- 1Engineering Research Center of Modern Preparation Technology of TCM, Innovation Research Institute of Traditional Chinese Medicine, Shanghai University of Traditional Chinese Medicine, Shanghai, China
- 2Department of Cultural Heritage and Museology, Zhejiang University, Hangzhou, China
- 3Zhejiang Provincial Key Laboratory of Silkworm Bioreactor and Biomedicine, College of Life Sciences and Medicine, Zhejiang Sci-Tech University, Hangzhou, China
Strategies for treating brain metastases of breast cancer have demonstrated limited efficacy due to the blood–brain barrier (BBB). Gene therapy could improve the efficacy of chemotherapeutic drugs. Exosomes derived from the mesenchymal stem cells (MSCs) are small membrane-based gene vectors that can pass through the BBB. CXCR4 is the most commonly found chemokine receptor in human cancer cells. Furthermore, the SDF-1/CXCR4 axis plays an important role in the homing of MSCs for tumor cell diffusion and metastasis. TRAIL can selectively induce apoptosis in transformed cells without significant toxic side effects in normal tissues. In this study, exosomes were isolated from MSCCXCR4+TRAIL transduced with CXCR4 and TRAIL using a lentiviral vector. Synergistic antitumor study showed that exosomeCXCR4+TRAIL exerted significant activity as a cooperative agent with carboplatin in an MDA-MB-231Br SCID mouse model, potentially engendering a novel strategy for advancing the treatment of brain metastases of breast cancer. Based on this study, further investigation of the effect of the vector on BBB and inducing apoptosis of brain tumors is warranted. In addition, the safety of the vector in animals during the treatment needs to be evaluated.
Introduction
Brain metastasis has become the hot spot in the treatment of breast cancer due to its high morbidity, aggressiveness, and inaccessibility. Unfortunately, more than a quarter of breast cancer patients finally develop brain metastasis (1). Considering the high risk of surgery and the apparent neurotoxicity of brain radiotherapy, the use of chemotherapy, such as carboplatin, which is a first-line drug used in metastatic breast cancer therapy, is beneficial for treating orthotopic brain tumor, and brain metastases (2). However, the chemotherapeutic agents used for brain tumor treatment cannot pass across the blood–brain barrier (BBB) efficiently (3, 4). Thus, it is difficult to use chemotherapy to treat brain metastasis (5).
Nano-carriers have been developed to increase the ability of drugs to penetrate the BBB. Nevertheless, rapid drug clearance by the mononuclear phagocyte system (MPS) and toxicity of the nanoparticles have frequently been observed (6). Although polyethylene glycol is used to decrease drug uptake by the MPS, this can still result in reduced interaction among the target cells, which can decrease the drug distribution in the brain (7, 8). By comparison, exosome, a natural substance derived from the body, can penetrate into the BBB, which can improve drug transport to the brain by decreasing the influence of the MPS (9, 10). All of these characteristics make this system a promising therapeutic tool for treating brain diseases.
Exosomes are nano-sized carriers (30–120 nm) that are derived from different cell types such as B cells, T cells, epithelial cells, tumor cells, and mesenchymal stem cells (MSCs) (11–13). An ideal source of exosomes produces an abundance of exosomes that are clinically applicable. MSC is a unique human cell type, which has a capacity for large-scale preparation of exosomes (14, 15). MSCs also have characteristics that are beneficial for producing exosomes such as being easily accessible and non-immunogenic and having intrinsic therapeutic properties (14). Thus, MSC is the appropriate cell type for the large-scale production of exosomes.
Based on these considerations, we obtained the isolated and purified exosomes from MSCs transduced with lentiviral CXCR4 and lentiviral tumor necrosis factor-related apoptosis-inducing ligand (TRAIL, also known as Apo2L) (ExoCXCR4+TRAIL). CXCR4 and its ligand, SDF-1, are expressed extensively, including in immune cells, the brain, and the heart (16). Many studies have demonstrated that the SDF-1/CXCR4 axis plays an important role in processes including chemotaxis, stem cell homing, engraftment, expression of adhesion molecules, proliferation, and survival (17, 18). Thus, CXCR4 should lead to improved delivery of exosomes from MSCs to disease sites. Meanwhile, the molecule TRAIL, a member of the tumor necrosis factor ligand family, serves as a ligand for the four cellular membrane receptors, which is a death receptor activating the apoptotic program. As a type 2 transmembrane protein, TRAIL is expressed on the cell surface. It can be released by soluble TRAIL homotrimers. It has been observed that soluble TRAIL mostly causes apoptosis in tumor cells but not in normal tissues (19). In previous reports, the application of a combined medication for tumor cells with TRAIL and drugs has been evaluated for whether TRAIL could synergize with chemotherapeutic drugs (20–22).
The main principle of the scheme to deal with cancer treatment is that combination therapies can improve selectivity and efficacy in comparison to single-agent treatments. To explore the effect of combination CXCR4/TRAIL/carboplatin treatment for brain metastasis of breast cancer, this study examined the anti-tumor effect of ExoCXCR4+TRAIL combined with carboplatin in an MDA-MB-231Br severe combined immunodeficiency (SCID) mouse model. ExoCXCR4+TRAIL has been used as a cooperative agent with carboplatin against brain metastasis of breast cancer in vivo, providing the possibility of using this agent as a novel biological method for enhancing the treatment of brain metastases of breast cancer.
Methods
Materials
Carboplatin (C2538) was purchased from Sigma-Aldrich; Dulbecco's modified Eagle's medium (DMEM), fetal bovine serum, penicillin, trypsin, and streptomycin were purchased from Gibco BRL (Gaithersberg, MD, USA). All of the other chemical reagents used were of analytical grade.
Animals
SCID mice and 3-week-old Sprague-Dawley (SD) male rats were supplied by the Experimental Animal Center, Zhejiang University, China. All of the animals were kept in 12-h light/dark cycle and (25 ± 1)°C temperature with free access to food and water. All animal procedures were performed in accordance with Health Guidelines for the Care and Use of Laboratory Animals of Zhejiang University, and the experiments were approved by the Animal Ethics Committee of Zhejiang University.
Cell Lines
Brain-seeking human metastatic breast cancer cells were obtained from ATCC (USA). Cells stably transfected to express firefly luciferase (MDA-MB-231Br-Luc) were cultured in DMEM supplemented with 10% fetal bovine serum. Only cells from passages 2–10 were used. The human embryonic kidney 293T (293T) cells (GNHu17) were obtained from the Cell Bank of the Chinese Academy of Sciences (China) and were grown in DMEM containing 10% fetal bovine serum, 100 U/ml penicillin, and 100 mg/ml streptomycin. All of the cell lines were cultured at 37°C with 5% CO2.
Isolation and Culture of MSCs
MSCs were isolated and cultured according to the protocol described in our previous report (23). MSCs were isolated from rat bone marrow cells obtained from the hind femurs of 3-week-old SD male rats. The cell suspension was placed into a 100-mm dish and cultured at 37°C in a humidified atmosphere containing 5% CO2. Sub-confluent cells from passages 2–6 were used for further study.
Transfection, Virus Preparation, and Titer Determination
The CXCR4 gene was amplified using gene-specific primers containing the EcoRI and BamHI restriction sites, respectively. Polymerase chain reaction was performed, and the recombinant lentivirus vector was generated by cloning CXCR4 gene into the pLV-Puro vector as previously described (24). Then, 293T cells were transfected with the lentivirus vectors (CXCR4-GFP) in the presence of polybrene (8 μg/ml) for 13 h and switched to fresh medium. The titer of lentivirus vectors was (6.50 ± 0.5) × 108 transforming units/ml. The supernatant containing the lentivirus containing CXCR4 and GFP (LVCXCR4) was collected, aliquoted, and stored at −80°C in a freezer. The lentivirus-containing TRAIL (LVTRAIL) plasmids were constructed as previously reported (25). Vector stocks were prepared according to published protocols (26, 27). Briefly, 1.8 × 107 293T cells in T150 flasks were transfected with 20 μg transfer vector, 14 μg helper plasmid, and 7 μg envelope plasmid by CaPO4 precipitation. The supernatant was collected after the transfection, filtered using 0.22 μm Stericups (Millipore, Bedford, MA), and centrifuged for 140 min at 20,000 rpm. The titer of lentivirus vectors was (7.25 ± 0.85) × 108 transforming units/ml. The concentrated virus was dissolved in 100 μl phosphate buffer saline per 30 ml supernatant.
Transduction of MSC by LVCXCR4
MSCs were cultured in six-well-plates at 5 × 105 cells/well-concentration containing 4 μg/ml polybrene, and LVCXCR4 was added. The plates were centrifuged (1,200 rpm, 37°C, 1 h), and the medium was replaced by fresh medium after 6–16 h. The infected MSCs were then selected by continuous incubation with 10 μg/ml puromycin (Sigma) added, starting 1 day after transduction. Prior to further experiments, the MSCs overexpressing CXCR4 (MSCCXCR4) were cultured for 48 h without puromycin.
Transduction of MSC CXCR4 by LVTRAIL
MSCCXCR4 samples were cultured in six-well-plates at 5 × 105 cells/well-containing 4 μg/ml polybrene, and LVTRAIL was added. The plates were centrifuged (1,200 rpm, 37°C, 1 h). After 6–16 h, the medium was replaced by fresh medium.
CXCR4 and TRAIL Expression in MSCs
The expression levels of CXCR4 and TRAIL in MSCs were further analyzed by Western blot. Briefly, MSCs and the MSCs overexpressing CXCR4 and TRAIL (MSCCXCR4+TRAIL) were lysed in a cell lysis buffer (Beyontime, China). Proteins of the two groups were separated by 10% sodium dodecyl sulfate-polyacrylamide gel electrophoresis. They were then transferred onto a nitrocellulose transfer membrane (Whatman). The membranes were first incubated with primary antibodies against CXCR4, TRAIL, and GAPDH (Abcam, Britain) and then with horseradish peroxidase labeled secondary antibodies. According to the manufacturer's instructions, the peroxidase activity was visualized with an enhanced chemiluminescence kit (Amersham Biosciences).
Exosome Isolation
Exosome isolation from MSCCXCR4+TRAIL and MSCs was carried out using total exosome isolation reagent (Invitrogen) as reported previously (28). The principal process was as follows: MSCs were cultured overnight and used when the confluence reached 80%. The MSCs were then washed with phosphate buffer saline and seeded in a hypoxic (1%) environment with serum-free media for 24 h. They were then centrifuged (Beckman Coulter Optima XPN-80, USA), and the supernatant was filtered through a 0.2-μm filter. About 100 ml of supernatant was concentrated to 500 μl using a membrane with a 100 kDa MWCO (Millipore). The concentrate and half the volume of total exosome isolation reagent were mixed well. The homogenous solution was centrifuged (4°C, 10,000 g, 1 h). The supernatant fluid was discarded, and the exosomes were resuspended in phosphate buffer saline. The isolated exosomes were stored at −80°C until use.
Identification and Characterization of ExoCXCR4+TRAIL
ExoCXCR4+TRAIL, the exosome isolated from MSCCXCR4+TRAIL, was observed and photographed under transmission electron microscopy (TEM) (Tecnai G2 F20 S-Twin; FEI, Hillsboro, OR, USA) to get a better understanding of it. Furthermore, to identify the characteristics of exosomes, Western blot was carried out using the standard protocols described in section CXCR4 and TRAIL Expression in MSCs. The membranes were incubated with primary antibodies against CD63, CD81, CXCR4, ALIX, or Beta-Actin (BD Biosciences). They were further incubated with a chemiluminescent horseradish peroxidase substrate to detect primary antibody bound to the antigen present. In addition, the effect of CXCR4 and TRAIL on the characters of exosomes isolated from MSCs or MSCCXCR4+TRAIL was identified by Western blot.
Animal Tumor Model
MDA-MB-231Br-Luc cells (5 × 105) were implanted intracranially into SCID mice as previously described (29). During the process, the appearance and behavior of the SCID mice were recorded daily, and no profound effects of distress and pain were observed. In order to evaluate the animal model, the brain of SCID mice was imaged with an in vivo imaging system on days 0, 14, 21, and 35 after cell implantation.
Anti-tumor Study of Carboplatin Combined With ExoCXCR4+TRAIL in Brain Tumors
Animal tumor models were grown for 14 days prior to carotid injection of carboplatin (5 mg/kg) combined with ExoCXCR4+TRAIL (4 mg/kg) or exosome (4 mg/kg) on alternative days for 14 days. The mouse brain tumors in the two groups were then imaged with the in vivo imaging system.
Statistical Analysis
The results were expressed as mean ± standard deviation. Statistical significance was set at P < 0.05.
Results
Identification of MSCCXCR4+TRAIL
To evaluate CXCR4 expression, the fluorescence of MSCCXCR4 transduced by LVCXCR4 was observed under fluorescent micrographs, with MSC transduced by the lentivirus containing GFP (LVGFP) as the positive control group. Green fluorescence was observed in the MSCCXCR4 group, similar to that of the MSC group (Figure 1A). The result revealed that CXCR4 gene did not influence MSCs in terms of the MSC morphology and fluorescence intensity. Moreover, Western blots performed after the transduction by LVCXCR4 revealed that MSCCXCR4 exhibited a significantly higher level of CXCR4 than the MSCs (Figure 1B). This demonstrated that the lentiviral vector had transduced CXCR4 into the MSCs successfully. Based on the results, the evaluation of MSCCXCR4+TRAIL was further carried out. The presence of CXCR4 and TRAIL in MSCCXCR4+TRAIL after transduction by LVCXCR4 and LVTRAIL was confirmed by Western blotting. Western blots revealed that MSCCXCR4+TRAIL exhibited an apparent increase in CXCR4 level, and expression of TRAIL was also identified (Figure 2). This demonstrated that the lentiviral vector had transduced CXCR4 and TRAIL into MSCs successfully.
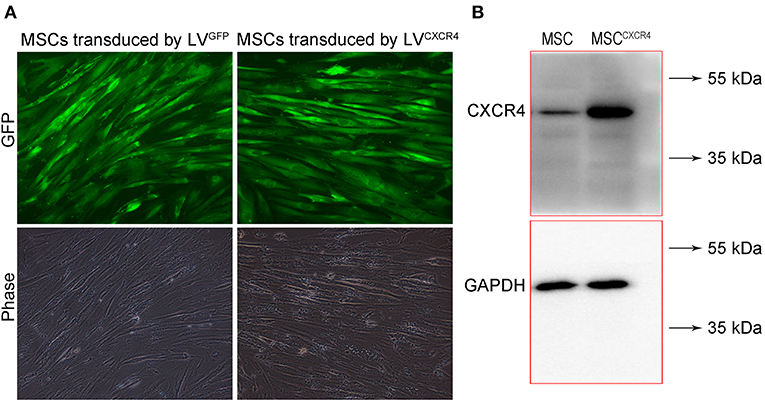
Figure 1. Characterization of MSCCXCR4 transduced by LVCXCR4. (A) Green fluorescence of MSCCXCR4 transduced by LVCXCR4 observed under fluorescent micrographs, with MSCs transduced by LVGFP as the positive control group. (B) Expression of CXCR4 in MSCs and MSCCXCR4 identified by Western blotting. LVCXCR4, the lentivirus containing CXCR4 and GFP; LVGFP, the lentivirus containing GFP; MSCCXCR4, MSCs overexpressing CXCR4.
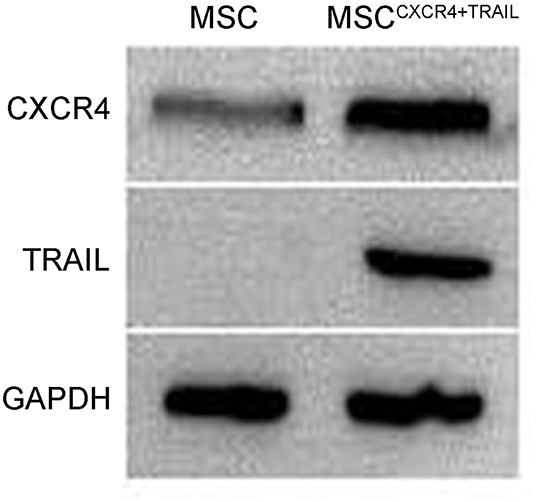
Figure 2. Expression of CXCR4 and TRAIL in MSCs and MSCCXCR4+TRAIL identified by Western blotting. MSCCXCR4+TRAIL, MSCs overexpressing CXCR4 and TRAIL.
Identification of ExoCXCR4+TRAIL
From the TEM results, ExoCXCR4+TRAIL showed double-membrane and rounded structures (red arrow) with a size of 40–90 nm (Figure 3A), which is similar to previous descriptions (30, 31). Western blot analysis confirmed that the ExoCXCR4+TRAIL fraction expressed CD63, CD81, and ALIX, all of these being well-established exosome markers (Figure 3B) (32). Furthermore, it was observed that ExoCXCR4+TRAIL could be influenced by LVCXCR4 and LVTRAIL transduction, as indicated by significant upregulation of the CXCR4 and TRAIL expression levels in comparison with exosome (Figures 3B, 4). The results revealed that CXCR4 and TRAIL were transduced into ExoCXCR4+TRAIL without changing the main phenotypic expressions of the specific surface molecules of exosomes, which is an advantage in gene modification of exosomes, as reported previously (32).
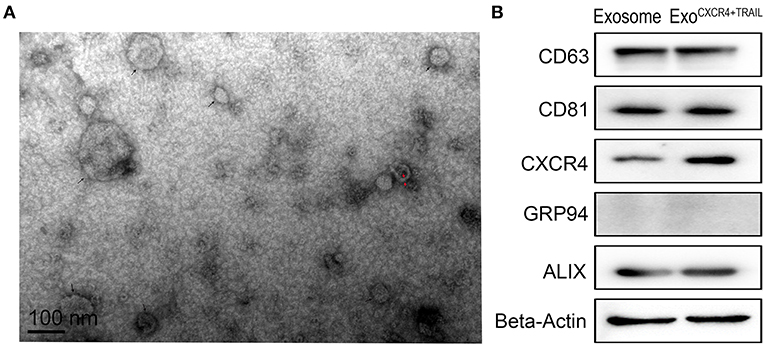
Figure 3. Characterization of ExoCXCR4+TRAIL. (A) Transmission electron micrograph of ExoCXCR4+TRAIL. Isolated exosomes are spherical (at the point of the black arrow) and membrane-encapsulated bodies (at the point of the red arrow) with a size of 40–90 nm. (B) Expression of exosomal markers in exosomes and ExoCXCR4+TRAIL identified by Western blotting. ExoCXCR4+TRAIL, exosomes isolated from MSCs transduced by CXCR4 and TRAIL with lentiviral vector.
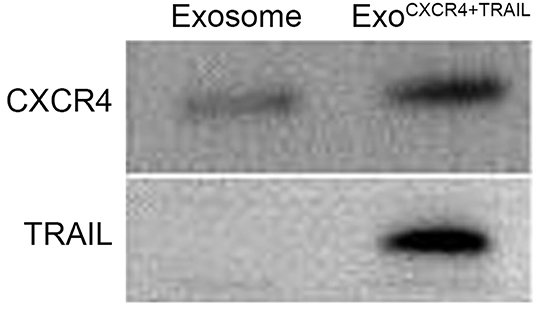
Figure 4. Expression of CXCR4 and TRAIL in exosomes and ExoCXCR4+TRAIL identified by Western blotting. ExoCXCR4+TRAIL, exosomes isolated from MSC transduced by CXCR4 and TRAIL with lentiviral vector.
Anti-tumor Study of Carboplatin Combined With ExoCXCR4+TRAIL in Brain Tumors
SCID mouse brains were imaged using the in vivo imaging system on days 0, 14, 21, and 35 after MDA-MB-231Br-Luc cells were implanted intracranially into the SCID mice (Figure 5A). The fluorescence intensity in mice was observed quantitatively on days 0, 14, 21, and 35, respectively (Figure 5B). Figure 5 shows that the bioluminescence value and area in the brain increased continuously with time. Moreover, at the beginning of the 14th day, the early stage of tumor growth in SCID mice brain was observed. Therefore, on the 14th day, after the brain metastasis of breast cancer model was established, the SCID mice were administered with carboplatin (5 mg/kg) in combination with ExoCXCR4+TRAIL (4 mg/kg) (Carboplatin + ExoCXCR4+TRAIL group) or exosome (4 mg/kg) (Carboplatin + exosome group) on alternate days for 14 days. The fluorescence intensity in both of the groups was observed by the in vivo imaging system (Figure 6A) and detected with quantitative analysis (Figure 6B) on day 0 and 14, respectively. Figure 6 shows that the signal of the tumor in the experimental group was decreased compared to the controls (P < 0.05), which indicates that ExoCXCR4+TRAIL has the potential to enhance the antitumor effect of carboplatin.
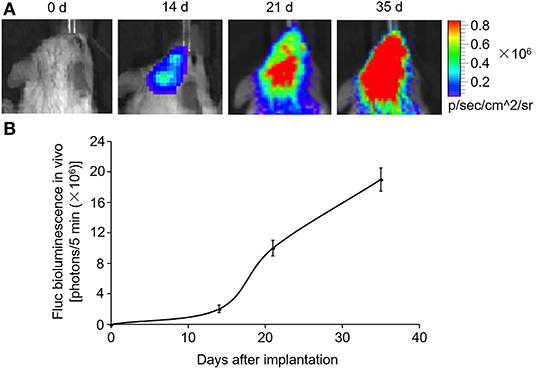
Figure 5. Construction of brain metastasis of a breast cancer model. MDA-MB-231Br-Luc cells (5 × 105) implanted intracranially into SCID mice. (A) SCID mouse brains imaged with the in vivo imaging system on days 0, 14, 21, and 35 after cell implantation. (B) Fluorescence intensity in mice detected by quantitative analysis on days 0, 14, 21, and 35, respectively. All values expressed as mean ± SD; n = 6 for each group.
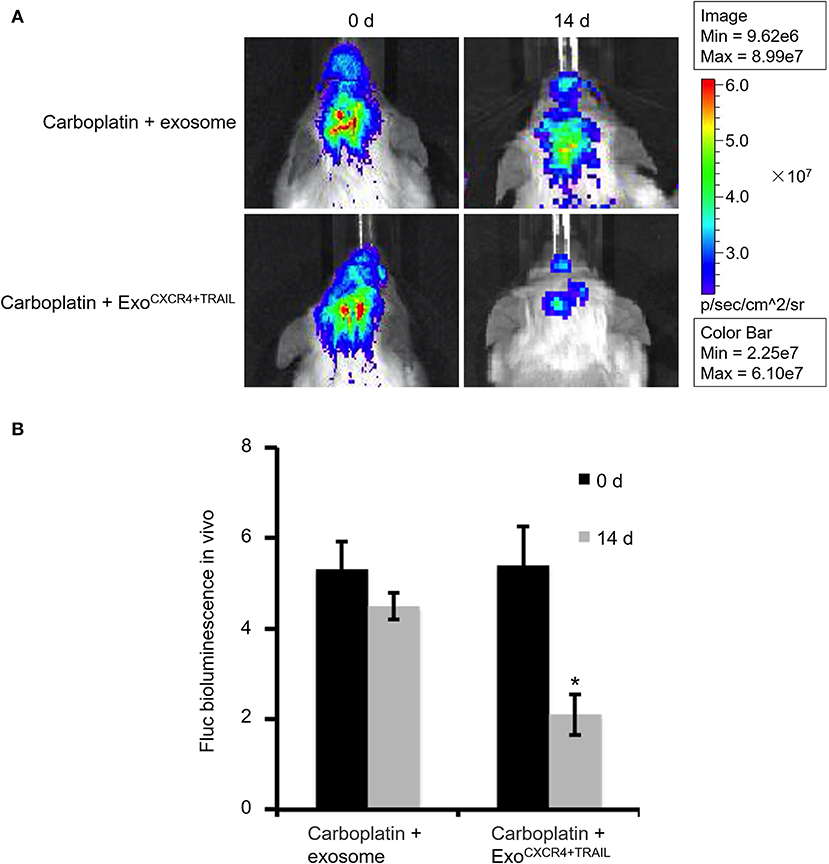
Figure 6. Fluorescence intensity in SCID mice administered with carboplatin (5 mg/kg) in combination with exosome (4 mg/kg) (Carboplatin + exosome group) and ExoCXCR4+TRAIL (4 mg/kg) (Carboplatin + ExoCXCR4+TRAIL group) on alternate days for 14 days after constructing the brain metastasis of breast cancer model (A) observed by the in vivo imaging system and (B) detected with quantitative analysis on days 0 and 14, respectively. All values expressed as mean ± SD. ExoCXCR4+TRAIL, exosomes isolated from MSCs transduced by CXCR4 and TRAIL with lentiviral vector. *P < 0.05: “Carboplatin + ExoCXCR4+TRAIL” group vs. “Carboplatin + exosome” group; n = 6 for each group.
Discussion
MSCs have been focused upon because of their unique biological properties in vivo, which enable them to be used for the treatment of many pathological conditions (33). In cancer, MSCs play a crucial role in promoting tumor progression. They also contribute to tumor growth and progression in different cancer types (34), although their anti-tumor activities have also been reported (35). MSCs provide a framework for anchoring tumor cells in the form of tumor stroma, and then secrete factors that facilitate tumor growth (36). Moreover, under the influence of cytokines or chemokines, they can transdifferentiate into M2-type microphages, myeloid-derived suppressor cells, or M2-type macrophages in the tumor microenvironment (37–39). Furthermore, Nakamizo A. et al. and our previous study have proved that during the initial period of administration, the majority of MSCs were reported to be filtered by the lung and that only rare MSCs were integrated into the tumor (40, 41). This property could impact the benefit of MSCs when applied for the treatment of brain diseases.
Nowadays, endosome-derived exosomes, a kind of extracellular vesicles, have emerged as physiologically related and meaningful components of the MSC secretome (42). Exosomes regulate neurite outgrowth (43), promote angiogenesis (44), reduce myocardial ischemia/reperfusion injury (45), and repair acute kidney injury (46). Proteomic analysis reveals that MSC-derived exosomes have characteristic signaling molecules and critical surface markers of the MSCs (47). In addition to the advantages mentioned above, exosomes are also applied to deliver drugs across the BBB by decreasing MPS drug clearance (9, 10). Thus, it is reasonable to hypothesize that exosomes have gene therapy potential for brain diseases.
Based on the reports mentioned above, exosome derived from MSCs was applied as a tool for gene delivery. Therefore, gene modification of MSCs is an important part of the study. In order to explore the therapeutic potential of MSCs, genetically modified MSCs expressing different factors have been studied (48). In terms of safety and efficacy, lentiviral vectors may be the best tool for producing stable genetically modified MSCs. The lentiviral vectors have little influence on the biology of the transduced cells and can realize multiple modifications in their backbone. They are available on the market and in research groups and can be used to obtain constitutive or inducible transgene expression in MSCs (49). In other words, lentiviral vectors have turned out to be one of the highest-potential and most useful vehicles for MSC gene modification.
TRAIL is a member of the tumor necrosis factor ligand superfamily (50). It exerts minimal activity against most normal cell types (50, 51) but is highly expressed in several kinds of solid tumors (22). Cytotoxic chemotherapeutics can regulate the expression of TRAIL receptors (52, 53). Moreover, they can modify response to TRAIL receptor agonists by changing the levels of pro- and anti-apoptotic regulatory proteins (54, 55). Based on this consideration, a combination of cytotoxic chemotherapy with agents targeting TRAIL receptors showed the potential of synergistic proapoptotic effects. Additionally, CXCR4, a G-protein-coupled receptor, can activate several related downstream signaling pathways after stimulation. This is a key factor related to several processes, such as homing and endothelial cell migration (17, 18). Thus, after CXCR4 gene modification, the ability of exosomes from MSCs to home to disease sites should be enhanced.
Based on the above considerations, this study is the first to present the preparation of ExoCXCR4+TRAIL derived from MSCs. Utilizing the ability of ExoCXCR4+TRAIL to cross the BBB and its advantages of being an excellent gene vector, we investigated whether a combination of CXCR4, TRAIL, and carboplatin used in the clinic was effective to treat a brain metastasis of breast cancer model. From Figure 6, we could find that ExoCXCR4+TRAIL has potential for use in the treatment of brain metastases of breast cancer, but further study is needed of its associated mechanisms, such as whether the vector can effectively cross the BBB and whether apoptosis occurs in the brain tumors. In addition, further evaluation of the safety of the vector in animals during the treatment is necessary. In future research, we will use carboplatin or only exosome as a single-treatment group to reveal the differences among the groups more comprehensively. In the meanwhile, a survival plot for the anti-tumor effect should be produced. Considering the problem that there are highly heterogeneous responses even in syngeneic mice, the images of more mice should also be investigated. Moreover, histological evaluation is a very important method for the further study of the relationship between the shrinkage of tumor on fluorescence imaging and tumor cell apoptosis. Besides, the uptake of ExoCXCR4+TRAIL and its effect on MDA-MB-231Br cells will be investigated in the next step, which could help us provide comprehensive evidence to show that CXCR4/TRAIL-enriched exosomes exerted this role by targeting and apoptosis induction. Furthermore, tumor marker quantification under the influence of exosomes needs further study. Analysis of proteins and cytokines involved in the tumor response will be needed to conclude that the present gene therapy strategy is working.
Conclusion
CXCR4/TRAIL-enriched exosomes were successfully obtained from MSCs overexpressing both CXCR4 and TRAIL, which were characterized by TEM and Western blotting. These exosomes exerted activity as a cooperative agent with carboplatin against brain metastasis of breast cancer in vivo. This novel application of CXCR4/TRAIL-enriched exosomes in improving the efficacy of chemotherapy highlights a new perspective for establishing a synergistic protocol with anticancer agents to treat brain disease. Furthermore, CXCR4-mediated targeting and TRAIL-mediated apoptosis induction in tumor cells suggests that ExoCXCR4+TRAIL may be used as an optional therapeutic tool, potentially providing a novel approach for advancing the treatment of brain metastases of breast cancer with anticancer agents. In addition, it is important to determine the effect of ExoCXCR4+TRAIL on BBB and in apoptosis induction in brain tumors. Furthermore, it is necessary to conduct a safety evaluation of the vector in animals during treatment.
Data Availability Statement
The original contributions presented in the study are included in the article/supplementary material, further inquiries can be directed to the corresponding author/s.
Ethics Statement
The animal study and experiments were reviewed and approved by the Animal Ethics Committee of Zhejiang University. All animal procedures were performed in accordance with Health Guidelines for the Care and Use of Laboratory Animals of Zhejiang University.
Author Contributions
ML and GC: conceptualization and validation. YH: methodology, resources, writing—review and editing, and project administration. ML: software, formal analysis, data curation, writing—original draft preparation, and visualization. GC: investigation. YH and GC: supervision. YH and ML: funding acquisition. All authors have read and agreed to the published version of the manuscript.
Funding
This work was financially supported by the National Natural Science Foundation of China (81472818), the Fundamental Research Funds for the Central Universities (YH), the Xinglin Young Talent Program of Shanghai University of Traditional Chinese Medicine (ML), the Zhejiang Provincial Natural Science Foundation, the Public Welfare Research Project of Zhejiang Province (LGF19H140002), a startup grant from Zhejiang SCI-TECH University (11612932618290), and the China Postdoctoral Science Foundation (2019M662032).
Conflict of Interest
The authors declare that the research was conducted in the absence of any commercial or financial relationships that could be construed as a potential conflict of interest.
Acknowledgments
We greatly appreciate Dr. Weiren Dong (College of Animal Science, Zhejiang University) for his technical assistance in the isolation of exosomes.
References
1. Li J, Cai P, Shalviri A, Henderson JT, He C, Foltz WD, et al. A multifunctional polymeric nanotheranostic system delivers doxorubicin and imaging agents across the blood-brain barrier targeting brain metastases of breast cancer. ACS Nano. (2014) 8:9925–40. doi: 10.1021/nn501069c
2. Murrell J, Board R. The use of systemic therapies for the treatment of brain metastases in metastatic melanoma: opportunities and unanswered questions. Cancer Treat Rev. (2013) 39:833–8. doi: 10.1016/j.ctrv.2013.06.004
3. Pardridge WM. Drug and gene targeting to the brain with molecular Trojan horses. Nature reviews Drug Discover. (2002) 1:131–9. doi: 10.1038/nrd725
4. Wong HL, Wu XY, Bendayan R. Nanotechnological advances for the delivery of CNS therapeutics. Adv Drug Delivery Rev. (2012) 64:686–700. doi: 10.1016/j.addr.2011.10.007
5. Pardridge WM. The blood-brain barrier: bottleneck in brain drug development. NeuroRx. (2005) 2:3–14. doi: 10.1602/neurorx.2.1.3
6. Peng Q, Zhang S, Yang Q, Zhang T, Wei XQ, Jiang L, et al. Preformed albumin corona, a protective coating for nanoparticles based drug delivery system. Biomaterials. (2013) 34:8521–30. doi: 10.1016/j.biomaterials.2013.07.102
7. Veronese FM, Caliceti P, Schiavon O, Sergi M. Polyethylene glycol-superoxide dismutase, a conjugate in search of exploitation. Adv Drug Delivery Rev. (2002) 54:587–606. doi: 10.1016/S0169-409X(02)00029-7
8. Yoshida K, Burton GF, McKinney JS, Young H, Ellis EF. Brain and tissue distribution of polyethylene glycol-conjugated superoxide dismutase in rats. Stroke. (1992) 23:865–9. doi: 10.1161/01.STR.23.6.865
9. Basso M, Bonetto V. Extracellular vesicles and a novel form of communication in the brain. Front Neurosci. (2016) 10:127. doi: 10.3389/fnins.2016.00127
10. Rajendran L, Bali J, Barr MM, Court FA, Kramer-Albers EM, Picou F, et al. Emerging roles of extracellular vesicles in the nervous system. J Neurosci. (2014) 34:15482–9. doi: 10.1523/JNEUROSCI.3258-14.2014
11. Mathivanan S, Ji H, Simpson RJ. Exosomes: extracellular organelles important in intercellular communication. J Proteomics. (2010) 73:1907–20. doi: 10.1016/j.jprot.2010.06.006
12. Tan A, Rajadas J, Seifalian AM. Exosomes as nano-theranostic delivery platforms for gene therapy. Adv Drug Delivery Rev. (2013) 65:357–67. doi: 10.1016/j.addr.2012.06.014
13. Vlassov AV, Magdaleno S, Setterquist R, Conrad R. Exosomes: current knowledge of their composition, biological functions, and diagnostic and therapeutic potentials. Biochimica et Biophys Acta. (2012) 1820:940–8. doi: 10.1016/j.bbagen.2012.03.017
14. Lai RC, Yeo RW, Tan KH, Lim SK. Exosomes for drug delivery - a novel application for the mesenchymal stem cell. Biotechnol Adv. (2013) 31:543–51. doi: 10.1016/j.biotechadv.2012.08.008
15. Yeo RW, Lai RC, Zhang B, Tan SS, Yin Y, Teh BJ, et al. Mesenchymal stem cell: an efficient mass producer of exosomes for drug delivery. Adv Drug Delivery Rev. (2013) 65:336–41. doi: 10.1016/j.addr.2012.07.001
17. Bowie MB, McKnight KD, Kent DG, McCaffrey L, Hoodless PA, Eaves CJ. Hematopoietic stem cells proliferate until after birth and show a reversible phase-specific engraftment defect. J Clin Invest. (2006) 116:2808–16. doi: 10.1172/JCI28310
18. Wojakowski W, Tendera M, Michalowska A, Majka M, Kucia M, Maslankiewicz K, et al. Mobilization of CD34/CXCR4+, CD34/CD117+, c-met+ stem cells, and mononuclear cells expressing early cardiac, muscle, and endothelial markers into peripheral blood in patients with acute myocardial infarction. Circulation. (2004) 110:3213–20. doi: 10.1161/01.CIR.0000147609.39780.02
19. Walczak H, Miller RE, Ariail K, Gliniak B, Griffith TS, Kubin M, et al. Tumoricidal activity of tumor necrosis factor-related apoptosis-inducing ligand in vivo. Nat Med. (1999) 5:157–63. doi: 10.1038/5517
20. Mizutani Y, Nakao M, Ogawa O, Yoshida O, Bonavida B, Miki T. Enhanced sensitivity of bladder cancer cells to tumor necrosis factor related apoptosis inducing ligand mediated apoptosis by cisplatin and carboplatin. J Urol. (2001) 165:263–70. doi: 10.1097/00005392-200101000-00076
21. Han SJ, Ahn TK, Choi HS, Shin JN, Piya S, Kim TH. TRAIL-induced cell death and caspase-8 activation are inhibited by cisplatin but not carboplatin. J Gynecol Oncol. (2009) 20:113–6. doi: 10.3802/jgo.2009.20.2.113
22. Leong S, Cohen RB, Gustafson DL, Langer CJ, Camidge DR, Padavic K, et al. Mapatumumab, an antibody targeting TRAIL-R1, in combination with paclitaxel and carboplatin in patients with advanced solid malignancies: results of a phase I and pharmacokinetic study. J Clin Oncol. (2009) 27:4413–21. doi: 10.1200/JCO.2008.21.7422
23. Liu M, Zhang L, Zhao Q, Jiang X, Wu L, Hu Y. Lower-molecular-weight chitosan-treated polyethyleneimine: a practical strategy for gene delivery to mesenchymal stem cells. Cell Physiol Biochem. (2018) 50:1255–69. doi: 10.1159/000494585
24. Yang WH, Yang C, Xue YQ, Lu T, Reiser J, Zhao LR, et al. Regulated expression of lentivirus-mediated GDNF in human bone marrow-derived mesenchymal stem cells and its neuroprotection on dopaminergic cells in vitro. PloS ONE. (2013) 8:e64389. doi: 10.1371/journal.pone.0064389
25. Wenger T, Mattern J, Penzel R, Gassler N, Haas TL, Sprick MR, et al. Specific resistance upon lentiviral TRAIL transfer by intracellular retention of TRAIL receptors. Cell Death Different. (2006) 13:1740–51. doi: 10.1038/sj.cdd.4401867
26. Naldini L, Blomer U, Gage FH, Trono D, Verma IM. Efficient transfer, integration, and sustained long-term expression of the transgene in adult rat brains injected with a lentiviral vector. Proc Natl Acad Sci USA. (1996) 93:11382–8. doi: 10.1073/pnas.93.21.11382
27. Zufferey R, Dull T, Mandel RJ, Bukovsky A, Quiroz D, Naldini L, et al. Self-inactivating lentivirus vector for safe and efficient in vivo gene delivery. J Virol. (1998) 72:9873–80. doi: 10.1128/JVI.72.12.9873-9880.1998
28. Liu L, Jin X, Hu CF, Li R, Zhou Z, Shen CX. Exosomes derived from mesenchymal stem cells rescue myocardial ischaemia/reperfusion injury by inducing cardiomyocyte autophagy via AMPK and Akt pathways. Cell Physiol Biochem. (2017) 43:52–68. doi: 10.1159/000480317
29. On NH, Mitchell R, Savant SD, Bachmeier CJ, Hatch GM, Miller DW. Examination of blood-brain barrier (BBB) integrity in a mouse brain tumor model. J Neurooncol. (2013) 111:133–43. doi: 10.1007/s11060-012-1006-1
30. Thery C, Ostrowski M, Segura E. Membrane vesicles as conveyors of immune responses. Nat Rev Immunol. (2009) 9:581–93. doi: 10.1038/nri2567
31. Sahoo S, Klychko E, Thorne T, Misener S, Schultz KM, Millay M, et al. Exosomes from human CD34(+) stem cells mediate their proangiogenic paracrine activity. Circ Res. (2011) 109:724–8. doi: 10.1161/CIRCRESAHA.111.253286
32. Zhang HG, Grizzle WE. Exosomes: a novel pathway of local and distant intercellular communication that facilitates the growth and metastasis of neoplastic lesions. Am J Pathol. (2014) 184:28–41. doi: 10.1016/j.ajpath.2013.09.027
33. Farini A, Sitzia C, Erratico S, Meregalli M, Torrente Y. Clinical applications of mesenchymal stem cells in chronic diseases. Stem Cell Int. (2014) 2014:306573. doi: 10.1155/2014/306573
34. Karnoub AE, Dash AB, Vo AP, Sullivan A, Brooks MW, Bell GW, et al. Mesenchymal stem cells within tumour stroma promote breast cancer metastasis. Nature. (2007) 449:557–63. doi: 10.1038/nature06188
35. Xu C, Lin L, Cao G, Chen Q, Shou P, Huang Y, et al. Interferon-alpha-secreting mesenchymal stem cells exert potent antitumor effect in vivo. Oncogene. (2014) 33:5047–52. doi: 10.1038/onc.2013.458
36. Ridge SM, Sullivan FJ, Glynn SA. Mesenchymal stem cells: key players in cancer progression. Mol Cancer. (2017) 16:31. doi: 10.1186/s12943-017-0597-8
37. Allavena P, Sica A, Garlanda C, Mantovani A. The Yin-Yang of tumor-associated macrophages in neoplastic progression and immune surveillance. Immunol Rev. (2008) 222:155–61. doi: 10.1111/j.1600-065X.2008.00607.x
38. Chen HW, Chen HY, Wang LT, Wang FH, Fang LW, Lai HY, et al. Mesenchymal stem cells tune the development of monocyte-derived dendritic cells toward a myeloid-derived suppressive phenotype through growth-regulated oncogene chemokines. J Immunol. (2013) 190:5065–77. doi: 10.4049/jimmunol.1202775
39. Waterman RS, Henkle SL, Betancourt AM. Mesenchymal stem cell 1 (MSC1)-based therapy attenuates tumor growth whereas MSC2-treatment promotes tumor growth and metastasis. PLoS ONE. (2012) 7:e45590. doi: 10.1371/journal.pone.0045590
40. Hu YL, Huang B, Zhang TY, Miao PH, Tang GP, Tabata Y, et al. Mesenchymal stem cells as a novel carrier for targeted delivery of gene in cancer therapy based on nonviral transfection. Mol Pharm. (2012) 9:2698–709. doi: 10.1021/mp300254s
41. Nakamizo A, Marini F, Amano T, Khan A, Studeny M, Gumin J, et al. Human bone marrow-derived mesenchymal stem cells in the treatment of gliomas. Cancer Res. (2005) 65:3307–18. doi: 10.1158/0008-5472.CAN-04-1874
42. Kordelas L, Rebmann V, Ludwig AK, Radtke S, Ruesing J, Doeppner TR, et al. MSC-derived exosomes: a novel tool to treat therapy-refractory graft-versus-host disease. Leukemia. (2014) 28:970–3. doi: 10.1038/leu.2014.41
43. Xin H, Li Y, Buller B, Katakowski M, Zhang Y, Wang X, et al. Exosome-mediated transfer of miR-133b from multipotent mesenchymal stromal cells to neural cells contributes to neurite outgrowth. Stem Cells. (2012) 30:1556–64. doi: 10.1002/stem.1129
44. Zhang HC, Liu XB, Huang S, Bi XY, Wang HX, Xie LX, et al. Microvesicles derived from human umbilical cord mesenchymal stem cells stimulated by hypoxia promote angiogenesis both in vitro and in vivo. Stem Cells Develop. (2012) 21:3289–97. doi: 10.1089/scd.2012.0095
45. Lai RC, Arslan F, Lee MM, Sze NS, Choo A, Chen TS, et al. Exosome secreted by MSC reduces myocardial ischemia/reperfusion injury. Stem Cell Res. (2010) 4:214–22. doi: 10.1016/j.scr.2009.12.003
46. Zhou Y, Xu H, Xu W, Wang B, Wu H, Tao Y, et al. Exosomes released by human umbilical cord mesenchymal stem cells protect against cisplatin-induced renal oxidative stress and apoptosis in vivo and in vitro. Stem Cell Res Ther. (2013) 4:34. doi: 10.1186/scrt194
47. Kim HS, Choi DY, Yun SJ, Choi SM, Kang JW, Jung JW, et al. Proteomic analysis of microvesicles derived from human mesenchymal stem cells. J Proteome Res. (2012) 11:839–49. doi: 10.1021/pr200682z
48. Hwang BW, Kim SJ, Park KM, Kim H, Yeom J, Yang JA, et al. Genetically engineered mesenchymal stem cell therapy using self-assembling supramolecular hydrogels. J Control Release. (2015) 220:119–29. doi: 10.1016/j.jconrel.2015.10.034
49. Martin F, Tristan-Manzano M, Maldonado-Perez N, Sanchez-Hernandez S, Benabdellah K, Cobo M. Stable genetic modification of mesenchymal stromal cells using lentiviral vectors. Methods Mol Biol. (2019) 1937:267–80. doi: 10.1007/978-1-4939-9065-8_17
50. Younes A, Kadin ME. Emerging applications of the tumor necrosis factor family of ligands and receptors in cancer therapy. J Clin Oncol. (2003) 21:3526–34. doi: 10.1200/JCO.2003.09.037
51. Lawrence D, Shahrokh Z, Marsters S, Achilles K, Shih D, Mounho B, et al. Differential hepatocyte toxicity of recombinant Apo2L/TRAIL versions. Nat Med. (2001) 7:383–5. doi: 10.1038/86397
52. Poh TW, Huang S, Hirpara JL, Pervaiz S. LY303511 amplifies TRAIL-induced apoptosis in tumor cells by enhancing DR5 oligomerization, DISC assembly, and mitochondrial permeabilization. Cell Death Differ. (2007) 14:1813–25. doi: 10.1038/sj.cdd.4402177
53. Miao L, Yi P, Wang Y, Wu M. Etoposide upregulates Bax-enhancing tumour necrosis factor-related apoptosis inducing ligand-mediated apoptosis in the human hepatocellular carcinoma cell line QGY-7703. Eur J Biochem. (2003) 270:2721–31. doi: 10.1046/j.1432-1033.2003.03639.x
54. Menoret E, Gomez-Bougie P, Geffroy-Luseau A, Daniels S, Moreau P, Le Gouill S, et al. Mcl-1L cleavage is involved in TRAIL-R1- and TRAIL-R2-mediated apoptosis induced by HGS-ETR1 and HGS-ETR2 human mAbs in myeloma cells. Blood. (2006) 108:1346–52. doi: 10.1182/blood-2005-12-007971
Keywords: brain metastasis of breast cancer, CXCR4, TRAIL, exosome, MSCs, lentiviral vector
Citation: Liu M, Hu Y and Chen G (2020) The Antitumor Effect of Gene-Engineered Exosomes in the Treatment of Brain Metastasis of Breast Cancer. Front. Oncol. 10:1453. doi: 10.3389/fonc.2020.01453
Received: 16 April 2020; Accepted: 08 July 2020;
Published: 30 July 2020.
Edited by:
Shiv K. Gupta, Mayo Clinic, United StatesReviewed by:
Ji Hoon Phi, Seoul National University Children's Hospital, South KoreaLuz Milbeth Cumba Garcia, Mayo Clinic Graduate School of Biomedical Sciences, United States
Copyright © 2020 Liu, Hu and Chen. This is an open-access article distributed under the terms of the Creative Commons Attribution License (CC BY). The use, distribution or reproduction in other forums is permitted, provided the original author(s) and the copyright owner(s) are credited and that the original publication in this journal is cited, in accordance with accepted academic practice. No use, distribution or reproduction is permitted which does not comply with these terms.
*Correspondence: Yulan Hu, hu_yulan@zju.edu.cn; Guiqian Chen, gqchen@zstu.edu.cn