- 1Adelaide Medical School, University of Adelaide, Adelaide, SA, Australia
- 2College of Science and Engineering, Flinders University, Adelaide, SA, Australia
The highly invasive nature of glioblastoma imposes poor prospects for patient survival. Molecular evidence indicates glioblastoma cells undergo an intriguing expansion of phenotypic properties to include neuron-like signaling using excitable membrane ion channels and synaptic proteins, augmenting survival and motility. Neurotransmitter receptors, membrane signaling, excitatory receptors, and Ca2+ responses are important candidates for the design of customized treatments for cancers within the heterogeneous central nervous system. Relatively few published studies of glioblastoma multiforme (GBM) have evaluated pharmacological agents targeted to signaling pathways in limiting cancer cell motility. Transcriptomic analyses here identified classes of ion channels, ionotropic receptors, and synaptic proteins that are enriched in human glioblastoma biopsy samples. The pattern of GBM-enriched gene expression points to a major role for glutamate signaling. However, the predominant role of AMPA receptors in fast excitatory signaling throughout the central nervous system raises a challenge on how to target inhibitors selectively to cancer cells while maintaining tolerability. This review critically evaluates a panel of ligand- and voltage-gated ion channels and synaptic proteins upregulated in GBM, and the evidence for their potential roles in the pathological disease progress. Evidence suggests combinations of therapies could be more effective than single agents alone. Natural plant products used in traditional medicines for the treatment of glioblastoma contain flavonoids, terpenoids, polyphenols, epigallocatechin gallate, quinones, and saponins, which might serendipitously include agents that modulate some classes of signaling compounds highlighted in this review. New therapeutic strategies are likely to exploit evidence-based combinations of selected agents, each at a low dose, to create new cancer cell-specific therapeutics.
Glioblastoma Invasiveness Severely Limits Patient Survival
The prospects for survival of patients diagnosed with glioblastoma are poor, even with the most powerful therapies, largely because of the highly invasive nature of the cancer. After diagnosis, average survival expectancy ranges from a few months to less than 2 years, depending on cancer subtype and treatment strategy (Stupp et al., 2005). As reviewed recently, glioblastoma is divided into primary GBM, constituting 80% of cases with a mean age of onset at 62 years, and secondary GBM progressing from lower grade astrocytomas or oligodendrogliomas with a mean age of onset of 45 years (Ghosh et al., 2018). Glioblastoma multiforme is designated as Grade IV by the World Health Organization, the most malignant type of brain tumor. Molecular markers that have been defined for GBM include DNA repair and methylation enzymes, epidermal growth factor receptor, proto-oncogenes, and tumor suppressor genes (Ghosh et al., 2018). Current treatments for GBM depend on a combination of surgical resection, radiation therapy and chemotherapy, but even with this multi-pronged approach, median survival time is only 14.6 months (Ghosh et al., 2018).
In historical records, the first intracranial procedure in 1884 to remove a glial tumor was attributed to Bennett and Godlee (Walker et al., 1976). Surgical resection can remove most of a tumor bulk, but the problem is that a subset of GBM cells at the time of treatment will have already spread a substantial distance beyond the visible boundary of the main mass. Postmortem analyses of sectioned whole brains from untreated GBM patients revealed that in none of the 15 cases studied did the computer-tomography defined area capture the full extent of the neoplasm, and in three cases, infiltration extended 3.5 to 5 cm beyond the observed electron-dense boundaries of the tumors (Burger et al., 1988). Conventional radiological evaluations frequently underestimate the full area over which an invasive glioma has extended (Claes et al., 2007). In the late 1970s, addition of radiotherapy after surgery was shown to further improve GBM patient survival, and has since been refined progressively to optimize focused applications (Mann et al., 2017). Radiation damage to DNA acts to decrease cell proliferation, but falls short of being curative as a result of the invasive nature of GBM, as well as radiation-induced necrosis, neuronal damage, and the insensitivity of some GBM subtypes to radiation treatment. Adjuvant chemotherapy was found to further improve survival. In 2005, a Phase 3 clinical trial conducted by the European Organization for Research and Treatment of Cancer (EORTC) 26981-22981/National Cancer Institute of Canada Clinical Trials Group (NCIC CTG) showed significantly better overall survival when patients were treated with temozolomide in combination with radiotherapy and maximal surgical resection (Stupp et al., 2005). However, recurrence remains a serious problem, as GBM cells escape resection by early migration, and show inherent or acquired resistance to chemo- and radio therapy treatments (Ghosh et al., 2018). More work is needed to understand the mechanisms of motility, to define new intervention strategies for this devastating disease, and potentially to aim treatments to specific subtypes of GBM.
GBM arises throughout the brain most frequently in the cerebral hemispheres, particularly in frontal lobes or both frontal and temporal lobes, whereas it is relatively rare in cerebellum (Iacob and Dinca, 2009). Categories of glioblastoma traditionally were defined based on histology; recently a systematic correlation of phenotypes with molecular markers identified diagnostic indicators of the major subtypes (Louis et al., 2016; Verhaak, 2016). The molecular markers themselves are not necessarily key drivers of glioblastoma pathology, but their usefulness in defining subtypes is expected to allow tailoring of treatment aggressiveness to optimize patient outcomes (Verhaak, 2016). Frequently altered pathways in cancers involve tyrosine and serine/threonine kinases and relevant receptors, including the epidermal growth factor receptor (EGFR), which alter the regulation of Ras oncogenes overexpressed in glioblastoma (Cancer Genome Atlas Research, 2008). Downstream cascades of kinases include the mitogen-activated protein kinase family that are associated with an array of outcomes including proliferation, invasion, and chemotherapy resistance. We suggest that additional contributions from neurotransmitters, membrane signaling, excitatory receptors, and Ca2+ responses could be similarly important, and might offer opportunities for customized treatment of cancer cells within the heterogeneous cell populations of the central nervous system by combinations of agents at subtoxic doses.
Limiting cell migration is a compelling goal in the broad cancer field. Tools for managing the invasive spread of cancer cells in general through the body are essential, given that metastasis is a major cause of cancer-related deaths (Welch and Hurst, 2019). In GBM, the cancer rarely metastasizes outside the central nervous system, yet within the brain the highly invasive properties of these cells constitute a major challenge for effective treatment. Controlling GBM spread using inhibitors of cell migration and invasion would constitute a powerful adjunct therapy that could be applied in parallel with other procedures aimed at direct eradication of primary tumors. This review critically evaluates classes of ligand- and voltage ion channels and synaptic proteins as new targets for experimental therapies in GBM, and integrates cross-disciplinary evidence for their potential roles in the pathological features of glioblastoma.
Pharmacological Targets for Cancer Treatment
The majority of published work in the drug discovery field for cancers including GBM has focused on cell death and reduced proliferation as the primary outcomes of interest for therapeutics. While these candidate agents would have desired effects of killing cancer cells, non-selective toxicity, side-effects, and efficacy in vivo remain constraints on effective drug development. Alkylating agents that modify DNA structure have been shown to improve patient survival by driving apoptosis of the cancer cells, but concurrent activity on non-cancerous cells creates side effects which limit tolerable doses. Alkylating agents for GBM include chloroethylating drugs such as carmustine and lomustine, and methylating agents such as temozolomide which, due to its comparatively lower toxicity, has in combination with radiotherapy become a standard of care for GBM patients in countries that can afford the high cost of this chemotherapeutic agent (Lonardi et al., 2005). A second tier of cytotoxic agents for non-responsive GBM cases includes carboplatin, etoposide, oxaliplatin, and irinotecan. These agents also alter DNA to reduce cell proliferation, with greatest effects exerted on populations of rapidly dividing cells such as cancers. Anti-angiogenic agents and antibodies against EGFR and other tyrosine kinase receptors also have been of interest for new experimental chemotherapy strategies (Iacob and Dinca, 2009). A major gap in knowledge in this field is how to constrain GBM cell motility while treatments of the primary tumor masses are in progress, preventing the escape that leads to recurrence. As summarized in this review, the discovery of pharmacological tools to intervene in processes of cell migration and invasion in GBM is a promising area of work, possibly utilizing traditional medicinal herbs as one source of novel agents, but this area remains largely unexplored to date. As an indicative survey, of more than 38,000 papers listed in the National Institutes of Health PubMed database that were identified as relevant to glioblastoma as of Nov 2019, 18% identified use of an inhibitor, 26% were linked to proliferation, and 11% considered effects on motility (Figure 1). Less than 4% of published studies in glioblastoma evaluated candidate therapeutics as tools for limiting cancer cell motility. Approximately 2% of the papers published evaluated the effects of inhibitors on both cell survival and motility.
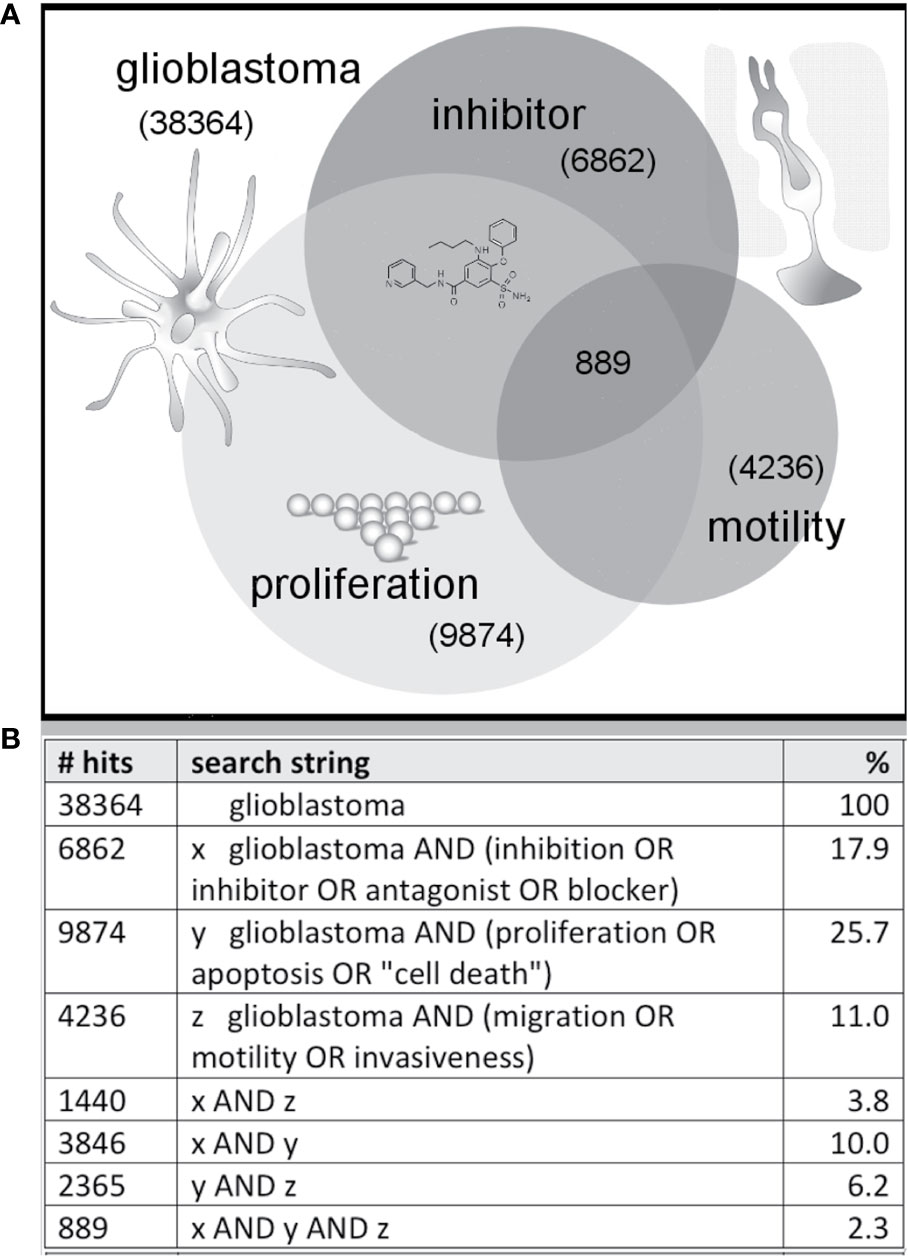
Figure 1 Illustration of a sample distribution of published glioblastoma studies suggesting less than 3% combine three themes (inhibitor, proliferation, motility). Venn diagram (A) summarizing the numbers of published articles on glioblastoma, with key words linked to inhibition, migration and growth, as of November 2019 based on a search of the NIH PubMed database, with search strings as defined in the table (B).
Evidence suggests combinations of therapies could be more effective than single agents alone. For example, a Chinese traditional medicinal extract known as Compound Kushing Injection has been fractionated, chemically defined, and evaluated for dose-dependent activities in a broad array of cancer cell types measured by changes in rates of migration, cell survival, proliferation, and gene expression (Nourmohammadi et al., 2019). The interesting conclusions drawn in this work were that no single compound in the Compound Kushing Injection mixture accounted for the full therapeutic effect of the whole extract; combinations of agents were required to reconstitute the beneficial activity, and the most effective combinations of compounds depended on the cancer cell type. Combined block of glioma cells with 5-nitro-2-(3-phenylpropylamino)-benzoate (a non-specific chloride channel blocker), covalently linked to temozolomide (an oral chemotherapeutic drug for glioblastoma) suppressed glioma cell proliferation, migration, and invasion (Park et al., 2016). Temozolomide modifies DNA or RNA at guanine and adenine bases by addition of methyl groups (Lee, 2016); however, a substantial proportion of tumors are insensitive to temozolamide due to expression of methyltransferase genes.
Limitations of current therapies highlight the need for new drugs that induce fewer side effects and can lead to a more favorable prognosis. While the combination of radiotherapy and temozolomide is a principal treatment strategy, a rising concern is that radiation exposure at therapeutic levels can alter gene expression and signaling to promote a highly invasive phenotype in the surviving glioblastoma cells (Birch et al., 2018). Promising results have indicated that a blocker of a key kinase (which regulates cytoskeletal contractility at the leading edges of glioblastoma tumors) could be co-administered to suppress the recurrence of the invasive cells after radiotherapy, with potential promise for extending patient survival time and reducing the neurological impairment associated with the disease (Birch et al., 2018). A major active ingredient of the herbal medicinal plant ginseng (ginsenoside Rg3), has additive anti-tumor and anti-angiogenic effects when applied in combination with temozolomide (Sun et al., 2016b). Epigallocatechin gallate from green tea synergizes with temozolomide to inhibit viability, migration, and drug resistance of glioma stem-like cells isolated from the U87 line (Zhang et al., 2015). The anti-invasive activity of a medicinal herbal extract from olive (Olea) leaf on glioblastoma stem cells derived from human primary tumors was increased when given in combination with bevacuazimab (Tezcan et al., 2017a). Further work seems likely to demonstrate that the most effective clinical strategies will include co-administration of multiple agents, targeted to subtype-specific panels of factors in glioblastoma, to enhance the successful outcomes of interventions.
Mechanisms Enabling Glioblastomal Progression
High motility, heterogeneity of cell types within the cancer clusters, as well as the ability of the glioblastoma cells to transition between proliferative and non-proliferative phases make these cancers difficult to treat, and complicate the accurate diagnoses of tumor types based on small biopsies (Scherer, 1940; Singh et al., 2004; Behnan et al., 2019). Migration pathways of glioblastoma through the human brain, described by Scherer (1940) and confirmed in rodents with human tumor cell implants (Laws et al., 1993), suggest a predominant pattern of reliance on myelinated tracts such as corpus callosum, basement membranes of blood vessels, pial surfaces, and subependymal layers surrounding the ventricles as routes for invasion. Insights from Scherer's work further indicated that interactions with the brain tissue environment were an important part of glioblastoma progression, a concept highly relevant to current discoveries.
Surprising capacities for membrane signaling in glioblastoma are reminiscent of properties characteristic of excitable cells, and appear to be important for disease progression (Soroceanu et al., 1999; Catacuzzeno et al., 2011; Molenaar, 2011; Wong et al., 2017; Venkataramani et al., 2019; Venkatesh et al., 2019). Although glioblastoma cells have been thought to arise from glia in the CNS, molecular evidence indicates they originate from stem cell precursors (Emlet et al., 2014), and undergo an intriguing expansion of phenotypic properties to include neuron-like signaling, which has been proposed to augment glioblastoma cell survival and motility (Venkataramani et al., 2019). As analyzed here, a select set of excitable membrane ion channels and synaptic proteins (usually assumed to be the province of cells such as neurons, not glia) are upregulated in glioblastoma. This unusual portfolio of signaling proteins could endow glioblastoma cells with functional capabilities that aid their robust persistence and merit investigation as targets for new therapeutics, though the signaling protein contributions to the underlying pathological mechanisms are yet to be fully defined.
Glutamate, the main excitatory neurotransmitter in the central nervous system, in early brain development promotes the proliferation of neuronal progenitor cells, but interestingly also has been found to enhance proliferation and migration in glioblastoma cells (Lyons et al., 2007; Corsi et al., 2019). Glutamate responses associated with glioblastoma are more than excitotoxicity mechanisms to kill neurons and create space for tumor growth (Sontheimer, 2008); the process is an integral part of bidirectional communication between neurons and glioblastoma cell networks (Robert and Sontheimer, 2014; Ribeiro et al., 2017; Corsi et al., 2019). Excitation of AMPA-activated classes of ionotropic glutamate receptors (GluA1 to 4) augments the cell migration and proliferation of human glioblastoma cells (Ishiuchi et al., 2002), mediated in part by Ca2+-dependent activation of a survival factor Akt kinase, as confirmed in surgical samples and in glioblastoma cell lines (Ishiuchi et al., 2007). Pathological tumor cells are not unique among non-neuronal brain cells in being responsive to glutamate; immature glia also show sensitivity. In oligodendrocyte precursor cells, functional synapses marked by fast depolarizing currents were measured in response to voltage- and calcium-dependent release of glutamate from pyramidal neurons in hippocampal slice preparations (Bergles et al., 2000). During oligodendrocyte maturation, signaling from axons is proposed to coordinate the process of myelination. In human glioblastoma, synapses from neurons onto glioblastoma cell processes were observed by electron microscopy in resected tumor samples, complete with hallmark structures including presynaptic docked vesicles, synaptic clefts, and postsynaptic densities carrying AMPA receptors, confirmed by molecular markers and electrophysiological recordings (Venkataramani et al., 2019). Prior work had showed glutamate-induced Ca2+ signals and suggested the occurrence of action potentials in some dissociated cells cultured from glioma samples (Labrakakis et al., 1998), though cell types in the mixed sample were not precisely identified. Postsynaptic responses characterized in identified glioblastoma depend directly on the activity of surrounding neurons and seem focused on increasing intracellular Ca2+; no action potentials were observed in more than 300 tumor cells recorded (Venkataramani et al., 2019). Signals driven by synaptic input spread through the gap-junction linked network of glioma cells to promote proliferation, and reciprocally drive hyperexcitability of neurons in the glioma microenvironment, further boosting glioblastoma synaptic input and amplifying the problem (Venkatesh et al., 2019). We propose that analysis of a transcriptomic database with a focus on classes of ion channels, ionotropic receptors, and synaptic proteins that are enriched in human glioblastoma biopsy samples, offers a timely opportunity to generate predictive hypotheses for further testing the possible functional roles of these signaling proteins in glioblastoma progression.
Quantitative data on transcript levels measured from biopsied samples from human glioblastoma multiforme (GBM) patients, available in the Human Protein Atlas, were queried here to compare the patterns of gene expression of an array of excitable membrane signaling proteins for GBM patients with the likelihood of their survival of at 3 years after diagnosis (Uhlen et al., 2005; Uhlen et al., 2017). Table 1 provides a summary of compiled data for the classes of ionotropic receptors, ion channels, and synaptic proteins that showed distinct upregulation in measured transcript levels in GBM patients. Transcriptomic data were based on analyses of biopsied samples from 153 patients diagnosed with GBM (54 female and 99 male). At the time of the data collection, 123 patients were deceased. Transcript levels were quantified as ‘Fragments per kilobase of transcript per million fragments mapped' (FPKM). The percent survival at 3 years is calculated from deceased patients whose samples showed transcript levels for a given signaling protein greater than the median value across the GBM group; these values ranged from 3% to 14%, depending on the gene. Several genes were significant as negative prognostic indicators of survival when upregulated, including the small-conductance Ca2+-activated K channel (KCa3.1), synaptotagmin 5, gap junction channel connexin 26, and the Na+ proton exchanger NHE1 (though upregulation of this transporter was seen in multiple types of cancers, not selectively in GBM). Among the classes of ionotropic glutamate receptors enriched in GBM patients (Table 1), transcript levels were high for the AMPA-activated classes GluA1, GluA2, and GluA3, and the kainate-activated class GluK5. Ionotropic receptors for GABA and acetylcholine were enriched in GBM, but were not expressed at levels as high as those seen for glutamate receptors. Analyses of gene expression for the voltage-gated ion channel family showed expression of CaV and NaV channels, as well as multiple types of K+ channels. The highest levels of ion channel transcripts were seen for the inward rectifier K+ channel Kir4.1, and were appreciable as well for voltage-gated Kv4.2 and Kv7.2, and Ca2+-activated KCa2.3 channel classes. High levels of synaptic proteins were seen for synaptosome-associated protein SNAP25, synaptic vesicle glycoprotein, synapsin, synaptophysin, and synaptotagmins. Other channels with higher levels of expression in GBM include Aquaporins 1 and 4, the acid-sensing channel ASIC1, cyclic nucleotide gated channel CNG3, and the chloride channel ClC3. Though downregulation of expression of other genes in GBM also could contribute to pathological features of the disease, work here has focused on the genes showing upregulation of transcript levels, based on the proposed goal of finding potential therapeutic targets for novel inhibitory agents.
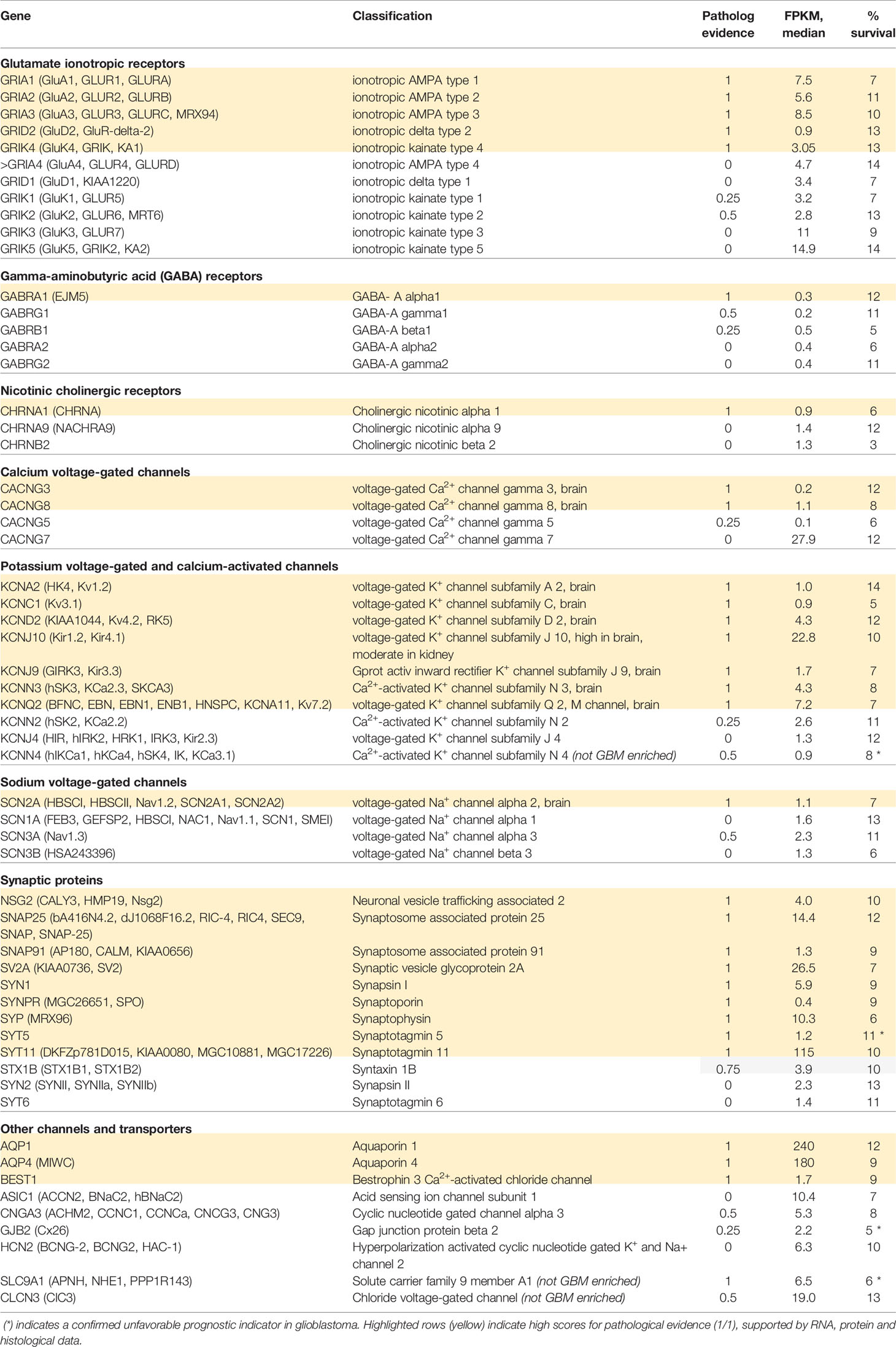
Table 1 Summary of ion channels and synaptic proteins showing enriched transcript levels (FPKM, fragments per kilobase of transcript per million fragments mapped) in human glioblastoma biopsy samples, analyzed using TGCA RNAseq (n=153; Human Protein Atlas database); and percent survival at 3 years for patients with FPKM values greater than median.
Transcriptomic Analyses of Ion Channel Genes Selectively Enriched in Glioblastoma
Transcriptomic data from RNAseq analysis of human glioblastoma samples (Human Protein Database) were further analyzed by banding data into pooled subsets based on patient survival duration, for intervals ranging from 0–3 months up to more than 3 years, and calculating the transcript levels (median FPKM values), depicted as a heat map (Figure 2). The negative prognostic indicators NHE1 and Cx26 as expected showed a general pattern in which GBM patients with the highest levels of transcript expression showed the shortest average survival times. A similar pattern might be suggested by data for the voltage gated Ca2+ channel, inward rectifier K+ channels, and Ca2+-activated K+ channel classes shown. No distinct correlation between survival and transcript level was evident in this analysis for AQP1 and ClC3 channels, both identified previously as targets of interest in pathological mechanisms of glioblastoma progression (McCoy and Sontheimer, 2007; Cuddapah and Sontheimer, 2010; El Hindy et al., 2013). However, this link between expression and survival is based on small biopsies of heterogeneous tumor masses, and does not rule out potential impacts for any of the channels or synaptic proteins that are enriched in GBM. Signaling molecules often have overlapping functions; thus, analyses that consider sets of related channels within a class, rather than a specific individual channel, might potentially be more meaningful predictors of survival outcomes (see further discussion and Figure 4 below).
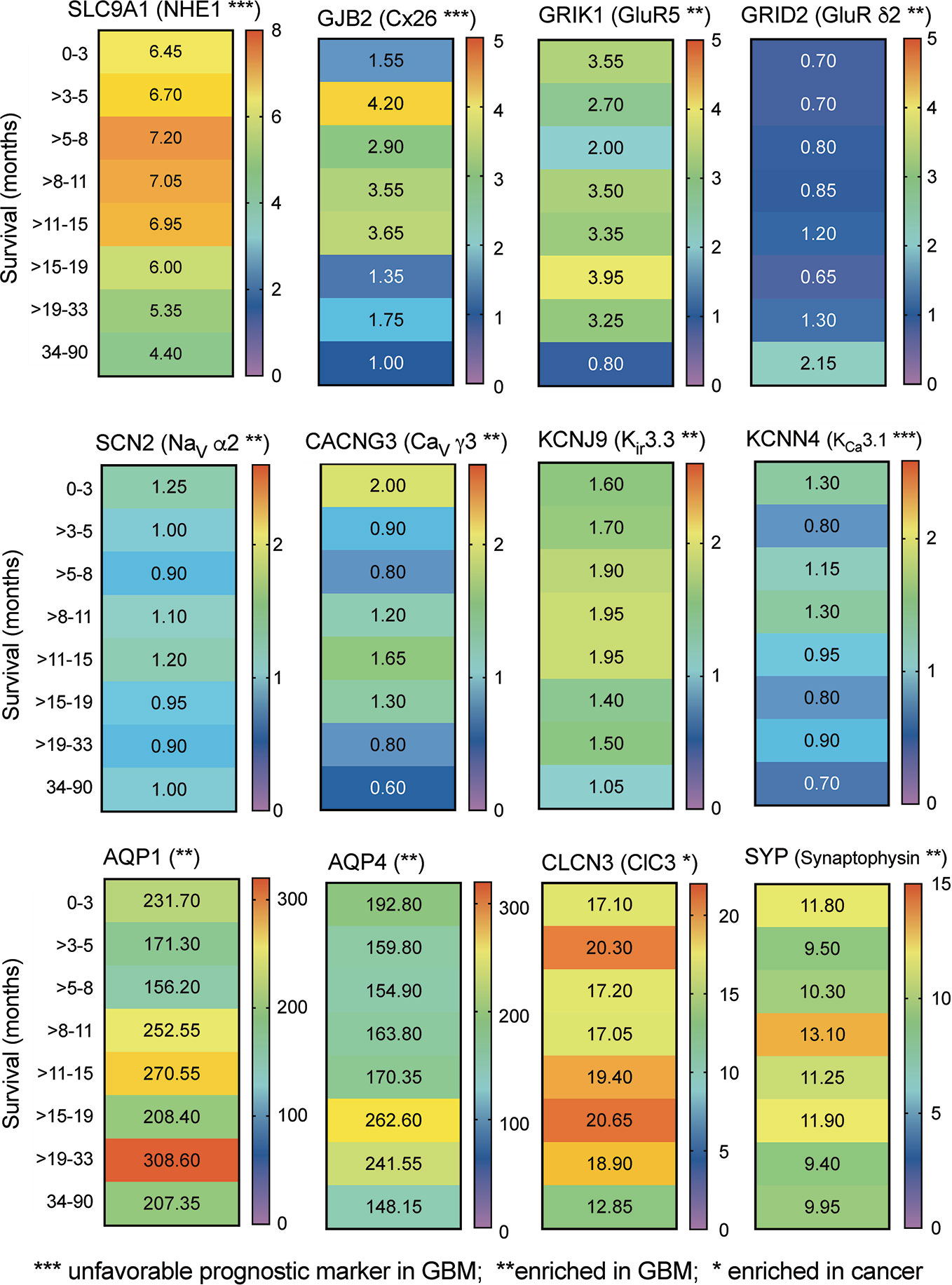
Figure 2 Correlation between relative expression levels of key genes and patient survival. Heat maps summarizing the quantitative median levels of transcripts (FPMK, color bars) for 12 genes, for results banded into bins based on durations of patient survival. Results were calculated from TCGA data available in the Human Protein Atlas. Asterisks indicate relative prognostic values: *** increased transcript expression is an unfavorable prognostic marker in GBM; ** transcript levels are selectively enriched in GBM; * transcript levels are enhanced in cancers.
Glutamate Receptors Enriched in Glioblastoma
Neurons in the central nervous system express diverse classes of direct ligand-gated ion channels that are activated by changes in membrane voltage, by the binding of intracellular ligands such as Ca2+ and cyclic nucleotides, by the binding of extracellular ligands such as neurotransmitters, or a combination. Glioblastoma cells express a subset of receptors and channels which are associated with excitable cells, and are of substantial interest for their potential roles in signaling pathways that promote the aggressive and lethal properties of the glioblastoma tumor. Of the direct ligand gated channels, the highest transcript levels were for glutamate receptor genes, with comparatively low levels seen for GABAA and nicotinic acetylcholine receptors (Table 1).
Glutamate is the major excitatory neurotransmitter in the brain and spinal cord, released from presynaptic terminals in response to an increase in intracellular Ca2+ mediated through voltage-gated Ca2+ channels, and detected at postsynaptic glutamate receptors, resulting in an excitatory change in membrane potential. As reviewed by Traynelis and colleagues (2010), direct ligand-gated glutamate receptors are cation-permeable channels built as tetramers of subunits, and have been subdivided by selective agonist sensitivities into the N-methyl-D-aspartate (NMDA) and the non-NMDA classes (AMPA, alpha-amino-3-hydroxy-5-methyl-4-isoxazolepropionic acid, and kainate), categories which align with genetic classifications based on amino acid sequence homology. Transcripts enriched in glioblastoma RNA seq data include receptors in the non-NMDA group activated by the agonist AMPA (GluA1 to -4) and by kainate (GluK1 to -5).
A key determinant of ionic permeability of AMPA and kainate receptors is the type of amino acid located in the second transmembrane domain. In GluA2, the residue is either glutamine (in the unedited form) yielding a receptor which conducts Ca2+ as well as monovalent cations, or arginine (in the edited form) which confers low Ca2+ permeability (Hume et al., 1991; Verdoorn et al., 1991). Co-assembly of edited GluA2 with GluA1, 3 or 4 subunits prevents Ca2+ entry through the receptor channel. In neurons, the efficiency of editing is high and thus the Ca2+ permeability of the majority of AMPA receptors is negligible; however, in malignant brain tumors, the editing efficiency is substantially reduced (Maas et al., 2001). Similarly in pediatric glioblastomas, only 50 to 70% of the GluA2 RNA is edited (Venkatesh et al., 2019), likely augmenting the Ca2+ signaling pathways that have been implicated in glioblastoma proliferation and migration.
Voltage-Gated Ion Channels Enriched in Glioblastoma
Voltage-gated Ca2+ (CaV) channels are involved in diverse functions, including regulating the entry of Ca2+ to control the release of neurotransmitters and hormones. CaV channels are composed of a number of subunits encoded by separate genes; the largest alpha-1 subunit forms the ion pore, and is associated with alpha-2, beta, gamma and delta auxiliary subunits that modulate functional properties. The Ca2+ channel-related genes showing transcript levels enriched in glioblastoma (Table 1) were CaV gamma3, -7, and -8. CaV alpha subunits were not represented in the GBM-enriched category, perhaps hinting at a role for gammas other than voltage-gated Ca2+ entry. As reviewed by Snutch and colleagues (2000-2013), there are eight types of CaV γ subunits. One of the first to be discovered was stargazin (classified as γ2), named after the Stargazer mouse strain which is prone to absence seizures caused by a mutation in the gamma2 gene. It is intriguing to note that gamma subunits γ3, γ7, and γ8 are expressed in brain and have been found to serve as transmembrane AMPA receptor regulatory proteins, known as TARPs (Tomita et al., 2003; Yamazaki et al., 2010). TARPs γ2 and γ7 cooperatively promote AMPA receptor expression in cerebellum, and γ7 also promotes expression in glia (Yamazaki et al., 2010). The primary AMPA receptor found in the hippocampus is a heteromeric combination of GluA1 and 2 subunits, associated with two γ8 auxiliary subunits which modulate gating by interacting with the GluA2 ligand-binding domain (Herguedas et al., 2019). The interaction of GluA2 receptors with TARP γ8 shifts the AMPA receptor channel conductance to a higher level (Carrillo et al., 2019). It will be interesting to test whether the augmentation of glutamate signal amplitudes by increasing expression or conductance is a key adaptive mechanism favoring gamma subunit expression in glioblastoma.
Based on transcriptomic data, the voltage-gated Na+ (NaV) alpha subunits 1, 2, and 3 appear to be expressed in glioblastoma at the RNA level. In excitable cells the primary role of NaV channels is to generate action potentials that can be conducted over distances, harnessing a regenerative loop fueled by the Na+ gradient. The Na+-dependent action potential typically links to voltage-gated Ca2+ entry to generate biological work such as the release of neurotransmitters, secretion of hormones, or contraction of muscle, for example. The reported absence of measurable action potentials in identified GBM cells (Venkataramani et al., 2019) however invites speculation on alternative hypotheses, including possibilities that NaV protein might not be assembled or functional, its distribution in plasma membrane might lack a requisite spatial organization, or shunting of currents through the gap junction-linked network might render depolarization responses undetectable. In the U87 GBM cell line, the effectiveness of a NaV channel blocker found to reduce migration and invasiveness was not dependent on Na+ channel inhibition, not replicated by application of tetrodotoxin, and was suggested to be due to side effects on RNA demethylase (Malacrida et al., 2020). In general, NaV channels serve as a mechanism for the amplification of small excitatory signals into large amplitude depolarizations, and in principle might contribute a role in boosting excitatory responses in glioblastoma, but a logical role for NaV channels in GBM remains to be proposed for testing.
Voltage-gated K+ channels are a large diverse group of channels that serve to maintain the negative resting membrane potential in many different types of cells, and to restore the resting voltage during the repolarization phase of action potential responses in excitatory cells such as neurons, muscle and sensory cells. Ca2+ dependent K+ channels add the capacity to shape membrane potential by controlling the amplitude and kinetics of repolarizing K+ currents in response to both voltage and intracellular Ca2+ levels. K+ channels identified at moderate transcript levels (Table 1) include the voltage-gated Kv4.2 (KCND2), an inactivating A-type channel expressed in brain and heart, and Kv7.2 (KCNQ2), which alone or with KCNQ3 forms M channels in the central and peripheral nervous system. M-currents activate and deactivate slowly, regulating excitability in neurons. Channel closure by Gq-protein-coupled muscarinic acetylcholine receptors enables repetitive firing (Brown and Passmore, 2009). The Ca2+-activated KCa2.2 (KCNN2) and 2.3 (KCNN3), members of the small conductance class labeled SK, are more sensitive to Ca2+ and less sensitive to voltage than the big conductance BK channels, and contribute to hyperpolarized interludes in neurons following bursts of firing, as reviewed by Kaczmarek and colleagues (2017). In glioblastoma, a proposed purpose of cation efflux through K+ channels (in parallel with anion efflux though Cl- channels) is to induce osmotic shrinking, decreasing glioma cell volume to expedite invasiveness through confined spaces (Olsen et al., 2003). Alternatively, in the absence of a substantial Cl- leak, K+ currents would be expected to buffer membrane potential at a negative voltage, maintaining the driving force for Ca2+ entry (through pathways including for example, the Ca2+ permeable glutamate receptors). Relatively high transcript levels in glioblastoma were indicated for the inward rectifier Kir4.1 (KCNQ2), summarized in Table 1. This channel is expressed in brain and kidney. In brain oligodendrocytes, Kir4.1 channels are clustered near nodes in the myelin, where they are thought to remove accumulations of K+ near electrically active membranes (Schirmer et al., 2018). Loss of function of Kir4.1 is associated with axonal degeneration. The pattern of subcellular localization of the water channel AQP4 in glial cells coincides with that of the potassium channel Kir4.1, suggesting a coordination of activities for the spatial buffering of K+ and water homeostasis (Nagelhus et al., 2004; Yool, 2007).
Synaptic Proteins Enriched in Glioblastoma
Since the synapses onto glioblastoma cells are made by neurons, and reverse synapses have not been observed (Venkataramani et al., 2019), at first glance it seems a mystery why glioblastoma cells would have measureable levels of transcripts for presynaptic proteins. However, a careful evaluation of the candidate genes indicates that these proteins might not be exclusively limited to presynaptic functions. Synaptic proteins in glioblastoma (Table 1) with moderate transcript levels include NSG2 and SYN1. Neuronal vesicle trafficking associated 2 (NSG2) protein is abundant in the dendrites and cell bodies of developing neurons, but its role until recently was unknown. Chander and colleagues discovered Nsg1 physically interacts with GluA1 and GluA2 in postsynaptic domains, where it promotes synapse formation, GluR expression, and synaptic activity (Chander et al., 2019). Synapsin I (SYN1) is regarded as a reliable presynaptic marker for immunocytochemistry. In axonal nerve terminals, it associates with synaptic vesicles to hold them in a reserve (non-released) pool. Phosphorylation in response to Ca2+ influx causes synapsin I to dissociate, freeing the vesicle for docking and transmitter release. Immunostaining and northern analyses have shown SYN1 protein and mRNA can expressed outside of neural tissues, in liver, epithelia, and cell lines, in which it is associated with the Golgi complex rather than plasma membrane, suggesting involvement in protein trafficking (Bustos et al., 2001). Synapsins have been identified in a variety of non-neuronal cells (Cesca et al., 2010) including chromaffin cells, PC12, immature astrocytes, pancreatic beta cells (in which synapsin I is associated with secretory granules), and in osteoblasts which are able to trigger glutamate release by processes mimicking neurotransmission (Bhangu et al., 2001).
It is interesting to note that human glioblastoma cells can release sufficient glutamate to raise extracellular concentrations up to 600 µM, which promotes GBM cell motility and growth, and causes excitotoxic cell death and seizures in peritumoral brain tissues (Corsi et al., 2019). The glutamate release process could be mediated by entirely by amino acid exchangers; but atypical neurotransmission-like mechanisms remain to be explored.
High transcript levels in glioblastoma (Table 1) were measured for SNAP-25, the synaptic vesicle glycoprotein 2A (SV2A), and for synaptophysin. Synaptosome associated protein 25 (SNAP-25) is involved in the control of synaptic vesicle exocytosis, and is essential for synaptic transmission (Jahn et al., 2003). SNAP-25-mediated effects do not point exclusively to a presynaptic role; it also has been suggested to govern postsynaptic morphology (Fossati et al., 2015) and receptor trafficking (Selak et al., 2009; Lau et al., 2010; Jurado et al., 2013) though some details remain controversial (Antonucci et al., 2016). SV2A, expressed throughout the brain, is a binding site for some anti-epileptic drugs such as levetiracetam, and is differentially associated with GABAergic and glutamatergic synapses, depending on the brain region, to regulate the balance of excitatory and inhibitory inputs (Venkatesan et al., 2012; Vanoye-Carlo and Gomez-Lira, 2019). Knock out of this gene in mice gives rise to violent spontaneous seizure activity leading to early death. Although viewed as a marker of neuronal synaptic vesicles, synaptophysin protein (SYP) was identified in medulloblastoma, neuroblastoma, and other tumors more than 30 years ago (Schwechheimer et al., 1987). It is linked to endo- and exocytotic mechanisms including formation of a fusion pore for the release of neurotransmitter, though genetic knock out does not result in an overt phenotype (McMahon et al., 1996). Continuing work has found synaptophysin immunoreactivity to be a diagnostic and prognostic tool in a wide array of cancer types, but the functional role of the protein remains unclear.
Very high transcript levels in glioblastoma (Table 1) were measured for synaptotagmin-11. Synaptotagmins generally are associated with evoked release of synaptic and secretory vesicles, as low affinity Ca2+ sensors that can bind to syntaxin and SNAP-25 (Burgoyne et al., 2019). Synaptotagmin 11 (Syt11), a substrate for ubiquitinylation by parkin, is one of the risk genes for Parkinson's disease; overexpression (resulting from parkin dysfunction) leads to impaired dopamine release, degeneration of dopaminergic neurons, and associated motor behavioral deficits (Wang et al., 2018a). These findings indicated a role for synaptotagmin-11 in the inhibition of endocytosis and vesicle recycling in neurons. Another member of the synaptotagmin family, Syt3 was shown to interact with GluA2 receptors in postsynaptic membranes, where it enhanced the endocytosis of receptors in response to Ca2+, generating an activity-dependent decrease in synaptic strength (Awasthi et al., 2019). It would be interesting to test the idea that Syt11 in glioblastoma slows endocytosis of GluA2 receptors, promoting glutamate-driven signaling.
Transcriptomic Analysis of Additional Channels and Transporters Linked to Glioblastoma
The specificity of enhanced channel and transporter gene expressions as markers for GBM can be assessed by comparing median transcript levels for a protein of interest across a broad panel of cancer types (Figure 3A) and assessing whether expression at levels greater than the median correlates with a poorer prospect of survival (Figure 3B). Aquaporins 1 and 4 (AQP1 and AQP4) are both strongly elevated in GBM; AQP1 is also upregulated in renal cancer. The high levels of AQP1 and AQP4 seen in GBM correlate with a poor chance of survival that is characteristic of the GBM diseases (Figure 2B). Conversely, the low levels of AQP1 and absence of detectable AQP4 transcripts in stomach and pancreatic cancers do not appear to offer any protective advantage in terms of survival, and the higher level of AQP1 in renal cancer does not coincide with a higher risk of death.
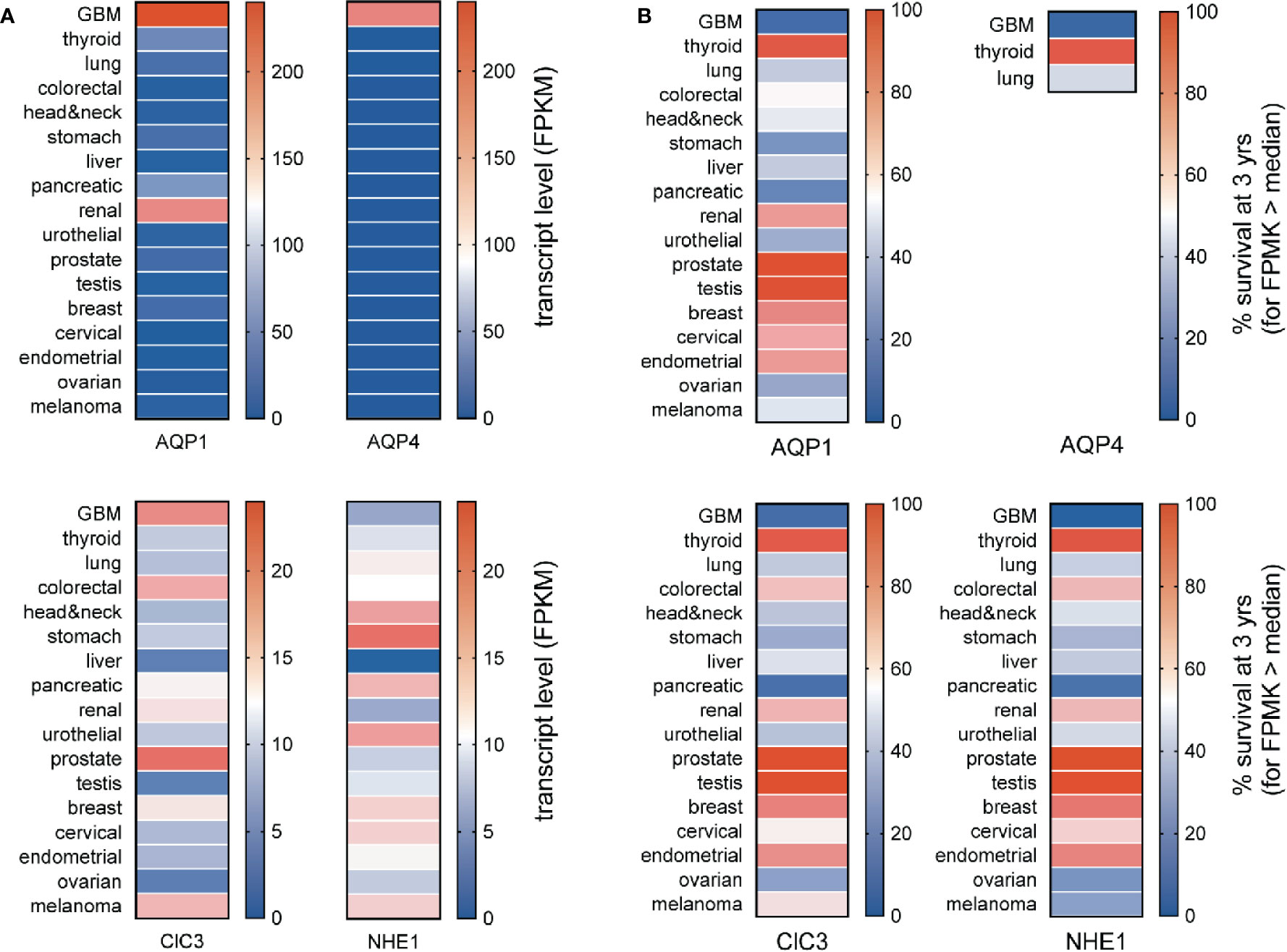
Figure 3 Comparison of transcript levels across different cancers. (A) Heat map comparison of median transcript levels (FPMK, color bars) across a panel of different cancers. (B) Heat map comparison of patient survival at 3 years (%, color bars) across a panel of different cancers. Results were calculated from TCGA data available in the Human Protein Atlas. TCGA, The Cancer Genome Atlas.
The expression of AQP1 in the leading edges of migrating cells has been linked to enhanced motility, as documented in neural crest (McLennan et al., 2020) and various types of cancer cells including colon cancer, gliomas, breast cancer, and endothelial cells involved in angiogenic responses (McCoy and Sontheimer, 2007; Pei et al., 2016a; Nakhjavani et al., 2019; Tomita et al., 2019). AQP1 is a dual water and ion channel (Anthony et al., 2000; Yu et al., 2006; Campbell et al., 2012; Kourghi et al., 2018), which has been suggested to accelerate cell migration by enabling parallel water and cation entry (Yool et al., 2009; Pei et al., 2019), promoting the cellular lamellipodial and filopodial extensions needed for forward movement. Inhibition of either the AQP1 water pores (in each subunit) or the ion channel (thought to reside in the central pore of the tetramer) slows migration in AQP1-expressing cancer cell lines (Kourghi et al., 2016; Pei et al., 2016b), and the inhibition of both shows an enhanced blocking effect on motility (De Ieso et al., 2019).
Transcript levels for the chloride channel ClC3 are seen across multiple classes of cancers, with the highest in prostate cancer, fairly high values in GBM, melanoma and colorectal cancers, and moderate levels in others. Molecular knockdown of ClC3 channels in glioma cells decreased Cl- efflux, and slowed the volume decrease associated with DNA condensation in mitosis, suggesting a role for this conductance in promoting proliferation (Habela et al., 2008). Block of outwardly rectifying Cl- currents in U251MG cells with chlorotoxin reduced motility in invasion assays in vitro and in rat cortical brain slice assays, suggesting glioma cell motility might be enhanced by a Cl–mediated decrease in cell volume, facilitating penetration through extracellular matrices (Soroceanu et al., 1999). Subsequent work showed the site of action of chlorotoxin in glioma invasion was via the selective inhibition of matrix metalloprotease-2 (Cao et al., 2013). Chlorotoxin binds preferentially to cancer cells including gliomas (Lyons et al., 2002), and its promise as a selective histopathological marker has proven valuable for targeting nanoparticles in situ in human GBM xenografted mice (Stephen et al., 2019), opening exciting possibilities for GBM cell-targeted delivery of chemotherapeutics (Fang et al., 2015).
The sodium proton exchanger NHE1, though a negative prognostic indicator for GBM, is most strongly expressed in cancer types such as head and neck, stomach, pancreatic, urothelial, and comparatively low in GBM. Nonetheless, increased levels of NHE1 expression found in the lamellipodial leading edges in primary glioma and GBM cell lines including U87, U251 and U118 are associated with increased export of protons, resulting in a more acidic environment and basic intracellular pH, associated with resistance to temozolomide, and enhanced tumor progression (Cong et al., 2014).
Other candidates meriting further analysis include gap junction channels (connexin 26) and bestrophin. Connexin 26, Cx30 and Cx43 are characteristic components of the majority of gap junctions between astrocytes in the rat central nervous system (Rash et al., 2001). Bestrophin Ca2+-activated anion channels are permeable to chloride as well as large organic anions such as glumate and GABA; they are expressed throughout the brain and in the retinal pigment epithelium of the eye, in which inherited mutations are associated with Best's type of macular degeneration (Oh and Lee, 2017). Roles of these channels in GBM remain to be determined.
Prognostic Value in Evaluating Sets of Signaling Proteins
The GBM Bio Discovery portal provides an interface for evaluating defined sets of multiple genes as prognostic indicators, drawing on the Human Protein Altas TCGA transcriptomics database. For the analysis here, clusters of genes were created from the GBM-enriched genes summarized in Table 1 that had strong reliability scores (i.e., links to GBM based on multiple lines of evidence including immunocytochemistry, protein and RNA analyses, as denoted by “pathological evidence” scores of 1.0). The three clusters created were ionotropic neurotransmiter receptors, ion channels, and synaptic proteins (Figure 4). Data are presented as heatmaps (above), and as Kaplan Meier survival curves (below) for each cluster. Each column in the heat map represents data from a single GBM patient, and illustrates the different transcript levels measured for the genes in the cluster, presented as Z-scores relative to the average transcript level across the GBM sample population. Subtypes of glioblastoma assigned per patient are depicted by a color key (top row). Column order in the heat map columns reflects overall transcript levels, with predominantly low (blue) on the left side of the heat map, ranging to high (red) on the right side.
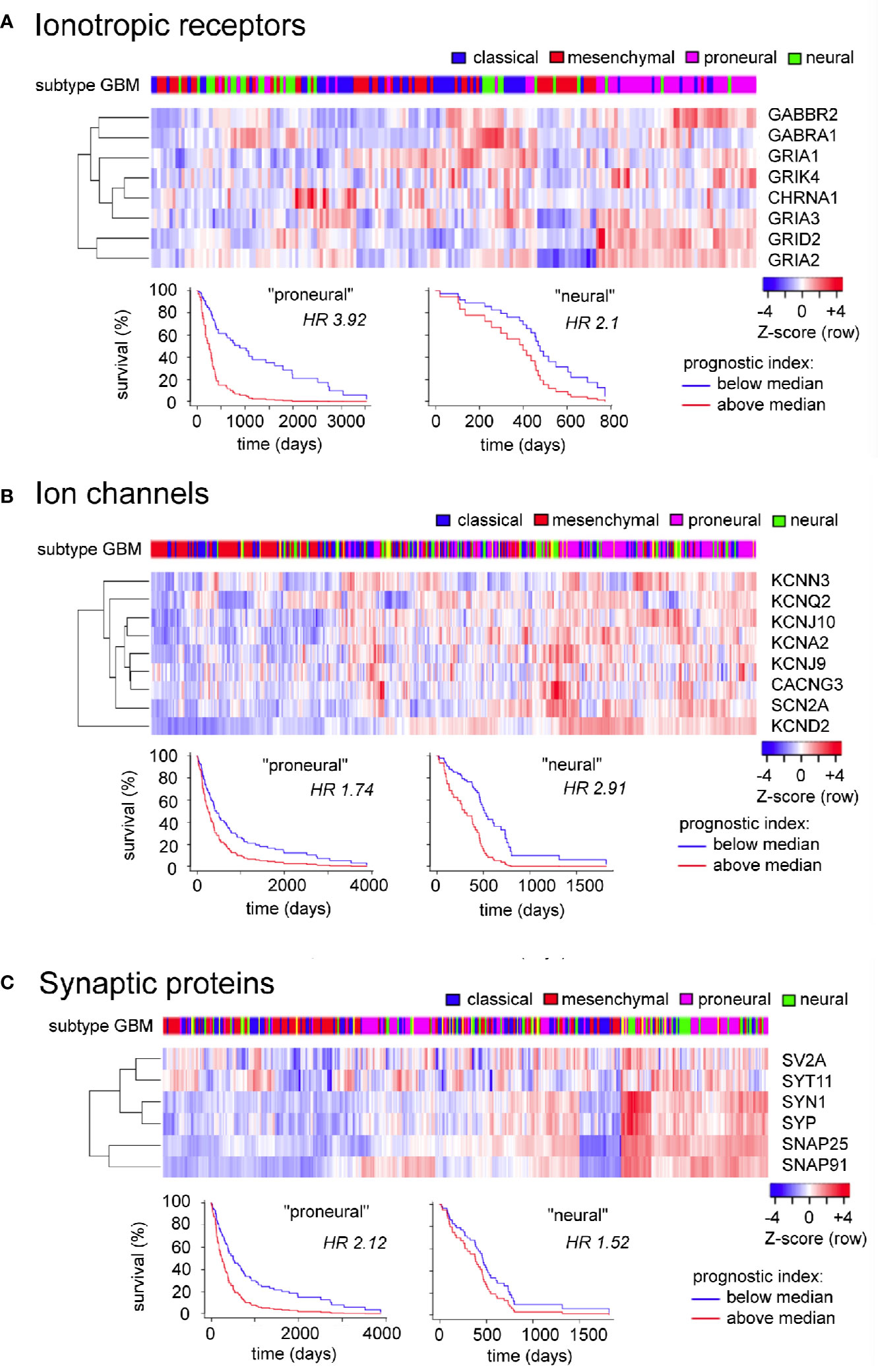
Figure 4 Evaluation of expression patterns and additional prognostic information available in analyzing sets of related signaling genes, for ionotropic neurotransmitter receptors (A), ion channels (B), and synaptic proteins (C) enriched in glioblastoma. Data were analyzed using the GBM Bio Discovery Portal, a resource for user-specified interrogation of The Cancer Genome Atlas (TCGA) database, for glioblastoma multiforme categorized by molecular subtype (Verhaak et al., 2010). GBM, glioblastoma multiforme.
The subtype categories (neural, proneural, mesenchymal, and classical) serve as a useful tool for TCGA data analysis but do not reflect a consensus opinion; evidence for the neural classification has been questioned (Behnan et al., 2019) leaving support for three subtypes only. Limitations of the TCGA database include the fact that many of the samples analyzed were taken from single random sites in the tumor bulk, not capturing the inherent heterogeneity of subtypes across different regions within the tumor (Puchalski et al., 2018). However, biological relevance for the classification scheme has been supported by similarities in expression profiles of glioma cells with those of brain cell populations, prompting the idea that a common source for the gliomas might be pleuripotent neuronal stem cells of adult brain, which become glioblastoma stem cells and differentiate into subtypes based on environmental and genetic factors. Proneural cells show an oligodendrocyte progenitor cell-like signature, and classical and mesenchymal subtypes reflect astrocyte-like lineages (Verhaak and Valk, 2010; Alcantara Llaguno et al., 2015).
For the Ionotropic receptors (Figure 4A) and Ion channels (Figure 4B) clusters, the samples with predominantly high levels of expression (right side) correlated most frequently with GBM subtypes diagnosed as proneural in character. For the Synaptic proteins cluster (Figure 4C), the high expression patterns correlated with both proneural and neural subtypes of GBM. The branched tree (left side of the heat map) summarizes the degree of covariance in transcript levels for genes within the cluster. The survival plots in each cluster demonstrated that the prognostic impact of expression of a set of genes was stronger than that of any individual gene alone, as indicated by Hazard Ratio (HR) scores nearing as high as four for clusters (i.e., a 4-fold increase in chance of death in 3 years for transcript levels > median), as compared with hazard ratios close to 1.0 (ranging mainly 0.8 to 1.2) when evaluated for individual genes.
A comparable analysis of genes in the ‘Other channels and transporters' cluster (Figure 5) based on Table 1 (not limited to pathological evidence scores of 1.0) shows that patterns of high transcript levels in this group correlated most strongly with the classical and proneural subtypes of GBM, with hazard ratios of 2.4 to 2.5 determined from survival curves.
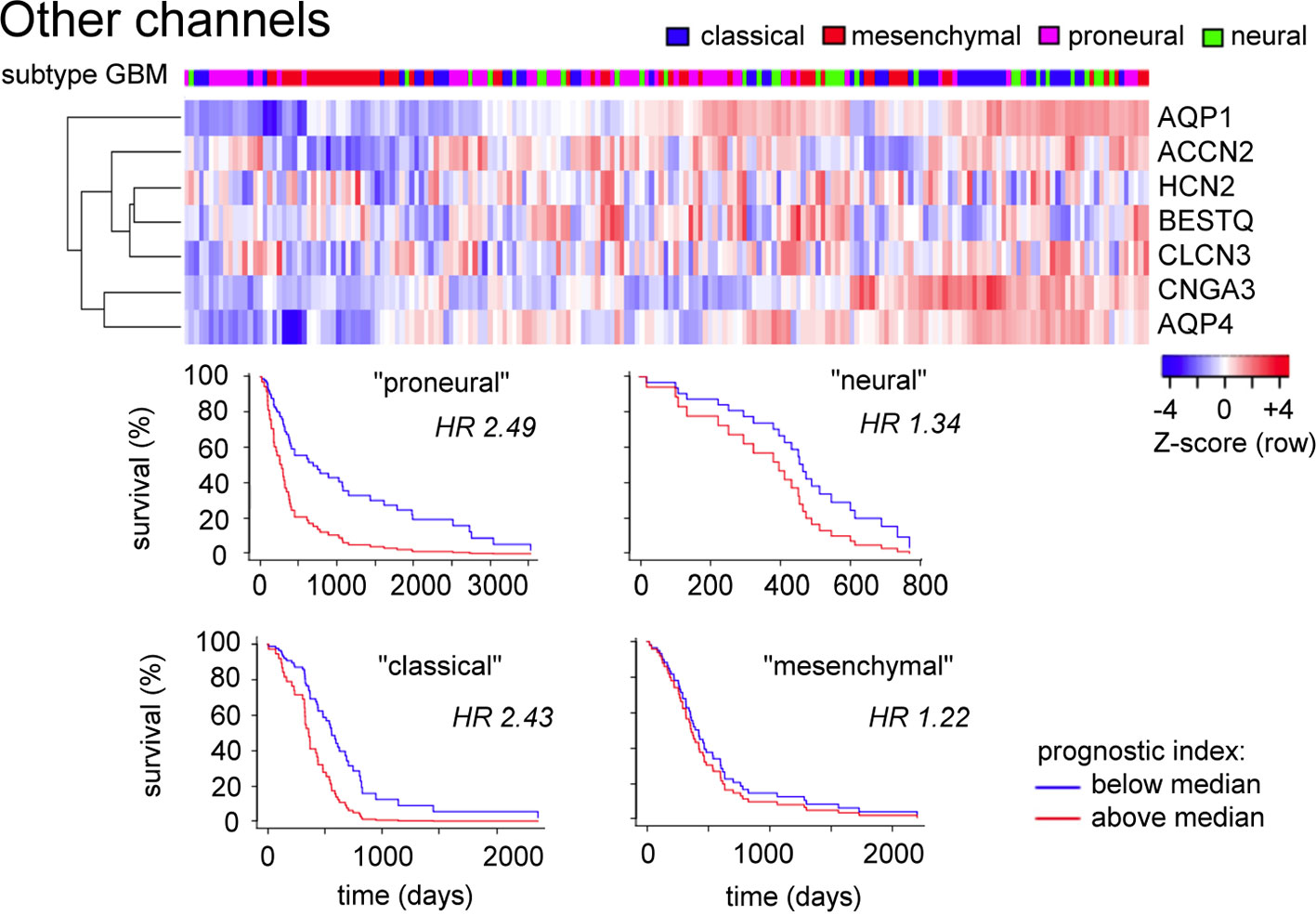
Figure 5 Evaluation of expression patterns for additional classes of channels enriched in glioblastoma. Data were analyzed using the GBM Bio Discovery Portal. GBM, glioblastoma multiforme.
Transcriptomic data analyses indicated that the proneural subtype is consistently correlated with patterns of transcript levels across several clusters of membrane signaling proteins associated with GBM, and illustrate the point that calculations of hazard ratios for related groups of genes in GBM could have a stronger prognostic significance with respect to evaluating patient survival time than predictions based on expression levels of single genes alone. This insight at the genomic level is likely to be meaningful from a functional point of view as well. A major limitation that must be kept in mind in these analyses is that the tumor biopsy samples used for generating the RNAseq database were taken from random locations in the tumor, and thus are unlikely to capture the full heterogeneity and range of cell phenotypes within the tumor mass, complicating determination of correlations with patient survival and transcript prognostic value. Nonetheless, the transcriptomic analyses highlight the concept that cellular signaling is carried out by sets of proteins with partial overlap in functional properties, providing cells with capacity for compensation following the loss of a given signaling component. Therapeutic strategies aimed at modulating multiple proteins within a class, and multiple targets across classes, might prove to be more effective in realizing a clinically relevant outcome. A key point is that an ideal treatment, tailored to a given cancer, would incorporate multiple pharmacological agents targeted across a set of key proteins, each administered at low doses to reduce the severity of side effects, but achieving potency by a synergistic complementation. In this regard, it will be of interest to screen herbal medicinal extracts comprising naturally complex combinations of chemical components, for effects on a panel of different classes of signaling proteins for potentially synergistic interactions.
Natural Plant Products as Sources of Therapeutic Agents
The current therapies for glioblastoma involving temozolomide chemotherapy, radiation, and surgical resection yield only limited improvements in survival periods. New drugs that induce fewer side effects and lead to more favorable prognoses are needed. Plant extracts have since ancient times been used traditionally as medicines in Egypt, China, India, Greece, and other countries. Some of the first written records on the medicinal uses of plants date back to 2600 BC. The plant kingdom comprises nearly 250,000 plant species of which only around 10% have been screened thus far for different diseases. Medicinal herbs found by experience to be valuable for treating diseases and ailments have served as original sources for many clinical compounds in the current therapeutic armamentarium. The National Cancer Institute (USA) has screened 35,000 plant samples (114,000 extracts) from 20 countries for anti-cancer activity (Developmental Therapeutics Program, NIH, USA). Approximately 60% of the clinically approved anti-cancer drugs available commercially are derived from medicinal plants (Khan, 2014). Many studies have considered compounds as effective potential anticancer treatments if they arrest the cell cycle or trigger apoptosis (reducing proliferation), impair angiogenesis (reducing metabolic supply), or decrease the expression of matrix metalloproteinases (impairing invasion) as reviewed by Park, Erices, and colleagues (Park et al., 2017; Erices et al., 2018). Ability to cross the blood brain barrier would facilitate drug delivery to the tumors.
Natural plant products tested in glioblastoma including flavonoids, terpenoids, polyphenols, epigallocatechin gallate, quinones, saponins, and others (Table 2), have been assayed mainly in glioblastoma cell lines, as well as xenografts in mice. A diverse set of plant products are effective in slowing proliferation and migration, and increasing cell death. The molecular mechanisms underpinning the effect of these compounds include decreased expression of angiogenic factors (VEGF and TGF-β1), disruption of signaling pathways (PI3K/AKT/mTOR, RAF-MEK-ERL, TLR4, IL-6 mediated JAK/STAT3, and Rho/ROCK), impaired mitosis (cell cycle arrest at G2/M phase), decreased expression of proliferation-associated genes (PCNA and cyclin D1), enhancement of apoptosis by increased Caspases (CASP 3, 9) and PARP 1 cleavage activities, decreased anti-apoptotic protein (Bcl-2) expression, downregulation of the activity of matrix metalloproteinases (MMP 2,9,14,16), and inhibition of actin assembly (see references in Table 2).
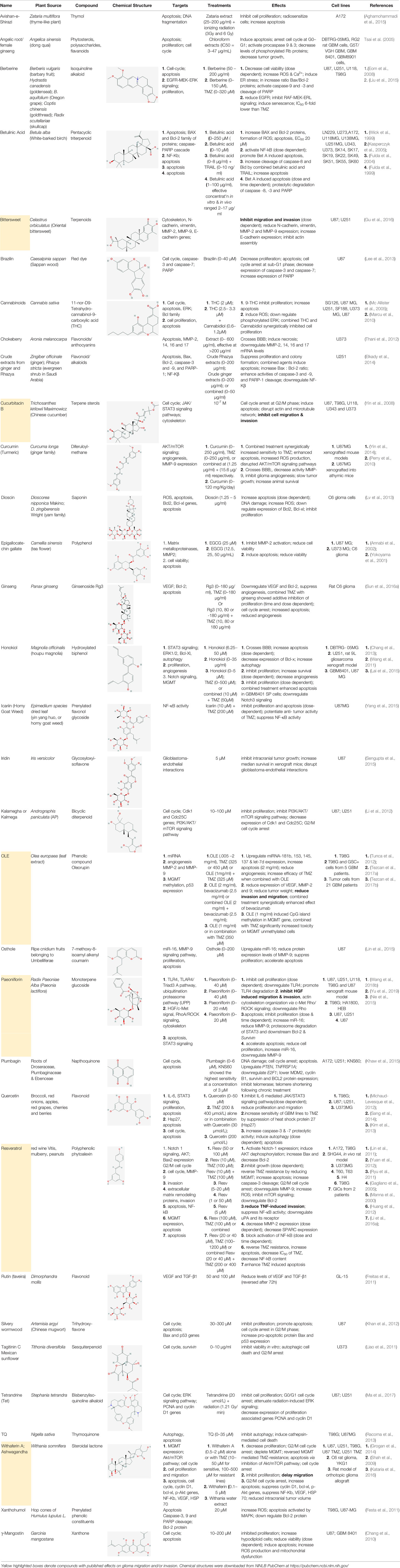
Table 2 Summary of alternative medicinal compounds and extracts reported to affect proliferation, viability, and/or motility of glioblastoma and malignant brain tumor cells.
The limited number of natural plant compounds identified as inhibitors of motility in glioblastoma (Table 2) included bittersweet (Celastrus orbiculatus), curcubitacin B (Trichosanthes kirilowii), OLE (Olea europaea leaf), paeoniflorin (Radix paeoniase alba), resveratrol (red wine and other sources), and withaferin A (Withania somnifera); however, it is important to note that potential anti-motility effects of other glioma-active plant preparations in Table 2 are not excluded, but remain to be evaluated. A useful starting point is to survey whether any of the motility-blocking herbal compounds have published effects on ion channels or ionotropic receptors. Evidence is limited in this under-researched field, but available publications suggest possible links. For example, celangulin I isolated from bittersweet activated high-voltage activated L-type Ca2+ channels in insect neurons, consistent with excitoxicity and insecticidal activity (Li et al., 2016b). Cucurbitacin IIa decreased the expression of glutamate receptors GluN2B (NMDA) and GluA1 (AMPA), and increased GABAAα2 expression in mouse brain amygdala (Zhou et al., 2017). OLE from Olea inhibited L-type Ca2+ currents in rat cardiac cells (Scheffler et al., 2008), and reduced L-type Ca2+ channel RNA levels in rat spinal cord (Zare et al., 2012). Oleuropein from Olea caused dose-dependent increases in intracellular Ca2+ in mesothelioma cells, suggested to be mediated by activation of low-threshold T-type Ca2+ channels (Marchetti et al., 2015). An analgesic action of paeoniflorin was suggested to be mediated by activating adenosine A1 receptors and reducing glutamate release in rat pain sensory fibers (Zhang et al., 2009). Resveratrol has been found alter activity in an array of ion channel classes, including the inhibition of Ca2+ channels involved in pain perception pathways in mice, consistent with an anti-nociceptive effect of systemic treatment (Pan et al., 2017), inhibition of cardiac L-type Ca2+ currents in rat ventricular myocytes (Zhang et al., 2006), and activation of T-type Ca2+ channels in a mesothelioma cell line (Marchetti et al., 2016), providing an interesting parallel with the effects of Olea extracts listed above. Resveratrol activated defined subtypes of GABAA receptors containing α1 subunits, expressed in Xenopus oocytes (Groundwater et al., 2015), and blocked the calcium-dependent K+ channel, KCa3.1, heterologously expressed in 3T3 fibroblasts (Olivan-Viguera et al., 2013). Withania extract activated mammalian brain GABAA and GABArho receptors recorded by voltage clamp in the Xenopus expression system, evoking currents comparable to those induced by the native neurotransmittter; however, responses were not activated by the isolated components withaferin A and withanolide A, suggesting other active agents are present in the extract (Candelario et al., 2015). These observations point to an important gap in knowledge regarding the ion channel and receptor modulatory effects of anti-cancer medicinal herb extracts.
It is interesting to note that some compounds such as curcumin [1.25 µg/ml] (Yin et al., 2014), OLE extract [1 mg/ml] (Tezcan et al., 2017a), resveratrol [10 µM] (Yuan et al., 2012) and quercetin [30 µmol/L] (Sang et al., 2014) when tested in combination with temozolomide have been effective in increasing survival in animal models, overcoming temozolomide resistance by increasing ROS production, disrupting AKT/mTOR signaling, reducing MGMT gene expression, suppressing Hsp27 protein expression, and downregulating the expression of MMP-2 and -9. The plant derived compounds are effective in low doses and intriguingly the effective concentration of temozolomide required in combination is also reduced (50–100 µM) leading to more effective treatments with fewer side effects. Thus, natural products from traditional medicinal plants could prove useful in combination with other plant products or chemotherapy drugs, as a new way forward in treating glioblastoma tumors and improving prognoses, alone and in combination with front-line chemotherapeutic agents.
Combining Modulators for Multiple Targets to Increase Selectivity and Potency of Treatments for Glioblastoma
Selected sets of membrane signaling proteins that are upregulated in glioblastoma might be added to the matrix of markers supporting diagnosis and targeted intervention. Moving from bench to bedside is challenging; many failures have resulted from approaches focused on single targets and single agents as therapies. Combinations of agents offer potential to focus treatments more precisely on a class of cells of interest, based on unique signature profiles reflecting expression of multiple key proteins from more than one essential pathway. However even combinations of approaches have not yet achieved the desired endpoints.
The standard current therapy for GBM does use a combined approach, employing radiotherapy, temozolomide chemotherapy and surgical resection. Studies have explored possible benefits of layering additional agents onto this standard therapy regime with the aim of further extending survival. Some logical pharmacological candidates such as gefitinib (an inhibitor of the EGF receptor) or bevacizumab (an inhibitor of angiogenesis) added to the standard therapy have not met the aims for improved overall survival (Okonogi et al., 2015).
With a diverse types of GBM-enriched proteins all appearing to point to a major role for glutamate signaling in glioblastoma, glutamate receptors are a clear target of interest for potential therapies, but have proven elusive thus far. Glutamate driven Ca2+ entry is linked to the invasion and growth, while release of excitotoxic glutamate in the local environment causes neuronal loss and further recruits tumor-associated microglia which produce growth factors enhancing tumor cell survival (Corsi et al., 2019). AMPA receptors are associated with integrin, and could contribute localized signaling at cytoskeletal anchor points in focal adhesion complexes (Piao et al., 2009) which might be key in invasive motility. A non-competitive antagonist of AMPA receptors, talampanel (also known as GYKI 53773 or LY300164) was found to increase the median survival of patients with GBM (20.3 months versus 14.6 months) in a cohort study of 60 patients (Grossman et al., 2009). Despite promise in Phase II, it has not been validated further and might be limited by a short half-life of 3 h (Langan et al., 2003). This agent, a derivative of benzodiazepine, also blocks kainate receptors (Donevan et al., 1994).
Targeting the AMPA glutamate receptors would seem to be a compelling approach. However, the predominant role of AMPA receptors in fast excitatory signaling throughout the central nervous system raises a challenge on how to target inhibitors selectively to cancer cells while maintaining tolerability. Most AMPA receptor antagonists tested thus far have proven unsuitable due to side effects and pharmacokinetics, in contrast to effectiveness in preclinical animal models (Lee et al., 2015). The natural extracts in traditional medicines that have been found to be effective might serendipitously include agents which modulate more than one of the classes of signaling molecules highlighted in this review, that are enriched in glioblastoma and could be important contributors to the pathological process. New approaches will be likely to exploit evidence-based combinations of selected agents, each at low doses, along with tools for cancer-selective targeting to GBM cells, to engineer new and potent therapeutic strategies for meeting the medical challenge of treating glioblastoma.
Data Availability Statement
The datasets analyzed for this study can be found in the following repositories:
The Human Protein Atlas available from http://www.proteinatlas.org
The National Cancer Institute Glioblastoma Bio Discovery Portal available from https://gbm-biodp.nci.nih.gov/
The National Institutes of Health NCBI PubMed search platform available from https://www.ncbi.nlm.nih.gov/pubmed
The US National Library of Medicine PubChem available at https://pubchem.ncbi.nlm.nih.gov/.
Author Contributions
All authors listed have made substantial, direct, and intellectual contribution to the work and approved it for publication.
Funding
Support was provided by Australian Research Council Discovery Project grant 19ARC_DP190101745.
Conflict of Interest
The authors declare that the research was conducted in the absence of any commercial or financial relationships that could be construed as a potential conflict of interest.
References
Aghamohammadi, A., Hosseinimehr, S. J., Ghasemi, A., Azadbakht, M., Pourfallah, T. A. (2015). Radiosensitization effects of a Zataria multiflora extract on human glioblastoma cells. Asian Pac J. Cancer Prev. 16, 7285–7290.sw. doi: 10.7314/APJCP.2015.16.16.7285
Alcantara Llaguno, S. R., Wang, Z., Sun, D., Chen, J., Xu, J., Kim, E., et al. (2015). Adult Lineage-Restricted CNS Progenitors Specify Distinct Glioblastoma Subtypes. Cancer Cell 28, 429–440. doi: 10.1016/j.ccell.2015.09.007
Annabi, B., Lachambre, M.-P., Bousquet-Gagnon, N., Pagë, M., Gingras, D., Bëliveau, R. (2002). Green tea polyphenol (–)-epigallocatechin 3-gallate inhibits MMP-2 secretion and MT1-MMP-driven migration in glioblastoma cells. Biochim. Biophys. Acta (BBA)-Mol. Cell Res. 1542, 209–220. doi: 10.1016/S0167-4889(01)00187-2
Anthony, T. L., Brooks, H. L., Boassa, D., Leonov, S., Yanochko, G. M., Regan, J. W., et al. (2000). Cloned human aquaporin-1 is a cyclic GMP-gated ion channel. Mol. Pharmacol. 57, 576–588. doi: 10.1124/mol.57.3.576
Antonucci, F., Corradini, I., Fossati, G., Tomasoni, R., Menna, E., Matteoli, M. (2016). SNAP-25, a Known Presynaptic Protein with Emerging Postsynaptic Functions. Front. Synaptic Neurosci. 8, 7. doi: 10.3389/fnsyn.2016.00007
Awasthi, A., Ramachandran, B., Ahmed, S., Benito, E., Shinoda, Y., Nitzan, N., et al. (2019). Synaptotagmin-3 drives AMPA receptor endocytosis, depression of synapse strength, and forgetting. Science 363. doi: 10.1126/science.aav1483
Behnan, J., Finocchiaro, G., Hanna, G. (2019). The landscape of the mesenchymal signature in brain tumours. Brain 142, 847–866. doi: 10.1093/brain/awz044
Bergles, D. E., Roberts, J. D., Somogyi, P., Jahr, C. E. (2000). Glutamatergic synapses on oligodendrocyte precursor cells in the hippocampus. Nature 405, 187–191. doi: 10.1038/35012083
Bhangu, P. S., Genever, P. G., Spencer, G. J., Grewal, T. S., Skerry, T. M. (2001). Evidence for targeted vesicular glutamate exocytosis in osteoblasts. Bone 29, 16–23. doi: 10.1016/S8756-3282(01)00482-3
Birch, J. L., Strathdee, K., Gilmour, L., Vallatos, A., Mcdonald, L., Kouzeli, A., et al. (2018). A Novel Small-Molecule Inhibitor of MRCK Prevents Radiation-Driven Invasion in Glioblastoma. Cancer Res. 78, 6509–6522. doi: 10.1158/0008-5472.CAN-18-1697
Brown, D. A., Passmore, G. M. (2009). Neural KCNQ (Kv7) channels. Br. J. Pharmacol. 156, 1185–1195. doi: 10.1111/j.1476-5381.2009.00111.x
Burger, P. C., Heinz, E. R., Shibata, T., Kleihues, P. (1988). Topographic anatomy and CT correlations in the untreated glioblastoma multiforme. J. Neurosurg. 68, 698–704. doi: 10.3171/jns.1988.68.5.0698
Burgoyne, R. D., Helassa, N., Mccue, H. V., Haynes, L. P. (2019). Calcium Sensors in Neuronal Function and Dysfunction. Cold Spring Harb. Perspect. Biol. 11 (5). doi: 10.1101/cshperspect.a035154
Bustos, R., Kolen, E. R., Braiterman, L., Baines, A. J., Gorelick, F. S., Hubbard, A. L. (2001). Synapsin I is expressed in epithelial cells: localization to a unique trans-Golgi compartment. J. Cell Sci. 114, 3695–3704.
Campbell, E. M., Birdsell, D. N., Yool, A. J. (2012). The activity of human aquaporin 1 as a cGMP-gated cation channel is regulated by tyrosine phosphorylation in the carboxyl-terminal domain. Mol. Pharmacol. 81, 97–105. doi: 10.1124/mol.111.073692
Cancer Genome Atlas Research, N (2008). Comprehensive genomic characterization defines human glioblastoma genes and core pathways. Nature 455, 1061–1068. doi: 10.1038/nature07385
Candelario, M., Cuellar, E., Reyes-Ruiz, J. M., Darabedian, N., Feimeng, Z., Miledi, R., et al. (2015). Direct evidence for GABAergic activity of Withania somnifera on mammalian ionotropic GABAA and GABArho receptors. J. Ethnopharmacol. 171, 264–272. doi: 10.1016/j.jep.2015.05.058
Cao, X. C., Zhang, W. R., Cao, W. F., Liu, B. W., Zhang, F., Zhao, H. M., et al. (2013). Aquaporin3 is required for FGF-2-induced migration of human breast cancers. PloS One 8, e56735. doi: 10.1371/journal.pone.0056735
Carrillo, E., Shaikh, S. A., Berka, V., Durham, R. J., Litwin, D. B., Lee, G., et al. (2019). Mechanism of modulation of AMPA receptors by TARP-gamma8. J. Gen. Physiol.
Catacuzzeno, L., Aiello, F., Fioretti, B., Sforna, L., Castigli, E., Ruggieri, P., et al. (2011). Serum-activated K and Cl currents underlay U87-MG glioblastoma cell migration. J. Cell Physiol. 226, 1926–1933. doi: 10.1002/jcp.22523
Cesca, F., Baldelli, P., Valtorta, F., Benfenati, F. (2010). The synapsins: key actors of synapse function and plasticity. Prog. Neurobiol. 91, 313–348. doi: 10.1016/j.pneurobio.2010.04.006
Chander, P., Kennedy, M. J., Winckler, B., Weick, J. P. (2019). Neuron-Specific Gene 2 (NSG2) Encodes an AMPA Receptor Interacting Protein That Modulates Excitatory Neurotransmission. eNeuro 6 (1). doi: 10.1523/ENEURO.0292-18.2018
Chang, H.-F., Huang, W.-T., Chen, H.-J., Yang, L.-L. (2010). Apoptotic effects of γ-mangostin from the fruit hull of Garcinia mangostana on human malignant glioma cells. Molecules 15, 8953–8966. doi: 10.3390/molecules15128953
Chang, K. H., Yan, M. D., Yao, C. J., Lin, P. C., Lai, G. M. (2013). Honokiol−induced apoptosis and autophagy in glioblastoma multiforme cells. Oncol. Lett. 6, 1435–1438. doi: 10.3892/ol.2013.1548
Claes, A., Idema, A. J., Wesseling, P. (2007). Diffuse glioma growth: a guerilla war. Acta Neuropathol. 114, 443–458. doi: 10.1007/s00401-007-0293-7
Cong, D., Zhu, W., Shi, Y., Pointer, K. B., Clark, P. A., Shen, H., et al. (2014). Upregulation of NHE1 protein expression enables glioblastoma cells to escape TMZ-mediated toxicity via increased H(+) extrusion, cell migration and survival. Carcinogenesis 35, 2014–2024. doi: 10.1093/carcin/bgu089
Corsi, L., Mescola, A., Alessandrini, A. (2019). Glutamate Receptors and Glioblastoma Multiforme: An Old “Route”. New Perspect. Int. J. Mol. Sci. 20.
Cuddapah, V. A., Sontheimer, H. (2010). Molecular interaction and functional regulation of ClC-3 by Ca2+/calmodulin-dependent protein kinase II (CaMKII) in human malignant glioma. J. Biol. Chem. 285, 11188–11196. doi: 10.1074/jbc.M109.097675
De Ieso, M. L., Pei, J. V., Nourmohammadi, S., Smith, E., Chow, P. H., Kourghi, M., et al. (2019). Combined pharmacological administration of AQP1 ion channel blocker AqB011 and water channel blocker Bacopaside II amplifies inhibition of colon cancer cell migration. Sci. Rep. 9, 12635. doi: 10.1038/s41598-019-49045-9
Donevan, S. D., Yamaguchi, S., Rogawski, M. A. (1994). Non-N-methyl-D-aspartate receptor antagonism by 3-N-substituted 2,3-benzodiazepines: relationship to anticonvulsant activity. J. Pharmacol. Exp. Ther. 271, 25–29.
El Hindy, N., Rump, K., Lambertz, N., Zhu, Y., Frey, U. H., Bankfalvi, A., et al. (2013). The functional Aquaporin 1 -783G/C-polymorphism is associated with survival in patients with glioblastoma multiforme. J. Surg. Oncol. 108, 492–498. doi: 10.1002/jso.23421
Elkady, A. I., Hussein, R., Abu-Zinadah, O. A. (2014). Effects of crude extracts from medicinal herbs Rhazya stricta and Zingiber officinale on growth and proliferation of human brain cancer cell line in vitro. BioMed. Res. Int. 2014. doi: 10.1155/2014/260210
Emlet, D. R., Gupta, P., Holgado-Madruga, M., Del Vecchio, C. A., Mitra, S. S., Han, S. Y., et al. (2014). Targeting a glioblastoma cancer stem-cell population defined by EGF receptor variant III. Cancer Res. 74, 1238–1249. doi: 10.1158/0008-5472.CAN-13-1407
Eom, K.-S., Hong, J.-M., Youn, M.-J., So, H.-S., Park, R., Kim, J.-M., et al. (2008). Berberine induces G1 arrest and apoptosis in human glioblastoma T98G cells through mitochondrial/caspases pathway. Biol. Pharmaceut. Bull. 31, 558–562. doi: 10.1248/bpb.31.558
Erices, J. I., Torres, Á., Niechi, I., Bernales, I., Quezada, C. (2018). Current natural therapies in the treatment against glioblastoma. Phytother. Res. 32, 2191–2201. doi: 10.1002/ptr.6170
Fang, C., Wang, K., Stephen, Z. R., Mu, Q., Kievit, F. M., Chiu, D. T., et al. (2015). Temozolomide nanoparticles for targeted glioblastoma therapy. ACS Appl. Mater Interfaces 7, 6674–6682. doi: 10.1021/am5092165
Festa, M., Capasso, A., D'acunto, C. W., Masullo, M., Rossi, A. G., Pizza, C., et al. (2011). Xanthohumol induces apoptosis in human malignant glioblastoma cells by increasing reactive oxygen species and activating MAPK pathways. J. Natural Products 74, 2505–2513. doi: 10.1021/np200390x
Fossati, G., Morini, R., Corradini, I., Antonucci, F., Trepte, P., Edry, E., et al. (2015). Reduced SNAP-25 increases PSD-95 mobility and impairs spine morphogenesis. Cell Death Differ. 22, 1425–1436. doi: 10.1038/cdd.2014.227
Freitas, S., Costa, S., Azevedo, C., Carvalho, G., Freire, S., Barbosa, P., et al. (2011). Flavonoids inhibit angiogenic cytokine production by human glioma cells. Phytother. Res. 25, 916–921. doi: 10.1002/ptr.3338
Fulda, S., Jeremias, I., Steiner, H. H., Pietsch, T., Debatin, K. M. (1999). Betulinic acid: A new cytotoxic agent against malignant brain-tumor cells. Int. J. Cancer 82, 435–441. doi: 10.1002/(SICI)1097-0215(19990730)82:3<435::AID-IJC18>3.0.CO;2-1
Fulda, S., Jeremias, I., Debatin, K. M. (2004). Cooperation of betulinic acid and TRAIL to induce apoptosis in tumor cells. Oncogene 23, 7611–7620. doi: 10.1038/sj.onc.1207970
Gagliano, N., Moscheni, C., Torri, C., Magnani, I., Bertelli, A. A., Gioia, M. (2005). Effect of resveratrol on matrix metalloproteinase-2 (MMP-2) and Secreted Protein Acidic and Rich in Cysteine (SPARC) on human cultured glioblastoma cells. Biomed. Pharmacother. 59, 359–364. doi: 10.1016/j.biopha.2005.06.001
Ghosh, D., Nandi, S., Bhattacharjee, S. (2018). Combination therapy to checkmate Glioblastoma: clinical challenges and advances. Clin. Transl. Med. 7, 33. doi: 10.1186/s40169-018-0211-8
Grogan, P. T., Sarkaria, J. N., Timmermann, B. N., Cohen, M. S. (2014). Oxidative cytotoxic agent withaferin A resensitizes temozolomide-resistant glioblastomas via MGMT depletion and induces apoptosis through Akt/mTOR pathway inhibitory modulation. Investigat. New Drugs 32, 604–617. doi: 10.1007/s10637-014-0084-7
Grossman, S. A., Ye, X., Chamberlain, M., Mikkelsen, T., Batchelor, T., Desideri, S., et al. (2009). Talampanel with standard radiation and temozolomide in patients with newly diagnosed glioblastoma: a multicenter phase II trial. J. Clin. Oncol. 27, 4155–4161. doi: 10.1200/JCO.2008.21.6895
Groundwater, P. W., Hamid, K., Ng, I., Tallapragada, V. J., Hibbs, D. E., Hanrahan, J. (2015). The Differential Effects of Resveratrol and trans-epsilon-Viniferin on the GABA-Induced Current in GABAA Receptor Subtypes Expressed in Xenopus Laevis Oocytes. J. Pharm. Pharm. Sci. 18, 328–338. doi: 10.18433/J3QW3K
Gu, H., Feng, J., Wang, H., Qian, Y., Yang, L., Chen, J., et al. (2016). Celastrus orbiculatus extract inhibits the migration and invasion of human glioblastoma cells in vitro. BMC Complement. Altern. Med. 16, 387. doi: 10.1186/s12906-016-1232-8
Habela, C. W., Olsen, M. L., Sontheimer, H. (2008). ClC3 is a critical regulator of the cell cycle in normal and malignant glial cells. J. Neurosci. 28, 9205–9217. doi: 10.1523/JNEUROSCI.1897-08.2008
Herguedas, B., Watson, J. F., Ho, H., Cais, O., Garcia-Nafria, J., Greger, I. H. (2019). Architecture of the heteromeric GluA1/2 AMPA receptor in complex with the auxiliary subunit TARP gamma8. Science 364. doi: 10.1126/science.aav9011
Huang, H., Lin, H., Zhang, X., Li, J. (2012). Resveratrol reverses temozolomide resistance by downregulation of MGMT in T98G glioblastoma cells by the NF-κB-dependent pathway. Oncol. Rep. 27, 2050–2056. doi: 10.3892/or.2012.1715
Hume, R. I., Dingledine, R., Heinemann, S. F. (1991). Identification of a site in glutamate receptor subunits that controls calcium permeability. Science 253, 1028–1031. doi: 10.1126/science.1653450
Iacob, G., Dinca, E. B. (2009). Current data and strategy in glioblastoma multiforme. J. Med. Life 2, 386–393.
Ishiuchi, S., Tsuzuki, K., Yoshida, Y., Yamada, N., Hagimura, N., Okado, H., et al. (2002). Blockage of Ca(2+)-permeable AMPA receptors suppresses migration and induces apoptosis in human glioblastoma cells. Nat. Med. 8, 971–978. doi: 10.1038/nm746
Ishiuchi, S., Yoshida, Y., Sugawara, K., Aihara, M., Ohtani, T., Watanabe, T., et al. (2007). Ca2+-permeable AMPA receptors regulate growth of human glioblastoma via Akt activation. J. Neurosci. 27, 7987–8001. doi: 10.1523/JNEUROSCI.2180-07.2007
Jahn, R., Lang, T., Sudhof, T. C. (2003). Membrane fusion. Cell 112, 519–533. doi: 10.1016/S0092-8674(03)00112-0
Jurado, S., Goswami, D., Zhang, Y., Molina, A. J., Sudhof, T. C., Malenka, R. C. (2013). LTP requires a unique postsynaptic SNARE fusion machinery. Neuron 77, 542–558. doi: 10.1016/j.neuron.2012.11.029
Kaczmarek, L. K., Aldrich, R. W., Chandy, K. G., Grissmer, S., Wei, A. D., Wulff, H. (2017). International Union of Basic and Clinical Pharmacology. C. Nomenclature and Properties of Calcium-Activated and Sodium-Activated Potassium Channels. Pharmacol. Rev. 69, 1–11. doi: 10.1124/pr.116.012864
Kasperczyk, H., La Ferla-Brühl, K., Westhoff, M. A., Behrend, L., Zwacka, R. M., Debatin, K.-M., et al. (2005). Betulinic acid as new activator of NF-κB: molecular mechanisms and implications for cancer therapy. Oncogene 24, 6945–6956. doi: 10.1038/sj.onc.1208842
Kataria, H., Kumar, S., Chaudhary, H., Kaur, G. (2016). Withania somnifera Suppresses Tumor Growth of Intracranial Allograft of Glioma Cells. Mol. Neurobiol. 53, 4143–4158. doi: 10.1007/s12035-015-9320-1
Khan, M., Yu, B., Rasul, A., Al Shawi, A., Yi, F., Yang, H., et al. (2012). Jaceosidin induces apoptosis in U87 glioblastoma cells through G2/M phase arrest. Evidence-Based Complement. Altern. Med. 2012. doi: 10.1155/2012/703034
Khan, H. (2014). Medicinal plants in light of history: recognized therapeutic modality. J. Evidence-Based Complement. Altern. Med. 19, 216–219. doi: 10.1177/2156587214533346
Khaw, A. K., Sameni, S., Venkatesan, S., Kalthur, G., Hande, M. P. (2015). Plumbagin alters telomere dynamics, induces DNA damage and cell death in human brain tumour cells. Mutat. Research/Genetic Toxicol. Environ. Mutagenesis 793, 86–95. doi: 10.1016/j.mrgentox.2015.06.004
Kim, H., Moon, J. Y., Ahn, K. S., Cho, S. K. (2013). Quercetin induces mitochondrial mediated apoptosis and protective autophagy in human glioblastoma U373MG cells. Oxid. Med. Cell. Longevity 2013. doi: 10.1155/2013/596496
Kourghi, M., Pei, J. V., De Ieso, M. L., Flynn, G., Yool, A. J. (2016). Bumetanide derivatives AqB007 and AqB011 selectively block the aquaporin-1 ion channel conductance and slow cancer cell migration. Mol. Pharmacol. 89, 133–140. doi: 10.1124/mol.115.101618
Kourghi, M., De Ieso, M. L., Nourmohammadi, S., Pei, J. V., Yool, A. J. (2018). Identification of Loop D domain amino acids in the human aquaporin-1 channel involved in activation of the ionic conductance and inhibition by AqB011. Front. Chem. 6, 142. doi: 10.3389/fchem.2018.00142
Labrakakis, C., Patt, S., Hartmann, J., Kettenmann, H. (1998). Glutamate receptor activation can trigger electrical activity in human glioma cells. Eur. J. Neurosci. 10, 2153–2162. doi: 10.1046/j.1460-9568.1998.00226.x
Lai, I. C., Shih, P. H., Yao, C. J., Yeh, C. T., Wang-Peng, J., Lui, T. N., et al. (2015). Elimination of cancer stem-like cells and potentiation of temozolomide sensitivity by Honokiol in glioblastoma multiforme cells. PloS One 10, e0114830. doi: 10.1371/journal.pone.0114830
Laws, E. R., Goldberg, W. J., Bernstein, J. J. (1993). Migration of human malignant astrocytoma cells in the mammalian brain: Scherer revisited. J. Developm. Neurosci. 11 (5), 691–697. doi: 10.1016/0736-5748(93)90056-J
Langan, Y. M., Lucas, R., Jewell, H., Toublanc, N., Schaefer, H., Sander, J. W., et al. (2003). Talampanel, a new antiepileptic drug: single- and multiple-dose pharmacokinetics and initial 1-week experience in patients with chronic intractable epilepsy. Epilepsia 44, 46–53. doi: 10.1046/j.1528-1157.2003.128902.x
Lau, C. G., Takayasu, Y., Rodenas-Ruano, A., Paternain, A. V., Lerma, J., Bennett, M. V., et al. (2010). SNAP-25 is a target of protein kinase C phosphorylation critical to NMDA receptor trafficking. J. Neurosci. 30, 242–254. doi: 10.1523/JNEUROSCI.4933-08.2010
Lee, D.-Y., Lee, M.-K., Kim, G.-S., Noh, H.-J., Lee, M.-H. (2013). Brazilin inhibits growth and induces apoptosis in human glioblastoma cells. Molecules 18, 2449–2457. doi: 10.3390/molecules18022449
Lee, K., Goodman, L., Fourie, C., Schenk, S., Leitch, B., Montgomery, J. (2015). “AMPA Receptors as Therapeutic Targets for Neurological Disorders,” in Advances in Protein Chemistry and Structural Biology.
Lee, S. Y. (2016). Temozolomide resistance in glioblastoma multiforme. Genes Dis. 3, 198–210. doi: 10.1016/j.gendis.2016.04.007
Li, Y., Zhang, P., Qiu, F., Chen, L., Miao, C., Li, J., et al. (2012). Inactivation of PI3K/Akt signaling mediates proliferation inhibition and G2/M phase arrest induced by andrographolide in human glioblastoma cells. Life Sci. 90, 962–967. doi: 10.1016/j.lfs.2012.04.044
Li, H., Liu, Y., Jiao, Y., Guo, A., Xu, X., Qu, X., et al. (2016a). Resveratrol sensitizes glioblastoma-initiating cells to temozolomide by inducing cell apoptosis and promoting differentiation. Oncol. Rep. 35, 343–351. doi: 10.3892/or.2015.4346
Li, Y., Lian, X., Wan, Y., Wang, D., Chen, W., Di, F., et al. (2016b). Modulation of the Ca(2+) signaling pathway by celangulin I in the central neurons of Spodoptera exigua. Pestic Biochem. Physiol. 127, 76–81. doi: 10.1016/j.pestbp.2015.09.010
Liao, M.-H., Lin, W.-C., Wen, H.-C., Pu, H.-F. (2011). Tithonia diversifolia and its main active component tagitinin C induce survivin inhibition and G2/M arrest in human malignant glioblastoma cells. Fitoterapia 82, 331–341. doi: 10.1016/j.fitote.2010.11.002
Lin, H., Xiong, W., Zhang, X., Liu, B., Zhang, W., Zhang, Y., et al. (2011). Notch-1 activation-dependent p53 restoration contributes to resveratrol-induced apoptosis in glioblastoma cells. Oncol. Rep. 26, 925–930. doi: 10.3892/or.2011.1380
Lin, K., Gao, Z., Shang, B., Sui, S., Fu, Q. (2015). Osthole suppresses the proliferation and accelerates the apoptosis of human glioma cells via the upregulation of microRNA-16 and downregulation of MMP-9. Mol. Med. Rep. 12, 4592–4597. doi: 10.3892/mmr.2015.3929
Liu, Q., Xu, X., Zhao, M., Wei, Z., Li, X., Zhang, X., et al. (2015). Berberine induces senescence of human glioblastoma cells by downregulating the EGFR–MEK–ERK signaling pathway. Mol. Cancer Ther. 14, 355–363. doi: 10.1158/1535-7163.MCT-14-0634
Lonardi, S., Tosoni, A., Brandes, A. A. (2005). Adjuvant chemotherapy in the treatment of high grade gliomas. Cancer Treat Rev. 31, 79–89. doi: 10.1016/j.ctrv.2004.12.005
Louis, D. N., Perry, A., Reifenberger, G., Von Deimling, A., Figarella-Branger, D., Cavenee, W. K., et al. (2016). The 2016 World Health Organization Classification of Tumors of the Central Nervous System: a summary. Acta Neuropathol. 131, 803–820. doi: 10.1007/s00401-016-1545-1
Lv, L., Zheng, L., Dong, D., Xu, L., Yin, L., Xu, Y., et al. (2013). Dioscin, a natural steroid saponin, induces apoptosis and DNA damage through reactive oxygen species: a potential new drug for treatment of glioblastoma multiforme. Food Chem. Toxicol. 59, 657–669. doi: 10.1016/j.fct.2013.07.012
Lyons, S. A., O'neal, J., Sontheimer, H. (2002). Chlorotoxin, a scorpion-derived peptide, specifically binds to gliomas and tumors of neuroectodermal origin. Glia 39, 162–173. doi: 10.1002/glia.10083
Lyons, S. A., Chung, W. J., Weaver, A. K., Ogunrinu, T., Sontheimer, H. (2007). Autocrine glutamate signaling promotes glioma cell invasion. Cancer Res. 67, 9463–9471. doi: 10.1158/0008-5472.CAN-07-2034
Ma, J.-W., Zhang, Y., Ye, J.-C., Li, R., Wen, Y.-L., Huang, J.-X., et al. (2017). Tetrandrine exerts a radiosensitization effect on human glioma through inhibiting proliferation by attenuating ERK phosphorylation. Biomol. Ther. 25, 186. doi: 10.4062/biomolther.2016.044
Maas, S., Patt, S., Schrey, M., Rich, A. (2001). Underediting of glutamate receptor GluR-B mRNA in malignant gliomas. Proc. Natl. Acad. Sci. U. S. A 98, 14687–14692. doi: 10.1073/pnas.251531398
Malacrida, A., Rivara, M., Di Domizio, A., Cislaghi, G., Miloso, M., Zuliani, V., et al. (2020). 3D proteome-wide scale screening and activity evaluation of a new ALKBH5 inhibitor in U87 glioblastoma cell line. Bioorg. Med. Chem. 28, 115300. doi: 10.1016/j.bmc.2019.115300
Mann, J., Ramakrishna, R., Magge, R., Wernicke, A. G. (2017). Advances in Radiotherapy for Glioblastoma. Front. Neurol. 8, 748. doi: 10.3389/fneur.2017.00748
Manna, S. K., Mukhopadhyay, A., Aggarwal, B. B. (2000). Resveratrol suppresses TNF-induced activation of nuclear transcription factors NF-κB, activator protein-1, and apoptosis: potential role of reactive oxygen intermediates and lipid peroxidation. J. Immunol. 164, 6509–6519. doi: 10.4049/jimmunol.164.12.6509
Marchetti, C., Clericuzio, M., Borghesi, B., Cornara, L., Ribulla, S., Gosetti, F., et al. (2015). Oleuropein-Enriched Olive Leaf Extract Affects Calcium Dynamics and Impairs Viability of Malignant Mesothelioma Cells. Evid. Based Complement. Alternat. Med. 2015, 908493. doi: 10.1155/2015/908493
Marchetti, C., Ribulla, S., Magnelli, V., Patrone, M., Burlando, B. (2016). Resveratrol induces intracellular Ca(2+) rise via T-type Ca(2+) channels in a mesothelioma cell line. Life Sci. 148, 125–131. doi: 10.1016/j.lfs.2016.01.048
Marcu, J. P., Christian, R. T., Lau, D., Zielinski, A. J., Horowitz, M. P., Lee, J., et al. (2010). Cannabidiol enhances the inhibitory effects of Δ9-tetrahydrocannabinol on human glioblastoma cell proliferation and survival. Mol. Cancer Ther. 9, 180–189. doi: 10.1158/1535-7163.MCT-09-0407
Mc Allister, S. D., Chan, C., Taft, R. J., Luu, T., Abood, M. E., Moore, D. H., et al. (2005). Cannabinoids selectively inhibit proliferation and induce death of cultured human glioblastoma multiforme cells. J. Neuro-oncol. 74, 31–40. doi: 10.1007/s11060-004-5950-2
McCoy, E., Sontheimer, H. (2007). Expression and function of water channels (aquaporins) in migrating malignant astrocytes. Glia 55, 1034–1043. doi: 10.1002/glia.20524
McLennan, R., Mckinney, M. C., Teddy, J. M., Morrison, J. A., Kasemeier-Kulesa, J. C., Ridenour, D. A., et al. (2020). Neural crest cells bulldoze through the microenvironment using Aquaporin 1 to stabilize filopodia. Development 147. doi: 10.1242/dev.185231
McMahon, H. T., Bolshakov, V. Y., Janz, R., Hammer, R. E., Siegelbaum, S. A., Sudhof, T. C. (1996). Synaptophysin, a major synaptic vesicle protein, is not essential for neurotransmitter release. Proc. Natl. Acad. Sci. U. S. A 93, 4760–4764. doi: 10.1073/pnas.93.10.4760
Michaud-Levesque, J., Bousquet-Gagnon, N., Béliveau, R. (2012). Quercetin abrogates IL-6/STAT3 signaling and inhibits glioblastoma cell line growth and migration. Exp. Cell Res. 318, 925–935. doi: 10.1016/j.yexcr.2012.02.017
Molenaar, R. J. (2011). Ion channels in glioblastoma. ISRN Neurol. 2011, 590249. doi: 10.5402/2011/590249
Nagelhus, E. A., Mathiisen, T. M., Ottersen, O. P. (2004). Aquaporin-4 in the central nervous system: cellular and subcellular distribution and coexpression with KIR4.1. Neuroscience 129, 905–913. doi: 10.1016/j.neuroscience.2004.08.053
Nakhjavani, M., Palethorpe, H. M., Tomita, Y., Smith, E., Price, T. J., Yool, A. J., et al. (2019). Stereoselective Anti-Cancer Activities of Ginsenoside Rg3 on Triple Negative Breast Cancer Cell Models. Pharmaceut. (Basel) 12. doi: 10.3390/ph12030117
Nie, X.-H., Ou-Yang, J., Xing, Y., Li, D.-Y., Dong, X.-Y., Liu, R.-E., et al. (2015). Paeoniflorin inhibits human glioma cells via STAT3 degradation by the ubiquitin–proteasome pathway. Drug Design Dev. Ther. 9, 5611. doi: 10.2147/DDDT.S93912
Nourmohammadi, S., Aung, T. N., Cui, J., Pei, J. V., De Ieso, M. L., Harata-Lee, Y., et al. (2019). Effect of Compound Kushen Injection, a Natural Compound Mixture, and Its Identified Chemical Components on Migration and Invasion of Colon, Brain, and Breast Cancer Cell Lines. Front. Oncol. 9, 314. doi: 10.3389/fonc.2019.00314
Oh, S. J., Lee, C. J. (2017). Distribution and Function of the Bestrophin-1 (Best1) Channel in the Brain. Exp. Neurobiol. 26, 113–121. doi: 10.5607/en.2017.26.3.113
Okonogi, N., Shirai, K., Oike, T., Murata, K., Noda, S. E., Suzuki, Y., et al. (2015). Topics in chemotherapy, molecular-targeted therapy, and immunotherapy for newly-diagnosed glioblastoma multiforme. Anticancer Res. 35, 1229–1235.
Olivan-Viguera, A., Valero, M. S., Murillo, M. D., Wulff, H., Garcia-Otin, A. L., Arbones-Mainar, J. M., et al. (2013). Novel phenolic inhibitors of small/intermediate-conductance Ca(2)(+)-activated K(+) channels, KCa3.1 and KCa2.3. PloS One 8, e58614. doi: 10.1371/journal.pone.0058614
Olsen, M. L., Schade, S., Lyons, S. A., Amaral, M. D., Sontheimer, H. (2003). Expression of voltage-gated chloride channels in human glioma cells. J. Neurosci. 23, 5572–5582. doi: 10.1523/JNEUROSCI.23-13-05572.2003
Pan, X., Chen, J., Wang, W., Chen, L., Wang, L., Ma, Q., et al. (2017). Resveratrol-induced antinociception is involved in calcium channels and calcium/caffeine-sensitive pools. Oncotarget 8, 9399–9409. doi: 10.18632/oncotarget.14090
Park, M., Song, C., Yoon, H., Choi, K. H. (2016). Double Blockade of Glioma Cell Proliferation and Migration by Temozolomide Conjugated with NPPB, a Chloride Channel Blocker. ACS Chem. Neurosci. 7, 275–285. doi: 10.1021/acschemneuro.5b00178
Park, M. N., Song, H. S., Kim, M., Lee, M.-J., Cho, W., Lee, H.-J., et al. (2017). Review of natural product-derived compounds as potent antiglioblastoma drugs. BioMed. Res. Int. 2017. doi: 10.1155/2017/8139848
Pei, J. V., Burton, J. L., Kourghi, M., De Ieso, M. L., Yool, A. J. (2016a). “Drug discovery and therapeutic targets for pharmacological modulators of aquaporin channels,” in Aquaporins in Health and Disease: New Molecular Targets For Drug Discovery. Eds. Soveral, G., Casinin, A., Nielsen, S. (Oxfordshire, UK: CRC Press), 275–297.
Pei, J. V., Kourghi, M., De Ieso, M. L., Campbell, E. M., Dorward, H. S., Hardingham, J. E., et al. (2016b). Differential inhibition of water and ion channel activities of mammalian aquaporin-1 by two structurally related bacopaside compounds derived from the medicinal plant Bacopa monnieri. Mol. Pharmacol. 90, 496–507. doi: 10.1124/mol.116.105882
Pei, J. V., Heng, S., De Ieso, M. L., Sylvia, G., Kourghi, M., Nourmohammadi, S., et al. (2019). Development of a photoswitchable lithium-sensitive probe to analyze nonselective cation channel activity in migrating cancer cells. Mol. Pharmacol. 95, 573–583. doi: 10.1124/mol.118.115428
Perry, M. C., Demeule, M., Régina, A., Moumdjian, R., Béliveau, R. (2010). Curcumin inhibits tumor growth and angiogenesis in glioblastoma xenografts. Mol. Nutr. Food Res. 54, 1192–1201. doi: 10.1002/mnfr.200900277
Piao, Y., Lu, L., De Groot, J. (2009). AMPA receptors promote perivascular glioma invasion via beta1 integrin-dependent adhesion to the extracellular matrix. Neuro Oncol. 11, 260–273. doi: 10.1215/15228517-2008-094
Puchalski, R. B., Shah, N., Miller, J., Dalley, R., Nomura, S. R., Yoon, J. G., et al. (2018). An anatomic transcriptional atlas of human glioblastoma. Science 360, 660–663. doi: 10.1126/science.aaf2666
Racoma, I. O., Meisen, W. H., Wang, Q.-E., Kaur, B., Wani, A. A. (2013). Thymoquinone inhibits autophagy and induces cathepsin-mediated, caspase-independent cell death in glioblastoma cells. PloS One 8, e72882. doi: 10.1371/journal.pone.0072882
Rash, J. E., Yasumura, T., Davidson, K. G., Furman, C. S., Dudek, F. E., Nagy, J. I. (2001). Identification of cells expressing Cx43, Cx30, Cx26, Cx32 and Cx36 in gap junctions of rat brain and spinal cord. Cell Commun. Adhes 8, 315–320. doi: 10.3109/15419060109080745
Ribeiro, M. P., Custodio, J. B., Santos, A. E. (2017). Ionotropic glutamate receptor antagonists and cancer therapy: time to think out of the box? Cancer Chemother. Pharmacol. 79, 219–225. doi: 10.1007/s00280-016-3129-0
Robert, S. M., Sontheimer, H. (2014). Glutamate transporters in the biology of malignant gliomas. Cell Mol. Life Sci. 71, 1839–1854. doi: 10.1007/s00018-013-1521-z
Ryu, J., Ku, B. M., Lee, Y. K., Jeong, J. Y., Kang, S., Choi, J., et al. (2011). Resveratrol reduces TNF-alpha-induced U373MG human glioma cell invasion through regulating NF-kappaB activation and uPA/uPAR expression. Anticancer Res. 31, 4223–4230.
Sang, D.-P., Li, R.-J., Lan, Q. (2014). Quercetin sensitizes human glioblastoma cells to temozolomide in vitro via inhibition of Hsp27. Acta Pharmacol Sin. 35, 832. doi: 10.1038/aps.2014.22
Scheffler, A., Rauwald, H. W., Kampa, B., Mann, U., Mohr, F. W., Dhein, S. (2008). Olea europaea leaf extract exerts L-type Ca(2+) channel antagonistic effects. J. Ethnopharmacol. 120, 233–240. doi: 10.1016/j.jep.2008.08.018
Scherer, H. J. (1940). A Critical Review: The Pathology of Cerebral Gliomas. J. Neurol. Psychiatry 3, 147–177. doi: 10.1136/jnnp.3.2.147
Schirmer, L., Mobius, W., Zhao, C., Cruz-Herranz, A., Ben Haim, L., Cordano, C., et al. (2018). Oligodendrocyte-encoded Kir4.1 function is required for axonal integrity. Elife 11 (7), e36428. doi: 10.7554/eLife.36428
Schwechheimer, K., Wiedenmann, B., Franke, W. W. (1987). Synaptophysin: a reliable marker for medulloblastomas. Virchows Arch. A Pathol. Anat. Histopathol. 411, 53–59. doi: 10.1007/BF00734514
Selak, S., Paternain, A. V., Aller, M. I., Pico, E., Rivera, R., Lerma, J. (2009). A role for SNAP25 in internalization of kainate receptors and synaptic plasticity. Neuron 63, 357–371. doi: 10.1016/j.neuron.2009.07.017
Sengupta, R., Barone, A., Marasa, J., Taylor, S., Jackson, E., Warrington, N. M., et al. (2015). Novel chemical library screen identifies naturally occurring plant products that specifically disrupt glioblastoma-endothelial cell interactions. Oncotarget 6, 18282. doi: 10.18632/oncotarget.4957
Shah, N., Kataria, H., Kaul, S. C., Ishii, T., Kaur, G., Wadhwa, R. (2009). Effect of the alcoholic extract of Ashwagandha leaves and its components on proliferation, migration, and differentiation of glioblastoma cells: combinational approach for enhanced differentiation. Cancer Sci. 100, 1740–1747. doi: 10.1111/j.1349-7006.2009.01236.x
Singh, S. K., Hawkins, C., Clarke, I. D., Squire, J. A., Bayani, J., Hide, T., et al. (2004). Identification of human brain tumour initiating cells. Nature 432, 396–401. doi: 10.1038/nature03128
Snutch, T. P., Peloquin, J., Mathews, E., Mcrory, J. E. (2000-2013). “Molecular Properties of Voltage-Gated Calcium Channels,” in Madame Curie Bioscience Database. Ed. Bookshelf, N. (Austin (TX): Landes Bioscience).
Sontheimer, H. (2008). A role for glutamate in growth and invasion of primary brain tumors. J. Neurochem. 105, 287–295. doi: 10.1111/j.1471-4159.2008.05301.x
Soroceanu, L., Manning, T. J., Jr., Sontheimer, H. (1999). Modulation of glioma cell migration and invasion using Cl(-) and K(+) ion channel blockers. J. Neurosci. 19, 5942–5954. doi: 10.1523/JNEUROSCI.19-14-05942.1999
Stephen, Z. R., Chiarelli, P. A., Revia, R. A., Wang, K., Kievit, F., Dayringer, C., et al. (2019). Time-Resolved MRI Assessment of Convection-Enhanced Delivery by Targeted and Nontargeted Nanoparticles in a Human Glioblastoma Mouse Model. Cancer Res. 79, 4776–4786. doi: 10.1158/0008-5472.CAN-18-2998
Stupp, R., Mason, W. P., Van Den Bent, M. J., Weller, M., Fisher, B., Taphoorn, M. J., et al. (2005). Radiotherapy plus concomitant and adjuvant temozolomide for glioblastoma. N. Engl. J. Med. 352, 987–996. doi: 10.1056/NEJMoa043330
Sun, C., Yu, Y., Wang, L., Wu, B., Xia, L., Feng, F., et al. (2016a). Additive antiangiogenesis effect of ginsenoside Rg3 with low-dose metronomic temozolomide on rat glioma cells both in vivo and in vitro. J. Exp. Clin. Cancer Res. 35, 32. doi: 10.1186/s13046-015-0274-y
Sun, C., Yu, Y., Wang, L., Wu, B., Xia, L., Feng, F., et al. (2016b). Additive antiangiogenesis effect of ginsenoside Rg3 with low-dose metronomic temozolomide on rat glioma cells both in vivo and in vitro. J. Exp. Clin. Cancer Res. 35, 32. doi: 10.1186/s13046-015-0274-y
Tezcan, G., Taskapilioglu, M. O., Tunca, B., Bekar, A., Demirci, H., Kocaeli, H., et al. (2017a). Olea europaea leaf extract and bevacizumab synergistically exhibit beneficial efficacy upon human glioblastoma cancer stem cells through reducing angiogenesis and invasion in vitro. BioMed. Pharmacother. 90, 713–723. doi: 10.1016/j.biopha.2017.04.022
Tezcan, G., Tunca, B., Demirci, H., Bekar, A., Taskapilioglu, M. O., Kocaeli, H., et al. (2017b). Olea europaea leaf extract improves the efficacy of temozolomide therapy by inducing MGMT methylation and reducing P53 expression in glioblastoma. Nutr. Cancer 69, 873–880. doi: 10.1080/01635581.2017.1339810
Thani, A., Azela, N., Sallis, B., Nuttall, R., Schubert, F. R., Ahsan, M., et al. (2012). Induction of apoptosis and reduction of MMP gene expression in the U373 cell line by polyphenolics in Aronia melanocarpa and by curcumin. Oncol. Rep. 28, 1435–1442. doi: 10.3892/or.2012.1941
Tomita, S., Chen, L., Kawasaki, Y., Petralia, R. S., Wenthold, R. J., Nicoll, R. A., et al. (2003). Functional studies and distribution define a family of transmembrane AMPA receptor regulatory proteins. J. Cell Biol. 161, 805–816. doi: 10.1083/jcb.200212116
Tomita, Y., Palethorpe, H. M., Smith, E., Nakhjavani, M., Townsend, A. R., Price, T. J., et al. (2019). Bumetanide-Derived Aquaporin 1 Inhibitors, AqB013 and AqB050 Inhibit Tube Formation of Endothelial Cells through Induction of Apoptosis and Impaired Migration In Vitro. Int. J. Mol. Sci. 20 (8), E1818. doi: 10.3390/ijms20081818
Traynelis, S. F., Wollmuth, L. P., Mcbain, C. J., Menniti, F. S., Vance, K. M., Ogden, K. K., et al. (2010). Glutamate receptor ion channels: structure, regulation, and function. Pharmacol. Rev. 62, 405–496. doi: 10.1124/pr.109.002451
Tsai, N. M., Lin, S. Z., Lee, C. C., Chen, S. P., Su, H. C., Chang, W. L., et al. (2005). The antitumor effects of Angelica sinensis on malignant brain tumors in vitro and in vivo. Clin. Cancer Res. 11, 3475–3484. doi: 10.1158/1078-0432.CCR-04-1827
Tunca, B., Tezcan, G., Cecener, G., Egeli, U., Ak, S., Malyer, H., et al. (2012). Olea europaea leaf extract alters microRNA expression in human glioblastoma cells. J. Cancer Res. Clin. Oncol. 138, 1831–1844. doi: 10.1007/s00432-012-1261-8
Uhlen, M., Bjorling, E., Agaton, C., Szigyarto, C. A., Amini, B., Andersen, E., et al. (2005). A human protein atlas for normal and cancer tissues based on antibody proteomics. Mol. Cell Proteomics 4, 1920–1932. doi: 10.1074/mcp.M500279-MCP200
Uhlen, M., Zhang, C., Lee, S., Sjostedt, E., Fagerberg, L., Bidkhori, G., et al. (2017). A pathology atlas of the human cancer transcriptome. Science 357 (6352), eaan2507. doi: 10.1126/science.aan2507
Vanoye-Carlo, A., Gomez-Lira, G. (2019). Differential expression of SV2A in hippocampal glutamatergic and GABAergic terminals during postnatal development. Brain Res. 1715, 73–83. doi: 10.1016/j.brainres.2019.03.021
Venkataramani, V., Tanev, D. I., Strahle, C., Studier-Fischer, A., Fankhauser, L., Kessler, T., et al. (2019). Glutamatergic synaptic input to glioma cells drives brain tumour progression. Nature 573, 532–538. doi: 10.1038/s41586-019-1564-x
Venkatesan, K., Alix, P., Marquet, A., Doupagne, M., Niespodziany, I., Rogister, B., et al. (2012). Altered balance between excitatory and inhibitory inputs onto CA1 pyramidal neurons from SV2A-deficient but not SV2B-deficient mice. J. Neurosci. Res. 90, 2317–2327. doi: 10.1002/jnr.23111
Venkatesh, H. S., Morishita, W., Geraghty, A. C., Silverbush, D., Gillespie, S. M., Arzt, M., et al. (2019). Electrical and synaptic integration of glioma into neural circuits. Nature 573, 539–545. doi: 10.1038/s41586-019-1563-y
Verdoorn, T. A., Burnashev, N., Monyer, H., Seeburg, P. H., Sakmann, B. (1991). Structural determinants of ion flow through recombinant glutamate receptor channels. Science 252, 1715–1718. doi: 10.1126/science.1710829
Verhaak, R. G., Valk, P. J. (2010). Genes predictive of outcome and novel molecular classification schemes in adult acute myeloid leukemia. Cancer Treat Res. 145, 67–83. doi: 10.1007/978-0-387-69259-3_5
Verhaak, R. G., Hoadley, K. A., Purdom, E., Wang, V., Qi, Y., Wilkerson, M. D., et al. (2010). Integrated genomic analysis identifies clinically relevant subtypes of glioblastoma characterized by abnormalities in PDGFRA, IDH1, EGFR, and NF1. Cancer Cell 17, 98–110. doi: 10.1016/j.ccr.2009.12.020
Verhaak, R. G. (2016). Moving the needle: Optimizing classification for glioma. Sci. Transl. Med. 8, 350fs314. doi: 10.1126/scitranslmed.aah4740
Walker, M. D., Hellman, K., Connors, T. A. (1976). “Chemotherapy of CNS Tumors,” in Chemotherapy (New York: Plenum Press), 271–275.
Wang, X., Duan, X., Yang, G., Zhang, X., Deng, L., Zheng, H., et al. (2011). Honokiol crosses BBB and BCSFB, and inhibits brain tumor growth in rat 9L intracerebral gliosarcoma model and human U251 xenograft glioma model. PloS One 6, e18490. doi: 10.1371/journal.pone.0018490
Wang, C., Kang, X., Zhou, L., Chai, Z., Wu, Q., Huang, R., et al. (2018a). Synaptotagmin-11 is a critical mediator of parkin-linked neurotoxicity and Parkinson's disease-like pathology. Nat. Commun. 9, 81. doi: 10.1038/s41467-017-02593-y
Wang, Z., Liu, Z., Yu, G., Nie, X., Jia, W., Liu, R.-E., et al. (2018b). Paeoniflorin Inhibits Migration and Invasion of Human Glioblastoma Cells via Suppression Transforming Growth Factor β-Induced Epithelial–Mesenchymal Transition. Neurochem. Res. 43, 760–774. doi: 10.1007/s11064-018-2478-y
Welch, D. R., Hurst, D. R. (2019). Defining the Hallmarks of Metastasis. Cancer Res. 79, 3011–3027. doi: 10.1158/0008-5472.CAN-19-0458
Wick, W., Grimmel, C., Wagenknecht, B., Dichgans, J., Weller, M. (1999). Betulinic acid-induced apoptosis in glioma cells: A sequential requirement for new protein synthesis, formation of reactive oxygen species, and caspase processing. J. Pharmacol. Exp. Ther. 289, 1306–1312.
Wong, R., Turlova, E., Feng, Z. P., Rutka, J. T., Sun, H. S. (2017). Activation of TRPM7 by naltriben enhances migration and invasion of glioblastoma cells. Oncotarget 8, 11239–11248. doi: 10.18632/oncotarget.14496
Yamazaki, M., Fukaya, M., Hashimoto, K., Yamasaki, M., Tsujita, M., Itakura, M., et al. (2010). TARPs gamma-2 and gamma-7 are essential for AMPA receptor expression in the cerebellum. Eur. J. Neurosci. 31, 2204–2220. doi: 10.1111/j.1460-9568.2010.07254.x
Yang, L., Wang, Y., Guo, H., Guo, M. (2015). Synergistic anti-cancer effects of icariin and temozolomide in glioblastoma. Cell Biochem. Biophys. 71, 1379–1385. doi: 10.1007/s12013-014-0360-3
Yin, D., Wakimoto, N., Xing, H., Lu, D., Huynh, T., Wang, X., et al. (2008). Cucurbitacin B markedly inhibits growth and rapidly affects the cytoskeleton in glioblastoma multiforme. Int. J. Cancer 123, 1364–1375. doi: 10.1002/ijc.23648
Yin, H., Zhou, Y., Wen, C., Zhou, C., Zhang, W., Hu, X., et al. (2014). Curcumin sensitizes glioblastoma to temozolomide by simultaneously generating ROS and disrupting AKT/mTOR signaling. Oncol. Rep. 32, 1610–1616. doi: 10.3892/or.2014.3342
Yokoyama, S., Hirano, H., Wakimaru, N., Sarker, K. P., Kuratsu, J.-I. (2001). Inhibitory effect of epigallocatechin-gallate on brain tumor cell lines in vitro. Neuro-oncology 3, 22–28. doi: 10.1093/neuonc/3.1.22
Yool, A. J., Brown, E. A., Flynn, G. A. (2009). Roles for novel pharmacological blockers of aquaporins in the treatment of brain oedema and cancer. Clin. Exp. Pharmacol. Physiol. 37, 403–409. doi: 10.1111/j.1440-1681.2009.05244.x
Yool, A. J. (2007). Aquaporins: multiple roles in the central nervous system. Neuroscientist 13, 470–485. doi: 10.1177/1073858407303081
Yu, J., Yool, A. J., Schulten, K., Tajkhorshid, E. (2006). Mechanism of gating and ion conductivity of a possible tetrameric pore in aquaporin-1. Structure 14, 1411–1423. doi: 10.1016/j.str.2006.07.006
Yu, G., Wang, Z., Zeng, S., Liu, S., Zhu, C., Xu, R., et al. (2019). Paeoniflorin Inhibits Hepatocyte Growth Factor-(HGF-) Induced Migration and Invasion and Actin Rearrangement via Suppression of c-Met-Mediated RhoA/ROCK Signaling in Glioblastoma. BioMed. Res. Int. 2019, 9053295. doi: 10.1155/2019/9053295
Yuan, Y., Xue, X., Guo, R. B., Sun, X. L., Hu, G. (2012). Resveratrol enhances the antitumor effects of temozolomide in glioblastoma via ROS-dependent AMPK-TSC-mTOR signaling pathway. CNS Neurosci. Ther. 18, 536–546. doi: 10.1111/j.1755-5949.2012.00319.x
Zare, L., Esmaeili-Mahani, S., Abbasnejad, M., Rasoulian, B., Sheibani, V., Sahraei, H., et al. (2012). Oleuropein, chief constituent of olive leaf extract, prevents the development of morphine antinociceptive tolerance through inhibition of morphine-induced L-type calcium channel overexpression. Phytother. Res. 26, 1731–1737. doi: 10.1002/ptr.4634
Zhang, L. P., Yin, J. X., Liu, Z., Zhang, Y., Wang, Q. S., Zhao, J. (2006). Effect of resveratrol on L-type calcium current in rat ventricular myocytes. Acta Pharmacol. Sin. 27, 179–183. doi: 10.1111/j.1745-7254.2006.00250.x
Zhang, X. J., Chen, H. L., Li, Z., Zhang, H. Q., Xu, H. X., Sung, J. J., et al. (2009). Analgesic effect of paeoniflorin in rats with neonatal maternal separation-induced visceral hyperalgesia is mediated through adenosine A(1) receptor by inhibiting the extracellular signal-regulated protein kinase (ERK) pathway. Pharmacol. Biochem. Behav. 94, 88–97. doi: 10.1016/j.pbb.2009.07.013
Zhang, Y., Wang, S. X., Ma, J. W., Li, H. Y., Ye, J. C., Xie, S. M., et al. (2015). EGCG inhibits properties of glioma stem-like cells and synergizes with temozolomide through downregulation of P-glycoprotein inhibition. J. Neurooncol. 121, 41–52. doi: 10.1007/s11060-014-1604-1
Keywords: ionotropic glutamate receptor, synaptic protein expression, transcriptomic, ion channels, traditional herbal medicines, aquaporin, glioblastoma
Citation: Yool AJ and Ramesh S (2020) Molecular Targets for Combined Therapeutic Strategies to Limit Glioblastoma Cell Migration and Invasion. Front. Pharmacol. 11:358. doi: 10.3389/fphar.2020.00358
Received: 18 December 2019; Accepted: 10 March 2020;
Published: 27 March 2020.
Edited by:
Jiang-Jiang Qin, Zhejiang Chinese Medical University, ChinaReviewed by:
Denisa Margina, Carol Davila University of Medicine and Pharmacy, RomaniaRyan Louis O‘Hare Doig, South Australian Health and Medical Research Institute, Australia
Cornelia Braicu, Iuliu Hațieganu University of Medicine and Pharmacy, Romania
Copyright © 2020 Yool and Ramesh. This is an open-access article distributed under the terms of the Creative Commons Attribution License (CC BY). The use, distribution or reproduction in other forums is permitted, provided the original author(s) and the copyright owner(s) are credited and that the original publication in this journal is cited, in accordance with accepted academic practice. No use, distribution or reproduction is permitted which does not comply with these terms.
*Correspondence: Andrea Yool, andrea.yool@adelaide.edu.au