The degeneration-pain relationship in the temporomandibular joint: Current understandings and rodent models
- Department of Neural and Pain Sciences, School of Dentistry, Program in Neuroscience, Center to Advance Chronic Pain Research, University of Maryland Baltimore, Baltimore, MD, United States
Temporomandibular disorders (TMD) represent a group of musculoskeletal conditions involving the temporomandibular joints (TMJ), the masticatory muscles and associated structures. Painful TMD are highly prevalent and conditions afflict 4% of US adults annually. TMD include heterogenous musculoskeletal pain conditions, such as myalgia, arthralgia, and myofascial pain. A subpopulations of TMD patients show structural changes in TMJ, including disc displacement or degenerative joint diseases (DJD). DJD is a slowly progressing, degenerative disease of the TMJ characterized by cartilage degradation and subchondral bone remodeling. Patients with DJD often develop pain (TMJ osteoarthritis; TMJ OA), but do not always have pain (TMJ osteoarthrosis). Therefore, pain symptoms are not always associated with altered TMJ structures, which suggests that a causal relationship between TMJ degeneration and pain is unclear. Multiple animal models have been developed for determining altered joint structure and pain phenotypes in response to various TMJ injuries. Rodent models of TMJOA and pain include injections to induce inflammation or cartilage destruction, sustained opening of the oral cavity, surgical resection of the articular disc, transgenic approaches to knockout or overexpress key genes, and an integrative approach with superimposed emotional stress or comorbidities. In rodents, TMJ pain and degeneration occur during partially overlapping time periods in these models, which suggests that common biological factors may mediate TMJ pain and degeneration over different time courses. While substances such as intra-articular pro-inflammatory cytokines commonly cause pain and joint degeneration, it remains unclear whether pain or nociceptive activities are causally associated with structural degeneration of TMJ and whether structural degeneration of TMJ is necessary for producing persistent pain. A thorough understanding of the determining factors of pain-structure relationships of TMJ during the onset, progression, and chronification by adopting novel approaches and models should improve the ability to simultaneously treat TMJ pain and TMJ degeneration.
Introduction
Orofacial pain is a highly prevalent condition. Approximately 15% of the general population experiences various orofacial pain symptoms, such as dento-alveolar pain, tenderness of the temporomandibular joint (TMJ) or masticatory muscles, pain with TMJ biomechanics or pre-auricular pain (1, 2). Temporomandibular disorders (TMD) represent heterogeneous musculoskeletal disorders involving pain conditions, such as myalgia, arthralgia, and myofascial pain, and structural changes, such as disc displacement and joint degeneration (3). A retrospective study involving 4,528 care-seeking TMD patients found that facial pain, ear discomfort, and discomfort or tenderness of the TMJ are typical presenting signs and symptoms of TMD (4). According to the OPPERA (Orofacial Pain: Prospective Evaluation and Risk Assessment) study, 260 people among 2,737 adults aged between 18 and 44 in the United States developed initial TMD during a 2.8 year follow-up period, indicating an incidence of 4% per annum (5). TMD is a multifactorial disease and the mechanisms underlying TMD pain and discomfort are not clearly understood.
TMD also include joint disorders, such as disc displacement and degenerative joint disease (DJD) (3). DJD is defined as a condition whereby articular cartilage deteriorates and the underlying subchondral bone undergoes concomitant remodeling. Radiographic and clinical examinations have shown that approximately 25%–55% of TMD patients have degenerative changes in the TMJ (6–10). DJD includes osteoarthritis and osteoarthrosis. TMJ osteoarthritis (TMJ OA) indicates degenerative changes with pain in the joint whereas TMJ osteoarthrosis is a degenerative condition without any pain-related symptoms. Degenerative changes are more prevalent in female by two folds and increase with aging (9, 11), and could be related to trauma and joint overloading or might be secondary to disc displacement (8, 12–14). However, the majority of TMJOA has a complicated and multiple etiology or is simply idiopathic. Current conservative therapy is successful in managing symptoms, restoring functions, and improving TMJOA (15). However, therapeutic approaches for regenerating the TMJ structures are limited due to a lack of understanding of its pathophysiology and the low healing ability of avascular cartilage (14, 16). It is not clearly known if peripheral pathology, i.e., TMJOA-associated degenerative changes, contributes to TMJ pain. DJD is not likely correlated with the entire spectrum of TMD pain (e.g., myalgia). However, pain from TMJ (arthralgia) is associated with DJD and joint pain is associated with a poor prognosis of TMJOA treatment (15, 17). Therefore, a clear mechanistic understanding of the causal factors associated with joint pain and degeneration should improve our ability to effectively manage the subpopulation of TMD patients with joint pain and TMJOA. In this review, we will focus on the relationship of TMJ degeneration and pain from TMJ rather than other painful TMD conditions, such as myalgia or myofascial pain.
Degenerative changes and pain in patients with TMJOA
Degenerative changes in the TMJ are highly prevalent in TMD patients (6–10). Radiographic examinations have shown that TMJOA involves the condylar head, articular eminence, and glenoid fossa. Condylar flattening and articular surface erosion are the most commonly observed changes in TMJOA patients (18, 19). While subcortical cysts, surface erosion, osteophytes, or generalized sclerosis are considered as positive criteria for diagnosing TMJOA, flattening of the articular surface and subcortical sclerosis are indeterminate, meaning, an indication of variation of normal (20).
Patients with TMJOA show poorer oral health-related quality of life than healthy individuals (21). Pain from the TMJ is a common and problematic symptom in TMJOA and is more prevalent in females and older adults (22). Self-reported pain from patients with TMJOA is described as dull, heavy, troublesome, and tiring although these are not specific to TMJOA but also reported in other subtypes of TMD patients (22). Pain in the patients with TMJOA often persists and the average pain duration is 6 years (1–408 months) in a study of patients with TMJOA seeking treatment (23). A common symptom of TMJOA is tenderness upon joint palpation. During quantitative sensory testing of the skin overlying the TMJ, TMJOA patients demonstrated distinct sensory phenotypes compared to patients with TMJ pain without degeneration (arthralgia) (24). Specifically, patients with TMJOA had more pronounced mechanical hyperalgesia to blunt pressure compared to those with arthralgia. Furthermore, patients with arthralgia showed reduced sensitivity to both innocuous thermal and mechanical stimuli whereas patients with TMJOA exhibited reduced sensitivity to a tactile stimulus only (24). Another frequent symptom of TMJOA is pain associated with jaw functioning, such as food intake (25). Arthritic TMJs with high inflammatory activity (based on the high concentrations of inflammatory mediators in the synovial fluid) show greater pain intensity on maximum mouth opening and higher pain upon mandibular movements than TMJs with low inflammatory activity (26). However, self-reported jaw pain, function, and disability of patients with DJD are not different from patients with disc displacement (27).
Radiographic studies have supported the association of condyle morphology and destructive condylar changes with pain symptomatology, with condylar flattening being most highly correlated with general pain complaints (19). Condylar erosion has been shown to be proportional to pain intensity, whereas osteophytes seem to be inversely proportional (28). Among patients with arthritic changes of the TMJ, those with pain at rest or during function displayed greater destructive change index and number of CT sections with erosion or subchondral cyst formation than those with no pain (15). In a study involving 89 TMD patients (with TMJOA, TMJ arthralgia, internal derangement, and muscle disorders) and their 178 TMJs, TMJ pain during mandibular movement was correlated with radiographic findings of degenerative changes in the articular surface (erosion, concavity, flattening, osteophytes, osteosclerosis, and subchondral cysts). However, it was not correlated with resorption of the lateral part of the condyle (29). TMJ pain on digital palpation is, however, correlated with both degenerative changes in the articular surfaces and resorption of the lateral part of the condyle. Furthermore, the pressure-pain threshold is lower in patients with resorption of the lateral part of the condyle compared to patients without the condition (29). Three- dimensional surface models from cone-beam CT images show that the condylar morphology of TMJOA with pain is significantly different from asymptomatic subjects: The condyles of TMJOA with pain show resorption of the lateral and medial poles and flattening of the articular surface. Moreover, self-reported pain intensity and duration was correlated with structural changes of the superior surface of the condyles, and pain duration is correlated with structural changes of the superior, posterior, and lateral surfaces of the condyles (23).
However, there are studies suggesting that the extent of degenerative changes in the TMJ is not associated with pain-related variables (6, 9, 30, 31). Several magnetic resonance imaging (MRI) studies focused on the TMJ also have shown equivocal results: In some studies, TMJ pain is associated with severe bone changes, bone marrow edema, and condylar erosion (10, 32–35). In contrast, other MRI studies have indicated that bone does not correlate with pain (30, 36). One clinical study has even suggested that progression of TMJ intra-articular pathologies from disc displacement to degeneration is not correlated with pain, functioning, or disability (37). Similarly, in knee-joint arthritis, knee pain is not strongly correlated with radiographic findings (38, 39). Approximately 15%–76% of patients with knee pain have radiographic knee osteoarthritis (OA), and 15%–81% of patients with radiographic knee OA have pain. However, MRI studies suggest there is a stronger correlation of pain with tissue changes such as synovitis, effusion, and bone marrow lesions (40). Reduction of bone marrow lesions using bisphosphonate is accompanied by reduced pain from knee-joint OA (41). Strontium ranelate is also used to reduce joint degradation and pain from knee-joint OA (42), which suggests a potential contribution of structural changes to pain in this condition.
Lack of unequivocal evidence for the close association of degenerative changes of the TMJ with pain suggests that pain is not a good predictor of degenerative changes or vice versa. However, studying the relationship between pain and degenerative changes remains important. Identifying definitive causal factors for joint degeneration and pain can serve as a biomarker of disease onset and progression, which will enable detection and treatment during the early phase of the disease. Persistent pain can adversely impact the prognosis of the treatment of degenerative conditions (15). Novel approaches for pain treatment should not aggravate the degenerative changes either. Therefore, determining factors that commonly or differentially contribute to the onset, progression, and chronification of joint degeneration and pain can help in the development of novel tailored treatments for the different stages of TMJOA and its symptomatology.
Preclinical studies for TMJOA and pain from TMJ
Multiple animal models have been developed for determining altered joint structures and pain phenotypes in response to TMJ injury. These models are essential for studying the pathogenic mechanisms of TMJOA and exploring effective treatment measures. Compared to human studies, animal models allow for more definitive analyses of cause-and-effect relationships. While there are some morphological dissimilarities between the rodent and human TMJ (e.g., a shallow glenoid fossa and no articular eminence) (43), rodent models of TMJOA and pain should be useful for determining detailed underlying mechanisms. These models typically produce inflammation or biomechanical loading within the TMJ through different methods and evaluate various outcomes, such as degenerative changes of the disc, cartilage or bone of the condyles, and pain-related behaviors. These rodent models have several limitations and there is no ideal model. However, they have provided vast amounts of information concerning neuroimmune, cellular and molecular mechanisms underlying the pathogenesis of the onset, progression, and recovery of TMJ degeneration, as well as peripheral and central plasticity related to pain in inflammatory TMJ. In the future, such studies should lead to the identification of novel biomarkers of disease risk and progression and to explore therapeutic modalities to better manage TMJOA and its relevant symptoms. Animal models can also be used to explore the efficacy or toxicity of new treatments.
TMD patients with pain are heterogeneous in terms of psychosocial and sensory phenotypes and systemic comorbid conditions (44). In addition, the mechanisms underlying the development and maintenance of pain and joint degeneration status are highly diverse. Therefore, to study the relationship between TMJ degeneration and pain, it is likely that no single animal model will ideally mimic the etiology and natural pathogenesis of TMJ DJD translating both condylar degeneration and pain development. Different models may result in a different time course and severity of TMJ degeneration and pain-like behaviors, and their translation into human conditions may be also different in various models. The extent of association between degeneration and pain-like behaviors can also be different. In rodent models of knee OA, models have found different correlations between bone/cartilage damage with pain-like behaviors and nociceptive gene expression in sensory ganglia (45). By critically reviewing the strengths and limitations of each experimental model of TMJOA, an appropriate model can be chosen, or a new model can be developed to test hypotheses involving TMJ-related pain and degeneration.
In addition, mechanistic insights gained through preclinical studies can provide opportunities for developing novel therapeutics to treat both structural changes and pain concurrently or independently. For example, an antibody against disintegrin and metalloproteinase with thrombospondin motifs (ADAMTS-5) decreases both mechanical allodynia and structural changes in a mouse model of knee OA (46). Transforming growth factor–α also decreases cartilage/bone degeneration and knee joint pain (47). Interleukin 6 (IL-6) mediates both cartilage degradation and pain associated with post-traumatic knee OA only in males (48). In contrast, protein kinase Cδ (PKCδ) null mice show reduced joint degeneration in knee OA but display greater pain (47). It is also critical to examine whether molecules enriched in nociceptors regulate joint degeneration since the pain treatment should not adversely impact the pathogenesis of joint degeneration. For example, nerve growth factor-targeted therapy to reduce knee OA pain shows adverse side effects including rapidly progressive OA and osteonecrosis in patients (49). Blockade of voltage-gated Ca2+ channels reduces knee arthritis-induced pain, but increases joint destruction (50). Neuropeptides such as substance P and calcitonin gene-related peptide (CGRP) are known to modulate the degeneration of bone and cartilage in a knee OA mouse model (51). Identifying such common and differential mechanisms underlying TMJ degeneration and pain should help in understanding the TMJ degeneration-pain relationship. In the following sections, we will review preclinical studies exploring the mechanisms of TMJ pain and degeneration using various rodent models. We will focus our review on rodent models. Since there are recent outstanding reviews available for rodent models of TMJ degeneration (43, 52, 53), we will focus on the aspects of the TMJ pain-degeneration relationship.
Injections of inflammatory agents
The etiology of TMJOA is complex and multifactorial, and may be secondary to disc displacement, trauma, parafunctioning, unstable occlusion, or functional overloading (14). These factors can lead to an altered matrix of cartilage, increased joint friction, altered levels of cytokines, and the maintenance of low levels of inflammation to produce focal degeneration. In TMJ synovial fluid, the levels of various proinflammatory and anti-inflammaotry cytokines are differentially altered in TMD patients with or without osseous changes compared to healthy controls (54). In some conditions, such as rheumatoid arthritis, infectious arthritis, or gout, inflammatory activity within the TMJ is high and degenerative changes are more diffuse. Therefore, the direct injection of chemical agents into the TMJ to produce inflammation is the most straightforward method for evoking TMJ degeneration and pain in rodents. Intra-TMJ injections of complete Freund's adjuvant (CFA), zymosan, carrageenan, mustard oil, monoiodo acetate (MIA), albumin, and formalin have been used for this purpose. This approach has been useful for reliably producing measurable nocifensive behaviors, which start quickly (within an hour) and last for a relatively long period (approximately 1–2 weeks). Therefore, intra-TMJ injections have been widely used for studying the mechanisms of TMJ inflammation and the neurobiology of associated acute and chronic pain.
Methods of measuring pain-related outcomes from TMJ have been developed in rodents to mimic various pain conditions frequently reported by TMD patients (Table 1). These assays have also been used to measure pain-related behaviors from craniofacial muscles. Tenderness of the TMJ in patients are evaluated by measuring mechanical sensitivity on the skin overlying the joint using Von Frey filaments, for which a decreased threshold is interpreted as mechanical hyperalgesia (55–57). For mimicking spontaneous pain in TMD patients, head flinching and orofacial rubbing behaviors are assessed in rodents (62, 63, 68, 69). A high facial grimace scale score is also associated with non-evoked, pain-like behaviors after TMJ loading or masseter inflammation (65, 69–71). Preference for a conditioned analgesic indirectly indicates the presence of ongoing pain after inflammation of TMJ or masseter muscle (67, 70). Bite-evoked pain has also been modeled in rats and mice by assessing changes in bite force or gnawing function, for which TMJ or masseter muscle inflammation decreases both (58–60, 69–72). A detailed analysis of meal duration in rats and mice has also shown functional discomfort in the presence of TMJ inflammation (61). Increased central sensitization following prolonged TMJ inflammation can be evaluated by measuring mechanical allodynia from remote sites such as the fore or hindpaw (67). Increased anxiety in TMD patients has also been modeled in rodents using anxiety-like behavioral assays such as the elevated plus maze and open field tests (66). The duration and extent of nocifensive behaviors are varied and depend on multiple factors including the agents used for producing TMJ inflammation, the behavioral assays used, and the strain, species, and sex of the animals.
Intra-TMJ injections of CFA induces mechanical hyperalgesia on the TMJ, which peaks after 1–4 days and lasts approximately 10–14 days in mice and rats (56, 57). Intra-TMJ CFA administration increase meal durations for 19 days in rats and 1 week in DBA/1LacJ mice, but does not affect C57bl/6 mice (61). Intra-TMJ CFA also reduces bite force for 1–9 days (59, 73) and increases the mouse grimace scale score for 2–3 days (56). Following MIA injection, mechanical sensitivity on TMJ skin increases from day 1 and lasts for 2 weeks (67, 74). This mechanical hyperalgesia declines from baseline at 3 weeks with recovery occurring after 4 weeks (74). MIA-injected rats display a conditioned place preference for the systemic administration of duloxetine at 7 days. They also exhibit increased feeding duration and total number of meals, suggesting the presence of ongoing pain (67). It appears that MIA tends to produce a longer duration of hyperalgesia than CFA. In addition, when it comes to injection method, most studies focused on degeneration and mechanism, while the studies of phenotype of pain are limited. Therefore, we conducted an example of phenotype experiment to figure out its effectiveness. Intra-TMJ injections of MIA increased mechanical sensitivity and mouse grimace scale scores and decreased the bite force for at least 2 weeks in mice (Figure 1). Establishing the mouse model of persistent TMJ pain can facilitate mechanistic study using a variety of transgenic models.
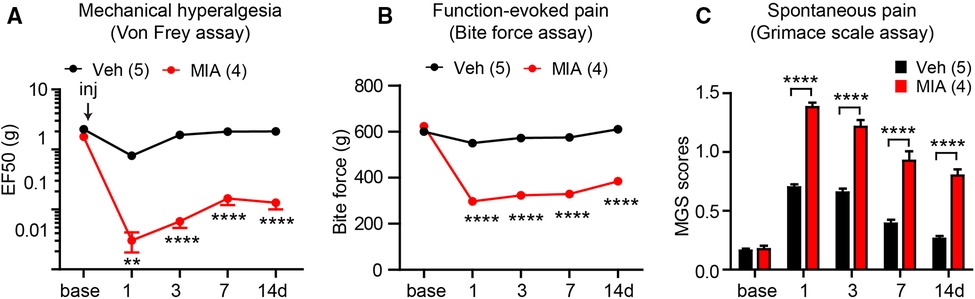
Figure 1. Examples of measuring different types of persistent pain induced by temporomandibular joint (TMJ) inflammation in mice. Bilateral TMJs of C57bl/6 mice were injected with monoiodo acetate (MIA). Controls were injected with saline. Mechanical sensitivity of the skin over the right TMJ using the Von Frey assay (A), bite-force (B), and mouse grimace scale (MGS) (C) were determined. **p < 0.01; ****p < 0.0001 in Bonferroni post-tests following two-way repeated measure ANOVAs. We followed methods described previously (69, 70, 75).
Studies using models that involve the artificial inflammation of the TMJ and other orofacial areas have led to important discoveries concerning the neurophysiological mechanisms in primary afferents and central neural transmissions associated with pain from the TMJ and other oral and craniofacial regions. These studies have laid a solid foundation for our current understanding of orofacial pain mechanisms. While a detailed discussion of these mechanisms is beyond the scope of this review, there are other publications that have reviewed these topics (57, 76–82). In brief, the identity of the subtypes of joint nociceptors and the peripheral sensitization of joint afferents leading to hypersensitivity has been defined (83), as has the dominant role TRPV1-expressing afferents play in TMJ pain (84). Furthermore, the causative role of pronociceptive ion channels in nociceptors (e.g., TRPV4 or Nav1.7) has been identified (58, 85). The anti-nociceptive role of opioids, cannabinoids, and β adrenergic receptors are also known (63, 86–88). Highly convergent nociceptive inputs from the TMJ and other orofacial tissues (such as skin, tooth pulp, and the masseter muscle) on dorsal horn neurons in the cervical spinal cord and trigeminal subnucleus caudalis (Vc) may underlie the diffuse and widespread nature of pain from TMD (89–92). Central sensitization mechanisms involving NMDA receptors, MARK, and non-neuronal cells (e.g., microglia and astrocytes) in the medullary dorsal horn are well known (93–96). Intraganglionic mechanisms can also mediate widespread pain via the spreading of nociceptive signals within the trigeminal ganglia (TG) through soluble mediators and gap junction channels involving satellite glia (97–99). Determining the mechanisms underlying sex differences has also been at the center of investigational interest. Sex hormones (e.g., estrogen, progesterone, and testosterone) differentially affect inflammatory responses in the TMJ (100–103) and the activities of joint afferents (104). Currently, sex differences and the influence of gonadal hormones on nocifensive behaviors have been determined (105–107), and the roles of sex hormones on the regulation of neuron activity in the Vc and upper cervical spinal cord are also well established (108, 109).
Injecting chemical agents into the TMJ has also been widely used to determine the mechanisms of inflammation-induced TMJ degeneration. CFA contains heat-killed mycobacteria that produces immune responses and inflammation, which recapitulates microbial infection-like acute inflammatory responses. Two weeks after intra-TMJ injections of CFA in mice, the TMJ showed deposition of extracellular matrix, such as aggrecan and collagen type II, and soft tissue changes that include pannus formation in TMJ disc attachments and synovitis with infiltration of inflammatory cells (110). However, cartilage degradation or bony changes do not occur, which suggests that this model cannot represent pathogenesis during the chronic phase of OA. Another study in rats demonstrated that intra-TMJ CFA injections induced histological changes in cartilage and subchondral bone that peaked after 2 weeks, but recovered thereafter without progressive, long-term changes (111). When CFA injections were administered into rat TMJs two weeks apart, inflammation in the synovium was sustained up to 5 weeks after the 1st CFA injection, and the TMJ disc was thickened with increased cellularity (112). However, there were no changes in the condylar cartilage or the subchondral bone. In these experiments, CFA was injected into the upper joint cavity. In contrast, injection of CFA into the lower joint cavity induced radiographic and histologic bony changes within 14 days (113), supporting the idea that CFA does not induce degenerative changes of the disc.
MIA has also been widely used to model degeneration and pain from knee joint OA in rodents (67, 74, 114, 115). MIA is an inhibitor of glyceraldehyde-3-phosphate dehydrogenase and interferes with the metabolism of glycolysis in nerves and chondrocytes (116, 117). Injections of 0.5 mg MIA into the upper compartment of the TMJ in female rats induces ultrastructural changes in chondrocytes and the disc as early as day 1. Chondrocytes are nearly lost in the cartilage of the temporal fossa and intermediate zone of the disc after 3 days. Exudates in the upper compartment is evident within 1 week, and infiltrated immune cells are present within 2 weeks. In µCT, changes in the condylar surface appear within 1 week, while erosion and defects are evident within 2–4 weeks. Sclerotic changes begin by 8 weeks (74). Hence, MIA may produce more global and long-lasting degenerative changes in the TMJ compared to CFA.
Although the injection of exogenous substances into the TMJ consequently produces both pain and degenerations, the model cannot ideally mimic the unknown real factors contributing to the progression and the maintenance of persistent pain and degeneration in humans. Despite the limitation, direct injection chemical agents can be still useful for understanding inflammatory processes leading to TMJ pain and degeneration.
Forced mouth opening
The TMJ is unique in its high mobility compared to other joints. Mandibular condyles not only make a hinge movement, but also rotate horizontally during mastication and glide over the temporal bone upon wide opening of the mouth. The structure of the TMJ has evolved to accommodate the wide and dynamic range of movements with high functionality and maximum efficiency. However, excessive functioning of the TMJ beyond its physiological limits can be traumatic and leads to the development of chronic pain and structural remodeling of the joint. Indeed, there is a strong association between prolonged mouth opening and the development of chronic TMD. In one prospective study, the TMD annual incidence was doubled in patients who had experienced a jaw injury (118). Both extrinsic injuries (e.g., tooth extractions or dental treatments, oral intubation, sports injury, or motor vehicle accidents) or intrinsic injuries (e.g., yawning or sustained mouth opening) can be risk factors for TMD. Interestingly, the association of jaw injury with painful TMD are higher in patients with increased heat pain (119). Not only pain, jaw trauma can also lead to the degenerative changes of TMJ (13, 120). Therefore, it is important to investigate pain responses and TMJ structure following TMJ trauma or injury. To achieve this, animal models have been developed for delivering intrinsic injuries to the TMJ by adding abnormal TMJ loading or inducing a non-physiological range of jaw openings (121–123). These models are non-invasive and do not involve open trauma or injecting artificial chemicals into the joint to cause structural destruction.
A single event of prolonged jaw opening for 20 min in rats induces increased head withdrawal to mechanical stimuli as early as 2 h after the event, which then lasts up to 7 days (123). The procedure increases pro-inflammatory cytokines in the TG and upper cervical spinal cord up to 14 days after induction (123). Sustained and repeated mouth opening (60 min/day for 7 days) in rats by applying 2 N to the jaw has produced mechanical hyperalgesia on the face as early as day 1 and has lasted for 3 days after termination of the induction. When loading force was increased to 3.5 N, mechanical hyperalgesia was not resolved—even after a week following the termination of the induction (122, 124). The rat grimace scale score was increased in the 3.5 N group, but not in the 2 N group, over a period of 1–7 days following the initiation of sustained mouth opening, during which condylar cartilage was not affected (65). However, damage to the condylar cartilage was evident on day 15. Both the 2 N and 3.5 N groups showed thinning of the condylar cartilage with irregular chondrocyte organization and there was no significant difference between two groups. However, the expression of MMP-13, hypoxia-inducible factor 1α (HIF-1α, and TNF in the condylar cartilage were higher in the group with greater loading (122). A preemptive, single intra-TMJ injection of etanercept (a biologic TNF inhibitor) decreased mechanical hyperalgesia and rat grimace scale scores until day 7 following the initiation of TMJ loading (125). Concomitantly, an intra-TMJ injection of etanercept reduced HIF-2α expression and hypoxia in TMJ cartilage on day 8 (125). Detailed investigations of the pericellular matrix surrounding chondrocytes within the TMJ cartilage following loading showed that collagen VI increased in both the 2 N and 3.5 N groups, whereas there was a greater increase in aggrecan non-epitopes in the 3.5 N group. These results suggest that aggrecan fragmentation may be relevant to pain responses and the severity of structural damage to the TMJ (126).
In C57BL/6 mice, sustained passive mouth opening by 11.5 mm (1.5 h per day for 5 consecutive days) produced mechanical hyperalgesia of the masseter muscle, which began as early as 1 day after the 1st opening event and lasted for 32 days afterwards (127). The amount of hard pellet food intake was also reduced. Sustained mouth opening increased the infiltration of macrophages into the TG and the activation of microglia in the Vc. Inhibition of colony stimulating factor 1 (CSF1) receptor reduced this infiltration into the TG, TMJ, and masseter muscles and decreased the activation of microglia in the Vc. Importantly, the CSF1 inhibitor significantly attenuated the development of mechanical hyperalgesia by sustained mouth opening, suggesting macrophages contribute to the peripheral sensitization of trigeminal nociceptors from TMJ injury. Sustained mouth opening also led to subtle changes in the condylar cartilage on day 5.
Mechanisms of TMJ structural degeneration after forced mouth opening in mice were studied in a sustained mouth-opening model that used a spring to maintain an open mouth by 14 mm and delivered 2 N force for 3 h per day for 5 days (128). Four weeks after the completion of the force loading sessions, destruction of TMJ cartilage and subchondral bone was obvious on mCT and in histological assays (128). Rebamipide, an anti-oxidant and anti-inflammatory agent, reduced the TMJ degeneration with reduced osteoclastogenesis (128). Although the effects of rebamipide on pain were not assessed in that study, the intra-articular injection of rebamipide into the knee joint attenuates hyperalgesia and cartilage degeneration following MIA injection (129).
Rodent forced mouth opening models can be useful for determining structural changes in the TMJ that mimic loading and trauma pathogenesis in humans. The model has translational potential since the associations of TMJ trauma with the development of painful TMD or TMJ degeneration is known (13, 118). Determining pain phenotypes using various assays during the time course of the pathogenesis of TMJOA and a more careful assessment of pain and TMJ degeneration over longer periods is necessary.
Of note, the non-physiological range of excessive occlusal interferences can lead to pain and TMJ degeneration in animals as reviewed previously (52, 130). However, it is important to emphasize that the animal models involving occlusal interference are built on a highly controversial assumption that occlusion is an etiologic factor leading to TMD development, but the evidence supporting this assumption is not established (131). There is a growing consensus among TMD experts that occlusion should not be considered a contributing factor for the common TMD (132). Therefore, translational potential of the models involving occlusion alteration is weak and we do not discuss this model in this review.
Surgical approaches
A surgical approach to destabilize the knee joint by transection of the medial meniscotibial ligament has been widely used in rodents to produce slow progression of structural changes and pain-like behaviors from 4 weeks following surgery (133, 134). Degeneration of the knee joint is accompanied by the development of pain-like behaviors such as weight bearing asymmetry or decreased paw withdrawal thresholds. Despite their correlation, it is still unclear if the extent of degeneration of the knee joint and pain-like behaviors are causative or mechanistically associated. For example, a modified surgical technique only attenuates pain-like behaviors after 13 weeks following surgery but did not affect joint pathology (135). However, comparable artificial surgical impairments of TMJ stability have been used to produce TMJOA in rodents (Table 2). Removal of the entire or a portion of the articular disc can affect the ability of the condylar surface of the TMJ to withstand forces and absorb loads, which could result in excessive mechanical forces on the condylar surface over a small area leading to TMJOA.
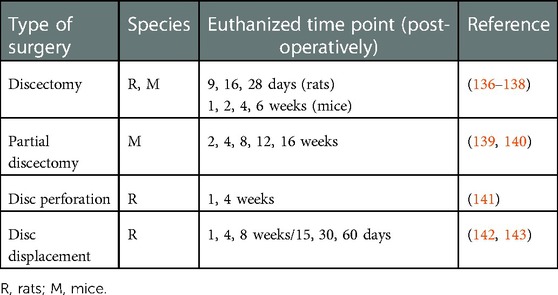
Table 2. Examples of surgical procedures to induce temporomandibular joint osteoarthritis in rodents.
Partial or total removal of the disc in the TMJ of rats and mice results in early-onset OA-like changes (136, 137, 139, 144). Partial discectomy that removes the lateral part of the disc of the TMJ in C67BL/6 mice produces alterations in the condylar cartilage as early as 4 weeks following surgery. At 16 weeks, destruction of the cartilage was more evident, but subchondral bone was not affected. Surgical perforation of the posterolateral portion of the TMJ disc in rats has led to the destruction of cartilage condyles and subchondral bone at 4 weeks (145). Intra-TMJ administration of interleukin 37b, an isoform of the anti-inflammatory cytokine interleukin 37, decreased post-surgical degenerative changes of the TMJ (145). Although pain phenotypes were not determined in the rat model, patients with TMJ synovitis exhibit a high expression of interleukin 37 in synovial fluid, which is correlated with self-reported pain levels (145). Surgical anterior displacement of the TMJ disc in rats also produces OA-like alterations in TMJ structure (142, 143), which is apparently less severe than other surgical approaches. Although pain-like behaviors were not directly measured, no visible behavioral changes or stress-related signs were reported.
Surgical models of TMJOA are primarily used for studying TMJ degeneration rather than pain phenotypes, and information regarding the correlation or association of pain and TMJ structure is unavailable. Since surgical manipulation itself can cause inflammation inside the affected joint, segregating the effects of acute, post-operational changes on TMJ structure from the effects of surgical manipulation of the disc is difficult. Interestingly, unilateral, partial discectomy not only leads to degeneration in the surgical-side of the TMJ, but also initiates early-onset articular cartilage degeneration in the contralateral, non-surgical side of the TMJ in mice (140). Therefore, the contralateral non-surgical side of the TMJ might serve as a model of TMJ degeneration that mimics the natural progression of TMJOA in patients. Nonetheless, differentiation of post-operative pain from the pain caused by structural alterations relevant to TMJOA can be challenging, which might be a limitation when using surgical models for determining the pain-degeneration relationship.
Genetic models of TMJOA
Mice carrying spontaneous or targeted mutations of multiple genes naturally develop TMJ degeneration in a non-invasive manner and without the need for injecting agents or inducing trauma (Table 3). These mouse models have been used to determine molecular contributions to the development and degeneration of the TMJ, which has been recently reviewed elsewhere (43). The mouse genetic model has deepened our understanding of the causal contributions of matrix proteins, transcription factors, and regulatory signaling such as growth factors. Mouse models that carry natural mutations of genes encoding matrix proteins have been useful for understanding their critical contributions to skeletal anomalies associated with human disease. For example, the disproportionate micromelia (Dmm) mutation is associated with mutations of Col2a1 that encodes collagen type II, which results in condylar cartilage abnormalities in the TMJ (147). Dmm TMJs exhibit degeneration of the condylar cartilage from 6 months of age, which continues to progress until 12 months of age. During the progression, expression markers, such as HtrA1, Ddr2 and Mmp13 were detected (147). The chondrodysplasia (Cho) mutation is caused by a single-nucleotide deletion of Col11a1, which encodes for collagen type XI (146). In the TMJ of Cho mice, changes in proteoglycans occur as early as 3 months and fissures of the condylar cartilage were evident at 9 month of age. Targeted mutations of multiple genes has also uncovered their roles in TMJOA. Transgenic mice expressing the deletion mutant of Col2a1 (Del1 mice) show defects in condylar cartilage as early as 3 months and progression of degenerative changes occurs until 15 months of age (148). Mice with double mutations of 2 genes encoding proteoglycans, Bgn (biglycan) and Fmod (fromodulin) develop degenerative changes at 6 months and show complete destruction of the TMJ at 18 months (149). Knockouts of proteoglycan 4 (Prg4), which encodes lubricin, which functions as a lubricant inside the TMJ, induces early signs of OA-like degenerative changes including increased thickness of the glenoid fossa, articular disc, and condylar head as early as 3 months. At 12 months, morphological and osseous abnormalities of the condyle was evident in radiographic analyses (150). Knockouts of discoidin domain receptor 1, a tyrosine kinase receptor, develop osteoarthritic degenerative changes of the TMJ as early as 9 weeks of age (151).
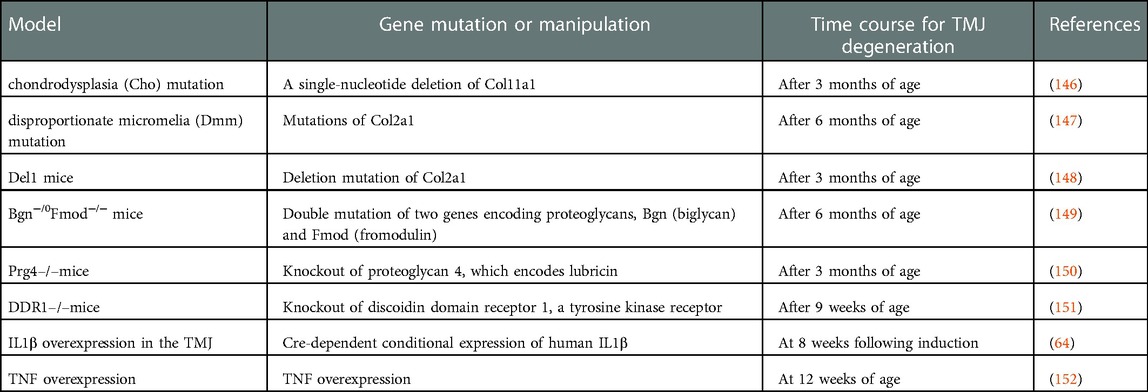
Table 3. Genetic mouse models producing temporomandibular joint (TMJ) osteoarthritis-like phenotypes.
Genetic models involving pro-inflammatory cytokines have contributed to thorough validations of their role in TMJOA pain and degeneration. Pro-inflammatory cytokines such as IL1β, TNF, and IL6 are elevated in the TMJs of patients with TMD (153). The IL1β level in TMJ synovial fluid from TMD patients is associated with pain and tenderness on palpation (154). Moreover, pain on TMJ palpation and mandibular movement is associated with increased levels of TNF in TMJ synovial fluid (155). Furthermore, intra-TMJ CFA injections elevate TNF and IL1β inside the joint (156). Sufficiency of IL1β in TMJ degeneration and pain was convincingly shown using a model that overexpressed the IL1β gene under the Col1a1 promoter within the TMJ (by intra-TMJ injection of the viral vector encoding Cre). In this model, joint degenerative changes were evident 8 weeks following Cre injection (157). Increases of CGRP in the TG and nocifensive behaviors such as facial grooming also occurred (157). Nocifensive behaviors in this model were attenuated by virally mediated overexpression of the mu-opioid receptor gene in the TMJ (64). IL1β plays an important role in the medullary dorsal horn (158), and TMJ degeneration via overexpression of IL1β is prevented by the overexpression of the IL1 receptor antagonist in the brainstem. Consistently, conditional overexpression of IL1β in the medullary dorsal horn driven GFAP promoter is sufficient to induce TMJ degeneration, albeit to a lesser extent than the overexpression of IL1β in the TMJ. Furthermore, facial grooming behaviors induced by the overexpression of IL1β in the TMJ is attenuated by the overexpression of the IL1 receptor antagonist in the brainstem (158). These results suggest that IL1β in both the TMJ and the medullary dorsal horn contribute to TMJ degeneration and pain.
TNF also contributes to TMJ pain. TNF is released not only by immune cells but also by the glia and neurons of the peripheral and central nervous systems (159). Overexpression of TNF is sufficient to develop OA changes in the TMJ such as articular and condylar bony changes, an influx of inflammatory cells in the synovium, and fissuring of the condylar surface at 12 weeks of age (152). TNF overexpression also causes severe destruction of the condyle, with condylar surface erosion, bone resorption, and loss of the disc at 14 weeks (160). Although pain phenotypes were not evaluated in this model, the contribution of TNFα to pain from rodent models of TMJ inflammation is known. For example, intra-TMJ TNF injections increases head withdrawal responses (161). Intra-TMJ CFA increases TNF in the TMJ, the TG, and the Vc (56, 162). Furthermore, CFA-induced nocifensive behaviors (mechanical hyperalgesia of facial skin and increased mouse grimace scale scores) are attenuated in mice lacking TNF (56).
These genetic mouse models are useful for determining the molecular contributors to degeneration of the TMJ that mimic natural pathogenesis in humans (with an onset at 2 to 6 months of age and further progression with aging). However, most studies are only focused on the time course of the disease and biological factors involved in degenerative changes within the TMJ and, therefore, do not pay much attention to pain-related phenotypes. Hence, determining pain phenotypes using the various assays discussed above during the time course of pathogenesis of TMJOA in these genetic models is warranted.
Models involving emotional stress and comorbid conditions
International Association for the Study of Pain defines pain as “an unpleasant sensory and emotional experience associated with, or resembling that associated with, actual or potential tissue damage, or described in terms of such damage”, meaning pain is not a simple sensation but involves emotional aspects. One prospective study showed that multiple psychosocial variables including somatic symptoms, general psychological symptoms, a negative mood, and multiple measures of stress are risk factors for the onset of painful TMD (163). Furthermore, in an unbiased clustering of subjects based on biopsychosocial measurements, a cluster showing greater pain sensitivity and greater psychological distress had a greater risk of painful TMD onset, as well as other comorbid pain conditions such as chronic headache, low-back pain, and irritable bowel syndrome (44). Therefore, chronic pain from TMD should be under the influence of psychosocial factors, although relationship between the psychosocial factors and pain associated with TMJOA is not clear. As surrogate models in rodents, the effects of psychological stress on TMJ pain have been actively studied. Intra-TMJ CFA injections increases anxiety-like behaviors (reduced entry into open arms in elevated plus mazes and light boxes in light/dark box assays) (66). Rats exposed to chronic restraint stress exhibit greater anxiety-like behavior and formalin-induced nocifensive behaviors (164). Stress-induced hyperalgesia from the TMJ is apparently derived from both peripheral and central mechanisms, while sleep deprivation increases inflammatory cytokines from the synovial membrane (165). Stress also enhances the activity of TMJ neurons in the deep lamina of the Vc and enhances the integration of nociceptive information from the masseter muscles (166). Interestingly, psychological stress also affects condyle structure (167). When rats experience psychological stress by visual, olfactory, and auditory stimuli from other rats receiving electric foot shocks in an adjacent chamber, anxiety-like behaviors increase. Furthermore, condylar cartilage and subchondral bony changes occur as early as 3 weeks, which is accompanied by increased expression of MMP3, IL1β and TNF in the TMJ (168, 169). Adrenergic signaling through α2 receptors in chondrocytes promotes TMJ degeneration (170). Chronic sleep deprivation activates extracellular, signal-regulated kinases (ERK) in the TMJ, and inhibition of ERK reduces the expression of matrix metalloproteinases genes and decreases pathological changes of condyle (171). Therefore, chronic stress likely affects both TMJ degeneration and pain.
The mechanisms of TMD and comorbid pain conditions, such as irritable bowel syndrome, have also been studied using rodent models. Masseter inflammation followed by swim stress induces months-long visceral hypersensitivity and concomitant cutaneous hyperalgesia in rats, which is mediated by ERK1/2 in the spinal cord (172, 173). Such sensitization between orofacial and gut tissues were also studied in another mouse model called the “double-hit model” (174). Intra-TMJ CFA injections produced mechanical hypersensitivity of the skin overlying the TMJ, which recovered within a week. After 3 weeks, colonic injections of mustard oil produced no hypersensitivity in wild type mice. In contrast, TNFR1/R2 double knockout mice developed mechanical hypersensitivity on the skin overlying the TMJ for up to 18 weeks. Systemic antagonism of TNF reverses mechanical hyperalgesia that has developed after colonic mustard oil (174), suggesting the role of circulating cytokines in trans-segmental sensitization. A recent study has reported a more sophisticated comorbid pain model: unilateral anterior cross bite in rats produces somatic pain hypersensitivity, which involves the cholecystokinin receptor-mediated descending facilitation system (175). Mechanistically, the gut microbiome was also proposed to be involved in TMJ pain (176). Intra-TMJ CFA injections induced mechanical hyperalgesia in the facial skin and alterations of the gut microbiota. This was reversed by systemic treatment with resveratrol and via transplantation of fecal microbiota from resveratrol-treated mice into mice with intra-TMJ CFA (176), which suggests a contribution of gut microbial dysbiosis to TMJ pain. In humans, an abundance of gut Streptococcus is associated with pain and inflammation in knee OA (177). While a microbiota-skeletal axis has been proposed, determining the association of TMJOA and pain with the gut microbiome and comorbid pain conditions is warranted.
Conclusion
Studies that have focused on the mechanisms of TMJ pain and structural degeneration using different models indicate that TMJ pain and degeneration occur over a partially overlapping time course. There are molecules that commonly cause pain and joint degeneration, e.g., intra-articular pro-inflammatory cytokines. However, it is unclear whether pain is causally associated with structural degeneration and whether structural degeneration is necessary for producing persistent pain. To experimentally address the pain-structure relationship, it will be important to determine whether the activity of peripheral and central pathways involved in TMJ pain contributes to TMJ degeneration or vice versa. Considering the broad impact of the brain on skeletal homeostasis (178), it is likely that nociceptors and brain plasticity associated with pain upon TMJ injury can modulate the degeneration of the TMJ. More detailed investigations of the pain-structure relationship in the TMJ can suggest new therapeutic options that treat pain without adverse effects on the TMJ structure. To determine the mechanistic association of pain and TMJ structure, it is important to select the right animal models. However, TMD are multifactorial conditions, and it may be that a single animal model cannot represent all aspects of pain and the structural changes in the TMJ with DJD. As different animal models mimic some aspects of the signs and symptoms of TMJOA patients, careful selection of one or combinations of animal models will be critical to meet the goal of investigations. Further development of non-invasive models mimicking natural progress of degenerative changes in TMJ with persistent pain conditions and comorbidities can improve the mechanistic understanding and the development of novel strategies for better managements of TMD.
Author contributions
MC, SW, IA, JH, and JR conceptualized the study. All authors wrote and revised the manuscript. All authors contributed to the article and approved the submitted version.
Funding
This work was supported by the National Institutes of Health grants R01 DE027731, R01 DE031477, and R35 DE030045 to MKC, and R01 DE016062 and R01 AG073136 to JYR.
Acknowledgments
The authors thank Dr. Marcela Romero-Reyes at the University of Maryland Baltimore, School of Dentistry, for critical reading of the manuscript.
Conflict of interest
The authors declare that the research was conducted in the absence of any commercial or financial relationships that could be construed as a potential conflict of interest.
Publisher's note
All claims expressed in this article are solely those of the authors and do not necessarily represent those of their affiliated organizations, or those of the publisher, the editors and the reviewers. Any product that may be evaluated in this article, or claim that may be made by its manufacturer, is not guaranteed or endorsed by the publisher.
References
1. Macfarlane TV, Glenny AM, Worthington HV. Systematic review of population-based epidemiological studies of oro-facial pain. J Dent. (2001) 29(7):451–67. doi: 10.1016/S0300-5712(01)00041-0
2. Horst OV, Cunha-Cruz J, Zhou L, Manning W, Mancl L, DeRouen TA. Prevalence of pain in the orofacial regions in patients visiting general dentists in the Northwest Practice-based REsearch Collaborative in Evidence-based DENTistry research network. J Am Dent Assoc. (2015) 146(10):721–8e3. doi: 10.1016/j.adaj.2015.04.001
3. Schiffman E, Ohrbach R, Truelove E, Look J, Anderson G, Goulet JP, et al. Diagnostic criteria for temporomandibular disorders (DC/TMD) for clinical and research applications: recommendations of the international RDC/TMD consortium network* and orofacial pain special interest groupdagger. J Oral Facial Pain Headache. (2014) 28(1):6–27. doi: 10.11607/jop.1151
4. Cooper BC, Kleinberg I. Examination of a large patient population for the presence of symptoms and signs of temporomandibular disorders. Cranio. (2007) 25(2):114–26. doi: 10.1179/crn.2007.018
5. Slade GD, Bair E, Greenspan JD, Dubner R, Fillingim RB, Diatchenko L, et al. Signs and symptoms of first-onset TMD and sociodemographic predictors of its development: the OPPERA prospective cohort study. J Pain. (2013) 14(12 Suppl):T20–32e1-3. doi: 10.1016/j.jpain.2013.07.014
6. Kim K, Wojczynska A, Lee JY. The incidence of osteoarthritic change on computed tomography of Korean temporomandibular disorder patients diagnosed by RDC/TMD; a retrospective study. Acta Odontol Scand. (2016) 74(5):337–42. doi: 10.3109/00016357.2015.1136678
7. Jeon KJ, Lee C, Choi YJ, Han SS. Analysis of three-dimensional imaging findings and clinical symptoms in patients with temporomandibular joint disorders. Quant Imaging Med Surg. (2021) 11(5):1921–31. doi: 10.21037/qims-20-857
8. Fan PD, Xiong X, Cheng QY, Xiang J, Zhou XM, Yi YT, et al. Risk estimation of degenerative joint disease in temporomandibular disorder patients with different types of sagittal and coronal disc displacements: MRI and CBCT analysis. J Oral Rehabil. (2023) 50(1):12-23. doi: 10.1111/joor.13385
9. Wiese M, Svensson P, Bakke M, List T, Hintze H, Petersson A, et al. Association between temporomandibular joint symptoms, signs, and clinical diagnosis using the RDC/TMD and radiographic findings in temporomandibular joint tomograms. J Orofac Pain. (2008) 22(3):239–51. PMID: 18780537.18780537
10. Bertram S, Rudisch A, Innerhofer K, Pumpel E, Grubwieser G, Emshoff R. Diagnosing TMJ internal derangement and osteoarthritis with magnetic resonance imaging. J Am Dent Assoc. (2001) 132(6):753–61. doi: 10.14219/jada.archive.2001.0272
11. Back K, Ahlqwist M, Hakeberg M, Dahlstrom L. Occurrence of signs of osteoarthritis/arthrosis in the temporomandibular joint on panoramic radiographs in Swedish women. Community Dent Oral Epidemiol. (2017) 45(5):478–84. doi: 10.1111/cdoe.12312
12. Lei J, Han J, Liu M, Zhang Y, Yap AU, Fu KY. Degenerative temporomandibular joint changes associated with recent-onset disc displacement without reduction in adolescents and young adults. J Craniomaxillofac Surg. (2017) 45(3):408–13. doi: 10.1016/j.jcms.2016.12.017
13. Norman JE. Post-traumatic disorders of the jaw joint. Ann R Coll Surg Engl. (1982) 64(1):29–36. PMID: 7055365; PMCID: 2494002.7055365
14. Tanaka E, Detamore MS, Mercuri LG. Degenerative disorders of the temporomandibular joint: etiology, diagnosis, and treatment. J Dent Res. (2008) 87(4):296–307. doi: 10.1177/154405910808700406
15. Song H, Lee JY, Huh KH, Park JW. Long-term changes of temporomandibular joint osteoarthritis on computed tomography. Sci Rep. (2020) 10(1):6731. doi: 10.1038/s41598-020-63493-8
16. de Souza RF, Lovato da Silva CH, Nasser M, Fedorowicz Z, Al-Muharraqi MA. Interventions for the management of temporomandibular joint osteoarthritis. Cochrane Database Syst Rev. (2012) 2012(4):CD007261. doi: 10.1002/14651858.CD007261.pub2
17. Wu M, Almeida FT, Friesen R. A systematic review on the association between clinical symptoms and CBCT findings in symptomatic TMJ degenerative joint disease. J Oral Facial Pain Headache. (2021) 35(4):332–45. doi: 10.11607/ofph.2953
18. Derwich M, Mitus-Kenig M, Pawlowska E. Morphology of the temporomandibular joints regarding the presence of osteoarthritic changes. Int J Environ Res Public Health. (2020) 17(8):2923. doi: 10.3390/ijerph17082923
19. Comert Kilic S, Kilic N, Sumbullu MA. Temporomandibular joint osteoarthritis: cone beam computed tomography findings, clinical features, and correlations. Int J Oral Maxillofac Surg. (2015) 44(10):1268–74. doi: 10.1016/j.ijom.2015.06.023
20. Ahmad M, Hollender L, Anderson Q, Kartha K, Ohrbach R, Truelove EL, et al. Research diagnostic criteria for temporomandibular disorders (RDC/TMD): development of image analysis criteria and examiner reliability for image analysis. Oral Surg Oral Med Oral Pathol Oral Radiol Endod. (2009) 107(6):844–60. doi: 10.1016/j.tripleo.2009.02.023
21. Ning W, Schmalz G, Li P, Huang S. Oral health-related quality of life in patients with osteoarthritis of the temporomandibular joint-results of a systematic review. J Oral Rehabil. (2022) 49(11):1106–14. doi: 10.1111/joor.13363
22. Kino K, Sugisaki M, Haketa T, Amemori Y, Ishikawa T, Shibuya T, et al. The comparison between pains, difficulties in function, and associating factors of patients in subtypes of temporomandibular disorders. J Oral Rehabil. (2005) 32(5):315–25. doi: 10.1111/j.1365-2842.2004.01439.x
23. Cevidanes LH, Hajati AK, Paniagua B, Lim PF, Walker DG, Palconet G, et al. Quantification of condylar resorption in temporomandibular joint osteoarthritis. Oral Surg Oral Med Oral Pathol Oral Radiol Endod. (2010) 110(1):110–7. doi: 10.1016/j.tripleo.2010.01.008
24. Kothari SF, Baad-Hansen L, Hansen LB, Bang N, Sorensen LH, Eskildsen HW, et al. Pain profiling of patients with temporomandibular joint arthralgia and osteoarthritis diagnosed with different imaging techniques. J Headache Pain. (2016) 17(1):61. doi: 10.1186/s10194-016-0653-6
25. Haketa T, Kino K, Sugisaki M, Amemori Y, Ishikawa T, Shibuya T, et al. Difficulty of food intake in patients with temporomandibular disorders. Int J Prosthodont. (2006) 19(3):266–70. PMID: 16752624.16752624
26. Alstergren P, Pigg M, Kopp S. Clinical diagnosis of temporomandibular joint arthritis. J Oral Rehabil. (2018) 45(4):269–81. doi: 10.1111/joor.12611
27. Kubota T, Kubota E, Matsumoto A, Kawai Y, Saito H, Mikuni-Takagaki Y, et al. Identification of matrix metalloproteinases (MMPs) in synovial fluid from patients with temporomandibular disorder. Eur J Oral Sci. (1998) 106(6):992–8. doi: 10.1046/j.0909-8836.1998.eos106603.x
28. Bae S, Park MS, Han JW, Kim YJ. Correlation between pain and degenerative bony changes on cone-beam computed tomography images of temporomandibular joints. Maxillofac Plast Reconstr Surg. (2017) 39(1):19. doi: 10.1186/s40902-017-0117-1
29. Kurita H, Kojima Y, Nakatsuka A, Koike T, Kobayashi H, Kurashina K. Relationship between temporomandibular joint (TMJ)-related pain and morphological changes of the TMJ condyle in patients with temporomandibular disorders. Dentomaxillofac Radiol. (2004) 33(5):329–33. doi: 10.1259/dmfr/13269559
30. Wurm MC, Behrends TK, Wust W, Wiesmuller M, Wilkerling A, Neukam FW, et al. Correlation between pain and MRI findings in TMD patients. J Craniomaxillofac Surg. (2018) 46(8):1167–71. doi: 10.1016/j.jcms.2017.12.029
31. Palconet G, Ludlow JB, Tyndall DA, Lim PF. Correlating cone beam CT results with temporomandibular joint pain of osteoarthritic origin. Dentomaxillofac Radiol. (2012) 41(2):126–30. doi: 10.1259/dmfr/60489374
32. Takahara N, Nakagawa S, Sumikura K, Kabasawa Y, Sakamoto I, Harada H. Association of temporomandibular joint pain according to magnetic resonance imaging findings in temporomandibular disorder patients. J Oral Maxillofac Surg. (2017) 75(9):1848–55. doi: 10.1016/j.joms.2017.03.026
33. Suenaga S, Nagayama K, Nagasawa T, Indo H, Majima HJ. The usefulness of diagnostic imaging for the assessment of pain symptoms in temporomandibular disorders. Jpn Dent Sci Rev. (2016) 52(4):93–106. doi: 10.1016/j.jdsr.2016.04.004
34. Limchaichana N, Nilsson H, Ekberg EC, Nilner M, Petersson A. Clinical diagnoses and MRI findings in patients with TMD pain. J Oral Rehabil. (2007) 34(4):237–45. doi: 10.1111/j.1365-2842.2006.01719.x
35. Emshoff R, Bertram A, Hupp L, Rudisch A. A logistic analysis prediction model of TMJ condylar erosion in patients with TMJ arthralgia. BMC Oral Health. (2021) 21(1):374. doi: 10.1186/s12903-021-01687-w
36. Ohlmann B, Rammelsberg P, Henschel V, Kress B, Gabbert O, Schmitter M. Prediction of TMJ arthralgia according to clinical diagnosis and MRI findings. Int J Prosthodont. (2006) 19(4):333–8. PMID: 16900815.16900815
37. Chantaracherd P, John MT, Hodges JS, Schiffman EL. Temporomandibular joint disorders’ impact on pain, function, and disability. J Dent Res. (2015) 94(3 Suppl):79S–86S. doi: 10.1177/0022034514565793
38. Hannan MT, Felson DT, Pincus T. Analysis of the discordance between radiographic changes and knee pain in osteoarthritis of the knee. J Rheumatol. (2000) 27(6):1513–7. PMID: 10852280.10852280
39. Jones G. Osteoarthritis: where are we for pain and therapy in 2013? Aust Fam Physician. (2013) 42(11):766–9. PMID: 24217094.24217094
40. Hunter DJ, Zhang W, Conaghan PG, Hirko K, Menashe L, Li L, et al. Systematic review of the concurrent and predictive validity of MRI biomarkers in OA. Osteoarthritis Cartilage. (2011) 19(5):557–88. doi: 10.1016/j.joca.2010.10.029
41. Laslett LL, Dore DA, Quinn SJ, Boon P, Ryan E, Winzenberg TM, et al. Zoledronic acid reduces knee pain and bone marrow lesions over 1 year: a randomised controlled trial. Ann Rheum Dis. (2012) 71(8):1322–8. doi: 10.1136/annrheumdis-2011-200970
42. Reginster JY, Badurski J, Bellamy N, Bensen W, Chapurlat R, Chevalier X, et al. Efficacy and safety of strontium ranelate in the treatment of knee osteoarthritis: results of a double-blind, randomised placebo-controlled trial. Ann Rheum Dis. (2013) 72(2):179–86. doi: 10.1136/annrheumdis-2012-202231
43. Suzuki A, Iwata J. Mouse genetic models for temporomandibular joint development and disorders. Oral Dis. (2016) 22(1):33–8. doi: 10.1111/odi.12353
44. Bair E, Gaynor S, Slade GD, Ohrbach R, Fillingim RB, Greenspan JD, et al. Identification of clusters of individuals relevant to temporomandibular disorders and other chronic pain conditions: the OPPERA study. Pain. (2016) 157(6):1266–78. doi: 10.1097/j.pain.0000000000000518
45. Zaki S, Smith MM, Little CB. Pathology-pain relationships in different osteoarthritis animal model phenotypes: it matters what you measure, when you measure, and how you got there. Osteoarthritis Cartilage. (2021) 29(10):1448–61. doi: 10.1016/j.joca.2021.03.023
46. Miller RE, Tran PB, Ishihara S, Larkin J, Malfait AM. Therapeutic effects of an anti-ADAMTS-5 antibody on joint damage and mechanical allodynia in a murine model of osteoarthritis. Osteoarthritis Cartilage. (2016) 24(2):299–306. doi: 10.1016/j.joca.2015.09.005
47. Wei Y, Luo L, Gui T, Yu F, Yan L, Yao L, et al. Targeting cartilage EGFR pathway for osteoarthritis treatment. Sci Transl Med. (2021) 13(576):eabb3946. doi: 10.1126/scitranslmed.abb3946
48. Liao Y, Ren Y, Luo X, Mirando AJ, Long JT, Leinroth A, et al. Interleukin-6 signaling mediates cartilage degradation and pain in posttraumatic osteoarthritis in a sex-specific manner. Sci Signal. (2022) 15(744):eabn7082. doi: 10.1126/scisignal.abn7082
49. Miller RE, Block JA, Malfait AM. Nerve growth factor blockade for the management of osteoarthritis pain: what can we learn from clinical trials and preclinical models? Curr Opin Rheumatol. (2017) 29(1):110–8. doi: 10.1097/BOR.0000000000000354
50. Baddack U, Frahm S, Antolin-Fontes B, Grobe J, Lipp M, Muller G, et al. Suppression of peripheral pain by blockade of voltage-gated calcium 2.2 channels in nociceptors induces RANKL and impairs recovery from inflammatory arthritis in a mouse model. Arthritis Rheumatol. (2015) 67(6):1657–67. doi: 10.1002/art.39094
51. Muschter D, Fleischhauer L, Taheri S, Schilling AF, Clausen-Schaumann H, Grassel S. Sensory neuropeptides are required for bone and cartilage homeostasis in a murine destabilization-induced osteoarthritis model. Bone. (2020) 133:115181. doi: 10.1016/j.bone.2019.115181
52. Liu Q, Yang H, Zhang M, Zhang J, Lu L, Yu S, et al. Initiation and progression of dental-stimulated temporomandibular joints osteoarthritis. Osteoarthritis Cartilage. (2021) 29(5):633–42. doi: 10.1016/j.joca.2020.12.016
53. Zhao Y, An Y, Zhou L, Wu F, Wu G, Wang J, et al. Animal models of temporomandibular joint osteoarthritis: classification and selection. Front Physiol. (2022) 13:859517. doi: 10.3389/fphys.2022.859517
54. Kellesarian SV, Al-Kheraif AA, Vohra F, Ghanem A, Malmstrom H, Romanos GE, et al. Cytokine profile in the synovial fluid of patients with temporomandibular joint disorders: a systematic review. Cytokine. (2016) 77:98–106. doi: 10.1016/j.cyto.2015.11.005
55. Ren K. An improved method for assessing mechanical allodynia in the rat. Physiol Behav. (1999) 67(5):711–6. doi: 10.1016/S0031-9384(99)00136-5
56. Bai Q, Liu S, Shu H, Tang Y, George S, Dong T, et al. TNFalpha in the trigeminal nociceptive system is critical for temporomandibular joint pain. Mol Neurobiol. (2019) 56(1):278–91. doi: 10.1007/s12035-018-1076-y
57. Imbe H, Iwata K, Zhou QQ, Zou S, Dubner R, Ren K. Orofacial deep and cutaneous tissue inflammation and trigeminal neuronal activation. Implications for persistent temporomandibular pain. Cells Tissues Organs. (2001) 169(3):238–47. doi: 10.1159/000047887
58. Chen Y, Williams SH, McNulty AL, Hong JH, Lee SH, Rothfusz NE, et al. Temporomandibular joint pain: a critical role for Trpv4 in the trigeminal ganglion. Pain. (2013) 154(8):1295–304. doi: 10.1016/j.pain.2013.04.004
59. Kim SH, Son CN, Lee HJ, Cho HC, Jung SW, Hur JA, et al. Infliximab partially alleviates the bite force reduction in a mouse model of temporomandibular joint pain. J Korean Med Sci. (2015) 30(5):552–8. doi: 10.3346/jkms.2015.30.5.552
60. Dolan JC, Lam DK, Achdjian SH, Schmidt BL. The dolognawmeter: a novel instrument and assay to quantify nociception in rodent models of orofacial pain. J Neurosci Methods. (2010) 187(2):207–15. doi: 10.1016/j.jneumeth.2010.01.012
61. Kramer PR, Kerins CA, Schneiderman E, Bellinger LL. Measuring persistent temporomandibular joint nociception in rats and two mice strains. Physiol Behav. (2010) 99(5):669–78. doi: 10.1016/j.physbeh.2010.01.037
62. Roveroni RC, Parada CA, Cecilia M, Veiga FA, Tambeli CH. Development of a behavioral model of TMJ pain in rats: the TMJ formalin test. Pain. (2001) 94(2):185–91. doi: 10.1016/S0304-3959(01)00357-8
63. Hartwig AC, Mathias SI, Law AS, Gebhart GF. Characterization and opioid modulation of inflammatory temporomandibular joint pain in the rat. J Oral Maxillofac Surg. (2003) 61(11):1302–9. doi: 10.1016/S0278-2391(03)00732-8
64. Kyrkanides S, Fiorentino PM, Miller JN, Gan Y, Lai YC, Shaftel SS, et al. Amelioration of pain and histopathologic joint abnormalities in the Col1-IL-1beta(XAT) mouse model of arthritis by intraarticular induction of mu-opioid receptor into the temporomandibular joint. Arthritis Rheum. (2007) 56(6):2038–48. doi: 10.1002/art.22635
65. Sperry MM, Yu YH, Welch RL, Granquist EJ, Winkelstein BA. Grading facial expression is a sensitive means to detect grimace differences in orofacial pain in a rat model. Sci Rep. (2018) 8(1):13894. doi: 10.1038/s41598-018-32297-2
66. do Nascimento GC, Leite-Panissi CR. Time-dependent analysis of nociception and anxiety-like behavior in rats submitted to persistent inflammation of the temporomandibular joint. Physiol Behav. (2014) 125:1–7.24291383
67. Sannajust S, Imbert I, Eaton V, Henderson T, Liaw L, May M, et al. Females have greater susceptibility to develop ongoing pain and central sensitization in a rat model of temporomandibular joint pain. Pain. (2019) 160(9):2036–49. doi: 10.1097/j.pain.0000000000001598
68. Romero-Reyes M, Akerman S, Nguyen E, Vijjeswarapu A, Hom B, Dong HW, et al. Spontaneous behavioral responses in the orofacial region: a model of trigeminal pain in mouse. Headache. (2013) 53(1):137–51. doi: 10.1111/j.1526-4610.2012.02226.x
69. Wang S, Lim J, Joseph J, Wei F, Ro JY, Chung MK. Spontaneous and bite-evoked muscle pain are mediated by a common nociceptive pathway with differential contribution by TRPV1. J Pain. (2017) 18(11):1333–45. doi: 10.1016/j.jpain.2017.06.005
70. Wang S, Brigoli B, Lim J, Karley A, Chung MK. Roles of TRPV1 and TRPA1 in spontaneous pain from inflamed masseter muscle. Neuroscience. (2018) 384:290–9. doi: 10.1016/j.neuroscience.2018.05.048
71. Joseph J, Qu L, Wang S, Kim M, Bennett D, Ro J, et al. Phosphorylation of TRPV1 S801 contributes to modality-specific hyperalgesia in mice. J Neurosci. (2019) 39(50):9954–66. doi: 10.1523/JNEUROSCI.1064-19.2019
72. Ro JY. Bite force measurement in awake rats: a behavioral model for persistent orofacial muscle pain and hyperalgesia. J Orofac Pain. (2005) 19(2):159–67. PMID: 15895839.15895839
73. Chen Y, Kanju P, Fang Q, Lee SH, Parekh PK, Lee W, et al. TRPV4 Is necessary for trigeminal irritant pain and functions as a cellular formalin receptor. Pain. (2014) 155(12):2662–72. doi: 10.1016/j.pain.2014.09.033
74. Wang XD, Kou XX, He DQ, Zeng MM, Meng Z, Bi RY, et al. Progression of cartilage degradation, bone resorption and pain in rat temporomandibular joint osteoarthritis induced by injection of iodoacetate. PLoS One. (2012) 7(9):e45036. doi: 10.1371/journal.pone.0045036
75. Wang S, Bian C, Yang J, Arora V, Gao Y, Wei F, et al. Ablation of TRPV1+ afferent terminals by capsaicin mediates long-lasting analgesia for trigeminal neuropathic pain. eNeuro. (2020) 7(3):ENEURO.0118-20.2020. doi: 10.1523/ENEURO.0118-20.2020
76. Sessle BJ. Peripheral and central mechanisms of orofacial inflammatory pain. Int Rev Neurobiol. (2011) 97:179–206. doi: 10.1016/B978-0-12-385198-7.00007-2
77. Bereiter DA, Okamoto K. Neurobiology of estrogen status in deep craniofacial pain. Int Rev Neurobiol. (2011) 97:251–84. doi: 10.1016/B978-0-12-385198-7.00010-2
78. Chichorro JG, Porreca F, Sessle B. Mechanisms of craniofacial pain. Cephalalgia. (2017) 37(7):613–26. doi: 10.1177/0333102417704187
79. Cairns BE. Pathophysiology of TMD pain--basic mechanisms and their implications for pharmacotherapy. J Oral Rehabil. (2010) 37(6):391–410. doi: 10.1111/j.1365-2842.2010.02074.x
80. Chung MK, Wang S, Yang J, Alshanqiti I, Wei F, Ro JY. Neural pathways of craniofacial muscle pain: implications for novel treatments. J Dent Res. (2020) 99(9):1004–12. doi: 10.1177/0022034520919384
81. Chung MK, Ro JY. Peripheral glutamate receptor and transient receptor potential channel mechanisms of craniofacial muscle pain. Mol Pain. (2020) 16:1744806920914204. doi: 10.1177/1744806920914204
82. Chung MK, Wang S, Oh SL, Kim YS. Acute and chronic pain from facial skin and oral Mucosa: unique neurobiology and challenging treatment. Int J Mol Sci. (2021) 22(11). doi: 10.3390/ijms22115810
83. Lam DK, Sessle BJ, Hu JW. Glutamate and capsaicin effects on trigeminal nociception I: activation and peripheral sensitization of deep craniofacial nociceptive afferents. Brain Res. (2009) 1251:130–9. doi: 10.1016/j.brainres.2008.11.029
84. Bellinger LL, Spears R, King CM, Dahm F, Hutchins B, Kerins CA, et al. Capsaicin sensitive neurons role in the inflamed TMJ acute nociceptive response of female and male rats. Physiol Behav. (2007) 90(5):782–9. doi: 10.1016/j.physbeh.2007.01.002
85. Bi RY, Meng Z, Zhang P, Wang XD, Ding Y, Gan YH. Estradiol upregulates voltage-gated sodium channel 1.7 in trigeminal ganglion contributing to hyperalgesia of inflamed TMJ. PLoS One. (2017) 12(6):e0178589. doi: 10.1371/journal.pone.0178589
86. Ahn DK, Choi HS, Yeo SP, Woo YW, Lee MK, Yang GY, et al. Blockade of central cyclooxygenase (COX) pathways enhances the cannabinoid-induced antinociceptive effects on inflammatory temporomandibular joint (TMJ) nociception. Pain. (2007) 132(1-2):23–32. doi: 10.1016/j.pain.2007.01.015
87. Zhang X, Hartung JE, Bortsov AV, Kim S, O’Buckley SC, Kozlowski J, et al. Sustained stimulation of beta2- and beta3-adrenergic receptors leads to persistent functional pain and neuroinflammation. Brain Behav Immun. (2018) 73:520–32. doi: 10.1016/j.bbi.2018.06.017
88. Favaro-Moreira NC, Parada CA, Tambeli CH. Blockade of beta(1)-, beta(2)- and beta(3)-adrenoceptors in the temporomandibular joint induces antinociception especially in female rats. Eur J Pain. (2012) 16(9):1302–10. doi: 10.1002/j.1532-2149.2012.00132.x
89. Kojima Y. Convergence patterns of afferent information from the temporomandibular joint and masseter muscle in the trigeminal subnucleus caudalis. Brain Res Bull. (1990) 24(4):609–16. doi: 10.1016/0361-9230(90)90167-X
90. Nishikawa T, Takeda M, Tanimoto T, Matsumoto S. Convergence of nociceptive information from temporomandibular joint and tooth pulp afferents on C1 spinal neurons in the rat. Life Sci. (2004) 75(12):1465–78. doi: 10.1016/j.lfs.2004.03.014
91. Yu XM, Sessle BJ, Vernon H, Hu JW. Effects of inflammatory irritant application to the rat temporomandibular joint on jaw and neck muscle activity. Pain. (1995) 60(2):143–9. doi: 10.1016/0304-3959(94)00104-M
92. Morch CD, Hu JW, Arendt-Nielsen L, Sessle BJ. Convergence of cutaneous, musculoskeletal, dural and visceral afferents onto nociceptive neurons in the first cervical dorsal horn. Eur J Neurosci. (2007) 26(1):142–54. doi: 10.1111/j.1460-9568.2007.05608.x
93. Wang S, Song L, Tan Y, Ma Y, Tian Y, Jin X, et al. A functional relationship between trigeminal astroglial activation and NR1 expression in a rat model of temporomandibular joint inflammation. Pain Med. (2012) 13(12):1590–600. doi: 10.1111/j.1526-4637.2012.01511.x
94. Won KA, Kang YM, Lee MK, Park MK, Ju JS, Bae YC, et al. Participation of microglial p38 MAPK in formalin-induced temporomandibular joint nociception in rats. J Orofac Pain. (2012) 26(2):132–41. PMID: 22558613.22558613
95. Cavalcante AL, Siqueira RM, Araujo JC, Gondim DV, Ribeiro RA, Quetz JS, et al. Role of NMDA receptors in the trigeminal pathway, and the modulatory effect of magnesium in a model of rat temporomandibular joint arthritis. Eur J Oral Sci. (2013) 121(6):573–83. doi: 10.1111/eos.12093
96. Lam DK, Sessle BJ, Hu JW. Glutamate and capsaicin effects on trigeminal nociception II: activation and central sensitization in brainstem neurons with deep craniofacial afferent input. Brain Res. (2009) 1253:48–59. doi: 10.1016/j.brainres.2008.11.056
97. Garrett FG, Durham PL. Differential expression of connexins in trigeminal ganglion neurons and satellite glial cells in response to chronic or acute joint inflammation. Neuron Glia Biol. (2008) 4(4):295–306. doi: 10.1017/S1740925X09990093
98. Freeman SE, Patil VV, Durham PL. Nitric oxide-proton stimulation of trigeminal ganglion neurons increases mitogen-activated protein kinase and phosphatase expression in neurons and satellite glial cells. Neuroscience. (2008) 157(3):542–55. doi: 10.1016/j.neuroscience.2008.09.035
99. Magni G, Merli D, Verderio C, Abbracchio MP, Ceruti S. P2y2 receptor antagonists as anti-allodynic agents in acute and sub-chronic trigeminal sensitization: role of satellite glial cells. Glia. (2015) 63(7):1256–69. doi: 10.1002/glia.22819
100. Kou XX, Li CS, He DQ, Wang XD, Hao T, Meng Z, et al. Estradiol promotes M1-like macrophage activation through cadherin-11 to aggravate temporomandibular joint inflammation in rats. J Immunol. (2015) 194(6):2810–8. doi: 10.4049/jimmunol.1303188
101. Flake NM, Hermanstyne TO, Gold MS. Testosterone and estrogen have opposing actions on inflammation-induced plasma extravasation in the rat temporomandibular joint. Am J Physiol Regul Integr Comp Physiol. (2006) 291(2):R343–8. doi: 10.1152/ajpregu.00835.2005
102. Xue XT, Kou XX, Li CS, Bi RY, Meng Z, Wang XD, et al. Progesterone attenuates temporomandibular joint inflammation through inhibition of NF-kappaB pathway in ovariectomized rats. Sci Rep. (2017) 7(1):15334. doi: 10.1038/s41598-017-15285-w
103. Torres-Chavez KE, Fischer L, Teixeira JM, Favaro-Moreira NC, Obando-Pereda GA, Parada CA, et al. Sexual dimorphism on cytokines expression in the temporomandibular joint: the role of gonadal steroid hormones. Inflammation. (2011) 34(5):487–98. doi: 10.1007/s10753-010-9256-6
104. Flake NM, Bonebreak DB, Gold MS. Estrogen and inflammation increase the excitability of rat temporomandibular joint afferent neurons. J Neurophysiol. (2005) 93(3):1585–97. doi: 10.1152/jn.00269.2004
105. Kramer PR, Bellinger LL. The effects of cycling levels of 17beta-estradiol and progesterone on the magnitude of temporomandibular joint-induced nociception. Endocrinology. (2009) 150(8):3680–9. doi: 10.1210/en.2008-1707
106. Fischer L, Clemente JT, Tambeli CH. The protective role of testosterone in the development of temporomandibular joint pain. J Pain. (2007) 8(5):437–42. doi: 10.1016/j.jpain.2006.12.007
107. Fischer L, Torres-Chavez KE, Clemente-Napimoga JT, Jorge D, Arsati F, de Arruda Veiga MC, et al. The influence of sex and ovarian hormones on temporomandibular joint nociception in rats. J Pain. (2008) 9(7):630–8. doi: 10.1016/j.jpain.2008.02.006
108. Okamoto K, Thompson R, Katagiri A, Bereiter DA. Estrogen status and psychophysical stress modify temporomandibular joint input to medullary dorsal horn neurons in a lamina-specific manner in female rats. Pain. (2013) 154(7):1057–64. doi: 10.1016/j.pain.2013.03.009
109. Bereiter DA, Shen S, Benetti AP. Sex differences in amino acid release from rostral trigeminal subnucleus caudalis after acute injury to the TMJ region. Pain. (2002) 98(1–2):89–99. doi: 10.1016/S0304-3959(01)00476-6
110. Morel M, Ruscitto A, Pylawka S, Reeve G, Embree MC. Extracellular matrix turnover and inflammation in chemically-induced TMJ arthritis mouse models. PLoS One. (2019) 14(10):e0223244. doi: 10.1371/journal.pone.0223244
111. Xu L, Guo H, Li C, Xu J, Fang W, Long X. A time-dependent degeneration manner of condyle in rat CFA-induced inflamed TMJ. Am J Transl Res. (2016) 8(2):556–67. PMID: 27158347; PMCID: 4846904.27158347
112. Wang XD, Kou XX, Mao JJ, Gan YH, Zhou YH. Sustained inflammation induces degeneration of the temporomandibular joint. J Dent Res. (2012) 91(5):499–505. doi: 10.1177/0022034512441946
113. Kuroki Y, Honda K, Kijima N, Wada T, Arai Y, Matsumoto N, et al. In vivo morphometric analysis of inflammatory condylar changes in rat temporomandibular joint. Oral Dis. (2011) 17(5):499–507. doi: 10.1111/j.1601-0825.2010.01782.x
114. Bapat S, Hubbard D, Munjal A, Hunter M, Fulzele S. Pros and cons of mouse models for studying osteoarthritis. Clin Transl Med. (2018) 7(1):36. doi: 10.1186/s40169-018-0215-4
115. Alves-Simoes M. Rodent models of knee osteoarthritis for pain research. Osteoarthritis Cartilage. (2022) 30(6):802–14. doi: 10.1016/j.joca.2022.01.010
116. Otte P. Basic cell metabolism of articular cartilage. Manometric studies. Z Rheumatol. (1991) 50(5):304–12. PMID: 1776367.1776367
117. Sabri MI, Ochs S. Inhibition of glyceraldehyde-3-phosphate dehydrogenase in mammalian nerve by iodoacetic acid. J Neurochem. (1971) 18(8):1509–14. doi: 10.1111/j.1471-4159.1971.tb00013.x
118. Sharma S, Wactawski-Wende J, LaMonte MJ, Zhao J, Slade GD, Bair E, et al. Incident injury is strongly associated with subsequent incident temporomandibular disorder: results from the OPPERA study. Pain. (2019) 160(7):1551–61. doi: 10.1097/j.pain.0000000000001554
119. Sharma S, Ohrbach R, Fillingim RB, Greenspan JD, Slade G. Pain sensitivity modifies risk of injury-related temporomandibular disorder. J Dent Res. (2020) 99(5):530–6. doi: 10.1177/0022034520913247
120. Ku JK, Baik SH, Kim JY, Huh JK. Follow-up evaluation of temporomandibular joints using magnetic resonance imaging after mandibular trauma: case series analysis of young adult males. Dent Traumatol. (2022) 38(2):136–42. doi: 10.1111/edt.12719
121. Fujisawa T, Kuboki T, Kasai T, Sonoyama W, Kojima S, Uehara J, et al. A repetitive, steady mouth opening induced an osteoarthritis-like lesion in the rabbit temporomandibular joint. J Dent Res. (2003) 82(9):731–5. doi: 10.1177/154405910308200914
122. Kartha S, Zhou T, Granquist EJ, Winkelstein BA. Development of a rat model of mechanically induced tunable pain and associated temporomandibular joint responses. J Oral Maxillofac Surg. (2016) 74(1):54 e1–10. doi: 10.1016/j.joms.2015.09.005
123. Hawkins JL, Durham PL. Prolonged jaw opening promotes nociception and enhanced cytokine expression. J Oral Facial Pain Headache. (2016) 30(1):34–41. doi: 10.11607/ofph.1557
124. Nicoll SB, Hee CK, Davis MB, Winkelstein BA. A rat model of temporomandibular joint pain with histopathologic modifications. J Orofac Pain. (2010) 24(3):298–304. PMID: 20664832.20664832
125. Sperry MM, Yu YH, Kartha S, Ghimire P, Welch RL, Winkelstein BA, et al. Intra-articular etanercept attenuates pain and hypoxia from TMJ loading in the rat. J Orthop Res. (2020) 38(6):1316–26. doi: 10.1002/jor.24581
126. Franklin M, Sperry MM, Phillips E, Granquist EJ, Marcolongo M, Winkelstein BA. Painful temporomandibular joint overloading induces structural remodeling in the pericellular matrix of that joint’s chondrocytes. J Orthop Res. (2022) 40(2):348–58. doi: 10.1002/jor.25050
127. Wang GYF, Shi XQ, Wu W, Gueorguieva M, Yang M, Zhang J. Sustained and repeated mouth opening leads to development of painful temporomandibular disorders involving macrophage/microglia activation in mice. Pain. (2018) 159(7):1277–88. doi: 10.1097/j.pain.0000000000001206
128. Izawa T, Mori H, Shinohara T, Mino-Oka A, Hutami IR, Iwasa A, et al. Rebamipide attenuates mandibular condylar degeneration in a murine model of TMJ-OA by mediating a chondroprotective effect and by downregulating RANKL-mediated osteoclastogenesis. PLoS One. (2016) 11(4):e0154107. doi: 10.1371/journal.pone.0154107
129. Moon SJ, Woo YJ, Jeong JH, Park MK, Oh HJ, Park JS, et al. Rebamipide attenuates pain severity and cartilage degeneration in a rat model of osteoarthritis by downregulating oxidative damage and catabolic activity in chondrocytes. Osteoarthritis Cartilage. (2012) 20(11):1426–38. doi: 10.1016/j.joca.2012.08.002
130. Cao Y. Occlusal disharmony and chronic oro-facial pain: from clinical observation to animal study. J Oral Rehabil. (2022) 49(2):116–24. doi: 10.1111/joor.13236
131. Clark GT, Tsukiyama Y, Baba K, Watanabe T. Sixty-eight years of experimental occlusal interference studies: what have we learned? J Prosthet Dent. (1999) 82(6):704–13. doi: 10.1016/S0022-3913(99)70012-0
132. National Academies of Sciences E, Medicine, Bond EC, Mackey S, English R, Liverman CT, Yost O, editors Temporomandibular disorders: Priorities for research and care. Washington, DC: The National Academies Press (2020). 426 p.
133. Glasson SS, Blanchet TJ, Morris EA. The surgical destabilization of the medial meniscus (DMM) model of osteoarthritis in the 129/SvEv mouse. Osteoarthritis Cartilage. (2007) 15(9):1061–9. doi: 10.1016/j.joca.2007.03.006
134. Inglis JJ, McNamee KE, Chia SL, Essex D, Feldmann M, Williams RO, et al. Regulation of pain sensitivity in experimental osteoarthritis by the endogenous peripheral opioid system. Arthritis Rheum. (2008) 58(10):3110–9. doi: 10.1002/art.23870
135. Gowler PRW, Mapp PI, Burston JJ, Shahtaheri M, Walsh DA, Chapman V. Refining surgical models of osteoarthritis in mice and rats alters pain phenotype but not joint pathology. PLoS ONE. (2020) 15(9):e0239663. doi: 10.1371/journal.pone.0239663
136. Hinton RJ. Alterations in rat condylar cartilage following discectomy. J Dent Res. (1992) 71(6):1292–7. doi: 10.1177/00220345920710060501
137. Lan L, Jiang Y, Zhang W, Li T, Ying B, Zhu S. Expression of Notch signaling pathway during osteoarthritis in the temporomandibular joint. J Craniomaxillofac Surg. (2017) 45(8):1338–48. doi: 10.1016/j.jcms.2017.05.029
138. Liu X, Cai HX, Cao PY, Feng Y, Jiang HH, Liu L, et al. TLR4 Contributes to the damage of cartilage and subchondral bone in discectomy-induced TMJOA mice. J Cell Mol Med. (2020) 24(19):11489–99. doi: 10.1111/jcmm.15763
139. Xu L, Polur I, Lim C, Servais JM, Dobeck J, Li Y, et al. Early-onset osteoarthritis of mouse temporomandibular joint induced by partial discectomy. Osteoarthritis Cartilage. (2009) 17(7):917–22. doi: 10.1016/j.joca.2009.01.002
140. Cohen WA, Servais JM, Polur I, Li Y, Xu L. Articular cartilage degeneration in the contralateral non-surgical temporomandibular joint in mice with a unilateral partial discectomy. J Oral Pathol Med. (2014) 43(2):162–5. doi: 10.1111/jop.12113
141. Luo P, Peng S, Yan Y, Ji P, Xu J. IL-37 inhibits M1-like macrophage activation to ameliorate temporomandibular joint inflammation through the NLRP3 pathway. Rheumatology (Oxford). (2020) 59(10):3070–80. doi: 10.1093/rheumatology/keaa192
142. Nguyen NGK, Nishiyama A, Shimada M. A rat model for inducing temporomandibular anterior disc displacement experimentally. J Oral Sci. (2020) 62(1):70–4. doi: 10.2334/josnusd.19-0093
143. Togni L, de Abreu MC, Augustin AH, da Silva RBM, Campos MM. Characterization of a rat model with temporomandibular joint osteoarthritis following a surgical anterior disc displacement. Am J Transl Res. (2018) 10(11):3806–17. PMID: 30662631; PMCID: 6291709.30662631
144. Lekkas C, Honee GL, van den Hooff A. Effects of experimental defects of the articular disc of the temporomandibular joint in rats. J Oral Rehabil. (1988) 15(2):141–8. doi: 10.1111/j.1365-2842.1988.tb00763.x
145. Luo P, Feng C, Jiang C, Ren X, Gou L, Ji P, et al. IL-37b alleviates inflammation in the temporomandibular joint cartilage via IL-1R8 pathway. Cell Prolif. (2019) 52(6):e12692. doi: 10.1111/cpr.12692
146. Xu L, Flahiff CM, Waldman BA, Wu D, Olsen BR, Setton LA, et al. Osteoarthritis-like changes and decreased mechanical function of articular cartilage in the joints of mice with the chondrodysplasia gene (cho). Arthritis Rheum. (2003) 48(9):2509–18. doi: 10.1002/art.11233
147. Ricks ML, Farrell JT, Falk DJ, Holt DW, Rees M, Carr J, et al. Osteoarthritis in temporomandibular joint of Col2a1 mutant mice. Arch Oral Biol. (2013) 58(9):1092–9. doi: 10.1016/j.archoralbio.2013.02.008
148. Rintala M, Metsaranta M, Saamanen AM, Vuorio E, Ronning O. Abnormal craniofacial growth and early mandibular osteoarthritis in mice harbouring a mutant type II collagen transgene. J Anat. (1997) 190(Pt 2):201–8. doi: 10.1046/j.1469-7580.1997.19020201.x
149. Wadhwa S, Embree M, Ameye L, Young MF. Mice deficient in biglycan and fibromodulin as a model for temporomandibular joint osteoarthritis. Cells Tissues Organs. (2005) 181(3–4):136–43. doi: 10.1159/000091375
150. Bechtold TE, Saunders C, Decker RS, Um HB, Cottingham N, Salhab I, et al. Osteophyte formation and matrix mineralization in a TMJ osteoarthritis mouse model are associated with ectopic hedgehog signaling. Matrix Biol. (2016):52–54:339–54. doi: 10.1016/j.matbio.2016.03.001
151. Schminke B, Muhammad H, Bode C, Sadowski B, Gerter R, Gersdorff N, et al. A discoidin domain receptor 1 knock-out mouse as a novel model for osteoarthritis of the temporomandibular joint. Cell Mol Life Sci. (2014) 71(6):1081–96. doi: 10.1007/s00018-013-1436-8
152. Puzas JE, Landeau JM, Tallents R, Albright J, Schwarz EM, Landesberg R. Degradative pathways in tissues of the temporomandibular joint. Use of in vitro and in vivo models to characterize matrix metalloproteinase and cytokine activity. Cells Tissues Organs. (2001) 169(3):248–56. doi: 10.1159/000047888
153. Kaneyama K, Segami N, Nishimura M, Suzuki T, Sato J. Importance of proinflammatory cytokines in synovial fluid from 121 joints with temporomandibular disorders. Br J Oral Maxillofac Surg. (2002) 40(5):418–23. doi: 10.1016/S0266-4356(02)00215-2
154. Alstergren P, Ernberg M, Kvarnstrom M, Kopp S. Interleukin-1beta in synovial fluid from the arthritic temporomandibular joint and its relation to pain, mobility, and anterior open bite. J Oral Maxillofac Surg. (1998) 56(9):1059–65; discussion 66. doi: 10.1016/S0278-2391(98)90256-7
155. Nordahl S, Alstergren P, Kopp S. Tumor necrosis factor-alpha in synovial fluid and plasma from patients with chronic connective tissue disease and its relation to temporomandibular joint pain. J Oral Maxillofac Surg. (2000) 58(5):525–30. doi: 10.1016/S0278-2391(00)90015-6
156. Spears R, Dees LA, Sapozhnikov M, Bellinger LL, Hutchins B. Temporal changes in inflammatory mediator concentrations in an adjuvant model of temporomandibular joint inflammation. J Orofac Pain. (2005) 19(1):34–40. PMID: 15779537.15779537
157. Lai YC, Shaftel SS, Miller JN, Tallents RH, Chang Y, Pinkert CA, et al. Intraarticular induction of interleukin-1beta expression in the adult mouse, with resultant temporomandibular joint pathologic changes, dysfunction, and pain. Arthritis Rheum. (2006) 54(4):1184–97. doi: 10.1002/art.21771
158. Fiorentino PM, Tallents RH, Miller JN, Brouxhon SM, O’Banion MK, Puzas JE, et al. Spinal interleukin-1beta in a mouse model of arthritis and joint pain. Arthritis Rheum. (2008) 58(10):3100–9. doi: 10.1002/art.23866
159. Gao YJ, Ji RR. Chemokines, neuronal-glial interactions, and central processing of neuropathic pain. Pharmacol Ther. (2010) 126(1):56–68. doi: 10.1016/j.pharmthera.2010.01.002
160. Dobsak T, Heimel P, Tangl S, Schwarze UY, Schett G, Gruber R. Impaired periodontium and temporomandibular joints in tumour necrosis factor-alpha transgenic mice. J Clin Periodontol. (2017) 44(12):1226–35. doi: 10.1111/jcpe.12799
161. Durham ZL, Hawkins JL, Durham PL. Tumor necrosis factor-Alpha stimulates cytokine expression and transient sensitization of trigeminal nociceptive neurons. Arch Oral Biol. (2017) 75:100–6. doi: 10.1016/j.archoralbio.2016.10.034
162. Spears R, Oakes R, Moore C, Bellinger LL, Hutchins B. A determination of tumor necrosis factor expression in TMJ inflammation with the use of microarray analysis. J Dent Res. (2003) 82(10):807–13. doi: 10.1177/154405910308201009
163. Fillingim RB, Ohrbach R, Greenspan JD, Knott C, Diatchenko L, Dubner R, et al. Psychological factors associated with development of TMD: the OPPERA prospective cohort study. J Pain. (2013) 14(12 Suppl):T75–90. doi: 10.1016/j.jpain.2013.06.009
164. Gameiro GH, Gameiro PH, Andrade Ada S, Pereira LF, Arthuri MT, Marcondes FK, et al. Nociception- and anxiety-like behavior in rats submitted to different periods of restraint stress. Physiol Behav. (2006) 87(4):643–9. doi: 10.1016/j.physbeh.2005.12.007
165. Wu G, Chen L, Wei G, Li Y, Zhu G, Zhao Z, et al. Effects of sleep deprivation on pain-related factors in the temporomandibular joint. J Surg Res. (2014) 192(1):103–11. doi: 10.1016/j.jss.2014.05.035
166. Okamoto K, Tashiro A, Chang Z, Thompson R, Bereiter DA. Temporomandibular joint-evoked responses by spinomedullary neurons and masseter muscle are enhanced after repeated psychophysical stress. Eur J Neurosci. (2012) 36(1):2025–34. doi: 10.1111/j.1460-9568.2012.08100.x
167. Li Q, Zhang M, Chen YJ, Zhou Q, Wang YJ, Liu J. Psychological stress alters microstructure of the mandibular condyle in rats. Physiol Behav. (2013) 110–111:129–39. doi: 10.1016/j.physbeh.2013.01.007
168. Huang X, Liu H, Xiao P, Wang Y, Zhang H. Effect of psychological stress on the structure of the temporomandibular joint and the expression of MMP-3 and TIMP-3 in the cartilage in rats. Br J Oral Maxillofac Surg. (2014) 52(8):709–14. doi: 10.1016/j.bjoms.2014.06.016
169. Wu G, Chen L, Su Y, Zhu G, Wang P, Wang Y, et al. The influence of psychological stress on the rat temporomandibular joint with the application of countermeasures. J Surg Res. (2012) 178(2):728–36. doi: 10.1016/j.jss.2012.06.016
170. Jiao K, Zeng G, Niu LN, Yang HX, Ren GT, Xu XY, et al. Activation of alpha2A-adrenergic signal transduction in chondrocytes promotes degenerative remodelling of temporomandibular joint. Sci Rep. (2016) 6:30085. doi: 10.1038/srep30085
171. Ma C, Wu G, Wang Z, Wang P, Wu L, Zhu G, et al. Effects of chronic sleep deprivation on the extracellular signal-regulated kinase pathway in the temporomandibular joint of rats. PLoS One. (2014) 9(9):e107544. doi: 10.1371/journal.pone.0107544
172. Traub RJ, Cao DY, Karpowicz J, Pandya S, Ji Y, Dorsey SG, et al. A clinically relevant animal model of temporomandibular disorder and irritable bowel syndrome comorbidity. J Pain. (2014) 15(9):956–66. doi: 10.1016/j.jpain.2014.06.008
173. Zhao YJ, Li JH, Hu B, Wang Y, Chang XF, Traub RJ, et al. Extracellular signal-regulated kinase activation in the spinal cord contributes to visceral hypersensitivity induced by craniofacial injury followed by stress. Neurogastroenterol Motil. (2018) 30(2):10.1111/nmo.13161. doi: 10.1111/nmo.13161
174. McIlwrath SL, Nesemeier R, Ma F, Oz HS, Zhang L, Westlund KN. Inflammatory “double hit” model of temporomandibular joint disorder with elevated CCL2, CXCL9, CXCL10, RANTES and behavioural hypersensitivity in TNFR1/R2-/- mice. Eur J Pain. (2017) 21(7):1209–23. doi: 10.1002/ejp.1021
175. Xiang T, Li JH, Su HY, Bai KH, Wang S, Traub RJ, et al. Spinal CCK1 receptors contribute to somatic pain hypersensitivity induced by malocclusion via a reciprocal neuron-glial signaling cascade. J Pain. (2022) 23(10):1629–45. doi: 10.1016/j.jpain.2022.05.009
176. Ma Y, Liu S, Shu H, Crawford J, Xing Y, Tao F. Resveratrol alleviates temporomandibular joint inflammatory pain by recovering disturbed gut microbiota. Brain Behav Immun. (2020) 87:455–64. doi: 10.1016/j.bbi.2020.01.016
177. Boer CG, Radjabzadeh D, Medina-Gomez C, Garmaeva S, Schiphof D, Arp P, et al. Intestinal microbiome composition and its relation to joint pain and inflammation. Nat Commun. (2019) 10(1):4881. doi: 10.1038/s41467-019-12873-4
Keywords: temporomandibular joint, osteoarthritis, degeneration, pain, models
Citation: Chung M, Wang S, Alshanqiti I, Hu J and Ro JY (2023) The degeneration-pain relationship in the temporomandibular joint: Current understandings and rodent models. Front. Pain Res. 4:1038808. doi: 10.3389/fpain.2023.1038808
Received: 7 September 2022; Accepted: 18 January 2023;
Published: 9 February 2023.
Edited by:
Brian E Cairns, University of British Columbia, CanadaReviewed by:
Jie Lei, Peking University Hospital of Stomatology, ChinaCarolina Beraldo Meloto, McGill University, Canada
© 2023 Chung, Wang, Alshanqiti, Hu and Ro. This is an open-access article distributed under the terms of the Creative Commons Attribution License (CC BY). The use, distribution or reproduction in other forums is permitted, provided the original author(s) and the copyright owner(s) are credited and that the original publication in this journal is cited, in accordance with accepted academic practice. No use, distribution or reproduction is permitted which does not comply with these terms.
*Correspondence: Man-Kyo Chung mchung@umaryland.edu
Specialty Section: This article was submitted to Musculoskeletal Pain, a section of the journal Frontiers in Pain Research