- 1Division of Pharmacology, Department of Pharmaceutical Sciences, Faculty of Science, Utrecht University, Utrecht, Netherlands
- 2Center for Translational Immunology, University Medical Center Utrecht, Utrecht University, Utrecht, Netherlands
- 3Department of Dermatology/Allergology, University Medical Center Utrecht, Utrecht University, Utrecht, Netherlands
- 4Global Centre of Excellence Immunology, Danone Nutricia Research B.V., Utrecht, Netherlands
The Mediterranean diet, containing valuable nutrients such as n-3 long chain poly-unsaturated fatty acids (LCPUFAs) and other fat-soluble micronutrients, is known for its health promoting and anti-inflammatory effects. Its valuable elements might help in the battle against the rising prevalence of non-communicable diseases (NCD), including the development of allergic diseases and other (chronic) inflammatory diseases. The fat fraction of the Mediterranean diet contains bioactive fatty acids but can also serve as a matrix to dissolve and increase the uptake of fat-soluble vitamins and phytochemicals, such as luteolin, quercetin, resveratrol and lycopene with known immunomodulatory and anti-inflammatory capacities. Especially n-3 LCPUFAs such as eicosapentaenoic acid (EPA) and docosahexaenoic acid (DHA) derived from marine oils can target specific receptors or signaling cascades, act as eicosanoid precursors and/or alter membrane fluidity and lipid raft formation, hereby exhibiting anti-inflammatory properties. Beyond n-3 LCPUFAs, fat-soluble vitamins A, D, E, and K1/2 have the potential to affect pro-inflammatory signaling cascades by interacting with receptors or activating/inhibiting signaling proteins or phosphorylation in immune cells (DCs, T-cells, mast cells) involved in allergic sensitization or the elicitation/effector phase of allergic reactions. Moreover, fat-soluble plant-derived phytochemicals can manipulate signaling cascades, mostly by interacting with other receptors or signaling proteins compared to those modified by fat-soluble vitamins, suggesting potential additive or synergistic actions by applying a combination of these nutrients which are all part of the regular Mediterranean diet. Research concerning the effects of phytochemicals such as polyphenols has been hampered due to their poor bio-availability. However, their solubility and uptake are improved by applying them within the dietary fat matrix. Alternatively, they can be prepared for targeted delivery by means of pharmaceutical approaches such as encapsulation within liposomes or even unique nanoparticles. This review illuminates the molecular mechanisms of action and possible immunomodulatory effects of n-3 LCPUFAs and fat-soluble micronutrients from the Mediterranean diet in allergic disease development and allergic inflammation. This will enable us to further appreciate how to make use of the beneficial effects of n-3 LCPUFAs, fat-soluble vitamins and a selection of phytochemicals as active biological components in allergy prevention and/or symptom reduction.
Fatty Acids and Fat-Soluble Components of the Mediterranean Diet and Allergy Development
Metabolic and immunological disturbances underlie the recent rise in non-communicable diseases (NCD). Beyond auto-immune diseases, the risk of allergic diseases and asthma has been steadily rising over the last decades, reaching the alarming prevalence of 5%–40% in Western populations (Agache et al., 2019). The Mediterranean diet is known for its health promoting and anti-inflammatory effects (Barbaresko et al., 2013; Sánchez-Sánchez et al., 2020). The fat component of the Mediterranean diet typically consists of olive and marine oils. Notably, particularly marine oil derived n-3 long chain poly-unsaturated fatty acids (LCPUFAs) such as eicosapentaenoic acid (EPA) and docosahexaenoic acid (DHA) may have immunomodulatory properties to mitigate unwanted inflammation and reduce the risk of allergy development (Venter et al., 2019). Additionally, the used oils may facilitate bioavailability of fat-soluble micronutrients such as vitamin A, D, K, E and phytochemicals such as polyphenols and carotenoids (Figure 1) (White et al., 2017). In contrast, n-9 mono-unsaturated oleic acid is olive oil’s main fatty acid, but it is not known for its anti-allergic properties. However, olive oils are also rich in anti-oxidants (e.g. tocopherols, carotenes), phenols, secoiridoids, lignans, and flavones (luteolin, apigenin) all known for their anti-inflammatory effects (Aludatt et al., 2017). Moreover, fruits and vegetables, accounting for a significant component of the Mediterranean diet, are rich sources of fat-soluble phytochemicals such as quercetin (flavonoid) in onions and apples, luteolin (flavonoid) in parsley, resveratrol (stilbene) in wine and berries and carotenoid lycopene in tomatoes (Figure 1 and Table 1) (Mazzocchi et al., 2019). The uptake and bioavailability of these fat-soluble micronutrients may be enhanced in the presence of olive oil. They can be absorbed via inclusion in micelles required for fatty acid uptake by the intestinal epithelium and released basolaterally in chylomicrons which traffic via the lymphatics into the bloodstream (Boileau et al., 1999; Arranz et al., 2015; Mashurabad et al., 2017; White et al., 2017; Rinaldi de Alvarenga et al., 2019). Enhanced bioavailability of fat-soluble bioactive components may enhance health benefits, including protection against allergic inflammation. Indeed, allergy protective effects of the Mediterranean diet have been suggested in several observational studies, but thus far data have been inconclusive (Biagi et al., 2019). In early life, one of the first outcomes of allergic disease is atopic dermatitis and/or food allergy while later in childhood and during adolescence allergic rhinitis and asthma are more prevalent (Figure 2).
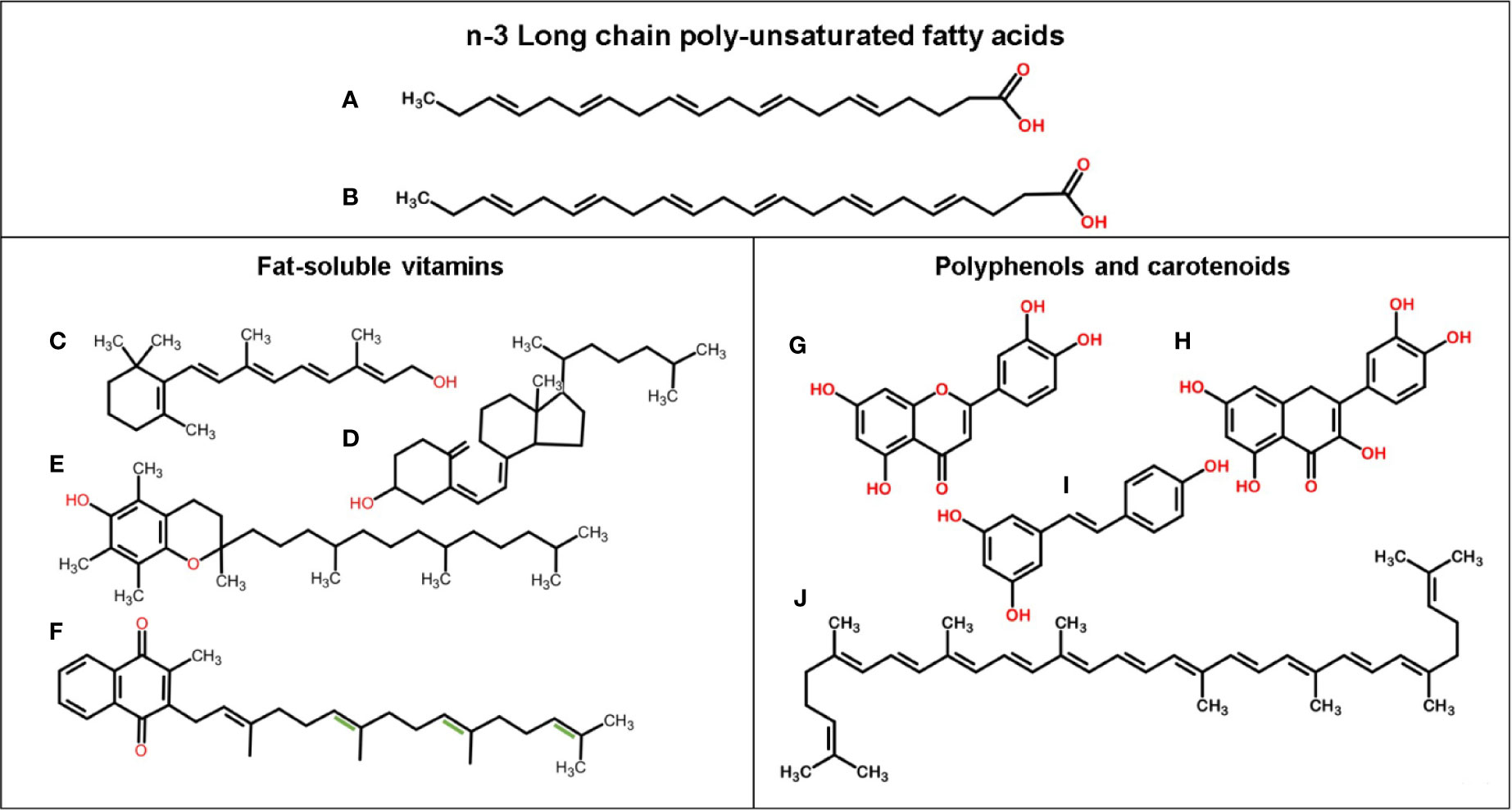
Figure 1 Chemical structure of n-3 LCPUFAs and fat-soluble bioactive components. (A) EPA, (B) DHA, (C) Vitamin A (retinol), (D) Vitamin D3 (cholecalciferol), (E) Vitamin E (alpha-tocopherol), (F) Vitamin K1 (phylloquinone), and with extra double bonds (in green) Vitamin K2 (menaquinone-4), (G) Luteolin, (H) Quercetin, (I) Resveratrol, and (J) Lycopene.
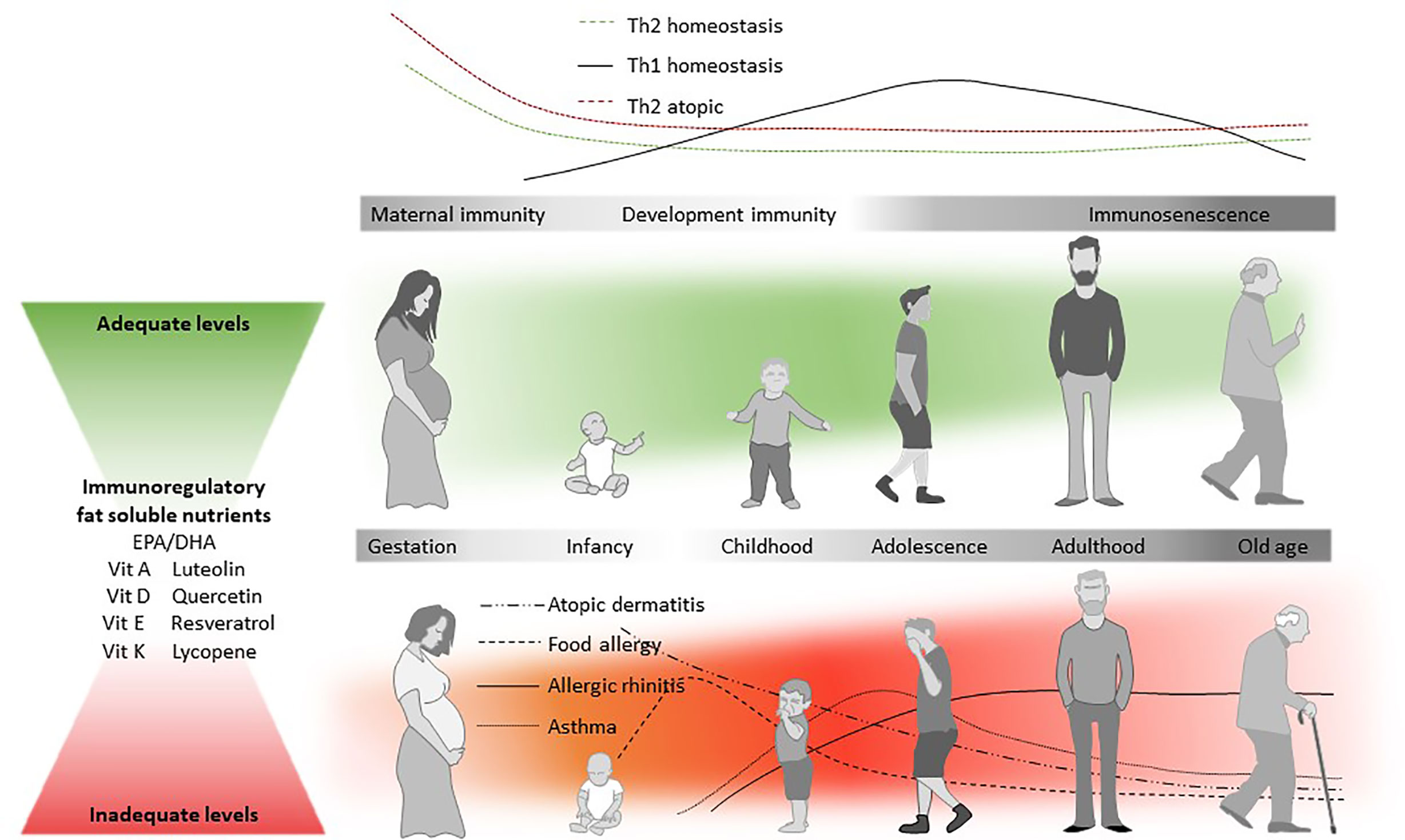
Figure 2 Diet and the atopic march. Immune responses are typically Th2-dominated in the early stages of life (top panel), but Th1-responses start developing after birth. In atopic individuals, Th2 responses tend to be higher already from an early age. The likelihood that an individual will develop allergic disease is partly determined by genetic predisposition, but genetic drift cannot explain the rise in prevalence (Agache et al., 2019). In the so-called Atopic March, atopic dermatitis (AD) is often the first clinical manifestation of allergic disease, which is typically followed by food allergy, rhinitis, and asthma (Spergel, 2010), all being characterized by a Th2 immune response. AD is followed by the development of allergen specific IgE (develop) and/or food allergy. In contrast to IgE-mediated food allergies, inhalant allergies and asthma are developed later in childhood. The increased susceptibility to atopic disease (red panel) is thought to be mediated at least in part by the nutritional environment during early development. Beyond other known immunoregulatory dietary components, n-3 LCPUFA and fat soluble nutrients may also help to reduce the allergy risk, and it is hypothesized that adequate levels (in green) of EPA/DHA, vitamin A, D, E, and K1/2, luteolin, quercetin, resveratrol, and lycopene could play an important role in maintaining immune-homeostasis throughout life.
Allergy evolves due to hampered immunological tolerance induction at mucosal sites such as the intestinal and pulmonary mucosa or the skin. Upon crossing this physical barrier, allergens are taken up by antigen-presenting cells such as dendritic cells (DCs) which subsequently present processed peptides originating from the allergens in a major histocompatibility complex class II (MHC-II) molecule to naïve T helper (Th) cells (Roche and Furuta, 2015). In a Th2 driving environment, characterized by increased interleukin (IL) 4 and IL-13 expression and/or release of epithelial factors such as IL-33, IL-25, and thymic stromal lymphopoietin (TSLP), these DCs differentiate into DC2s that instruct naïve Th-cells to differentiate into Th2-cells (Van Dyken et al., 2016). Interaction of these Th2-cells with naïve B-cells recognizing the allergen via the B-cell receptor and CD40-CD40 ligand co-stimulatory interaction supports the class-switch of naïve IgM+ B-cells to IgE+ B cells. Upon activation, these B-cells differentiate into IgE-secreting plasma cells (Iciek et al., 1997). These IgE-antibodies can be bound by the high-affinity FcϵRI receptor located on the surface of mast cells and basophils (effector cells) (Figure 3). Upon re-exposure, the allergen is recognized by IgE antibodies and cross-linking of at least two different FcϵRI receptors triggers the release of pre-formed (e.g. histamines) and de-novo synthesized mediators (e.g. lipid mediators like prostaglandins) and cytokines/chemokines driving allergic symptoms (Kambayashi and Koretzky, 2007).
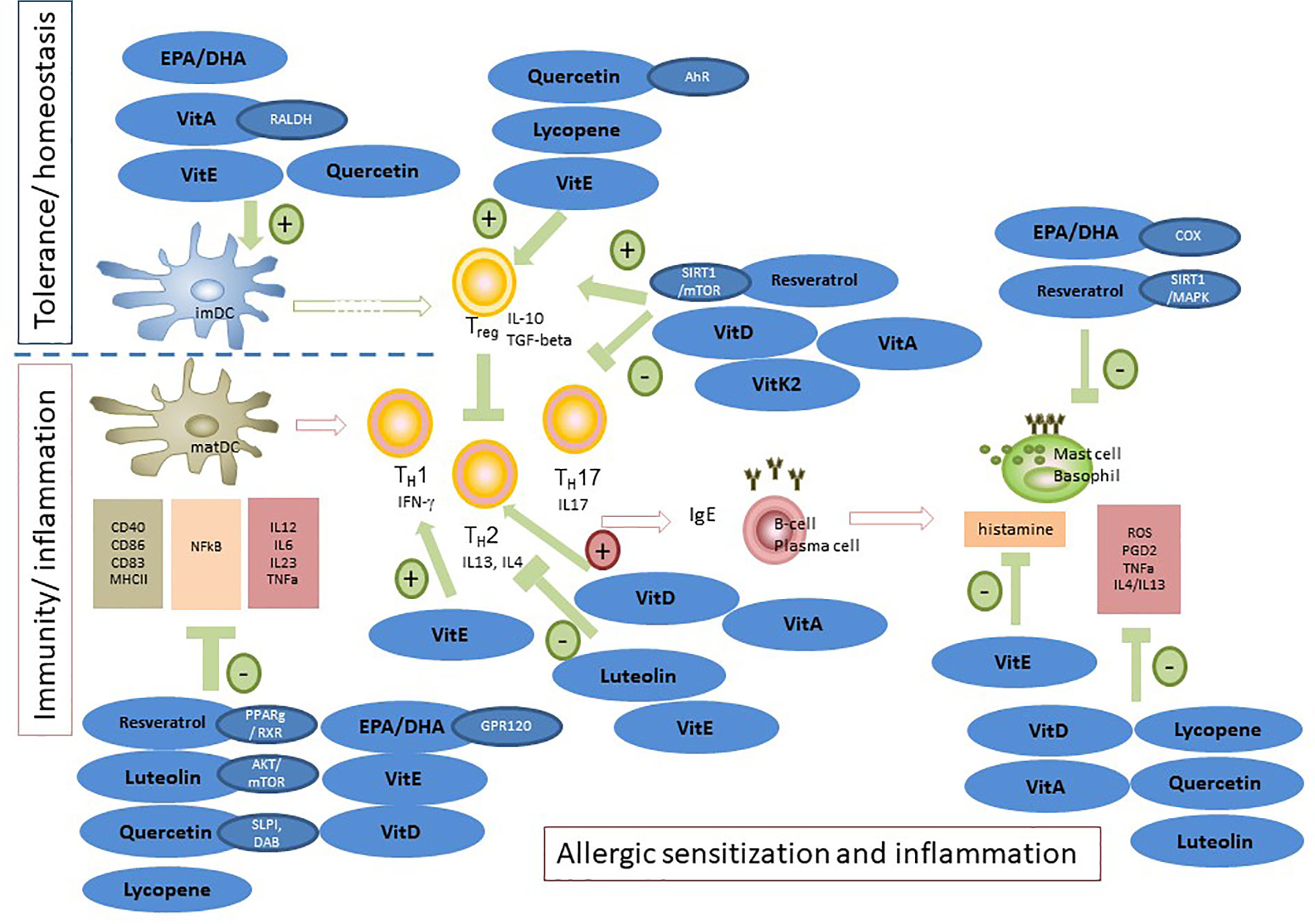
Figure 3 Modulation of allergic sensitization and effector phase by n-3 LCPUFAs and fat-soluble vitamins, polyphenols and carotenoids. In in vitro and pre-clinical studies, the potency of n-3 LCPUFAs and several fat-soluble micronutrients to instruct DC silencing was indicated, rendering DCs that support Treg development. In addition, LPS or inflammatory induced maturation of DCs can be suppressed by multiple of these nutrients, resulting in reduced proliferation and activation of consequent effector T-cells responses, hence attenuating pro-inflammatory responses. Also, Th2 driven allergy development can be mitigated by these micronutrients, either by directly suppressing Th2 development or via enhancing Treg or Th1 responsiveness, known to down regulate Th2 activation. In addition, mast cell or basophil activation is modified or suppressed in various ways by n-3 LCPUFA and the selected fat-soluble micronutrients. Some micronutrients play an ambivalent role since they can lower pro-inflammatory responses via enhancing not only Treg but also Th2 function (VitD and VitA). This may be a point of concern in case of allergic predisposition. Of note is that the beneficial immunomodulatory effects of vitamin E are mainly linked to the alpha-tocopherol form and even though not much is known about immune effects of VitK, the main immunomodulatory effects appear to relate to the VitK2 isoform. These micronutrients can act via several receptors or signaling transduction cascades and are frequently tested as single component for their immunomodulatory capacities. However, since they target similar cells involved in the allergic sensitization and effector cascade their effects may by additive or synergistic when combined aiming to prevent allergy development or reduce severity of symptoms.
Modulation of Allergic Inflammation by N-3 LCPUFAs
Fatty fish are rich in n-3 LCPUFAs such as EPA (C20:5) and DHA (C22:6). EPA and DHA are essential components of cell lipid bilayers and play an important role in visual and neurodevelopment and cardioprotection (Miles and Calder, 2012; van den Elsen et al., 2012). In humans, these n-3 LCPUFAs can be synthesized from the essential fatty acid alpha-linolenic acid (ALA; C18:3) (present in vegetables, oils such as linseed oil and nuts such as walnuts) by fatty acid desaturase encoded by the FADS genes and elongase activity (Figure 4). However, the conversion rate of ALA into n-3 LCPUFA is only 10% or lower, depending on gene expression and activity of the rate limiting enzymes Δ5 or Δ6 desaturase and elongase (Tanaka et al., 2009). Additionally, the ratio of n-3 LCPUFAs to n-6 LCPUFA linoleic acid (LA, C18:2) and n-6 LCPUFA arachidonic acid (AA, C20:4) is unfavorably low (1:10–20) in the Western diet, due to its high content of n-6 PUFAs from vegetable oils such as sunflower and corn oil, eggs and meat [reviewed by (Miles and Calder, 2012; van den Elsen et al., 2012)]. A more preferable n-3:n-6 ratio would be 1:4 since n-3 ALA and n-6 LA compete for conversion by desaturases and elongase and also for inter-exchange in cell membranes phosphobilayers (Sprecher et al., 1995). Thus, a disbalance in favor of n-6 PUFAs may result in enhanced inflammatory responses since the n-6 LCPUFA AA will be converted by cyclo-oxygenase (COX) and lipoxygenase (LOX) enzymes into 2 and 4 series of prostaglandins (PGE2, PGD2) and leukotriens (LTB4), respectively (Barden et al., 2016). By contrast, n-3 LCPUFA EPA conversion by the same enzymes results in less inflammatory 3 and 5 series eicosanoids. Additionally, EPA and DHA are precursors for resolvins and maresins that restrict and silence inflammation, hence contributing to homeostasis [reviewed by (van den Elsen et al., 2012)]. LCPUFA can also be converted by cytochrome P450 epoxygenases, resulting in anti-inflammatory and cardioprotective epoxins such as epoxyeicosatrienoic acid (EET) derived from n-6 AA, and epoxyeicosatetraenoic acid (EpETE) and epoxydocosapentaenoic acid (EpDPE) from n-3 EPA or DHA respectively (Spector and Kim, 2016; Ostermann et al., 2019). In contrast, AA can also be converted in several pro-inflammatory hydroxyeicosatetraenoic acids (HETE) (reviewed by Calder, 2015). In addition, the epoxins may be further metabolized in PUFA-diols by soluble epoxidehydrolase which may cause undesirable side effects, related to—among others—increased oxidative stress responses (Fleming, 2019; dos Santos and Fleming, 2020). This underpins the importance of adequate levels of anti-oxidant intake when consuming high doses of LCPUFAs.
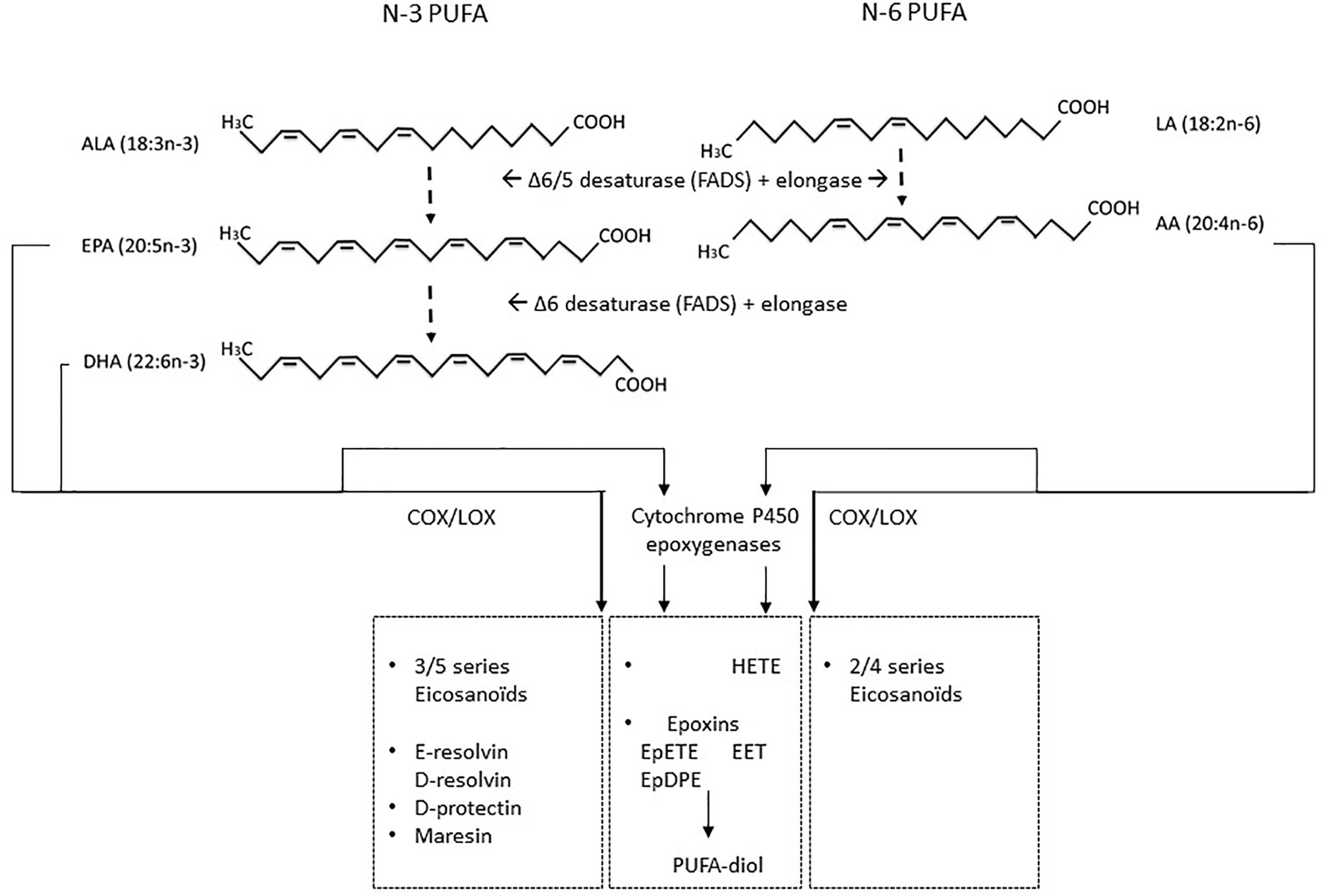
Figure 4 LCPUFAs and their metabolites N-3 ALA and n-6 LA are essential PUFAs that can be formed into either n-3 series of long chain PUFA EPA and DHA or n-6 LCPUFAs AA via fatty acid elongases and desaturases enzymes. These LCPUFAs are substrates for COX and LOX which can convert EPA into 3–5 series of eicosanoids or AA into 2–4 series of eicosanoids. In addition, EPA and DHA can be converted into anti-inflammatory protectins, resolvins, or maresins. Beyond COX and LOX, cytochrome P450 epoxygenases can convert n-6 AA or n-3 LA, EPA, and DHA in anti-inflammatory and cardiovascular protective epoxins such as epoxyeicosatrienoic acid (EET) derived from AA, and EPA or DHA derived epoxyeicosatetraenoic acid (EpETE) and epoxydocosapentaenoic acid (EpDPE). However, these may be further converted into PUFA-diols by soluble epoxidehydrolase, resulting in loss of their protective function and induction of possible harmful effects. In addition, cytochrome P450 epoxygenase conversion of AA can contribute to several classes of hydroxyeicosatetraenoic acid (HETE) which can promote inflammation.
N-3 LCPUFAs EPA and DHA can also directly enhance anti-inflammatory signaling cascades [reviewed by (van den Elsen et al., 2012)]. DHA is a well-known ligand for the transmembrane G-protein coupled receptor 120 (GPR120) and intracellular receptor PPARγ. By binding to these receptors, DHA can generate its anti-inflammatory effect by lowering NFkB (nuclear factor kappa-light-chain-enhancer of activated B-cells) activation (Hara et al., 2011; Scirpo et al., 2015). Furthermore, n-3 LCPUFAs enhance membrane fluidity due to their high number of unsaturated bonds, thus impacting cellular function by altering lipid raft formation and cellular signaling (van den Elsen et al., 2012). However, the high amount of unsaturated bonds leads to the risk of oxidation and formation of reactive oxygen species (ROS). In this regard, fat-soluble vitamin E (tocopherol) has an important function, since it is present in the cell membrane and can scavenge formed radicals and prevent cell damage due to lipid peroxidation.
N-3 LCPUFA and DCs and T-Cells
DCs are key players in host defense and the maintenance of tolerance (Steinman and Banchereau, 2007; Coquerelle and Moser, 2010). Several papers reported effects of EPA and DHA on DC functioning. In human monocyte-derived DCs (moDCs), DHA and EPA decreased expression of lipid presenting protein CD1, IL-6 secretion, and expression of GPR120 (Oh et al., 2010; Rajnavolgyi et al., 2014). Moreover, differentiation of human moDCs in the presence of EPA diminished lipopolysaccharide (LPS)-induced maturation and cytokine release (Zeyda et al., 2005). Correspondingly, moDCs treated with EPA have a decreased potency to stimulate allogeneic T-cells (Zeyda et al., 2005). These inhibitory effects were associated with a dose-dependent suppression of LPS-induced p38 mitogen-activated protein kinase (MAPK) phosphorylation by EPA and DHA (Wang et al., 2007). Furthermore, PPARγ target genes in human moDCs are induced by DHA-exposure, suggesting DHA to be involved in activation of the heterodimer of PPARγ and retinoid X receptor (RXR). PPARs are highly expressed in DCs, and DHA derivatives act as potent PPAR agonists (Yamamoto et al., 2005), resulting in lower IL-12 expression in murine bone marrow derived DCs (BMDCs). However, DHA-induced inhibition of NFκB p65 nuclear translocation appeared to be PPARγ-independent (Kong et al., 2010; Draper et al., 2011). Overall, treatment with DHA led to an immature DC phenotype (Kong et al., 2010). Interestingly, T-cells co-cultured with DHA-treated murine BMDCs expressed higher levels of transforming growth factor (TGF) β and forkhead box P3 (Foxp3), but a functional regulatory T-cell (Treg) phenotype was not found (Kong et al., 2010). In contrast, DHA treatment of murine DCs has been observed to increase expression levels of co-stimulatory molecules (Carlsson et al., 2015). However, T-cell stimulation was similarly inhibited, accompanied by an increased proportion of Treg in co-cultures with DHA-primed DCs (Carlsson et al., 2015). Hence, EPA and DHA have the capacity to affect the phenotype and function of DCs, which in turn can alter immune outcomes by lowering T-cell activation and inducing regulatory responses (Figure 3).
N-3 LCPUFA and B-Cells
In the presence of DHA, ex-vivo B-cell IgE class-switching (induced by anti-CD40 and IL-4) is reduced in peripheral blood mononuclear cells (PBMCs) from AD patients (Koch et al., 2008). Potentially, IgE class-switching ability is reduced by direct interference with CD40 and IL-4 signaling pathways, subsequently specifically blocking transcription of the epsilon germline transcript (ϵGLT) in DHA-treated PBMCs from non-atopic patients (Weise et al., 2011). Moreover, ex-vivo treatment of PBMCs from non-atopic donors with DHA metabolites reduced transcription of ϵGLT by stabilizing B-cell lymphoma 6 protein (Bcl-6), acting as a suppressor of the ϵGLT transcription factor STAT6. However, due to low numbers of IgE-producing B-cells, significant suppression of class-switching was not confirmed (Kim et al., 2015).
N-3 LCPUFA and Mast Cells
Cross-linking of high affinity FcϵRI receptors on effector cell membrane leads to the release of (lipid) mediators. Pre-incubation with n-3 LCPUFAs EPA and/or DHA dose-dependently reduced PGD2 production by human cord blood derived mast cells (CBMCs) and HMC-1 cells compared to AA pre-incubation by competing for conversion via COX (Obata et al., 1999; van den Elsen et al., 2013a). This reducing effect was also observed in NC/Nga mice (PGE2 and LTB4) (Suzuki et al., 2002; Yoshida et al., 2016). Moreover, n-3 LCPUFAs reduce production of pro-inflammatory cytokines such as IL-4 and IL-13, probably by decreasing ROS levels and further downstream inhibition of MAPK phosphorylation without affecting NFκB (van den Elsen et al., 2013a). In contrast, ROS production was increased in a canine mastocytoma cell line (C2) after pre-administration of AA and EPA. Adding antioxidants like vitamin E (VitE) diminished this activating effect of EPA (Schmutzler et al., 2010). Administration of n-3 LCPUFAs may also decrease Th2 cytokine expression by inhibiting transcription factor GATA-1 phosphorylation in murine mast cells (Park et al., 2013). However, in-vivo experiments on n-3 LCPUFAs supplementation in allergy management are controversial which might be explained by experiments performed by Shimanaka et al. (Matsuda et al., 1997; Sierra et al., 2008; Jang et al., 2018; Lee et al., 2019). Phospholipase PAF-AH2 knock-out mice (C57BL and BALB/c) with an impaired conversion of n-3 LCPUFAs into n-3 epoxins showed attenuated allergic symptoms compared to wild-type mice upon n-3 LCPUFAs supplementation and antigen-induced activation was restored by applying n-3 epoxins. Double knockout of PAF-AH2 and Src-kinase-signaling inhibitor 1 (Srcin 1) in bone marrow-derived mast cells (BMMCs) also restored antigen-induced activation, suggesting an important role of Srcin 1 in negatively regulating FcϵRI signaling, being inhibited by n-3 epoxins (Shimanaka et al., 2017). N-3 LCPUFAs also modulate lipid raft compositions in C57BL/6 BMMCs, resulting in more fluid membrane regions and altered assembling of signaling proteins. Fat-1 mice, converting n-6 to n-3 PUFAs (43), show an abnormal shuttling of FcϵRI within lipid rafts at baseline compared to C57BL/6 mice (Wang et al., 2015). Overall, n-3 LCPUFAs may negatively modulate mast cell activation by interfering at different stages, although the tendency to be oxidized, potentially leading to mast cell activation, should be considered and may be circumvented by supplementing antioxidants (Figure 5).
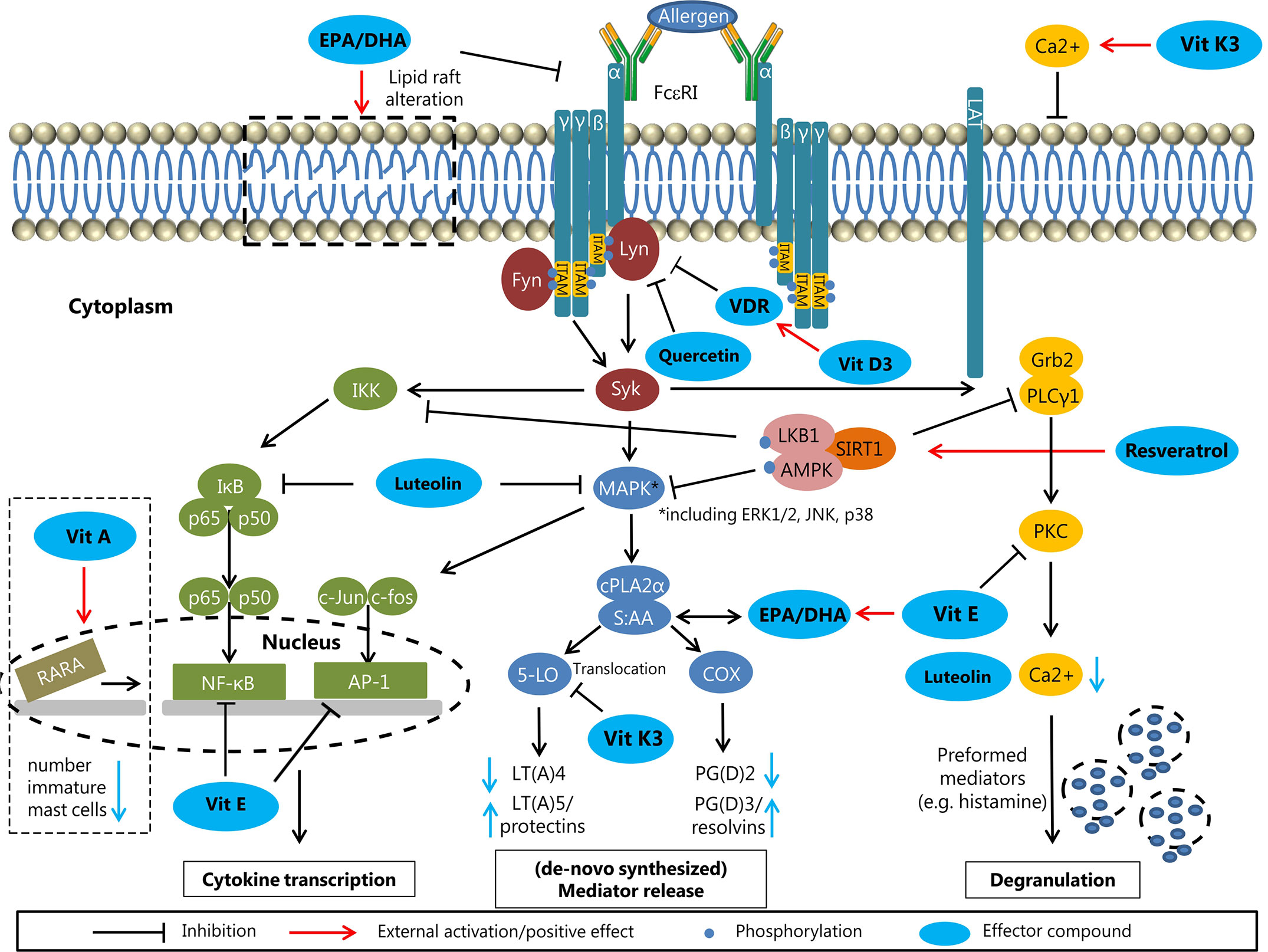
Figure 5 Molecular effects of n-3 LCPUFAs and micronutrients on effector cells (effector compounds are highlighted in blue). N-3 LCPUFAs: Alteration of the lipid raft composition as indicated at the top left, potentially affecting the assembly of FcϵRI signaling proteins and attenuating the FcϵRI signaling. Fatty acids from the phospholipid bilayer are used for the formation of lipid mediators. Replacing AA by EPA or DHA results in the production of less inflammatory mediators (LT(A)5/protectins and PG(D)2/resolvins). Vitamins: The fat-soluble vitamins A, D, and E can influence mast cell signaling at different stages. Vitamin A influences the gene transcription by RARA in the nucleus, resulting in lower numbers of immature mast cells. The metabolite vitamin D3 from vitamin D can upregulate its receptor VDR, resulting in inhibition of Lyn, an upstream protein of the FcϵRI signaling cascade. Vitamin E has the potential to inhibit the transcription factors NFκB and AP-1, resulting in less (pro-inflammatory) cytokine transcription. Phytochemicals: The flavonoids luteolin and quercetin have directly inhibitory effects on FcϵRI signaling by affecting Lyn (Quercetin) and MAPK and IκB (luteolin) upstream of the cytokine transcription and mediator forming. The stilbene resveratrol can activate the inhibitory Sirt1 complex, resulting in less cytokine transcription, less mediator forming and reduced degranulation potency. Synthetic vitamin K3 (not allowed in human) is converted into vitamin K2 in the intestine.
N-3 LCPUFAs in Pre-Clinical Allergy Models
Allergic sensitization itself has been shown to decrease serum triacylglycerol levels of EPA and DHA in mice (Ruhl et al., 2008), which may be linked to altered lipoprotein distribution (Schweigert, 2001). House dust mite (HDM) sensitized asthmatic mice had increased serum levels of AA, while di-homo-γ-linolenic acid was decreased (Fussbroich et al., 2019). In a study by Sierra et al, contact dermatitis was induced in Balb/c mice after a 3-week dietary intervention with diets containing fish oil (Sierra et al., 2008), with either 1.42% EPA or 1.42% DHA of total fatty acid content compared to the control diet. Although, both diets reduced ear inflammation (Sierra et al., 2008), the EPA-rich diet reduced the local and systemic inflammatory response and Th2 responses, while increasing IL-10 production (Sierra et al., 2008). In contrast, a diet supplemented with 5% EPA was not able to prevent AD-like dermatitis in NC/Nga mice (Lee et al., 2019) developing AD-like symptoms spontaneously (Matsuda et al., 1997). These contrasting outcomes may be explained by differences in experimental models and mouse strains, although Fat-1 mice were recently observed to exhibit markedly reduced AD-like skin lesions (Jang et al., 2018) suggesting that EPA and DHA play a protective role in AD. Cow’s milk, hen’s egg and peanut are major food allergens (Sicherer et al., 2010). In mice with orally induced hen’s egg allergy [ovalbumin (OVA)], a 13% salmon oil diet (6.1% EPA and 7.5% DHA) partially prevented development of food allergic symptoms (Hogenkamp et al., 2011) although no differences in immunoglobulin levels or effector cell-populations were observed. However, a 7% fish oil diet (15.9% EPA and 7.9% DHA) provided directly after weaning of mice prevented increase in OVA-specific IgE and IgG1 levels after subcutaneous sensitization and oral challenges with OVA (de Matos et al., 2012). Furthermore, edema, eosinophil infiltration and mucus production in the proximal jejunum were reduced in the fish oil group (de Matos et al., 2012). In mice with orally induced cow’s milk allergy (CMA), allergic sensitization and whey-induced symptoms were largely prevented by a diet in which 6% of the soybean oil was replaced by tuna oil (7.0% EPA and 27.8% DHA) (van den Elsen et al., 2013c). Mice fed the tuna oil diet had lower serum whey specific IgE levels, and Th2 and Th1-cell frequency in the mesenteric lymph nodes (MLN) and/or spleen, in sham and whey sensitized mice. Also increased frequencies of tolerogenic DCs in MLN and Foxp3+Tregs in spleen and intestinal lamina propria were observed in sensitized tuna oil fed mice (van den Elsen et al., 2013c). Adoptive transfer of these splenic Tregs protected naïve mice from allergy development (van den Elsen et al., 2013b; van den Elsen et al., 2013c). In a similar follow-up study, EPA (28.8% EPA and 13.7% DHA) or DHA (7% EPA and 27.8% DHA) rich fish oil were compared in whey or peanut sensitized mice (van den Elsen et al., 2014). Both fish oil diets attenuated the acute allergic skin response to whey, but peanut specific skin responses were only suppressed in mice fed the DHA enriched diet. Oral peanut sensitization was not prevented by either of the oils, but whey-specific IgE and IgG1 levels were lower in mice fed the DHA-enriched diet (van den Elsen et al., 2014). Hence, the quality of the fish oil (i.e. the ratio of the n-3 LCPUFAs) is of importance for its potential to prevent food allergy (van den Elsen et al., 2014). Asthma is a chronic inflammatory disorder of the respiratory tract, characterized by reversible airflow obstruction, bronchial hyperresponsiveness, and eosinophil and T-cell infiltration in the lungs (Lemanske and Busse, 2010). In murine OVA-induced allergic pulmonary inflammation, menhaden fish oil supplementation reduced pulmonary oxidative stress. Remarkably, fish oil supplementation enhanced the production of IL-5, IL-13, and reduced production of protective pulmonary PGE2 (Yin et al., 2009). Oral fish oil supplementation in a rat model for allergic airway inflammation lowered concentrations of nitrite lipid hydroperoxide, and increased activities of superoxide dismutase and glutathione peroxidase (Zanatta et al., 2014). However, fish oil did not affect proinflammatory platelet activating factor (PAF) bioactivity in lung tissue, suggesting a dual effect of fish oil on oxidative stress and inflammation in asthma (Zanatta et al., 2014). In contrast, OVA-induced allergic airway inflammation led to less severe airway inflammation and bronchoconstriction in Fat-1 mice (Bilal et al., 2011). Higher levels of endogenous n-3 LCPUFAs, and the counter-regulatory mediators protectin D1 (PD1) and resolvin E1 (RvE1) were measured in Fat-1 mice lung tissue. PD1 and RvE1 have anti-inflammatory and pro-resolving effects (Serhan et al., 2008). Interestingly, another DHA-derived pro-resolving mediator maresin-1 also suppressed murine allergic airway inflammation (Krishnamoorthy et al., 2015). Maresin-1 augmented de-novo generation of Tregs interacting with type 2 innate lymphoid cells (ILC2s) to suppress cytokine production in a TGF-β–dependent manner (Krishnamoorthy et al., 2015). In line with earlier observations (Bilal et al., 2011), OVA-specific Th-cell activation and proliferation was suppressed as a consequence of reduced T-cell receptor signaling in Fat-1 mice (Jang et al., 2014). Additionally, lower Th1 and Th2 cytokine levels in bronchoalveolar lavage fluid (BALF) of OVA-sensitized and challenged mice were found (Jang et al., 2014). Dietary fish oil intervention (6.3% menhaden oil containing 13% EPA, 12% DHA, and 0.7% soybean oil) prevented OVA-induced airway inflammation, hyperresponsiveness, eosinophilia, and airway fibrosis, accompanied by a reduction of OVA-specific IgE and Th2 and Th17 type cytokines in the lung (Bargut et al., 2013).
N-3 LCPUFAs in Allergy Prevention in Human Observational Studies
N-3 LCPUFAs blood levels in early life have been linked to allergy protection in several studies. In infants between 6 and 14 months of age, cord blood EPA and DHA and total n-3 LCPUFAs correlated negatively with AD development (50% risk reduction) (Montes et al., 2013). Breastmilk DHA levels correlated with asthma prevention at 4 years of age (60% risk reduction), and the risk for AD was reduced by 50% at 1 year and 4 years of age. However, this effect was only observed in atopic mothers with n-3 LCPUFAs levels above the median (Wijga et al., 2006). Moreover, high breastmilk n-3 LCPUFAs levels, protected against sensitization for inhalant allergens in children up to 2 years of age (50% risk reduction) (Rosenlund et al., 2016). High content of long chain fatty acids and double bond numbers in maternal blood triacylglycerols was associated with up to 40% risk reduction of food allergy in the offspring (Hong et al., 2019). High n-3 LCPUFAs plasma levels at 3 years of age (and n-6 LCPUFAs), were inversely associated with asthma and/or wheeze and allergic sensitization for inhalant and food allergens, which was most pronounced in children with high cord blood 25-hydroxyvitamin D at birth (≥ 50% risk reduction) (Lee-Sarwar et al., 2019). Imprinting effects of early n-3 LCPUFAs exposure were still observed in children at 6–7 years of age (Stratakis et al., 2018). Strikingly, n-3 LCPUFAs EPA and DHA, and a higher total n-3/n-6 LCPUFA ratio were associated with a risk reduction in wheeze and asthma of approximately 50%, but not with allergic rhinitis or AD. However, in another recent study, higher n-3 LCPUFAs blood levels at 8 years of age were associated with a 20-30% risk reduction for aeroallergen sensitization, rhinitis and asthma (Magnusson et al., 2015). Of note, n-6 LCPUFA AA was also linked with allergic asthma and rhinitis protection and remission of airway allergies within this period. In young adults, n-3 LCPUFAs plasma levels were no longer associated with protection against airborne and food allergens sensitization (Woods et al., 2004). Interestingly, asthma severity was negatively associated with total n-3 LCPUFAs plasma levels and bronchial hyperreactivity with DHA, while n-6 LCPUFAs did not show protective effects. Also in adults diagnosed with asthma, EPA blood levels were associated with a lower risk of non-specific bronchial hyperresponsiveness (aOR 0.66), while AA increased this risk (aOR 1.21) (Adams et al., 2018). In addition, in serum or PBMCs of adult AD patients, n-6 LCPUFA patterns were found to differ from healthy controls, and in the skin AA levels and some of its metabolites were significantly increased (Lindskov and Holmer, 1992; Mihály et al., 2013; Mihály et al., 2014; Töröcsik et al., 2019).
These observational studies consistently show strong positive associations between cord blood or plasma n-3 LCPUFAs levels in current and long-term allergy protection during infancy. In addition, altered LCPUFA status and enhanced AA levels are linked with current asthma or AD in adults. However, although these studies do correct for lifestyle factors, no direct causal relationships can be drawn from observational studies.
N-3 LCPUFAs in Placebo Controlled Dietary Intervention Studies
Placebo-controlled dietary intervention studies mostly use fish oil as an n-3 LCPUFAs source, and early life intervention already starts during early gestation. In the DOMinO trial, pregnant women (n=706) with a familiar risk of allergy were provided daily with 900 mg n-3 LCPUFAs (1.5 g fish oil DHA concentrate) starting from 21 weeks of gestation until delivery, but this intervention did not protect the offspring against airborne and food allergen sensitization, nor allergy (rhinitis, eczema, wheeze) at 3 or 6 years of age (Best et al., 2018). However, at 1 year of age, hen’s egg sensitization was reduced by more than 30%, and at 1–3 years of age the prevalence of AD was lowered by 25% (tendency p=0.10) (Palmer et al., 2012; Palmer et al., 2013). In an earlier study, atopic women (n=98) were provided a higher dose n-3 LCPUFAs (3.7 g) starting from week 20 of gestation until delivery. This resulted in a mean increase of 4% n-3 LCPUFAs in the erythrocyte membranes of the neonates at the expense of n-6 LCPUFA AA, and slightly reduced ex-vivo allergen specific cytokine release of cord blood mononuclear cells (CBMNCs) (Dunstan et al., 2003b). Additionally, hen’s egg sensitization (skin prick test) was reduced by more than 50% and although the frequency of AD at 1 year of age was unaltered, children in the fish oil group had significantly less severe symptoms with the Scoring atopic dermatitis (SCORAD) test. IL-13 plasma levels were also lower in the neonates, correlating with an increase of n-3 LCPUFAs incorporated in cell membranes (Dunstan et al., 2003a). Daily administration of 0.65g n-3 LCPUFAs (fish oil) during pregnancy enhanced mRNA levels of regulatory TGF-β in both maternal blood and cord blood, while IL-1 and interferon (IFN)-γ plasma levels were reduced in the mothers and IL-4, IL-13 were lowered in the neonatal cord blood (Krauss-Etschmann et al., 2008). The protective effect of n-3 LCPUFAs may result from epigenetic changes when provided early in life or during pregnancy, hereby affecting the health outcome also later in life. Daily administration of 400 mg DHA starting from day 18–22 of gestation until delivery resulted in enhanced methylation of both IL-13 and IFN-γ gene transcription sites compared to the control group, hence lowering their expression (Lee et al., 2013). The preventive effect of n-3 LCPUFA intervention may further increase when also provided during breastfeeding. Daily maternal supplementation with 2.7 g n-3 LCPUFAs starting from week 25th of gestation until 3rd–4th month of breastfeeding in an at risk population significantly lowered the prevalence of AD and food allergy in the offspring at 1 year of age (Furuhjelm et al., 2009). Even though this encouraging study concerned a small cohort, it appeared that the dosing, timing, and duration of maternal intervention may have contributed to this successful prevention of allergy in some of these children. This protective effect was still measured at 2 years of age, although new cases were not prevented. Children (or their mothers) with the highest EPA and DHA plasma levels had the lowest chance of developing allergy and severity of developed allergies was reduced (Furuhjelm et al., 2011). Providing asthmatic women or their offspring with a lower daily dose of n-3 LCPUFAs (158 mg from week 36 of gestation until 6 months of breastfeeding or formula feeding) did not prevent allergic sensitization. However, it reduced wheeze and bronchodilator use especially in offspring with relatively high n-3 LCPUFAs plasma levels at 18 month and atopic cough at 3 years of age (Mihrshahi et al., 2004; Peat et al., 2004). In another study, high risk children were supplemented with n-3 LCPUFAs (390 mg) from birth until 6 months of age. Although HDM IgE levels were reduced at 1 year of age, the children were not protected against food allergy or wheeze. However, infants without wheeze at 1 year of age had significantly higher n-3 LCPUFAs plasma levels and lower allergen specific Th2 responses (D’Vaz et al., 2012a; D’Vaz et al., 2012b). Formula milk with added DHA and n-6 LCPUFA AA reduced the risk of all allergies in children from the general population, while the prevalence of wheezing was reduced in children at risk in the first year of life (Foiles et al., 2016). Furthermore, daily supplementation of 2.4 g n-3 LCPUFAs (fish oil) in 736 pregnant women from week 24 of gestation until 1 week after delivery reduced the infant’s risk on pediatrician diagnosed persistent wheeze or asthma for 30% at 3 to 7 years of age. This was associated with reduced risk of lower respiratory tract infections as compared to a control group supplemented with olive oil (Bisgaard et al., 2016). The protective effect was particularly evident upon supplementation of women with low EPA and DHA blood levels (<4.3%), resulting in a risk reduction of more than 50%. Low blood levels were due to low n-3 LCPUFAs intake and a specific FADS genotype hampering LCPUFA conversion. The n-3 LCPUFAs supplementation appeared to be less effective in children receiving high dose of vitamin D3 (Bisgaard et al., 2016). In asthmatic infants (8–12 years), daily n-3 LCPUFAs (1.2 g) supplementation for 6 months reduced inflammatory marker TNF-α in plasma. However, no clinical benefit was observed although blood eosinophilia tended to reduce (p=0.011) (Hodge et al., 1998). Similar results were obtained in adult allergic asthmatics [5 weeks daily 0.7 g n-3 PUFA (630 mg DHA+EPA)], although pro-inflammatory markers as exhaled nitric oxide and the number of sputum and serum eosinophils reduced (Schubert et al., 2009). Pollen-induced hay fever and asthma in adults was also not prevented by a 6 month intervention with daily 5.4 g of EPA and DHA (Thien et al., 1993). These studies indicate that early life intervention with n-3 LCPUFAs may help to reduce the asthma risk, but treatment of allergic asthma using n-3 LCPUFAs is not effective in lowering symptoms. In contrast, daily supplementation of 5.2 g of EPA and DHA for 3 weeks did ameliorate exercise-induced bronchoconstriction in asthmatics, as determined by improved lung function and reduced bronchodilator use. This was associated with reduced sputum concentrations of pro-inflammatory LTC4-LTE4 and LTB4, PGD2, IL-1β and TNF-α and an increase on LTB5 (Mickleborough et al., 2006). A more recent study showed a protective effect when using 3.1 g per day (Williams et al., 2017).
Hence, n-3 LCPUFA may modulate allergic sensitization and/or inflammation as indicated in these clinical trials. Fat-soluble components like vitamins, polyphenols, stilbenes, and carotenoids may be capable of adding to these effects, since pre-clinical studies clearly show their potential in allergy protection.
Effects on Allergic Inflammation by Fat-Soluble Micronutrients
Vitamin A
Vitamin A (VitA) plays a key role in various processes such as embryogenesis (Metzler and Sandell, 2016) and immune function (Julia et al., 2015). This dietary component is obtained from plants as carotenoids (β-carotene) or from animal-derived food sources as retinyl esters or all-trans-retinol (Blomhoff and Blomhoff, 2006; Li et al., 2014). After uptake, VitA is eventually oxidized to all-trans-retinoic acid (RA) by retinal dehydrogenases (RALDHs) (Blomhoff and Blomhoff, 2006) expressed in epithelial cells (Lampen et al., 2000; Iwata et al., 2004) and DCs (Iwata et al., 2004; Coombes et al., 2007) in the gut. RA is a high-affinity ligand for the nuclear receptors RA receptor-α (RARα), RARβ, and RARγ forming heterodimers with RXR. RXR/RAR heterodimers can bind to RAR response elements (RAREs) or RXR response elements (RXREs) in the promoter regions of target genes, thereby controlling gene transcription in various cells including immune cells (Chambon, 1996; Raverdeau and Mills, 2014). In addition, carotenoids can affect the NFκB pathway, which decreases DNA-binding activity and inhibits inflammatory cytokines (Kaulmann and Bohn, 2014).
Vitamin A and DCs and T-cells
VitA is important for DC and T-cell functioning. Intestinal DCs express high levels of RALDH2, allowing them to synthesize RA (Iwata et al., 2004; Coombes et al., 2007). RA regulates the development and homeostasis of intestinal CD11b+(CD103+)+CCR7+ DCs (Klebanoff et al., 2013), which can induce gut-tropism and gut-homing in T-cells by inducing CCR9 (C-C chemokine receptor 9) and α4β7 expression (Johansson-Lindbom et al., 2003; Mora et al., 2003; Iwata et al., 2004). Importantly, DC-derived RA enhanced TGF-β driven differentiation of naïve T-cells into Tregs (Coombes et al., 2007; Mucida et al., 2007) and inhibited development of Th17-cells (Xiao et al., 2008). Moreover, VitA inhibits Th1 and supports Th2 differentiation (Iwata et al., 2003) by inducing IL-4 expression (Lovett-Racke and Racke, 2002), but it may also indirectly promote Th2 differentiation by modulating DCs (Hoag et al., 2002). Favoring Th2 responses in the gut can stimulate protective effects on the gut mucosa, but it may also enhance allergic responses (Schuster et al., 2008; Matheu et al., 2009). However, VitA deficiency has been shown to increase airway hyperreactivity (AHR) in humans (Chen et al., 2014) and MLN DCs from vitamin-A deficient mice induce inflammatory Th2-cells (Yokota-Nakatsuma et al., 2014). Furthermore, RA supplementation inhibited detrimental Th17 responses, while promoting Treg responses in a murine asthma model (Zhao et al., 2013). It is possible that these contrasting outcomes depend on the RA availability, i.e. RA may induce Tregs and inhibit or promote Th17 differentiation dependent on the concentration of biologically available RA (Julia et al., 2015) (Figure 3).
Vitamin A and Mast Cells
Compared to other vitamins, VitA surprisingly has a potential pro-inflammatory effect on mast cells. Ex-vivo stimulation of human-derived skin mast cells with RA increased the secretion of IL-1β, IL-6, IL-8, and TNF-α in a dose-dependent manner by binding of RA to the RARα (Babina et al., 2015). One of the most important effects of this mechanism is proliferation restriction in mast cells whereby immature mast cells are the dominated target (Kinoshita et al., 2000; Hjertson et al., 2003). The anti-proliferative trait of RA has been described for human mast cells in and ex-vivo, HMC-1-cells and murine peritoneal mast cells (Astorquiza et al., 1980; Alexandrakis et al., 2003; Ishida et al., 2003). Moreover, RA can enhance degranulation of human-skin derived mast cells, probably due to the wide range of genes regulated by RARα (Babina et al., 2017). Overall, the potential effect of VitA in humans must be critically evaluated since VitA can in fact diminish the number of mast cells, but has a potential pro-inflammatory effect. The combination with VitD and VitE might have beneficial impact on mast cell stabilization since these vitamins can negatively regulate FcϵRI signaling and subsequently diminish degranulation (Figure 5).
Vitamin A in Pre-Clinical Allergy Models
As outlined above, VitA and its metabolite RA can affect immune responses substantially and may therefore have impact on allergic outcomes. In a murine model for OVA-induced allergic asthma, RA supplementation attenuated airway inflammation and decreased Th17 and Th2 differentiation and functions while promoting Treg differentiation, which is partly in contrast to in-vitro observations (Wu et al., 2013). Lower Th17 and greater Treg responses were also observed by Zhao et al. in a chronic asthma model (Zhao et al., 2013). Interestingly, RA supported OVA-specific oral tolerance induction in OVA-sensitized mice and reduced OVA-induced AHR of recipient mice transferred with pulmonary Th-cells of tolerized RA treated donor mice, implying an immune imprinting effect of RA (Sakamoto et al., 2015). In line with these findings, VitA deficiency exacerbated lung inflammation and type 2 cytokine production in a similar murine model for allergic asthma (Cui et al., 2016). However, others have reported that disease severity in allergic asthma can be attenuated by VitA deficiency, while excessive VitA intake exacerbates pulmonary hyperresponsiveness (Schuster et al., 2008), indicating a small therapeutic window for VitA.
Vitamin A in Human Allergy Prevention
The impact of VitA supplementation on human allergic diseases has recently been reviewed by Hufnagl et al. (Hufnagl and Jensen-Jarolim, 2019). Lower levels of serum VitA have been observed in asthmatic children and adults (Allen et al., 2009; Nurmatov et al., 2011), although other studies do not confirm this (Hamalainen et al., 2017). Maternal intake of VitA has been suggested to lower the risk of asthma in children (Maslova et al., 2014), although other studies did not show this (Checkley et al., 2011; Nwaru et al., 2011). Neonatal supplementation with VitA is recommended in areas with VitA deficiency (serum (plasma) retinol <0.35 µmol/l), but this does not affect the risk of atopy (Kiraly et al., 2013). Similarly, dietary VitA supplementation in children does not affect allergic outcomes (Kim et al., 2016), although a systematic review showed weak but nonetheless supportive effects of VitA in asthma prevention (Nurmatov et al., 2011). In studies involving adults, VitA supplementation was provided in the context of dietary interventions such as the Mediterranean diet, but not as a single component (Hufnagl and Jensen-Jarolim, 2019). Increasing the dietary intake of fruit and vegetables rich in carotenoids can improve lung function and reduce risk of asthma exacerbation, associated with decreased systemic inflammation (Wood et al., 2012).
Vitamin D3
Humans acquire vitamin D (VitD) primarily from exposure to sunlight. Vitamin D3 (VitD3) is synthesized in the skin from a derivative of cholesterol (Holick, 2007). In addition, VitD3 (or VitD2) can be obtained from the diet. In the liver, 25-hydroxylase (CYP2R1) hydroxylates VitD3 to 25-dihydroxyvitamin D3 (25(OH)D3), which is measured to assess VitD status (DeLuca, 2004). 25(OH)D3 is metabolically inactive and must be hydroxylated in the kidneys by 1-a-hydroxylase (CYP27B1) to 1,25(OH)2D3 (Holick et al., 1972). Interestingly, CYP27B1 is expressed in various immune cells, but only macrophages and some DCs also express CYP2R1, allowing these latter cell types to convert VitD3 to 1,25(OH)2D3 (Monkawa et al., 2000; Fritsche et al., 2003; Chen et al., 2007; Sigmundsdottir et al., 2007).
1,25(OH)2D3 binds to the Vitamin D receptor (VDR), a member of the nuclear receptor superfamily (Kutner and Brown, 2018). VDR heterodimerizes with nuclear receptors of the RXR family after binding to 1,25(OH)2D3, allowing the VDR-RXR heterodimer to bind Vitamin D Responsive Elements (VDREs) (Kutner and Brown, 2018). Depending on the target gene, this induces or represses gene transcription (Baeke et al., 2010; Haussler et al., 2011).
Vitamin D3 and DCs and T-Cells
1,25(OH)2D3 decreases expression of MHC class II molecules and of CD40, CD80 and CD86 on DCs, thereby downregulating differentiation, maturation and immunostimulatory capacity (Penna and Adorini, 2000; Griffin et al., 2001; Fritsche et al., 2003; van Etten and Mathieu, 2005). 1,25(OH)2D3 produced by DCs also leads to skin tropism by inducing CCR10 expression in T-cells (Sigmundsdottir et al., 2007), suggesting DCs can respond to local metabolites and subsequently direct the imprinting of tissue-specific tropism in T-cells (Iwata et al., 2004; Dudda et al., 2005; Sigmundsdottir et al., 2007). Additionally, 1,25(OH)2D3 suppresses IL-12 (D’Ambrosio et al., 1998; Penna and Adorini, 2000) and increases IL-10 production by DCs (Penna et al., 2006), suggesting that 1,25(OH)2D3 can steer DC-driven differentiation of T-cells, but 1,25(OH)2D3 also suppresses IL-2 expression in T-cells, potentially inhibiting their proliferation (Lemire et al., 1984; Lemire et al., 1985; Alroy et al., 1995). Furthermore, VitD3 treatment results in polarizing murine T-cells towards a Th2 phenotype (IL-4 release) (Boonstra et al., 2001), but others did not confirm this (Staeva-Vieira and Freedman, 2002). Furthermore, 1,25(OH)2D3 can suppress IL-6 and IL-23 synthesis (Penna et al., 2006; Daniel et al., 2008), which decreases Th17-responses. Interestingly, 1,25(OH)2D3 can also induce FoxP3 expression in T-cells (Penna et al., 2005; Gorman et al., 2007; Daniel et al., 2008), which—taken together with the findings discussed above—suggests that 1,25(OH)2D3 induces regulatory responses while inhibiting Th1 and Th17 immune outcomes. However, it is not clear whether involvement of 1,25(OH)2D3-driven DC responses are necessary for Treg induction as this is also observed in absence of DCs (Barrat et al., 2002; Jeffery et al., 2009) (Figure 3).
Vitamin D3 and Mast Cells
In epidemiological studies, deficiency of VitD has been associated with severe asthma, and spontaneous mast cell release is increased in VitD-deficient BALB/c mice (Poon et al., 2013; Liu et al., 2017). Vice versa, BALB/c mice fed a VitD-supplemented diet exhibited lower levels of histamine and TNF-α, indicators of mast cell release, compared to mice fed VitD-deficient or VitD-sufficient diets, suggesting stabilization of mast cells by VitD (Liu et al., 2017). This stabilization might be explained by upregulation of VDR expression by 1,25(OH)2D in-vitro (Babina et al., 2000; Baroni et al., 2007; Yip et al., 2014) VDR, in turn, binds to the tyrosine-protein kinase Lyn resulting in decreased phosphorylation of Syk and activation of the MAPK complex and NFκB in murine BMMCs. Moreover, VitD decreased TNF-α levels by binding to the promotor region of the TNF-α transcript (Liu et al., 2017). Impaired TNF-α expression upon VitD supplementation has also been observed in human CBMCs (Yip et al., 2014). These effects on mast cell modulation suggest a potential treatment option to attenuate allergic symptoms, especially in patients with severe reactions (Figure 5).
Vitamin D3 in Pre-Clinical Allergy Models
VitD deficiency affects lung function and volume in mice, which may affect pulmonary health (Zosky et al., 2011). Indeed, in mice sensitized to OVA at 8 weeks of age, perinatal VitD deficiency increased the capacity of airway-draining lymph node cells to proliferate in response to OVA stimulation ex-vivo (Gorman et al., 2013). Although increases in OVA-specific cytokine production were observed, pulmonary cell infiltration, BALF cytokine levels and serum IgE levels were unaffected by VitD deficiency (Gorman et al., 2013). In contrast, VitD deficiency worsened AHR, pulmonary eosinophilia, increased BALF pro-inflammatory cytokines and reduced IL-10 levels and lowered numbers of Tregs in OVA-sensitized mice at 6 weeks of age (Agrawal et al., 2013). Furthermore, VitD supplementation attenuated the pro-inflammatory effects, although allergic airway inflammation was not completely reversed (Agrawal et al., 2013). The contrast between these two studies may be explained by the timing and doses for OVA sensitization. Perinatal VitD deficiency induces Th2 skewing and a reduction of IL-10 secreting Tregs (Vasiliou et al., 2014). This was further enhanced in HDM-sensitized mice at one week of age. Although VitD insufficiency in early life did not affect AHR in this study, eosinophilic inflammation and airway remodeling was more severe in VitD-deficient mice sensitized to HDM. VitD supplementation after weaning reduced serum IgE levels, pulmonary eosinophilia and airway remodeling (Vasiliou et al., 2014). OVA-specific IgE and IgG1 levels were increased in VitD deficient mice (Heine et al., 2014), and co-administration of 1,25(OH)2D3 enhanced OVA-specific immunotherapy indicated by reduced allergic airway inflammation and AHR. However, treating OVA-sensitized mice with intraperitoneal 1,25(OH)2D3 at the time of intranasal OVA-challenge reduced allergic inflammation in non-deficient mice (Lai et al., 2013; Wang et al., 2016). Hence, indicating an important role in maintaining pulmonary homeostasis and allergic asthma protection.
VitD deficiency also exacerbated food allergic symptoms in mice sensitized intraperitoneally to OVA, which was suggested to be mediated by increased expression of IL-4 in MLN (Matsui et al., 2018). VitD deficiency could be involved in the development of food allergy, potentially by modulating immune responses and maintaining intestinal microbe homeostasis (Vassallo and Camargo, 2010). In humans, full VitD3 deficiency is unlikely, however reduced serum VitD3 levels are often observed.
Vitamin D3 in Human Allergy Studies
VitD status has been linked to differences in geographical locations with different degrees of sun exposures (Yeum et al., 2016). Interestingly, sun exposure has been inversely related to food sensitization during infancy (Matsui et al., 2015), suggesting a link between VitD status and risk of allergic sensitization. However, data from studies are inconclusive. Some studies report that allergic sensitization is more common in children and adolescents with low 1,25(OH)2D3 levels (Sharief et al., 2011; Baek et al., 2014). Furthermore, low cord blood VitD levels have been associated with increased cow’s milk sensitization but not with asthma, AD, or allergic rhinitis in early childhood (Chiu et al., 2014). Additionally, VitD insufficiency increased the risk of developing food allergies up to 11-fold in infants of Australian parents, depending on the allergen (Allen et al., 2013). Others have made similar associations as higher rates of VitD deficiency were found in children with persistent egg allergy (Neeland et al., 2018). In contrast, high maternal 1,25(OH)2D3 status has also been associated with increased risk of allergic disease in the offspring (Hansen et al., 2015). Another study reported that VitD deficiency during the first 6 months of infancy was not associated to an increased risk for food allergy at 1 year of age (Molloy et al., 2017). Moreover, no convincing associations between prenatal VitD status and allergic outcomes in childhood were found in a recent meta-analysis (Pacheco-Gonzalez et al., 2018).
The effects of (maternal) supplementation with VitD on food allergy development are also conflicting. One study reported inverse associations between maternal intake of VitD and sensitization to food allergens at 5 years of age (Nwaru et al., 2010). In contrast, in a randomized, double-blind, placebo-controlled trial maternal supplementation with VitD did not improve infant AD but rather appeared to increase the risk of developing food allergy (Norizoe et al., 2014). To further complicate matters, VitD supplementation during pregnancy increased the risk of food allergy in the offspring, whereas food-derived VitD during pregnancy was associated with a decreased risk (Tuokkola et al., 2016). However, no correlation between maternal VitD supplementation during pregnancy and food sensitization in the offspring at 2 years of age were found (Savage et al., 2018).
VitD-related outcomes for pulmonary (allergic) diseases appear to differ from food allergic outcomes. In a long-term prospective study, early life 1,25(OH)2D3 deficiency was associated with increased risk of persistent asthma at 10 years of age (Hollams et al., 2017; Pfeffer and Hawrylowicz, 2018), possibly resulting from the association between 1,25(OH)2D3 deficiency and increased risk for early allergic sensitization and upper respiratory tract colonization with bacterial pathogens (Hollams et al., 2017). Based on a systematic review and meta-analysis, VitD supplementation reduces the rate of asthma exacerbations in patients requiring treatment with systemic corticosteroids (Jolliffe et al., 2017). Furthermore, maternal VitD supplementation reduced the risk of persistent wheeze in the offspring throughout the first 3 years of life (Chawes et al., 2016). Additionally, VitD tended to reduce the incidence of asthma and recurrent wheezing in children from pregnant women at risk (Litonjua et al., 2016). In conclusion, it appears that—depending on the window of opportunity and the overall VitD status—VitD would contribute to maintaining homeostasis and may counteract the development of allergic disease.
Vitamin E
VitE is the umbrella term for four tocopherols (α–δ- tocopherol) and four tocotrienols (α–δ-tocotrienol) (Traber, 2007), mainly available in edible oils (Slover, 1971). α-Tocopherol and γ-tocopherol are most abundant in food, and although the intake of γ-tocopherol from the diet is generally higher, α-tocopherol is predominantly found in mammalian plasma and tissues (Wolf, 2006). Both α-tocopherol and γ-tocopherol are lipid peroxyl radical scavengers, making them potent antioxidants (Jiang, 2014). Additionally, γ-tocopherol is capable of detoxifying nitrogen dioxide and peroxy-nitrite (Jiang, 2014). However, these tocopherols also affect signal transduction (e.g. modifying protein C kinase (PKC) activity (Mahoney and Azzi, 1988)). The outcome is dependent on the isoform, as α-tocopherol acts as an antagonist and γ-tocopherol is an agonist of PKC, leading to opposing roles in inflammation (Cook-Mills, 2013). α-Tocopherol can stimulate cyclic adenosine monophosphate (cAMP) production in human PBMCs, thereby attenuating proinflammatory cytokine and chemokine production (Salinthone et al., 2013). Moreover, VitE can affect the activity of many transcription factors like PPARγ and NFκB via modulation of signal transduction enzymes (Zingg, 2015). Interestingly, lipid rafts can also be altered by VitE, resulting in altered membrane protein interaction and translocation, modified signal transduction (Zingg, 2015). Overall, VitE appears to modulate cell functioning at multiple levels which cannot only be explained by its antioxidant function.
Vitamin E and DCs and T-Cells
VitE reduces human moDCs activation upon proinflammatory cytokine stimulation (Tan et al., 2005) and the capacity of DCs to induce T-cell proliferation, resulting in generation of anergic T-cells that have regulatory properties, suggesting a role for VitE in tolerance induction (Tan et al., 2005). Similarly, α-tocopherol exposure of murine BMDC lowered LPS-induced maturation (Xuan et al., 2016). Interestingly, maternal supplementation with α-tocopherol to allergic female mice reduced numbers of pulmonary CD11b+ DCs, but not CD11b− DCs (Abdala-Valencia et al., 2014), while γ-tocopherol exerts an opposing effect (Abdala-Valencia et al., 2016). This points to an important role of the different isoforms of VitE, as shifts in different subsets of DCs could affect maintenance of tolerance and the development of allergy. Notably, high average human plasma γ-tocopherol levels (relatively abundant in soy bean oil) are reported in countries with the highest asthma prevalence (Abdala-Valencia et al., 2014). The effects of VitE on T-cells have been studied in relation to immunosenescence, where VitE showed improved proliferative ability of old T-cells (Lee and Han, 2018). Furthermore, re-stimulated human PBMCs were protected from apoptosis in the presence of VitE accompanied by a reduction in CD95L expression, suggesting a protection from activation-induced cellular death (Li-Weber et al., 2002). VitE also dose-dependently reduced IL-4 production in activated human peripheral T cells (Li-Weber et al., 2002). Interestingly, this effect was observed both in T-cells isolated from allergic patients as well as in T-cells from non-allergic donors (Li-Weber et al., 2002), suggesting a role for VitE in modulating the Th1/Th2 balance (Han et al., 2006) (Figure 3).
Vitamin E and Mast Cells
In an allergic dermatitis mouse model (NC/Nga), scratching behavior, epidermis thickness and serum histamine levels upon sensitization were lower in mice fed a VitE supplemented diet (Tsuduki et al., 2013). Additionally, OVA-sensitized Brown Norway rats experienced less severe reaction upon challenge after treatment with VitE between sensitization and challenge, accompanied by reduced eosinophil infiltration (Wagner et al., 2008). As a potential mechanism, in-vitro experiments (HMC-1) showed inhibition of NFκB predominately reducing phosphorylation of protein kinase B (PKB). Hence, VitE can directly interfere with FcϵRI signaling (Kempna et al., 2004) (Figure 5).
Vitamin E and Pre-Clinical Allergy Mouse Models
Use of experimental animal models has led to further insight in the role of VitE on allergy. In a model for OVA-induced allergic airway inflammation, pulmonary levels of IL-5 and plasma levels of IgE were blunted in VitE deficient allergic mice (Lim et al., 2008). However, no differences in pulmonary eosinophils were observed, suggesting that the effects of VitE deficiency are most pronounced for early sensitization to allergens (Lim et al., 2008). The observed effects are possibly induced by Th1 skewing, as VitE supplementation has been shown to enhance antiviral Th1 responses in old mice (Han et al., 2000). In addition, a dose-dependent reduction of allergic inflammation in the offspring of α-tocopherol supplemented allergic mice was observed (Abdala-Valencia et al., 2014), whereas lung eosinophilia, inflammatory mediators and inflammatory CD11b+ DCs increased in offspring of γ-tocopherol supplemented allergic mice (Abdala-Valencia et al., 2016). In addition, daily administration of α-tocopherol during the OVA-challenge decreased pulmonary eosinophils and monocytes infiltration, γ-tocopherol treatment however increased airway inflammation (Berdnikovs et al., 2009). Similar anti-inflammatory effects of α-tocopherol supplementation have been described linking to protection against mitochondrial dysfunctions related to asthmatic inflammation (Mabalirajan et al., 2009). Interestingly, in mice treated with both γ-tocopherol and α-tocopherol, the beneficial effects of α-tocopherol were inhibited (Berdnikovs et al., 2009). These proinflammatory effects of γ-tocopherol could be partly reversed by supplemental levels of α-tocopherol (McCary et al., 2011).
Vitamin E in Human Allergy Studies
In a recent systematic review, maternal supplementation with VitE during pregnancy was found to reduce odds of asthma development and was negatively associated with childhood wheezing (Wu et al., 2018). However, the opposing effects of α-tocopherol and γ-tocopherol were not considered in this study. In asthmatics, α-tocopherol levels in airway fluid were reduced, although plasma concentrations were normal (Kelly et al., 1999; Kalayci et al., 2000). Supplementation with α-tocopherol of asthmatic patients decreased allergic inflammation and AHR (Hoskins et al., 2012), although older studies demonstrated mixed effects on wheeze (Troisi et al., 1995; Dow et al., 1996; Weiss, 1997; Smit et al., 1999; Tabak et al., 1999). These differential outcomes may reflect the opposing effects of α-tocopherol and γ-tocopherol, as beneficial effects were found in Italy and Finland (low average γ-tocopherol levels), but not in the United States or Netherlands (high average γ-tocopherol levels) (Cook-Mills et al., 2013). Overall, α-tocopherol has anti-inflammatory effects with potential clinical significance for the treatment of allergic lung diseases, but dietary patterns should be considered.
Vitamin K
Vitamin K (VitK) exists in two natural forms, phylloquinone (K1) and menaquinone (K2), and in a synthetic form named menadione (vitamin K3). The number of studies examining the effects of VitK on immune functioning is limited, therefore, this section will briefly discuss VitK in relation to allergy (development).
In human PBMCs, VitK2, but not VitK1, inhibited T-cell proliferation (Myneni and Mezey, 2018) and VitK2 derivatives inhibit proliferative responses and cytokine production by T-cells isolated from human PBMCs (Checker et al., 2011; Hatanaka et al., 2014). However, in human macrophagic THP-1 cells, VitK1 suppressed IL-6 production (Ohsaki et al., 2006), and dietary supplementation with VitK1 suppressed LPS-induced inflammation in rats (Ohsaki et al., 2006). The underlying mechanism may be linked to suppression of extracellular signal-regulated kinases (ERK), c-Jun N-terminal kinases (JNK) and NFκB in lymphocytes (Checker et al., 2011). Interestingly, VitK derivatives were also observed to increase Treg cell-frequencies in activated PBMCs (Hatanaka et al., 2014). In RBL-2H3, a model for mast cells, pre-incubation with VitK3 slightly inhibited degranulation and Ca2+ influx (Kawamura et al., 2006). Additionally, VitK3 treatment dose-dependently decreased leukotriene-secretion without affecting ERK or p38 phosphorylation (Kawamura et al., 2010). However, VitK3 is converted to VitK2 in the intestine but currently not allowed as a supplement for humans.
Taken together, these potential anti-inflammatory effects may point to an interesting role for VitK in allergy prevention/treatment. This is consistent with the findings from the Framingham Offspring Study in which inverse associations between VitK status and inflammatory biomarkers were observed (Shea et al., 2008). However, further investigation are warranted as data from the Danish National Birth Cohort suggest that maternal VitK intake increases the risk of admitted asthma and current asthma in 7-year-old children (Maslova et al., 2014).
Luteolin and Quercetin
Fat-soluble phytochemicals can be separated into flavonoids and non-flavonoids. Flavonoids are polyphenolic components consisting of a three hydroxyflavone backbone and a diversity of side chains, making up 2,000 different components which are categorized in subgroups: flavones, flavanones, flavonols, flavononols, isoflavones, flavanols (catechins), and anthocyanidins. The average total human intake of flavonoids is 100–650 mg per day (Pan et al., 2010). Luteolin and quercetin act as anti-oxidants, with luteolin being more potent than several types of polyphenols including quercetin and resveratrol (Hofer et al., 2014). Quercetin however is also far more effective than VitE and VitC in lowering lipid peroxidation of albumin bound linoleic acid and PUFA oxidation (Fremont et al., 1998; Dufour and Loonis, 2007). Additionally, quercetin accumulates in mitochondria and protects against lipid peroxidase-induced mitochondrial damage (Fiorani et al., 2010). Both luteolin and quercetin are known for their anti-inflammatory capacity, lowering LPS-induced activation of AKT, MAPK (p38) and NFκB signaling cascades and pro-inflammatory cytokine release, possibly via disruption of lipid raft formation (Kim and Jobin, 2005; Kaneko et al., 2008). In addition, a metabolic breakdown product of quercetin was identified as competitive inhibitor of LOX (Borbulevych et al., 2004). However, pharmacokinetic studies showed poor absorption and fast metabolism of bioactive flavonoids like quercetin due to metabolization by gut microbiota. Currently, drug delivery systems are being developed to enhance delivery and bioavailability (Caddeo et al., 2019).
Luteolin and Quercetin and DCs and T-Cells
Luteolin completely blocked LPS-induced TNF-α and IL-12 expression in BMDCs, and i.p. injections prevented NFκB activation of LPS-activated PBMCs and splenocytes in-vivo (Kim and Jobin, 2005; Kim et al., 2019). Similarly, quercetin lowered TNF-α and IL-6 release by LPS-activated BMDCs, which was causally related to induced expression of secretory leukoprotease inhibitor (SLPI) known to suppress LPS-induced NFκB activation (Cavalcanti et al., 2014; De Santis et al., 2016). Quercetin improved extracellular iron transport contributing to an anti-inflammatory DC phenotype (Galleggiante et al., 2017). However, luteolin not only lowered IL-4 and IL-13 release by human basophils, but also IL-4 by human PBMCs with an IC50 at much lower dosing than quercetin (Hirano et al., 2004). Luteolin also inhibited murine and human inflammatory T-cell responses, while quercetin was ineffective (Verbeek et al., 2004). Furthermore, luteolin reduced effector DC maturation and T-cell responses via AKT/mTOR inhibition, while enhancing Treg responses in mice equally effective as rapamycin (Ye et al., 2019). However, in mice orally provided with lipid droplets containing quercetin and piperin, DC trafficking and consequent antigen-specific T-cell proliferation in lymph nodes was also strongly reduced (Delvecchio et al., 2015). A diet containing 0.1% quercetin prevented rhinovirus-induced exacerbation of COPD and airway inflammation (Farazuddin et al., 2018). In BMDCs quercetin enhanced disabled adapter protein (DAB) expression, linked to suppression of DC maturation, and reduced activation of NFκB and AKT signaling proteins (Lin et al., 2017). In addition, quercetin enhanced DAB2 expression in human DCs, and suppressed DC maturation via binding of AhR while inducing a regulatory DC phenotype directing regulatory T-cell development and TGF-ß release and inhibiting activation of Th-cells (Michalski et al., 2020). Hence, these studies clearly show the anti-inflammatory actions of both luteolin as well as quercetin by silencing DC activation and consequent T-cell proliferation, while supporting immunoregulatory functions (Figure 3).
Luteolin, Quercetin, and Mast Cells
Luteolin metabolites are characterized as IL-6 and COX-2 inhibitors (Quan et al., 2013) and luteolin inhibited secretion of pro-inflammatory cytokines like IL-1ß and TNF-α in HMC-1 cells (Jeon et al., 2014). These inhibitory effects may originate from reduced phosphorylation of MAPK complex, including JNK 1/2 and ERK 1/2 but not p38 MAPK. In HMC-1 cells, inhibition of the MAPK complex resulted downstream in reduced intracellular Ca2+ content, suppressed cytokine expression (IL-8, IL-6, TNF-α) and inhibition of NFκB (Kang et al., 2010). Inhibition of NFκB was also described for human CBMCs, resulting in reduced CCL2 release (Weng et al., 2015). Interestingly, luteolin can inhibit mast cell degranulation as well as its structurally related polyphenol quercetin at lower concentrations, indicating, like observed for the suppression of T-cell activation, a greater potency compared to quercetin (Baolin et al., 2004). Moreover, luteolin and its metabolite methlut were respectively 2,5 and 3 times more potent than the mast cell stabilizer chromolyn in human LAD-2 cells (Weng et al., 2015). Quercetin lowered mast cell degranulation of BMMCs and human cultured mast cells, while no effect was observed for the glycosylated form quercitrin (Kimata et al., 2000; Cruz et al., 2012). Protection by quercetin involves the inhibition of Lyn phosphorylation as assessed in human LAD2 cells and human basophilic KU812 cells. Reduced Lyn phosphorylation results indirectly in reduced phosphoinositide phospholipase C γ (PLCγ)-IP3R–Ca2+ signaling, ERK1/2 phosphorylation, IκB kinase (IKK) phosphorylation and NFκB expression (Kimata et al., 2000; Li et al., 2016; Ding et al., 2020) (Figure 5).
Luteolin and Quercetin in Pre-Clinical Allergy Models
In a guinea pig asthma model, luteolin and apigenin were more effective in suppressing acute and/or late phase allergen-induced airway hyperresponsiveness and inflammation than structurally related flavones and as effective as dexamethason (Lee et al., 2014). In a murine model for OVA-induced allergic asthma, dietary intervention with quercetin (10 mg/kg) for 5 days after sensitization but prior to and during challenge, reduced airway and systemic eosinophilia for more than 50% but less effective than dexamethasone (Rogerio et al., 2007). Quercetin (i.p.) supplementation for 3 days prior to OVA challenge reduced airway eosinophilia and AHR for more than 50%, while effectively lowering eosinophil peroxidase (EPO) activity and reducing pulmonary Th2/ILC2 markers GATA-3, IL-4 and IL-5, and enhancing Th1 marker T-bet (Park et al., 2009). In guinea pigs, both orally and pulmonary supplied quercetin reduced both immediate and late phase airway resistance, and inflammatory cell infiltration and histamine levels similar to effects of dexamethason (Moon et al., 2008). To improve bioavailability, a quercetin containing oil-in-water microemulsion was prepared (Rogerio et al., 2010). This was equally effective as dexamethasone in reducing airway eosinophilia in mice with OVA-induced asthma, while quercetin suspended in 0.5% carboxymethylcellulose was ineffective. The quercetin loaded micro-emulsion also suppressed Th2 cytokines IL-4 and IL-5 in BALF and NFκB activation in pulmonary tissue comparable to dexamethasone (Rogerio et al., 2010). Luteolin and quercetin have a great effect on attenuating allergic asthma symptoms and their bioavailability can be increased by encapsulation, making these bioactive micronutrients interesting candidates for allergy modulation.
Resveratrol
Resveratrol (3,4,5-trihydroxy-trans-stilbene) is known for its strong anti-inflammatory potency. It is a ligand for the AhR receptor, which is known to favor the development of Tregs at the expense of inflammation-related Th17 effector cells development (Wang et al., 2013). Additionally, it can act as a Sirtin 1 agonist, enabling the deacetylation of transcription factors and lowering pro-inflammatory T-cell responses, amongst others NFκB-mediated inflammation (Malaguarnera, 2019; Delmas et al., 2020).
Resveratrol and Dendritic Cells and T-Cells
Resveratrol loaded nanostructured lipid carriers or free resveratrol suppressed NFκB activation in TNF-α activated moDCs (Barbosa et al., 2016). This effect was confirmed in another study showing impaired nuclear NFκB translocation, lowered co-stimulatory molecule expression and reduced DC induced allogenic T-cell proliferation (Silva et al., 2014). Similar results were obtained upon moDCs maturation with glycated albumin as a model for unwanted DC activation by advanced glycemic end products (Buttari et al., 2013). Resveratrol blocked DC activation and consequently T-cell proliferation and cytokine release, while largely preventing NFκB and MAPK, p38 and ERK activation (Buttari et al., 2013). Resveratrol also reduced CD80 and MHCII expression upon LPS-induced maturation of BMDCs, while increasing phagocytotic capacity and lowering DC-induced allogenic T-cell proliferation (Kim et al., 2004a). Monocytes exposed to resveratrol during differentiation to moDCs resulted in development of tolerogenic DCs instructing IL-10 secretion by allogenic T-cells (Svajger et al., 2010). Both splenic murine T-cell and B-cell proliferation and inflammatory cytokine release were suppressed by resveratrol, which coincided with enhanced regulatory IL-10 secretion (Sharma et al., 2007). Also, in T-cell receptor activated CD4+ T-cells, resveratrol blocked proliferation and reduced IL-2 receptor CD25 expression. Furthermore, IFN-γ release was blocked via suppression of the AKT/mTOR and MAPK(ERK) pathway, and T-cell metabolism was suppressed by modifying regulator 2 deacetylase (Sirt2) and p53. However, lower concentrations of resveratrol reduced proliferation but enhanced metabolic activity and induced IFN-γ release (Craveiro et al., 2017). The inhibition of T-cell proliferation and cytokine secretion by resveratrol was associated with increased Sirt1 expression (T-cell tolerance maintenance factor) upon resveratrol exposure (Zou et al., 2013). A recent study showed the interference of resveratrol with the interaction between PDL-1 and PD-1, known to contribute to Treg generation (Verdura et al., 2020). In a murine model of high fat (45%) induced regulatory T-cell dysfunction, resveratrol (0.06%) was indeed capable of restoring Treg levels and Treg related transcription factors, however this was achieved via prevention of ROS generation and stabilization of mitochondria preventing Treg cell death (Wang et al., 2014).
Resveratrol and Mast Cells
The strong inhibitory effect on mast cell degranulation by resveratrol was shown in BMMCs ex-vivo. The release of LTE4 and PGD2 was already inhibited upon administrating 10 µM resveratrol while histamine release was decreased at a dose of 100 µM. Interestingly, luteolin had to be administered tenfold less to achieve comparable effects (Baolin et al., 2004). Moreover, inhibition of IgE-mediated histamine release by orally administered resveratrol prior challenge was shown in BALB/c mice sensitized with anti-dinitrophenyl (DNP)-IgE (Han et al., 2013). Inhibition of mediator release by resveratrol is potentially induced by activation of negative signalling pathways, including Sirt1, which deacetylates several transcription factors. Activation of Sirt1 in BMMCs sensitized with DNP-IgE resulted in reduced phosphorylation of all MAPK, PLCγ1 and IKK. The effect of resveratrol was mostly inverted in Sirt1 knockout mice. (Li et al., 2017). Reduced phosphorylation of MAPK and PLCγ was also observed in human-derived skin mast cells, RBL-2H3 and HMC-1 (Koo et al., 2006; Kang et al., 2009; Han et al., 2015; Shirley et al., 2016). Overall, resveratrol can reduce mast cell degranulation by interfering with all three main pathways involved in cytokine/chemokine production, regulation of Ca2+ influx and mediator generation and release (Shirley et al., 2016).
Resveratrol in Pre-Clinical Allergy Models and Human Intervention Studies
Resveratrol not only abrogated IgE-allergen-induced ß-hexosaminidase and histamine release in RBL-2H3 and BMMCs, but also prevented OVA-induced diarrhea, temperature drop and anaphylactic symptom scores in OVA sensitized mice (Zhang et al., 2019). OVA-specific IgE levels were low, although IgG1 and IgG2a levels remained high. Serum histamine and murine mucosal mast cell protease 1 (mMCP-1) levels were significantly reduced, implying the potential of resveratrol to also lower mast cell degranulation in vivo in association with protection against food allergy symptoms (Zhang et al., 2019). These allergy protective effects were also shown in an OVA-induced food allergic model adding 0.01% resveratrol to the diet of mice (Okada et al., 2012). Resveratrol largely prevented sensitization since OVA-specific IgE was lowered for more than 50%, the challenge-induced drop in body temperature and the generation of allergen specific T-cell responses in both MLN and spleen was prevented (Okada et al., 2012). The resveratrol intervention inhibited DC-activation by lowering intracellular cAMP and expression of CD80 and CD86 on BMDCs (Okada et al., 2012). In a murine model of allergic asthma, resveratrol (daily via oral gavage, starting after the first sensitization) prevented OVA-induced pulmonary and systemic inflammation and type 2 responses (IL-5 and IL-13), while enhancing CD25+Foxp3+ Treg frequency and IL-10 miRNA expression in the lungs and downregulating miR34a expression (Alharris et al., 2018). Treatment of asthma via daily oral gavage with resveratrol reduced AHR and eosinophilia for 50% in association with lower type 2 cytokine levels in the BALF and reduced goblet cells and smooth muscle hyperplasia and collagen deposition as markers for tissue remodeling in the lung (Lee et al., 2017). Resveratrol inhibited pulmonary TGF-ß levels and mothers against decapentaplegic homolog 2 (SMAD2) activation, hence inhibiting a pathway known to contribute to airway remodeling (Lee et al., 2017). Although no randomized placebo controlled double blinded intervention studies in human have been done for the prevention of food allergy and asthma, resveratrol was tested in patients diagnosed with allergic rhinitis (n=151). Effects of a nose spray containing 0.1% resveratrol (0.6 µg/nostril/day) were compared with budesonide (400 µg/spray) or placebo for 1 month (Lv et al., 2018). After 2 and 4 weeks of treatment the nasal symptom score was reduced equally effective as the standard corticosteroid budesonide treatment. Hence, this study indicates the efficacy of resveratrol in suppressing allergic symptoms upon topical application in allergic rhinitis patients.
Lycopene
Beyond flavonoids, other groups of fat-soluble phytochemicals like non-pro-vitamin A carotenoid lycopene and pro-vitamin A carotenoid ß-carotene also have immunomodulatory abilities, being the most abundant carotenoids in human nutrition (Rinaldi de Alvarenga et al., 2019). Among other carotenoids, ß-carotene can be converted into retinal, retinol (vitamin A) and bioactive all trans retinoic acid via beta-carotene oxygenase 1 or RALDH. Conversion of lycopene, the red pigment of tomatoes, is mainly done via ß-carotene oxygenase 2; it is less clear if it also effectively can be converted into VitA. Carotenoids, including lycopene are known ligands for RAR, RXR, and PPAR and may modify NFκB-induced inflammatory pathways via this way (Ruhl, 2013). The exact contribution of pro-vitamin A and non-pro-vitamin A carotenoids in relation to allergy risk remains to be revealed. Although the total amount of ß-carotene and lycopene were comparable between healthy volunteers and AD patients, levels of retinol (VitA) and all trans retinoic acid as well as lycopene metabolites lutein and zeaxanthin, were significantly reduced in plasma of AD patients (Lucas et al., 2018). Asthma patients with AHR had reduced ß-carotene and tocopherol blood levels (Wood and Gibson, 2010), while in stable asthma patients serum lycopene concentrations were reduced (Riccioni et al., 2006). The latter studies indicate the relevance of not only studying ß-carotene or VitA, but also lycopene in the context of allergic diseases. Lycopene is known as an anti-oxidant, but also has anti-inflammatory capacities. It can suppress pro-inflammatory signaling cascades like the MAPK and NFκB and reduce inflammation in humans (Kim et al., 2004b; Colman-Martinez et al., 2017).
Lycopene and DCs and T-Cells
In BMDCs, lycopene lowered LPS-induced maturation resulting in reduced CD80, CD86 and MHCII expression, increased phagocytotic capacity, and reduced DC-induced allogenic T-cell proliferation and cytokine release (Kim et al., 2004b). These results were obtained not only upon in-vitro exposure of BMDCs to lycopene, but also in splenic DCs obtained after in-vivo lycopene exposure and LPS challenge. Also, mitogen-induced activation of PBMCs isolated from healthy adults was dampened, and lycopene enriched liposomes lowered lymphocyte activation, proliferation, and cytokine release (Mills et al., 2012).
Lycopene and Mast Cells
The effect of lycopene on mast cell degranulation was studied by administrating lycopene, amongst other carotenoids, to RBL-2H3 cells prior stimulation. Overall, RBL-2H3 cells showed a 50% reduced ß-hexosaminidase release upon 4 h pre-incubation with 10 µM lycopene. Reduction by other carotenoids ranged from 75% (Fucoxanthin) to less than 10% (Lutein), indicating a moderate ability of lycopene to reduce degranulation in RBL-2H3 cells (Manabe et al., 2014). Feeding HR-1 hairless mice with lycopene prevented low mineral diet AD development in association with reduced number of skin mast cells (Hiragun et al., 2016). Overall, lycopene seems to have similar effects on mast cells like VitA reducing mast cell numbers by inhibition of mast cell maturation via binding to the RAR.
Lycopene in Pre-Clinical Allergy Models and Human Intervention Studies
Pre-clinical studies investigating ß-carotenoids or lycopene are mainly focused on AD and asthma development. In HR-1 hairless mice, ß-carotenoid and lycopene both reduced skin infiltration of inflammatory cells, partially restored the moisture level in the skin, but only lycopene lowered trans-epidermal water loss and mast cell counts (Hiragun et al., 2016). This indicates a protective role for carotenoids in the development of skin inflammation and barrier defects. In a murine model for OVA-induced asthma, i.p. lycopene injections of sensitized mice for 3 days before OVA challenge lowered AHR and eosinophilia for 50%, while normalizing IL-4 levels in the BALF and increasing IFN-γ, indicating a shift in T-cell response (Lee et al., 2008). Dietary supplementation for 14 days prior to and during OVA sensitization and challenge with lycopene (Lyc-O-Mato) partially prevented airway eosinophilia in mice, while OVA-specific IgG1 levels as marker for sensitization were not affected (Hazlewood et al., 2011). However, prophylactic lycopene treatment was unable to ameliorate AHR responses (Hazlewood et al., 2011). In humans, dietary supplementation of asthmatic adults with high vegetable/fruit intake (>7 servings of vegetables/fruits/day) slightly improved lung function, significantly reduced sputum eosinophil counts and increased the time to exacerbation in association with increased serum carotenoids, however, lycopene supplementation alone was not protective (Wood et al., 2012). Daily supplementation of 30 mg/mL lycopene (Lyc-o-Mato capsules for one week), however, was capable of protecting against an exercise-induced drop in airway function (forced expiratory volume in 1 s, FEV1) for more than 10% in almost half of the patients (n=21), while in the non-supplemented group only one fifth had a lower than 15% drop in FEV1. Supplementation resulted in a doubling of the serum lycopene levels (Neuman et al., 2000). The authors suggest lycopene to act as an anti-oxidant scavenging ROS that is released upon exercise and can provoke AHR in allergic asthma. Another intervention study showed reduced inflammation markers and enhanced total antioxidant capacity when 250 mg n-3 LCPUFAs were added to a lycopene rich tomato juice intervention (Garcia-Alonso et al., 2012).
Combining n-3 LCPUFAs With Bioactive Micronutrients in Allergic Disease
Beyond n-3 LCPUFAs, fat-soluble vitamins, polyphenols and carotenoids have been studied for their allergy protective effects in-vitro and in pre-clinical models. The interaction between n-3 LCPUFAs and bio-active fat-soluble nutrients have been investigated in a few studies, showing their combinatorial value. For example when combined with n-3 LCPUFAs, resveratrol enhanced the anti-inflammatory action of DHA, and synergized with EPA in reducing LPS activation of macrophages. Furthermore, EPA plus resveratrol downregulated the expression of multiple inflammatory and oxidative stress related genes, all indicating the combination of several components can make the difference (Pallares et al., 2012; Kuhn et al., 2018). These studies are promising but were not topic of clinical trials. In this regard only few studies have addressed a combination approach. In an open label study, a combination of n-3 and n-6 PUFA, VitE, VitC, zinc as well as multivitamin capsules for 4 months was found to reduce AD symptoms of adult severe AD patients with 50% (Eriksen and Kåre, 2006). However, a study using i.v. infusion with n-3 and n-6 PUFA containing lipid emulsions obtained a similar result, so the added value of combining the PUFA with the micronutrients remains to be revealed (Mayser et al., 2002). Combining VitD with a high dose of EPA/DHA (6 g daily) for 3 weeks did not improve lung function in exercise-induced asthma, while in a previous study intervention with only n-3 LCPUFAs was effective (Mickleborough et al., 2003; Price et al., 2015). Hence, in this patient group, the added value of VitD was not shown, and another strategy may be considered. Combining these type of bioactive nutrients for optimized efficacy in allergy prevention or attenuation of allergic symptoms is not often studied and requires additional studies.
Discussion/Conclusion
Hence dietary intervention studies, aiming to determine effects of specific components on allergy prevention and/or amelioration of symptoms, mainly focus on a single active ingredient or food group per trial. n-3 LCPUFAs for example may be able to reduce the risk of atopic disease, albeit only in high doses starting already during early pregnancy (Dunstan et al., 2003a; Furuhjelm et al., 2009; Best et al., 2018). This approach may also help to prevent childhood asthma development and is especially indicated for those having low serum n-3 LCPUFAs levels due to low dietary intake or a specific FADS genotype (Tanaka et al., 2009). However, many other dietary components like fat-soluble nutrients possess anti-inflammatory activity targeting multiple cellular targets, potentially acting in an additive or synergistical manner to n-3 LCPUFAs which may help to optimize their full potential and gain efficacy in allergy prevention or treatment (Figure 2).
Despite their acknowledged anti-oxidant and anti-inflammatory capacities, therapeutic use of natural/biological polyphenols is thought to be limited due to their low bioavailability. Although the food matrix and oil composition can provide improved bioavailability, pharmaceutical concepts are under development to enforce their therapeutic value. Nanoparticle developments for enhanced gastrointestinal delivery and uptake or skin application is ongoing and promising, including diverse types of lipid-rich nanoparticles like liposomes or nanoparticles with additional functional groups as the recently developed quercetin loaded fucoidan/chitosan nanoparticles (Barbosa et al., 2019). Solid lipid nanoparticles containing n-3 LCPUFAs and esterified resveratrol are also being generated for pharmaceutical purposes (Serini et al., 2018). This allows combined delivery and solid lipid nanoparticles containing DHA and resveratrol indeed effectively abrogated the chemically induced inflammatory response in keratinocytes (Serini et al., 2019). Furthermore, unique nanoparticles (e.g. specific liposomes) loaded with antigen and quercetin achieved antigen specific immune silencing (NFκB inhibition similar to chemical inhibitor Bay) (Capini et al., 2009), showing the immunomodulatory and anti-inflammatory potency of these bioactive micronutrients in an antigen-specific manner. This is also exemplified by topical application of quercetin or resveratrol in murine models, being able to block skin inflammation in a contact hypersensitivity model (Carbone et al., 2018).
Overall, Mediterranean diet derived components like n-3 LCPUFAs, fat-soluble vitamins, polyphenols and carotenoids including lycopene are known for their anti-inflammatory and/or anti-allergic capacities with diverse underlying mechanisms, indicating their potencies to improve allergy protection by combining these elements. This hypothesis needs further investigation to reveal their capacity in fighting the progression of NCDs and especially the rising incidence of allergic disorders in industrialized countries.
Author Contributions
AH, AE, and LW have written the review. JG has critically read the review. All authors contributed to the article and approved the submitted version.
Conflict of Interest
AE is employed at the University Medical Centre Utrecht. JG is head of the Division of Pharmacology, Utrecht Institute for Pharmaceutical Sciences, Faculty of Science at Utrecht University and partly employed by Danone Nutricia Research B.V. AH and LW are employed at the Division of Pharmacology of the Utrecht University that collaborates within a strategic alliance with Danone Nutricia Research B.V.
References
Abdala-Valencia, H., Berdnikovs, S., Soveg, F. W., Cook-Mills, J. M. (2014). alpha-Tocopherol supplementation of allergic female mice inhibits development of CD11c+CD11b+ dendritic cells in utero and allergic inflammation in neonates. Am. J. Physiol. Lung Cell Mol. Physiol. 307 (6), L482–L496. doi: 10.1152/ajplung.00132.2014
Abdala-Valencia, H., Soveg, F., Cook-Mills, J. M. (2016). gamma-Tocopherol supplementation of allergic female mice augments development of CD11c+CD11b+ dendritic cells in utero and allergic inflammation in neonates. Am. J. Physiol. Lung Cell Mol. Physiol. 310 (8), L759–L771. doi: 10.1152/ajplung.00301.2015
Adams, S., Lopata, A., Smuts, C., Baatjies, R., Jeebhay, M. (2018). Relationship between Serum Omega-3 Fatty Acid and Asthma Endpoints. Int. J. Environ. Res. Public Health 16 (1), 43. doi: 10.3390/ijerph16010043
Agache, I., Annesi-Maesano, I., Bonertz, A., Branca, F., Cant, A., Fras, Z., et al. (2019). Prioritizing research challenges and funding for allergy and asthma and the need for translational research-The European Strategic Forum on Allergic Diseases. Allergy 74 (11), 2064–2076. doi: 10.1111/all.13856
Agrawal, T., Gupta, G. K., Agrawal, D. K. (2013). Vitamin D supplementation reduces airway hyperresponsiveness and allergic airway inflammation in a murine model. Clin. Exp. Allergy 43 (6), 672–683. doi: 10.1111/cea.12102
Alexandrakis, M., Kyriakou, D., Seretakis, D., Boucher, W., Letourneau, R., Kempuraj, D., et al. (2003). Inhibitory Effect of Retinoic Acid on Proliferation, Maturation and Tryptase Level in Human Leukemic Mast Cells (HMC-1). Int. J. Immunopath. Pharm. 16 (1), 43–47. doi: 10.1177/039463200301600106
Alharris, E., Alghetaa, H., Seth, R., Chatterjee, S., Singh, N. P., Nagarkatti, M., et al. (2018). Resveratrol Attenuates Allergic Asthma and Associated Inflammation in the Lungs Through Regulation of miRNA-34a That Targets FoxP3 in Mice. Front. Immunol. 9, 2992. doi: 10.3389/fimmu.2018.02992
Allen, S., Britton, J. R., Leonardi-Bee, J. A. (2009). Association between antioxidant vitamins and asthma outcome measures: systematic review and meta-analysis. Thorax 64 (7), 610–619. doi: 10.1136/thx.2008.101469
Allen, K. J., Koplin, J. J., Ponsonby, A. L., Gurrin, L. C., Wake, M., Vuillermin, P., et al. (2013). Vitamin D insufficiency is associated with challenge-proven food allergy in infants. J. Allergy Clin. Immunol. 131 (4), 1109–1116, 1116.e1101-1106. doi: 10.1016/j.jaci.2013.01.017
Alroy, I., Towers, T. L., Freedman, L. P. (1995). Transcriptional repression of the interleukin-2 gene by vitamin D3: direct inhibition of NFATp/AP-1 complex formation by a nuclear hormone receptor. Mol. Cell Biol. 15 (10), 5789–5799. doi: 10.1128/mcb.15.10.5789
Aludatt, M., Rababah, T., Alhamad, M., Gammoh, S., Ereifej, K., Al-Mahasneh, M. (2017). “Application of Olive Oil as Nutraceutical and Pharmaceutical Food: Composition and Biofunctional Constituents and Their Roles in Functionality, Therapeutic, and Nutraceutical Properties,” in Soft Chemistry and Food Fermentation. Eds. Grumezescu, A., Holban, A. (London, United Kingdom: Elsevier), 265–298.
Arranz, S., Martinez-Huelamo, M., Vallverdu-Queralt, A., Valderas-Martinez, P., Illan, M., Sacanella, E., et al. (2015). Influence of olive oil on carotenoid absorption from tomato juice and effects on postprandial lipemia. Food Chem. 168, 203–210. doi: 10.1016/j.foodchem.2014.07.053
Astorquiza, M., Helle, B., Vergara, R. (1980). Effect of vitamin A on the in vitro degranulation of mouse mast cells. Allergol. Immunopathol. 8 (2), 87–90.
Babina, M., Krautheim, M., Grützkau, A., Henz, B. (2000). Human Leukemic (HMC-1) Mast Cells Are Responsive to 1α,25-Dihydroxyvitamin D3: Selective Promotion of ICAM-3 Expression and Constitutive Presence of Vitamin D3 Receptor. Biochem. Biophys. Res. Com. 273 (3), 1104–1110. doi: 10.1006/bbrc.2000.3083
Babina, M., Guhl, S., Motakis, E., Artuc, M., Hazzan, T., Worm, M., et al. (2015). Retinoic acid potentiates inflammatory cytokines in human mast cells: identification of mast cells as prominent constituents of the skin retinoid network. Mol. Cell Endocrinol. 406, 49–59. doi: 10.1016/j.mce.2015.02.019
Babina, M., Artuc, M., Guhl, S., Zuberbier, T. (2017). Retinoic Acid Negatively Impacts Proliferation and MCTC Specific Attributes of Human Skin Derived Mast Cells, but Reinforces Allergic Stimulability. Int. J. Mol. Sci. 18 (3), 525. doi: 10.3390/ijms18030525
Baek, J. H., Shin, Y. H., Chung, I. H., Kim, H. J., Yoo, E. G., Yoon, J. W., et al. (2014). The link between serum vitamin D level, sensitization to food allergens, and the severity of atopic dermatitis in infancy. J. Pediatr. 165 (4), 849–854.e841. doi: 10.1016/j.jpeds.2014.06.058
Baeke, F., Takiishi, T., Korf, H., Gysemans, C., Mathieu, C. (2010). Vitamin D: modulator of the immune system. Curr. Opin. Pharmacol. 10 (4), 482–496. doi: 10.1016/j.coph.2010.04.001
Baolin, L., Inami, Y., Tanaka, H., Inagaki, N., Iinuma, M., Nagai, H. (2004). Resveratrol inhibits the release of mediators from bone marrow-derived mouse mast cells in vitro. Planta Med. 70 (4), 305–309. doi: 10.1055/s-2004-818940
Barbaresko, J., Koch, M., Schulze, M., Nöthlings, U. (2013). Dietary pattern analysis and biomarkers of low-grade inflammation: a systematic literature review. Nutr. Rev. 71 (8), 511–527. doi: 10.1111/nure.12035
Barbosa, J. P., Neves, A. R., Silva, A. M., Barbosa, M. A., Reis, M. S., Santos, S. G. (2016). Nanostructured lipid carriers loaded with resveratrol modulate human dendritic cells. Int. J. Nanomed. 11, 3501–3516. doi: 10.2147/IJN.S108694
Barbosa, A. I., Costa Lima, S. A., Reis, S. (2019). Application of pH-Responsive Fucoidan/Chitosan Nanoparticles to Improve Oral Quercetin Delivery. Molecules 24 (2), 346. doi: 10.3390/molecules24020346
Barden, A., Mas, E., Mori, T. (2016). n-3 Fatty acid supplementation and pro-resolving mediators of inflammation. Curr. Opin. Lipidol. 27 (1), 26–32. doi: 10.1097/MOL.0000000000000262
Bargut, T. C., Ferreira, T. P., Daleprane, J. B., Martins, M. A., Silva, P. M., Aguila, M. B. (2013). Fish oil has beneficial effects on allergen-induced airway inflammation and hyperreactivity in mice. PloS One 8 (9), e75059. doi: 10.1371/journal.pone.0075059
Baroni, E., Biffi, M., Benigni, F., Monna, A., Carlucci, D., Carmeliet, G., et al. (2007). VDR-dependent regulation of mast cell maturation mediated by 1,25-dihydroxyvitamin D3. J. Leuk Biol. 81 (1), 250–262. doi: 10.1189/jlb.0506322
Barrat, F. J., Cua, D. J., Boonstra, A., Richards, D. F., Crain, C., Savelkoul, H. F., et al. (2002). In vitro generation of interleukin 10-producing regulatory CD4(+) T cells is induced by immunosuppressive drugs and inhibited by T helper type 1 (Th1)- and Th2-inducing cytokines. J. Exp. Med. 195 (5), 603–616. doi: 10.1084/jem.20011629
Berdnikovs, S., Abdala-Valencia, H., McCary, C., Somand, M., Cole, R., Garcia, A., et al. (2009). Isoforms of vitamin E have opposing immunoregulatory functions during inflammation by regulating leukocyte recruitment. J. Immunol. 182 (7), 4395–4405. doi: 10.4049/jimmunol.0803659
Best, K., Sullivan, R., Palmer, D., Gold, M., Martin, J., Kennedy, D., et al. (2018). Prenatal omega-3 LCPUFA and symptoms of allergic disease and sensitization throughout early childhood - a longitudinal analysis of long-term follow-up of a randomized controlled trial. World Allergy Organ J. 11 (1), 10. doi: 10.1186/s40413-018-0190-7
Biagi, C., Nunzio, M. D., Bordoni, A., Gori, D., Lanari, M. (2019). Effect of Adherence to Mediterranean Diet during Pregnancy on Children’s Health: A Systematic Review. Nutrients 11 (5), 997. doi: 10.3390/nu11050997
Bilal, S., Haworth, O., Wu, L., Weylandt, K. H., Levy, B. D., Kang, J. X. (2011). Fat-1 transgenic mice with elevated omega-3 fatty acids are protected from allergic airway responses. Biochim. Biophys. Acta 1812 (9), 1164–1169. doi: 10.1016/j.bbadis.2011.05.002
Bisgaard, H., Stokholm, J., Chawes, B. L., Vissing, N. H., Bjarnadottir, E., Schoos, A. M., et al. (2016). Fish Oil-Derived Fatty Acids in Pregnancy and Wheeze and Asthma in Offspring. N. Engl. J. Med. 375, 2530–2539. doi: 10.1056/NEJMoa1503734
Blomhoff, R., Blomhoff, H. K. (2006). Overview of retinoid metabolism and function. J. Neurobiol. 66 (7), 606–630. doi: 10.1002/neu.20242
Boileau, A. C., Merchen, N. R., Wasson, K., Atkinson, C. A., Erdman, J. W., Jr. (1999). Cis-lycopene is more bioavailable than trans-lycopene in vitro and in vivo in lymph-cannulated ferrets. J. Nutr. 129 (6), 1176–1181. doi: 10.1093/jn/129.6.1176
Boonstra, A., Barrat, F. J., Crain, C., Heath, V. L., Savelkoul, H. F., O’Garra, A. (2001). 1alpha,25-Dihydroxyvitamin d3 has a direct effect on naive CD4(+) T cells to enhance the development of Th2 cells. J. Immunol. 167 (9), 4974–4980. doi: 10.4049/jimmunol.167.9.4974
Borbulevych, O. Y., Jankun, J., Selman, S. H., Skrzypczak-Jankun, E. (2004). Lipoxygenase interactions with natural flavonoid, quercetin, reveal a complex with protocatechuic acid in its X-ray structure at 2.1 A resolution. Proteins 54 (1), 13–19. doi: 10.1002/prot.10579
Burns, J., Yokota, T., Ashihara, H., Lean, M., Crozier, A. (2002). Plant Foods and Herbal Sources of Resveratrol. J. Agric. Food Chem. 50 (11), 3337–3340. doi: 10.1021/jf0112973
Buttari, B., Profumo, E., Facchiano, F., Ozturk, E. I., Segoni, L., Saso, L., et al. (2013). Resveratrol prevents dendritic cell maturation in response to advanced glycation end products. Oxid. Med. Cell Longev. 2013, 574029. doi: 10.1155/2013/574029
Caddeo, C., Gabriele, M., Fernandez-Busquets, X., Valenti, D., Fadda, A. M., Pucci, L., et al. (2019). Antioxidant activity of quercetin in Eudragit-coated liposomes for intestinal delivery. Int. J. Pharm. 565, 64–69. doi: 10.1016/j.ijpharm.2019.05.007
Calder, P. (2015). Marine omega-3 fatty acids and inflammatory processes: Effects, mechanisms and clinical relevance. Biochim. Biophys. Acta 1851 (4), 469–484. doi: 10.1016/j.bbalip.2014.08.010
Capini, C., Jaturanpinyo, M., Chang, H. I., Mutalik, S., McNally, A., Street, S., et al. (2009). Antigen-specific suppression of inflammatory arthritis using liposomes. J. Immunol. 182 (6), 3556–3565. doi: 10.4049/jimmunol.0802972
Carbone, M. L., Lulli, D., Passarelli, F., Pastore, S. (2018). Topical Plant Polyphenols Prevent Type I Interferon Signaling in the Skin and Suppress Contact Hypersensitivity. Int. J. Mol. Sci. 19 (9), 2652. doi: 10.3390/ijms19092652
Carlsson, J. A., Wold, A. E., Sandberg, A. S., Östman, S. M. (2015). The Polyunsaturated Fatty Acids Arachidonic Acid and Docosahexaenoic Acid Induce Mouse Dendritic Cells Maturation but Reduce T-Cell Responses In Vitro. PloS One 10 (11), e0143741. doi: 10.1371/journal.pone.0143741
Cavalcanti, E., Vadrucci, E., Delvecchio, F. R., Addabbo, F., Bettini, S., Liou, R., et al. (2014). Administration of reconstituted polyphenol oil bodies efficiently suppresses dendritic cell inflammatory pathways and acute intestinal inflammation. PloS One 9 (2), e88898. doi: 10.1371/journal.pone.0088898
Chambon, P. (1996). A decade of molecular biology of retinoic acid receptors. FASEB J. 10 (9), 940–954. doi: 10.1096/fasebj.10.9.8801176
Chawes, B. L., Bonnelykke, K., Stokholm, J., Vissing, N. H., Bjarnadottir, E., Schoos, A. M., et al. (2016). Effect of Vitamin D3 Supplementation During Pregnancy on Risk of Persistent Wheeze in the Offspring: A Randomized Clinical Trial. Jama 315 (4), 353–361. doi: 10.1001/jama.2015.18318
Checker, R., Sharma, D., Sandur, S. K., Khan, N. M., Patwardhan, R. S., Kohli, V., et al. (2011). Vitamin K3 suppressed inflammatory and immune responses in a redox-dependent manner. Free Radic. Res. 45 (8), 975–985. doi: 10.3109/10715762.2011.585647
Checkley, W., West, K. P., Jr., Wise, R. A., Wu, L., LeClerq, S. C., Khatry, S., et al. (2011). Supplementation with vitamin A early in life and subsequent risk of asthma. Eur. Respir. J. 38 (6), 1310–1319. doi: 10.1183/09031936.00006911
Chen, S., Sims, G. P., Chen, X. X., Gu, Y. Y., Chen, S., Lipsky, P. E. (2007). Modulatory effects of 1,25-dihydroxyvitamin D3 on human B cell differentiation. J. Immunol. 179 (3), 1634–1647. doi: 10.4049/jimmunol.179.3.1634
Chen, F., Marquez, H., Kim, Y. K., Qian, J., Shao, F., Fine, A., et al. (2014). Prenatal retinoid deficiency leads to airway hyperresponsiveness in adult mice. J. Clin. Invest. 124 (2), 801–811. doi: 10.1172/jci70291
Chiu, C. Y., Yao, T. C., Chen, S. H., Tsai, M. H., Tu, Y. L., Hua, M. C., et al. (2014). Low cord blood vitamin D levels are associated with increased milk sensitization in early childhood. Pediatr. Allergy Immunol. 25 (8), 767–772. doi: 10.1111/pai.12304
Colman-Martinez, M., Martinez-Huelamo, M., Valderas-Martinez, P., Arranz-Martinez, S., Almanza-Aguilera, E., Corella, D., et al. (2017). trans-Lycopene from tomato juice attenuates inflammatory biomarkers in human plasma samples: An intervention trial. Mol. Nutr. Food Res. 61 (11). doi: 10.1002/mnfr.201600993
Conly, J., Stein, K. (1992). The Production of Menaquinones (Vitamin K2) by Intestinal Bacteria and Their Role in Maintaining Coagulation Homeostasis. Prog. Food Nutr. Sci. 16 (4), 307–343.
Cook-Mills, J. M., Abdala-Valencia, H., Hartert, T. (2013). Two faces of vitamin E in the lung. Am. J. Respir. Crit. Care Med. 188 (3), 279–284. doi: 10.1164/rccm.201303-0503ED
Cook-Mills, J. M. (2013). Isoforms of Vitamin E Differentially Regulate PKC alpha and Inflammation: A Review. J. Clin. Cell Immunol. 4 (137), 1000137. doi: 10.4172/2155-9899.1000137
Coombes, J. L., Siddiqui, K. R., Arancibia-Carcamo, C. V., Hall, J., Sun, C. M., Belkaid, Y., et al. (2007). A functionally specialized population of mucosal CD103+ DCs induces Foxp3+ regulatory T cells via a TGF-beta and retinoic acid-dependent mechanism. J. Exp. Med. 204 (8), 1757–1764. doi: 10.1084/jem.20070590
Coquerelle, C., Moser, M. (2010). DC subsets in positive and negative regulation of immunity. Immunol. Rev. 234 (1), 317–334. doi: 10.1111/j.0105-2896.2009.00887.x
Craveiro, M., Cretenet, G., Mongellaz, C., Matias, M. I., Caron, O., de Lima, M. C. P., et al. (2017). Resveratrol stimulates the metabolic reprogramming of human CD4(+) T cells to enhance effector function. Sci. Signal 10 (501), eaal3024. doi: 10.1126/scisignal.aal3024
Cruz Bojórquez, R., González Gallego, J., Sánchez Collado, P. (2013). Functional Properties and Health Benefits of Lycopene. Nutr. Hosp. 28 (1), 6–15. doi: 10.3305/nh.2013.28.1.6302
Cruz, E., Reuter, S., Martin, H., Dehzad, N., Muzitano, M., Costa, S., et al. (2012). Kalanchoe pinnata inhibits mast cell activation and prevents allergic airway disease. Phytomedicine 19 (2), 115–121. doi: 10.1016/j.phymed.2011.06.030
Cui, W., Zhang, P., Gu, J., Tian, Y., Gao, X., Liu, Y., et al. (2016). Vitamin A Deficiency Promotes Inflammation by Induction of Type 2 Cytokines in Experimental Ovalbumin-Induced Asthma Murine Model. Inflammation 39 (5), 1798–1804. doi: 10.1007/s10753-016-0415-2
Dabeek, W., Marra, M. (2019). Dietary Quercetin and Kaempferol: Bioavailability and Potential Cardiovascular-Related Bioactivity in Humans. Nutrients 11 (10), 2288. doi: 10.3390/nu11102288
Daniel, C., Sartory, N. A., Zahn, N., Radeke, H. H., Stein, J. M. (2008). Immune modulatory treatment of trinitrobenzene sulfonic acid colitis with calcitriol is associated with a change of a T helper (Th) 1/Th17 to a Th2 and regulatory T cell profile. J. Pharmacol. Exp. Ther. 324 (1), 23–33. doi: 10.1124/jpet.107.127209
de Matos, O. G., Amaral, S. S., Pereira da Silva, P. E., Perez, D. A., Alvarenga, D. M., Ferreira, A. V., et al. (2012). Dietary supplementation with omega-3-PUFA-rich fish oil reduces signs of food allergy in ovalbumin-sensitized mice. Clin. Dev. Immunol. 2012, 236564. doi: 10.1155/2012/236564
De Santis, S., Kunde, D., Serino, G., Galleggiante, V., Caruso, M. L., Mastronardi, M., et al. (2016). Secretory leukoprotease inhibitor is required for efficient quercetin-mediated suppression of TNFalpha secretion. Oncotarget 7 (46), 75800–75809. doi: 10.18632/oncotarget.12415
Delmas, D., Limagne, E., Ghiringhelli, F., Aires, V. (2020). Immune Th17 lymphocytes play a critical role in the multiple beneficial properties of resveratrol. Food Chem. Toxicol. 137, 111091. doi: 10.1016/j.fct.2019.111091
DeLuca, H. F. (2004). Overview of general physiologic features and functions of vitamin D. Am. J. Clin. Nutr. 80 (6 Suppl), 1689S–1696S. doi: 10.1093/ajcn/80.6.1689S
Delvecchio, F. R., Vadrucci, E., Cavalcanti, E., De Santis, S., Kunde, D., Vacca, M., et al. (2015). Polyphenol administration impairs T-cell proliferation by imprinting a distinct dendritic cell maturational profile. Eur. J. Immunol. 45 (9), 2638–2649. doi: 10.1002/eji.201545679
Ding, Y., Li, C., Zhang, Y., Ma, P., Zhao, T., Che, D., et al. (2020). Quercetin as a Lyn kinase inhibitor inhibits IgE-mediated allergic conjunctivitis. Food Chem. Tox. 135, 110924. doi: 10.1016/j.fct.2019.110924
DiNicolantonio, J., Bhutani, J., O’Keefe, J. (2015). The health benefits of vitamin K. Open Heart 2 (1), e000300. doi: 10.1136/openhrt-2015-000300
dos Santos, L., Fleming, I. (2020). Role of cytochrome P450-derived, polyunsaturated fatty acid mediators in diabetes and the metabolic syndrome. Prostaglandins Other Lipid Mediat. 148, 106407. doi: 10.1016/j.prostaglandins.2019.106407
Dow, L., Tracey, M., Villar, A., Coggon, D., Margetts, B. M., Campbell, M. J., et al. (1996). Does dietary intake of vitamins C and E influence lung function in older people? Am. J. Respir. Crit. Care Med. 154 (5), 1401–1404. doi: 10.1164/ajrccm.154.5.8912755
D’Ambrosio, D., Cippitelli, M., Cocciolo, M. G., Mazzeo, D., Di Lucia, P., Lang, R., et al. (1998). Inhibition of IL-12 production by 1,25-dihydroxyvitamin D3. Involvement of NF-kappaB downregulation in transcriptional repression of the p40 gene. J. Clin. Invest. 101 (1), 252–262. doi: 10.1172/jci1050
Draper, E., Reynolds, C. M., Canavan, M., Mills, K. H., Loscher, C. E., Roche, H. M. (2011). Omega-3 fatty acids attenuate dendritic cell function via NF-κB independent of PPARγ. J. Nutr. Biochem. 22 (8), 784–790. doi: 10.1016/j.jnutbio.2010.06.009
D’Vaz, N., Meldrum, S. J., Dunstan, J. A., Lee-Pullen, T. F., Metcalfe, J., Holt, B. J., et al. (2012a). Fish oil supplementation in early infancy modulates developing infant immune responses. Clin. Exp. Allergy 42 (8), 1206–1216. doi: 10.1111/j.1365-2222.2012.04031.x
D’Vaz, N., Meldrum, S. J., Dunstan, J. A., Martino, D. J., McCarthy, S., Metcalfe, J., et al. (2012b). Postnatal fish oil supplementation in high-risk infants to prevent allergy: randomized controlled trial. Pediatrics 130 (4), 674–682. doi: 10.1542/peds.2011-3104
Dudda, J. C., Lembo, A., Bachtanian, E., Huehn, J., Siewert, C., Hamann, A., et al. (2005). Dendritic cells govern induction and reprogramming of polarized tissue-selective homing receptor patterns of T cells: important roles for soluble factors and tissue microenvironments. Eur. J. Immunol. 35 (4), 1056–1065. doi: 10.1002/eji.200425817
Dufour, C., Loonis, M. (2007). Flavonoids and their oxidation products protect efficiently albumin-bound linoleic acid in a model of plasma oxidation. Biochim. Biophys. Acta 1770 (6), 958–965. doi: 10.1016/j.bbagen.2007.02.005
Dunstan, J. A., Mori, T. A., Barden, A., Beilin, L. J., Taylor, A. L., Holt, P. G., et al. (2003a). Maternal fish oil supplementation in pregnancy reduces interleukin-13 levels in cord blood of infants at high risk of atopy. Clin. Exp. Allergy 33 (4), 442–448. doi: 10.1046/j.1365-2222.2003.01590.x
Dunstan, J. A., Mori, T. A., Barden, A., Beilin, L. J., Taylor, A. L., Holt, P. G., et al. (2003b). Fish oil supplementation in pregnancy modifies neonatal allergen-specific immune responses and clinical outcomes in infants at high risk of atopy: a randomized, controlled trial. J. Allergy Clin. Immunol. 112, 1178–1184. doi: 10.1016/j.jaci.2003.09.009
Eriksen, B. B., Kåre, D. L. (2006). Open Trial of Supplements of Omega 3 and 6 Fatty Acids, Vitamins and Minerals in Atopic Dermatitis. J. Dermatolog. Treat 17 (2), 82–85. doi: 10.1080/09546630600621946
Farazuddin, M., Mishra, R., Jing, Y., Srivastava, V., Comstock, A. T., Sajjan, U. S. (2018). Quercetin prevents rhinovirus-induced progression of lung disease in mice with COPD phenotype. PloS One 13 (7), e0199612. doi: 10.1371/journal.pone.0199612
Fiorani, M., Guidarelli, A., Blasa, M., Azzolini, C., Candiracci, M., Piatti, E., et al. (2010). Mitochondria accumulate large amounts of quercetin: prevention of mitochondrial damage and release upon oxidation of the extramitochondrial fraction of the flavonoid. J. Nutr. Biochem. 21 (5), 397–404. doi: 10.1016/j.jnutbio.2009.01.014
Fleming, I. (2019). New Lipid Mediators in Retinal Angiogenesis and Retinopathy. Front. Pharmacol. 10, 739. doi: 10.3389/fphar.2019.00739
Foiles, A. M., Kerling, E. H., Wick, J. A., Scalabrin, D. M. F., Colombo, J., Carlson, S. E. (2016). Formula with long-chain polyunsaturated fatty acids reduces incidence of allergy in early childhood. Pediatr. Allergy Immunol. 27 (2), 156–161. doi: 10.1111/pai.12515
Fremont, L., Gozzelino, M. T., Franchi, M. P., Linard, A. (1998). Dietary flavonoids reduce lipid peroxidation in rats fed polyunsaturated or monounsaturated fat diets. J. Nutr. 128 (9), 1495–1502. doi: 10.1093/jn/128.9.1495
Fritsche, J., Mondal, K., Ehrnsperger, A., Andreesen, R., Kreutz, M. (2003). Regulation of 25-hydroxyvitamin D3-1 alpha-hydroxylase and production of 1 alpha,25-dihydroxyvitamin D3 by human dendritic cells. Blood 102 (9), 3314–3316. doi: 10.1182/blood-2002-11-3521
Furuhjelm, C., Warstedt, K., Larsson, J., Fredriksson, M., Böttcher, M. F., Fälth-Magnusson, K., et al. (2009). Fish oil supplementation in pregnancy and lactation may decrease the risk of infant allergy. Acta Paediatr. 98 (9), 1461–1467. doi: 10.1111/j.1651-2227.2009.01355.x
Furuhjelm, C., Warstedt, K., Fageras, M., Fälth-Magnusson, K., Larsson, J., Fredriksson, M., et al. (2011). Allergic disease in infants up to 2 years of age in relation to plasma omega-3 fatty acids and maternal fish oil supplementation in pregnancy and lactation. Pediatr. Allergy Immunol. 22 (5), 505–514. doi: 10.1111/j.1399-3038.2010.01096.x
Fussbroich, D., Zimmermann, K., Gopel, A., Eickmeier, O., Trischler, J., Zielen, S., et al. (2019). A specific combined long-chain polyunsaturated fatty acid supplementation reverses fatty acid profile alterations in a mouse model of chronic asthma. Lipids Health Dis. 18 (1), 16. doi: 10.1186/s12944-018-0947-6
Galleggiante, V., De Santis, S., Cavalcanti, E., Scarano, A., De Benedictis, M., Serino, G., et al. (2017). Dendritic Cells Modulate Iron Homeostasis and Inflammatory Abilities Following Quercetin Exposure. Curr. Pharm. Des. 23 (14), 2139–2146. doi: 10.2174/1381612823666170112125355
Garcia-Alonso, F. J., Jorge-Vidal, V., Ros, G., Periago, M. J. (2012). Effect of consumption of tomato juice enriched with n-3 polyunsaturated fatty acids on the lipid profile, antioxidant biomarker status, and cardiovascular disease risk in healthy women. Eur. J. Nutr. 51 (4), 415–424. doi: 10.1007/s00394-011-0225-0
Gebauer, S., Psota, T., Harris, W., Kris-Etherton, P. (2006). n-3 Fatty Acid Dietary Recommendations and Food Sources to Achieve Essentiality and Cardiovascular Benefits. Am. J. Clin. Nutr. 83 (6 Suppl), 1526S–1535S. doi: 10.1093/ajcn/83.6.1526S
Gorman, S., Kuritzky, L. A., Judge, M. A., Dixon, K. M., McGlade, J. P., Mason, R. S., et al. (2007). Topically applied 1,25-dihydroxyvitamin D3 enhances the suppressive activity of CD4+CD25+ cells in the draining lymph nodes. J. Immunol. 179 (9), 6273–6283. doi: 10.4049/jimmunol.179.9.6273
Gorman, S., Weeden, C. E., Tan, D. H., Scott, N. M., Hart, J., Foong, R. E., et al. (2013). Reversible control by vitamin D of granulocytes and bacteria in the lungs of mice: an ovalbumin-induced model of allergic airway disease. PloS One 8 (6), e67823. doi: 10.1371/journal.pone.0067823
Griffin, M. D., Lutz, W., Phan, V. A., Bachman, L. A., McKean, D. J., Kumar, R. (2001). Dendritic cell modulation by 1alpha,25 dihydroxyvitamin D3 and its analogs: a vitamin D receptor-dependent pathway that promotes a persistent state of immaturity in vitro and in vivo. Proc. Natl. Acad. Sci. U.S.A. 98 (12), 6800–6805. doi: 10.1073/pnas.121172198
Hamalainen, N., Nwaru, B. I., Erlund, I., Takkinen, H. M., Ahonen, S., Toppari, J., et al. (2017). Serum carotenoid and tocopherol concentrations and risk of asthma in childhood: a nested case-control study. Clin. Exp. Allergy 47 (3), 401–409. doi: 10.1111/cea.12904
Han, S. N., Wu, D., Ha, W. K., Beharka, A., Smith, D. E., Bender, B. S., et al. (2000). Vitamin E supplementation increases T helper 1 cytokine production in old mice infected with influenza virus. Immunology 100 (4), 487–493. doi: 10.1046/j.1365-2567.2000.00070.x
Han, S. N., Adolfsson, O., Lee, C. K., Prolla, T. A., Ordovas, J., Meydani, S. N. (2006). Age and vitamin E-induced changes in gene expression profiles of T cells. J. Immunol. 177 (9), 6052–6061. doi: 10.4049/jimmunol.177.9.6052
Han, S., Bae, J., Park, S., Kim, Y., Park, J., Kang, Y. (2013). Resveratrol inhibits IgE-mediated basophilic mast cell degranulation and passive cutaneous anaphylaxis in mice. J. Nutr. 143 (5), 632–639. doi: 10.3945/jn.112.173302
Han, S., Choi, Y., Kang, M., Park, J., Kang, Y. (2015). Resveratrol Suppresses Cytokine Production Linked to FcϵRI-MAPK Activation in IgE-Antigen Complex-Exposed Basophilic Mast Cells and Mice. Am. J. Chin. Med. 43 (8), 1605–1623. doi: 10.1142/S0192415X15500913
Hansen, S., Maslova, E., Strom, M., Linneberg, A., Halldorsson, T. I., Granstrom, C., et al. (2015). The long-term programming effect of maternal 25-hydroxyvitamin D in pregnancy on allergic airway disease and lung function in offspring after 20 to 25 years of follow-up. J. Allergy Clin. Immunol. 136 (1), 169–176.e162. doi: 10.1016/j.jaci.2014.12.1924
Hara, T., Hirasawa, A., Ichimura, A., Kimura, I., Tsujimoto, G. (2011). Free Fatty Acid Receptors FFAR1 and GPR120 as Novel Therapeutic Targets for Metabolic Disorders. J. Pharm. Sci. 100 (9), 3594–3601. doi: 10.1002/jps.22639
Hatanaka, H., Ishizawa, H., Nakamura, Y., Tadokoro, H., Tanaka, S., Onda, K., et al. (2014). Effects of vitamin K3 and K5 on proliferation, cytokine production, and regulatory T cell-frequency in human peripheral-blood mononuclear cells. Life Sci. 99 (1-2), 61–68. doi: 10.1016/j.lfs.2014.01.068
Haussler, M. R., Jurutka, P. W., Mizwicki, M., Norman, A. W. (2011). Vitamin D receptor (VDR)-mediated actions of 1alpha,25(OH)(2)vitamin D(3): genomic and non-genomic mechanisms. Best Pract. Res. Clin. Endocrinol. Metab. 25 (4), 543–559. doi: 10.1016/j.beem.2011.05.010
Hazlewood, L. C., Wood, L. G., Hansbro, P. M., Foster, P. S. (2011). Dietary lycopene supplementation suppresses Th2 responses and lung eosinophilia in a mouse model of allergic asthma. J. Nutr. Biochem. 22 (1), 95–100. doi: 10.1016/j.jnutbio.2009.12.003
Heine, G., Tabeling, C., Hartmann, B., Gonzalez Calera, C. R., Kuhl, A. A., Lindner, J., et al. (2014). 25-hydroxvitamin D3 promotes the long-term effect of specific immunotherapy in a murine allergy model. J. Immunol. 193 (3), 1017–1023. doi: 10.4049/jimmunol.1301656
Hiragun, M., Hiragun, T., Oseto, I., Uchida, K., Yanase, Y., Tanaka, A., et al. (2016). Oral administration of beta-carotene or lycopene prevents atopic dermatitis-like dermatitis in HR-1 mice. J. Dermatol. 43 (10), 1188–1192. doi: 10.1111/1346-8138.13350
Hirano, T., Higa, S., Arimitsu, J., Naka, T., Shima, Y., Ohshima, S., et al. (2004). Flavonoids such as luteolin, fisetin and apigenin are inhibitors of interleukin-4 and interleukin-13 production by activated human basophils. Int. Arch. Allergy Immunol. 134 (2), 135–140. doi: 10.1159/000078498
Hjertson, M., Dimberg, L., Nilsson, K., Nilsson, G., Kivinen, P., Harvima, I. (2003). Retinoic acid inhibits in vitro development of mast cells but has no marked effect on mature human skin tryptase- and chymase-positive mast cells. J. Invest. Dermatol. 120 (2), 239–245. doi: 10.1046/j.1523-1747.2003.12030.x
Hoag, K. A., Nashold, F. E., Goverman, J., Hayes, C. E. (2002). Retinoic acid enhances the T helper 2 cell development that is essential for robust antibody responses through its action on antigen-presenting cells. J. Nutr. 132 (12), 3736–3739. doi: 10.1093/jn/132.12.3736
Hodge, L., Salome, C. M., Hughes, J. M., Liu-Brennan, D., Rimmer, J., Allman, M., et al. (1998). Effect of dietary intake of omega-3 and omega-6 fatty acids on severity of asthma in children. Eur. Respir. J. 11, 361–365. doi: 10.1183/09031936.98.11020361
Hofer, T., Jorgensen, T. O., Olsen, R. L. (2014). Comparison of food antioxidants and iron chelators in two cellular free radical assays: strong protection by luteolin. J. Agric. Food Chem. 62 (33), 8402–8410. doi: 10.1021/jf5022779
Hogenkamp, A., van Vlies, N., Fear, A. L., van Esch, B. C., Hofman, G. A., Garssen, J., et al. (2011). Dietary fatty acids affect the immune system in male mice sensitized to ovalbumin or vaccinated with influenza. J. Nutr. 141 (4), 698–702. doi: 10.3945/jn.110.135863
Holick, M. F., Schnoes, H. K., DeLuca, H. F., Gray, R. W., Boyle, I. T., Suda, T. (1972). Isolation and identification of 24,25-dihydroxycholecalciferol, a metabolite of vitamin D made in the kidney. Biochemistry 11 (23), 4251–4255. doi: 10.1021/bi00773a009
Holick, M. F. (2007). Vitamin D deficiency. N. Engl. J. Med. 357 (3), 266–281. doi: 10.1056/NEJMra070553
Hollams, E. M., Teo, S. M., Kusel, M., Holt, B. J., Holt, K. E., Inouye, M., et al. (2017). Vitamin D over the first decade and susceptibility to childhood allergy and asthma. J. Allergy Clin. Immunol. 139 (2), 472–481.e479. doi: 10.1016/j.jaci.2016.07.032
Hong, X., Liang, L., Sun, Q., Keet, C., Tsai, H.-J., Ji, Y., et al. (2019). Maternal triacylglycerol signature and risk of food allergy in offspring. J. Allergy Clin. Immunol. 144 (3), 729–737. doi: 10.1016/j.jaci.2019.03.033
Hoskins, A., Roberts, J. L., Milne, G., Choi, L., Dworski, R. (2012). Natural-source d-α-tocopheryl acetate inhibits oxidant stress and modulates atopic asthma in humans in vivo. Allergy 67 (5), 676–682. doi: 10.1111/j.1398-9995.2012.02810.x
Hufnagl, K., Jensen-Jarolim, E. (2019). Does a carrot a day keep the allergy away? Immunol. Lett. 206, 54–58. doi: 10.1016/j.imlet.2018.10.009
Iciek, L., Delphin, S., Stavnezer, J. (1997). CD40 cross-linking induces Ig epsilon germline transcripts in B cells via activation of NF-kappaB: synergy with IL-4 induction. J. Immunol. 158 (10), 4769–4779.
Ishida, S., Kinoshita, T., Sugawara, N., Yamashita, K., Koike, K. (2003). Serum inhibitors for human mast cell growth: possible role of retinol. Allergy 58 (10), 1044–1052. doi: 10.1034/j.1398-9995.2003.00270.x
Iwata, M., Eshima, Y., Kagechika, H. (2003). Retinoic acids exert direct effects on T cells to suppress Th1 development and enhance Th2 development via retinoic acid receptors. Int. Immunol. 15 (8), 1017–1025. doi: 10.1093/intimm/dxg101
Iwata, M., Hirakiyama, A., Eshima, Y., Kagechika, H., Kato, C., Song, S. Y. (2004). Retinoic acid imprints gut-homing specificity on T cells. Immunity 21 (4), 527–538. doi: 10.1016/j.immuni.2004.08.011
Jang, H. Y., Lim, K., Lee, S. M., Park, B. H. (2014). Effects of n-3 PUFA on the CD4(+) type 2 helper T-cell-mediated immune responses in Fat-1 mice. Mol. Nutr. Food Res. 58 (2), 365–375. doi: 10.1002/mnfr.201300194
Jang, H. Y., Koo, J. H., Lee, S. M., Park, B. H. (2018). Atopic dermatitis-like skin lesions are suppressed in fat-1 transgenic mice through the inhibition of inflammasomes. Exp. Mol. Med. 50 (6), 73. doi: 10.1038/s12276-018-0104-3
Jeffery, L. E., Burke, F., Mura, M., Zheng, Y., Qureshi, O. S., Hewison, M., et al. (2009). 1,25-Dihydroxyvitamin D3 and IL-2 combine to inhibit T cell production of inflammatory cytokines and promote development of regulatory T cells expressing CTLA-4 and FoxP3. J. Immunol. 183 (9), 5458–5467. doi: 10.4049/jimmunol.0803217
Jeon, I., Kim, H., Kang, H., Lee, H., Jeong, S., Kim, S., et al. (2014). Anti-inflammatory and antipruritic effects of luteolin from Perilla (P. frutescens L.) leaves. Molecules 19 (6), 6941–6951. doi: 10.3390/molecules19066941
Jiang, Q. (2014). Natural forms of vitamin E: metabolism, antioxidant, and anti-inflammatory activities and their role in disease prevention and therapy. Free Radic. Biol. Med. 72, 76–90. doi: 10.1016/j.freeradbiomed.2014.03.035
Johansson-Lindbom, B., Svensson, M., Wurbel, M. A., Malissen, B., Marquez, G., Agace, W. (2003). Selective generation of gut tropic T cells in gut-associated lymphoid tissue (GALT): requirement for GALT dendritic cells and adjuvant. J. Exp. Med. 198 (6), 963–969. doi: 10.1084/jem.20031244
Jolliffe, D. A., Greenberg, L., Hooper, R. L., Griffiths, C. J., Camargo, C. A., Jr., Kerley, C. P., et al. (2017). Vitamin D supplementation to prevent asthma exacerbations: a systematic review and meta-analysis of individual participant data. Lancet Respir. Med. 5 (11), 881–890. doi: 10.1016/s2213-2600(17)30306-5
Julia, V., Macia, L., Dombrowicz, D. (2015). The impact of diet on asthma and allergic diseases. Nat. Rev. Immunol. 15 (5), 308–322. doi: 10.1038/nri3830
Kalayci, O., Besler, T., Kilinç, K., Sekerel, B. E., Saraçlar, Y. (2000). Serum levels of antioxidant vitamins (alpha tocopherol, beta carotene, and ascorbic acid) in children with bronchial asthma. Turk. J. Pediatr. 42 (1), 17–21.
Kambayashi, T., Koretzky, G. (2007). Proximal signaling events in FceRI-mediatedmast cell activation. J. Allergy Clin. Immunol. 119 (3), 544–552. doi: 10.1016/j.jaci.2007.01.017
Kaneko, M., Takimoto, H., Sugiyama, T., Seki, Y., Kawaguchi, K., Kumazawa, Y. (2008). Suppressive effects of the flavonoids quercetin and luteolin on the accumulation of lipid rafts after signal transduction via receptors. Immunopharmacol. Immunotoxicol. 30 (4), 867–882. doi: 10.1080/08923970802135690
Kang, O., Jang, H., Chae, H., Oh, Y., Choi, J., Lee, Y., et al. (2009). Anti-inflammatory mechanisms of resveratrol in activated HMC-1 cells: pivotal roles of NF-kappaB and MAPK. Pharmacol. Res. 59 (5), 330–337. doi: 10.1016/j.phrs.2009.01.009
Kang, O., Choi, J., Lee, J., Kwon, D. (2010). Luteolin Isolated from the Flowers of Lonicera japonica Suppresses Inflammatory Mediator Release by Blocking NF-κB and MAPKs Activation Pathways in HMC-1 Cells. Molecules 15 (1), 385–398. doi: 10.3390/molecules15010385
Kaulmann, A., Bohn, T. (2014). Carotenoids, inflammation, and oxidative stress–implications of cellular signaling pathways and relation to chronic disease prevention. Nutr. Res. 34 (11), 907–929. doi: 10.1016/j.nutres.2014.07.010
Kawamura, F., Hirashima, N., Furuno, T., Nakanishi, M. (2006). Effects of 2-methyl-1,4-naphtoquinone (menadione) on cellular signaling in RBL-2H3 cells. Biol. Pharm. Bull. 29 (4), 605–607. doi: 10.1248/bpb.29.605
Kawamura, F., Nakanishi, M., Hirashima, N. (2010). Effects of menadione, a reactive oxygen generator, on leukotriene secretion from RBL-2H3 cells. Biol. Pharm. Bull. 33 (5), 881–885. doi: 10.1248/bpb.33.881
Kelly, F. J., Mudway, I., Blomberg, A., Frew, A., Sandström, T. (1999). Altered lung antioxidant status in patients with mild asthma. Lancet 354 (9177), 482–483. doi: 10.1016/s0140-6736(99)01812-7
Kempna, P., Reiter, E., Arock, M., Azzi, A., Zingg, J. (2004). Inhibition of HMC-1 mast cell proliferation by vitamin E: involvement of the protein kinase B pathway. J. Biol. Chem. 279 (49), 50700–50709. doi: 10.1074/jbc.M410800200
Kim, J. S., Jobin, C. (2005). The flavonoid luteolin prevents lipopolysaccharide-induced NF-kappaB signalling and gene expression by blocking IkappaB kinase activity in intestinal epithelial cells and bone-marrow derived dendritic cells. Immunology 115 (3), 375–387. doi: 10.1111/j.1365-2567.2005.02156.x
Kim, G. Y., Cho, H., Ahn, S. C., Oh, Y. H., Lee, C. M., Park, Y. M. (2004a). Resveratrol inhibits phenotypic and functional maturation of murine bone marrow-derived dendritic cells. Int. Immunopharmacol. 4 (2), 245–253. doi: 10.1016/j.intimp.2003.12.009
Kim, G. Y., Kim, J. H., Ahn, S. C., Lee, H. J., Moon, D. O., Lee, C. M., et al. (2004b). Lycopene suppresses the lipopolysaccharide-induced phenotypic and functional maturation of murine dendritic cells through inhibition of mitogen-activated protein kinases and nuclear factor-kappaB. Immunology 113 (2), 203–211. doi: 10.1111/j.1365-2567.2004.01945.x
Kim, J., Seo, Y., Park, S., Park, S., Lim, S., Lee, S., et al. (2015). Spiraeoside inhibits mast cells activation and IgE-mediated allergic responses by suppressing phospholipase C-γ-mediated signaling. Biochem. Cell Biol. 93 (3), 227–235. doi: 10.1139/bcb-2014-0055
Kim, S. Y., Sim, S., Park, B., Kim, J. H., Choi, H. G. (2016). High-Fat and Low-Carbohydrate Diets Are Associated with Allergic Rhinitis But Not Asthma or Atopic Dermatitis in Children. PloS One 11 (2), e0150202. doi: 10.1371/journal.pone.0150202
Kim, W. S., Song, H. Y., Han, J. M., Byun, E. B. (2019). GLM, a novel luteolin derivative, attenuates inflammatory responses in dendritic cells: Therapeutic potential against ulcerative colitis. Biochem. Biophys. Res. Commun. 518 (1), 87–93. doi: 10.1016/j.bbrc.2019.08.012
Kimata, M., Shichijo, M., Miura, T., Serizawa, I., Inagaki, N., Nagai, H. (2000). Effects of luteolin, quercetin and baicalein on immunoglobulin E-mediated mediator release from human cultured mast cells. Clin. Exp. Allergy 30 (4), 501–508. doi: 10.1046/j.1365-2222.2000.00768.x
Kinoshita, T., Koike, K., Mwamtemi, H., Ito, S., Ishida, S., Nakazawa, Y., et al. (2000). Retinoic acid is a negative regulator for the differentiation of cord blood-derived human mast cell progenitors. Blood 95 (9), 2821–2828. doi: 10.1182/blood.V95.9.2821.009k11_2821_2828
Kiraly, N., Balde, A., Lisse, I. M., Eriksen, H. B., Aaby, P., Benn, C. S. (2013). Vitamin A supplementation and risk of atopy: long-term follow-up of a randomized trial of vitamin A supplementation at six and nine months of age. BMC Pediatr. 13, 190. doi: 10.1186/1471-2431-13-190
Klebanoff, C. A., Spencer, S. P., Torabi-Parizi, P., Grainger, J. R., Roychoudhuri, R., Ji, Y., et al. (2013). Retinoic acid controls the homeostasis of pre-cDC-derived splenic and intestinal dendritic cells. J. Exp. Med. 210 (10), 1961–1976. doi: 10.1084/jem.20122508
Koch, C., Dölle, S., Metzger, M., Rasche, C., Jungclas, H., Rühl, R., et al. (2008). Docosahexaenoic Acid (DHA) Supplementation in Atopic Eczema: A Randomized, Double-Blind, Controlled Trial. Br. J. Dermatol. 158 (4), 786–792. doi: 10.1111/j.1365-2133.2007.08430.x
Kong, W., Yen, J. H., Vassiliou, E., Adhikary, S., Toscano, M. G., Ganea, D. (2010). Docosahexaenoic acid prevents dendritic cell maturation and in vitro and in vivo expression of the IL-12 cytokine family. Lipids Health Dis. 9, 12. doi: 10.1186/1476-511x-9-12
Koo, N., Cho, D., Kim, Y., Choi, H., Kim, K. (2006). Effects of resveratrol on mast cell degranulation and tyrosine phosphorylation of the signaling components of the IgE receptor. Planta Med. 72 (7), 659–661. doi: 10.1055/s-2006-931568
Krauss-Etschmann, S., Hartl, D., Rzehak, P., Heinrich, J., Shadid, R., Del Carmen Ramírez-Tortosa, M., et al. (2008). Decreased cord blood IL-4, IL-13, and CCR4 and increased TGF-beta levels after fish oil supplementation of pregnant women. J. Allergy Clin. Immunol. 121 (2), 464–470. doi: 10.1016/j.jaci.2007.09.018
Krishnamoorthy, N., Burkett, P. R., Dalli, J., Abdulnour, R. E., Colas, R., Ramon, S., et al. (2015). Cutting edge: maresin-1 engages regulatory T cells to limit type 2 innate lymphoid cell activation and promote resolution of lung inflammation. J. Immunol. 194 (3), 863–867. doi: 10.4049/jimmunol.1402534
Kuhn, G., Pallauf, K., Schulz, C., Birringer, M., Diaz-Rica, B., de Pascual-Teresa, S., et al. (2018). Resveratrol Modulates Desaturase Expression and Fatty Acid Composition of Cultured Hepatocytes. Front. Nutr. 5, 106. doi: 10.3389/fnut.2018.00106
Kutner, A., Brown, G. (2018). Vitamins D: Relationship between Structure and Biological Activity. Int. J. Mol. Sci. 19 (7), 2119. doi: 10.3390/ijms19072119
Lai, G., Wu, C., Hong, J., Song, Y. (2013). 1,25-Dihydroxyvitamin D(3) (1,25-(OH)(2)D(3)) attenuates airway remodeling in a murine model of chronic asthma. J. Asthma 50 (2), 133–140. doi: 10.3109/02770903.2012.738269
Lampen, A., Meyer, S., Arnhold, T., Nau, H. (2000). Metabolism of vitamin A and its active metabolite all-trans-retinoic acid in small intestinal enterocytes. J. Pharmacol. Exp. Ther. 295 (3), 979–985.
Lee, G. Y., Han, S. N. (2018). The Role of Vitamin E in Immunity. Nutrients 10 (11), 1614. doi: 10.3390/nu10111614
Lee, C. M., Chang, J. H., Moon, D. O., Choi, Y. H., Choi, I. W., Park, Y. M., et al. (2008). Lycopene suppresses ovalbumin-induced airway inflammation in a murine model of asthma. Biochem. Biophys. Res. Commun. 374 (2), 248–252. doi: 10.1016/j.bbrc.2008.07.032
Lee, H. S., Barraza-Villarreal, A., Hernandez-Vargas, H., Sly, P. D., Biessy, C., Ramakrishnan, U., et al. (2013). Modulation of DNA methylation states and infant immune system by dietary supplementation with omega-3 PUFA during pregnancy in an intervention study. Am. J. Clin. Nutr. 98 (2), 480–487. doi: 10.3945/ajcn.112.052241
Lee, J. Y., Kim, J. M., Kim, C. J. (2014). Flavones derived from nature attenuate the immediate and late-phase asthmatic responses to aerosolized-ovalbumin exposure in conscious guinea pigs. Inflammation Res. 63 (1), 53–60. doi: 10.1007/s00011-013-0670-8
Lee, H. Y., Kim, I. K., Yoon, H. K., Kwon, S. S., Rhee, C. K., Lee, S. Y. (2017). Inhibitory Effects of Resveratrol on Airway Remodeling by Transforming Growth Factor-beta/Smad Signaling Pathway in Chronic Asthma Model. Allergy Asthma Immunol. Res. 9 (1), 25–34. doi: 10.4168/aair.2017.9.1.25
Lee, H. C., Tominaga, M., Yasukawa, K., Ohba, M., Takahashi, N., Honda, K., et al. (2019). Dietary supplementation of omega-3 fatty acid eicosapentaenoic acid does not ameliorate pruritus in murine models of atopic dermatitis and psoriasis. J. Dermatol. Sci. 95 (3), 130–133. doi: 10.1016/j.jdermsci.2019.07.010
Lee-Sarwar, K., Kelly, R. S., Lasky-Su, J., Kachroo, P., Zeiger, R. S., O’Connor, G. T., et al. (2019). Dietary and Plasma Polyunsaturated Fatty Acids Are Inversely Associated with Asthma and Atopy in Early Childhood. J. Allergy Clin. Immunol. Pract. 7 (2), 529–538. doi: 10.1016/j.jaip.2018.07.039
Lemanske, R. F., Jr., Busse, W. W. (2010). Asthma: clinical expression and molecular mechanisms. J. Allergy Clin. Immunol. 125 (2 Suppl 2), S95–102. doi: 10.1016/j.jaci.2009.10.047
Lemire, J. M., Adams, J. S., Sakai, R., Jordan, S. C. (1984). 1 alpha,25-dihydroxyvitamin D3 suppresses proliferation and immunoglobulin production by normal human peripheral blood mononuclear cells. J. Clin. Invest. 74 (2), 657–661. doi: 10.1172/jci111465
Lemire, J. M., Adams, J. S., Kermani-Arab, V., Bakke, A. C., Sakai, R., Jordan, S. C. (1985). 1,25-Dihydroxyvitamin D3 suppresses human T helper/inducer lymphocyte activity in vitro. J. Immunol. 134 (5), 3032–3035.
Li, Y., Wongsiriroj, N., Blaner, W. S. (2014). The multifaceted nature of retinoid transport and metabolism. Hepatobil. Surg. Nutr. 3 (3), 126–139. doi: 10.3978/j.issn.2304-3881.2014.05.04
Li, L., Zhang, X., Liu, G., Liu, C., Dong, Y. (2016). Isoquercitrin suppresses the expression of histamine and pro-inflammatory cytokines by inhibiting the activation of MAP Kinases and NF-κB in human KU812 cells. Chin. J. Nat. Med. 14 (6), 407–412. doi: 10.1016/S1875-5364(16)30036-X
Li, X., Lee, Y., Jin, F., Park, Y., Deng, Y., Kang, Y., et al. (2017). Sirt1 negatively regulates FcϵRImediated mast cell activation through AMPK- and PTP1Bdependent processes. Sci. Rep. 7 (1), 6444. doi: 10.1038/s41598-017-06835-3
Lim, Y., Vasu, V. T., Valacchi, G., Leonard, S., Aung, H. H., Schock, B. C., et al. (2008). Severe vitamin E deficiency modulates airway allergic inflammatory responses in the murine asthma model. Free Radic. Res. 42 (4), 387–396. doi: 10.1080/10715760801976600
Lin, Y., Shi, R., Wang, X., Shen, H.-M. (2008). Luteolin, a flavonoid with potentials for cancer prevention and therapy. Curr. Cancer Drug Targets 8 (7), 634–646. doi: 10.2174/156800908786241050
Lin, W., Wang, W., Wang, D., Ling, W. (2017). Quercetin protects against atherosclerosis by inhibiting dendritic cell activation. Mol. Nutr. Food Res. 61 (9). doi: 10.1002/mnfr.201700031
Lindskou, R., Holmer, G. (1992). Polyunsaturated fatty acids in plasma, red blood cells and mononuclear cell phospholipids of patients with atopic dermatitis. Allergy 47 (5), 517–521. doi: 10.1111/j.1398-9995.1992.tb00675.x
Litonjua, A. A., Carey, V. J., Laranjo, N., Harshfield, B. J., McElrath, T. F., O’Connor, G. T., et al. (2016). Effect of Prenatal Supplementation With Vitamin D on Asthma or Recurrent Wheezing in Offspring by Age 3 Years: The VDAART Randomized Clinical Trial. Jama 315 (4), 362–370. doi: 10.1001/jama.2015.18589
Liu, Z., Li, X., Qiu, S., Yu, Y., Li, M., Yang, L., et al. (2017). Vitamin D contributes to mast cell stabilization. Allergy 72 (8), 1184–1192. doi: 10.1111/all.13110
Li-Weber, M., Weigand, M. A., Giaisi, M., Süss, D., Treiber, M. K., Baumann, S., et al. (2002). Vitamin E inhibits CD95 ligand expression and protects T cells from activation-induced cell death. J. Clin. Invest. 110 (5), 681–690. doi: 10.1172/jci15073
Lovett-Racke, A. E., Racke, M. K. (2002). Retinoic acid promotes the development of Th2-like human myelin basic protein-reactive T cells. Cell Immunol. 215 (1), 54–60. doi: 10.1016/s0008-8749(02)00013-8
Lucas, R., Mihaly, J., Lowe, G. M., Graham, D. L., Szklenar, M., Szegedi, A., et al. (2018). Reduced Carotenoid and Retinoid Concentrations and Altered Lycopene Isomer Ratio in Plasma of Atopic Dermatitis Patients. Nutrients 10 (10), 1390. doi: 10.3390/nu10101390
Lv, C., Zhang, Y., Shen, L. (2018). Preliminary Clinical Effect Evaluation of Resveratrol in Adults with Allergic Rhinitis. Int. Arch. Allergy Immunol. 175 (4), 231–236. doi: 10.1159/000486959
Mabalirajan, U., Aich, J., Leishangthem, G. D., Sharma, S. K., Dinda, A. K., Ghosh, B. (2009). Effects of vitamin E on mitochondrial dysfunction and asthma features in an experimental allergic murine model. J. Appl. Physiol. (1985) 107 (4), 1285–1292. doi: 10.1152/japplphysiol.00459.2009
Magnusson, J., Kull, I., Westman, M., Håkansson, N., Wolk, A., Melén, E., et al. (2015). Fish and polyunsaturated fat intake and development of allergic and nonallergic rhinitis. J. Allergy Clin. Immunol. 136 (5), 1247–1253. doi: 10.1016/j.jaci.2015.05.030
Mahoney, C. W., Azzi, A. (1988). Vitamin E inhibits protein kinase C activity. Biochem. Biophys. Res. Commun. 154 (2), 694–697. doi: 10.1016/0006-291x(88)90195-7
Malaguarnera, L. (2019). Influence of Resveratrol on the Immune Response. Nutrients 11 (5), 946. doi: 10.3390/nu11050946
Manabe, Y., Hirata, T., Sugawara, T. (2014). Suppressive Effects of Carotenoids on the Antigen-induced Degranulation in RBL-2H3 Rat Basophilic Leukemia Cells. J. Oleo Sci. 63 (3), 291–294. doi: 10.5650/jos.ess13169
Mashurabad, P. C., Palika, R., Jyrwa, Y. W., Bhaskarachary, K., Pullakhandam, R. (2017). Dietary fat composition, food matrix and relative polarity modulate the micellarization and intestinal uptake of carotenoids from vegetables and fruits. J. Food Sci. Technol. 54 (2), 333–341. doi: 10.1007/s13197-016-2466-7
Maslova, E., Hansen, S., Strøm, M., Halldorsson, T. I., Olsen, S. F. (2014). Maternal intake of vitamins A, E and K in pregnancy and child allergic disease: a longitudinal study from the Danish National Birth Cohort. Br. J. Nutr. 111 (6), 1096–1108. doi: 10.1017/s0007114513003395
Matheu, V., Berggard, K., Barrios, Y., Barrios, Y., Arnau, M. R., Zubeldia, J. M., et al. (2009). Impact on allergic immune response after treatment with vitamin A. Nutr. Metab. (Lond) 6, 44. doi: 10.1186/1743-7075-6-44
Matsuda, H., Watanabe, N., Geba, G. P., Sperl, J., Tsudzuki, M., Hiroi, J., et al. (1997). Development of atopic dermatitis-like skin lesion with IgE hyperproduction in NC/Nga mice. Int. Immunol. 9 (3), 461–466. doi: 10.1093/intimm/9.3.461
Matsui, T., Tanaka, K., Nakagawa, T., Sasaki, K., Nakata, J., Sugiura, S., et al. (2015). Sun exposure inversely related to food sensitization during infancy. Pediatr. Allergy Immunol. 26 (7), 628–633. doi: 10.1111/pai.12445
Matsui, T., Yamashita, H., Saneyasu, K. I., Tanaka, H., Ito, K., Inagaki, N. (2018). Vitamin D deficiency exacerbates sensitization and allergic diarrhea in a murine food allergy model. Allergol. Int. 67 (2), 289–291. doi: 10.1016/j.alit.2017.08.010
Mayser, P., Mayer, K., Mahloudjian, M., Benzing, S., Krämer, H.-J., Schill, W.-B., et al. (2002). A double-blind, randomized, placebo-controlled trial of n-3 versus n-6 fatty acid-based lipid infusion in atopic dermatitis. JPEN J. Parenter Enteral Nutr. 26 (3), 151–158. doi: 10.1177/0148607102026003151
Mazzocchi, A., Leone, L., Agostoni, C., Pali-Scholl, I. (2019). The Secrets of the Mediterranean Diet. Does [Only] Olive Oil Matter? Nutrients 11 (12), 2941. doi: 10.3390/nu11122941
McCary, C. A., Abdala-Valencia, H., Berdnikovs, S., Cook-Mills, J. M. (2011). Supplemental and highly elevated tocopherol doses differentially regulate allergic inflammation: reversibility of alpha-tocopherol and gamma-tocopherol’s effects. J. Immunol. 186 (6), 3674–3685. doi: 10.4049/jimmunol.1003037
Metzler, M. A., Sandell, L. L. (2016). Enzymatic Metabolism of Vitamin A in Developing Vertebrate Embryos. Nutrients 8 (12), 812. doi: 10.3390/nu8120812
Michalski, J., Deinzer, A., Stich, L., Zinser, E., Steinkasserer, A., Knippertz, I. (2020). Quercetin induces an immunoregulatory phenotype in maturing human dendritic cells. Immunobiology 151929. doi: 10.1016/j.imbio.2020.151929
Mickleborough, T. D., Murray, R. L., Ionescu, A. A., Lindley, M. R. (2003). Fish oil supplementation reduces severity of exercise-induced bronchoconstriction in elite athletes. Am. J. Respir. Crit. Care Med. 168 (10), 1181–1189. doi: 10.1164/rccm.200303-373OC
Mickleborough, T. D., Lindley, M. R., Ionescu, A. A., Fly, A. D. (2006). Protective effect of fish oil supplementation on exercise-induced bronchoconstriction in asthma. Chest 129 (1), 39–49. doi: 10.1378/chest.129.1.39
Mihály, J., Gericke, J., Töröcsik, D., Gaspar, K., Szegedi, A., Rühl, R. (2013). Reduced lipoxygenase and cyclooxygenase mediated signaling in PBMC of atopic dermatitis patients. Prostaglandins Other Lipid Mediat. 107, 35–42. doi: 10.1016/j.prostaglandins.2013.03.003
Mihály, J., Marosvölgyi, T., Szegedi, A., Köröskényi, K., Lucas, R., Töröcsik, D., et al. (2014). Increased FADS2-derived n-6 PUFAs and reduced n-3 PUFAs in plasma of atopic dermatitis patients. Skin Pharmacol. Physiol. 27 (5), 242–248. doi: 10.1159/000358290
Mihrshahi, S., Peat, J. K., Webb, K., Oddy, W., Marks, G. B., Mellis, C. M., et al. (2004). Effect of omega-3 fatty acid concentrations in plasma on symptoms of asthma at 18 months of age. Pediatr. Allergy Immunol. 15 (6), 517–522. doi: 10.1111/j.1399-3038.2004.00187.x
Miles, E. A., Calder, P. C. (2012). Influence of marine n-3 polyunsaturated fatty acids on immune function and a systematic review of their effects on clinical outcomes in rheumatoid arthritis. Br. J. Nutr. 107 Suppl 2, S171–S184. doi: 10.1017/S0007114512001560
Mills, L. M., Wilson, H., Thies, F. (2012). Lycopene inhibits lymphocyte proliferation through mechanisms dependent on early cell activation. Mol. Nutr. Food Res. 56 (7), 1034–1042. doi: 10.1002/mnfr.201200047
Molloy, J., Koplin, J. J., Allen, K. J., Tang, M. L., Collier, F., Carlin, J. B., et al. (2017). Vitamin D insufficiency in the first 6 months of infancy and challenge-proven IgE-mediated food allergy at 1 year of age: a case-cohort study. Allergy 72 (8), 1222–1231. doi: 10.1111/all.13122
Monkawa, T., Yoshida, T., Hayashi, M., Saruta, T. (2000). Identification of 25-hydroxyvitamin D3 1alpha-hydroxylase gene expression in macrophages. Kidney Int. 58 (2), 559–568. doi: 10.1046/j.1523-1755.2000.00202.x
Montes, R., Chisaguano, A. M., Castellote, A. I., Morales, E., Sunyer, J., López-Sabater, M. C. (2013). Fatty-acid composition of maternal and umbilical cord plasma and early childhood atopic eczema in a Spanish cohort. Eur. J. Clin. Nutr. 67 (6), 658–663. doi: 10.1038/ejcn.2013.68
Moon, H., Choi, H. H., Lee, J. Y., Moon, H. J., Sim, S. S., Kim, C. J. (2008). Quercetin inhalation inhibits the asthmatic responses by exposure to aerosolized-ovalbumin in conscious guinea-pigs. Arch. Pharm. Res. 31 (6), 771–778. doi: 10.1007/s12272-001-1225-2
Mora, J. R., Bono, M. R., Manjunath, N., Weninger, W., Cavanagh, L. L., Rosemblatt, M., et al. (2003). Selective imprinting of gut-homing T cells by Peyer’s patch dendritic cells. Nature 424 (6944), 88–93. doi: 10.1038/nature01726
Mucida, D., Park, Y., Kim, G., Turovskaya, O., Scott, I., Kronenberg, M., et al. (2007). Reciprocal TH17 and regulatory T cell differentiation mediated by retinoic acid. Science 317 (5835), 256–260. doi: 10.1126/science.1145697
Myneni, V. D., Mezey, E. (2018). Immunomodulatory effect of vitamin K2: Implications for bone health. Oral. Dis. 24 (1-2), 67–71. doi: 10.1111/odi.12759
Neeland, M. R., Koplin, J. J., Dang, T. D., Dharmage, S. C., Tang, M. L., Prescott, S. L., et al. (2018). Early life innate immune signatures of persistent food allergy. J. Allergy Clin. Immunol. 142857-864 (3), e853. doi: 10.1016/j.jaci.2017.10.024
Neuman, I., Nahum, H., Ben-Amotz, A. (2000). Reduction of exercise-induced asthma oxidative stress by lycopene, a natural antioxidant. Allergy 55 (12), 1184–1189. doi: 10.1034/j.1398-9995.2000.00748.x
Norizoe, C., Akiyama, N., Segawa, T., Tachimoto, H., Mezawa, H., Ida, H., et al. (2014). Increased food allergy and vitamin D: randomized, double-blind, placebo-controlled trial. Pediatr. Int. 56 (1), 6–12. doi: 10.1111/ped.12207
Nurmatov, U., Devereux, G., Sheikh, A. (2011). Nutrients and foods for the primary prevention of asthma and allergy: systematic review and meta-analysis. J. Allergy Clin. Immunol. 127724-733 (3), e721–e730. doi: 10.1016/j.jaci.2010.11.001
Nwaru, B. I., Ahonen, S., Kaila, M., Erkkola, M., Haapala, A. M., Kronberg-Kippila, C., et al. (2010). Maternal diet during pregnancy and allergic sensitization in the offspring by 5 yrs of age: a prospective cohort study. Pediatr. Allergy Immunol. 21 (1 Pt 1), 29–37. doi: 10.1111/j.1399-3038.2009.00949.x
Nwaru, B. I., Erkkola, M., Ahonen, S., Kaila, M., Kronberg-Kippila, C., Ilonen, J., et al. (2011). Intake of antioxidants during pregnancy and the risk of allergies and asthma in the offspring. Eur. J. Clin. Nutr. 65 (8), 937–943. doi: 10.1038/ejcn.2011.67
Obata, T., Nagakura, T., Masaki, T., Maekawa, K., Yamashita, K. (1999). Eicosapentaenoic acid inhibits prostaglandin D2 generation by inhibiting cyclo-oxygenase-2 in cultured human mast cells. Clin. Exp. Allergy 29 (8), 1129–1135. doi: 10.1046/j.1365-2222.1999.00604.x
Oh, D. Y., Talukdar, S., Bae, E. J., Imamura, T., Morinaga, H., Fan, W., et al. (2010). GPR120 is an omega-3 fatty acid receptor mediating potent anti-inflammatory and insulin-sensitizing effects. Cell 142 (5), 687–698. doi: 10.1016/j.cell.2010.07.041
Ohsaki, Y., Shirakawa, H., Hiwatashi, K., Furukawa, Y., Mizutani, T., Komai, M. (2006). Vitamin K suppresses lipopolysaccharide-induced inflammation in the rat. Biosci. Biotechnol. Biochem. 70 (4), 926–932. doi: 10.1271/bbb.70.926
Okada, Y., Oh-oka, K., Nakamura, Y., Ishimaru, K., Matsuoka, S., Okumura, K., et al. (2012). Dietary resveratrol prevents the development of food allergy in mice. PloS One 7 (9), e44338. doi: 10.1371/journal.pone.0044338
Ostermann, A., West, A., Schoenfeld, K., Browning, L., Walker, C., Jebb, S., et al. (2019). Plasma oxylipins respond in a linear dose-response manner with increased intake of EPA and DHA: results from a randomized controlled trial in healthy humans. Am. J. Clin. Nutr. 109 (5), 1251–1263. doi: 10.1093/ajcn/nqz016
Pacheco-Gonzalez, R. M., Garcia-Marcos, L., Morales, E. (2018). Prenatal vitamin D status and respiratory and allergic outcomes in childhood: A meta-analysis of observational studies. Pediatr. Allergy Immunol. 29 (3), 243–253. doi: 10.1111/pai.12876
Pallares, V., Calay, D., Cedo, L., Castell-Auvi, A., Raes, M., Pinent, M., et al. (2012). Enhanced anti-inflammatory effect of resveratrol and EPA in treated endotoxin-activated RAW 264.7 macrophages. Br. J. Nutr. 108 (9), 1562–1573. doi: 10.1017/S0007114511007057
Palmer, D. J., Sullivan, T., Gold, M. S., Prescott, S. L., Heddle, R., Gibson, R. A., et al. (2012). Effect of n-3 long chain polyunsaturated fatty acid supplementation in pregnancy on infants’ allergies in first year of life: randomised controlled trial. BMJ 344, e184. doi: 10.1136/bmj.e184
Palmer, D. J., Sullivan, T., Gold, M. S., Prescott, S. L., Heddle, R., Gibson, R. A., et al. (2013). Randomized controlled trial of fish oil supplementation in pregnancy on childhood allergies. Allergy 68 (11), 1370–1376. doi: 10.1111/all.12233
Pan, M. H., Lai, C. S., Ho, C. T. (2010). Anti-inflammatory activity of natural dietary flavonoids. Food Funct. 1 (1), 15–31. doi: 10.1039/c0fo00103a
Park, H. J., Lee, C. M., Jung, I. D., Lee, J. S., Jeong, Y. I., Chang, J. H., et al. (2009). Quercetin regulates Th1/Th2 balance in a murine model of asthma. Int. Immunopharmacol. 9 (3), 261–267. doi: 10.1016/j.intimp.2008.10.021
Park, B., Park, S., Park, J., Park, M., Min, T., Jin, M. (2013). Omega-3 fatty acids suppress Th2-associated cytokine gene expressions and GATA transcription factors in mast cells. J. Nutr. Biochem. 24 (5), 868–876. doi: 10.1016/j.jnutbio.2012.05.007
Peat, J. K., Mihrshahi, S., Kemp, A. S., Marks, G. B., Tovey, E. R., Webb, K., et al. (2004). Three-year outcomes of dietary fatty acid modification and house dust mite reduction in the Childhood Asthma Prevention Study. J. Allergy Clin. Immunol. 114 (4), 807–813. doi: 10.1016/j.jaci.2004.06.057
Penna, G., Adorini, L. (2000). 1 Alpha,25-dihydroxyvitamin D3 inhibits differentiation, maturation, activation, and survival of dendritic cells leading to impaired alloreactive T cell activation. J. Immunol. 164 (5), 2405–2411. doi: 10.4049/jimmunol.164.5.2405
Penna, G., Roncari, A., Amuchastegui, S., Daniel, K. C., Berti, E., Colonna, M., et al. (2005). Expression of the inhibitory receptor ILT3 on dendritic cells is dispensable for induction of CD4+Foxp3+ regulatory T cells by 1,25-dihydroxyvitamin D3. Blood 106 (10), 3490–3497. doi: 10.1182/blood-2005-05-2044
Penna, G., Amuchastegui, S., Cossetti, C., Aquilano, F., Mariani, R., Sanvito, F., et al. (2006). Treatment of experimental autoimmune prostatitis in nonobese diabetic mice by the vitamin D receptor agonist elocalcitol. J. Immunol. 177 (12), 8504–8511. doi: 10.4049/jimmunol.177.12.8504
Pfeffer, P. E., Hawrylowicz, C. M. (2018). Vitamin D in Asthma: Mechanisms of Action and Considerations for Clinical Trials. Chest 153 (5), 1229–1239. doi: 10.1016/j.chest.2017.09.005
Poon, A., Mahboub, B., Hamid, Q. (2013). Vitamin D deficiency and severe asthma. Pharmacol. Ther. 140 (2), 148–155. doi: 10.1016/j.pharmthera.2013.06.006
Price, O., Hull, J., Howatson, G., Robson-Ansley, P., Ansley, L. (2015). Vitamin D and omega-3 polyunsaturated fatty acid supplementation in athletes with exercise-induced bronchoconstriction: a pilot study. Expert Rev. Respir. Med. 9 (3), 369–378. doi: 10.1586/17476348.2015.1036032
Quan, G., Chae, H., Song, H., Ahn, K., Lee, H., Kim, Y., et al. (2013). Anti-allergic flavones from Arthraxon hispidus. Chem. Pharm. Bull. 61 (9), 920–926. doi: 10.1248/cpb.c13-00239
Rajnavolgyi, E., Laczik, R., Kun, V., Szente, L., Fenyvesi, E. (2014). Effects of RAMEA-complexed polyunsaturated fatty acids on the response of human dendritic cells to inflammatory signals. Beilstein J. Org. Chem. 10, 3152–3160. doi: 10.3762/bjoc.10.332
Raverdeau, M., Mills, K. H. (2014). Modulation of T cell and innate immune responses by retinoic Acid. J. Immunol. 192 (7), 2953–2958. doi: 10.4049/jimmunol.1303245
Riccioni, G., Bucciarelli, T., Mancini, B., Di Ilio, C., D’Orazio, N. (2006). Plasma lycopene concentration is low in subjects with stable asthma. Allergy 61 (11), 1371–1372. doi: 10.1111/j.1398-9995.2006.01100.x
Rinaldi de Alvarenga, J. F., Quifer-Rada, P., Francetto Juliano, F., Hurtado-Barroso, S., Illan, M., Torrado-Prat, X., et al. (2019). Using Extra Virgin Olive Oil to Cook Vegetables Enhances Polyphenol and Carotenoid Extractability: A Study Applying the sofrito Technique. Molecules 24 (8), 1555. doi: 10.3390/molecules24081555
Roche, P., Furuta, K. (2015). The ins and outs of mhc class-II-mediated antigen processing and presentation. Nat. Rev. Immunol. 15 (4), 203–216. doi: 10.1038/nri3818
Rogerio, A. P., Kanashiro, A., Fontanari, C., da Silva, E. V., Lucisano-Valim, Y. M., Soares, E. G., et al. (2007). Anti-inflammatory activity of quercetin and isoquercitrin in experimental murine allergic asthma. Inflammation Res. 56 (10), 402–408. doi: 10.1007/s00011-007-7005-6
Rogerio, A. P., Dora, C. L., Andrade, E. L., Chaves, J. S., Silva, L. F., Lemos-Senna, E., et al. (2010). Anti-inflammatory effect of quercetin-loaded microemulsion in the airways allergic inflammatory model in mice. Pharmacol. Res. 61 (4), 288–297. doi: 10.1016/j.phrs.2009.10.005
Rosenlund, H., Fagerstedt, S., Alm, J., Mie, A. (2016). Breastmilk fatty acids in relation to sensitization - the ALADDIN birth cohort. Allergy 71 (10), 1444–1452. doi: 10.1111/all.12896
Ruhl, R., Koch, C., Marosvolgyi, T., Mihaly, J., Schweigert, F. J., Worm, M., et al. (2008). Fatty acid composition of serum lipid classes in mice following allergic sensitisation with or without dietary docosahexaenoic acid-enriched fish oil substitution. Br. J. Nutr. 99 (6), 1239–1246. doi: 10.1017/s0007114507862374
Ruhl, R. (2013). Non-pro-vitamin A and pro-vitamin A carotenoids in atopy development. Int. Arch. Allergy Immunol. 161 (2), 99–115. doi: 10.1159/000345958
Sakamoto, H., Koya, T., Tsukioka, K., Shima, K., Watanabe, S., Kagamu, H., et al. (2015). The Effects of All-Trans Retinoic Acid on the Induction of Oral Tolerance in a Murine Model of Bronchial Asthma. Int. Arch. Allergy Immunol. 167 (3), 167–176. doi: 10.1159/000437326
Salinthone, S., Kerns, A. R., Tsang, V., Carr, D. W. (2013). alpha-Tocopherol (vitamin E) stimulates cyclic AMP production in human peripheral mononuclear cells and alters immune function. Mol. Immunol. 53 (3), 173–178. doi: 10.1016/j.molimm.2012.08.005
Sánchez-Sánchez, M., García-Vigara, A., Hidalgo-Mora, J., García-Pérez, M.-A., Tarín, J., Cano, A. (2020). Mediterranean diet and health: A systematic review of epidemiological studies and intervention trials. Maturitas 136, 25–37. doi: 10.1016/j.maturitas.2020.03.008
Savage, J. H., Lee-Sarwar, K. A., Sordillo, J., Bunyavanich, S., Zhou, Y., O’Connor, G., et al. (2018). A prospective microbiome-wide association study of food sensitization and food allergy in early childhood. Allergy 73 (1), 145–152. doi: 10.1111/all.13232
Schmutzler, S., Bachmann, L., Fuhrmann, H., Schumann, J. (2010). PUFA-dependent alteration of oxidative parameters of a canine mastocytoma cell line. Acta Vet. Hung. 58 (4), 453–464. doi: 10.1556/avet.58.2010.4.6
Schubert, R., Kitz, R., Beermann, C., Rose, M. A., Lieb, A., Sommerer, P. C., et al. (2009). Effect of n-3 polyunsaturated fatty acids in asthma after low-dose allergen challenge. Int. Arch. Allergy Immunol. 148 (4), 321–329. doi: 10.1159/000170386
Schuster, G. U., Kenyon, N. J., Stephensen, C. B. (2008). Vitamin A deficiency decreases and high dietary vitamin A increases disease severity in the mouse model of asthma. J. Immunol. 180 (3), 1834–1842. doi: 10.4049/jimmunol.180.3.1834
Schweigert, F. J. (2001). Inflammation-induced changes in the nutritional biomarkers serum retinol and carotenoids. Curr. Opin. Clin. Nutr. Metab. Care 4 (6), 477–481. doi: 10.1097/00075197-200111000-00002
Scirpo, R., Fiorotto, R., Villani, A., Amenduni, M., Spirli, C., Strazzabosco, M. (2015). Stimulation of nuclear receptor PPAR-γ limits NF-kB-dependent inflammation in mouse cystic fibrosis biliary epithelium. Hepatology 62 (5), 1551–1562. doi: 10.1002/hep.28000
Serhan, C. N., Chiang, N., Van Dyke, T. E. (2008). Resolving inflammation: dual anti-inflammatory and pro-resolution lipid mediators. Nat. Rev. Immunol. 8 (5), 349–361. doi: 10.1038/nri2294
Serini, S., Cassano, R., Corsetto, P. A., Rizzo, A. M., Calviello, G., Trombino, S. (2018). Omega-3 PUFA Loaded in Resveratrol-Based Solid Lipid Nanoparticles: Physicochemical Properties and Antineoplastic Activities in Human Colorectal Cancer Cells In Vitro. Int. J. Mol. Sci. 19 (2), 586. doi: 10.3390/ijms19020586
Serini, S., Cassano, R., Facchinetti, E., Amendola, G., Trombino, S., Calviello, G. (2019). Anti-Irritant and Anti-Inflammatory Effects of DHA Encapsulated in Resveratrol-Based Solid Lipid Nanoparticles in Human Keratinocytes. Nutrients 11 (6), 1400. doi: 10.3390/nu11061400
Sharief, S., Jariwala, S., Kumar, J., Muntner, P., Melamed, M. L. (2011). Vitamin D levels and food and environmental allergies in the United States: results from the National Health and Nutrition Examination Survey 2005-2006. J. Allergy Clin. Immunol. 127 (5), 1195–1202. doi: 10.1016/j.jaci.2011.01.017
Sharma, S., Chopra, K., Kulkarni, S. K., Agrewala, J. N. (2007). Resveratrol and curcumin suppress immune response through CD28/CTLA-4 and CD80 co-stimulatory pathway. Clin. Exp. Immunol. 147 (1), 155–163. doi: 10.1111/j.1365-2249.2006.03257.x
Shea, M. K., Booth, S. L., Massaro, J. M., Jacques, P. F., D’Agostino, R. B., Dawson-Hughes, B., et al. (2008). Vitamin K and vitamin D status: associations with inflammatory markers in the Framingham Offspring Study. Am. J. Epidemiol. 167 (3), 313–320. doi: 10.1093/aje/kwm306
Shimanaka, Y., Kono, N., Taketomi, Y., Arita, M., Okayama, Y., Tanaka, Y., et al. (2017). Omega-3 fatty acid epoxides are autocrine mediators that control the magnitude of IgE-mediated mast cell activation. Nat. Med. 23 (11), 1287–1297. doi: 10.1038/nm.4417
Shirley, D., McHale, C., Gomez, G. (2016). Resveratrol preferentially inhibits IgE-dependent PGD2 biosynthesis but enhances TNF production from human skin mast cells. Biochim. Biophys. Acta 1860 (4), 678–685. doi: 10.1016/j.bbagen.2016.01.006
Sicherer, S. H., Munoz-Furlong, A., Godbold, J. H., Sampson, H. A. (2010). US prevalence of self-reported peanut, tree nut, and sesame allergy: 11-year follow-up. J. Allergy Clin. Immunol. 125 (6), 1322–1326. doi: 10.1016/j.jaci.2010.03.029
Sierra, S., Lara-Villoslada, F., Comalada, M., Olivares, M., Xaus, J. (2008). Dietary eicosapentaenoic acid and docosahexaenoic acid equally incorporate as decosahexaenoic acid but differ in inflammatory effects. Nutrition 24 (3), 245–254. doi: 10.1016/j.nut.2007.11.005
Sigmundsdottir, H., Pan, J., Debes, G. F., Alt, C., Habtezion, A., Soler, D., et al. (2007). DCs metabolize sunlight-induced vitamin D3 to ‘program’ T cell attraction to the epidermal chemokine CCL27. Nat. Immunol. 8 (3), 285–293. doi: 10.1038/ni1433
Silva, A. M., Oliveira, M. I., Sette, L., Almeida, C. R., Oliveira, M. J., Barbosa, M. A., et al. (2014). Resveratrol as a natural anti-tumor necrosis factor-alpha molecule: implications to dendritic cells and their crosstalk with mesenchymal stromal cells. PloS One 9 (3), e91406. doi: 10.1371/journal.pone.0091406
Smit, H. A., Grievink, L., Tabak, C. (1999). Dietary influences on chronic obstructive lung disease and asthma: a review of the epidemiological evidence. Proc. Nutr. Soc. 58 (2), 309–319. doi: 10.1017/s0029665199000427
Spector, A., Kim, H.-Y. (2016). Cytochrome P450 epoxygenase pathway of polyunsaturated fatty acid metabolism. Biochim. Biophys. Acta 1851 (4), 356–365. doi: 10.1016/j.bbalip.2014.07.020
Spergel, J. M. (2010). From atopic dermatitis to asthma: the atopic march. Ann. Allergy Asthma Immunol. 105 (2), 99–106; quiz 107-109, 117. doi: 10.1016/j.anai.2009.10.002
Sprecher, H., Luthria, D., Mohammed, B., Baykousheva, S. (1995). Reevaluation of the pathways for the biosynthesis of polyunsaturated fatty acids. J. Lipid Res. 36 (12), 2471–2477. doi: 10.1201/9781439831939.ch2
Staeva-Vieira, T. P., Freedman, L. P. (2002). 1,25-dihydroxyvitamin D3 inhibits IFN-gamma and IL-4 levels during in vitro polarization of primary murine CD4+ T cells. J. Immunol. 168 (3), 1181–1189. doi: 10.4049/jimmunol.168.3.1181
Steinman, R. M., Banchereau, J. (2007). Taking dendritic cells into medicine. Nature 449 (7161), 419–426. doi: 10.1038/nature06175
Stratakis, N., Gielen, M., Margetaki, K., de Groot, R. H. M., Apostolaki, M., Chalkiadaki, G., et al. (2018). PUFA status at birth and allergy-related phenotypes in childhood: a pooled analysis of the Maastricht Essential Fatty Acid Birth (MEFAB) and RHEA birth cohorts. Br. J. Nutr. 119 (2), 202–210. doi: 10.1017/S0007114517003348
Suzuki, R., Shimizu, T., Kudo, T., Ohtsuka, Y., Yamashiro, Y., Oshida, K. (2002). Effects of n-3 polyunsaturated fatty acids on dermatitis in NC/Nga mice. Prostaglandins Leukot. Essent. Fatty Acids 66 (4), 435–440. doi: 10.1054/plef.2002.0370
Svajger, U., Obermajer, N., Jeras, M. (2010). Dendritic cells treated with resveratrol during differentiation from monocytes gain substantial tolerogenic properties upon activation. Immunology 129 (4), 525–535. doi: 10.1111/j.1365-2567.2009.03205.x
Tabak, C., Smit, H. A., Räsänen, L., Fidanza, F., Menotti, A., Nissinen, A., et al. (1999). Dietary factors and pulmonary function: a cross sectional study in middle aged men from three European countries. Thorax 54 (11), 1021–1026. doi: 10.1136/thx.54.11.1021
Tan, P. H., Sagoo, P., Chan, C., Yates, J. B., Campbell, J., Beutelspacher, S. C., et al. (2005). Inhibition of NF-kappa B and oxidative pathways in human dendritic cells by antioxidative vitamins generates regulatory T cells. J. Immunol. 174 (12), 7633–7644. doi: 10.4049/jimmunol.174.12.7633
Tanaka, T., Shen, J., Abecasis, G., Kisialiou, A., Ordovas, J., Guralnik, J., et al. (2009). Genome-wide association study of plasma polyunsaturated fatty acids in the InCHIANTI study. PloS Genet. 5 (1), e1000338. doi: 10.1371/journal.pgen.1000338
Thien, F. C., Mencia-Huerta, J. M., Lee, T. H. (1993). Dietary fish oil effects on seasonal hay fever and asthma in pollen-sensitive subjects. Am. Rev. Respir. Dis. 147 (5), 1138–1143. doi: 10.1164/ajrccm/147.5.1138
Töröcsik, D., Weise, C., Gericke, J., Szegedi, A., Lucas, R., Mihály, J., et al. (2019). Transcriptomic and lipidomic profiling of eicosanoid/docosanoid signalling in affected and non-affected skin of human atopic dermatitis patients. Exp. Dermatol. 28 (2), 177–189. doi: 10.1111/exd.13867
Traber, M. G. (2007). Vitamin E regulatory mechanisms. Annu. Rev. Nutr. 27, 347–362. doi: 10.1146/annurev.nutr.27.061406.093819
Troisi, R. J., Willett, W. C., Weiss, S. T., Trichopoulos, D., Rosner, B., Speizer, F. E. (1995). A prospective study of diet and adult-onset asthma. Am. J. Respir. Crit. Care Med. 151 (5), 1401–1408. doi: 10.1164/ajrccm.151.5.7735592
Tsuduki, T., Kuriyama, K., Nakagawa, K., Miyazawa, T. (2013). Tocotrienol (unsaturated vitamin E) suppresses degranulation of mast cells and reduces allergic dermatitis in mice. J. Oleo Sci. 62 (10), 825–834. doi: 10.5650/jos.62.825
Tuokkola, J., Luukkainen, P., Kaila, M., Takkinen, H. M., Niinisto, S., Veijola, R., et al. (2016). Maternal dietary folate, folic acid and vitamin D intakes during pregnancy and lactation and the risk of cows’ milk allergy in the offspring. Br. J. Nutr. 116 (4), 710–718. doi: 10.1017/S0007114516002464
van den Elsen, L., Garssen, J., Willemsen, L. (2012). Long chain N-3 polyunsaturated fatty acids in the prevention of allergic and cardiovascular disease. Curr. Pharm. Des. 18 (16), 2375–2392. doi: 10.2174/138161212800165960
van den Elsen, L. W., Nusse, Y., Balvers, M., Redegeld, F., Knol, E., Garssen, J., et al. (2013a). n-3 Long-chain PUFA reduce allergy-related mediator release by human mast cells in vitro via inhibition of reactive oxygen species. Brit. J. Nutr. 109, 1–11. doi: 10.1017/S0007114512003959
van den Elsen, L. W., Meulenbroek, L. A., van Esch, B. C., Hofman, G. A., Boon, L., Garssen, J., et al. (2013b). CD25+ regulatory T cells transfer n-3 long chain polyunsaturated fatty acids-induced tolerance in mice allergic to cow’s milk protein. Allergy 68 (12), 1562–1570. doi: 10.1111/all.12300
van den Elsen, L. W., van Esch, B. C., Hofman, G. A., Kant, J., van de Heijning, B. J., Garssen, J., et al. (2013c). Dietary long chain n-3 polyunsaturated fatty acids prevent allergic sensitization to cow’s milk protein in mice. Clin. Exp. Allergy 43 (7), 798–810. doi: 10.1111/cea.12111
van den Elsen, L. W., Bol-Schoenmakers, M., van Esch, B. C., Hofman, G. A., van de Heijning, B. J., Pieters, R. H., et al. (2014). DHA-rich tuna oil effectively suppresses allergic symptoms in mice allergic to whey or peanut. J. Nutr. 144 (12), 1970–1976. doi: 10.3945/jn.114.198515
Van Dyken, S., Nussbaum, J., Lee, J., Molofsky, A., Liang, H., Pollack, J., et al. (2016). A tissue checkpoint regulates type 2 immunity. Nat. Immunol. 17 (12), 1381–1387. doi: 10.1038/ni.3582
van Etten, E., Mathieu, C. (2005). Immunoregulation by 1,25-dihydroxyvitamin D3: basic concepts. J. Steroid Biochem. Mol. Biol. 97 (1-2), 93–101. doi: 10.1016/j.jsbmb.2005.06.002
Vasiliou, J. E., Lui, S., Walker, S. A., Chohan, V., Xystrakis, E., Bush, A., et al. (2014). Vitamin D deficiency induces Th2 skewing and eosinophilia in neonatal allergic airways disease. Allergy 69 (10), 1380–1389. doi: 10.1111/all.12465
Vassallo, M. F., Camargo, C. A., Jr. (2010). Potential mechanisms for the hypothesized link between sunshine, vitamin D, and food allergy in children. J. Allergy Clin. Immunol. 126 (2), 217–222. doi: 10.1016/j.jaci.2010.06.011
Venter, C., Meyer, R., Nwaru, B., Roduit, C., Untersmayr, E., Adel-Patient, K., et al. (2019). EAACI position paper: Influence of dietary fatty acids on asthma, food allergy, and atopic dermatitis. Allergy 74 (8), 1429–1444. doi: 10.1111/all.13764
Verbeek, R., Plomp, A. C., van Tol, E. A., van Noort, J. M. (2004). The flavones luteolin and apigenin inhibit in vitro antigen-specific proliferation and interferon-gamma production by murine and human autoimmune T cells. Biochem. Pharmacol. 68 (4), 621–629. doi: 10.1016/j.bcp.2004.05.012
Verdura, S., Cuyas, E., Cortada, E., Brunet, J., Lopez-Bonet, E., Martin-Castillo, B., et al. (2020). Resveratrol targets PD-L1 glycosylation and dimerization to enhance antitumor T-cell immunity. Aging (Albany NY) 12 (1), 8–34. doi: 10.18632/aging.102646
Wagner, J., Jiang, Q., Harkema, J., Ames, B., Illek, B., Roubey, R., et al. (2008). Gamma-tocopherol prevents airway eosinophilia and mucous cell hyperplasia in experimentally induced allergic rhinitis and asthma. Clin. Exp. Allergy 38 (3), 501–511. doi: 10.1111/j.1365-2222.2007.02855.x
Wang, H., Hao, Q., Li, Q. R., Yan, X. W., Ye, S., Li, Y. S., et al. (2007). Omega-3 polyunsaturated fatty acids affect lipopolysaccharide-induced maturation of dendritic cells through mitogen-activated protein kinases p38. Nutrition 23 (6), 474–482. doi: 10.1016/j.nut.2007.04.002
Wang, B., Sun, J., Li, X., Zhou, Q., Bai, J., Shi, Y., et al. (2013). Resveratrol prevents suppression of regulatory T-cell production, oxidative stress, and inflammation of mice prone or resistant to high-fat diet-induced obesity. Nutr. Res. 33 (11), 971–981. doi: 10.1016/j.nutres.2013.07.016
Wang, B., Sun, J., Ma, Y., Wu, G., Tian, Y., Shi, Y., et al. (2014). Resveratrol preserves mitochondrial function, stimulates mitochondrial biogenesis, and attenuates oxidative stress in regulatory T cells of mice fed a high-fat diet. J. Food Sci. 79 (9), H1823–H1831. doi: 10.1111/1750-3841.12555
Wang, X., Ma, D., Kang, J., Kulka, M. (2015). n-3 Polyunsaturated fatty acids inhibit Fc ϵ receptor I-mediated mast cell activation. J. Nutr. Biochem. 26 (12), 1580–1588. doi: 10.1016/j.jnutbio.2015.07.027
Wang, Z., Zhang, H., Sun, X., Ren, L. (2016). The protective role of vitamin D3 in a murine model of asthma via the suppression of TGF-beta/Smad signaling and activation of the Nrf2/HO-1 pathway. Mol. Med. Rep. 14 (3), 2389–2396. doi: 10.3892/mmr.2016.5563
Weise, C., Hilt, K., Milovanovic, M., Ernst, D., Rühl, R., Worm, M. (2011). Inhibition of IgE production by docosahexaenoic acid is mediated by direct interference with STAT6 and NFκB pathway in human B cells. J. Nutr. Biochem. 22, 269–275. doi: 10.1016/j.jnutbio.2010.02.004
Weiss, S. T. (1997). Diet as a risk factor for asthma. Ciba Found. Symp. 206, 244–257: discussion 253-247. doi: 10.1016/j.jaci.2004.12.1139
Weng, Z., Patel, A., Panagiotidou, S., Theoharides, T. (2015). The novel flavone tetramethoxyluteolin is a potent inhibitor of human mast cells. J. Allergy Clin. Immunol. 135 (4), 1044–1052. doi: 10.1016/j.jaci.2014.10.032
White, W. S., Zhou, Y., Crane, A., Dixon, P., Quadt, F., Flendrig, L. M. (2017). Modeling the dose effects of soybean oil in salad dressing on carotenoid and fat-soluble vitamin bioavailability in salad vegetables. Am. J. Clin. Nutr. 106 (4), 1041–1051. doi: 10.3945/ajcn.117.153635
Wijga, A. H., van Houwelingen, A. C., Kerkhof, M., Tabak, C., de Jongste, J. C., Gerritsen, J., et al. (2006). Breast milk fatty acids and allergic disease in preschool children: the Prevention and Incidence of Asthma and Mite Allergy birth cohort study. J. Allergy Clin. Immunol. 117 (2), 440–447. doi: 10.1016/j.jaci.2005.10.022
Williams, N. C., Hunter, K. A., Shaw, D. E., Jackson, K. G., Sharpe, G. R., Johnson, M. A. (2017). Comparable reductions in hyperpnoea-induced bronchoconstriction and markers of airway inflammation after supplementation with 6.2 and 3.1 g/d of long-chain n-3 PUFA in adults with asthma. Br. J. Nutr. 117 (10), 1379–1389. doi: 10.1017/S0007114517001246
Wolf, G. (2006). How an increased intake of alpha-tocopherol can suppress the bioavailability of gamma-tocopherol. Nutr. Rev. 64 (6), 295–299. doi: 10.1111/j.1753-4887.2006.tb00213.x
Wolpowitz, D., Gilchrest, B. (2006). The vitamin D questions: how much do you need and how should you get it? J. Am. Acad. Dermatol. 54 (2), 301–317. doi: 10.1016/j.jaad.2005.11.1057
Wood, L. G., Gibson, P. G. (2010). Reduced circulating antioxidant defences are associated with airway hyper-responsiveness, poor control and severe disease pattern in asthma. Br. J. Nutr. 103 (5), 735–741. doi: 10.1017/S0007114509992376
Wood, L. G., Garg, M. L., Smart, J. M., Scott, H. A., Barker, D., Gibson, P. G. (2012). Manipulating antioxidant intake in asthma: a randomized controlled trial. Am. J. Clin. Nutr. 96 (3), 534–543. doi: 10.3945/ajcn.111.032623
Woods, R. K., Raven, J. M., Walters, E. H., Abramson, M. J., Thien, F. C. (2004). Fatty acid levels and risk of asthma in young adults. Thorax 59 (2), 105–110. doi: 10.1136/thorax.2003.009498
Wu, J., Zhang, Y., Liu, Q., Zhong, W., Xia, Z. (2013). All-trans retinoic acid attenuates airway inflammation by inhibiting Th2 and Th17 response in experimental allergic asthma. BMC Immunol. 14, 28. doi: 10.1186/1471-2172-14-28
Wu, H., Zhang, C., Wang, Y., Li, Y. (2018). Does vitamin E prevent asthma or wheeze in children: A systematic review and meta-analysis. Paediatr. Respir. Rev. 27, 60–68. doi: 10.1016/j.prrv.2017.08.002
Xiao, S., Jin, H., Korn, T., Liu, S. M., Oukka, M., Lim, B., et al. (2008). Retinoic acid increases Foxp3+ regulatory T cells and inhibits development of Th17 cells by enhancing TGF-beta-driven Smad3 signaling and inhibiting IL-6 and IL-23 receptor expression. J. Immunol. 181 (4), 2277–2284. doi: 10.4049/jimmunol.181.4.2277
Xuan, N. T., Trang, P. T., Van Phong, N., Toan, N. L., Trung, D. M., Bac, N. D., et al. (2016). Klotho sensitive regulation of dendritic cell functions by vitamin E. Biol. Res. 49 (1), 45. doi: 10.1186/s40659-016-0105-4
Yamamoto, K., Itoh, T., Abe, D., Shimizu, M., Kanda, T., Koyama, T., et al. (2005). Identification of putative metabolites of docosahexaenoic acid as potent PPARgamma agonists and antidiabetic agents. Bioorg. Med. Chem. Lett. 15 (3), 517–522. doi: 10.1016/j.bmcl.2004.11.053
Ye, S., Liu, H., Chen, Y., Qiu, F., Liang, C. L., Zhang, Q., et al. (2019). A Novel Immunosuppressant, Luteolin, Modulates Alloimmunity and Suppresses Murine Allograft Rejection. J. Immunol. 203 (12), 3436–3446. doi: 10.4049/jimmunol.1900612
Yeum, K. J., Song, B. C., Joo, N. S. (2016). Impact of Geographic Location on Vitamin D Status and Bone Mineral Density. Int. J. Environ. Res. Public Health 13 (2), 184. doi: 10.3390/ijerph13020184
Yin, H., Liu, W., Goleniewska, K., Porter, N. A., Morrow, J. D., Peebles, R. S., Jr. (2009). Dietary supplementation of omega-3 fatty acid-containing fish oil suppresses F2-isoprostanes but enhances inflammatory cytokine response in a mouse model of ovalbumin-induced allergic lung inflammation. Free Radic. Biol. Med. 47 (5), 622–628. doi: 10.1016/j.freeradbiomed.2009.05.033
Yip, K., Kolesnikoff, N., Yu, C., Hauschild, N., Taing, H., Biggs, L., et al. (2014). Mechanisms of vitamin D3metabolite repressionof IgE-dependent mast cell activation. J. Allergy Clin. Immunol. 133 (5), 1356–1364. doi: 10.1016/j.jaci.2013.11.030
Yokota-Nakatsuma, A., Takeuchi, H., Ohoka, Y., Kato, C., Song, S. Y., Hoshino, T., et al. (2014). Retinoic acid prevents mesenteric lymph node dendritic cells from inducing IL-13-producing inflammatory Th2 cells. Mucosal. Immunol. 7 (4), 786–801. doi: 10.1038/mi.2013.96
Yoshida, S., Yasutomo, K., Watanabe, T. (2016). Treatment with DHA/EPA ameliorates atopic dermatitis-like skin disease by blocking LTB4 production. J. Med. Invest. 63 (3-4), 187–191. doi: 10.2152/jmi.63.187
Zanatta, A. L., Miranda, D. T., Dias, B. C., Campos, R. M., Massaro, M. C., Michelotto, P. V., Jr., et al. (2014). Fish oil supplementation decreases oxidative stress but does not affect platelet-activating factor bioactivity in lungs of asthmatic rats. Lipids 49 (7), 665–675. doi: 10.1007/s11745-014-3914-7
Zeyda, M., Säemann, M. D., Stuhlmeier, K. M., Mascher, D. G., Nowotny, P. N., Zlabinger, G. J., et al. (2005). Polyunsaturated fatty acids block dendritic cell activation and function independently of NF-kappaB activation. J. Biol. Chem. 280 (14), 14293–14301. doi: 10.1074/jbc.M410000200
Zhang, Y. F., Liu, Q. M., Gao, Y. Y., Liu, B., Liu, H., Cao, M. J., et al. (2019). Attenuation of allergic responses following treatment with resveratrol in anaphylactic models and IgE-mediated mast cells. Food Funct. 10 (4), 2030–2039. doi: 10.1039/c9fo00077a
Zhao, J., Lloyd, C. M., Noble, A. (2013). Th17 responses in chronic allergic airway inflammation abrogate regulatory T-cell-mediated tolerance and contribute to airway remodeling. Mucosal. Immunol. 6 (2), 335–346. doi: 10.1038/mi.2012.76
Zingg, J. M. (2015). Vitamin E: A Role in Signal Transduction. Annu. Rev. Nutr. 35, 135–173. doi: 10.1146/annurev-nutr-071714-034347
Zosky, G. R., Berry, L. J., Elliot, J. G., James, A. L., Gorman, S., Hart, P. H. (2011). Vitamin D deficiency causes deficits in lung function and alters lung structure. Am. J. Respir. Crit. Care Med. 183 (10), 1336–1343. doi: 10.1164/rccm.201010-1596OC
Zou, T., Yang, Y., Xia, F., Huang, A., Gao, X., Fang, D., et al. (2013). Resveratrol Inhibits CD4+ T cell activation by enhancing the expression and activity of Sirt1. PloS One 8 (9), e75139. doi: 10.1371/journal.pone.0075139
Glossary
Keywords: n-3 long chain poly-unsaturated fatty acids, fat-soluble micronutrients, vitamins, polyphenols, allergy
Citation: Hogenkamp A, Ehlers A, Garssen J and Willemsen LEM (2020) Allergy Modulation by N-3 Long Chain Polyunsaturated Fatty Acids and Fat Soluble Nutrients of the Mediterranean Diet. Front. Pharmacol. 11:1244. doi: 10.3389/fphar.2020.01244
Received: 10 April 2020; Accepted: 29 July 2020;
Published: 21 August 2020.
Edited by:
Gerard Bannenberg, Global Organization for EPA and DHA Omega-3s (GOED), United StatesReviewed by:
Cristina López-Vicario, Hospital Clínic de Barcelona, SpainJelena Radosavljević, University of Belgrade, Serbia
Berber Vlieg-Boerstra, Onze Lieve Vrouwe Gasthuis (OLVG), Netherlands
Copyright © 2020 Hogenkamp, Ehlers, Garssen and Willemsen. This is an open-access article distributed under the terms of the Creative Commons Attribution License (CC BY). The use, distribution or reproduction in other forums is permitted, provided the original author(s) and the copyright owner(s) are credited and that the original publication in this journal is cited, in accordance with accepted academic practice. No use, distribution or reproduction is permitted which does not comply with these terms.
*Correspondence: Linette Willemsen, l.e.m.willemsen@uu.nl