Modulation of Gut Microbiota and Oxidative Status by β-Carotene in Late Pregnant Sows
- 1College of Animal Science and Technology, Hunan Agricultural University, Changsha, China
- 2Hunan Xinguang'an Agricultural Husbandry Co., Ltd., Changsha, China
- 3Department of Food Science and Biotechnology, Faculty of Agriculture, Kagoshima University, Kagoshima, Japan
Recent evidences suggest that gut microbiota plays an important role in regulating physiological and metabolic activities of pregnant sows, and β-carotene has a potentially positive effect on reproduction, but the impact of β-carotene on gut microbiota in pregnant sows remains unknown. This study aimed to explore the effect and mechanisms of β-carotene on the reproductive performance of sows from the aspect of gut microbiota. A total of 48 hybrid pregnant sows (Landrace × Yorkshire) with similar parity were randomly allocated into three groups (n = 16) and fed with a basal diet or a diet containing 30 or 90 mg/kg of β-carotene from day 90 of gestation until parturition. Dietary supplementation of 30 or 90 mg/kg β-carotene increased the number of live birth to 11.82 ± 1.54 and 12.29 ± 2.09, respectively, while the control group was 11.00 ± 1.41 (P = 0.201). Moreover, β-carotene increased significantly the serum nitric oxide (NO) level and glutathione peroxidase (GSH-Px) activity (P < 0.05). Characterization of fecal microbiota revealed that 90 mg/kg β-carotene increased the diversity of the gut flora (P < 0.05). In particular, β-carotene decreased the relative abundance of Firmicutes including Lachnospiraceae AC2044 group, Lachnospiraceae NK4B4 group and Ruminococcaceae UCG-008, but enriched Proteobacteria including Bilophila and Sutterella, and Actinobacteria including Corynebacterium and Corynebacterium 1 which are related to NO synthesis. These data demonstrated that dietary supplementation of β-carotene may increase antioxidant enzyme activity and NO, an important vasodilator to promote the neonatal blood circulation, through regulating gut microbiota in sows.
Introduction
Beta-(β-)carotene is a widely distributed phytochromes (1), and is generally considered as a precursor of vitamin A (2, 3). It belongs to the fat-soluble substance which is incorporated into chylomicrons and absorbed in the intestine through passive diffusion (4). When ingested into the intestine, β-carotene is partially transformed into vitamin A, and the remaining β-carotene is passed by blood circulation to target organs such as the liver, ovary, adipose tissue and adrenal gland (5). Around 17–45% of β-carotene can be absorbed into the circulatory system (1). The importance of vitamin A in female reproductive health has been well-documented (6). Previous studies have found a high concentration of β-carotene in the luteum, and the lack of β-carotene results in delayed ovulation, luteal phase defect, and increased risk of ovarian cyst (1, 7). Moreover, the secretion of pregnancy hormones is linked to serum β-carotene concentrations, indicating that β-carotene can play an important role in reproduction (8, 9).
Multiple studies have shown that low-level inflammation, progressive oxidative stress, and metabolic disorders occur during the perinatal period. During pregnancy, the rapid cell proliferation of the uterus and the placenta, growth of the fetus, and childbirth will progressively increase the reactive oxygen species (ROS) and decrease the antioxidant capacity of the body (10). The pro-inflammatory interleukins (ILs) such as IL-6 can be substantially increased in maternal serum as pregnancy progresses (11). Furthermore, insulin resistance during pregnancy will decrease glucose utilization, while excessive energy intake and obesity during pregnancy will exacerbate maternal inflammation and oxidants stress, thereby triggering insulin resistance and having adverse effects on pregnancy (12). Recent studies have shown that significant change in the gut microbiota during different periods of pregnancy can affect the physiological state and metabolic process of host, indicating that gut microbiota plays a vital role during pregnancy (13, 14). In modern pig farming industry, constipation occurs frequently in pregnant sows due to intestinal disorder, which is largely determined by the dysfunction of gut microbiota. β-carotene has been considered as a potent inhibitor of oxidative stress and inflammation both in vitro and in vivo (15, 16), and it can suppress the expression of proinflammatory cytokines such as IL-1β and IL-6 to alleviate inflammation and oxidative stress induced by ischemia injury (17). However, it is not clear whether β-carotene exerts biological functions by modulating the gut microbiota.
Therefore, this study aimed to investigate the effect of β-carotene on the reproductive performance of sows, and challenged to clarify the potential mechanisms from the aspect of gut microbiota.
Materials and Methods
Experimental Design and Diets
The animal model and experimental procedures used in this experiment were approved by the Hunan Agricultural University Institutional Animal Care and Use Committee (No. 201903). A total of 48 hybrid pregnant sows (Landrace × Yorkshire), with similar parity (3–7 fetuses) were used in this study. The experimental animals which kept in gestation stalls with fully slatted floors measuring under environment temperature and had free access to water, were randomly allocated into three treatments (n = 16). Based on previous studies, sows in the treatments were fed with a basal diet (control group, CTL), a diet containing 30 mg/kg of β-carotene (β-carotene low dose group, CAR-L), or a diet containing 90 mg/kg of β-carotene (β-carotene high dose group, CAR-H), respectively, based on previous studies (8, 18). The sows were fed at 6:00 a.m., 12:00 p.m., and 6:00 p.m. with ~3.2 kg of feed/sow/day. The experiment started on day 90 of gestation and continued until delivery. The composition of the basal diet, which meets the nutritional requirements of pigs according to NRC (2012), was shown in Supplementary Table 1.
Reproductive Performance Markers
The number of live birth, litter weight at parturition and average weight of piglets born alive were measured within 24 h after farrowing.
Sample Collections
At the day of parturition, blood samples (5 mL) were collected from the marginal auricular vein into anticoagulant-free vacuum tubes and centrifuged on 1,500 × g for 10 min after standing at room temperature for 30 min to get the serum. Fecal samples (around 2 g from each sow) were collected from the innermost of feces into sterile tubes after defecation in the morning, and snap-frozen in liquid nitrogen before storage at −80°C for further DNA extraction.
Measurement of Serum Biochemical Indices
Total antioxidant capacity (T-AOC), total superoxide dismutase (T-SOD) activity, glutathione peroxidase (GSH-Px) activity, the level of thiobarbituric acid reactive substances (TBARS), and the nitric oxide (NO) level were determined in serum by using respective assay kits (Nanjing Jiancheng Bioengineering Institute, Nanjing, China) according to the manufacturer's instructions as described previously (19). The levels of glucose (GLU, 0.06–27.8 mmol/L), total protein (TP, 1.74–100 g/L), total cholesterol (TC, 0.09–25.85 mmol/L), triglycerides (TG, 0.05–11.3 mmol/L), high-density lipoproteins (HDL-c, 0.065–3.8 mmol/L), low-density lipoprotein cholesterol (LDL-c, 0.2–12 mmol/L), immunoglobulin G (IgG, 0.25–35 g/L) and immunoglobulin M (IgM, 0.25–5.00 g/L) were measured with respective kits from Mindray Medical International Ltd., China by using an automated biochemical analyser BS-200 (Mindray, China).
Characterization of Gut Microbiota
Fecal microbiota was characterized by 16S rRNA gene sequencing. Briefly, total DNA was extracted from fecal samples (six random samples from each group) by using a DNA Isolation Kit (MoBio Laboratories, Carlsbad, CA, USA) following the manufacturer's manual. Purity and quality of the genomic DNA were checked on 0.8% agarose gels. The V3–4 hypervariable region of the bacterial 16S rRNA gene was amplified with the primers 338F (5′-ACTCCTACGGGAGGCAGCA-3′) and 806R (5′-GGACTACHVGGGTWTCTAAT-3′). For each sample, 10-digit barcode sequence was added to the 5′ end of the forward and reverse primers (provided by Allwegene Technology Inc., Beijing, China). The PCR was carried out on a Mastercycler Gradient (Eppendorf, Germany) using 25 μL reaction volumes, containing 12.5 μL KAPA 2G Robust Hot Start Ready Mix, 1 μL Forward Primer (5 μmol/L), 1 μL Reverse Primer (5 μmol/L), 5 μL DNA (total template quantity is 30 ng), and 5.5 μL H2O. Cycling parameters were 95°C for 5 min, followed by 28 cycles of 95°C for 45 s, 55°C for 50 s, and 72°C for 45 s with a final extension at 72°C for 10 min. Three PCR products per sample were pooled to mitigate reaction-level PCR biases. The PCR products were purified using a QIAquick Gel Extraction Kit (QIAGEN, Germany), and quantified using Real Time PCR, and sequenced on Miseq platform at Allwegene Technology Inc., Beijing, China. After the run, image analysis, base calling and error estimation were performed using Illumina Analysis Pipeline Version 2.6. The raw data were first screened and sequences were removed from consideration if they were shorter than 200 bp, had a low quality score (≤ 20), contained ambiguous bases or did not exactly match to primer sequences and barcode tags. Qualified reads were separated using the sample-specific barcode sequences and trimmed with Illumina Analysis Pipeline Version 2.6. And then the dataset was analyzed using QIIME (Version 1.8.0). The sequences were clustered into operational taxonomic units (OTUs) at a similarity level of 97%, to generate rarefaction curves and to calculate the richness and diversity index. The Ribosomal Database Project (RDP) Classifier tool was used to classify all sequences into different taxonomic groups.
Statistical Analysis
Results were expressed as means ± SD. The significant differences between groups were analyzed by one-way analysis of variance tests, followed by Fisher's least significant difference (LSD) and Duncan's multiple range tests with the SPSS statistical program (SPSS19, IBM Corp., Armonk, NY, USA). A probability of P < 0.05 was considered significant.
Results
The Influence of β-Carotene on the Reproductive Performance of Sows
As shown in Table 1, the number of live birth was increased to 11.82 ± 1.54 and 12.29 ± 2.09 in CAR-L (30 mg/kg β-carotene) group and CAR-H (90 mg/kg β-carotene) group respectively, while the CTL group was 11.00 ± 1.41, although there had no statistical significance (P = 0.201). The litter weight at parturition showed a similar trend with the number of live birth, and the average weight of piglets born alive kept no change.
The Effect of β-Carotene on Serum Biochemical Markers in Sows
As progressive oxidative stress plays a negative role during the perinatal period, the oxidative stress markers including T-AOC, TBARS, GSH-Px, and T-SOD were measured in the serum of sows. As shown in Figure 1, the activity of GSH-Px (C) was increased significantly (P < 0.05) in both CAR-L (85.99 ± 4.75 U/mL) and CAR-H (86.8 ± 1.67 U/mL) group, as compared with CTL group (77.94 ± 4.54 U/mL). However, no significant differences were observed in the levels of T-AOC (A), MDA (B), and T-SOD (D) among the three groups (P > 0.05).
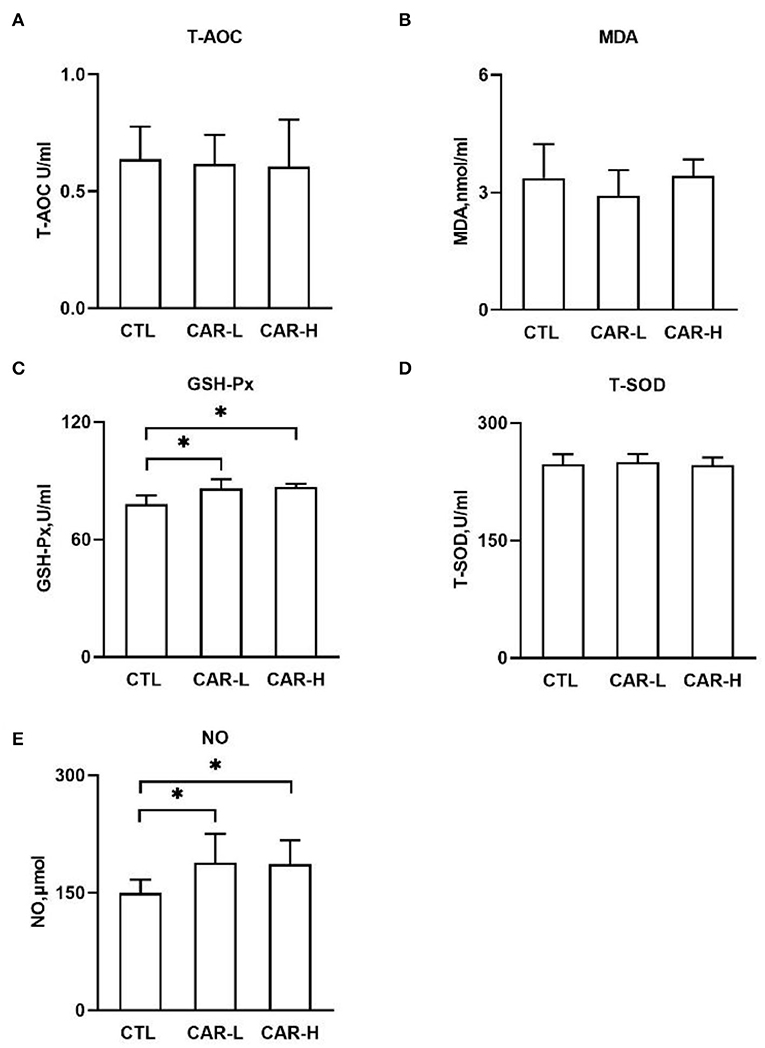
Figure 1. The effect of β-carotene on serum levels of antioxidant indicators and NO. Serum levels of T-AOC (A), TBARS (B), GSH-Px (C), T-SOD (D), and NO (E) were determined by using respective kits. CTL, a basal diet; CAR-L, a basal diet containing 30 mg/kg β-carotene; CAR-H, a basal diet containing 90 mg/kg β-carotene. Data were shown as means ± SD (n = 16), *P < 0.05.
Nitric oxide (NO) is a signaling molecule involved in oxidative stress and inflammation, and also considered as an important vasodilator to promote the neonatal blood circulation (14). Thus, NO was further measured in serum, and the results showed that the level of NO was increased to 188.33 ± 37.13 μmol and 186.95 ± 30.06 μmol in CAR-L and CAR-H group respectively, which were significantly (P < 0.05) higher than that in CTL group (149.54 ± 17.12 μmol) (Figure 1E).
In addition, indicators of immune response and glucolipid metabolism were also measured in serum due to their important roles in pregnancy. However, β-carotene had no significant effects on serum levels of immunoglobulins (IgM & IgG), lipids (Tc, Tg, HDL-c & LDL-c), Glu, and Tp (Supplementary Table 2).
Modulation of Gut Microbiota by β-Carotene
To gain an insight into the effect of β-carotene on gut microbiota, the composition and relative abundance of fecal microbiota was characterized by using high throughput 16S rRNA gene sequencing. As shown in Figure 2, supplementation of β-carotene dose-dependently increased the Shannon index (A) of the microbiota, and a significant difference was observed between CTL and CAR-H group (P < 0.05). Further analysis on the relative abundance of bacterial phyla showed that the microbial community was dominated by Firmicutes, Bacteroidetes, Proteobacteria, Spirochaetae, and Euryarchaeota, which account for 97% of total microbes, and supplementation of β-carotene increased the relative abundance of Proteobacteria.
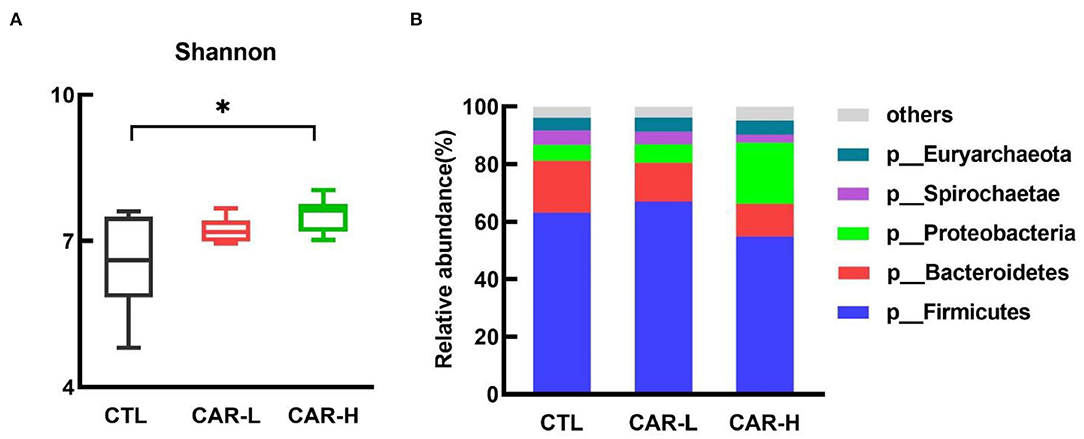
Figure 2. The effect of β-carotene on fecal microbial community. The effect of β-carotene on the Shannon index (A) and the relative abundances of microbial phyla (B) were analyzed by 16S rRNA gene sequencing. CTL, a basal diet; CAR-L, a basal diet containing 30 mg/kg β-carotene; CAR-H, a basal diet containing 90 mg/kg β-carotene. Data were shown as means ± SD (n = 6), *P < 0.05.
At the genus level, a total number of 299 microbial genera were analyzed, and nine genera were found to be significantly different among the three groups. As shown in Figure 3, β-carotene decreased the relative abundances of Lachnospiraceae AC2044 group (A), Lachnospiraceae NK4B4 group (B), and Ruminococcaceae UCG-008 (C), which belong to the phylum of Firmicutes. Meanwhile, β-carotene reduced the relative abundance of Prevotellaceae UCG-001 (D), a genera belong to the phylum of Bacteroidete. On the other hand, β-carotene increased the relative abundance of Sedimentibacter (E) belonging to the phylum of Firmicutes, Bilophila (F) and Sutterella (G) belonging to the phylum of Proteobacteria, as well as Corynebacterium 1 (H) and Corynebacterium (I) belonging to the phylum of Actinobacteria. Based on the results of gut microbiota and serum biochemical markers, the correlation between GSH-Px, NO and the changed microbial genera were further analyzed. The results showed that Corynebacterium 1 and Corynebacterium were positively correlated (P < 0.01) with the NO level, while Prevotellaceae UCG-001 and Lachnospiraceae AC2044 group were negatively correlated (P < 0.01) with the GSH-Px level (J).
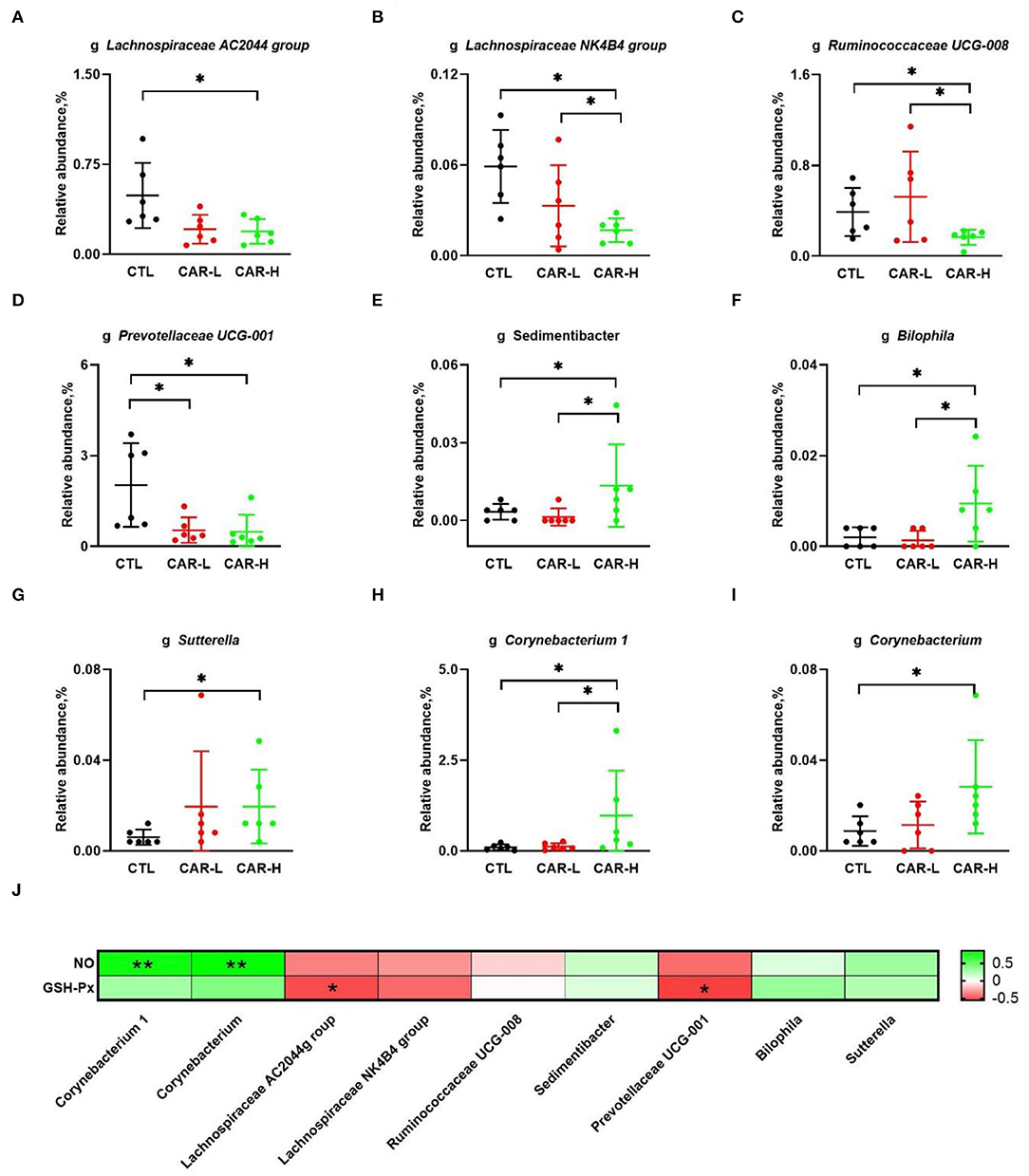
Figure 3. Modulation of fecal microbiota by β-carotene at the genus level. The relative abundance of microbial genera including Lachnospiraceae AC2044 group (A), Lachnospiraceae NK4B4 group (B), Ruminococcaceae UCG-008 (C), Prevotellaceae UGC-001 (D), Sedimentibacter (E), Bilophila (F), Sutterella (G), Corynebacterium 1 (H), and Corynebacterium (I) in each group were characterized by 16S rRNA gene sequencing, and the correlation between NO, GSH-Px and the changed gut microbiota (J) were analyzed by Spearman's correlation analysis. The intensity of the colors represent the degree of association (red, negative correlation; green, positive correlation). Significant correlations were marked by *P < 0.05, **P < 0.01. CTL, a basal diet; CAR-L, a basal diet containing 30 mg/kg β-carotene; CAR-H, a basal diet containing 90 mg/kg β-carotene. Data were shown as means ± SD (n = 6), *P < 0.05.
Discussion
It has been reported that injection or dietary supplementation of β-carotene during early gestation can enhance embryo survival, litter size and litter weight of piglets (9, 20, 21), but less information on its impact in late pregnancy has been obtained. However, in this study, dietary supplementation of β-carotene at late stage of pregnancy showed limited effect on the number of live birth and litter weight at parturition. During late pregnancy, maternal metabolism will be increased to adapt the nutritional requirements of fetal growth and placenta metabolism, which usually manifested by the increase in TG, TC, HDL-c and LDL-c levels (22–24). However, supplementation of β-carotene had no significant effect on the serum levels of lipids (TC, TG, HDL-c, and LDL-c) and immunoglobulins (IgM & IgG) in sows, which suggesting that β-carotene has limited effects on lipid metabolism and immunoglobulin production. Oxidative stress has a negative effect on oocyte maturation, ovulation, implantation and blastocyst formation (25). In the perinatal period of mammals, fetal growth, lactation and increased metabolism can induce the production of progressive ROS, and negatively impact sow reproductive performance including reduces litter size, survival ratio of piglets and the ability for lactation (10, 22). Our results revealed that β-carotene significantly increased the activity of GSH-Px, an antioxidant enzyme against ROS. The findings were consistent with a previous cell model analysis in which β-carotene increased Nrf2 expression (2). Moreover, adequate uterine blood flow throughout gestation is reported to be essential for placental and fetal growth, especially in the late phase of pregnancy (26), as the increased blood flow velocity in placenta can enhance fetus to uptake nutrients absorption (10, 26). As a signaling molecule that conveys information between cells, NO is considered to be an important vasodilator to promote the neonatal blood circulation (27). Thus, serum NO levels in the sow serum were further tested, and the findings showed that β-carotene substantially increased NO levels, implying that β-carotene has a possible beneficial effect on uterine and placenta blood circulation.
Gut microbiota plays an essential role in the regulation of nutrient utilization and metabolism (28), and the microbial diversity can be used as a biomarker to reflect health and metabolic capacity of animals (14). A previous study has also pointed out that gut microbiota is closely related to the reproductive performance of sows (13). The intestinal microbial composition changes significantly during pregnancy, and α-diversity will gradually increase throughout the lactation period (29). Furthermore, the structure of intestinal flora differ at different stages of pregnancy, and enrichment of α-diversity can enhance metabolic capacity and increase the flux of nutrients to the fetus for growth and development (30). Clarke et al. (14) revealed that people with rapid metabolism show a higher α-diversity of gut microbiota. In this study, β-carotene increased the Shannon index, which suggested that it may enhance the metabolic capacity and promote fetal development. Further analysis at the genus level revealed that β-carotene mainly down-regulated the relative abundance of genera Lachnospiraceae AC2044 group, Lachnospiraceae NK4B4 group, and Ruminococcaceae UCG-008 belonging to the phylum of Firmicutes, as well as Prevotellaceae UCG-001 belonging to the phylum of Bacteroidete. On the other hand, β-carotene enriched Sutterella and Bilophila belonging to the phylum of Proteobacteria, Corynebacterium 1 and Corynebacterium belonging to the phylum of Actinobacteria, as well as Sedimentibacter belonging to the phylum of Firmicutes. Lachnospiraceae and Ruminococcaceae are closely related to the production of butyrate (31, 32). Prevotellaceae UCG-001 belongs to the family of Prevotellace showed a positive correlation with the expression of inflammatory factors in our recent study (33). Sutterella is positively correlated with neutral detergent fiber (NDF) digestibility (34), and Bilophila has a positive correlation with obesity-related markers (18), while Sedimentibacter can secrete cellulase enzyme to digest cellulose into glucose (19, 34). Our results revealed that Corynebacterium 1 and Corynebacterium were positively correlated with NO level, while Prevotellaceae UCG-001 and Lachnospiraceae AC2044 group were negatively correlated with GSH-Px level. Other studies have also shown that Corynebacterium can induce the expression of NO synthase in a number of tissues in mice, and the decrease in blood pressure induced by Corynebacterium is associated with the induction of NO synthase (35). This can partially explain the increased NO level induced by β-carotene in this study. A recent study has also indicated that the abundance of Corynebacterium is increased with reproductive performance (36). Therefore, β-carotene potentially enhanced NO production by up-regulating the relative abundance of Corynebacterium, although more direct evidences are need.
Conclusions
In conclusion, dietary supplementation of β-carotene showed limited effect on the reproductive performance of sows but increased the activity of GSH-Px and NO production. Analysis on fecal microbiota revealed that β-carotene may increase the diversity of the microbial flora with enriched Bilophila, Sutterella, Sedimentibacter, Corynebacterium 1 and Corynebacterium which related to the synthesis of NO, an important vasodilator that can promote the neonatal blood circulation.
Data Availability Statement
The raw data supporting the conclusions of this article will be made available by the authors, without undue reservation.
Ethics Statement
The animal study was reviewed and approved by Hunan Agricultural University Institutional Animal Care and Use Committee.
Author Contributions
XY and JY were the primary investigator in this study. RH, YL, and YW participated in the animal experiments. HC participated in sample analysis. D-XH revised the manuscript. SW and JH designed this study and wrote the manuscript as corresponding author. The authors read and approved the final manuscript.
Funding
This work was partially supported by the funds from the National Natural Science Foundation of China (31772819 and 31972600), Hunan Provincial Natural Science Foundation for Distinguished Young Scholars (2019JJ30012), and Scientific Research Fund of Hunan Provincial Education Department (18B098).
Conflict of Interest
XY was employed by Hunan Xinguang'an Agricultural Husbandry Co., Ltd., and the sows for experiment were provided by this company.
The remaining authors declare that the research was conducted in the absence of any commercial or financial relationships that could be construed as a potential conflict of interest.
Acknowledgments
We thanked Dr. Muhammed Adebayo Arowolo for the language editing.
Supplementary Material
The Supplementary Material for this article can be found online at: https://www.frontiersin.org/articles/10.3389/fnut.2020.612875/full#supplementary-material
References
1. von Lintig J. Colors with functions: elucidating the biochemical and molecular basis of carotenoid metabolism. Annu Rev Nutr. 30:35–56 doi: 10.1146/annurev-nutr-080508-141027
2. Bonet ML, Canas JA, Ribot J, Palou A. Carotenoids and their conversion products in the control of adipocyte function, adiposity and obesity. Arch Biochem Biophys. (2015) 572:112–25. doi: 10.1016/j.abb.2015.02.022
3. Grune T, Lietz G, Palou A, Ross AC, Stahl W, Tang GW, et al. Beta-carotene is an important vitamin a source for human. J Nutr. (2010) 140:2268–85. doi: 10.3945/jn.109.119024
4. Weber D, Grune T. The contribution of beta-carotene to vitamin A supply of humans. Mol Nutr Food Res. (2012) 56:251–8. doi: 10.1002/mnfr.201100230
5. Shapiro SS, Mott DJ, Machlin LJ. Kinetic characteristics of β-carotene uptake and depletion in rat tissue. J Nutr. (1984) 114:1924–33.
6. Paik J, Haenisch M, Muller CH, Goldstein AS, Arnold S, Isoherranen N, et al. Inhibition of retinoic acid biosynthesis by the bisdichloroacetyldiamine WIN 18,446 markedly suppresses spermatogenesis and alters retinoid metabolism in mice. J Biol Chem. (2014) 289:15104–17. doi: 10.1074/jbc.M113.540211
7. Kawashima C, Nagashima S, Sawada K, Schweigert FJ, Miyamoto A, Kida K. Effect of beta-carotene supply during close-up dry period on the onset of first postpartum luteal activity in dairy cows. Reprod Domest Anim. (2010) 45:282–7. doi: 10.1111/j.1439-0531.2009.01558.x
8. Talavera F, Chew BP. Comparative role of retinol, retinoic acid and beta-carotene on progesterone secretion by pig corpus luteum in vitro. J Reprod Infertil. (1988) 82:611–5. doi: 10.1530/jrf.0.0820611
9. Chew B, Rasmussen H, Pubols MH, Preston RL. Effects of vitamin and B-carotene on plasma progesterone and uterine secretions in gilts. Theriogenology. (1982) 18:643–54. doi: 10.1016/0093-691X(82)90030-9
10. Burton GJ. Oxygen, the Janus gas; its effects on human placental development and function. J Anat. (2009) 215:27–35. doi: 10.1111/j.1469-7580.2008.00978.x
11. Palm M, Axelsson O, Wernroth L, Larsson A, Basu S. Involvement of inflammation in normal pregnancy. Acta Obstet Gynecol Scand. (2013) 92:601–5. doi: 10.1111/aogs.12093
12. Chen ZH, Watanabe RM, Stram DO, Buchanan TA, Xiang AH. High calorie intake is associated with worsening insulin resistance and beta-cell function in hispanic women after gestational diabetes mellitus. Diabetes Care. (2014) 37:3294–300. doi: 10.2337/dc14-1433
13. Shao YR, Zhou J, Xiong X, Zou LJ, Kong XF, Tan B, et al. Differences in gut microbial and serum biochemical indices between sows with different productive capacities during perinatal period. Front Microbiol. (2020) 10:3047. doi: 10.3389/fmicb.2019.03047
14. Clarke SF, Murphy EF, O'Sullivan O, Lucey AJ, Humphreys M, Hogan A, et al. Exercise and associated dietary extremes impact on gut microbial diversity. Gut. (2014) 63:1913–20. doi: 10.1136/gutjnl-2013-306541
15. Zhou LH, Ouyang L, Lin SZ, Chen S, Liu YJ, Zhou W, et al. Protective role of beta-carotene against oxidative stress and neuroinflammation in a rat model of spinal cord injury. Int Immunopharmacol. (2018) 61:92–9. doi: 10.1016/j.intimp.2018.05.022
16. Lee S-J, Bai S-K, Lee K-S, Namkoong S, Na H-J, Ha K-S, et al. Astaxanthin inhibits nitric oxide production and inflammatory gene expression by suppressing I(kappa)B kinase-dependent NF-kappaB activation. Molecules Cells. (2003) 16:97–105.
17. Karamese SA, Toktay E, Unal D, Selli J, Karamese M, Malkoc I. The protective effects of beta-carotene against ischemia/reperfusion injury in rat ovarian tissue. Acta Histochem. (2015) 117:790–7. doi: 10.1016/j.acthis.2015.07.006
18. Hou D, Zhao Q, Yousaf L, Khan J, Xue Y, Shen Q. Consumption of mung bean (Vigna radiata L.) attenuates obesity, ameliorates lipid metabolic disorders and modifies the gut microbiota composition in mice fed a high-fat diet. J Funct Foods. (2020) 64:103687. doi: 10.1016/j.jff.2019.103687
19. Kanokratana P, Wongwilaiwalin S, Mhuantong W, Tangphatsornruang S, Eurwilaichitr L, Champreda V. Characterization of cellulolytic microbial consortium enriched on Napier grass using metagenomic approaches. J Biosci Bioeng. (2018) 125:439–47. doi: 10.1016/j.jbiosc.2017.10.014
20. Krammer G, Aurich J. Effect of intramuscularly administered beta-carotene on reproductive performance in sows. Berliner Munchener Tierarztl Wochenschr. (2010) 123:496–9. doi: 10.2376/0005-9366-123-496
21. Lindemann MD, Brendemuhl JH, Chiba LI, Darroch CS, Dove CR, Estienne MJ, et al. A regional evaluation of injections of high levels of vitamin A on reproductive performance of sows. J Anim Sci. (2008) 86:333–8. doi: 10.2527/jas.2007-0153
22. Burton GJ, Jauniaux E, Charnock-Jones DS. The influence of the intrauterine environment on human placental development. Int J Dev Biol. (2010) 54:303–11. doi: 10.1387/ijdb.082764gb
23. Zhao Y, Flowers WL, Saraiva A, Yeum KJ, Kim SW. Effect of social ranks and gestation housing systems on oxidative stress status, reproductive performance, and immune status of sows. J Anim Sci. (2013) 91:5848–58. doi: 10.2527/jas.2013-6388
24. Tanghe S, Missotten J, Raes K, De Smet S. The effect of different concentrations of linseed oil or fish oil in the maternal diet on the fatty acid composition and oxidative status of sows and piglets. J Anim Physiol Anim Nutr. (2015) 99:938–49. doi: 10.1111/jpn.12243
25. Kim SW, Weaver AC, Shen YB, Zhao Y. Improving efficiency of sow productivity: nutrition and health. J Anim Sci Biotechnol. (2013) 4:26. doi: 10.1186/2049-1891-4-26
26. Shao XM, López-Valdés HE, Liang J, Feldman JL. Inhaled nicotine equivalent to cigarette smoking disrupts systemic and uterine hemodynamics and induces cardiac arrhythmia in pregnant rats. Sci Rep. (2017) 7:16974. doi: 10.1038/s41598-017-17301-5
27. Angelis D, Savani R, Chalak L. Nitric oxide and the brain. Part 1: mechanisms of regulation, transport and effects on the developing brain. Pediatr Res. (2020). doi: 10.1038/s41390-020-1017-0. [Epub ahead of print].
28. Tremaroli V, Backhed F. Functional interactions between the gut microbiota and host metabolism. Nature. (2012) 489:242–9. doi: 10.1038/nature11552
29. Ji YJ, Li H, Xie PF, Li ZH, Li HW, Yin YL, et al. Stages of pregnancy and weaning influence the gut microbiota diversity and function in sows. J Appl Microbiol. (2019) 127:867–79. doi: 10.1111/jam.14344
30. Koren O, Goodrich JK, Cullender TC, Spor A, Laitinen K, Backhed HK, et al. Host remodeling of the gut microbiome and metabolic changes during pregnancy. Cell. (2012) 150:470–80. doi: 10.1016/j.cell.2012.07.008
31. Chen R, Wu P, Cai Z, Fang Y, Zhou H, Lasanajak Y, et al. Puerariae Lobatae Radix with chuanxiong Rhizoma for treatment of cerebral ischemic stroke by remodeling gut microbiota to regulate the brain-gut barriers. J Nutr Biochem. (2019) 65:101–14. doi: 10.1016/j.jnutbio.2018.12.004
32. Hu R, Zeng F, Wu L, Wan X, Chen Y, Zhang J, et al. Fermented carrot juice attenuates type 2 diabetes by mediating gut microbiota in rats. Food Funct. (2019) 10:2935–46. doi: 10.1039/C9FO00475K
33. Hu RZ, He ZY, Liu M, Tan JJ, Zhang HF, Hou DX, et al. Dietary protocatechuic acid ameliorates inflammation and up-regulates intestinal tight junction proteins by modulating gut microbiota in LPS-challenged piglets. J Anim Sci Biotechnol. (2020) 11:12. doi: 10.1186/s40104-020-00492-9
34. Niu Q, Li P, Hao S, Kim SW, Du T, Hua J, et al. Characteristics of gut microbiota in sows and their relationship with apparent nutrient digestibility. Int J Mol Sci. (2019) 20:870. doi: 10.3390/ijms20040870
35. Rees DD, Cunha FQ, Assreuy J, Herman AG, Moncada S. Sequential induction of nitric oxide synthase by Corynebacterium parvum in different organs of the mouse. Br J Pharmacol. (1995) 114:689–93. doi: 10.1111/j.1476-5381.1995.tb17193.x
Keywords: β-carotene, nitric oxide, antioxidant, gut microbiota, pregnant sows
Citation: Yuan X, Yan J, Hu R, Li Y, Wang Y, Chen H, Hou D-X, He J and Wu S (2020) Modulation of Gut Microbiota and Oxidative Status by β-Carotene in Late Pregnant Sows. Front. Nutr. 7:612875. doi: 10.3389/fnut.2020.612875
Received: 01 October 2020; Accepted: 20 November 2020;
Published: 14 December 2020.
Edited by:
Hui Han, Chinese Academy of Sciences (CAS), ChinaReviewed by:
Hongkui Wei, Huazhong Agricultural University, ChinaShengyu Xu, Sichuan Agricultural University, China
Copyright © 2020 Yuan, Yan, Hu, Li, Wang, Chen, Hou, He and Wu. This is an open-access article distributed under the terms of the Creative Commons Attribution License (CC BY). The use, distribution or reproduction in other forums is permitted, provided the original author(s) and the copyright owner(s) are credited and that the original publication in this journal is cited, in accordance with accepted academic practice. No use, distribution or reproduction is permitted which does not comply with these terms.
*Correspondence: Jianhua He, jianhuahy@hunau.net; Shusong Wu, wush688@hunau.edu.cn