Biotechnological Processes Simulating the Natural Fermentation Process of Bee Bread and Therapeutic Properties—An Overview
- 1Institute of Life Sciences, University of Agricultural Sciences and Veterinary Medicine Cluj-Napoca, Cluj-Napoca, Romania
- 2Faculty of Food Science and Technology, University of Agricultural Sciences and Veterinary Medicine Cluj-Napoca, Cluj-Napoca, Romania
- 3Advanced Horticultural Research Institute of Transylvania, University of Agricultural Sciences and Veterinary Medicine Cluj-Napoca, Cluj-Napoca, Romania
Recent signs of progress in functional foods and nutraceuticals highlighted the favorable impact of bioactive molecules on human health and longevity. As an outcome of the fermentation process, an increasing interest is developed in bee products. Bee bread (BB) is a different product intended for humans and bees, resulting from bee pollen's lactic fermentation in the honeycombs, abundant in polyphenols, nutrients (vitamins and proteins), fatty acids, and minerals. BB conservation is correlated to bacteria metabolites, mainly created by Pseudomonas spp., Lactobacillus spp., and Saccharomyces spp., which give lactic acid bacteria the ability to outperform other microbial groups. Because of enzymatic transformations, the fermentation process increases the content of new compounds. After the fermentation process is finalized, the meaningful content of lactic acid and several metabolites prevent the damage caused by various pathogens that could influence the quality of BB. Over the last few years, there has been an increase in bee pollen fermentation processes to unconventional dietary and functional supplements. The use of the chosen starters improves the bioavailability and digestibility of bioactive substances naturally found in bee pollen. As a consequence of enzymatic changes, the fermentation process enhances BB components and preserves them against loss of characteristics. In this aspect, the present review describes the current biotechnological advancements in the development of BB rich in beneficial components derived from bee pollen fermentation and its use as a food supplement and probiotic product with increased shelf life and multiple health benefits.
Introduction
Nowadays, the concept of “food as medicine and medicine as food” assimilated to Hippocrates is increasingly common in the food sector, where the production of functional foods is a significant part of human lifestyle (1). All over the world, consumers have extensive knowledge regarding the effect of food on wellbeing and having positive food expectations (2).
Bee products have been used to treat and prevent disorders such as burns, wounds, diabetic foot ulcers, allergic rhinitis, hyperlipidemia, and rheumatoid arthritis through the history of the traditional medicine (3–6). Recently, bee products developed due to the fermentation process have gained colossal interest and became a severe topic for future research due to the positive impact on the food market.
Bee bread (BB) is a fermented assortment of bee pollen (BP), nectar, and bee saliva, having a caramel-like color and a sharp taste given by flowers, citrus, or other fruit flavors (7). The outcome of lactic acid fermentation of BP collected from flowers by bees and combined with their digestive enzymes is the primary food for larvae and young bees in the hive (8). In the cells of the honeycombs, bees pack the components and then secure the mixture with honey and wax, protecting the pollen mass from oxygen, starting an anaerobic lactic fermentation process, which after approximately seven days generates BB (9).
According to Habryka et al. BB incorporates a well-balanced nutritional content and more prosperous chemical composition than BP, having a significant assimilation rate and a better absorption at the level of the human body (10). Since the BB components are fermented in part, the high BP content is more straightforward incorporated and utilized as the walls have partly deteriorated through the lactic fermentation (9). The studies on its chemical composition demonstrated that BB is a trustworthy source of proteins, vitamins (B, C, E, K, and P), and polyphenols, like quercetin, kaempferol, apigenin, naringenin, chrysin, caffeic, gallic and ferulic acids (11). Besides, free amino acids, carbohydrates, and fatty acids are essential elements of BB composition, which depend on environmental conditions, seasonal discrepancy and differ from region to region, based on the melliferous plant's (12).
The recent studies on BB chemical composition established that it has a higher nutritional value than BP; nevertheless, research on BB is scarce and focused only on its chemical composition (13, 14). Because collecting BB from the hive is too costly and time-consuming, BB purchases for human consumption are limited; moreover, beekeepers feel more comfortable extracting BP using traps. Unfortunately, due to this method, there is a danger of harmful mold growth due to the high humidity. Furthermore, storage treatments are required to avoid spoiling (15–17). The traditional methods used have several disadvantages; the nutritional value is affected, and the operating cost is high; therefore, alternative opportunities should be considered to overcome these impediments. A unique opportunity is the biotechnological fermentation of BP, simulating the natural process in the hive leading to a high nutritional product, as in BB (18).
Fermentation is one of the most widely used methods in the production and economic preservation of food, being perceived as an essential constituent of the nutritional culture of every world's society supporting the cultural history of ethnic communities (19). Globally, a multifariousness of fermented products is widely consumed as daily human food, from yogurt, kefir, sausages to pickles and fermented cereals, thanks to their biological functions and enrichment of nutritional value. In the beehive, the natural fermentation improves the bioavailability of fresh BP and the possibility of long-time storage, avoiding losing its nutritional value as it is converted to BB (20). Because of its beneficial components, BP represents a valuable raw material that allows the microorganisms' development during the fermentation process (21). In the past years, researchers attempted to induce the natural fermentation of BP at a lab scale by inoculating diverse microorganisms under specific process conditions.
The purpose of the review was to present an overview of the biotechnological processes used to obtain BB from harvested BP in laboratory conditions by an assortment of chosen starters reproducing the microbial consortium implicated in the fermentation of BB. Changes in the bioactive compounds and antioxidant activity are also pointed out along with the final's probiotic product shelf life and health benefits. Furthermore, the effectiveness of BB and strains isolated or found in BB are investigated in the prevention and treatment of several anti-cancer agent-induced toxicities in animal models and patients with cancer.
Similarities and Differences Between Bee Pollen and Bee Bread
Because of their nutritional and medicinal properties, natural products such as unique bee brands have piqued the curiosity of academics in recent years. Even though these have been well-known products for millennia, they have only lately become the subject of recorded scientific investigation (2).
BP is recognized as the oldest nutritive supplement in history and includes roughly all of the dietary nutrition compounds, the main ingredient of BB (14). BP is the male gametophyte of flowering plants. After gathering pollen grains from flowers, bees combine their saliva and secretions. This procedure enables BP to be hydrated and pelleted, which subsequently sticks to the pollen basket on the bees' rear leg and carries to hive. BP is a source of nutrients that honeybees need to grow and develop appropriately throughout their larval stage until maturity (22, 23). BP is deposited into honeycomb cells by bees, which seal them with honey and wax. Collected and stored, BP is exposed to lactic fermentation under the beehive conditions, resulting in BB, which is fermented BP (2).
BP and BB are used for apitherapeutic benefits as they are rich in vitamins, valuable bio-elements, and nutrients. Still nevertheless, the two components differ from a biochemical point of view (Table 1) (25).
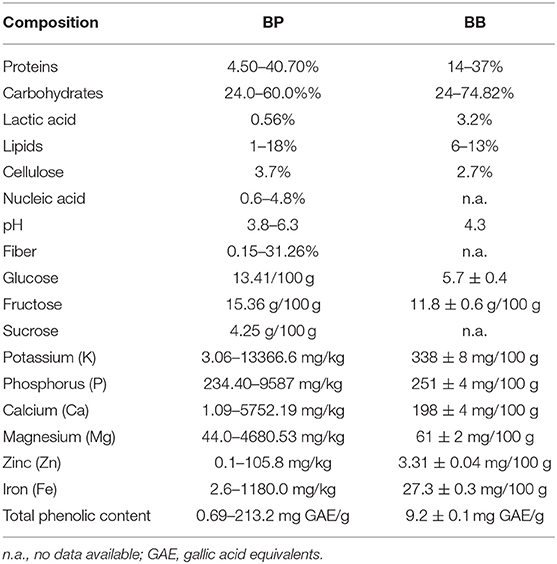
Table 1. Chemical and nutritious compounds of BP and BB adapted after Kieliszek et al. and Bakour et al. (2, 14, 24).
The nutritional composition of BB varies based on the pollen's local and seasonal value and availability to different plant species. Compared to BP, BB is high in reducing sugars and has significant amounts of tocopherols (26, 27). BB has low protein and fat content but increased carbohydrate and lactic acid content. Due to its structure, it possesses increased bioavailability, which can be partly assimilated by fermentation and demonstrates high resistance against the degradation action of digestive media. Furthermore, the functional and actively rich components of BP are easily absorbed and utilized (Figure 1) (2, 28).
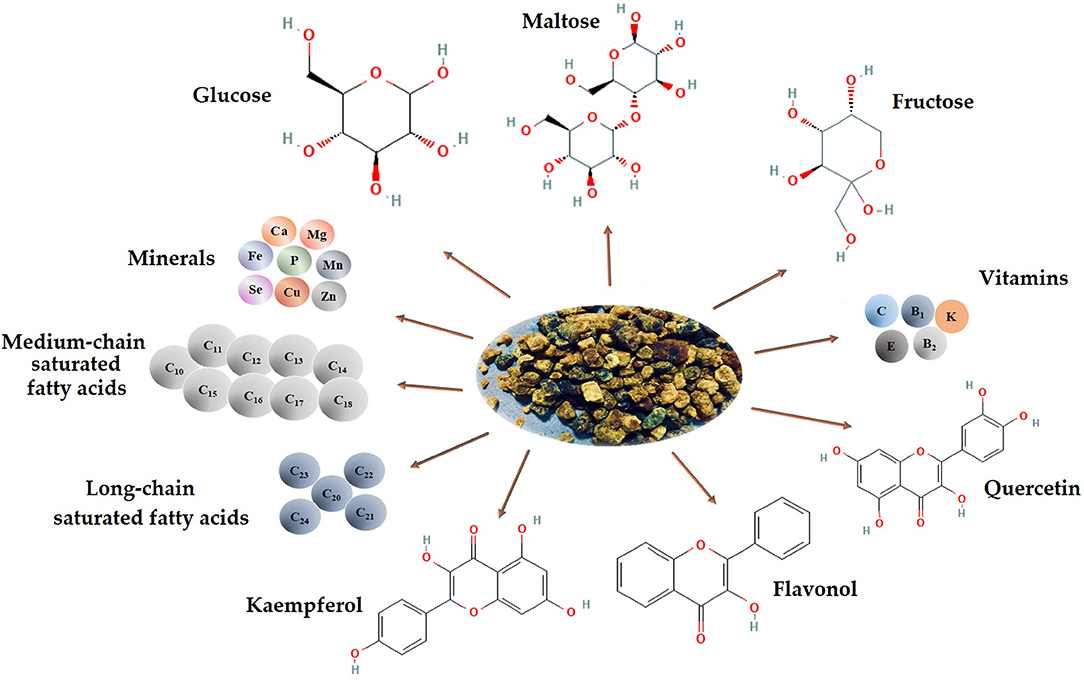
Figure 1. Chemical composition and bioactive compounds of BB (image created using BioRender and King Draw applications).
BB is more digestible than BP due to the breakdown of the multilayer wall during fermentation. Moreover, this process enhances BB bioavailability, resulting in higher absorption by human intestinal epithelial cells (3, 18). Also, during fermentation, bacteria break down cellulose, which constitutes the internal coating (also known as intine) of BP, lowering BB's cellulose ratio in contrast to BP (22). Furthermore, throughout the fermentation process, certain innovative products are deliberated. For instance, several proteins in BP are converted to amino acids by digestive enzymes, increasing the protein level, whereas, in BB, an increasing content in amino acids is noticed (2). According to DeGrandi-Hoffman et al., the content of threonine and leucine in BB is approximately 60% higher than BP (29). As a primary food source for bees, BP includes amino acids such as leucine, isoleucine, histidine, lysine, valine, arginine, phenylalanine, methionine threonine, and tryptophan (30). According to Bayram et al., in comparison to BP, BB samples had considerably less L-asparagine (5891.1–2475.4 μg/g), but L-proline (22212.8–4939.2 μg/g), L-aspartic acid (5207.37–2833.3 μg/g), and GABA (4588.4–2703.2 μg/g) were detected as significant amino acids. Furthermore, considerable amounts of L-phenylalanine were found in BP and BB samples, with values ranging from 3353.8–1298.9 μg/g to 3345.6–1308.4 μg/g, respectively (30). The lower content in L-asparagine in BB may be due to the fermentation development and acidic environment that cause this amino acid field's deamination (29).
In 2008, Venskutonis et al. investigated the fatty acid content of BB in summer and spring and discovered 22 fatty acids, as well as five ω-3, four ω-6, and three ω-9 polyunsaturated fatty acids (PUFAs). The primary fatty acids found in BB were arachidonic and oleic acids, with α-linolenic concentrations varying the most between the botanical origins of samples. There was also a substantial difference in the α-linolenic and eicosapentaenoic acids (31).
BP has a high concentration of polyphenolic chemicals, primarily flavonoids and phenolic acids (32). Flavonoids in BP vary from 3.7 to 10.1 mg/g, according to Pascoal et al. (33). At the same time, the total flavonoid content of five BB samples showed values ranging between 13.56 and 18.24 g QE/g DW (quercetin equivalents/g dry weight) (34). Also, in 2015 Zuluaga et al. established that BB originated from Colombia has a total flavonoid content between 1.9 and 4.5 mg QE/g (18).
Vitamins are a diverse group of active ingredients required for the optimal health and growth of all organisms. BB is characterized by higher levels of phenolics with 4.87 mg GAE/g, flavonoids with 59.06 mg QE/g, vitamin C (0.36 ng/g), and E (32.55 ng/g) (35). In addition, K, P, Ca, Mg, Zn, Fe, and Mn (Manganese) levels in spring BB collected from honey bee colonies were also tested at the Institute for Forage Crops, the results demonstrating a strong relationship between the mineral content and the sources of floral pollen (25). Adequate amounts of macro-and microelements in the human organism are critical for the effective operation of many distinct metabolic processes. Minerals are required for appropriate physiological functions and metabolic pathways regulation (36).
BB includes a variety of enzymes, as well as acid leucine aminopeptidase, phosphatase, and glucosidase, which hydrolyze carbohydrates such as amygdalin, salicin, cellobiose, and centipoise (3). The BB fermentation technique results in higher amounts of lactic acid due to microbial metabolism, which provides long-term defense against pathogens while also increasing the nutritious qualities of BB (3).
In summary, BB includes a wide range of nutritional phytochemicals with valuable characteristics, including vitamins, carotenoids, phenolic acids, and, most notably, flavonoids. BB was found to possess antioxidant, antibacterial, antiviral, anti-inflammatory, and anti-cancer benefits because of their complex chemical composition. In recent years, there has been a surge in interest in the chemicals responsible for these effects; hence, taking into account food productions and the beneficial impacts on human wellbeing, these bee products, with enormous production perspectives and usage as natural and valuable components, provide a broad topic of study (37–41).
The growing global interest in functional products and rising health consciousness adds burden to the upcoming production of bee products. BB has the advantage, apart from a distinct chemical profile, the further production of microbial fermentation, which contributes to its nutritional and health properties. BB is quickly absorbed and digested and contains a variety of macro-and micronutrients that are beneficial to the human body, including flavonoids and polyphenols (3). In addition to the well-known bee products, such as BP, honey, and royal jelly, BB might be the potentially exploited gold mine in the culinary sector and medicine development.
BB Natural Fermentation Process
After fermentation of BP, the resulting product (BB) has an amber-like color and a rich aroma of flowers and citrus flavor (7). The digestive enzymes of bees naturally contain lactic acid bacteria (LAB), due to which a lactic fermentation begins, leading to BB (15, 22, 29).
According to the indoor beehive conditions, the process of obtaining BB is based on the progression of the appearance/disappearance of colonizing microorganisms, particularly LAB, under anaerobic conditions (18). Also, through the saliva of bees, enzymes are segregated, causing fermentation and enzymatic processes, biochemical transformations necessary to break the outer layer known as exine, that covers the pollen, consisting of sporopollenin that provides resistance to chemicals and preservation of bioactive substances inside the pollen grain (42). As a result of microbial metabolism and biochemical changes, BP is transformed to BB via lactic acid fermentation generated mainly by bacteria, like Pseudomonas spp., Lactobacilli spp., and yeasts as Saccharomyces spp. (Figure 2).
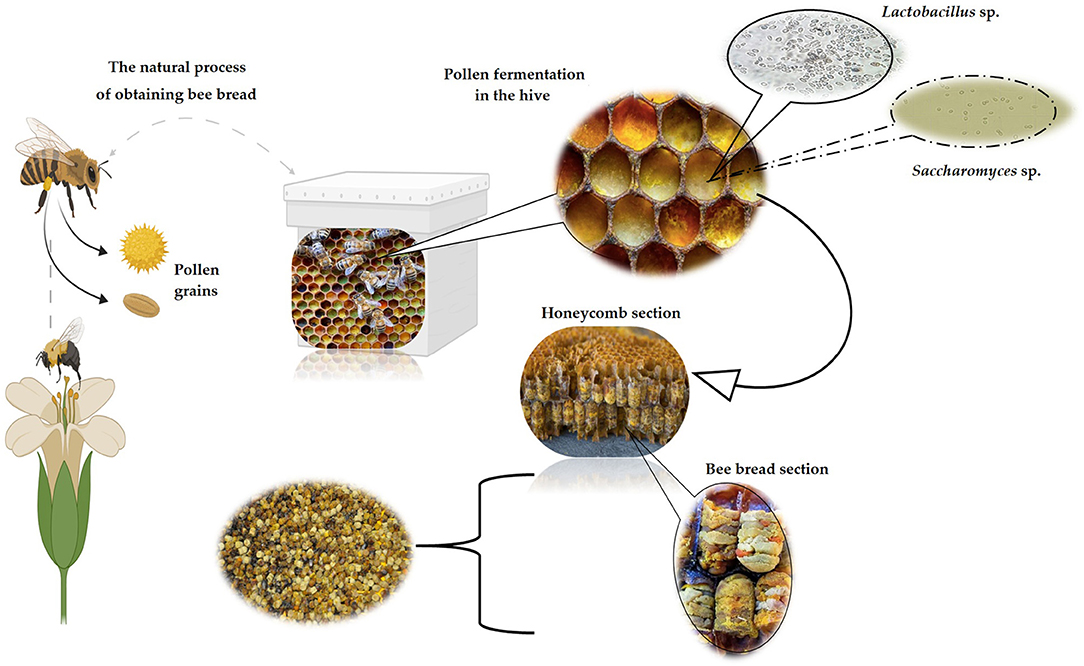
Figure 2. The natural process of producing BB by bees (image created using BioRender and King Draw applications).
In addition, Bacillus species in Melipona panamica nests were shown to secrete enzymes that catalyze the breakdown of lipids, carbohydrates, and proteins. This bacterial genus was found predominant in BP, and some species are known to ferment glucose. Therefore, this suggests that Bacillus species is a significant genus that could be involved in the BB formation (43, 44).
Inside the hive, BP turns into BB in about 7 days, fulfilling several biochemical stages, starting after the development of LAB, indole-producing bacteria (Escherichia spp.), aerobic bacteria, and yeast. In the next step, the anaerobic LAB (Streptococcus spp.) use the nutrients created by bacteria and yeast, causing the pH of BP to decrease. Then, when the concentration of Streptococcus bacteria decreases, Lactobacilli bacteria begin to increase at the end of the 7 days when the LAB and yeasts die. Moreover, due to lactic acid fermentation, the BB reaches a pH of 4.0, becoming microbiologically sterile, all in the last stage (3). Certainly, BB is pollen stored in the hive, which undergoes a fermentation process, most likely due to the glandular secretions of bees and its microbial group, involving mold, yeast, and bacteria, and LAB with a critical role in this process (45). The resulted product is more stable and more nutritious than fresh BP and has higher vitamin content, especially vitamin K because of the pollen degradation (3, 46). Due to the metabolism of microorganisms involved in the fermentation process, the content of LAB increases, resulting in long-term protection against microorganisms and strengthening the nutritional properties of BB (47).
The chemical composition of BB, such as flavor, color, and texture, changes considerably after being stored, and these characteristics vary among bee species. For example, the BB of stingless bee Frieseomelitta and Tetragonisca are dry and sweet, while the ones produced by Melipona and Scaptotrigona are moist and sour (48).
The process of collecting BB from the hive is more laborious than BP, where the traps placed at the hive entrance are sufficient to manage it. Complex techniques like machinery separation or freezing followed by a manual crushing of the combs are required to obtain BB as it is fixed with beeswax and tightly stuffed in the combs by bees (22). Therefore, BB is more costly than other bee products (49).
The current tendency relating to the consumer's behavior and food inclination determined the production of novel products. Therefore, new technologies for their development, challenging companies from several food enterprises to commence the action of organic products. As a result of fermentation, the obtained bee products have become an increasingly studied niche. Solid-state fermentation is a bioprocess naturally produced in the hive when BP is used as the primary nutrient source for microorganisms (21). The advantage of using BP in future processes, like solid-state fermentation, will be discussed in the following section to demonstrate the necessity of obtaining BB via the biotechnological route.
Biotechnological Processes Simulating the Natural Fermentation Process of BB
Nowadays, more and more scientific research proposes using LAB as a noteworthy part of future production chains. The food industries focused on producing value-added products with significant bio-elements, macro and micro-nutrients, vitamins, and health benefits (50). LAB are gram-positive bacteria, non-spore-forming, fermentative, facultative anaerobic, with a significant impact on the food industry (51). Furthermore, LAB has important significance since they fulfill the safety conditions for people and animals (GRAS—-Generally Recognized as Safe). Each has a distinct fermentation metabolism and energy gained due to the saccharides conversion (52).
In consequence, throughout fermentation, in addition to lactic acid, the specific LAB strains produce multiple metabolites like exopolysaccharides, enzymes, diacetyl, hydrogen peroxide, and bactericidal proteins or bacteriocins. These compounds confer the functional properties of LAB, for instance, probiotic and fibrinolytic effects antioxidant activity, in addition to providing fermented products with their remarkable consistency, color, flavor, and aroma (53). LAB has a long history of application in various industrial sectors used as starter cultures. Thus, microbial preparations of many microorganisms are introduced in raw material to develop a fermented product by an accelerated and guided process (54, 55). In recent years, numerous researchers attempted to simulate the natural fermentation of BP via microorganisms' inoculation under various process conditions at the lab scale to obtain nutritional and functional BB (Figure 3).
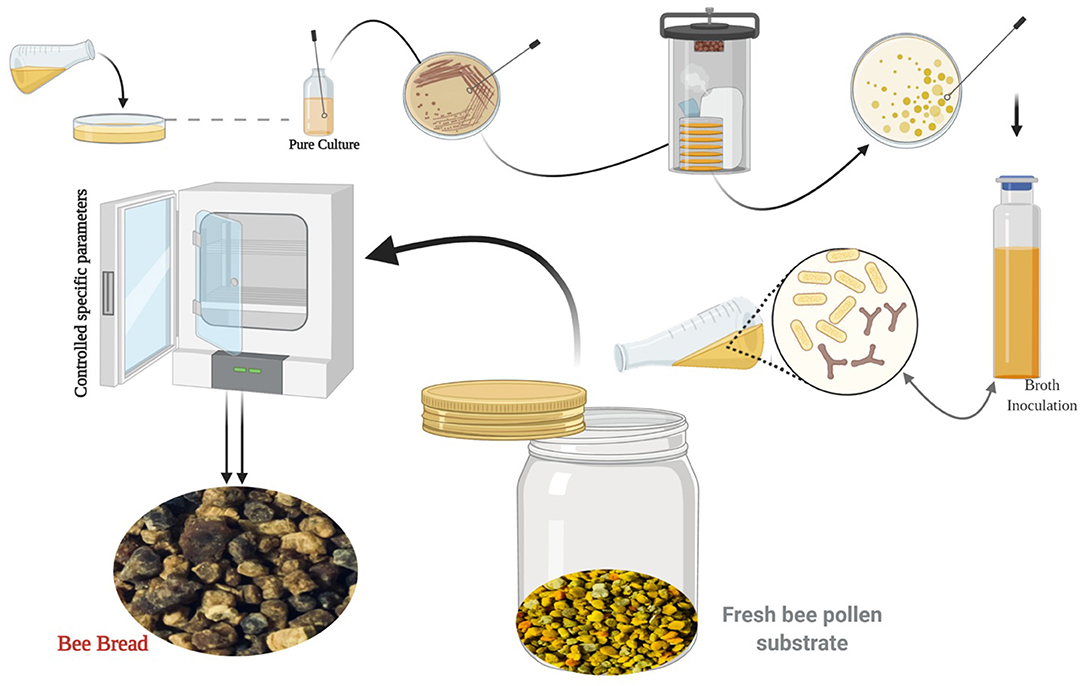
Figure 3. The biotechnological process of simulating the natural fermentation process of BB (image created using BioRender and King Draw applications).
Moreover, other studies have carried out enzymatic hydrolysis and sonication as alternative methods to improve BP nutrient bioavailability (56). Concerning LAB, 45 bacteria species were identified and isolated from honeybees, flowers, and bee products, as shown in Table 2 (87). Studies have shown that bees possess specific microbiota, different from other beings, but closely related to the thread Firmicutes, Actinobacteria, and Proteobacteria, the essential intestinal bacteria (88). A unique LAB group, different from the classic consortium that prefers glucose, has been identified in the stomach of bees, fructophilic lactic acid bacteria (FLAB), which can use fructose from richer sources, for instance, flowers (57). Recently, FLAB was regarded as unconventional LAB, as they have a unique growth characteristic due to a partially bi-functional alcohol/acetaldehyde dehydrogenase encoded gene, generating a disequilibrium in NAD/NADH (nicotinamide adenine dinucleotide/reduced nicotinamide adenine dinucleotide) and the necessity of supplementary acceptors for metabolizing glucose (58). According to recent studies, fructose-feeding insects, like bees, possess in their guts high amounts of FLAB cells belonging to the Fructobacillus and Lactobacilli spp. (59).
Foods fermented with selected Bifidobacterium strains are known to present a variety of probiotics, prophylactic and therapeutic properties (89). Members of the Bifidobacterium genus are found as gut microbiota components. They are thought to play a significant function in sustaining and improving human health by evoking various positive qualities. Bifidobacteria may use a wide variety of dietary carbohydrates, most of them being oligo- and polysaccharides derived from plants, that avoid decomposing in the intestine's upper section (90). Bifidobacteria spp. are Gram-positive, hetero-fermentative microorganisms that do not generate spores (90). The Bifidobacteria pathway consists of a carbohydrate metabolism mechanism found in all Bifidobacterium spp., due to their ability to metabolize different polysaccharides leading to the degradation of those undigested sugars (91). Vamanu et al. (92) used a substrate of prebiotics such as lactulose, inulin, and raffinose with Bifidobacterium bifidum 1 and 2 to evaluate their impact on lactic acid production, cell viability, and antioxidant activity. The BP and honey-based medium supplemented with prebiotics, ground pollen, and inulin provided the best results in viability and total antioxidant activity (92). These findings demonstrate the feasibility of BB functional foods that can be used in both medicine and food sectors (93).
Lactobacilli spp. on the Biotechnological Process and the Use of Probiotics
Lactobacilli are gram-positive, non-spore-forming bacteria that at the end of fermentation release lactic acid as the primary end product of fermentation, which also contributes to the texture and sensory profile of a food (94). Lactobacillus was the most numerous genera within the LAB group belonging to the phylum Firmicutes, class Bacilli, order II Lactobacillales and family Lactobacillaceae. According to the recent reclassification, the Lactobacillus genus has been split into 23 new genera (95). Some of them (for example, Lacticaseibacillus casei and Ligilactobacillus salivarius) provide particularly L (+) lactic acid, while other microorganisms like Lactobacillus bulgaricus and Lactobacillus jensenii generate only D – lactic acid, and microorganisms like Lactobacillus acidophilus and Lactobacillus helveticus can create a combination of D (+) and L– lactic acid. (63). The live microorganism belonging to these genera has recently gained further attention. They are immune to gastrointestinal acidity, improve the intestinal microbiota, and reduce the growth of undesirable bacteria (65). Further, the available studies in the literature describe a biotechnological way to obtain BB by using collected BP as substrate and specific Lactobacilli spp. as starter culture according to their particular growing conditions (Table 3).
The most important mechanism for the fermenting of microorganisms is carbon metabolism, in which carbohydrates are converted into essential compounds such as alcohols, acids, and carbon dioxide as the main end–products (100). To produce lactic acid, L. bulgaricus, and Streptococcus thermophilus consume sugar up to 3.2%, but in an acidic environment, those species become inactive (101). In this aspect, following fermentation, the levels of maltose and turanose decreased. The contents of free and total phenolic compounds increased by 18.3 and 17.8% after fermentation, whereas the content of bound phenolic compounds decreased. Fermentation with various microbial strains was demonstrated to enhance the quality of phenolic compounds in BB attributed to BP's structure (23, 102). Most bioactive compounds can be changed during fermentation due to the microbes' metabolic actions; thus phenolic acids are released under acidic conditions—field (103). Furthermore, peptides play critical roles in enhancing food taste and flavor. Most people cannot eat BP due to its distinctive aroma; however, active–taste peptides may solve this limitation (104).
BP is well-known to be rich in nicotinic, pantothenic acids, and riboflavin, while the riboflavin content of BP is the highest among all plant-based materials. The contents of riboflavin, nicotinamide, nicotinic acid, and amino acids were increased after fermentation in both pollen mediums, emphasizing the fermentation with L. bulgaricus and S. thermophilus can enhance vitamin content (105). In addition, the proteins degrade into smaller molecules through the fermentation process, making digestion and consumption much more accessible. Aside from that, wall-breaking pollen demonstrated more significant advantages in nutrient transformation during the fermentation process (96). In 2019, Di Cagno et al. (45) attempted to reproduce the natural process of BB fermentation by performing an actual solid-state fermentation process with BP as the primary substrate. Furthermore, they established a biotechnological protocol in which the role of LAB is precisely emphasized, and specific parameters, like temperature, pH, use of selected starters, time, influence the quality of the product developed at the fermentation end (Table 3).
Since fermentation is the most cost-effective method to improve the availability of high-quality nutrients for the human body, Knazovická et al. (73) simulated the function of bees with BP using a natural fermentation model analyzed the final product, which they dubbed pollen can. Physico-chemical analyzes of pollen can (BB) showed a 60% increase in water content, a 40% increase in free acidity, and a 17% decrease in pH, along with a reduction of 2% of fat compared to the results of analyzes performed on raw BP. The drop in pH is caused by lactic and acetic acid fermentation and alcoholic fermentation, thus ensuring a defensive role and spoilage avoidance of BB.
In 2019, another study found a potential improvement in the nutrient content of the resulting product through the solid fermentation of BP with LAB (96). The study aimed to obtain a nutritionally improved novel natural food product from BP that can be used as a nutritional supplement or a valuable ingredient in other foods. The LAB produced lactic acid by using the carbohydrates found in BP. During fermentation, the lactic acid content increased slightly after 168 h, with the final level of 6.10%, while in control, it remained unchanged, and the total sugar content decreased by 31.60%. The protein level in BP before fermentation was 26.80 mg/g, which increased by 12.53 mg/g after fermentation. Further details can be seen in Table 3.
Yeast on the Biotechnological Process and Probiotic Usage
Foods have been fermented to enhance their organoleptic and nutritional characteristics from ancient times. In the 20th century, industrial microbiology grew even further when new opportunities for producing a wide range of goods by fermentative processes emerged (90). Recent developments in yeast taxonomy, ecology, biochemistry, genetics, and molecular biology have piqued the interest in their role and importance in beverages and foods. This has led to a better understanding of the fermentation functions of well-known products and their role in the fermentation processes of other substrates (93). During their development in beverages and foods, they absorb nitrogen and carbon substrates and produce a wide range of volatile and non-volatile metabolites that influence the product's chemosensory properties. At the same time, other yeasts create extracellular amylases, proteases, lipases, and pectinases which also affect the aroma and texture of products (106). Yeast enzymatic activity is now critical in the processing of wide varieties of fermented food products; thus through a fermentation process under anaerobic or oxygen rich-conditions, it is likely to obtain ethanol and carbon dioxide (107). Compared to LAB, yeasts are not especially nutritionally demanding, but even so, the presence of simple compounds such as fermentable sugars, vitamins, minerals, amino acids, and oxygen stimulate their growth (108).
During a study, Yan et al. (96) fermented BP with various yeast or yeast mixtures the following fermentation. The overall phenolic compound contents of BP or wall-breaking pollen increased in the next order: fermentation with a microbial combination> fermentation with yeasts > LAB fermentation. The findings indicate that peptides with lower molecular weight significantly increased throughout fermentation. Peptides are either released during protein hydrolysis or formed by microorganisms involved in fermentation (97). A surprising aspect is that the following fermentation with yeasts, peptides with lower molecular weight (<1,000 Da), also known as oligopeptides, carry out a variety of active functions such as blood pressure regulators, anticoagulants, and antioxidants (109).
Yeast fermented BP may contain more carbohydrates, generate oligopeptides, free essential amino acids, PUFAs, and polyphenols than BP fermented by LAB; thus, yeast fermentation is a viable option for improving BP's nutritional properties (29). In this aspect, Zhang et al. (99) investigated the fermentation mechanisms of Canola BP by S. cerevisiae and Ganoderma lucidum to facilitate the breaking of the pollen wall. The pollen wall cannot fully decompose in the human digestive system, and its contents are only released through the germinal aperture, resulting in a nutrient consumption reduction. As a result, deterioration of the pollen wall is needed to maximize the use of nutrients present inside BP (58). On day 1, the Canola BP coating disappeared, the germinal apertures continued to expand with fermentation until the 8th day when the contents were released, and the wall's structure was damaged. G. lucidum broth comprises higher amounts of nutrients compared to S. cerevisiae. The results showed a suitable fermentation method for breaking the pollen wall and releasing nutritional compounds (e.g., polysaccharides, ganoderic acid) (110).
Hanseniaspora uvarum, a unique yeast species that can grow at 1.5 pH (most yeasts grow better at pH 4.5–7.0), along with A. kunkeei, was used by Di Cagno et al. to reproduce the natural process of BB by performing an initial solid-state fermentation process with BP as the main substrate (45). Yeasts were detected at the uppermost cell densities in raw BP throughout their first 20 days of storage and unstarted BP throughout their last 20 days. H. uvarum pectin-degrading enzymes were required to break down the pollen walls, resulting in nutrient release; this hypothesis emphasizes the close interaction between LAB and other microbial groups during BB fermentation (111). This study linked the growth process of BB to the active role of native LAB in collaboration with yeasts. Furthermore, it highlighted the critical role of A. kunkeei and the development of a fermentation protocol for BP that nearly reproduces the natural process of BB fermentation, resulting in a well-constructed and consistent fermented product with a high nutritional value, ideal for human consumption (45). Further details can be seen in Table 3.
The present studies indicate the favorable influence of BP solid-state fermentation on the nutritional content of the resulting product, BB, by boosting the bioavailability and digestibility of nutrients and bioactive substances. Furthermore, since all of the studies listed above have achieved positive features in their field of research, biotechnological BB can be considered a different food with a great source of natural nutrients and a product that is beneficial to human nutrition and health (14, 112).
Potential Therapeutic Properties of LABs Found in BB and/or Used in the Fermentation Process of BP
Probiotics from bee products possess several health benefits, including controlling gastrointestinal infections, improvement in lactose metabolism, anticarcinogenic and antimutagenic properties, cholesterol reduction, immune system stimulation, and improvement in inflammatory bowel disease (Table 4). Probiotics from BB produce bacteriocins and short-chain fatty acids, which help lower gut pH, enhance the available nutrients, colonize the colon with available microorganisms, stimulate mucosal barrier function, and increase immunity (Table 5). Moreover, several studies demonstrated the stimulatory effect of probiotics of the natural and gained immune response by inducing secretory and systemic IgA secretion (135–137).
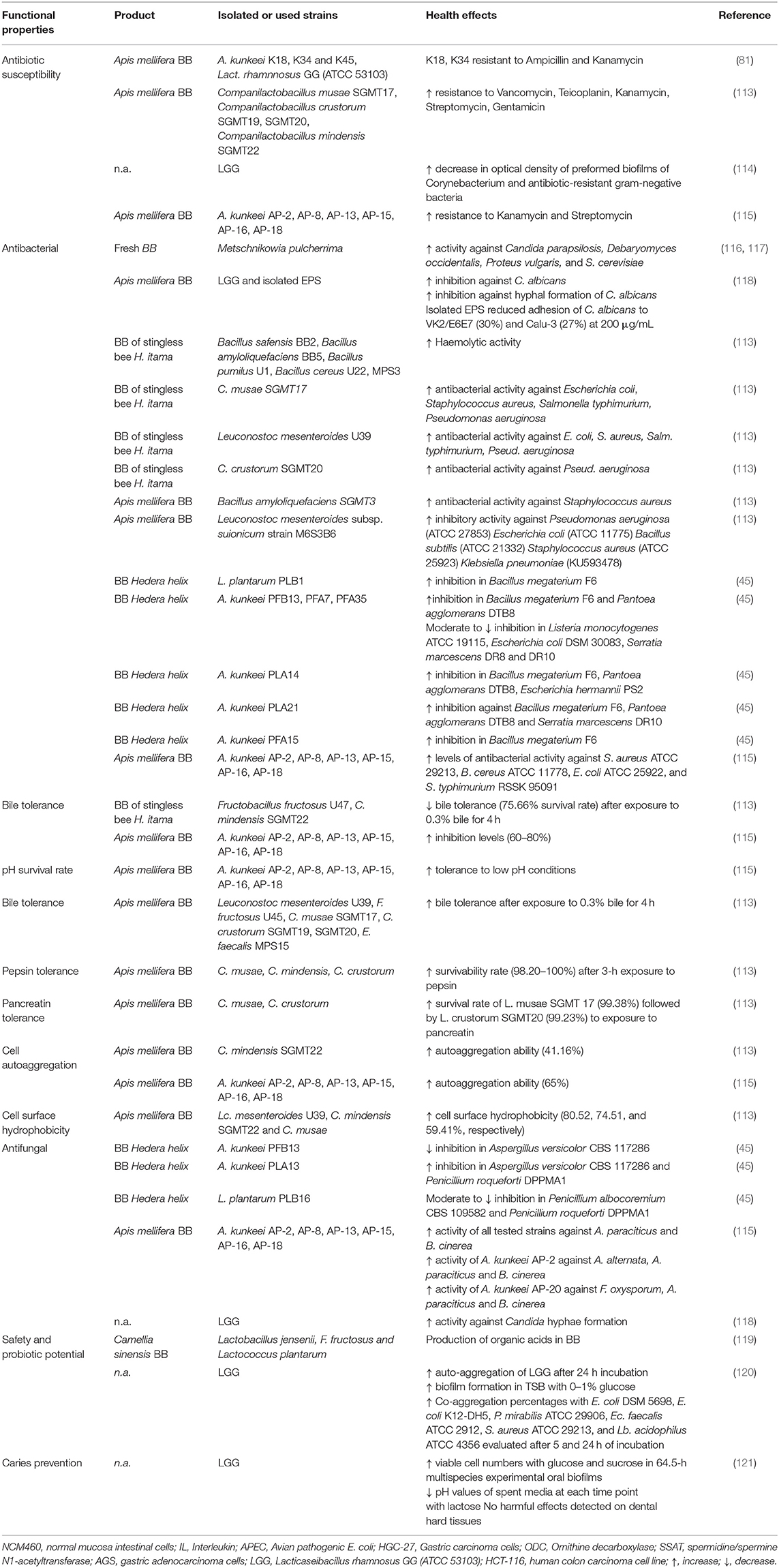
Table 4. Therapeutic properties of probiotic bacterial isolates found in BB and/or used in the fermentation process of BP.
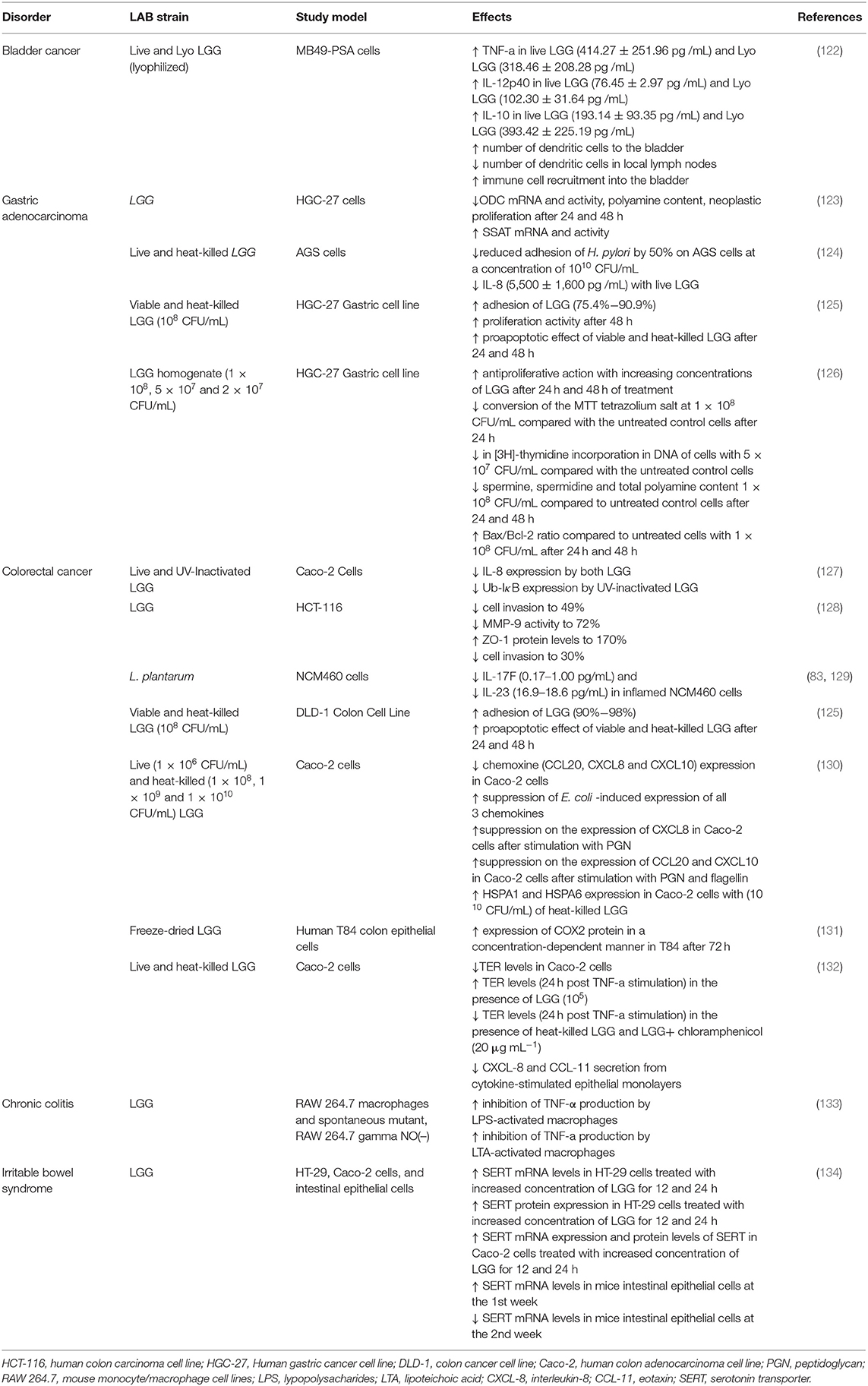
Table 5. In vitro studies regarding the potential therapeutic properties of LABs found in BB and/or used in the fermentation process of BP.
Multiple human and animal studies have been conducted and suggest that probiotics are GRAS and practical for clinical application on human diseases, such as acute pediatric diarrhea (138), rotavirus-related diarrhea (139), infantile colic (140), necrotizing enterocolitis in shallow birth weight infants (141), type 1 diabetes (142), allergic asthma (143) inflammatory bowel disease (144), bone loss (145), and bacterial vaginosis (146), and virus infection (147). Further details can be seen in Table 6.
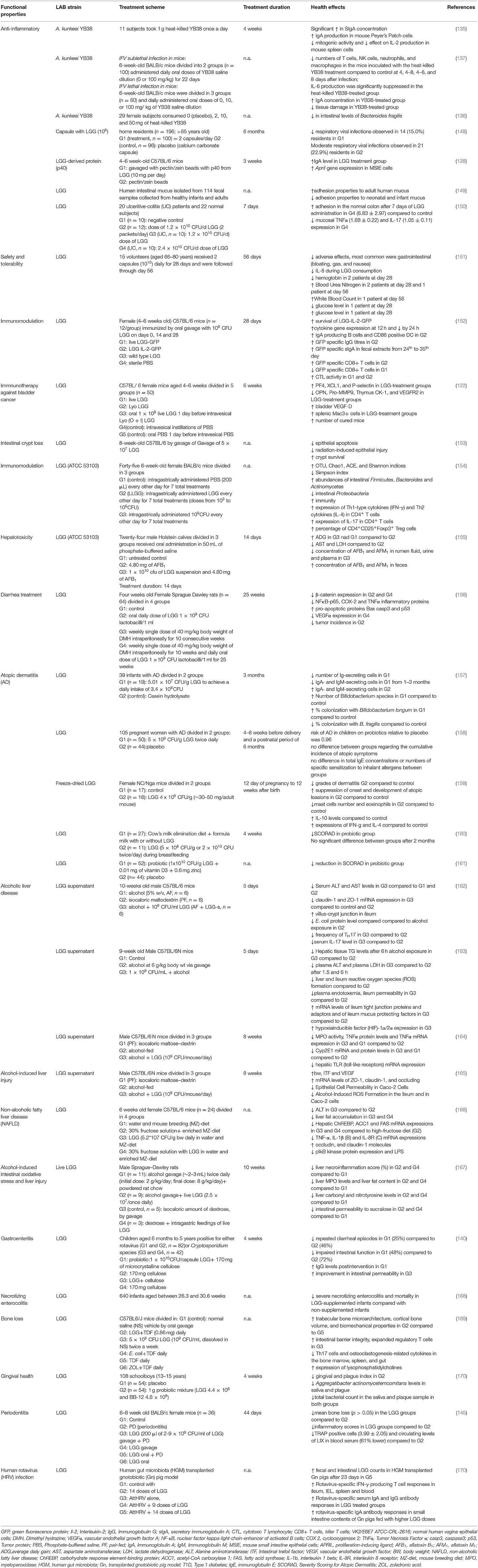
Table 6. In vivo studies regarding the potential therapeutic properties of LABs found in BB and/or used in the fermentation process of BP.
So far, Lacticaseibacillus rhamnosus ATCC 53103 (formerly Lactobacillus rhamnosus LGG) is the most characterized, and studied probiotics, extensively used in the fermentation process of BP with promising results (171). It has multiple health benefits; for instance, it produces a biofilm that acts as a mucosa-protecting agent, enhances the intestinal pit survival through diverse soluble factors beneficial to the gut, diminishes apoptosis of the intestinal epithelium, preserves cytoskeletal strength along with pathogen inhibition, promotes immune awareness by reducing expression of inflammation markers and increasing production of IL-10 (interleukin-10), IL-12 and TNF- α (α-tumor necrosis factor) (172). Thus, the combination of BP fermented with different lactobacilli strains is significant for developing a novel product used both as food and medicine.
Antibiotic Susceptibility
Several reports demonstrated that Lactobacilli strains have antibiotic resistance genes which can be transferred to the host gut of other bacteria. Therefore, it is significant to evaluate the antibiotic resistance properties of probiotics. Furthermore, characteristics linked to safety, survival in the host gut, and colonizing abilities are substantial to assess the proposed probiotic bacteria.
The prevalence of antibiotic-associated diarrhea varies based on diagnosis, with values between 5 and 30% in children and up to 70% in adults. In this aspect, treatment with LGG reduced the risk of antibiotic-associated diarrhea from 22 to 12% in adults and from 23 to 9.6% in children. In the LGG-groups, there was no need for antibiotic treatment discontinuation or intravenous rehydration (173). Korpela et al. evaluated whether long-term consumption of probiotics influences the antibiotic-associated changes in children's microbiota. Their results showed that long-term LGG-supplementation increased the levels of Lactococcus, Prevotella, and Ruminococcus and decreased the levels of Escherichia, as well as reduced the frequency of gastrointestinal complaints after the use of macrolides (174).
Salmonellosis is usually treated with antibiotics, which can cause side effects, including antibiotic resistance and enteric dysbacteriosis. Also, antibiotic therapy can worsen Salmonella-induced diarrhea. In this aspect, Lactobacilli strains are a promising alternative for Salmonella infection as they help prevent it, have fewer side effects, and have higher safety. Several Lactobacilli strains were found to attenuate the intestinal epithelial barrier dysfunction induced by Salmonella lipopolysaccharide (175). De Keersmaecker et al. demonstrated the potential antibacterial activity of LGG-spent culture supernatant (SCS) against S. typhimurium (176). Their results showed that several compounds, such as acetic, pyroglutamic, formic, and lactic acids, might be responsible for the antibacterial efficiency against Salmonella spp. Furthermore, infection with Salmonella spp. causes gut inflammation, which changes in the cytokine profile can detect. LGG reduced the levels of TNF-α in the colon while maintaining IL-10 and significantly reducing MPO (myeloperoxidase) levels (177).
Antibacterial Activity
Several reports showed the antibacterial activity of BB due to its high content of polyphenols and LAB. Also, the antimicrobial potential of BB for the prevention and treatment of bacterial and fungal infections in animals and humans has been demonstrated in (1, 178). In a recent study, several polish BB samples exerted higher inhibitory potential against Staphylococcus aureus ATCC 25923 and ATCC 29213. Furthermore, several pieces effectively inhibited the growth of S. aureus and MRSA (methicillin-resistant S. aureus), strains at concentrations between 2.5 and 5.0% (179). In the following study, the bacterial isolates of mature BB exhibited moderate antagonistic effects against S. aureus ATCC 25923, ATCC 29213, S. epidermidis ATCC 12228, P. aeruginosa ATCC 27853, and lower results against Escherichia coli ATCC 25922 (180).
Despite the progress in antimicrobial therapy, sepsis and meningitis caused by E. coli K1 remain the primary cause of mortality and severe neurological morbidity, particularly in preterm and very-low-birth-weight infants (181). In this aspect, He et al. in 2017 evaluated whether LGG supernatant has a preventive effect against gut-derived systemic neonatal E. coli K1 infection in human colonic carcinoma cell line Caco-2 and neonatal rat models. The in vitro results showed that LGG up-regulated mucin and maintained intestinal integrity by blocking the adhesion of E. coli K1 to Caco-2 monolayer. In contrast, the in vivo results showed a decrease of E. coli K1 infection susceptibility in neonatal rats who received oral administration of LGG and reduced bacterial intestinal colonization (182). Furthermore, the LGG-treated rats had increased intestinal expression of MUC2 (intestinal mucin), Ki67 (intestinal epithelial cell proliferation marker), IgA (immunoglobulin A), ZO-1 (zonula occludens-1), and mucin layer, and decreased barrier permeability compared to untreated rats.
Antiviral Activity
Influenza is an infectious disease that affects both animals and humans. The most common symptoms in humans are fever, coughing, muscle pain, chills, headaches, and weakness. In severe cases, it can cause pneumonia which can be fatal in children and the elderly. To investigate whether probiotics protect the host against influenza virus (IFV), BALB/c mice were infected with IFV A/PR/8/34 (H1N1) and administered intranasal LGG. It was shown that mice treated with LGG had lower symptoms frequency and higher survival rates than control. Also, increased mRNA expression of IL-8, TNF, and MCP-1 (monocyte chemotactic protein) were noticed in Ispirli and Dertli (183).
It was reported to reduce IL-2, IL-6, and IL-8, either by oral feeding or intraperitoneal injection with Apilactobacillus spp. The administration of heat-killed A. kunkeei YB38 reduced the symptoms of murine influenza pneumonia by enhancing SIgA production in mice infected with the influenza virus. The intake of A. kunkeei YB38 by healthy adults significantly improved secretory immunoglobulin A (SIgA) levels in saliva compared with baseline concentrations. Also, it decreased levels of the Bacteroides fragilis group.
Gastrointestinal Disorders
Based on their viability, probiotics can survive in simulated gastrointestinal conditions. LAB and their bioactive specific cellular components exert many pivotal effects on the ecosystem of the human GI tract, including maintenance of the gut microbiota and control of the enteric mucosal pathogens, and modulation of the cell-mediated immune response. Auto-aggregation and cell surface hydrophobicity properties of the bacterial cells are essential characteristics to reveal their potential as probiotics which can be associated with the cells adhesion to the gastrointestinal, demonstrating their health-promoting functions. The EPS (exopolysaccharide) production levels in A. kunkeei strains from BB had values between 0.17 (A. kunkeei AP-13) and 2.79 (A. kunkeei AP-15) g/L (115). Also, supplementation with LGG in colicky breastfed infants resulted in reduced daily crying and fecal calprotectin and increased total bacteria and Lactobacilli compared to the placebo group (140).
LGG has beneficial effects on the organism as to be considered probiotic. In particular, LGG can withstand gastric acidity and bile salts, effectively adhering to the gastrointestinal mucosa. The ability to resist gastric acidity and bile salts is a consequence of the power of the bacterium to produce anti-stress proteins that give it greater survival capacity in intestinal transit after oral intake (Table 4).
As neonatal necrotizing enterocolitis (NEC) is one of the significant causes of mortality in premature infants, oral administration of Bifidobacterium bifidum has been suggested as a promising preventive treatment. Thus, B. bifidum (5 × 106 CFU/day) administered to premature rat models of NEC decreased the ailment incidence from 57 to 17% and increased the levels of IL-6, mucin-3, and Tff3 (trefoil factor 3). The protective effect of B. bififdum is related to a decreased inflammatory reaction in the ileum, regulation of main components of the mucus layer, and intestinal integrity improvement (184).
Bone Loss Prevention
Osteoporosis is a chronic syndrome of excessive skeletal fragility characterized by bone mass loss and bone micro-architecture deterioration. Patients with human immunodeficiency virus (HIV) (185) or subjected to antiretroviral therapy with tenofovir disoproxil fumarate (TDF) (186) are strongly correlated to bone loss. Recently, it has been suggested that the gut microbiota is linked to bone homeostasis (187). Thus, Liu et al. evaluated the effectiveness of LGG in attenuation of TDF-induced bone loss in 6-week old C57BL6/J mice. LGG treatment reconstructed the gut microbiota structure and increased the intestinal barrier integrity, proving an effective treatment against TDF-induced osteoporosis (169).
Periodontitis, a chronic inflammatory condition, affects the soft and hard tissues that support teeth. It is influenced by specific pathogenic bacteria (i.e., Porphyromonas gingivalis and Fusobacterium nucleatum), which have been shown to aggravate inflammation and alveolar bone loss in mice (188). In a recent study, LGG administration to P. gingivalis and F. nucleatum-inoculated mice reduced tissue inflammation in the duodenum and IL-6 levels in ileum compared to control. Oral gavage with LGG induced a protective effect against intestinal inflammation and reduced the changes in the gut microbiome (189). LGG also attenuated bone loss in ovariectomy-induced postmenopausal mice models. Inhibition of bone loss was due to increased anti-osteoclastogenic CD4+Foxp3+Tregs and CD8+Foxp3+Tregs and reduced bone marrow, peyer's patch, spleen, and lymph nodes levels (190).
Liver Disease
Alcoholic liver disease is one of the major liver diseases and has increased morbidity and mortality rates. Continued alcohol consumption might cause intestinal microbiota homeostasis, intestinal tight junction barrier dysfunction, endotoxemia, and ultimately steatohepatitis. LGG and bacteria-free LGG culture supernatant have shown promising results in terms of liver protection, such as promoting intestinal epithelial integrity and protecting the intestinal barrier in both animal and human subjects. In this aspect, Zhao et al. demonstrated that supplementation with LGG (109 CFU/day/mouse) decreased ethanol-elevated miR122a expression levels and attenuated ethanol-induced liver injury in mice (191).
Cholestatic liver disease is characterized by gut dysbiosis and increased toxic hepatic bile acids.
The mechanism of action by LGG on hepatic bile acids, liver injury, fibrosis, were evaluated in bile duct ligation (BDL) and multidrug resistance protein two knockout (Mdr2−/−) mice. LGG-treated mice had reduced hepatic concentration of taurine-β-muricholic acid and normalized levels of chenodeoxycholic acid compared to BDL mice. Also, the LGG-group mice had increased serum and ileum fibroblast growth factor 15 expression levels and reduced hepatic cholesterol 7α-hydroxylase (192). Further details can be seen in Table 6.
Immunomodulatory Effects
In recent years, immunotherapy has become an increasing anti-cancer treatment due to its fewer side effects compared to conventional ones. LABs in the gut microbiome of patients under immunotherapy had favorable, responsive rates compared to patients lacking them. Viable and heat-killed LGG were administered to colonic cancer mice models to assess the gut immune background changes. LGG-supplemented rats had increased colonic CD8 T-cell (cytotoxic T lymphocytes) responses and decreased tumor burden in the murine gut cancer models by a CD8 Tcell–dependent manner (193).
Mast cells are multifunctional regulator cells responsible for defense against pathogenic microbes. Thus, the effect of LGG on human mast cell gene expression using microarray analysis was investigated. LGG suppressed genes encoded allergy-related high-affinity IgE receptor subunits α and γ (FCER1A and FCER1G) and histamine H4 receptor. Also, LGG up-regulated the expression levels of genes involved in mast cell immune system regulation and genes that encode proteins with a pro-inflammatory impact (IL-8) and with anti-inflammatory functions (IL-10) (194).
Giardiasis causes intestinal malabsorption and diarrhea in malnourished and immunodeficient individuals, but frequently in children <3 years old. Thus, the immunomodulatory effect of orally administered LGG in Giardia-infected mice was investigated. Pre-and post-probiotic oral administration for 25 days modulated the mucosal immune system response, as the levels of IgA antibody, IgA+ cells, and CD4+ T lymphocytes increased, as well as increased levels of anti-inflammatory cytokines (i.e., IL-6 and Il-10) and decreased levels of pro-inflammatory cytokine INF-γ (195).
Using several BP fermentation variants, Knazovická et al. noticed a decrease in Enterobacteriaceae, making the fermented products appropriate for consumption by people with lower immunity. Apart from the types of fermentation involved in the process, natural products with antibiotic attributes developed by the existing microbiota in stored BP, interactions among living microorganisms can control and enhance the microbiota (73). Furthermore, the resulting BB is more suitable for the organism due to its increased digestibility (196).
Allergic Asthma
Asthma is an airway inflammatory disorder, and its prevalence is increasing by each year. Recent epidemiological studies demonstrated the relationship between asthma, allergic disorder development, and altered intestinal microbiota in both animal models (197–200) and human clinical trials (201–203). Intestinal colonization with freeze-dried LGG in pregnant and during lactation of BALB/c female mice for 48 days resulted in reduced allergic airway, peribronchial inflammation, goblet cell hyperplasia, and increased TNF-α levels (198). Also, pre-and post-treatment with LGG suppressed the airway hyper-responsiveness to methacholine and metalloproteinase nine expressions in lung tissue. Also, it significantly decreased the number of infiltrating inflammatory cells and Th2 cytokines in bronchoalveolar lavage fluid and serum compared with the OVA-sensitized mice (204). Recently, it was demonstrated that pre-and post-supplementation with LGG suppressed Th2 cytokine (T helper type II cells), TNF-α, IL-17, and HMGB (high mobility group box 1) in the BALF (Bronchoalveolar Lavage Fluid) levels and increased the levels of proteins involved in immune system responses, namely T-bet and Foxp3 in ARN (205).
The efficacy of LGG administration was studied on at-risk infants, as children of allergic mothers have an increased risk of developing allergies. Thus, LGG administration for 6 months diminished by almost 50% the incidence of allergy to expecting mothers and their infant's (197). No significant differences in asthma and allergic rhinitis were shown between early probiotic and placebo supplementation in infants (206).
Atopic Dermatitis
Atopic dermatitis (AD) is an increasing chronic skin disease in children with a prevalence reaching 10% in many industrialized countries (207). The rising incidence of atopic dermatitis might be associated with shifts in gastrointestinal microbiota, namely from a rich flora in non-pathogenic bacteria (i.e., Lactobacilli or Bifidobacterium) to one with increased pathogenic bacteria (i.e., Clostridium) (208). The preventive effect of Lactobacillus on the incidence of atopic dermatitis was demonstrated in pre-and postnatal infants who were less likely to develop this disease compared to placebo group infants. In a randomized, double-blind trial, pregnant women with atopic eczema, allergic rhinitis, or asthma received LGG (1 × 1010 CFU/day) between 2 and 4 weeks before the estimated delivery date. After delivery, infants received LGG for 6 months. In the 4th year, at the end of the trial, 46% of the children in the placebo group developed AD, compared to 26% in the probiotic group (201, 209). In a subsequent randomized, placebo-controlled trial, the children completing the previous studies were invited to complete a 7-year treatment. The LGG group had a lower risk of developing eczema (42%) compared to the placebo group (66%) (210). Rautava, Kalliomäki, and Isolauri in 2002 found that the levels of transforming growth factor (TGF)-β was significantly higher in the breast milk of LGG-administered mothers (2 × 1010 CFU/day) before labor and 3 months after birth (211). Furthermore, AD development was significantly lower in infants receiving LGG in the first 2 years compared to the placebo group. In multiple studies, no significant differences were observed between the probiotic and placebo-treated patients (212–214) or in IL-4, IL-5, and interferon γ (IFN γ) (215). Also, no significant differences between the probiotic and placebo groups regarding the eczema frequencies and atopic eczema were noticed (206, 216). Recently, Filipovic et al., in 2020, demonstrated that LGG formulation with Zn and vitamin D3 supplementation in infancy and early childhood proves to be an effective treatment against AD (161).
Lowering Cholesterol Levels
Excess cholesterol is associated with coronary artery disease, the most common cardiovascular disease with increased worldwide mortality by each year. Thus, there is a need for natural therapeutic products that lower cholesterol levels besides the existing treatment schemes. In this aspect, Kim et al. orally administered LGG to high-fat diet (HFD) mice for 13 weeks. A significant reduction of weight and adipose tissues were noticed in LGG-treated HFD-fed mice compared to control. The triglyceride serum levels and cholesterol were also significantly reduced (217).
In 2008, Vamanu et al. performed a study analyzing the evolution of L. acidophilus 1a, and L. plantarum 2s strains on a media with BP and honey. The study aimed to demonstrate the outcome of fermented products on cholesterol levels in Wistar rats (n = 40) and possible bio-productive effects. An increase in sugar consumption after 72 h of fermentation was noticed in the milled pollen grains groups and an aroma specific to BP and lactic acid. The results showed that increased weight was seen in all treated groups with the symbiotic product compared to control. Other monitored parameters were taste and aroma, highlighting that once lactic acid is produced, the sweet flavor converts to a mix between sweet and sour (218).
Safety and Digestibility
Understanding intestinal health and disease is strongly linked to identifying the functional elements behind the gut epithelia's effective growth and homeostasis. Recently, it was demonstrated that the intake of LGG elevated the leptin levels in the gut epithelia important in normal metabolism function and intestinal development and triggered cell proliferation (219).
According to the results presented in previous studies, in 2011, Fuenmayor et al. assessed the conditions required for a better BP fermentation from Columbia. Their results showed the possibility to use L. acidophilus inoculum in a matrix of BP to induce lactic fermentation and generate a probiotic product with additional functional characteristics, which might be used as a protein food supplement suitable for human consumption or as an ingredient in the development of other functional foods (98).
The massive role of A. kunkeei in preserving BB has been demonstrated in previous studies that have recognized BB's stability to the influences caused by nectar, honey, bee secretions, and BP natural properties (7). The fermentation protocol developed during this study emulates the natural BB fermentation process, generating a stable and safe fermented product with increased digestibility and bioavailability of nutrients due to the use of specific strains of lactobacilli as A. kunkeei (45).
Confectionary foods contain large numbers of titanium dioxide nanoparticles (TiO2 NPs), proving to be at a high risk of developing diet-induced metabolism syndrome (MetS). In this aspect, LGG-oral supplementation to MetS mice ameliorates TiO2 NPs-induced inflammation and metabolic abnormality caused by fructose. In LGG-supplemented mice, the gut dysbiosis was improved, and the inflammation-related bacteria (Clostridia, Desulfovibrionaceae, and Proteobacteria) were decreased; thus LGG acted as a defensive system against TiO2 NPs-induced severe inflammation damage (220).
Urinary symptoms are common in people suffering from neurogenic lower urinary tract dysfunction (NLUTD). As there are no non-prescription treatment methods (221), assessed the safety and tolerability of LGG in adults and children with NLUTD due to spinal cord injury or disease. At the end of the 18-month study, two doses of self-installed intravesical LGG were safe and well-tolerated among the tested individuals. This aspect was further confirmed by LGG installation in asymptomatic patients with neuropathic bladder. No adverse effects were reported after installation (222).
Impact Assessment of Current BB Production
Because collecting BB from the hive is time-consuming and harmful, it's marketing for human use is hampered. On the other hand, pollen removal through traps is handy for beekeepers and harmless to the hive (17). Harvesting BB can be a profitable option, but it is rarely applied in apiaries due to high costs and time-consuming. However, it is an auspicious opportunity, thanks to the rising consumer awareness of functional foods (i.e., food with positive and pro-health effects on human individuals, primarily due to the naturally present bioactive substances) (223).
The process of acquiring the final product can technically be entirely automated. However, in conventional apiaries, which are the most common in EU nations and have an average of 21 hives per beekeeper, it is not economically justified (224). A 3-year study began in the spring of 2015 to establish the volume of BB production in honeybee colonies and analyze the economic effects of such output. Each year, 28 honeybee colonies participated in the experiment, separated into four groups. Each group tried alternative brood nest configurations or frame positioning against the hive entrance for collected BB. All costs, including labor, were associated with the BB production process. Depending on the group, it gathered between 0.51 and 1.23 kg of BB from a single colony. The average yield was 0.7 kg, while the apiary as a whole produced 20 kg of BB each year.
Moreover, the annual expenditures associated with BB manufacturing were 679.5 EUR, whereas the projected sales gain was 1110 EUR. As a result, the income was 430.5 EUR or 21.5 EUR per kilogram of collected BB. The most significant expenses were associated with labor, which might be a factor restricting the growth of BB manufacturing in apiaries (49).
Challenges and Opportunities for Developing Improved Fermented Functional Foods
Fermented foods and beverages are global edible products of significant scientific, social, and economic importance. A fermented nutritive matrix represents one-third of all foods produced for human consumption (225). Furthermore, the attention of prestigious international institutions, such as the World Health Organization (WHO) and the Food and Agriculture Organization (FAO) of the United Nations (UN), to the importance of microbiological risks assessment and of traditional fermented food and beverages contribute to testify to the relevance of the discussed topic (226). The intake of fermented foods and drinks is typically connected with beneficial properties (227). This increased nutritional and functional quality would serve as an additional reason to establish a risk management plan to minimize contamination (225). The establishment of a multi-strain starter culture based on genotypic and technical characterization of microbial diversity connected with natural food fermentation to increase the “unique characteristics” defined as fermented attributes (228). It is critical to differentiate between the risk associated with microbial genera/species that are not generally present in fermented matrices and the risks associated with spontaneous fermentation. Pathogens are classified as the first class, whereas mycotoxins, ethyl carbamate, and biogenic amines are second. Because the same species includes both technologically beneficial and harmful strains, the second category is more subtle (229). Controlling the microbiology of fermented foods may be an essential step toward harnessing the biotechnological potential of strains developed via spontaneous fermentation (230). Recent economic, productive, and social trends have rediscovered the possibility of spontaneous fermentation in increasing the uniqueness of fermented goods.
Future Perspectives and Conclusions
Over the last decade, aspects of artificial food additives and consumer demands for balanced and healthy diets may have accelerated research on natural products, such as BB. As a result, researchers are working to uncover its nutritional importance and health-promoting effects. A comparison of expenses and earnings generated by the production of BB, obtained in nature in the hive, showed that the financial impact could sometimes be less satisfactory. Labor costs can be a problem that restricts the development of this beekeeping product, so a biotechnological approach may be a better way to improve the market's presence. In the case of fermented BP consumed by humans, the chosen starters improved the bioavailability and digestibility of nutrients and bioactive compounds naturally found in BP.
Moreover, from an economic standpoint, the biotechnological development of BB may be an alternative and efficient way to diversify the beekeeping activity, which is gradually susceptible to frequent problems and increasing beekeepers' incomes. Further research into the classification of aromatic compounds, the flavor impact of organic acid-aroma interactions, chosen LAB starters and fermentation protocols, as well as consumer demands, will lead to noteworthy advancements in the flavor of fermented bee products for a promising market. Additionally, the need for value-added products with numerous health properties, macro-, and micronutrients, important bio-elements, probiotics, and vitamins is gaining popularity.
Author Contributions
DGB and MC-C: conceptualization, methodology, and writing—original draft preparation. RM: validation, supervision, and writing—review & editing. DCV: funding acquisition, supervision, and writing—review and editing. All authors read and approved the manuscript.
Funding
This work was supported by the National Research, Development, and Innovations Programme for 2015-2020-PNII, developed with the support of UEFISCDI PN-III-P1-1.1-TE-2019-1748 (TE 184/2020), and the publication was supported by funds from the National Research Development Projects to finance excellence (PFE)-14/2022-2024 granted by the Romanian Ministry of Research and Innovation.
Conflict of Interest
The authors declare that the research was conducted in the absence of any commercial or financial relationships that could be construed as a potential conflict of interest.
Publisher's Note
All claims expressed in this article are solely those of the authors and do not necessarily represent those of their affiliated organizations, or those of the publisher, the editors and the reviewers. Any product that may be evaluated in this article, or claim that may be made by its manufacturer, is not guaranteed or endorsed by the publisher.
References
1. Mărgăoan R, Stran? M, Varadi A, Topal E, Yücel B, Cornea-Cipcigan M, et al. Bee collected pollen and bee bread: bioactive constituents and health benefits. Antioxidants. (2019) 8:568. doi: 10.3390/antiox8120568
2. Kieliszek M, Piwowarek K, Kot AM, Błazejak S, Chlebowska-Smigiel A, Wolska I. Pollen and bee bread as new health-oriented products: A review. Trends Food Sci Technol. (2018) 71:170–80. doi: 10.1016/j.tifs.2017.10.021
3. Khalifa SAM, Elashal M, Kieliszek M, Ghazala NE, Farag MA, Saeed A, et al. Recent insights into chemical and pharmacological studies of bee bread. Trends Food Sci Technol. (2020) 97:300–16. doi: 10.1016/j.tifs.2019.08.021
4. Mărgăoan R, Topal E, Balkanska R, Yücel B, Oravecz T, Cornea-Cipcigan M, et al. Monofloral honeys as a potential source of natural antioxidants, minerals and medicine. Antioxidants. (2021) 10:1023. doi: 10.3390/antiox10071023
5. Mărgăoan R, Özkök A, Keskin S, Mayda N, Urcan AC, Cornea-Cipcigan M. Bee collected pollen as a value-added product rich in bioactive compounds and unsaturated fatty acids: A comparative study from Turkey and Romania. LWT. (2021) 149:111925. doi: 10.1016/j.lwt.2021.111925
6. Mărgăoan R, Zăhan M, Mărghitaş LA, Dezmirean DS, Erler S, Bobiş O. Antiproliferative activity and apoptotic effects of Filipendula ulmaria pollen against C26 mice colon tumour cells. J Apicult Sci. (2016) 60:135–44. doi: 10.1515/jas-2016-0014
7. Anderson KE, Carroll MJ, Sheehan T, Mott BM, Maes P, Corby-Harris V. Hive-stored pollen of honey bees: many lines of evidence are consistent with pollen preservation, not nutrient conversion. Mol Ecol. (2014) 23:5904–17. doi: 10.1111/mec.12966
8. Degrandi-Hoffman G, Chen Y, Simonds R. The effects of pesticides on queen rearing and virus titers in honey bees (Apis mellifera L.). Insects. (2013) 4:71–89. doi: 10.3390/insects4010071
9. Siksna S, Daberte I, Barene I. Investigation of bee bread and development of its dosage forms. Medicinos teorija ir praktika. (2014) 21:16–22. doi: 10.15591/mtp.2015.003
10. Celina Habryka, Marek Kruczek, Drygaś B. Bee Products Used in Apitherapy. Darwin, Poland: Scientific Publishing House. (2016).
11. Dranca F, Ursachi F, Oroian M. Bee bread: physicochemical characterization and phenolic content extraction optimization. Foods. (2020) 9:1358. doi: 10.3390/foods9101358
12. Urcan AC, Marghitas LA, Dezmirean DS, Bobis O, Bonta V, Muresan CI, et al. Chemical composition and biological activities of beebread – review. Bull University Agri Sci Vet Med Cluj-Napoca Animal Sci Biotechnol. (2017) 74:6. doi: 10.15835/buasvmcn-asb:12646
13. Bakour M, Al-Waili NS, El Menyiy N, Imtara H, Figuira AC, Al-Waili T, et al. Antioxidant activity and protective effect of bee bread (honey and pollen) in aluminum-induced anemia, elevation of inflammatory makers and hepato-renal toxicity. J Food Sci Technol. (2017) 54:4205–12. doi: 10.1007/s13197-017-2889-9
14. Bakour M, Fernandes Â, Barros L, Sokovic M, Ferreira ICFR, Badiaa L. Bee bread as a functional product: Chemical composition and bioactive properties. LWT. (2019) 109:276–82. doi: 10.1016/j.lwt.2019.02.008
15. Medina Á, González G, Sáez JM, Mateo R, Jiménez M. Bee Pollen, a Substrate that Stimulates Ochratoxin A Production by Aspergillus ochraceus Wilh. Syst Appl Microbiol. (2004) 27:261–7. doi: 10.1078/072320204322881880
16. González G, Hinojo MJ, Mateo R, Medina A, Jiménez M. Occurrence of mycotoxin producing fungi in bee pollen. Int J Food Microbiol. (2005) 105:1–9. doi: 10.1016/j.ijfoodmicro.2005.05.001
17. Nardoni S, D'Ascenzi C, Rocchigiani G, Moretti V, Mancianti F. Occurrence of moulds from bee pollen in Central Italy – A preliminary study. Ann Agri Environ Med. (2015) 23:103–5. doi: 10.5604/12321966.1196862
18. Carlos M, Zuluaga JCS, Marta C. Chemical, Nutritional and Bioactive Characterization of Colombian Bee-Bread (2015).
19. Karaçil MS, Tek NA. Dünyada Üretilen Fermente Ürünler: Tarihsel Süreç ve Saglik ile Ilişkileri. (2013).
20. Kaškoniene V, Adaškevičiute V, Kaškonas P, Mickiene R, Maruška A. Antimicrobial and antioxidant activities of natural and fermented bee pollen. Food Biosci. (2020) 34:100532. doi: 10.1016/j.fbio.2020.100532
21. Salazar-González C, Díaz-Moreno C. The nutritional and bioactive aptitude of bee pollen for a solid-state fermentation process. J Apicult Res. (2016) 55:161–75. doi: 10.1080/00218839.2016.1205824
22. Aylanc V, Falcão SI, Ertosun S, Vilas-Boas M. From the hive to the table: Nutrition value, digestibility and bioavailability of the dietary phytochemicals present in the bee pollen and bee bread. Trends Food Sci Technol. (2021) 109:464–81. doi: 10.1016/j.tifs.2021.01.042
23. Tomás A, Falcão SI, Russo-Almeida P, Vilas-Boas M. Potentialities of beebread as a food supplement and source of nutraceuticals: Botanical origin, nutritional composition and antioxidant activity. J Apicultural Res. (2017) 56:219–30. doi: 10.1080/00218839.2017.1294526
24. Filannino P, Di Cagno R, Addante R, Pontonio E, Gobbetti M. Metabolism of fructophilic lactic acid bacteria isolated from the Apis mellifera L. bee gut: phenolic acids as external electron acceptors. Appl Environ Microbiol. (2016) 82:6899–911. doi: 10.1128/AEM.02194-16
25. Bojan A, Goran J, Mića M, Jordan M, Mirjana P, Nedić N. Quality of pollen and honey bee bread collected in spring. J Hyg Eng Des. (2012).
26. Donkersley P, Rhodes G, Pickup RW, Jones KC, Wilson K. Honeybee nutrition is linked to landscape composition. Ecol Evol. (2014) 4:4195–206. doi: 10.1002/ece3.1293
27. Andjelković MS, Petrović V, Stamenković Z, Ristić GS, Jovanović GS. Circuit-level simulation of the single event transients in an on-chip single event latchup protection switch. J Electr Test. (2015) 31:275–89. doi: 10.1007/s10836-015-5529-1
28. Fatrcová-Šramková K, NôŽková J, Kačániová M, Máriássyová M, Rovná K, Stričík M. Antioxidant and antimicrobial properties of monofloral bee pollen. J Environ Sci Health Part B Pesticides Food Contaminants Agri Wastes. (2013) 48:133–8. doi: 10.1080/03601234.2013.727664
29. Degrandi-Hoffman G, Eckholm BJ, Huang MH. A comparison of bee bread made by Africanized and European honey bees (Apis mellifera) and its effects on hemolymph protein titers. Apidologie. (2013) 44:52–63. doi: 10.1007/s13592-012-0154-9
30. Bayram NE, Gercek YC, Çelik S, Mayda N, Kostić AŽ, Dramićanin AM, et al. Phenolic and free amino acid profiles of bee bread and bee pollen with the same botanical origin – similarities and differences. Arab J Chem. (2021) 14:103004. doi: 10.1016/j.arabjc.2021.103004
31. Venskutonis PR, Kaškoniene V, Račys J, Ceksteryte V. Fatty acid composition in beebread. Biologija. (2008) 54:253–7. doi: 10.2478/v10054-008-0052-2
32. Mărgăoan R, Chirilă F. Bee Pollen Methanolic Extracts: Total Polyphenols Content and Antibacterial Activity. (2021).
33. Pascoal A, Rodrigues S, Teixeira A, Feás X, Estevinho LM. Biological activities of commercial bee pollens: Antimicrobial, antimutagenic, antioxidant and anti-inflammatory. Food Chem Toxicol. (2014) 63:233–9. doi: 10.1016/j.fct.2013.11.010
34. Ivanišová E, Kačániová M, Frančáková H, Petrová J, Hutková J, Brovarskyi V, et al. Bee bread - perspective source of bioactive compounds for future. Potravinarstvo. (2015) 9:558. doi: 10.5219/558
35. Sawicki T, Baczek N, Starowicz M. Characterisation of the total phenolic, vitamins C and E content and antioxidant properties of the beebread and honey from the same batch. Czech J Food Sci. (2020) 38:158–63. doi: 10.17221/312/2019-CJFS
36. Sattler JAG, De-Melo AAM, Nascimento KSD, Melo ILPD, Mancini-Filho J, Sattler A, et al. Essential minerals and inorganic contaminants (barium, cadmium, lithium, lead and vanadium) in dried bee pollen produced in Rio Grande do Sul State, Brazil. Food Sci Technol. (2016) 36:505–9. doi: 10.1590/1678-457X.0029
37. Nakajima Y, Tsuruma K, Shimazawa M, Mishima S, Hara H. Comparison of bee products based on assays of antioxidant capacities. BMC Complement Alternative Med. (2009) 9:4. doi: 10.1186/1472-6882-9-4
38. Veiga RS, De Mendonça S, Mendes PB, Paulino N, Mimica MJ, Lagareiro Netto AA, et al. Artepillin C and phenolic compounds responsible for antimicrobial and antioxidant activity of green propolis andBaccharis dracunculifoliaDC. J Appl Microbiol. (2017) 122:911–20. doi: 10.1111/jam.13400
39. Yildirim A, Duran GG, Duran N, Jenedi K, Bolgul BS, Miraloglu M, et al. Antiviral activity of hatay propolis against replication of herpes simplex virus type 1 and type 2. Med Sci Monit. (2016) 22:422–30. doi: 10.12659/MSM.897282
40. Rimbach G, Fischer A, Schloesser A, Jerz G, Ikuta N, Ishida Y, et al. Anti-inflammatory properties of Brazilian green propolis encapsulated in a γ-cyclodextrin complex in mice fed a western-type diet. Int J Mol Sci. (2017) 18:1141. doi: 10.3390/ijms18061141
41. Liu C-C, Hao D-J, Zhang Q, An J, Zhao J-J, Chen B, et al. Application of bee venom and its main constituent melittin for cancer treatment. Cancer Chemother Pharmacol. (2016) 78:1113–30. doi: 10.1007/s00280-016-3160-1
42. Atkin SL, Barrier S, Cui Z, Fletcher PDI, Mackenzie G, Panel V, et al. UV and visible light screening by individual sporopollenin exines derived from Lycopodium clavatum (club moss) and Ambrosia trifida (giant ragweed). J Photochem Photobiol B. (2011) 102:209–17. doi: 10.1016/j.jphotobiol.2010.12.005
43. Gilliam M, Roubik D, Lorenz B. Microorganisms associated with pollen, honey, and brood provisions in the nest of a stingless bee, Melipona fasciata. Apidologie. (1990) 21:89–97. doi: 10.1051/apido:19900201
44. Gilliam M, Buchmann SL, Lorenz BJ, Roubik DWJB. Microbiology of the larval provisions of the stingless bee, Trigona hypogea, an obligate necrophage. Biotropica. (1985) 17:28. doi: 10.2307/2388374
45. Di Cagno R, Filannino P, Cantatore V, Gobbetti M. Novel solid-state fermentation of bee-collected pollen emulating the natural fermentation process of bee bread. Food Microbiol. (2019) 82:218–30. doi: 10.1016/j.fm.2019.02.007
46. Tamarit D, Ellegaard KM, Wikander J, Olofsson T, Vasquez A, Andersson SGE. Functionally structured genomes in Lactobacillus kunkeei colonizing the honey crop and food products of honeybees and stingless bees. Genome Biol Evol. (2015) 7:1455–73. doi: 10.1093/gbe/evv079
47. Mayda N, Ozkok A, Bayram NE, Gercek YC, Sorkun K. Bee bread and bee pollen of different plant sources: determination of phenolic content, antioxidant activity, fatty acid and element profiles. J Food Meas Charact. (2020) 14:1795–809. doi: 10.1007/s11694-020-00427-y
48. Souza RCdS, Yuyama LKO, Aguiar JPL, Oliveira FPM. Valor nutricional do mel e pólen de abelhas sem ferrão da região amazônica. (2014).
49. Semkiw P, Skubida P. Bee bread production—a new source of income for beekeeping farms? Agriculture. (2021) 11:6. doi: 10.3390/agriculture11060468
50. Mărgăoan R, Cornea-Cipcigan M, Topal E, Kösoglu M. Impact of fermentation processes on the bioactive profile and health-promoting properties of bee bread, mead and honey vinegar. Processes. (2020) 8:1081. doi: 10.3390/pr8091081
52. Waśko A, Kieliszek M, Targoński Z. Purification and characterization of a proteinase from the probiotic Lactobacillus rhamnosus OXY. Preparative Biochem Biotechnol. (2012) 42:476–88. doi: 10.1080/10826068.2012.656869
53. García C, Rendueles M, Díaz M. Liquid-phase food fermentations with microbial consortia involving lactic acid bacteria: A review. Food Res Int. (2019) 119:207–20. doi: 10.1016/j.foodres.2019.01.043
54. Florou-Paneri P, Christaki E, Bonos E. Lactic acid bacteria as source of functional ingredients. InTech. (2013) 25:589–614. doi: 10.5772/47766
55. Peng K, Koubaa M, Bals O, Vorobiev E. Recent insights in the impact of emerging technologies on lactic acid bacteria: A review. Food Res Int. (2020) 137:109544. doi: 10.1016/j.foodres.2020.109544
56. Mora WI, Fuenmayor CA, Benavides MA, Algecira NA, Quicazán MC. Bee pollen as a novel substrate in pilot-scale probiotic-mediated lactic fermentation processes. LWT. (2021) 2021:110868. doi: 10.1016/j.lwt.2021.110868
57. Koch H, Schmid-Hempel P. Bacterial communities in central european bumblebees: low diversity and high specificity. Microbial Ecol. (2011) 62:121–33. doi: 10.1007/s00248-011-9854-3
58. Van De Guchte M, Penaud S, Grimaldi C, Barbe V, Bryson K, Nicolas P, et al. The complete genome sequence of Lactobacillus bulgaricus reveals extensive and ongoing reductive evolution. Proc Natl Acad Sci USA. (2006) 103:9274–9. doi: 10.1073/pnas.0603024103
59. Endo A, Maeno S, Tanizawa Y, Kneifel W, Arita M, Dicks L, et al. Fructophilic lactic acid bacteria, a unique group of fructose-fermenting microbes. Appl Environ Microbiol. (2018) 84:19. doi: 10.1128/AEM.01290-18
60. Endo A, Tanizawa Y, Tanaka N, Maeno S, Kumar H, Shiwa Y, et al. Comparative genomics of Fructobacillus spp. and Leuconostoc spp. reveals niche-specific evolution of Fructobacillus spp. BMC Genomics. (2015) 16:1117. doi: 10.1186/s12864-015-2339-x
61. Zaunmüller T, Eichert M, Richter H, Unden G. Variations in the energy metabolism of biotechnologically relevant heterofermentative lactic acid bacteria during growth on sugars and organic acids. Appl Microbiol Biotechnol. (2006) 72:421–9. doi: 10.1007/s00253-006-0514-3
62. Calasso M, Gobbetti M. Lactic acid bacteria | Lactobacillus spp.: other species. Encyclopedia of Dairy Sciences. Amsterdam: Elsevier Ltd. (2011). doi: 10.1016/B978-0-12-374407-4.00265-X
63. Batt CA. Lactobacillus | Introduction. Encyclopedia Food Microbiol. (2014) 2014:409–11. doi: 10.1016/B978-0-12-384730-0.00176-2
64. Filannino P, Di Cagno R, Gambacorta G, Tlais AZ, Cantatore V, Gobbetti M. Volatilome and bioaccessible phenolics profiles in lab-scale fermented bee pollen. Foods. (2021) 10:20286. doi: 10.3390/foods10020286
65. Abushelaibi A, Al-Mahadin S, El-Tarabily K, Shah NP, Ayyash M. Characterization of potential probiotic lactic acid bacteria isolated from camel milk. LWT. (2017) 79:316–25. doi: 10.1016/j.lwt.2017.01.041
66. Mašková Z, Knazovická V, Tančinová D, Panáková S. Production of pollen cans by fermentation of bee pollen in model conditions with regard to filamentous micromycetes occurrence. J Microbiol Biotechnol Food Sci. (2019) 8:1223–7. doi: 10.15414/jmbfs.2019.8.5.1223-1227
67. Zuluaga-Dominguez CM, Quicazan M. Effect of fermentation on structural characteristics and bioactive compounds of bee-pollen based food. J Apicultural Sci. (2019) 63:209–22. doi: 10.2478/jas-2019-0016
68. Araneda X, Velásquez C, Morales D, Martínez I. Producción de pan de abejas (Apis mellifera L.) bajo condiciones de laboratorio. Idesia (Arica). (2014) 32:63–9. doi: 10.4067/S0718-34292014000400008
69. Risco-Ríos CAd, Pérez-Piñeiro A, Álvarez-Rivera VP, RodríguezCastro i, Virginia Leiva-Castillo, Puig-Peña Y, et al. Bacterias ácido-lácticas para ensilar polen apícola. (2012).
70. Endo A, Futagawa-Endo Y, Dicks LMT. Isolation and characterization of fructophilic lactic acid bacteria from fructose-rich niches. Syst Appl Microbiol. (2009) 32:593–600. doi: 10.1016/j.syapm.2009.08.002
71. Yang S, Wu Y, Luo C, Di Z, Wu Y, Zhang J, et al. A Bacillus coagulans and its application in pine pollen fermentation. Food Sci Biotechnol. (2015) 24:2129–35. doi: 10.1007/s10068-015-0283-9
72. Mohr KI, Tebbe CC. Diversity and phylotype consistency of bacteria in the guts of three bee species (Apoidea) at an oilseed rape field. Environ Microbiol. (2006) 8:258–72. doi: 10.1111/j.1462-2920.2005.00893.x
73. Knazovická V, Mašková Z, Vlková E, Švejstil R, Salmonová H, Ivanišová E, et al. Pollen can - testing of bee pollen fermentation in model conditions. J Microbiol Biotechnol Food Sci. (2018) 8:805–11. doi: 10.15414/jmbfs.2018.8.2.805-811
74. Vamanu A, Vamanu E, Popa O, Câmpeanu G, Albulescu R, Drugulescu M, et al. Obtaining of a symbiotic product based on lactic bacteria, pollen and honey. Pakistan J Biol Sci. (2008) 11:613–7. doi: 10.3923/pjbs.2008.613.617
75. Linjordet MS. A Comparative Analysis of Lactic Acid Bacteria Isolated from Honeybee Gut and Flowers, with Focus on Phylogeny and Plasmid Profile. Ås: Norwegian University of Life Sciences. (2016).
76. Yang S, Li H. Optimization of pine pollen fermentation conditions using Lactobacillus paracasei. Food Sci Biotechnol. (2015) 24:155–60. doi: 10.1007/s10068-015-0021-3
77. du Plessis HW, Dicks LMT, Pretorius IS, Lambrechts MG, du Toit M. Identification of lactic acid bacteria isolated from South African brandy base wines. Int J Food Microbiol. (2004) 91:19–29. doi: 10.1016/S0168-1605(03)00335-0
78. Endo A, Tanaka N, Oikawa Y, Okada S, Dicks L. Fructophilic Characteristics of Fructobacillus spp. may be due to the Absence of an Alcohol/Acetaldehyde Dehydrogenase Gene (adhE). Curr Microbiol. (2014) 68:531–5. doi: 10.1007/s00284-013-0506-3
79. Vamanu E, Vamanu A, Popa O, Babeanu NJSPASB. The antioxidant effect of a functional product based on probiotic biomass, pollen and honey. Animal Sci Biotechnol. (2010) 43:331–6. Available online at: https://www.usab-tm.ro/fileadmin/fzb/Simp%202010/vol1/BIOTECHNOLOGIES/Vamanu1.pdf
80. Vergalito F, Testa B, Cozzolino A, Letizia F, Succi M, Lombardi SJ, et al. Potential application of Apilactobacillus kunkeei for human use: evaluation of probiotic and functional properties. Foods. (2020) 9:1535. doi: 10.3390/foods9111535
81. CadeŽ N, Fülöp L, Dlauchy D, Péter G. Zygosaccharomyces favi sp. nov., an obligate osmophilic yeast species from bee bread and honey. Antonie van Leeuwenhoek. (2015) 107:645–54. doi: 10.1007/s10482-014-0359-1
82. Iorizzo M, Pannella G, Lombardi SJ, Ganassi S, Testa B, Succi M, et al. Inter- and intra-species diversity of lactic acid bacteria in Apis mellifera ligustica colonies. Microorganisms. (2020) 8:1578. doi: 10.3390/microorganisms8101578
83. Endo A, Okada S. Reclassification of the genus Leuconostoc and proposals of Fructobacillus fructosus gen. nov., comb. nov., Fructobacillus durionis comb. nov., Fructobacillus ficulneus comb. nov. and Fructobacillus pseudoficulneus comb. nov. Int J Syst Evol Microbiol. (2008) 58:2195–205. doi: 10.1099/ijs.0.65609-0
84. Tang QH, Miao CH, Chen YF, Dong ZX, Cao Z, Liao SQ, et al. The composition of bacteria in gut and beebread of stingless bees (Apidae: Meliponini) from tropics Yunnan, China. Antonie Van Leeuwenhoek. (2021) 114:1293–305. doi: 10.1007/s10482-021-01602-x
85. Ngalimat MS, Raja Abd., Rahman RNZ, Yusof MT, Syahir A, Sabri S. Characterisation of bacteria isolated from the stingless bee, Heterotrigona itama, honey, bee bread and propolis. PeerJ. (2019) 7:e7478. doi: 10.7717/peerj.7478
86. Mohammad SM, Mahmud-Ab-Rashid N-K, Zawawi N. Probiotic properties of bacteria isolated from bee bread of stingless bee Heterotrigona itama. J Apicultural Res. (2021) 60:172–87. doi: 10.1080/00218839.2020.1801152
87. Ramos OY, Basualdo M, Libonatti C, Vega MF. Current status and application of lactic acid bacteria in animal production systems with a focus on bacteria from honey bee colonies. J Appl Microbiol. (2020) 128:1248–60. doi: 10.1111/jam.14469
88. Martinson VG, Danforth BN, Minckley RL, Rueppell O, Tingek S, Moran NA. A simple and distinctive microbiota associated with honey bees and bumble bees. Mol Ecol. (2011) 20:619–28. doi: 10.1111/j.1365-294X.2010.04959.x
89. Shah NP. Bifidobacterium spp.: morphology and physiology. (2011). doi: 10.1016/B978-0-12-374407-4.00043-1
90. Pokusaeva K, Fitzgerald GF, Van Sinderen D. Carbohydrate metabolism in Bifidobacteria. Genes Nutr. (2011) 6:285–306. doi: 10.1007/s12263-010-0206-6
91. Van Der Meulen R, Adriany T, Verbrugghe K, De Vuyst L. Kinetic analysis of bifidobacterial metabolism reveals a minor role for succinic acid in the regeneration of NAD+ through its growth-associated production. Appl Environ Microbiol. (2006) 72:5204–10. doi: 10.1128/AEM.00146-06
92. Emanuel Vamanu, Vamanu A, Popa O, Băbeanu N. The Antioxidant Effect of a Functional Product Based on Probiotic Biomass, Pollen and Honey. (2010).
93. Duan C, Feng Y, Zhou H, Xia X, Shang Y, Cui Y. Optimization of fermentation condition of man-made bee-bread by response surface methodology. Adv Appl Biotechnol. (2015) 2015:353–63. doi: 10.1007/978-3-662-46318-5_38
95. Zheng J, Wittouck S, Salvetti E, Franz C, Harris HMB, Mattarelli P, et al. A taxonomic note on the genus Lactobacillus: Description of 23 novel genera, emended description of the genus Lactobacillus Beijerinck 1901 and union of Lactobacillaceae and Leuconostocaceae. Int J Syst Evol Microbiol. (2020) 70:2782–858. doi: 10.1099/ijsem.0.004107
96. Yan S, Li Q, Xue X, Wang K, Zhao L, Wu L. Analysis of improved nutritional composition of bee pollen (Brassica campestris L.) after different fermentation treatments. Int J Food Sci Technol. (2019) 54:2169–81. doi: 10.1111/ijfs.14124
97. Kaškoniene V, Katilevičiute A, Kaškonas P, Maruška A. The impact of solid-state fermentation on bee pollen phenolic compounds and radical scavenging capacity. Chem Papers. (2018) 72:2115–20. doi: 10.1007/s11696-018-0417-7
98. Zhao Y, Liu S, Tang Y, You T, Xu H. Lactobacillus rhamnosus GG ameliorated long-term exposure to TiO(2) nanoparticles induced microbiota-mediated liver and colon inflammation and fructose-caused metabolic abnormality in metabolism syndrome mice. J Agri Food Chem. (2021) 69:9788–99. doi: 10.1021/acs.jafc.1c03301
99. Zhang Z, Cao H, Chen C, Chen X, Wei Q, Zhao F. Effects of fermentation by Ganoderma lucidum and Saccharomyces cerevisiae on rape pollen morphology and its wall. J Food Sci Technol. (2017) 54:4026–34. doi: 10.1007/s13197-017-2868-1
100. Vina I, Semjonovs P, Linde R, Denina I. Current evidence on physiological activity and expected health effects of kombucha fermented beverage. J Med Food. (2013) 17:179–88. doi: 10.1089/jmf.2013.0031
101. Jayabalan R, Malbaša RV, Lončar ES, Vitas JS, Sathishkumar M. A review on kombucha tea—microbiology, composition, fermentation, beneficial effects, toxicity, and tea fungus. Comprehen Rev Food Sci Food Safety. (2014) 13:538–50. doi: 10.1111/1541-4337.12073
102. Özkaya H, Özkaya B, Duman B, Turksoy S. Effect of dephytinization by fermentation and hydrothermal autoclaving treatments on the antioxidant activity, dietary fiber, and phenolic content of oat bran. J Agri Food Chem. (2017) 65:5713–9. doi: 10.1021/acs.jafc.7b01698
103. Zhou J, Qi Y, Ritho J, Zhang Y, Zheng X, Wu L, et al. Flavonoid glycosides as floral origin markers to discriminate of unifloral bee pollen by LC–MS/MS. Food Control. (2015) 57:54–61. doi: 10.1016/j.foodcont.2015.03.035
104. De-Melo AAM, Estevinho MLMF, Almeida-Muradian LB. A diagnosis of the microbiological quality of dehydrated bee-pollen produced in Brazil. Lett Appl Microbiol. (2015) 61:477–83. doi: 10.1111/lam.12480
105. Ekinci R. The effect of fermentation and drying on the water-soluble vitamin content of tarhana, a traditional Turkish cereal food. Food Chem. (2005) 90:127–32. doi: 10.1016/j.foodchem.2004.03.036
106. Chitte RR, Dey S. Production of a fibrinolytic enzyme by thermophilic Streptomyces species. World J Microbiol Biotechnol. (2002) 18:289. doi: 10.1023/A:1015252607118
107. Peng Y, Huang Q, Zhang R-H, Zhang Y-Z. Purification and characterization of a fibrinolytic enzyme produced by Bacillus amyloliquefaciens DC-4 screened from douchi, a traditional Chinese soybean food. Comparat Biochem Physiol Part B: Biochem Mol Biol. (2003) 134:45–52. doi: 10.1016/S1096-4959(02)00183-5
108. Sumi H, Hamada H, Tsushima H, Mihara H, Muraki H. A novel fibrinolytic enzyme (nattokinase) in the vegetable cheese Natto; a typical and popular soybean food in the Japanese diet. Experientia. (1987) 43:1110–1. doi: 10.1007/BF01956052
109. Amores-Arrocha A, Roldán A, Jiménez-Cantizano A, Caro I, Palacios V. Effect on white grape must of multiflora bee pollen addition during the alcoholic fermentation process. Molecules. (2018) 23:1321. doi: 10.3390/molecules23061321
110. Kostić AŽ, Petrović TS, Krnjaja VS, Nedić NM, Tešić ŽL, Milojković-Opsenica DM, et al. Mold/aflatoxin contamination of honey bee collected pollen from different Serbian regions. J Apicultural Res. (2016) 56:13–20. doi: 10.1080/00218839.2016.1259897
111. Kostić AŽ, Milinčić DD, Barać MB, Ali Shariati M, Tešić ŽL, Pešić MB. The application of pollen as a functional food and feed ingredient—the present and perspectives. Biomolecules. (2020) 10:84. doi: 10.3390/biom10010084
112. Asama T, Arima TH, Gomi T, Keishi T, Tani H, Kimura Y, et al. Lactobacillus kunkeeiYB38 from honeybee products enhances IgA production in healthy adults. J Appl Microbiol. (2015) 119:818–26. doi: 10.1111/jam.12889
113. Isayenko OY, Knysh OV, Babych YM, Ryzhkova TN, Dyukareva GI. Effect of disintegrates and metabolites of Lactobacillus rhamnosus and Saccharomyces boulardii on biofilms of antibiotic resistant conditionally pathogenic and pathogenic bacteria. Regul Mechan Biosyst. (2019) 10:3–8. doi: 10.15421/021901
114. Detry R, Simon-Delso N, Bruneau E, Daniel H-M. Specialisation of yeast genera in different phases of bee bread maturation. Microorganisms. (2020) 8:1789. doi: 10.3390/microorganisms8111789
115. Khailova L, Dvorak K, Arganbright KM, Halpern MD, Kinouchi T, Yajima M, et al. Bifidobacterium bifidum improves intestinal integrity in a rat model of necrotizing enterocolitis. Am J Physiol Gastrointest Liver Physiol. (2009) 297:G940–9. doi: 10.1152/ajpgi.00141.2009
116. Türkel S, Ener B. Isolation and Characterization of New Metschnikowia pulcherrima Strains as Producers of the Antimicrobial Pigment Pulcherrimin. Zeitschrift für Naturforschung C. (2009) 64:405–10. doi: 10.1515/znc-2009-5-618
117. Allonsius CN, van den Broek MFL, De Boeck I, Kiekens S, Oerlemans EFM, Kiekens F, et al. Interplay between Lactobacillus rhamnosus GG and Candida and the involvement of exopolysaccharides. Microbial Biotechnol. (2017) 10:1753–63. doi: 10.1111/1751-7915.12799
118. Songkun S, Shenglu C, Xuping Y, Xuezhen L, Fuliang H. The influence of bacteria isolated from bee bread on pH. Apiculture of China. (2002) 53: 4–5.
119. Cozzolino A, Vergalito F, Tremonte P, Iorizzo M, Lombardi SJ, Sorrentino E, et al. Preliminary evaluation of the safety and probiotic potential of Akkermansia muciniphila DSM 22959 in comparison with Lactobacillus rhamnosus GG. Microorganisms. (2020) 8:189. doi: 10.3390/microorganisms8020189
120. Jiang Q, Kainulainen V, Stamatova I, Korpela R, Meurman JH. Lactobacillus rhamnosus GG in experimental oral biofilms exposed to different carbohydrate sources. Caries Res. (2018) 52:220–9. doi: 10.1159/000479380
121. Seow SW, Cai S, Rahmat JN, Bay BH, Lee YK, Chan YH, et al. Lactobacillus rhamnosus GG induces tumor regression in mice bearing orthotopic bladder tumors. Cancer Sci. (2010) 101:751–8. doi: 10.1111/j.1349-7006.2009.01426.x
122. Linsalata M, Cavallini A, Messa C, Orlando A, Refolo MG, Russo F. Lactobacillus rhamnosus GG influences polyamine metabolism in HGC-27 gastric cancer cell line: a strategy toward nutritional approach to chemoprevention of gastric cance. Curr Pharmaceut Design. (2010) 16:847–53. doi: 10.2174/138161210790883598
123. Rokka S, Myllykangas S, Joutsjoki V. Effect of specific colostral antibodies and selected lactobacilli on the adhesion of Helicobacter pylori on AGS cells and the Helicobacter-induced IL-8 production. Scand J Immunol. (2008) 68:280–6. doi: 10.1111/j.1365-3083.2008.02138.x
124. Orlando A, Refolo MG, Messa C, Amati L, Lavermicocca P, Guerra V, et al. Antiproliferative and proapoptotic effects of viable or heat-killed Lactobacillus paracasei IMPC2.1 and Lactobacillus rhamnosus GG in HGC-27 gastric and DLD-1 colon cell lines. Nutr Cancer. (2012) 64:1103–11. doi: 10.1080/01635581.2012.717676
125. Russo F, Orlando A, Linsalata M, Cavallini A, Messa C. Effects of Lactobacillus rhamnosus GG on the cell growth and polyamine metabolism in HGC-27 human gastric cancer cells. Nutr Cancer. (2007) 59:106–14. doi: 10.1080/01635580701365084
126. Lopez M, Li N, Kataria J, Russell M, Neu J. Live and ultraviolet-inactivated Lactobacillus rhamnosus GG decrease flagellin-induced interleukin-8 production in Caco-2 cells. J Nutr. (2008) 138:2264–8. doi: 10.3945/jn.108.093658
127. Escamilla J, Lane MA, Maitin V. Cell-free supernatants from probiotic Lactobacillus casei and Lactobacillus rhamnosus GG decrease colon cancer cell invasion in vitro. Nutr Cancer. (2012) 64:871–8. doi: 10.1080/01635581.2012.700758
128. Prete R, Garcia-Gonzalez N, Di Mattia CD, Corsetti A, Battista N. Food-borne Lactiplantibacillus plantarum protect normal intestinal cells against inflammation by modulating reactive oxygen species and IL-23/IL-17 axis. Sci Rep. (2020) 10:16340. doi: 10.1038/s41598-020-73201-1
129. Toki S, Kagaya S, Shinohara M, Wakiguchi H, Matsumoto T, Takahata Y, et al. Lactobacillus rhamnosus GG and Lactobacillus casei suppress Escherichia coli-induced chemokine expression in intestinal epithelial cells. Int Arch Allergy Immunol. (2009) 148:45–58. doi: 10.1159/000151505
130. Uribe G, Villéger R, Bressollier P, Dillard RN, Worthley DL, Wang TC, et al. Lactobacillus rhamnosus GG increases cyclooxygenase-2 expression and prostaglandin E2 secretion in colonic myofibroblasts via a MyD88-dependent mechanism during homeostasis. Cell Microbiol. (2018) 20:e12871. doi: 10.1111/cmi.12871
131. Donato KA, Gareau MG, Wang YJJ, Sherman PM. Lactobacillus rhamnosus GG attenuates interferon-γ and tumour necrosis factor-α-induced barrier dysfunction and pro-inflammatory signalling. Microbiology. (2010) 156:3288–97. doi: 10.1099/mic.0.040139-0
132. Peña JA, Versalovic J. Lactobacillus rhamnosus GG decreases TNF-alpha production in lipopolysaccharide-activated murine macrophages by a contact-independent mechanism. Cell Microbiol. (2003) 5:277–85. doi: 10.1046/j.1462-5822.2003.t01-1-00275.x
133. Wang YM, Ge XZ, Wang WQ, Wang T, Cao HL, Wang BL, et al. Lactobacillus rhamnosus GG supernatant upregulates serotonin transporter expression in intestinal epithelial cells and mice intestinal tissues. Neurogastroenterol Motility. (2015) 27:1239–48. doi: 10.1111/nmo.12615
134. Wang B, Hylwka T, Smieja M, Surrette M, Bowdish DME, Loeb M. Probiotics to prevent respiratory infections in nursing homes: a pilot randomized controlled trial. J Am Geriatrics Soc. (2018) 66:1346–52. doi: 10.1111/jgs.15396
135. Asama T, Kimura Y, Kono T, Tatefuji T, Hashimoto K, Benno Y. Effects of heat-killed Lactobacillus kunkeei YB38 on human intestinal environment and bowel movement: a pilot study. Benef Microbes. (2016) 7:337–44. doi: 10.3920/BM2015.0132
136. Asama T, Uematsu T, Kobayashi N, Tatefuji T, Hashimoto K. Oral administration of heat-killed Lactobacillus kunkeei YB38 improves murine influenza pneumonia by enhancing IgA production. Biosci Microbiota Food Health. (2017) 36:1–9. doi: 10.12938/bmfh.16-010
137. Li Y-T, Xu H, Ye J-Z, Wu W-R, Shi D, Fang D-Q, et al. Efficacy of Lactobacillus rhamnosus GG in treatment of acute pediatric diarrhea: A systematic review with meta-analysis. World J Gastroenterol. (2019) 25:4999–5016. doi: 10.3748/wjg.v25.i33.4999
138. Jiang Y, Ye L, Cui Y, Yang G, Yang W, Wang J, et al. Effects of Lactobacillus rhamnosus GG on the maturation and differentiation of dendritic cells in rotavirus-infected mice. Benef Microbes. (2017) 8:645–56. doi: 10.3920/BM2016.0157
139. Savino F, Montanari P, Galliano I, Daprà V, Bergallo M. Lactobacillus rhamnosus GG (ATCC 53103) for the management of infantile colic: a randomized controlled trial. Nutrients. (2020) 12:1693. doi: 10.3390/nu12061693
140. Kane AF, Bhatia AD, Denning PW, Shane AL, Patel RM. Routine supplementation of Lactobacillus rhamnosus GG and risk of necrotizing enterocolitis in very low birth weight infants. J Pediatrics. (2018) 195:73–9.e2. doi: 10.1016/j.jpeds.2017.11.055
141. Groele L, Szajewska H, Szypowska A. Effects of Lactobacillus rhamnosus GG and Bifidobacterium lactis Bb12 on beta-cell function in children with newly diagnosed type 1 diabetes: protocol of a randomised controlled trial. BMJ Open. (2017) 7:e017178. doi: 10.1136/bmjopen-2017-017178
142. Spacova I, Petrova MI, Fremau A, Pollaris L, Vanoirbeek J, Ceuppens JL, et al. Intranasal administration of probiotic Lactobacillus rhamnosus GG prevents birch pollen-induced allergic asthma in a murine model. Allergy. (2019) 74:100–10. doi: 10.1111/all.13502
143. Han X, Lee A, Huang S, Gao J, Spence JR, Owyang C. Lactobacillus rhamnosus GG prevents epithelial barrier dysfunction induced by interferon-gamma and fecal supernatants from irritable bowel syndrome patients in human intestinal enteroids and colonoids. Gut Microbes. (2019) 10:59–76. doi: 10.1080/19490976.2018.1479625
144. Gatej SM, Marino V, Bright R, Fitzsimmons TR, Gully N, Zilm P, et al. Probiotic Lactobacillus rhamnosus GG prevents alveolar bone loss in a mouse model of experimental periodontitis. J Clin Periodontol. (2018) 45:204–12. doi: 10.1111/jcpe.12838
145. Sabbatini S, Monari C, Ballet N, Decherf AC, Bozza S, Camilloni B, et al. Anti-biofilm properties of Saccharomyces cerevisiae CNCM I-3856 and Lacticaseibacillus rhamnosus ATCC 53103 probiotics against G. vaginalis. Microorganisms. (2020) 8:91294. doi: 10.3390/microorganisms8091294
146. Kumova OK, Fike AJ, Thayer JL, Nguyen LT, Mell JC, Pascasio J, et al. Lung transcriptional unresponsiveness and loss of early influenza virus control in infected neonates is prevented by intranasal Lactobacillus rhamnosus GG. PLoS Pathog. (2019) 15:e1008072. doi: 10.1371/journal.ppat.1008072
147. Strauss M, Mičetić-Turk D, Pogačar MŠ, Fijan S. Probiotics for the prevention of acute respiratory-tract infections in older people: systematic review. Healthcare. (2021) 9:690. doi: 10.3390/healthcare9060690
148. Harata G, Yoda K, Wang R, Miyazawa K, Sato M, He F, et al. Species- and age/generation-dependent adherence of bifidobacterium bifidum to human intestinal mucus in vitro. Microorganisms. (2021) 9:542. doi: 10.3390/microorganisms9030542
149. Pagnini C, Corleto VD, Martorelli M, Lanini C, D'Ambra G, Di Giulio E, et al. Mucosal adhesion and anti-inflammatory effects of Lactobacillus rhamnosus GG in the human colonic mucosa: A proof-of-concept study. World J Gastroenterol. (2018) 24:4652–62. doi: 10.3748/wjg.v24.i41.4652
150. Hibberd PL, Kleimola L, Fiorino AM, Botelho C, Haverkamp M, Andreyeva I, et al. No evidence of harms of probiotic Lactobacillus rhamnosus GG ATCC 53103 in healthy elderly-a phase I open label study to assess safety, tolerability and cytokine responses. PLoS ONE. (2014) 9:e113456. doi: 10.1371/journal.pone.0113456
151. Vilander AC, Dean GA. Adjuvant strategies for lactic acid bacterial mucosal vaccines. Vaccines. (2019) 7:150. doi: 10.3390/vaccines7040150
152. Ciorba MA, Riehl TE, Rao MS, Moon C, Ee X, Nava GM, et al. Lactobacillus probiotic protects intestinal epithelium from radiation injury in a TLR-2/cyclo-oxygenase-2-dependent manner. Gut. (2012) 61:829–38. doi: 10.1136/gutjnl-2011-300367
153. Shi C-w, Cheng M-y, Yang X, Lu Y-y, Yin H-d, Zeng Y, et al. Probiotic Lactobacillus rhamnosus GG promotes mouse gut microbiota diversity and T cell differentiation. Front Microbiol. (2020) 11:e607735. doi: 10.3389/fmicb.2020.607735
154. Zhang LY, Liu S, Zhao XJ, Wang N, Jiang X, Xin HS, et al. Lactobacillus rhamnosus GG modulates gastrointestinal absorption, excretion patterns, and toxicity in Holstein calves fed a single dose of aflatoxin B1. J Dairy Sci. (2019) 102:1330–40. doi: 10.3168/jds.2018-15444
155. Gamallat Y, Meyiah A, Kuugbee ED, Hago AM, Chiwala G, Awadasseid A, et al. Lactobacillus rhamnosus induced epithelial cell apoptosis, ameliorates inflammation and prevents colon cancer development in an animal model. Biomed Pharmacother. (2016) 83:536–41. doi: 10.1016/j.biopha.2016.07.001
156. Nermes M, Kantele JM, Atosuo TJ, Salminen S, Isolauri E. Interaction of orally administered Lactobacillus rhamnosus GG with skin and gut microbiota and humoral immunity in infants with atopic dermatitis. Clin Experi Allergy. (2011) 41:370–7. doi: 10.1111/j.1365-2222.2010.03657.x
157. Kopp MV, Hennemuth I, Heinzmann A, Urbanek R. Randomized, double-blind, placebo-controlled trial of probiotics for primary prevention: no clinical effects of Lactobacillus GG supplementation. Pediatrics. (2008) 121:e850–6. doi: 10.1542/peds.2007-1492
158. Sawada J, Morita H, Tanaka A, Salminen S, He F, Matsuda H. Ingestion of heat-treated Lactobacillus rhamnosus GG prevents development of atopic dermatitis in NC/Nga mice. Clin Experi Allergy. (2007) 37:296–303. doi: 10.1111/j.1365-2222.2006.02645.x
159. Majamaa H, Isolauri E. Probiotics: a novel approach in the management of food allergy. J Allergy Clin Immunol. (1997) 99:179–85. doi: 10.1016/S0091-6749(97)70093-9
160. Chen RC, Xu LM, Du SJ, Huang SS, Wu H, Dong JJ, et al. Lactobacillus rhamnosus GG supernatant promotes intestinal barrier function, balances Treg and TH17 cells and ameliorates hepatic injury in a mouse model of chronic-binge alcohol feeding. Toxicol Lett. (2016) 241:103–10. doi: 10.1016/j.toxlet.2015.11.019
161. Kim B, Park KY, Ji Y, Park S, Holzapfel W, Hyun CK. Protective effects of Lactobacillus rhamnosus GG against dyslipidemia in high-fat diet-induced obese mice. Biochem Biophys Res Commun. (2016) 473:530–6. doi: 10.1016/j.bbrc.2016.03.107
162. Wang Y, Liu Y, Sidhu A, Ma Z, McClain C, Feng W. Lactobacillus rhamnosus GG culture supernatant ameliorates acute alcohol-induced intestinal permeability and liver injury. Am J Physiol Gastrointest Liver Physiol. (2012) 303:G32–41. doi: 10.1152/ajpgi.00024.2012
163. Wang Y, Liu Y, Kirpich I, Ma Z, Wang C, Zhang M, et al. Lactobacillus rhamnosus GG reduces hepatic TNFα production and inflammation in chronic alcohol-induced liver injury. J Nutr Biochem. (2013) 24:1609–15. doi: 10.1016/j.jnutbio.2013.02.001
164. Wang Y, Kirpich I, Liu Y, Ma Z, Barve S, McClain CJ, et al. Lactobacillus rhamnosus GG treatment potentiates intestinal hypoxia-inducible factor, promotes intestinal integrity and ameliorates alcohol-induced liver injury. Am J Pathol. (2011) 179:2866–75. doi: 10.1016/j.ajpath.2011.08.039
165. Ritze Y, Bárdos G, Claus A, Ehrmann V, Bergheim I, Schwiertz A, et al. Lactobacillus rhamnosus GG protects against non-alcoholic fatty liver disease in mice. PLoS ONE. (2014) 9:e80169. doi: 10.1371/journal.pone.0080169
166. Forsyth CB, Farhadi A, Jakate SM, Tang Y, Shaikh M, Keshavarzian A. Lactobacillus GG treatment ameliorates alcohol-induced intestinal oxidative stress, gut leakiness, and liver injury in a rat model of alcoholic steatohepatitis. Alcohol (Fayetteville, NY). (2009) 43:163–72. doi: 10.1016/j.alcohol.2008.12.009
167. Sindhu KN, Sowmyanarayanan TV, Paul A, Babji S, Ajjampur SS, Priyadarshini S, et al. Immune response and intestinal permeability in children with acute gastroenteritis treated with Lactobacillus rhamnosus GG: a randomized, double-blind, placebo-controlled trial. Clin Infect Dis. (2014) 58:1107–15. doi: 10.1093/cid/ciu065
168. Alanzi A, Honkala S, Honkala E, Varghese A, Tolvanen M, Söderling E. Effect of Lactobacillus rhamnosus and Bifidobacterium lactis on gingival health, dental plaque, and periodontopathogens in adolescents: a randomised placebo-controlled clinical trial. Benef Microbes. (2018) 9:593–602. doi: 10.3920/BM2017.0139
169. Polak D, Wilensky A, Shapira L, Halabi A, Goldstein D, Weiss EI, et al. Mouse model of experimental periodontitis induced by Porphyromonas gingivalis/Fusobacterium nucleatum infection: bone loss and host response. J Clin Periodontol. (2009) 36:406–10. doi: 10.1111/j.1600-051X.2009.01393.x
170. Wen K, Tin C, Wang H, Yang X, Li G, Giri-Rachman E, et al. Probiotic Lactobacillus rhamnosus GG enhanced Th1 cellular immunity but did not affect antibody responses in a human gut microbiota transplanted neonatal gnotobiotic pig model. PLoS ONE. (2014) 9:e94504. doi: 10.1371/journal.pone.0094504
171. Capurso L. Thirty years of Lactobacillus rhamnosus GG: A review. J Clin Gastroenterol. (2019) 53 Suppl 1: S1–s41. doi: 10.1097/MCG.0000000000001170
172. Szajewska H, Kołodziej M. Systematic review with meta-analysis: Lactobacillus rhamnosus GG in the prevention of antibiotic-associated diarrhoea in children and adults. Alimentary Pharmacol Therap. (2015) 42:1149–57. doi: 10.1111/apt.13404
173. Korpela K, Salonen A, Virta LJ, Kumpu M, Kekkonen RA, de Vos WM. Lactobacillus rhamnosus GG intake modifies preschool children's intestinal microbiota, alleviates penicillin-associated changes, and reduces antibiotic use. PLoS ONE. (2016) 11:e0154012. doi: 10.1371/journal.pone.0154012
174. Yeung C-Y, Chiang Chiau J-S, Chan W-T, Jiang C-B, Cheng M-L, Liu H-L, et al. In vitro prevention of Salmonella lipopolysaccharide-induced damages in epithelial barrier function by various Lactobacillus Strains. Gastroenterol Res Pract. (2013) 2013:1–6. doi: 10.1155/2013/973209
175. De Keersmaecker SC, Verhoeven TL, Desair J, Marchal K, Vanderleyden J, Nagy I. Strong antimicrobial activity of Lactobacillus rhamnosus GG against Salmonella typhimurium is due to accumulation of lactic acid. FEMS Microbiol Lett. (2006) 259:89–96. doi: 10.1111/j.1574-6968.2006.00250.x
176. Liu J, Gu Z, Song F, Zhang H, Zhao J, Chen W. Lactobacillus plantarum ZS2058 and Lactobacillus rhamnosus GG Use different mechanisms to prevent salmonella infection in vivo. Front Microbiol. (2019) 10:299. doi: 10.3389/fmicb.2019.00299
177. Urcan AC, Criste AD, Dezmirean DS, Bobiş O, Victorit, a B, Dulf FV, et al. Botanical origin approach for a better understanding of chemical and nutritional composition of beebread as an important value-added food supplement. LWT. (2021) 2021:111068. doi: 10.1016/j.lwt.2021.111068
178. Pełka K, Otłowska O, Worobo RW, Szweda P. Bee bread exhibits higher antimicrobial potential compared to bee pollen. Antibiotics. (2021) 10:125. doi: 10.3390/antibiotics10020125
179. Pełka K, Worobo RW, Walkusz J, Szweda P. Bee pollen and bee bread as a source of bacteria producing antimicrobials. Antibiotics. (2021) 10:713. doi: 10.3390/antibiotics10060713
180. Kim KS. Current concepts on the pathogenesis of Escherichia coli meningitis: implications for therapy and prevention. Curr Opin Infect Dis. (2012) 25:273–8. doi: 10.1097/QCO.0b013e3283521eb0
181. He X, Zeng Q, Puthiyakunnon S, Zeng Z, Yang W, Qiu J, et al. Lactobacillus rhamnosus GG supernatant enhance neonatal resistance to systemic Escherichia coli K1 infection by accelerating development of intestinal defense. Sci Rep. (2017) 7:43305. doi: 10.1038/srep43305
182. Harata G, He F, Hiruta N, Kawase M, Kubota A, Hiramatsu M, et al. Intranasal administration of Lactobacillus rhamnosus GG protects mice from H1N1 influenza virus infection by regulating respiratory immune responses. Lett Appl Microbiol. (2010) 50:597–602. doi: 10.1111/j.1472-765X.2010.02844.x
183. Ispirli H, Dertli E. Detection of fructophilic lactic acid bacteria (FLAB) in bee bread and bee pollen samples and determination of their functional roles. J Food Process Preserv. (2021) 45:e15414. doi: 10.1111/jfpp.15414
184. Premaor MO, Compston JE. The hidden burden of fractures in people living with HIV. JBMR Plus. (2018) 2:247–56. doi: 10.1002/jbm4.10055
185. Post FA, Hamzah L, Fox J. Tenofovir disoproxil fumarate-associated bone loss: does vitamin D-binding protein play a role? AIDS. (2017) 31:178–9. doi: 10.1097/QAD.0000000000001288
186. Ohlsson C, Sjögren K. Effects of the gut microbiota on bone mass. Trends Endocrinol Metab. (2015) 26:69–74. doi: 10.1016/j.tem.2014.11.004
187. Liu H, Gu R, Li W, Zhou W, Cong Z, Xue J, et al. Lactobacillus rhamnosus GG attenuates tenofovir disoproxil fumarate-induced bone loss in male mice via gut-microbiota-dependent anti-inflammation. Therap Adv Chronic Dis. (2019) 10:2040622319860653. doi: 10.1177/2040622319860653
188. Gatej SM, Bright R, Weyrich LS, Marino V, Christophersen CT, Gibson RJ, et al. Probiotic Lactobacillus Rhamnosus GG Protects Against P. Gingivalis and F. Nucleatum gut dysbiosis. J Int Acad Periodontol. (2020) 22:18–27.
189. Sapra L, Dar HY, Bhardwaj A, Pandey A, Kumari S, Azam Z, et al. Lactobacillus rhamnosus attenuates bone loss and maintains bone health by skewing Treg-Th17 cell balance in Ovx mice. Sci Rep. (2021) 11:80536. doi: 10.1038/s41598-020-80536-2
190. Zhao H, Zhao C, Dong Y, Zhang M, Wang Y, Li F, et al. Inhibition of miR122a by Lactobacillus rhamnosus GG culture supernatant increases intestinal occludin expression and protects mice from alcoholic liver disease. Toxicol Lett. (2015) 234:194–200. doi: 10.1016/j.toxlet.2015.03.002
191. Liu Y, Chen K, Li F, Gu Z, Liu Q, He L, et al. Probiotic Lactobacillus rhamnosus GG prevents liver fibrosis through inhibiting hepatic bile acid synthesis and enhancing bile acid excretion in mice. Hepatology (Baltimore, Md). (2020) 71:2050–66. doi: 10.1002/hep.30975
192. Owens JA, Saeedi BJ, Naudin CR, Hunter-Chang S, Barbian ME, Eboka RU, et al. Lactobacillus rhamnosus GG orchestrates an antitumor immune response. Cell Mol Gastroenterol Hepatol. (2021) 12:1311–27. doi: 10.1016/j.jcmgh.2021.06.001
193. Oksaharju A. Probiotic Lactobacillus rhamnosus downregulates FCER1 and HRH4 expression in human mast cells. World J Gastroenterol. (2011) 17:750. doi: 10.3748/wjg.v17.i6.750
194. Goyal N, Shukla G. Probiotic Lactobacillus rhamnosus GG modulates the mucosal immune response in Giardia intestinalis-infected BALB/c mice. Digest Dis Sci. (2013) 58:1218–25. doi: 10.1007/s10620-012-2503-y
195. Menezes C, Paludo CR, Pupo MT. A review of the artificial diets used as pot-pollen substitutes. Pot-Pollen Stingless Bee Melittol. (2018) 2018:253–62. doi: 10.1007/978-3-319-61839-5_18
196. Ouwehand AC. Antiallergic effects of probiotics. J Nutr. (2007) 137: 794S−7S. doi: 10.1093/jn/137.3.794S
197. Blümer N, Sel S, Virna S, Patrascan CC, Zimmermann S, Herz U, et al. Perinatal maternal application of Lactobacillus rhamnosus GG suppresses allergic airway inflammation in mouse offspring. Clin Experi Allergy. (2007) 37:348–57. doi: 10.1111/j.1365-2222.2007.02671.x
198. Feleszko W, Jaworska J, Rha RD, Steinhausen S, Avagyan A, Jaudszus A, et al. Probiotic-induced suppression of allergic sensitization and airway inflammation is associated with an increase of T regulatory-dependent mechanisms in a murine model of asthma. Clin Experi Allergy. (2007) 37:498–505. doi: 10.1111/j.1365-2222.2006.02629.x
199. Thang CL, Baurhoo B, Boye JI, Simpson BK, Zhao X. Effects of Lactobacillus rhamnosus GG supplementation on cow's milk allergy in a mouse model. Allergy, Asthma Clin Immunol. (2011) 7:20. doi: 10.1186/1710-1492-7-20
200. Kalliomäki M, Salminen S, Poussa T, Arvilommi H, Isolauri E. Probiotics and prevention of atopic disease: 4-year follow-up of a randomised placebo-controlled trial. Lancet. (2003) 361:1869–71. doi: 10.1016/S0140-6736(03)13490-3
201. Kukkonen K, Savilahti E, Haahtela T, Juntunen-Backman K, Korpela R, Poussa T, et al. Probiotics and prebiotic galacto-oligosaccharides in the prevention of allergic diseases: A randomized, double-blind, placebo-controlled trial. J Allergy Clin Immunol. (2007) 119:192–8. doi: 10.1016/j.jaci.2006.09.009
202. Simpson MR, Dotterud CK, Storrø O, Johnsen R, Øien T. Perinatal probiotic supplementation in the prevention of allergy related disease: 6 year follow up of a randomised controlled trial. BMC Dermatol. (2015) 15:13. doi: 10.1186/s12895-015-0030-1
203. Wu CT, Chen PJ, Lee YT, Ko JL, Lue KH. Effects of immunomodulatory supplementation with Lactobacillus rhamnosus on airway inflammation in a mouse asthma model. J Microbiol Immunol Infect. (2016) 49:625–35. doi: 10.1016/j.jmii.2014.08.001
204. Wu CT, Lin FH, Lee YT, Ku MS, Lue KH. Effect of Lactobacillus rhamnosus GG immunopathologic changes in chronic mouse asthma model. J Microbiol Immunol Infect. (2019) 52:911–9. doi: 10.1016/j.jmii.2019.03.002
205. Kuitunen M, Kukkonen K, Juntunen-Backman K, Korpela R, Poussa T, Tuure T, et al. Probiotics prevent IgE-associated allergy until age 5 years in cesarean-delivered children but not in the total cohort. J Allergy Clin Immunol. (2009) 123:335–41. doi: 10.1016/j.jaci.2008.11.019
206. Betsi GI, Papadavid E, Falagas ME. Probiotics for the treatment or prevention of atopic dermatitis: a review of the evidence from randomized controlled trials. Am J Clin Dermatol. (2008) 9:93–103. doi: 10.2165/00128071-200809020-00002
207. Sepp E, Julge K, Vasar M, Naaber P, Björksten B, Mikelsaar M. Intestinal microflora of Estonian and Swedish infants. Acta Paediatri. (1997) 86:956–61. doi: 10.1111/j.1651-2227.1997.tb15178.x
208. Kalliomäki M, Salminen S, Arvilommi H, Kero P, Koskinen P, Isolauri E. Probiotics in primary prevention of atopic disease: a randomised placebo-controlled trial. Lancet (London, England). (2001) 357:1076–9. doi: 10.1016/S0140-6736(00)04259-8
209. Kalliomäki M, Salminen S, Poussa T, Isolauri E. Probiotics during the first 7 years of life: a cumulative risk reduction of eczema in a randomized, placebo-controlled trial. J Allergy Clin Immunol. (2007) 119:1019–21. doi: 10.1016/j.jaci.2006.12.608
210. Rautava S, Kalliomäki M, Isolauri E. Probiotics during pregnancy and breast-feeding might confer immunomodulatory protection against atopic disease in the infant. J Allergy Clin Immunol. (2002) 109:119–21. doi: 10.1067/mai.2002.120273
211. Fölster-Holst R, Müller F, Schnopp N, Abeck D, Kreiselmaier I, Lenz T, et al. Prospective, randomized controlled trial on Lactobacillus rhamnosus in infants with moderate to severe atopic dermatitis. Br J Dermatol. (2006) 155:1256–61. doi: 10.1111/j.1365-2133.2006.07558.x
212. Sistek D, Kelly R, Wickens K, Stanley T, Fitzharris P, Crane J. Is the effect of probiotics on atopic dermatitis confined to food sensitized children? Clin Experi Allergy. (2006) 36:629–33. doi: 10.1111/j.1365-2222.2006.02485.x
213. Boyle RJ, Ismail IH, Kivivuori S, Licciardi PV, Robins-Browne RM, Mah LJ, et al. Lactobacillus GG treatment during pregnancy for the prevention of eczema: a randomized controlled trial. Allergy. (2011) 66:509–16. doi: 10.1111/j.1398-9995.2010.02507.x
214. Brouwer ML, Wolt-Plompen SA, Dubois AE, van der Heide S, Jansen DF, Hoijer MA, et al. No effects of probiotics on atopic dermatitis in infancy: a randomized placebo-controlled trial. Clin Experi Allergy. (2006) 36:899–906. doi: 10.1111/j.1365-2222.2006.02513.x
215. Dotterud CK, Storrø O, Johnsen R, Oien T. Probiotics in pregnant women to prevent allergic disease: a randomized, double-blind trial. Br J Dermatol. (2010) 163:616–23. doi: 10.1111/j.1365-2133.2010.09889.x
216. Filipovic I, Ostojic O, Vekovic V, Lackovic M, Zivkovic Z. Combination of Lactobacillus Rhamnosus LGG, Vitamin D3 and Zn in preventing atopic dermatitis in infancy. Am J Pediatrics. (2020) 6:273. doi: 10.11648/j.ajp.20200603.26
217. Vamanu A, Vamanu E, Popa O, Campeanu G, Albulescu R, Drugulescu M, et al. Obtaining of a symbiotic product based on lactic bacteria, pollen and honey. Pak J Biol Sci. (2008) 11:613–7.
218. Darby TM, Naudin CR, Luo L, Jones RM. Lactobacillus rhamnosus GG-induced expression of leptin in the intestine orchestrates epithelial cell proliferation. Cell Mol Gastroenterol Hepatol. (2020) 9:627–39. doi: 10.1016/j.jcmgh.2019.12.004
219. Fuenmayor CA, Quicazán MC, Figueroa J. Desarrollo De Un Suplemento Nutricional Mediante La Fermentación En Fase Sólida De Polen De Abejas Empleando Bacterias Ácido Lácticas Probióticas. (2011).
220. Groah SL, Rounds AK, Ljungberg IH, Sprague BM, Frost JK, Tractenberg RE. Intravesical Lactobacillus rhamnosus GG is safe and well tolerated in adults and children with neurogenic lower urinary tract dysfunction: first-in-human trial. Therap Adv Urol. (2019) 11:1756287219875594. doi: 10.1177/1756287219875594
221. Forster CS, Hsieh MH, Pérez-Losada M, Caldovic L, Pohl H, Ljungberg I, et al. A single intravesical instillation of Lactobacillus rhamnosus GG is safe in children and adults with neuropathic bladder: A phase Ia clinical trial. J Spinal Cord Med. (2021) 44:62–9. doi: 10.1080/10790268.2019.1616456
222. Hasler CM. Functional foods: benefits, concerns and challenges—a position paper from the American council on science and health. J Nutr. (2002) 132:3772–81. doi: 10.1093/jn/132.12.3772
223. European Union. Honey. Detailed Information on Honey Production, National Apiculture Programmes, Budget and Legal Bases. (2021).
224. Capozzi V, Fragasso M, Russo P. Microbiological safety and the management of microbial resources in artisanal foods and beverages: the need for a transdisciplinary assessment to conciliate actual trends and risks avoidance. Microorganisms. (2020) 8:20306. doi: 10.3390/microorganisms8020306
225. Marshall E, Mejia D. Traditional Fermented Food and Beverages for Improved Livelihoods. Rome: FAO Diversification booklet; Rural Infrastructure and Agro-Industries Division, Food and Agriculture Organization of the United Nations (2011).
226. Melini F, Melini V, Luziatelli F, Ficca AG, Ruzzi M. Health-promoting components in fermented foods: an up-to-date systematic review. Nutrients. (2019) 11:1189. doi: 10.3390/nu11051189
227. Capozzi V, Fragasso M, Romaniello R, Berbegal C, Russo P, Spano G. Spontaneous food fermentations and potential risks for human health. Fermentation. (2017) 3:49. doi: 10.3390/fermentation3040049
228. Tamang JP, Watanabe K, Holzapfel WH. Review: diversity of microorganisms in global fermented foods and beverages. Front Microbiol. (2016) 7:377. doi: 10.3389/fmicb.2016.00377
229. Berbegal C, Garofalo C, Russo P, Pati S, Capozzi V, Spano G. Use of autochthonous yeasts and bacteria in order to control brettanomyces bruxellensis in wine. Fermentation. (2017) 3:65. doi: 10.3390/fermentation3040065
Keywords: bee bread, lactic acid bacteria, fermented product, food supplement, a probiotic product
Citation: Barta DG, Cornea-Cipcigan M, Margaoan R and Vodnar DC (2022) Biotechnological Processes Simulating the Natural Fermentation Process of Bee Bread and Therapeutic Properties—An Overview. Front. Nutr. 9:871896. doi: 10.3389/fnut.2022.871896
Received: 08 February 2022; Accepted: 21 March 2022;
Published: 27 April 2022.
Edited by:
Sandrina A. Heleno, Polytechnic Institute of Bragança (IPB), PortugalReviewed by:
Elena Sorrentino, University of Molise, ItalyFilomena Nazzaro, National Research Council (CNR), Italy
Copyright © 2022 Barta, Cornea-Cipcigan, Margaoan and Vodnar. This is an open-access article distributed under the terms of the Creative Commons Attribution License (CC BY). The use, distribution or reproduction in other forums is permitted, provided the original author(s) and the copyright owner(s) are credited and that the original publication in this journal is cited, in accordance with accepted academic practice. No use, distribution or reproduction is permitted which does not comply with these terms.
*Correspondence: Dan Cristian Vodnar, dan.vodnar@usamvcluj.ro; Rodica Margaoan, rodica.margaoan@usamvcluj.ro
†These authors have contributed equally to this work and share first authorship