™ II (#RR820A, TaKaRa). Relative expression levels of mRNA were determined using the 2−ΔΔCt formula, and results were normalized with GAPDH. The primers were from the sangon bioech company (Jinan, China), and the sequences are shown in Table 1.
Western blot
Harvested protein from clinical sample tissue (grind with a homogenizer on ice)/cell by using the whole protein extraction kit (Keygen, China). Then sodium dodecyl sulfate-polyacrylamide gel electrophoresis was performed and transferred to a polyvinylidene fluoride membrane. Results were quantified by ImageJ software (NIH, United States) after being incubated with the primary antibodies overnight. p-AMPK, AMPK, p-mTOR, mTOR, p-P70S6K, and P70S6K antibodies were from Cell Signaling Technology (Massachusetts, United States), LC3 II/I, SESN2, GAPDH and β-actin were from proteintech (State of New Jersey, United States).
Whole transcriptome microarray bioinformatics analysis
The B-CPAP cells were grown with calycosin (0.100 μM) for 24 h and then sent to GENE Matrix for sequencing (http://gcloud.taogene.com). The significantly different genes were screened with |Fold Change|≥1.5 and p-value<0.05 as the criteria, and parallel Gene Ontology (GO) and Kyoto Encyclopedia of Genes and Genomes (KEGG) analyses were performed.
Clinical samples
The selected clinical samples (16 cases of PTC tissues, 16 cases of normal thyroid tissue) in this study were all from the Affiliated Hospital of Weifang Medical University and agreed upon by the patients. This study was approved by the Ethics Committee of Affiliated Hospital of Weifang Medical University.
Statistical analysis
Statistical analyses were from GraphPad Prism 8.0 and expressed as means ± standard errors. Comparisons were assessed using unpaired Student’s t-tests/ANOVA according to different situations. Statistically significant at p < 0.05.
Results
Calycosin inhibited proliferation of B-CPAP cells
To determine whether calycosin has the anti-proliferative effects, the B-CPAP cells grown with calycosin was determined by CCK8 assay. With the increased calycosin concentration, cells' proliferation ability was gradually suppressed (Figure 1A). Afterward, we used a soft agar assay to analyze the effect of calycosin on B-CPAP cell-independent growth. The cell colonies formed by B-CPAP treated with calycosin (100 μM) were significantly less than that of the untreated cells after 12 days of culture, indicating that calycosin can inhibit B-CPAP cell proliferation (Figure 1B).
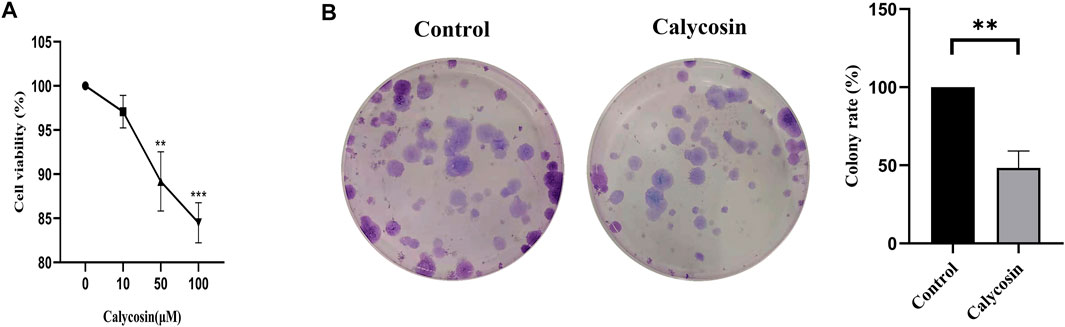
FIGURE 1. Calycosin inhibited the proliferation of B-CPAP cells. (A) CCK8 was used to measure the cell proliferation rate of BCPAP cells incubated with different concentrations of calycosin (0, 10, 50, 100 µM) for 24 h. (B) Colony formation assay was used to measure the number of clones formed in BCPAP cells incubated with calycosin (0, 100 µM) for 12 days. Results are representative of three independent experiments. *p < 0.05, **p < 0.01,***p < 0.001.
Calycosin inhibited migration and invasion of B-CPAP cells
To analyze the effect of calycosin on cell migration and invasion, we further performed wound healing (Figure 2A) and transwell experiments (Figure 2B). With the increasing concentration of calycosin, the wound healing width after 24 h was higher (Figure 2C), and the cell migration and invasion rate were lower (Figure 2D). These data suggested that calycosin significantly inhibited the migration and invasion ability of B-CPAP cells.
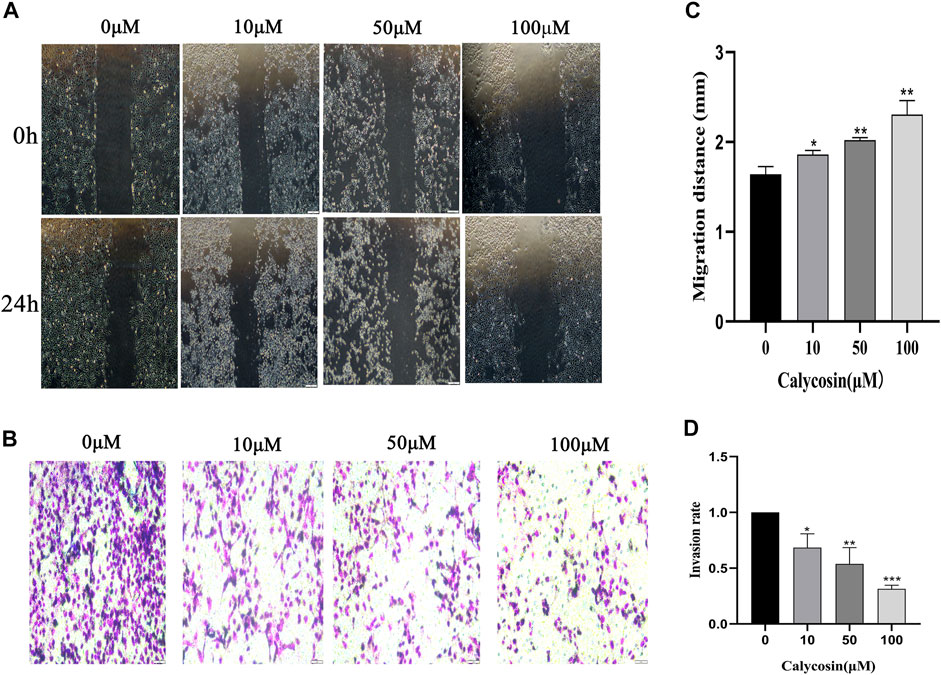
FIGURE 2. Calycosin inhibited the migration and invasion of B-CPAP cells. (A) Wound healing assay results in control or calycosin-treated B-CPAP cells at 0 and 24 h. (C) The distance between wound edges was measured. (B) Transwell invasion assay results showed the invasiveness of B-CPAP cells treated with calycosin. (D) The number of invasion cells was counted with ImageJ software. Results are representative of three independent experiments. *p < 0.05, **p < 0.01,***p < 0.001.
Calycosin induced apoptosis of B-CPAP cells
To identify potential protective mechanisms of calycosin on B-CPAP cells, the cells were grown with calycosin (100 μM) and performed expression microarray sequencing. Differentially expressed genes (DEGs) were screened out between the two groups (524 genes were upregulated and 328 genes were downregulated) (Figures 3A,B), and GO and KEGG of DEGs were analyzed: Adjusted.P.Value < 0.01 (Figures 3C,D). The pathway enrichment suggested that the role of calycosin in B-CPAP is closely related to apoptosis-related genes. To verify this result, we performed flow cytometry analysis (Figure 4A). The apoptosis rate of BCPAP cells treated with calycosin increased (Figure 4B) compared to control group. The relative expression of Bax/Bcl-2 in the calycosin group was higher than in untreated cells (Figure 4C). These results suggested that the calycosin treatment promoted the apoptosis of B-CPAP cells.
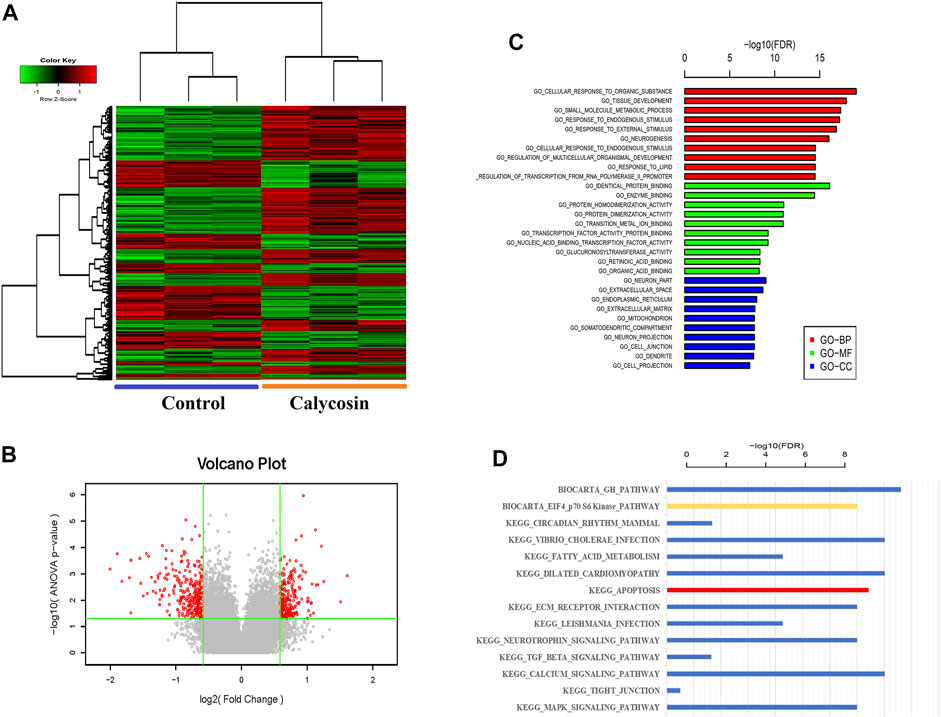
FIGURE 3. Whole Transcriptome Microarray Bioinformatics Analysis. (A) The DEGs of B-CPAP cells were treated with calycosin (0.100 µM) for 24 h. DEG screening criteria were: |Fold Change|≥1.5 and p-value<0.05. (B) Volcano plot: the red dots are points with |Fold Change|≥1.5 and p-value < 0.05 is a significant difference gene for standard screening, and the gray points are other genes with no significant difference. (C) The GO terms with the most significant DEG enrichment are listed. (D) The pathways with the most significant enrichment of KEGG in DEGs are listed.
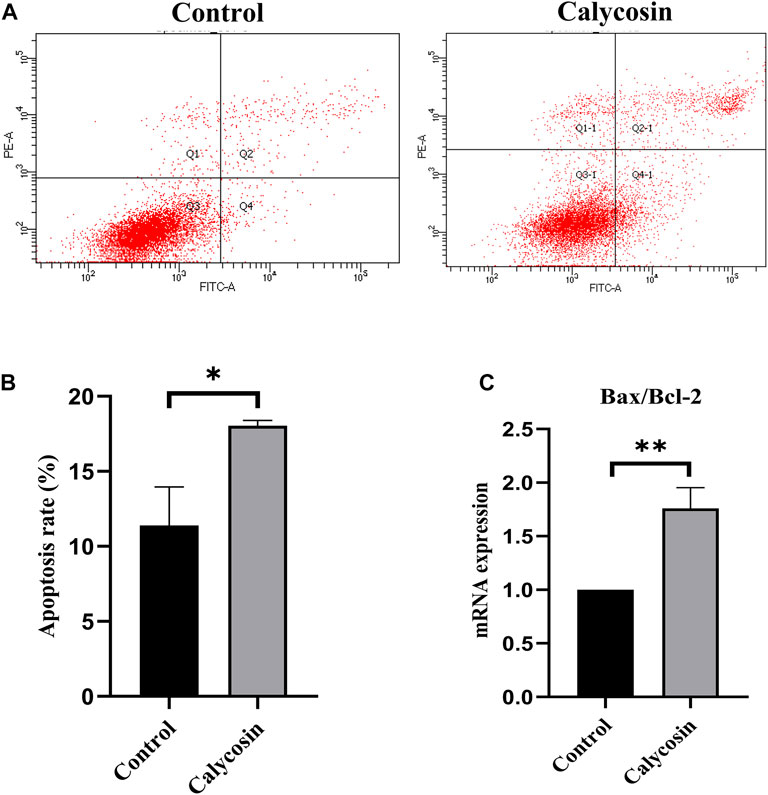
FIGURE 4. Calycosin promoted B-CPAP cell apoptosis. (A) B-CPAP cells were treated with calycosin (0.100 µM) for 24 h, and cell apoptosis was measured by flow cytometry. (B) The results were analyzed to obtain the apoptosis rate of each group. (C) Bax/Bcl-2 mRNA levels in B-CPAP cells. The experiment was repeated three times. *p < 0.05,**p < 0.01.
Calycosin induced autophagy in B-CPAP cells
The pathways enriched by the microarray sequencing results also showed that calycosin-treated B-APAP cells were associated with p70S6 Kinase (Figure 3D), a downstream target molecule of mTOR, which is a negative regulator of autophagy. To verify whether calycosin is related to autophagy, we observed cells by transmission electron microscopy and found an increase in autophagosomes in calycosin treatment group (Figure 5A). SESN2 is reported to activate autophagy via the AMPK/mTOR pathway in cancers. To investigate whether the antitumor effect of calycosin in PTC is also related to SESN2, we performed qRT-PCR and western blot experiments to verify the expression of SESN2 in cells and clinical sample tissue. We found that SESN2 in normal thyroid cells and tissues was higher than in B-CPAP cells (Figures 5B,C) and human PTC tissues (Figures 5D–F). The results indicated that calycosin promotes autophagy in B-CPAP cells and may be associated with SESN2 gene.
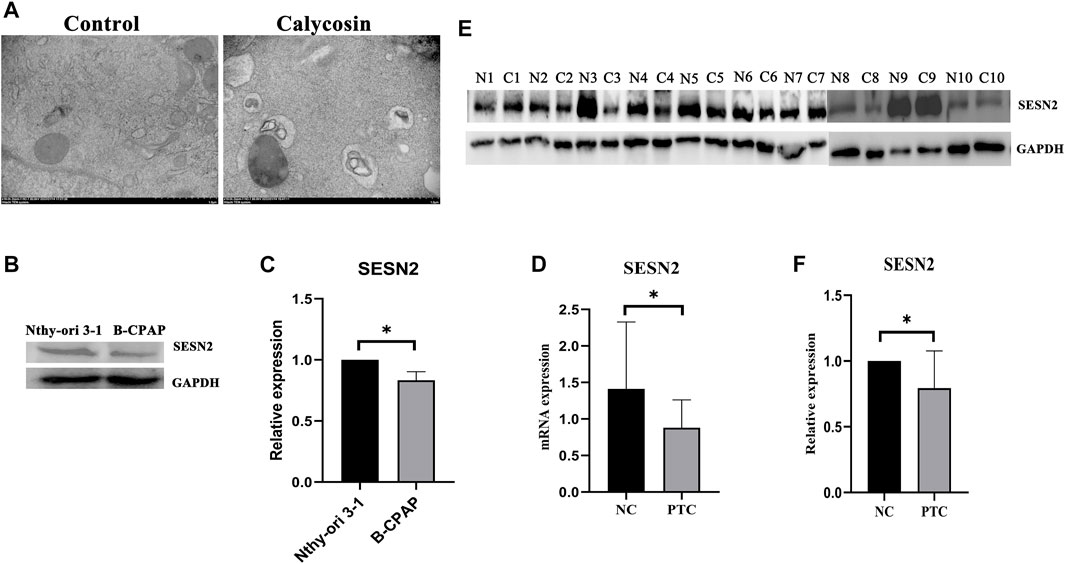
FIGURE 5. Calycosin induced B-CPAP cell autophagy (A) B-CPAP cells were treated with calycosin (100 µM) or control group for 24 h, washed with PBS, fixed with 2% glutaraldehyde, and imaged by transmission electron microscope to observe changes in cells. (B,C) SESN2 protein expression in Nthy-ori 3-1 and B-CPAP cells. (D) SESN2 mRNA expression in PTC tissue and human normal thyroid tissue. (E,F) SESN2 protein in human PTC tissue (C) and normal thyroid tissue (N). *p < 0.05.
Calycosin induced autophagy and apoptosis through the SESN2-AMPK-mTOR pathway
To further explore whether SESN2 was related to the protective effects of calycosin on autophagy and apoptosis, cells were transfected with siRNA targeting SESN2 (si-SESN2). We found that the protein expression of SESN2 was significantly increased after calycosin application (Figures 6A,B), which proved that the role of calycosin was indeed related to SESN2. The autophagic proteins (LC3II/I, Beclin1) and apoptosis markers (Bax/Bcl-2, Cleaved caspase-9) in the calycosin-treated cells did not increase after the SESN2-siRNA transfection (Figures 6C–G). These indicate that calycosin enhanced apoptosis and autophagy through SESN2 activation. Afterward, we continued to explore the SESN2-mediated downstream pathway. Calycosin significantly increased p-AMPK levels and inhibited p-mTOR and p-p70S6 kinase levels (Figure 7). These alterations were reversed when silencing SESN2, indicating that the antitumor effect of calycosin in B-CPAP cells was mediated by the SESN2/AMPK/mTOR pathway.
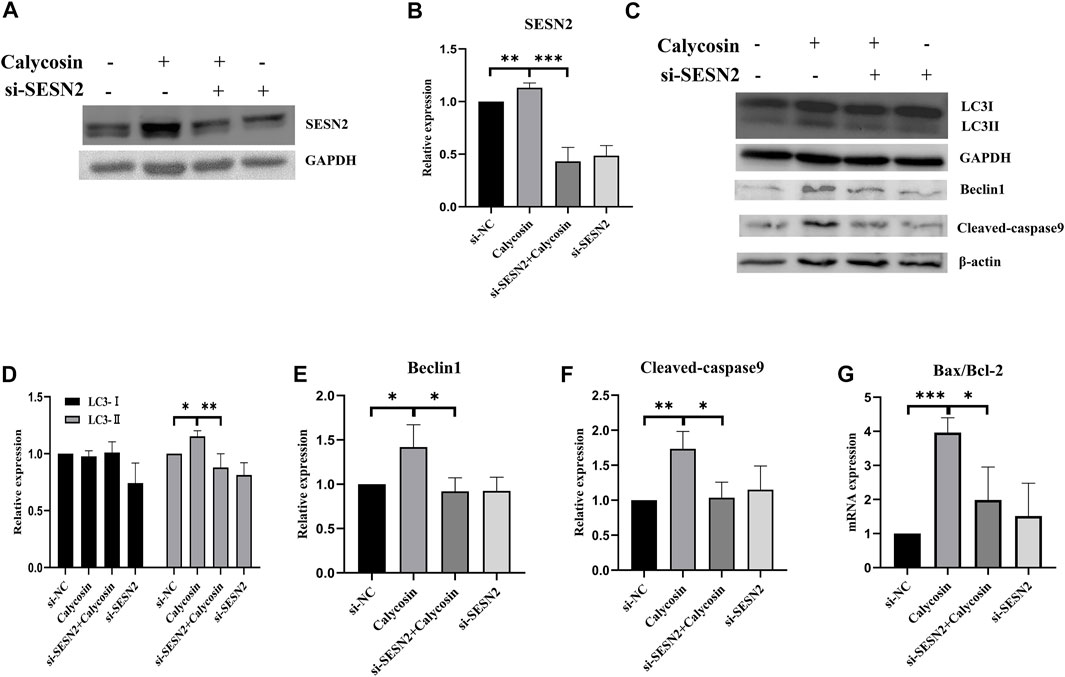
FIGURE 6. Calycosin induced apoptosis of B-CPAP cell and promoted autophagy related to SESN2. (A,B) The expression of SESN2 protein in B-CPAP cells treated with si-NC, si-SESN2 and/or calycosin (100 µM). (C–F) LC3Ⅰ/Ⅱ, Beclin1, Cleaved-caspase 9 proteins expression in B-CPAP cells treated with si-NC, si-SESN2 and/or calycosin (100 µM). (G) qRT-PCR analysis showing relative levels of Bax/Bcl-2 transcripts in si-NC, si-SESN2 and/or calycosin (100 µM)-treated B-CPAP cells. Results are representative of three independent experiments. *p < 0.05, **p < 0.01,***p < 0.001.
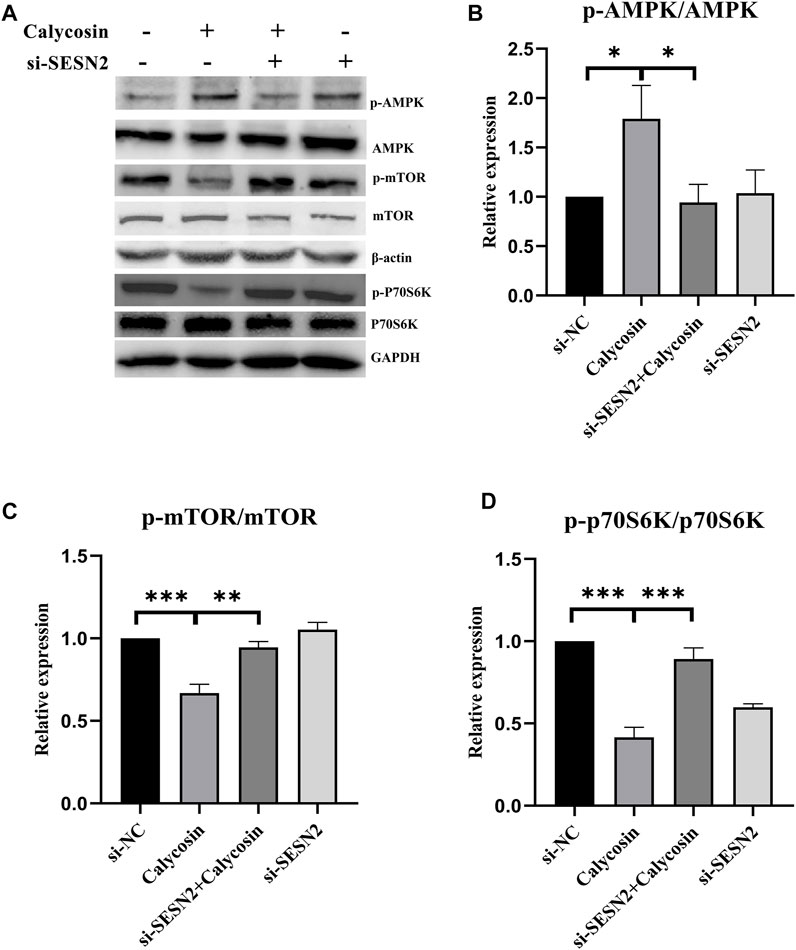
FIGURE 7. The pharmacological action of calycosin was through the SESN2-AMPK-mTOR pathway. The levels of p-AMPK/AMPK (A,B), p-mTOR/mTOR (A,C) and p-p70S6K/p70S6K (A,D) proteins in si-NC, si-SESN2/calycosin (100 μM)-treated B-CPAP cells. Results are representative of three independent experiments. *p < 0.05, **p < 0.01, ***p < 0.001.
Discussion
In this study, we found that calycosin reduced cell proliferation, inhibited migration and invasion, and promoted apoptosis of B-CPAP cells. This protective effect was associated with increased autophagy and activation of SESN2/AMPK/mTOR signaling pathway. Calycosin is a phytohormone with a molecular structure similar to mammalian estrogens and exerts biological activities, including anti-inflammatory activity, antioxidant activity, anti-osteoporosis activity, and anti-obese activity (Han et al., 2018; Deng et al., 2021). Several studies have demonstrated that calycosin has potential anti-metastatic effects on various tumors with low toxicity (Chen et al., 2011). However, until now, the role of calycosin in the PTC has never been studied. In this study, we examined the effect of calycosin on the growth of B-CPAP cells. Both the CCK8 assay and colony formation assay confirmed the inhibitory effect of calycosin on B-CPAP cells with increased concentration. Further transwell and wound healing experiments confirmed that calycosin could inhibit the proliferation, migration and invasion of B-CPAP cells. These findings proved that calycosin significantly repressed cell proliferation, induced apoptosis, and suppressed cell invasion in human PTC cells.
The regulation of autophagy is a complex process involving several mechanisms (Li et al., 2020). The role of autophagy in tumors is twofold, including inhibiting tumor initiation and supporting tumor progression (Levy et al., 2017). Previous studies confirmed that activation of autophagy is beneficial to the treatment of PTC. In this study, the autophagy-related proteins in the calycosin group were significantly increased. And autophagosomes in B-CPAP cells after calycosin application was more than that in untreated control cells by transmission electron microscopy. The results confirmed that calycosin indeed activated autophagy in B-CPAP cells.
Different from autophagy, apoptosis is an active and orderly cellular process. Malignant cells reduce apoptosis to achieve tumor growth in various ways; thus, drugs promoting apoptosis can inhibit tumor progression. Autophagy can directly activate apoptosis upstream or promote cell survival and antagonize apoptosis. Previously, calycosin was reported to activate autophagy in colorectal cancer cells HT29 and induce cell death (El-Kott et al., 2019). Thus, we analyzed the relationship between autophagy and apoptosis in calycosin-treated B-CPAP cells. The pathways enriched by the microarray sequencing showed that calycosin-treated B-APAP cells were associated with apoptosis. Bax/Bcl-2 ratio was increased in B-CPAP cells after calycosin application. Further studies showed that the cleaved caspase-9 in the calycosin-treated cells was elevated compared to control. Moreover, flow cytometry verified that the apoptosis rate of B-CPAP cells treated with calycosin was higher than control. These results support that calycosin can activate autophagy and apoptosis of B-CPAP cells.
The initiation of autophagy is due to the induction of autophagy-related gene complexes and inhibition of the activity of mTORC1 (Kim and Guan, 2015). AMPK can inhibit cell growth by inhibiting mTOR/P70S6K signaling, thereby exerting anti-tumor effects (Egan et al., 2011). However, AMPK activator/mTOR inhibitor can effectively inhibit various thyroid cancer cell lines growth (Plews et al., 2015). SESN2 could regulate autophagy and apoptosis mainly by activating AMPK and inhibiting the mTOR/P70S6K pathway. Pathways enriched by microarray sequencing results also showed that calycosin-treated B-APAP cells were associated with p70S6Kinase. To investigate whether the antitumor effect of calycosin in PTC is also related to SESN2, we performed qRT-PCR and western blot experiments to verify the expression of SESN2 in PTC. The results showed that SESN2 was higher in PTC and B-CPAP than in normal thyroid tissue/cells, and also higher in which calycosin (100 μM) was applied than in untreated B-CPAP. After silencing SESN2, we found that the Bax/Bcl-2 ratio did not increase, and the LC3, Beclin1, and Cleaved caspase-9 did not change significantly. These indicate that calycosin did not affect activating autophagy/promoting apoptosis in B-CPAP cells after silencing SESN2, indicating that SESN2 played a role in promoting autophagy or apoptosis. Thus, we confirmed the role of calycosin in autophagy and apoptosis in B-CPAP was consistent. We then studied whether the antitumor effects of calycosin were related to the SESN2/AMPK/mTOR signaling pathway. We found that calycosin increased AMPK phosphorylation while decreasing p-mTOR and p-p70S6K protein. After SESN2 silencing, these proteins were not significantly changed, indicating that the apoptosis and autophagy of calycosin in B-CPAP cells were regulated through the SESN2/AMPK/mTOR signaling pathway.
This study also has some limitations. This study mainly focuses on the research in B-CPAP cells and small clinical samples; it has not been validated in multiple tumor cell lines, nor has it been validated for other types of thyroid cancer and in vivo experiments. More importantly, there is no further drug application and research on animal and clinical samples. In the next step, we will further conduct related experiments to deeply study the antitumor mechanism of calycosin on PTC, which is brought novel insights into the pathogenesis of PTC and may lead to new avenues for treatment.
Conclusion
In conclusion, we found that calycosin can restrain B-CPAP cell proliferation, migration and invasion. Calycosin promoted apoptosis and autophagy via the SESN2/AMPK/mTOR pathway. Our study has revealed the anti-tumor pharmacological effects and the mechanism of calycosin on treatment of PTC. This supplies valuable rationale for clinical use of calycosin in treating PTC.
Data availability statement
The original contributions presented in the study are included in the article/Supplementary Material, further inquiries can be directed to the corresponding authors.
Ethics statement
The studies involving human participants were reviewed and approved by the Ethics Committee of Affiliated Hospital of Weifang Medical University. Written informed consent for participation was not required for this study in accordance with the national legislation and the institutional requirements.
Author contributions
NQ, JQ, and NH analyzed the data, performed the statistical analyses and drafted the manuscript. RP and XS conceived and designed the study and participated in the revision of the manuscript. The other authors participated in partial data collection, data curation and analysis.
Funding
This study was supported by grants from the National Natural Science Foundation of China (81870593 and 82170865), Natural Science Foundation of Shandong Province of China (ZR2019BH036, ZR2020MH106), Doctor Fund of Affiliated Hospital of Weifang Medical University (2019wyfybsjj01, NH), Medical Research and Cultivation Fund of Affiliated Hospital of Weifang Medical University (2021wyfyzzjj03), Special Funds for Taishan Scholars Project of Shandong Province, Yuandu scholars (2021) and Municipal Science and Technology Development Program of Weifang (2020YX088).
Conflict of interest
The authors declare that the research was conducted in the absence of any commercial or financial relationships that could be construed as a potential conflict of interest.
Publisher’s note
All claims expressed in this article are solely those of the authors and do not necessarily represent those of their affiliated organizations, or those of the publisher, the editors and the reviewers. Any product that may be evaluated in this article, or claim that may be made by its manufacturer, is not guaranteed or endorsed by the publisher.
References
Ambrosio, S., Saccà, C. D., Amente, S., Paladino, S., Lania, L., and Majello, B. (2017). Lysine-specific demethylase LSD1 regulates autophagy in neuroblastoma through SESN2-dependent pathway. Oncogene 36, 6701–6711. doi:10.1038/onc.2017.267
Ancker, O. V., Krüger, M., Wehland, M., Infanger, M., and Grimm, D. (2019). Multikinase inhibitor treatment in thyroid cancer. Int. J. Mol. Sci. 21, 10. doi:10.3390/ijms21010010
Beierwaltes, W. H., Nishiyama, R. H., Thompson, N. W., Copp, J. E., and Kubo, A. (1982). Survival time and "cure" in papillary and follicular thyroid carcinoma with distant metastases: Statistics following university of Michigan therapy. J. Nucl. Med. 23, 561–568.
Byun, J. K., Choi, Y. K., Kim, J. H., Jeong, J. Y., Jeon, H. J., Kim, M. K., et al. (2017). A positive feedback loop between Sestrin2 and mTORC2 is required for the survival of glutamine-depleted lung cancer cells. Cell Rep. 20, 586–599. doi:10.1016/j.celrep.2017.06.066
Chen, J., Xiong, W. B., Xiong, Y., Wu, Y. Y., Chen, X. J., Shao, Z. J., et al. (2011). Calycosin stimulates proliferation of estrogen receptor-positive human breast cancer cells through downregulation of Bax gene expression and upregulation of Bcl-2 gene expression at low concentrations. JPEN. J. Parenter. Enter. Nutr. 35, 763–769. doi:10.1177/0148607111413900
Deng, M., Chen, H., Long, J., Song, J., Xie, L., and Li, X. (2021). Calycosin: A review of its pharmacological effects and application prospects. Expert Rev. anti. Infect. Ther. 19, 911–925. doi:10.1080/14787210.2021.1863145
Dong, L., Yin, L., Chen, R., Zhang, Y., Hua, S., Quan, H., et al. (2018). Anti-inflammatory effect of Calycosin glycoside on lipopolysaccharide-induced inflammatory responses in RAW 264.7 cells. Gene 675, 94–101. doi:10.1016/j.gene.2018.06.057
Egan, D. F., Shackelford, D. B., Mihaylova, M. M., Gelino, S., Kohnz, R. A., Mair, W., et al. (2011). Phosphorylation of ULK1 (hATG1) by AMP-activated protein kinase connects energy sensing to mitophagy. Science 331, 456–461. doi:10.1126/science.1196371
El-Kott, A. F., Al-Kahtani, M. A., and Shati, A. A. (2019). Calycosin induces apoptosis in adenocarcinoma HT29 cells by inducing cytotoxic autophagy mediated by SIRT1/AMPK-induced inhibition of Akt/mTOR. Clin. Exp. Pharmacol. Physiol. 46, 944–954. doi:10.1111/1440-1681.13133
Filetti, S., Durante, C., Hartl, D., Leboulleux, S., Locati, L. D., Newbold, K., et al. (2019). Thyroid cancer: ESMO clinical practice guidelines for diagnosis, treatment and follow-up. Ann. Oncol. 30, 1856–1883. doi:10.1093/annonc/mdz400
Gong, A. G., Lau, K. M., Xu, M. L., Lin, H. Q., Dong, T. T., Zheng, K. Y., et al. (2016). The estrogenic properties of Danggui Buxue Tang, a Chinese herbal decoction, are triggered predominantly by calycosin in MCF-7 cells. J. Ethnopharmacol. 189, 81–89. doi:10.1016/j.jep.2016.05.035
Gudipaty, S. A., Conner, C. M., Rosenblatt, J., and Montell, D. J. (2018). Unconventional ways to live and die: Cell death and survival in development, homeostasis, and disease. Annu. Rev. Cell Dev. Biol. 34, 311–332. doi:10.1146/annurev-cellbio-100616-060748
Han, F., Li, K., Pan, R., Xu, W., Han, X., Hou, N., et al. (2018). Calycosin directly improves perivascular adipose tissue dysfunction by upregulating the adiponectin/AMPK/eNOS pathway in obese mice. Food Funct. 9, 2409–2415. doi:10.1039/c8fo00328a
JiaZhang, M. X., and Wang, P. (2019). Calycosin attenuates MPTP-induced Parkinson's disease by suppressing the activation of TLR/NF-κB and MAPK pathways. Phytother. Res. 33, 309–318. doi:10.1002/ptr.6221
Kim, Y. C., and Guan, K. L. (2015). mTOR: a pharmacologic target for autophagy regulation. J. Clin. Invest. 125, 25–32. doi:10.1172/JCI73939
Lee, J. H., Budanov, A. V., and Karin, M. (2013). Sestrins orchestrate cellular metabolism to attenuate aging. Cell Metab. 18, 792–801. doi:10.1016/j.cmet.2013.08.018
Levy, J., Towers, C. G., and Thorburn, A. (2017). Targeting autophagy in cancer. Nat. Rev. Cancer 17, 528–542. doi:10.1038/nrc.2017.53
Li, W., He, P., Huang, Y., Li, Y. F., Lu, J., Li, M., et al. (2021). Selective autophagy of intracellular organelles: Recent research advances. Theranostics 11, 222–256. doi:10.7150/thno.49860
Li, X., He, S., and Ma, B. (2020). Autophagy and autophagy-related proteins in cancer. Mol. Cancer 19, 12. doi:10.1186/s12943-020-1138-4
Liang, Y., Zhu, J., Huang, H., Xiang, D., Li, Y., Zhang, D., et al. (2016). SESN2/sestrin 2 induction-mediated autophagy and inhibitory effect of isorhapontigenin (ISO) on human bladder cancers. Autophagy 12, 1229–1239. doi:10.1080/15548627.2016.1179403
Lin, C. I., Whang, E. E., Donner, D. B., Du, J., Lorch, J., He, F., et al. (2010). Autophagy induction with RAD001 enhances chemosensitivity and radiosensitivity through Met inhibition in papillary thyroid cancer. Mol. Cancer Res. 8, 1217–1226. doi:10.1158/1541-7786.MCR-10-0162
Maiuri, M. C., Malik, S. A., Morselli, E., Kepp, O., Criollo, A., Mouchel, P. L., et al. (2009). Stimulation of autophagy by the p53 target gene Sestrin2. Cell Cycle 8, 1571–1576. doi:10.4161/cc.8.10.8498
Peng, M., Yin, N., and Li, M. O. (2014). Sestrins function as guanine nucleotide dissociation inhibitors for Rag GTPases to control mTORC1 signaling. Cell 159, 122–133. doi:10.1016/j.cell.2014.08.038
Plews, R. L., Mohd Yusof, A., Wang, C., Saji, M., Zhang, X., Chen, C. S., et al. (2015). A novel dual AMPK activator/mTOR inhibitor inhibits thyroid cancer cell growth. J. Clin. Endocrinol. Metab. 100, E748–E756. doi:10.1210/jc.2014-1777
Qu, J., Luo, M., Zhang, J., Han, F., Hou, N., Pan, R., et al. (2021). A paradoxical role for sestrin 2 protein in tumor suppression and tumorigenesis. Cancer Cell Int. 21, 606. doi:10.1186/s12935-021-02317-9
Schlumberger, M. J. (1998). Papillary and follicular thyroid carcinoma. N. Engl. J. Med. 338, 297–306. doi:10.1056/NEJM199801293380506
Shin, J., Bae, J., Park, S., Kang, H. G., Shin, S. M., Won, G., et al. (2020). mTOR-Dependent role of Sestrin2 in regulating tumor progression of human endometrial cancer. Cancers (Basel) 12, 2515. doi:10.3390/cancers12092515
Siegel, R. L., Miller, K. D., Fuchs, H. E., and Jemal, A. (2021). Cancer statistics, 2021. Ca. Cancer J. Clin. 71, 7–33. doi:10.3322/caac.21654
Sun, H., Yin, M., Qian, W., and Yin, H. (2018). Calycosin, a phytoestrogen isoflavone, induces apoptosis of estrogen receptor-positive MG-63 osteosarcoma cells via the phosphatidylinositol 3-kinase (PI3K)/AKT/mammalian target of rapamycin (mTOR) pathway. Med. Sci. Monit. 24, 6178–6186. doi:10.12659/MSM.910201
Sun, X., Han, F., Lu, Q., Li, X., Ren, D., Zhang, J., et al. (2020). Empagliflozin ameliorates obesity-related cardiac dysfunction by regulating sestrin2-mediated AMPK-mTOR signaling and redox homeostasis in high-fat diet-induced obese mice. Diabetes 69, 1292–1305. doi:10.2337/db19-0991
Tang, Z., Wei, X., Li, T., Wang, W., Wu, H., Dong, H., et al. (2021). Sestrin2-Mediated autophagy contributes to drug resistance via endoplasmic reticulum stress in human osteosarcoma. Front. Cell Dev. Biol. 9, 722960. doi:10.3389/fcell.2021.722960
Tian, J., Wang, Y., Zhang, X., Ren, Q., Li, R., Huang, Y., et al. (2017). Calycosin inhibits the in vitro and in vivo growth of breast cancer cells through WDR7-7-GPR30 Signaling. J. Exp. Clin. Cancer Res. 36, 153. doi:10.1186/s13046-017-0625-y
Wang, Q., Lu, W., Yin, T., and Lu, L. (2019). Calycosin suppresses TGF-β-induced epithelial-to-mesenchymal transition and migration by upregulating BATF2 to target PAI-1 via the Wnt and PI3K/Akt signaling pathways in colorectal cancer cells. J. Exp. Clin. Cancer Res. 38, 240. doi:10.1186/s13046-019-1243-7
Wei, J. L., Fu, Z. X., Fang, M., Guo, J. B., Zhao, Q. N., Lu, W. D., et al. (2015). Decreased expression of sestrin 2 predicts unfavorable outcome in colorectal cancer. Oncol. Rep. 33, 1349–1357. doi:10.3892/or.2014.3701
Wells, S. A. (2016). Progress in endocrine neoplasia. Clin. Cancer Res. 22, 4981–4988. doi:10.1158/1078-0432.CCR-16-0384
Wolfson, R. L., Chantranupong, L., Saxton, R. A., Shen, K., Scaria, S. M., Cantor, J. R., et al. (2016). Sestrin2 is a leucine sensor for the mTORC1 pathway. Science 351, 43–48. doi:10.1126/science.aab2674
Zhai, J., Tao, L., Zhang, S., Gao, H., Zhang, Y., Sun, J., et al. (2020). Calycosin ameliorates doxorubicin-induced cardiotoxicity by suppressing oxidative stress and inflammation via the sirtuin 1-NOD-like receptor protein 3 pathway. Phytother. Res. 34, 649–659. doi:10.1002/ptr.6557
Zhang, L., Cheng, X., Gao, Y., Zheng, J., Xu, Q., Sun, Y., et al. (2015). Apigenin induces autophagic cell death in human papillary thyroid carcinoma BCPAP cells. Food Funct. 6, 3464–3472. doi:10.1039/c5fo00671f
Keywords: calycosin, thyroid cancer, small molecules, therapy, autophagy, Sestrin2
Citation: Qu N, Qu J, Huang N, Zhang K, Ye T, Shi J, Chen B, Kan C, Zhang J, Han F, Hou N, Sun X and Pan R (2022) Calycosin induces autophagy and apoptosis via Sestrin2/AMPK/mTOR in human papillary thyroid cancer cells. Front. Pharmacol. 13:1056687. doi: 10.3389/fphar.2022.1056687
Received: 29 September 2022; Accepted: 06 December 2022;
Published: 16 December 2022.
Edited by:
Chao Guo, Guigang City People’s Hospital, ChinaReviewed by:
Zhiyu Wang, Guangzhou University of Chinese Medicine, ChinaXiao-yuan Li, The Affiliated Hospital of Qingdao University, China
Copyright © 2022 Qu, Qu, Huang, Zhang, Ye, Shi, Chen, Kan, Zhang, Han, Hou, Sun and Pan. This is an open-access article distributed under the terms of the Creative Commons Attribution License (CC BY). The use, distribution or reproduction in other forums is permitted, provided the original author(s) and the copyright owner(s) are credited and that the original publication in this journal is cited, in accordance with accepted academic practice. No use, distribution or reproduction is permitted which does not comply with these terms.
*Correspondence: Ruiyan Pan, panry@wfmc.edu.cn; Xiaodong Sun, xiaodong.sun@wfmc.edu.cn
†These authors have contributed equally to this work