- 1Laboratory of Molecular Neuro-Oncology, Department of Neurology, Clinical Neuroscience Center, University of Zurich, Zurich, Switzerland
- 2Laboratory of Molecular Neuro-Oncology, Department of Neurology, Clinical Neuroscience Center, University Hospital Zurich, Zurich, Switzerland
- 3Institute of Neuropathology, Medical Faculty, Heinrich Heine University and University Hospital Düsseldorf, Düsseldorf, Germany
- 4Department of Neurosurgery, Clinical Neuroscience Center, University Hospital and University of Zurich, Zurich, Switzerland
The methylation status of the O6-methylguanine DNA methyltransferase (MGMT) promoter region is a critical predictor of response to alkylating agents in glioblastoma. However, current approaches to study the MGMT status focus on analyzing models with non-identical backgrounds. Here, we present an epigenetic editing approach using CRISPRoff to introduce site-specific CpG methylation in the MGMT promoter region of glioma cell lines. Sanger sequencing revealed successful introduction of methylation, effectively generating differently methylated glioma cell lines with an isogenic background. The introduced methylation resulted in reduced MGMT mRNA and protein levels. Furthermore, the cell lines with MGMT promoter region methylation exhibited increased sensitivity to temozolomide, consistent with the impact of methylation on treatment outcomes in patients with glioblastoma. This precise epigenome-editing approach provides valuable insights into the functional relevance of MGMT promoter regional methylation and its potential for prognostic and predictive assessments, as well as epigenetic-targeted therapies.
Introduction
O6-methylguanine DNA methyltransferase (MGMT) is a DNA repair protein, encoded by the MGMT gene. Its promoter region is made up of a cytosine-phosphate-guanine (CpG) island, which is a stretch of DNA containing 98 CpG sites within a region spanning approximately 1.2 kilobases around the transcription start site (1). This CpG island has been observed to be methylated in a variety of cancers, including glioblastoma, colorectal cancer, non-small cell lung cancer, pancreatic cancer, and others (2–4).
Aberrant methylation of the promoter region of the MGMT gene is of particular interest in glioblastoma patients, as it is frequently described as the most relevant predictor of response to alkylating agents such as temozolomide (TMZ) (5). Most approaches to explore the biological role of MGMT have focused on analyzing the MGMT promoter region in cells from non-identical backgrounds, by means such as siRNA (6), enzymatic inhibition (7) as well as gene knock-out (8).
Here we present an experimental approach based on recent advancements in epigenetic editing, namely CRISPRoff (9), to enable the study of the impact of different MGMT promoter region methylation patterns in isogenic glioma cell lines.
Results
Targeting the MGMT promoter region using CRISPRoff in T-325 cells
Using a catalytically impaired CRISPR/Cas9 system conjugated to a methyltransferase, we introduced CpG site methylation specifically across the MGMT promoter region. This epigenetic modification was then quantified by sodium bisulfite conversion of DNA followed by Sanger sequencing of the CpG island spanning 98 CpG sites within the MGMT promoter region (Figure 1A). While the MGMT promoter region was mostly unmethylated in T-325 wildtype (WT) cells, methylation was successfully introduced across the MGMT promoter region, with averaged methylation levels of 36% after a single transfection (T-325 M.OFF) and 33% after repeat transfections (T-325 M.OFF3), compared to 12% for T-325 WT. These results were reproduced in LN-18 cells where the averaged methylation levels were increased from 8% for LN-18 WT to 30% for the edited cells (LN-18 M.OFF). These results were corroborated for T-325 WT and T-325 M.OFF using pyrosequencing of sodium bisulfite converted DNA spanning a short segment of the MGMT promoter region (Figure 1B).
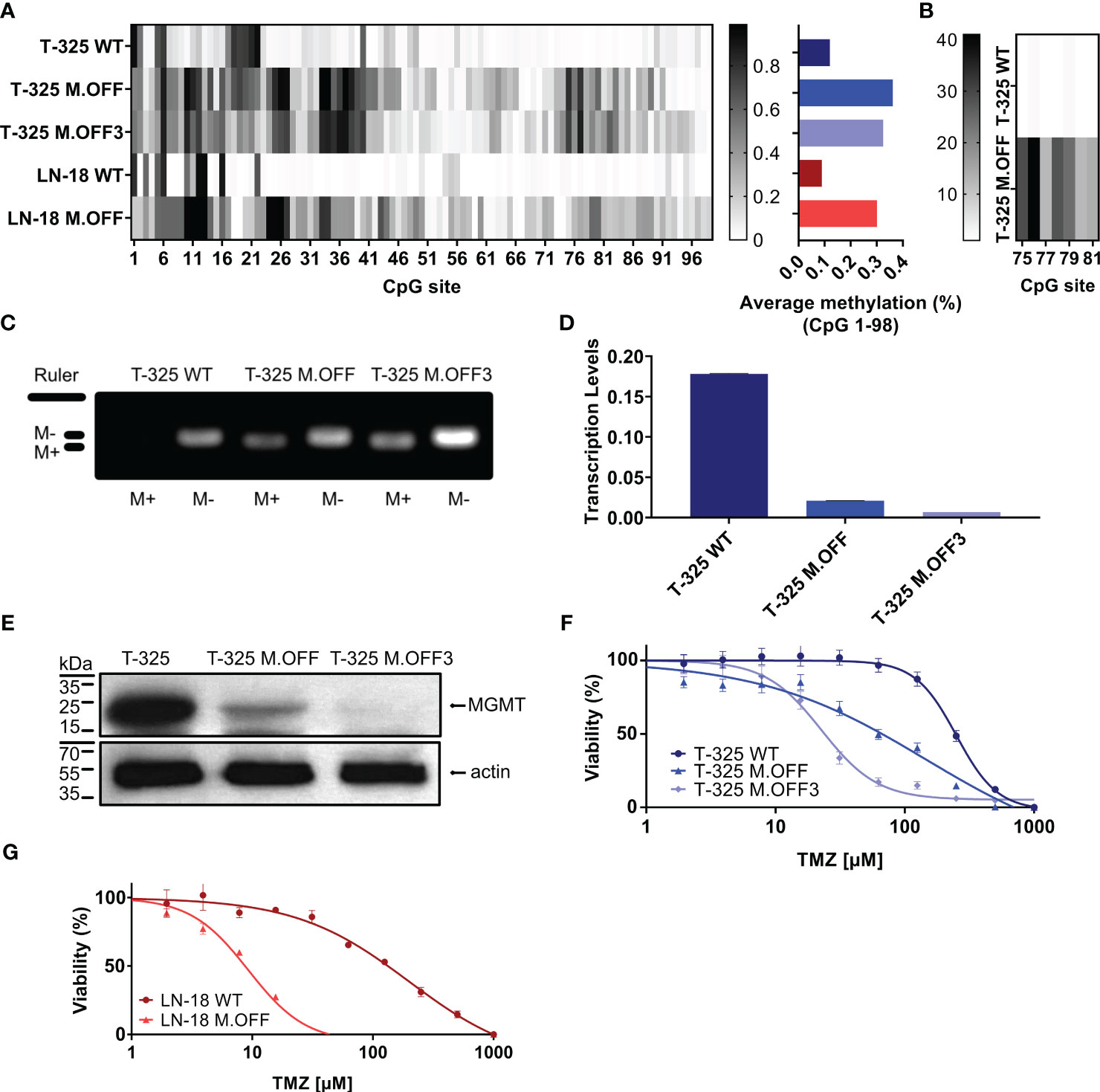
Figure 1 Epigenetic modification of the MGMT promotor region in glioma cells. (A) Methylation profile of the MGMT promotor region, determined by Sanger sequencing of sodium bisulfite-treated DNA (EpiTect Bisulfite Kit, Qiagen) of untreated cells (T-325 WT, LN-18 WT) and cells transiently transfected with the CRISPRoff system targeting MGMT once (T-325 M.OFF, LN-18 M.OFF) or three times (T-325 M.OFF3) with six sgRNAs. (B) Pyrosequencing of a short segment of the MGMT promoter region spanning 7 CpG sites in T-325 cells. (C) Agarose gel of methylation-specific PCR products to quantify MGMT promoter methylation of the three T-325 cell lines (M+/M-: primers specific to methylated/unmethylated DNA (1)), cropped (original presented in Supplementary Figure 1A). (D) Transcript levels of MGMT in T-325 cells measured by qPCR normalized to HPRT. (E) MGMT protein levels in T-325 cells quantified by immunoblot, cropped (original presented in Supplementary Figures 1B, C). F,G. MTT assay to assess temozolomide sensitivity with and without epigenetic modification of the MGMT promoter region in T-325 (F) and LN-18 (G).
To further substantiate the induced change in methylation status, we analyzed the three T-325 cell lines by methylation-specific PCR (MSP), an assay commonly applied in the clinical setting (1) (Figure 1C). Visualizing the MSP products on an agarose gel showed bands for the unmethylated PCR product in T-325 wildtype cells, while T-325 M.OFF and T-325 M.OFF3 showed bands for both unmethylated and methylated PCR products. This epigenetic modification resulted in a decrease in transcript levels in comparison to the T-325 WT cells for T-325 M.OFF, with an even more pronounced decrease in T-325 M.OFF3 (Figures 1D, E). At the same time, quantification of protein levels with immunoblot showed a reduction of the MGMT protein bands that correlates to the mRNA levels.
We next assessed the relative sensitivity to temozolomide in the T-325 and LN-18 cell lines. A strong decrease in the EC50 for temozolomide was observed after introducing the MGMT promotor region methylation for both cell lines. In T-325 cells, the TMZ EC50 decreased from 250 µM to 150 µM and 20 µM (T-325 WT, T-325 M.OFF, T-325 M.OFF3), while LN-18 cells experienced a similar reduction in their TMZ EC50 from 200 µM to 10 µM (LN-18 WT, LN-18 M.OFF) (Figures 1F, G).
Impact of MGMT promoter region methylation on cell proliferation and radiosensitivity
To further elucidate the impact of the MGMT promoter region methylation, proliferation and radiosensitivity of modified cell lines were analyzed. The analysis of cell growth showed a significant difference between the edited and unedited cell lines with a positive correlation between degree of methylation and proliferation (Figure 2A). This was further analyzed in limited dilution conditions, where again, cells with higher methylation levels in the MGMT promoter region showed an increase in proliferation (Figure 2B). To determine whether the MGMT promoter region methylation status has an impact on radiosensitivity, the three cell lines were subjected to different doses of irradiation. While higher radiation dose led to a lower cell viability, no differences were observed between the three cell lines (Figure 2C).
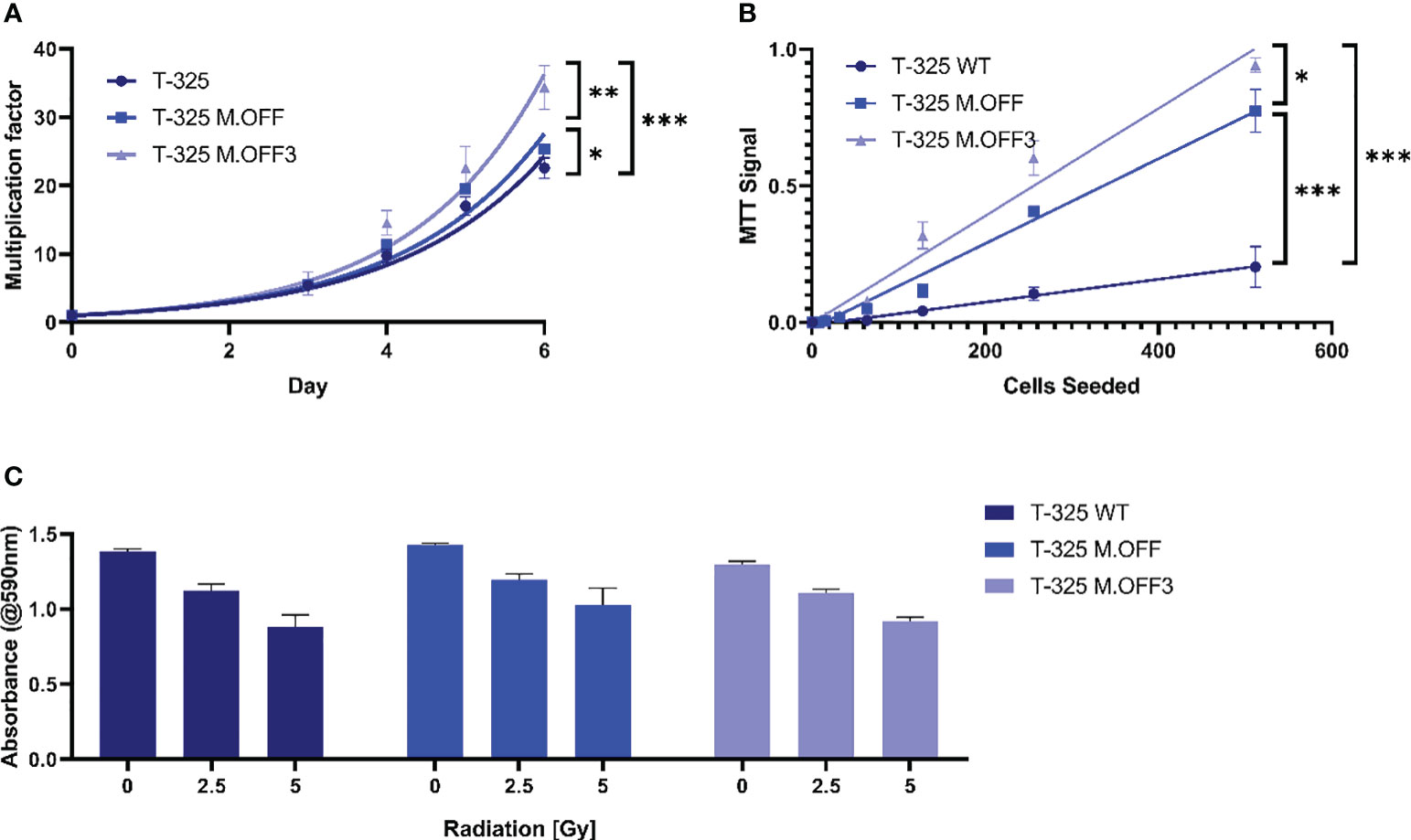
Figure 2 The impact of the MGMT promoter region methylation on cell proliferation and radiosensitivity. (A) Cell proliferation assessment of T-325 WT, M.OFF and M.OFF3 as determined by counting Hoechst 33342 stained nuclei over the course of 7 days. (B) Cell proliferation under limited dilution conditions, as determined by an MTT assay performed 10 days after seeding the specified cell number indicated on the x-axis. (C) Radiosensitivity, as determined by an MTT assay after irradiating cells with 0, 2.5 or 5 Gy twice with a 5-day interval followed by a 5-day incubation. *p<0.05; **p<0.01; ***p<0.001.
Discussion
While there have been multiple attempts to overcome MGMT-mediated TMZ resistance in glioma cells, by means such as siRNA (6), enzymatic inhibition (7) or knock-out (8), the specific induction of individual CpG methylation has so far not been recreated in a laboratory environment. Here we effectively introduced this epigenetic modification, as confirmed not just by Sanger sequencing, but also by pyrosequencing and MSP assay.
This proof of concept for the application of the CRISPRoff tool opens new opportunities for a better understanding of the methylation of the MGMT promoter region.
First, by the application of the epigenetic modification we demonstrated the relationship between methylation and transcript respectively protein levels in our cell lines, where both mRNA and protein levels were depleted (Figures 1A-E). This relationship has been confirmed in cell lines (10), but not patient tissue (11), likely due to technical limitations resulting from the heterogeneous composition of tumor samples. While repeat transfections of T-325 cells did not result in increased average methylation levels, the methylation levels of T.325 M.OFF 3 cells differed in some CpG sites compared to T.325 M.OFF (Figures 1A, B). Of note, the effects on transcript and protein levels did not correlate to the averaged methylation levels across the 98 CpG sites, potentially indicating that certain CpG sites confer a stronger impact on mRNA and protein levels than others. This result is in line with the observation, that certain regions within the MGMT promoter region have a stronger impact on gene expression (12). Identifying the CpG sites that confer a stronger impact on protein levels would be especially interesting and may lead to more accurate prognostication and prediction, as current MGMT promoter region methylation assays only cover short segments of the MGMT promoter region with unclear relative impact compared to other CpG sites (13, 14). While the MGMT promoter region was successfully methylated, off target effects are a possible downside of this approach. However, since these off target effects have previously been analyzed, and found to be infrequent (9), we did not further focus on this topic in this study.
Second, the MGMT methylation-dependent sensitivity to TMZ was demonstrated in vitro (Figures 1F, G). This approach enabled a direct comparison of the impact mediated solely by the methylation of the MGMT promoter region, circumventing added variables that are present in the literature due to intertumoral heterogeneity (12). This observation experimentally validates the MGMT promoter methylation-dependent treatment outcomes observed in patients (15), and was further substantiated in a murine glioblastoma xenograft model based on MGMT overexpression (16).
Third, the previously discussed reduction in variables within the edited and T-325 WT cells enabled the correlation between methylation levels and phenotypic changes in proliferation as well as radiosensitivity. We showed that methylation of the MGMT promoter region resulted in a higher proliferation rate among the three cell lines tested (Figures 2A, B), which has also been described in cholangiocarcinoma. In cholangiocarcinoma, MGMT methylation increases the number of cells entering S-phase by inhibiting p21, p27, and Cyclin E expression, which could be a possible explanation for the observed effects in the glioma cell lines (17).
We furthermore demonstrated that the MGMT promoter region methylation does not correlate with radiosensitivity in vitro (Figure 2C), contrary to results reported in a previous study (18). While others have described a dose-dependent effect of pharmacologic MGMT inhibition on radiosensitivity (19), the prevailing consensus does not support a significant influence of MGMT promoter region methylation on the response to radiotherapy in glioblastoma (20–22).
There are several limitations with regard to the present study, and further validation of our results using other cell lines and patient tissue will be crucial. While tissue heterogeneity and varied methylation profiles within cancer cells represent additional challenges that will need to be addressed when investigating the biological relevance of individual CpG sites, unraveling the intricacies of MGMT promoter region methylation might be achievable through single-cell methylation analysis. While this study was finalized and prepared for submission, a similar article was published that confirms our overall approach and the perspective of MGMT gene modifications as a therapeutic strategy (23).
In conclusion, we have generated insight into the impact of epigenetic modification of the MGMT promoter region for temozolomide sensitivity of glioma cells and may serve as a proof of concept for future studies. In the future, this precision epigenome-editing approach could be used to determine the biological relevance of methylation of individual CpG sites, improving the prognostic and predictive value of MGMT promoter region methylation assessments, as well as serve as a starting point for epigenetic therapeutic interventions.
Methods
Cell culture and reagents
The T-325 cells were generated at the University Hospital Zurich (Zurich, Switzerland), while the LN-18 cells were kindly provided by Dr. N. de Tribolet (Lausanne, Switzerland). The cells were cultured in Dulbecco’s modified Eagle’s medium (#11965084, Gibco Life Technologies, Paisley, UK) with 2 mM L-glutamine (#25030081, Gibco Life Technologies), 100 IU/mL penicillin (#15140122, Gibco Life Technologies), 100 μg/mL streptomycin ((#15140122, Gibco Life Technologies) and 10% fetal calf serum (#16000044, Gibco Life Technologies).
Metabolic activity assay
Culture medium was replaced with 100 µL medium containing 5 mg/mL filtered 3-(4,5-Dimethylthiazol-2-yl)-2,5-diphenyltetrazolium bromide (MTT) (#M5655, Sigma-Aldrich) and incubated for 2 h at 37°C. Subsequently, 100 µL of lysis buffer (10% SDS (#71729, Fluka, Buchs, Switzerland), 10 mM HCl (#320331, Sigma-Aldrich) in 100 mL water) were added. After overnight incubation at 37°C the absorbance was measured at a wavelength of 590 nm using a plate reader (Infinite 200 PRO, Tecan, Mannedorf, Switzerland). Blank values were obtained by measuring wells containing all components except the cells.
Epigenetic editing
Cells were seeded at densities between 5 × 103 to 20 × 103 cells/well in a 96 well plate in their respective medium 24 h before transfection. Transfections were performed using TransIT™-LT1 transfection reagent (#MIR2304, Mirus Bio) using 300 ng of the CRISPR plasmid and 100 ng of each sgRNA plasmid per well of a 96 well plate. In an attempt to further increase average methylation levels within the target loci, cell populations were transfected multiple times and subsequently underwent antibiotic selection. Antibiotic selection was applied two days after transfection (2 μg/mL puromycin (#ant-pr-1, Invivogen) for 3 days).
Plasmids and sgRNAs
CRISPRoff-v2.1 was a gift from Luke Gilbert (Addgene plasmid # 167981;a Addgene, Watertown, MA, USA) (9). The following 6 sgRNAs were used to target MGMT: 1. 5’-tgcgcatcctcgctggacgc-3’, 2. 5’-gacactcaccaagtcgcaaa-3’, 3. 5’-gacccggatggcccttcggc-3’, 4. 5’-acccggtcgggcgggaacac-3’, 5. 5’-gcgaggatgcgcagactgcc-3’, 6. 5’-cggctccgccccgctctaga-3’. The six sgRNA were distributed across the MGMT promotor region, to increase the level of methylation, since approaches using only one sgRNA are known to lead to insufficient methylation levels (9).
The qPCR was run with the following primers: HPRT1 for: 5’-cattatgctgaggatttggaaagg-3’, HPRT1 rev: 5’-cttgagcacacagagggctaca-3’, MGMT for: 5’-cctggctgaatgcctatttccac-3’, MGMT rev: 5’-gcagcttccataacacctgtctg-3’.
Evaluation of epigenetic editing
105 cells were harvested for each sample. DNA was extracted and bisulfite converted using the EpiTect Bisulfite Kit (#59104, Qiagen, Venlo, Netherlands). 50 ng of the bisulfite converted, purified DNA was PCR amplified in a 20 µL reaction either using the MSP assay primers (MGMT MSP Met for: 5’-tttcgacgttcgtaggttttcgc-3’, MGMT MSP Met rev: 5’-gcactcttccgaaaacgaaacg-3’, MGMT MSP UnMet for: 5’-tttgtgttttgatgtttgtaggtttttgt-3’, MGMT MSP UnMet rev: 5’-aactccacactcttccaaaaacaaaaca-3’, annealing temperature: 49°C) or first the amplicon large (MGMT amplicon large for: 5’-ggattttaatatagtttttttggtggata-3’, MGMT amplicon large rev: 5’-gttttaggaagtttttagaagtttttg-3’, annealing temperature: 45°C) followed by the amplicon nested primers (MGMT amplicon nested for: 5’-taattttaagtagggtttggtattttgtgt-3’, MGMT amplicon nested rev: 5’-aagtgttttttaggtgttgtttagtttttt-3’, annealing temperature: 49°C) using the EpiMark® Hot Start Taq DNA Polymerase (#M0490L, New England Biolabs, Ipswich, MA, USA).
Amplicons were prepared for sequencing by ExoSAP-IT™ Express PCR Product Cleanup Reagent (#75001.4X.1.ML, Thermo Fischer Scientific) and sent to MicroSynth for Sanger sequencing using three sequencing primers (MGMT seq 1: 5’-ttaggttttggtagtgtttaggtta-3’, MGMT seq 2: 5’-gttatttggtaaattaaggtatagagtt-3’, MGMT seq 3: 5’-gcgtttttttgttttttttaggtttt-3’). The resulting.ab1 files were deconvoluted using Tracy (24). The obtained numeric data of each base for every position were used to determine the percentage of methylation at each position. Pyrosequencing for MGMT promoter methylation analysis was performed as reported (25).
Proliferation assay
Cells were seeded in their respective medium in a ClearView 96 well plate (#6005182, Perkin Elmer, Waltham, MA, USA) with a quadruplicate for each timepoint. Imaging started one day after seeding. Nuclei were stained with Hoechst 33342 (0.2 μM, incubation for 2 h, #62249, Thermo Fischer Scientific), and five fields of view per well were imaged under a 4x objective in the bright and DAPI channel of a MuviCyte Live-Cell Imaging system (#HH40000000, Perkin Elmer). Cell numbers were determined by ImageJ analysis (V1.53t, National institute of health, Bethesda, MD, USA) of DAPI images.
To determine proliferation under limited dilution conditions, cells were seeded at increasingly low cell number by serial dilution with triplicates for every condition. After 10 days, viability was measured using an MTT assay.
Immunoblot analysis
Freshly harvested cells were supplemented with phosphatase and protease inhibitor cocktail (#04906837001 and #04693132001, Sigma-Aldrich) and lysed using RIPA lysis buffer (#20-188, Merck Millipore, Burlington, VT, USA). Protein concentration in the cell lysate was quantified using the Bradford protein assay (#500-0006, Bio-Rad). 30 μg of each sample was boiled with 4x Laemmli Sample Buffer containing 10% 2-mercaptoethanol (#1610747, BioRad, Hercules, CA, USA) and run on a Mini-PROTEAN® TGX™ Precast Gel (#4561083, BioRad) and transferred to a methanol-activated nitrocellulose membrane (#10600002, Sigma-Aldrich) using a Mini Gel Tank followed by a Blot Module (#A25977 and #B1000, Thermo Fischer Scientific). Subsequently, the membrane was stained using the respective antibody (MGMT: 1:500 dilution, 4°C overnight incubation, #MA5-13506, Invitrogen, Waltham, MA, USA β-Actin: 1:5000 dilution, 20°C, 1 h incubation, #sc-47778, Santa Cruz, Dallas, TX, USA). For multiple stainings on a single membrane, Western blot Strip-It buffer (#R-03722-D50, Advansta, San Jose, CA, USA) was used. To visualize the stained proteins, SuperSignal West Femto Maximum Sensitivity Substrate (#34095, Thermo Fischer Scientific) was applied and detected using the CURIX 60 processing System (AGFA, Mortsel, Belgium).
Methylation-specific polymerase chain reaction assay
The PCR amplification reactions for the MSP assay (described above) were loaded onto a 3% agarose gel and ran at 80 V for 2 h. The presence of DNA bands was evaluated qualitatively using a UV lamp.
Quantitative polymerase chain reaction
Total RNA was extracted from 10 (6) cells using the RNeasy Mini Kit (#74104, Qiagen) and reverse transcription was performed using the High-Capacity cDNA Reverse Transcription Kit (#4368814, Applied Biosystems, Foster City, CA, USA). The qPCR was conducted using the respective primers including normalization to HPRT1 and SYBR™ Green PCR Master Mix (#4309155, Applied Biosystems). Amplification was carried out in a QuantStudio™ 6 Flex Real-Time PCR System (#4485691, Applied Biosystems) under the following conditions: UDG activation: 50°C, 2 min; Taq polymerase activation: 95°C, 2 min; Denature 95°C, 15 sec; Anneal/Extend: 60°C, 1 min, 40 cycles.
Radiosensitivity assay
To perform radiosensitivity assays, 5 x 103 cells were placed in each well of a 96-well plate. Cells were given 24 h to attach before being irradiated (RS 2000, Radsource, Brentwood, CA, USA) with either 0, 2.5 or 5 Gy twice in 5-day intervals. After another 5 days the viability was determined by MTT assay.
Cytotoxicity assays
A total of 5 × 103 cells were placed in each well of a 96-well plate. After 24 h, they were subjected to TMZ treatment as specified for a duration of 72 h in a serum-free medium. The viability of the cells, as measured by metabolic activity, was assessed after incubation for another 3 days in serum containing medium by MTT assay.
Statistical analysis
All data are represented as mean ± standard deviation (SD) of 3 replicates unless indicated differently. Statistical analyses were performed by multiple t-tests with the two-stage step-up method of Benjamini, Krieger and Yekutieli (26). Significance thresholds were defined as *p<0.05; **p<0.01; ***p<0.001. Data was analyzed and visualized using Prism (GraphPad, San Diego, CA, USA).
Data availability statement
The original contributions presented in the study are included in the article/Supplementary Materials, further inquiries can be directed to the corresponding author/s.
Ethics statement
Ethical approval was not required for the studies on humans in accordance with the local legislation and institutional requirements because only commercially available established cell lines were used.
Author contributions
RW: Conceptualization, Data curation, Formal Analysis, Investigation, Methodology, Visualization, Writing – original draft, Writing – review & editing. MW: Conceptualization, Funding acquisition, Resources, Supervision, Writing – review & editing. GR: Methodology, Resources, Writing – review & editing. FV: Conceptualization, Formal Analysis, Supervision, Writing – original draft, Writing – review & editing.
Funding
The author(s) declare that no financial support was received for the research, authorship, and/or publication of this article.
Conflict of interest
MW has received research grants from Quercis and Versameb, and honoraria for lectures or advisory board participation or consulting from Bayer, Curevac, Medac, Novartis, Novocure, Orbus, Philogen, Roche and Sandoz.
The remaining authors declare that the research was conducted in the absence of any commercial or financial relationships that could be construed as a potential conflict of interest.
Publisher’s note
All claims expressed in this article are solely those of the authors and do not necessarily represent those of their affiliated organizations, or those of the publisher, the editors and the reviewers. Any product that may be evaluated in this article, or claim that may be made by its manufacturer, is not guaranteed or endorsed by the publisher.
Supplementary material
The Supplementary Material for this article can be found online at: https://www.frontiersin.org/articles/10.3389/fonc.2024.1342114/full#supplementary-material
References
1. Esteller M, Garcia-Foncillas J, Andion E, Goodman SN, Hidalgo OF, Vanaclocha V, et al. Inactivation of the DNA-repair gene MGMT and the clinical response of gliomas to alkylating agents. N Engl J Med (2000) 343:1350–4. doi: 10.1056/NEJM200011093431901
2. Toyota M, Ahuja N, Ohe-Toyota M, Herman JG, Baylin SB, Issa JPJ. CpG island methylator phenotype in colorectal cancer. Proc Natl Acad Sci U.S.A. (1999) 96:8681–6. doi: 10.1073/PNAS.96.15.8681
3. Gu C, Lu J, Cui T, Lu C, Shi H, Xu W, et al. Association between MGMT promoter methylation and non-small cell lung cancer: A meta-analysis. PloS One (2013) 8. doi: 10.1371/journal.pone.0072633
4. Majumdar S, Buckles E, Estrada J, Koochekpour S. Aberrant DNA methylation and prostate cancer. Curr Genomics (2011) 12:486. doi: 10.2174/138920211797904061
5. Hegi ME, Diserens A-C, Gorlia T, Hamou M-F, de Tribolet N, Weller M, et al. MGMT gene silencing and benefit from temozolomide in glioblastoma. N Engl J Med (2005) 352:997–1003. doi: 10.1056/NEJMoa043331
6. Kato T, Natsume A, Toda H, Iwamizu H, Sugita T, Hachisu R, et al. Efficient delivery of liposome-mediated MGMT-siRNA reinforces the cytotoxity of temozolomide in GBM-initiating cells. Gene Ther (2010) 17:1363–71. doi: 10.1038/gt.2010.88
7. Wu F, Cheng G, Yao Y, Kogiso M, Jiang H, Li X-N, et al. Inhibition of mutated isocitrate dehydrogenase 1 in cancer. Med Chem (Los Angeles) (2018) 14:715–24. doi: 10.2174/1573406414666180524093659
8. Glassner BJ, Weeda G, Allan JM, Broekhof JLM, Carls NHE, Donker I, et al. DNA repair methyltransferase (Mgmt) knockout mice are sensitive to the lethal effects of chemotherapeutic alkylating agents. Mutagenesis (1999) 14:339–47. doi: 10.1093/mutage/14.3.339
9. Nuñez JK, Chen J, Pommier GC, Cogan JZ, Replogle JM, Adriaens C, et al. Genome-wide programmable transcriptional memory by CRISPR-based epigenome editing. Cell (2021) 184:2503–2519.e17. doi: 10.1016/J.CELL.2021.03.025
10. Zappe K, Pühringer K, Pflug S, Berger D, Böhm A, Spiegl-Kreinecker S, et al. Association between MGMT enhancer methylation and MGMT promoter methylation, MGMT protein expression, and overall survival in glioblastoma. Cells (2023) 12:1639. doi: 10.3390/cells12121639
11. Uno M, Oba-Shinjo SM, Camargo AA, Moura RP, de Aguiar PH, Cabrera HN, et al. Correlation of MGMT promoter methylation status with gene and protein expression levels in glioblastoma. Clinics (2011) 66:1747. doi: 10.1590/S1807-59322011001000013
12. Everhard S, Tost J, El Abdalaoui H, Crinière E, Busato F, Marie Y, et al. Identification of regions correlating MGMT promoter methylation and gene expression in glioblastomas. Neuro Oncol (2009) 11:348. doi: 10.1215/15228517-2009-001
13. Wick W, Weller M, Van Den Bent M, Sanson M, Weiler M, Von Deimling A, et al. MGMT testing–the challenges for biomarker-based glioma treatment. Nat Rev Neurol (2014) 10:372–85. doi: 10.1038/nrneurol.2014.100
14. Weller M, Stupp R, Reifenberger G, Brandes AA, Van Den Bent MJ, Wick W, et al. MGMT promoter methylation in Malignant gliomas: ready for personalized medicine? Nat Rev Neurol (2010) 6:39–51. doi: 10.1038/nrneurol.2009.197
15. Siller S, Lauseker M, Karschnia P, Niyazi M, Eigenbrod S, Giese A, et al. The number of methylated CpG sites within the MGMT promoter region linearly correlates with outcome in glioblastoma receiving alkylating agents. Acta Neuropathol Commun (2021) 9:35. doi: 10.1186/s40478-021-01134-5
16. Kitange GJ, Carlson BL, Schroeder MA, Grogan PT, Lamont JD, Decker PA, et al. Induction of MGMT expression is associated with temozolomide resistance in glioblastoma xenografts. Neuro Oncol (2009) 11:281. doi: 10.1215/15228517-2008-090
17. Chen J, Li Z, Chen J, Du Y, Song W, Xuan Z, et al. Downregulation of MGMT promotes proliferation of intrahepatic cholangiocarcinoma by regulating p21. Clin Transl Oncol (2020) 22:392–400. doi: 10.1007/s12094-019-02140-9
18. Rivera AL, Pelloski CE, Gilbert MR, Colman H, De La Cruz C, Sulman EP, et al. MGMT promoter methylation is predictive of response to radiotherapy and prognostic in the absence of adjuvant alkylating chemotherapy for glioblastoma. Neuro Oncol (2010) 12:116. doi: 10.1093/neuonc/nop020
19. Kirstein A, Schilling D, Combs SE, Schmid TE. Lomeguatrib increases the radiosensitivity of MGMT unmethylated human glioblastoma multiforme cell lines. Int J Mol Sci (2021) 22:13, 6781. doi: 10.3390/ijms22136781
20. Wick W, Platten M, Meisner C, Felsberg J, Tabatabai G, Simon M, et al. Temozolomide chemotherapy alone versus radiotherapy alone for Malignant astrocytoma in the elderly: the NOA-08 randomised, phase 3 trial. Lancet Oncol (2012) 13:707–15. doi: 10.1016/S1470-2045(12)70164-X
21. Wen PY, Weller M, Lee EQ, Alexander BM, Barnholtz-Sloan JS, Barthel FP, et al. Glioblastoma in adults: a Society for Neuro-Oncology (SNO) and European Society of Neuro-Oncology (EANO) consensus review on current management and future directions. Neuro Oncol (2020) 22:1073. doi: 10.1093/neuonc/noaa106
22. Reifenberger G, Hentschel B, Felsberg J, Schackert G, Simon M, Schnell O, et al. Predictive impact of MGMT promoter methylation in glioblastoma of the elderly. Int J Cancer (2012) 131:1342–50. doi: 10.1002/ijc.27385
23. Han X, Abdallah MOE, Breuer P, Stahl F, Bakhit Y, Potthoff AL, et al. Downregulation of MGMT expression by targeted editing of DNA methylation enhances temozolomide sensitivity in glioblastoma. Neoplasia (2023) 44:100929. doi: 10.1016/j.neo.2023.100929
24. Rausch T, Fritz MHY, Untergasser A, Benes V. Tracy: Basecalling, alignment, assembly and deconvolution of sanger chromatogram trace files. BMC Genomics (2020) 21:1–9. doi: 10.1186/s12864-020-6635-8
25. Felsberg J, Thon N, Eigenbrod S, Hentschel B, Sabel MC, Westphal M, et al. Promoter methylation and expression of MGMT and the DNA mismatch repair genes MLH1, MSH2, MSH6 and PMS2 in paired primary and recurrent glioblastomas. Int J Cancer (2011) 129:659–70. doi: 10.1002/ijc.26083
Keywords: glioblastoma, epigenetic editing, CRISPRoff, MGMT promoter, temozolomide
Citation: Weber R, Weller M, Reifenberger G and Vasella F (2024) Epigenetic modification and characterization of the MGMT promoter region using CRISPRoff in glioblastoma cells. Front. Oncol. 14:1342114. doi: 10.3389/fonc.2024.1342114
Received: 21 November 2023; Accepted: 04 January 2024;
Published: 31 January 2024.
Edited by:
Pankaj Pathak, National Institute of Neurological Disorders and Stroke (NIH), United StatesReviewed by:
Laveniya Satgunaseelan, Royal Prince Alfred Hospital, AustraliaFahim Ahmad, National Cancer Institute at Frederick (NIH), United States
Subhashree Nayak, National Institute of Arthritis and Musculoskeletal and Skin Diseases (NIH), United States
Copyright © 2024 Weber, Weller, Reifenberger and Vasella. This is an open-access article distributed under the terms of the Creative Commons Attribution License (CC BY). The use, distribution or reproduction in other forums is permitted, provided the original author(s) and the copyright owner(s) are credited and that the original publication in this journal is cited, in accordance with accepted academic practice. No use, distribution or reproduction is permitted which does not comply with these terms.
*Correspondence: Flavio Vasella, flavio.vasella@usz.ch
†ORCID: Remi Weber, orcid.org/0000-0001-7639-3347
Michael Weller, orcid.org/0000-0002-1748-174X
Guido Reifenberger, orcid.org/0000-0002-1419-9837
Flavio Vasella, orcid.org/0000-0002-8953-0862