- 1ImmuNext Inc., Lebanon, NH, United States
- 2Department of Microbiology and Immunology, Geisel School of Medicine at Dartmouth, Hanover, NH, United States
- 3Department of Microbiology and Immunology, Dartmouth Cancer Center, Geisel School of Medicine at Dartmouth, Hanover, NH, United States
- 4Section of Clinical Pharmacology, Department of Medicine, Geisel School of Medicine at Dartmouth and Dartmouth Cancer Center, Hanover, NH, United States
- 5Curis, Inc., Lexington, MA, United States
- 6Division of Hematology/Oncology, Tufts Medical Center, Boston, MA, United States
- 7Kineta Inc., Seattle, WA, United States
- 8Yale University School of Medicine, New Haven, CT, United States
- 9Department of Medical, Division of Medical Oncology, Beth Israel Deaconess Medical Center, Harvard Medical School, Boston, MA, United States
- 10Department of Medical Oncology, Dana-Farber Cancer Institute, Harvard Medical School, Boston, MA, United States
- 11Department of Dermatology, Yale School of Medicine, New Haven, CT, United States
- 12Hummingbird Bioscience, Houston, TX, United States
- 13Department of Radiation Oncology, Stanford School of Medicine, Stanford, CA, United States
- 14Olivia Newton-John Cancer Research Institute and School of Cancer Medicine, La Trobe University, Melbourne, VIC, Australia
- 15Department of Molecular Imaging and Therapy, Austin Health and Faculty of Medicine, University of Melbourne, Melbourne, VIC, Australia
Immune checkpoints limit the activation of the immune system and serve an important homeostatic function but can also restrict immune responses against tumors. Inhibition of specific immune checkpoint proteins such as the B7:CD28 family members programmed cell death protein-1 (PD-1) and cytotoxic T-lymphocyte antigen-4 (CTLA-4) has transformed the treatment of various cancers by promoting the anti-tumor activation of immune cells. In contrast to these effects, the V-domain immunoglobulin suppressor of T-cell activation (VISTA) regulates the steady state of the resting immune system and promotes homeostasis by mechanisms distinct from PD-1 and CTLA-4. The effects of VISTA blockade have been shown to include a decrease in myeloid suppression coupled with proinflammatory changes by mechanisms that are separate and distinct from other immune checkpoint proteins; in some preclinical studies these immune effects appear synergistic. Given the potential benefits of VISTA blockade in the context of cancer therapy, the second Annual VISTA Symposium was convened virtually on September 23, 2022, to review new research from investigators and immuno-oncology experts. Discussions in the meeting extended the knowledge of VISTA biology and the effects of VISTA inhibition, particularly on cells of the myeloid lineage and resting T cells, as three candidate anti-VISTA antibodies are in, or nearing, clinical development.
Introduction to the VISTA immune checkpoint
The landscape of cancer management has been transformed enormously by advances in our understanding of immune system checkpoints and the blockade of their downstream signaling pathways. Treatment of appropriate malignancies with immune checkpoint inhibitors (ICIs) targeting cytotoxic T-lymphocyte antigen-4 (CTLA-4) and programmed cell death protein-1 (PD-1, along with its ligand, PD-L1) has markedly improved outcomes for patients with tumors by promoting activation of the adaptive immune system. In contrast, a related immune checkpoint protein, V-domain immunoglobulin (Ig) suppressor of T-cell activation (VISTA), serves in a homeostatic role to regulate the steady state of both the lymphoid and myeloid lineages of the resting immune system (1). VISTA is also known as PD-1H, c10orf54, VSIR, SISP1, B7-H5, DD1a, Gi24, and Dies1 (2, 3).
VISTA demonstrates similarity to the B7 family of ligands – sharing 25% protein sequence identity with PD-L1 – and receptors such as the CD28 family, but has unique structural features, expression patterns, and functions (4, 5). The expression of VISTA on tumor or immune cells may significantly affect tumor growth and antitumor immunity, with VISTA acting as a receptor, a ligand, or both (1, 6). Early studies by Chen and colleagues identified VISTA as a negative regulatory receptor on T cells (2, 7, 8). Ligands that have been identified as potential binding partners for VISTA include PSGL-1 (at low pH), VSIG-3, Galectin-9, LRIG1, and Syndecan-2 (9–13). Additionally, VISTA has been shown to form homophilic interactions at intercellular junctions of apoptotic cells and macrophages (14). The physiological relevance of these potential interactions remains uncertain at present and requires further investigation (1).
VISTA is expressed on most hematopoietic cells and is highly expressed on many cells in the myeloid lineage (1, 4). Resting T cells also express VISTA, which is transiently downregulated upon activation and then re-expressed on memory T cells (1, 4). VISTA serves as a checkpoint for resting T cells that negatively regulates the earliest stages of T-cell activation and the transition from quiescence to priming, suggesting that the VISTA checkpoint regulates the activation of resting T cells prior to the involvement of the PD-(L)1 and CTLA-4 checkpoints (1, 15).
Given its role in the regulation of the immune system, the expression and functions of VISTA in cancers are currently under active investigation. In preclinical models, high levels of VISTA expression are found on tumor-infiltrating leukocytes and myeloid-derived suppressor cells (MDSCs) (1, 6, 15–17). VISTA expression levels have also been examined on malignant cells. While several tumor types have been reported to express elevated levels of VISTA, careful assessment is still needed to interpret the significance of this finding, given that infiltrating VISTA-expressing immune cells may also be present (1, 6, 15–17). In several tumor types, such as melanoma and ovarian cancer, VISTA expression appears to correlate with disease progression and is indicative of poor survival in some settings (4). Encouragingly, VISTA-targeting agents have demonstrated promising findings in multiple preclinical tumor models (1, 4).
As an emerging immune checkpoint, VISTA represents a compelling new molecular treatment target under active investigation. Three distinct anti-VISTA antibodies are currently approaching or moving forward into clinical development. For the second consecutive year, a VISTA symposium was held virtually on September 23, 2022, to support efforts by investigators and immuno-oncology experts, accelerate our understanding of VISTA, and promote the development of potential therapeutics targeting this protein. Among the key findings of the second Annual VISTA Symposium was a growing awareness that VISTA inhibition exerts multiple effects on the immune system that are distinct from other ICIs (Figure 1). In particular, several presentations outlined the effects of VISTA blockade, including a decrease in myeloid suppression of immune responses coupled with proinflammatory changes in the tumor microenvironment (TME) by mechanisms separate and distinct from PD-1 and CTLA-4 inhibition. New preclinical and clinical data were described for three candidate anti-VISTA antibodies currently in development (CI-8993 [Curis, Inc.], HMBD-002 [Hummingbird Bioscience], and KVA12123 [Kineta, Inc.]).
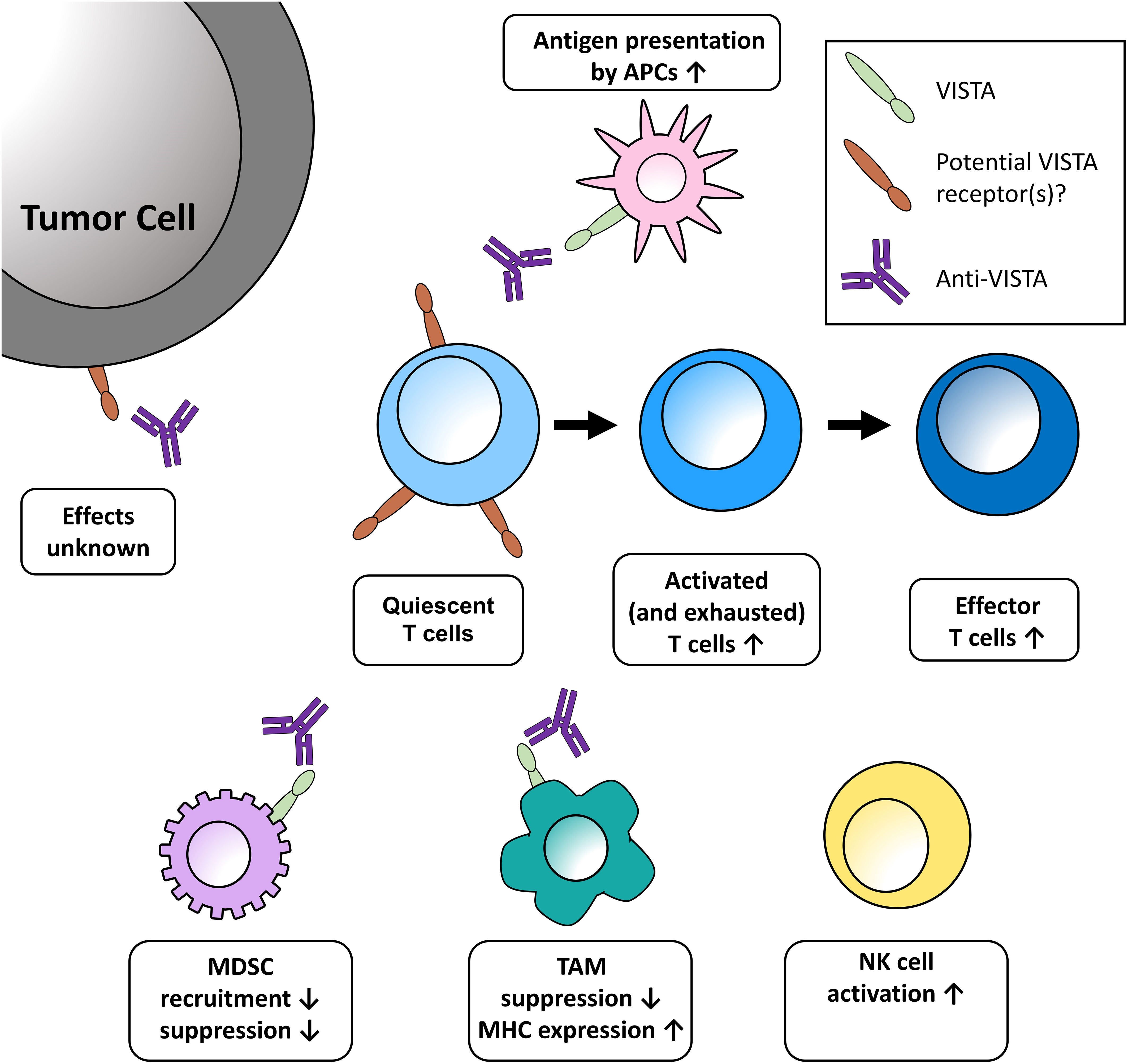
Figure 1 Potential effects of VISTA blockade in the tumor microenvironment discussed at the meeting. One role of VISTA is to serve as a checkpoint on naïve T cells, impeding the earliest stages of T-cell activation. Blockade of VISTA brings T cells out of quiescence, then acts to expand and transform activated and exhausted T cells into effector cells. Further effects of VISTA blockade in the TME include enhanced presentation of antigens on monocytes, decreased recruitment of MDSCs, increased release of T-cell activating factors, and increased maturation of NK cells. These effects are further bolstered by increased MHC expression on TAMs and the reduced expression of genes promoting T-cell quiescence. The functional relevance of VISTA expression on tumor cells has yet to be uncovered. APC, antigen-presenting cell; MDSC, myeloid-derived suppressor cells; MHC, major histocompatibility complex; NK, natural killer; TAM, tumor-associated macrophage.
VISTA and the suppression of immune responses by myeloid cells
It is now well established that myeloid cell reprogramming by tumors is an important way in which they evade the immune response (18, 19). Research suggests that the VISTA checkpoint plays an important role in the regulation of MDSCs, which inhibit the responses of the immune system in some malignancies. MDSCs exhibit potent immunosuppressive activity and higher numbers of circulating MDSCs are closely linked to poor clinical outcomes in cancer (18). In this way, VISTA shares some functional similarity to leukocyte Ig-like receptor subfamily B ICIs such as LAIR1, which have been demonstrated to support the immunosuppressive activity of myeloid cells (20). A defining characteristic of MDSCs is their interference with the immune responses mediated by T cells, B cells, and natural killer (NK) cells (18). Patients with acute myeloid leukemia have increased expression of VISTA on MDSCs, and VISTA is a critical component of the MDSC-mediated inhibition of CD8+ T-cell responses (21). In murine cancer models, VISTA knockout resulted in reduced migration of macrophages and MDSCs into the TME. In contrast, treatment with an anti-VISTA monoclonal antibody (mAb) resulted in a reduction of tumor-infiltrating MDSCs accompanied by an increase of tumor-infiltrating CD4+ and CD8+ T cells (16, 22). An emerging model hypothesizes that VISTA promotes both migration of inhibitory myeloid cells (such as MDSCs) to the TME and their suppressive effects on the immune system within the TME (1, 4). This is supported by another study using the CT26 colon cancer model that shows the role of VISTA in MDSC-mediated T-cell suppression under hypoxic conditions and indicates that targeting VISTA may counteract the dampening effect of hypoxia on antitumor immunity (23).
Several presentations at the symposium described aspects of VISTA regulation within the myeloid compartment, specifically regarding the activity of MDSCs. Research from Dr. Sam W. Lee’s group at Yale School of Medicine revealed that the in vitro inhibition of CD4+ T cells by MDSCs was reversed by treatment with antibodies targeting VISTA and in VISTA–/– syngeneic murine tumor models. A second preclinical murine study presented by Dr. Louise Lines of Dartmouth demonstrated that treatment with an anti-VISTA antibody exerted multiple effects on tumor-associated macrophages (TAMs), including diminished myeloid suppression of T-cell proliferation, increased major histocompatibility complex expression on TAMs, and reduced expression of genes promoting T-cell quiescence. Taken in combination, these findings extend the knowledge of the roles of VISTA in myeloid cells and further support the rationale for targeting VISTA.
Clinical prospects for VISTA inhibition
High levels of VISTA expression have been reported in patient samples from multiple forms of cancer (Table 1) (21, 24–39). However, the conclusions drawn from these patterns of expression are still emerging. For example, in patients with melanoma, any level of VISTA expression in the tumor-infiltrating inflammatory cells of their primary melanoma decreased survival, whereas in renal cell carcinoma (RCC), VISTA-positive immune cells homing to the venous tumor thrombus, but not to the primary RCC itself, indicated poor prognosis (29, 40). Conversely, high levels of VISTA expression have also been linked with improved survival outcomes in some settings, suggesting that further research is required to assess VISTA expression and function within the TME (41).
New research was presented at the symposium exploring the opportunities for VISTA blockade in potential target pathologies. New data from the laboratory of Dr. Kathleen Mahoney at Dana-Farber Cancer Institute and Beth Israel Deaconess Medical Center revealed a high expression of VISTA in samples of kidney cancer at levels that exceed those of PD-1 and PD-L1. Histology studies of clear cell kidney cancer found VISTA expression on tumor cells in a subset of cases, but the highest VISTA expression was observed on infiltrating immune cells. In the case of head and neck cancer (HNC), Dr. Dhanya Nambiar of Stanford University School of Medicine shared findings demonstrating that radiation therapy contributed to the migration of circulating MDSCs with an enhanced expression of VISTA, and VISTA blockade significantly improved radiation treatment response in mouse models of HNC.
Cutaneous malignancies, including melanoma and cutaneous squamous cell carcinoma (cSCC), were discussed by Dr. Matthew Vesely of Yale University School of Medicine, who described new immunological findings demonstrating frequent detection of VISTA expression in melanoma (~50% of samples), particularly in CD11b myeloid cells, and this specific form of expression was found to be associated with recurrence and poor survival. Consistent with findings from HNC, the levels of VISTA expression were shown to exceed that of PD-L1, and there was a poor correlation between VISTA and PD-L1 expression in the melanoma samples studied. In cSCC, VISTA and PD-L1 were expressed in a subset of samples, again with poor correlation between the two checkpoints and the highest VISTA expression seen in CD11b cells.
VISTA inhibition promotes a proinflammatory TME by mechanisms distinct from PD-1 and CTLA-4 inhibition
It is believed that the suppressive effects of MDSCs and TAMs can, in some cases, limit the therapeutic impact of T-cell–directed checkpoint therapies such as those targeting PD-1 or CTLA-4. The constitutive expression of VISTA observed in multiple immune cell lineages (particularly myeloid lineages and resting T cells) suggests that this regulatory protein may act as a “rheostat” to actively normalize immune responses by dampening excessive activation at the earliest stages of the immune response (4). This model of VISTA’s function is important compared to other negative checkpoint regulators since CTLA-4 and PD-1 are induced after activation, indicating that the VISTA checkpoint may be a hurdle to overcome to initiate an immune response (4, 17).
Ongoing research in preclinical models has established the role of the VISTA checkpoint in limiting immune responses. In the CT26 model of colorectal cancer, single-agent anti-VISTA treatment of small tumors resulted in slowed tumor growth (42). Similarly, while large CT26 tumors showed complete adaptive resistance to treatment with anti-PD-1/CTLA-4 antibodies, the addition of an anti-VISTA antibody led to the resolution of half the previously resistant tumors (42). Sequencing of tumor-specific CD8+ T cells revealed that anti-VISTA therapy activated T-cell pathways highly distinct from, and complementary to, those activated by anti-PD-1 therapy, with VISTA-targeting therapy reducing the regulators of T-cell quiescence (42). VISTA blockade in tumor models has been reviewed more comprehensively than this paper allows, for example by Huang et al. and Yum et al. (43, 44). Importantly, VISTA blockade has also demonstrated a combinatorial effect combined with CTLA-4 blockade to induce tumor regression in a murine model of SCC (45). These data indicate that anti-VISTA treatment has the potential to overcome adaptive resistance that arises in treatment strategies inhibiting PD-1 and/or CTLA-4.
From a therapeutic perspective, there are potential risks associated with immune system stimulation via anti-VISTA therapy (3). These risks could potentially be amplified when combined with other ICIs, such as those targeting PD-1 and CTLA-4, which have already been associated with immune-related adverse events (3, 46). Blocking VISTA may result in decreased peripheral tolerance through T-cell quiescence and thus development of auto-inflammatory conditions (3). Another concern is cytokine release syndrome (CRS), discussed below.
Presentations during this symposium reinforced the premise that inhibiting or removing VISTA increases activation of the immune system in a manner distinct from the most common ICIs currently employed in clinical practice. Dr. Randolph J. Noelle of Dartmouth proposed a conceptual framework that VISTA and CD28 function as mirror images of one another, with CD28 acting to amplify the development of immune responses while VISTA serves to dampen activation and promote quiescence. This model was supported by new research presented from the Noelle laboratory demonstrating that a loss of VISTA activity enhances the development of autoimmunity in mouse models.
Dr. Lines presented findings from a murine model of colon cancer, revealing that anti-VISTA treatment increased immune cell density in the TME. Findings from her laboratory showed increased post-treatment infiltration of NK cells in addition to CD45+, CD8+, and CD4+ T cells relative to the non-responders. Synergy was observed with the addition of anti-PD-1 and anti-CTLA-4 treatments relative to monotherapy, with further reductions in tumor growth and a reduction of the suppressive character of myeloid cells in the TME. The combination of VISTA and PD-1/CTLA-4 blockade was also shown to exert complementary effects on T cells, leading to an increased population of mature cells required for tumor cell killing and supporting the hypothesis that anti-VISTA treatment may ameliorate the adaptive resistance that can develop with contemporary ICI therapies (42).
An area of active investigation is the role of VISTA in T cells. The Noelle group previously demonstrated that the VISTA checkpoint limits the activation of naïve T cells and maintains peripheral tolerance (15). New findings from the Noelle group described an intriguing role for VISTA on both CD4+ and CD8+ memory T cells. Studies of VISTA knockout mice revealed that memory T cells were increasingly proinflammatory in mice that lacked VISTA, with reduced expression of co-inhibitory molecules. These findings raised multiple questions for future study, including the influences of VISTA on the exhaustion and future phenotype of memory T cells and VISTA’s influence on the survival and effector functions of regulatory T cells.
Antibodies targeting VISTA are currently in development
The promise of targeting the VISTA checkpoint in multiple cancers has motivated the development of mAbs targeting VISTA to block its function, and updates regarding three unique anti-VISTA mAbs that are currently in or about to enter human clinical trials were presented at the symposium.
The first anti-VISTA antibody to be assessed in humans is CI-8993 (Curis, Inc.). CI-8993 has putative binding sites at 4 residues within the C-C’ loop of VISTA, has been demonstrated to block interaction with both with PSGL-1 and VSIG-3 binding, and is constructed with an active IgG1 Fc domain that facilitates interactions with Fc gamma receptors and myeloid cells and supports antibody-dependent cell cytotoxicity (ADCC) (13, 47–49). Prior studies showed signs of transient grade 3 CRS (13). Current studies were designed to anticipate this possibility, as CRS was considered an on-target effect reflecting target engagement and the activation of an immune response (49). Furthermore, the oncology community has become more familiar with CRS management, and guidelines for management have been published (50). An ongoing phase 1 dose-escalation study in patients with advanced, refractory solid tumors is assessing CI-8993 (NCT04475523). Based on data published to date, the safety profile appears to be manageable, with no dose-limiting side effects observed up to the 0.6 mg/kg dose level (51). Pharmacokinetic analyses indicate that CI-8993 is demonstrating saturation binding kinetics to VISTA (51). Examination of the pharmacodynamic effects of CI-8993 has revealed increased maturation of NK cells, decreased recruitment of MDSCs, increased release of T-cell activating factors, and enhanced presentation of antigens on monocytes, suggesting that treatment may activate multiple anti-cancer mechanisms (51).
The complexity of targeting a relatively abundant protein such as VISTA with therapeutics that have a very strong binding affinity (Kd in the 350–500 picomolar range) leads to the phenomenon of target-mediated drug disposition (TMDD) (52). In TMDD, an abundant protein (such as VISTA) serves as a sink for the therapeutic agent, which then impacts the pharmacokinetics and therapeutic localization of the agent. Dr. Lionel D. Lewis of the Geisel School of Medicine at Dartmouth & The Dartmouth Cancer Center reviewed the properties of therapeutic agents (including mAbs) that undergo TMDD. Dr. Lewis emphasized the nonlinear and time-dependent change in the pharmacokinetics of such therapeutic agents and how there is a failure to saturate binding to VISTA, especially at lower doses, which in the setting of a high-affinity binding therapeutic can make the determination of optimal schedule and dosing very challenging. This phenomenon has been modeled across multiple therapeutics, reviewed by Dua et al. and Dostalek et al. (53, 54). Preclinical studies demonstrated bioavailability of a subcutaneously injected mAb that was inversely related to the dose level, likely due to saturable binding (55). Dr. Andrew M. Scott of the Olivia Newton-John Cancer Research Institute presented new data in a trio of tumorigenic mouse models using radiolabeled CI-8993 in conjunction with positron emission tomography imaging to demonstrate specific localization of CI-8993 to tumor sites, with evidence of VISTA receptor saturation at high doses. However, the amount of peripheral VISTA saturation needed to achieve the optimal antitumor effect dose in humans is pending further study.
A second anti-VISTA antibody, HMBD-002 (Hummingbird Bioscience), is also being investigated in clinical trials. HMBD-002 was rationally designed to bind to VISTA by targeting key residues within the C-C’ loop predicted to be involved in mediating interactions with VISTA binding partners (13, 47). In this case, the antibody is designed with an IgG4 Fc, which does not activate ADCC, allowing the preservation of important VISTA+ immune cells while stimulating the desired proinflammatory cytokine response from VISTA blockade (13). HMBD-002 has demonstrated significant inhibition of tumor growth in multiple solid tumor models, accompanied by increased numbers of antigen-presenting cells and reduced numbers of MDSCs in the TME (13). Additional preclinical validation was demonstrated by enhanced activation of CD8+ T cells and proinflammatory reprogramming of TAMs (56). Responses to HMBD-002 have also been evaluated in combination with pembrolizumab in a colon cancer model, demonstrating a synergistic antitumor effect (56). HMBD-002 is currently being assessed in an ongoing phase 1/2 dose-escalation trial (NCT05082610), which will include a combination arm with pembrolizumab. Thus far, HMBD-002 has shown a promising profile and has been safely tolerated at doses of up to 180 mg weekly (57).
The anti-VISTA antibody KVA12123 (Kineta, Inc.) is a fully human-engineered IgG1 mAb designed to bind to VISTA through a unique epitope in the extracellular domain, blocking interactions with putative VISTA binding partners, and blocking VISTA function (58). It has been engineered to provide strong single-agent tumor growth inhibition with no demonstrated CRS-associated cytokine release observed in preclinical models (59). Additionally, the effects of KVA12123 have been shown to reverse immunosuppression in the TME by increasing infiltrating T effector cells (CD4+ and CD8+), increasing the ratio of M1 macrophages, and enhancing the activation of NK cells (60). Tumor growth inhibition has been observed with KVA12123 treatment in multiple preclinical models, including colon, bladder, and T-cell lymphoma, as a monotherapy and in combination with PD-1 inhibitors (60). Studies in non-human primates have shown KVA12123 to be well tolerated at doses up to 100 mg/kg (60). A phase 1/2 open-label trial of KVA12123 alone and in combination with pembrolizumab in patients with advanced solid tumors (NCT05708950) has been initiated with planned increasing dose cohorts of KVA12123 from 3 mg up to 1000 mg.
Determination of the crystal structure of the extracellular domain of VISTA has provided insights into binding of both putative VISTA ligands and VISTA antibodies (47). This includes an extended C-C’ loop, which contributes to epitopes for multiple VISTA antibodies, including the previously described CI-8993 and HMBD-002 (13, 47). CI-8993 and two preclinical anti-VISTA antibodies, BMS767 and SG7, have been reported to have overlapping epitopes but different crucial amino acids for VISTA binding (13, 48). The three antibodies have been demonstrated to block both each other and interactions with human PSGL-1 and VSIG-3, with BMS767 doing so in a pH-dependent manner, reviewed elsewhere (48).
Conclusions and future directions
Research shows that the VISTA checkpoint is differentiated from similar molecules with important roles in the regulation of myeloid cells and the promotion of immune system quiescence. Presentations at the VISTA symposium further demonstrated that anti-VISTA treatment might show synergy with anti-PD-1 and anti-CTLA-4 agents due to a reduction in the suppressive character of myeloid cells in the TME and complementary pro-activation effects on T cells. These findings suggest that anti-VISTA treatment may help to overcome the emergence of adaptive resistance to common ICI therapies, and combinations of these approaches will be explored in clinical trials. Antibodies targeting VISTA entering the clinic are beginning to yield promising outcomes; however, more assessment and development are needed to understand the clinical consequences of different antibody Fc isotypes and to manage treatment-related adverse effects like CRS. Beyond its role in the regulation of tumor immunity, VISTA has been shown to play a central role in the development of immunity and autoimmune disease through its negative regulatory role in controlling the innate and adaptive arms of the immune response (5, 61–64).
Despite the progress in understanding VISTA, much remains to be learned. Future research must examine the physiological relevance of the proposed ligands for VISTA and under what conditions these interactions are most likely to occur. The functions of VISTA within the immune system must be further explored, both within the TME and in its roles in innate and adaptive immunity. The significance and prognostic relevance of VISTA expression in different tumor samples require further analysis to guide the selection of patients most likely to benefit. While VISTA expression measured by immunohistochemistry could be developed as a biomarker to guide patient selection for VISTA-targeted therapies, currently no standardized scoring method exists (3). Similar to that established for PD-L1, there is likely a need to assess the proportion of VISTA expression on tumor cells versus immune cells in the TME to properly guide patient selection.
Beyond the TME, there is an increasing evidence base indicating that gut microbiota-immune system interactions affect cancer progression and response to therapies (65). The efficacy of anti-PD-1-based immunotherapy has been associated with particular commensal microbial composition (66). Compositional deviations of gut microbiota have been correlated with both cancer prognosis and likelihood of response to specific ICIs (67). The composition of a patient’s gut microbiota may play a role in clinical response to anti-VISTA therapy and potentially could be a consideration in patient selection.
Further preclinical research, clinical trials, and discussions will be necessary to address the issues of specific tumor types most responsive to VISTA blockade, the effects of prior therapies, and potential biomarkers most predictive of clinical response, all of which are likely to substantially impact the beneficial effects of VISTA blockade in cancer patients.
Author contributions
All authors listed have made a substantial, direct, and intellectual contribution to the work, and approved it for publication.
Funding
This work received funding from Curis, Inc., Lexington, MA, USA. This manuscript received medical writing support funded by Curis, Inc.
Conflict of interest
JB-K is the Chief Scientific Officer at, and has ownership interest including patents in, Hummingbird Bioscience. TG is the Chief Scientific Officer at, and has ownership interest including patents in, Kineta Inc. SL receives research support from the NIH. LL has served on advisory boards and as a consultant for Curis, Inc. and has received research support from Curis, Inc; and reports additional research support from AbbVie, AstraZeneca, and Bristol Myers Squibb, has served on a data safety monitoring board for G1 Therapeutics, and is the vice chair of the pharmacogenomics and population pharmacology committee of the Alliance for Clinical Trials in Oncology. JLL has patents issued and licensed to Curis, Inc. through ImmuNext. KM has received research support from the United States Department of Defense, Kidney Cancer Association, NextPoint Therapeutics, and Bristol Myers Squibb. RM is Head of Research and Development and has ownership interest at Curis, Inc. DN receives research support from the NCI and NIDCR. RN is employed by ImmuNext, has served on advisory boards and as a consultant for Curis, Inc, and reports research support from the NIH. AS has received research support to his institution from Curis, Inc.; and additional research support to his institution from Medimmune, AVID, Telix Pharmaceuticals, ITM, Fusion, Cyclotek, Adalta, TheraMyc, Humanigen, Antengene, National Health and Medical Research Council, National Imaging Facility, National Breast Cancer Foundation, Medical Research Future Fund, Australian Cancer Research Foundation, Victorian Cancer Agency.
The remaining author declares that the research was conducted in the absence of any commercial or financial relationships that could be construed as a potential conflict of interest.
Publisher’s note
All claims expressed in this article are solely those of the authors and do not necessarily represent those of their affiliated organizations, or those of the publisher, the editors and the reviewers. Any product that may be evaluated in this article, or claim that may be made by its manufacturer, is not guaranteed or endorsed by the publisher.
Abbreviations
ADCC, antibody-dependent cell cytotoxicity; AML, acute myeloid leukemia; APC, antigen-presenting cell; CRS, cytokine release syndrome; cSCC, cutaneous squamous cell carcinoma; CTLA-4, cytotoxic T-lymphocyte antigen-4; HNC, head and neck cancer; HR, hazard ratio; ICI, immune checkpoint inhibitor; IDC, invasive ductal carcinoma; Ig, immunoglobulin; IPMN, intraductal papillary mucinous neoplasm; mAb, monoclonal antibody; MDSC, myeloid-derived suppressor cells; MHC, major histocompatibility complex; MPM, malignant pleural mesothelioma; MSI+, microsatellite unstable; MSS, microsatellite stable; NK, natural killer; NSCLC, non-small cell lung cancer; PD-1, programmed cell death protein-1; PDAC, pancreatic ductal adenocarcinoma; PD-L1, programmed cell death ligand-1; PET, positron emission tomography; RCC, renal cell carcinoma; TAM, tumor-associated macrophage; TIL, tumor-infiltrating leukocytes; TMDD, target-mediated drug disposition; TME, tumor microenvironment; VISTA, V-domain immunoglobulin suppressor of T-cell activation.
References
1. ElTanbouly MA, Schaafsma E, Noelle RJ, Lines JL. VISTA: Coming of age as a multi-lineage immune checkpoint. Clin Exp Immunol (2020) 200:120–30. doi: 10.1111/cei.13415
2. Flies DB, Han X, Higuchi T, Zheng L, Sun J, Ye JJ, et al. Coinhibitory receptor PD-1H preferentially suppresses CD4+ T cell-mediated immunity. J Clin Invest (2014) 124:1966–75. doi: 10.1172/JCI74589
3. Martin AS, Molloy M, Ugolkov A, von Roemeling RW, Noelle RJ, Lewis LD, et al. VISTA expression and patient selection for immune-based anticancer therapy. Front Immunol (2023) 14:1086102. doi: 10.3389/fimmu.2023.1086102
4. ElTanbouly MA, Croteau W, Noelle RJ, Lines JL. VISTA: A novel immunotherapy target for normalizing innate and adaptive immunity. Semin Immunol (2019) 42:101308. doi: 10.1016/j.smim.2019.101308
5. Nowak EC, Lines JL, Varn FS, Deng J, Sarde A, Mabaera R, et al. Immunoregulatory functions of VISTA. Immunol Rev (2017) 276:66–79. doi: 10.1111/imr.12525
6. Wang L, Rubinstein R, Lines JL, Wasiuk A, Ahonen C, Guo Y, et al. VISTA, a novel mouse Ig superfamily ligand that negatively regulates T cell responses. J Exp Med (2011) 208:577–92. doi: 10.1084/jem.20100619
7. Flies DB, Higuchi T, Chen L. Mechanistic assessment of PD-1H coinhibitory receptor–induced T cell tolerance to allogeneic antigens. J Immunol (2015) 194:5294. doi: 10.4049/jimmunol.1402648
8. Flies DB, Wang S, Xu H, Chen L. Cutting edge: A monoclonal antibody specific for the programmed death-1 homolog prevents graft-versus-host disease in mouse models. J Immunol (2011) 187:1537–41. doi: 10.4049/jimmunol.1100660
9. Johnston RJ, Su LJ, Pinckney J, Critton D, Boyer E, Krishnakumar A, et al. VISTA is an acidic pH-selective ligand for PSGL-1. Nature (2019) 574:565–70. doi: 10.1038/s41586-019-1674-5
10. Wang J, Wu G, Manick B, Hernandez V, Renelt M, Erickson C, et al. VSIG-3 as a ligand of VISTA inhibits human T-cell function. Immunology (2019) 156:74–85. doi: 10.1111/imm.13001
11. Yasinska IM, Meyer NH, Schlichtner S, Hussain R, Siligardi G, Casely-Hayford M, et al. Ligand-receptor interactions of galectin-9 and VISTA suppress human T lymphocyte cytotoxic activity. Front Immunol (2020) 11:580557. doi: 10.3389/fimmu.2020.580557
12. Rogers BM, Smith L, Dezso Z, Shi X, DiGiammarino E, Nguyen D, et al. VISTA is an activating receptor in human monocytes. J Exp Med (2021) 218:e20201601. doi: 10.1084/jem.20201601
13. Thakkar D, Paliwal S, Dharmadhikari B, Guan S, Liu L, Kar S, et al. Rationally targeted anti-VISTA antibody that blockades the C-C’ loop region can reverse VISTA immune suppression and remodel the immune microenvironment to potently inhibit tumor growth in an Fc independent manner. J Immunother Cancer (2022) 10:e003382. doi: 10.1136/jitc-2021-003382
14. Yoon KW, Byun S, Kwon E, Hwang S-Y, Chu K, Hiraki M, et al. Control of signaling-mediated clearance of apoptotic cells by the tumor suppressor p53. Science (2015) 349:1261669. doi: 10.1126/science.1261669
15. ElTanbouly MA, Zhao Y, Nowak E, Li J, Schaafsma E, Le Mercier I, et al. VISTA is a checkpoint regulator for naïve T cell quiescence and peripheral tolerance. Science (2020) 367:eaay0524. doi: 10.1126/science.aay0524
16. Broughton TWK, ElTanbouly MA, Schaafsma E, Deng J, Sarde A, Croteau W, et al. Defining the signature of VISTA on myeloid cell chemokine responsiveness. Front Immunol (2019) 10:2641. doi: 10.3389/fimmu.2019.02641
17. Lines JL, Pantazi E, Mak J, Sempere LF, Wang L, O’Connell S, et al. VISTA is an immune checkpoint molecule for human T cells. Cancer Res (2014) 74:1924–32. doi: 10.1158/0008-5472.CAN-13-1504
18. Veglia F, Sanseviero E, Gabrilovich DI. Myeloid-derived suppressor cells in the era of increasing myeloid cell diversity. Nat Rev Immunol (2021) 21:485–98. doi: 10.1038/s41577-020-00490-y
19. Gabrilovich DI, Ostrand-Rosenberg S, Bronte V. Coordinated regulation of myeloid cells by tumours. Nat Rev Immunol (2012) 12:253–68. doi: 10.1038/nri3175
20. Zhang CC. A perspective on LILRBs and LAIR1 as immune checkpoint targets for cancer treatment. Biochem Biophys Res Commun (2022) 633:64–7. doi: 10.1016/j.bbrc.2022.09.019
21. Wang L, Jia B, Claxton DF, Ehmann WC, Rybka WB, Mineishi S, et al. VISTA is highly expressed on MDSCs and mediates an inhibition of T cell response in patients with AML. OncoImmunology (2018) 7:e1469594. doi: 10.1080/2162402X.2018.1469594
22. Le Mercier I, Chen W, Lines JL, Day M, Li J, Sergent P, et al. VISTA regulates the development of protective antitumor immunity. Cancer Res (2014) 74:1933–44. doi: 10.1158/0008-5472.CAN-13-1506
23. Deng J, Li J, Sarde A, Lines JL, Lee Y-C, Qian DC, et al. Hypoxia-induced VISTA promotes the suppressive function of myeloid-derived suppressor cells in the tumor microenvironment. Cancer Immunol Res (2019) 7:1079–90. doi: 10.1158/2326-6066.CIR-18-0507
24. Cao X, Ren X, Zhou Y, Mao F, Lin Y, Wu H, et al. VISTA expression on immune cells correlates with favorable prognosis in patients with triple-negative breast cancer. Front Oncol (2021) 10:583966. doi: 10.3389/fonc.2020.583966
25. Xie X, Zhang J, Shi Z, Liu W, Hu X, Qie C, et al. The expression pattern and clinical significance of the immune checkpoint regulator VISTA in human breast cancer. Front Immunol (2020) 11:563044. doi: 10.3389/fimmu.2020.563044
26. Zong L, Mo S, Yu S, Zhou Y, Zhang M, Chen J, et al. Expression of the immune checkpoint VISTA in breast cancer. Cancer Immunol Immunother (2020) 69:1437–46. doi: 10.1007/s00262-020-02554-3
27. Xie S, Huang J, Qiao Q, Zang W, Hong S, Tan H, et al. Expression of the inhibitory B7 family molecule VISTA in human colorectal carcinoma tumors. Cancer Immunol Immunother (2018) 67:1685–94. doi: 10.1007/s00262-018-2227-8
28. Zaravinos A, Roufas C, Nagara M, de Lucas Moreno B, Oblovatskaya M, Efstathiades C, et al. Cytolytic activity correlates with the mutational burden and deregulated expression of immune checkpoints in colorectal cancer. J Exp Clin Cancer Res (2019) 38:364. doi: 10.1186/s13046-019-1372-z
29. Kuklinski LF, Yan S, Li Z, Fisher JL, Cheng C, Noelle RJ, et al. VISTA expression on tumor-infiltrating inflammatory cells in primary cutaneous melanoma correlates with poor disease-specific survival. Cancer Immunol Immunother (2018) 67:1113–21. doi: 10.1007/s00262-018-2169-1
30. Chung YS, Kim M, Cha YJ, Kim KA, Shim HS. Expression of V-set immunoregulatory receptor in malignant mesothelioma. Modern Pathol (2020) 33:263–70. doi: 10.1038/s41379-019-0328-3
31. Muller S, Victoria Lai W, Adusumilli PS, Desmeules P, Frosina D, Jungbluth A, et al. V-domain Ig-containing suppressor of T-cell activation (VISTA), a potentially targetable immune checkpoint molecule, is highly expressed in epithelioid malignant pleural mesothelioma. Modern Pathol (2020) 33:303–11. doi: 10.1038/s41379-019-0364-z
32. Rooney C, Nixon C, Blyth K, Sethi T, Murphy D, McCaughan F. S45 VISTA expression in malignant pleural mesothelioma. Thorax (2019) 74:A29–A30. doi: 10.1136/thorax-2019-BTSabstracts2019.51
33. Villarroel-Espindola F, Yu X, Datar I, Mani N, Sanmamed M, Velcheti V, et al. Spatially resolved and quantitative analysis of VISTA/PD-1H as a novel immunotherapy target in human non–small cell lung cancer. Clin Cancer Res (2018) 24:1562–73. doi: 10.1158/1078-0432.CCR-17-2542
34. Zong L, Zhou Y, Zhang M, Chen J, Xiang Y. VISTA expression is associated with a favorable prognosis in patients with high-grade serous ovarian cancer. Cancer Immunol Immunother (2020) 69:33–42. doi: 10.1007/s00262-019-02434-5
35. Blando J, Sharma A, Higa MG, Zhao H, Vence L, Yadav SS, et al. Comparison of immune infiltrates in melanoma and pancreatic cancer highlights VISTA as a potential target in pancreatic cancer. Proc Natl Acad Sci (2019) 116:1692–7. doi: 10.1073/pnas.1811067116
36. Byers JT, Paniccia A, Kaplan J, Koenig M, Kahn N, Wilson L, et al. Expression of the novel costimulatory molecule B7-H5 in pancreatic cancer. Ann Surg Oncol (2015) 22:1574–9. doi: 10.1245/s10434-014-4293-2
37. Liu J, Xie X, Xuan C, Li T, Wang L, Teng L, et al. High-density infiltration of V-domain immunoglobulin suppressor of T-cell activation up-regulated immune cells in human pancreatic cancer. Pancreas (2018) 47:725–31. doi: 10.1097/MPA.0000000000001059
38. Gao J, Ward JF, Pettaway CA, Shi LZ, Subudhi SK, Vence LM, et al. VISTA is an inhibitory immune checkpoint that is increased after ipilimumab therapy in patients with prostate cancer. Nat Med (2017) 23:551–5. doi: 10.1038/nm.4308
39. Hong S, Yuan Q, Xia H, Zhu G, Feng Y, Wang Q, et al. Analysis of VISTA expression and function in renal cell carcinoma highlights VISTA as a potential target for immunotherapy. Protein Cell (2019) 10:840–5. doi: 10.1007/s13238-019-0642-z
40. Zapała Ł, Kunc M, Sharma S, Pęksa R, Popęda M, Biernat W, et al. Immune checkpoint receptor VISTA on immune cells is associated with expression of T-cell exhaustion marker TOX and worse prognosis in renal cell carcinoma with venous tumor thrombus. J Cancer Res Clin Oncol (2022) 149(7):4131–39. doi: 10.1007/s00432-022-04329-y
41. He X-L, Zhou Y, Lu H-Z, Li Q-X, Wang Z. Prognostic value of VISTA in solid tumours: a systematic review and meta-analysis. Sci Rep (2020) 10:2662. doi: 10.1038/s41598-020-59608-w
42. Schaafsma E, Croteau W, ElTanbouly M, Nowak EC, Smits NC, Deng J, et al. VISTA targeting of T-cell quiescence and myeloid suppression overcomes adaptive resistance. Cancer Immunol Res (2023) 11:38–55. doi: 10.1158/2326-6066.CIR-22-0116
43. Yum J-EI, Hong Y-K. Terminating Cancer by Blocking VISTA as a Novel Immunotherapy: Hasta la vista, baby. Front Oncol (2021) 11:658488. doi: 10.3389/fonc.2021.658488
44. Huang X, Zhang X, Li E, Zhang G, Wang X, Tang T, et al. VISTA: an immune regulatory protein checking tumor and immune cells in cancer immunotherapy. J Hematol Oncol (2020) 13:83. doi: 10.1186/s13045-020-00917-y
45. Kondo Y, Ohno T, Nishii N, Harada K, Yagita H, Azuma M. Differential contribution of three immune checkpoint (VISTA, CTLA-4, PD-1) pathways to antitumor responses against squamous cell carcinoma. Oral Oncol (2016) 57:54–60. doi: 10.1016/j.oraloncology.2016.04.005
46. Conroy M, Naidoo J. Immune-related adverse events and the balancing act of immunotherapy. Nat Commun (2022) 13:392. doi: 10.1038/s41467-022-27960-2
47. Mehta N, Maddineni S, Kelly RL, Lee RB, Hunter SA, Silberstein JL, et al. An engineered antibody binds a distinct epitope and is a potent inhibitor of murine and human VISTA. Sci Rep (2020) 10:15171. doi: 10.1038/s41598-020-71519-4
48. Yuan L, Tatineni J, Mahoney KM, Freeman GJ. VISTA: A mediator of quiescence and a promising target in cancer immunotherapy. Trends Immunol (2021) 42:209–27. doi: 10.1016/j.it.2020.12.008
49. Johnson M, Lines JL, Carriere C, Molloy M, Martell R, von Roemeling R, et al. Phase 1 Study of CI-8993 anti-VISTA antibody in patients with advanced solid tumor Malignancies. Soc Immunother Cancer (2020) 8:A417–A417. doi: 10.1136/jitc-2020-SITC2020.0392
50. NCCN. Management of immunotherapy-related toxicities V2 (2023). Available at: https://www.nccn.org/professionals/physician_gls/pdf/immunotherapy.pdf.
51. Noelle R, Johnson M, Rodon J, Zauderer M, Lewis L, Severgini M, et al. Pharmacokinetic and pharmacodynamic data from a phase 1 study of CI-8993 anti-VISTA antibody in patients with advanced solid tumors. Soc Immunother Cancer (2022) 10. doi: 10.1136/jitc-2022-SITC2022.0761
52. Levy G. Pharmacologic target-mediated drug disposition. Clin Pharmacol Ther (1994) 56:248–52. doi: 10.1038/clpt.1994.134
53. Dua P, Hawkins E, van der Graaf PH. A tutorial on target-mediated drug disposition (TMDD) models. CPT Pharmacometrics Syst Pharmacol (2015) 4:324–37. doi: 10.1002/psp4.41
54. Dostalek M, Gardner I, Gurbaxani BM, Rose RH, Chetty M. Pharmacokinetics, pharmacodynamics and physiologically-based pharmacokinetic modelling of monoclonal antibodies. Clin Pharmacokinet (2013) 52:83–124. doi: 10.1007/s40262-012-0027-4
55. Kagan L, Turner MR, Balu-Iyer SV, Mager DE. Subcutaneous absorption of monoclonal antibodies: role of dose, site of injection, and injection volume on rituximab pharmacokinetics in rats. Pharm Res (2012) 29:490–9. doi: 10.1007/s11095-011-0578-3
56. Dharmadhikari B, Thakkar D, Zharkova O, Ray D, Tirado-Magallanes R, Lai J, et al. Anti-VISTA antibody HMBD-002 reprograms tumor associated macrophages and promotes cytotoxic T cell response. J Immunother Cancer (2022) 10. doi: 10.1136/jitc-2022-SITC2022.0532
57. Rodon Ahnert J, Gruber JJ, Telli ML, Mita MM, Mita AC, Kim JW, et al. A phase 1 first-in-human clinical trial of HMBD-002, an IgG4 monoclonal antibody targeting VISTA, in advanced solid tumors. JCO (2023) 41:TPS2664–TPS2664. doi: 10.1200/JCO.2023.41.16_suppl.TPS2664
58. Iadonato S, Tarcha E, Bader R, Dutzar B, Eyde N, Frazier E, et al. 76P Highly potent fully human anti-VISTA antibodies efficiently abrogate the interaction of this new target checkpoint inhibitor to its different putative receptors at different pH. Ann Oncol (2020) 31:S1448–9. doi: 10.1016/j.annonc.2020.10.564
59. Guillaudeux T, Lustig K, Frazier E, Kabi N, Katz C, Peckham D, et al. 425 KVA12.1: an anti-VISTA monoclonal antibody with strong single agent anti-tumor activity and no evidence of cytokine mediated toxicity. J Immunother Cancer (2022) 10. doi: 10.1136/jitc-2022-SITC2022.0425
60. Guillaudeux T. (2022). KVA12123, a VISTA blocking immunotherapy, in: Antibody Engineering and Therapeutics Conference.
61. Wang L, Le Mercier I, Putra J, Chen W, Liu J, Schenk AD, et al. Disruption of the immune-checkpoint VISTA gene imparts a proinflammatory phenotype with predisposition to the development of autoimmunity. Proc Natl Acad Sci U.S.A. (2014) 111:14846–51. doi: 10.1073/pnas.1407447111
62. Ceeraz S, Eszterhas SK, Sergent PA, Armstrong DA, Ashare A, Broughton T, et al. VISTA deficiency attenuates antibody-induced arthritis and alters macrophage gene expression in response to simulated immune complexes. Arthritis Res Ther (2017) 19:270. doi: 10.1186/s13075-017-1474-y
63. Ceeraz S, Sergent PA, Plummer SF, Schned AR, Pechenick D, Burns CM, et al. VISTA deficiency accelerates the development of fatal murine lupus nephritis. Arthritis Rheumatol (2017) 69:814–25. doi: 10.1002/art.40020
64. Borggrewe M, Grit C, Den Dunnen WFA, Burm SM, Bajramovic JJ, Noelle RJ, et al. VISTA expression by microglia decreases during inflammation and is differentially regulated in CNS diseases. Glia (2018) 66:2645–58. doi: 10.1002/glia.23517
65. Sepich-Poore GD, Zitvogel L, Straussman R, Hasty J, Wargo JA, Knight R. The microbiome and human cancer. Science (2021) 371:eabc4552. doi: 10.1126/science.abc4552
66. Matson V, Fessler J, Bao R, Chongsuwat T, Zha Y, Alegre M-L, et al. The commensal microbiome is associated with anti-PD-1 efficacy in metastatic melanoma patients. Science (2018) 359:104–8. doi: 10.1126/science.aao3290
Keywords: VISTA, checkpoint inhibitors (ICIs), immunotherapy, PD-1, myeloid, immune checkpoint, immuno-oncology (IO)
Citation: Noelle RJ, Lines JL, Lewis LD, Martell RE, Guillaudeux T, Lee SW, Mahoney KM, Vesely MD, Boyd-Kirkup J, Nambiar DK and Scott AM (2023) Clinical and research updates on the VISTA immune checkpoint: immuno-oncology themes and highlights. Front. Oncol. 13:1225081. doi: 10.3389/fonc.2023.1225081
Received: 18 May 2023; Accepted: 21 August 2023;
Published: 15 September 2023.
Edited by:
Yutian Zou, Sun Yat-sen University Cancer Center (SYSUCC), ChinaReviewed by:
Jamal Majidpoor, Iran University of Medical Sciences, IranMohamed ElTanbouly, The Rockefeller University, United States
Tiezheng Hou, University College London, United Kingdom
Copyright © 2023 Noelle, Lines, Lewis, Martell, Guillaudeux, Lee, Mahoney, Vesely, Boyd-Kirkup, Nambiar and Scott. This is an open-access article distributed under the terms of the Creative Commons Attribution License (CC BY). The use, distribution or reproduction in other forums is permitted, provided the original author(s) and the copyright owner(s) are credited and that the original publication in this journal is cited, in accordance with accepted academic practice. No use, distribution or reproduction is permitted which does not comply with these terms.
*Correspondence: Randolph J. Noelle, Randolph.J.Noelle@dartmouth.com; Andrew M. Scott, Andrew.Scott@onjcri.org.au