- Department of Behavioural Neurobiology, Max Planck Institute for Ornithology, Seewiesen, Germany
Singing occurs in songbirds of both sexes, but some species show typical degrees of sex-specific performance. We studied the transcriptional sex differences in the HVC, a brain nucleus critical for song pattern generation, of the forest weaver (Ploceus bicolor), the blue-capped cordon-bleu (Uraeginthus cyanocephalus), and the canary (Serinus canaria), which are species that show low, medium, and high levels of sex-specific singing, respectively. We observed persistent sex differences in gene expression levels regardless of the species-specific sexual singing phenotypes. We further studied the HVC transcriptomes of defined phenotypes of canary, known for its testosterone-sensitive seasonal singing. By studying both sexes of canaries during both breeding and non-breeding seasons, non-breeding canaries treated with testosterone, and spontaneously singing females, we found that the circulating androgen levels and sex were the predominant variables associated with the variations in the HVC transcriptomes. The comparison of natural singing with testosterone-induced singing in canaries of the same sex revealed considerable differences in the HVC transcriptomes. Strong transcriptional changes in the HVC were detected during the transition from non-singing to singing in canaries of both sexes. Although the sex-specific genes of singing females shared little resemblance with those of males, our analysis showed potential functional convergences. Thus, male and female songbirds achieve comparable singing behaviours with sex-specific transcriptomes.
Introduction
Most of the genomes of male and female individuals of the same species are the same but stark sex differences in physiological, phenotypical, or behavioural traits between the sexes are common and widespread in the animal kingdom. The songbird clade (a suborder of the perching birds) consists of more than 4000 extant avian species, and these exhibit a great diversity of sex differences in singing behaviour. Among domesticated canaries (Serinus canaria), males are known for their singing behaviour and have been selected for their sophisticated songs for centuries, whereas female canaries seldom sing (Shoemaker, 1939; Herrick and Harris, 1957; Pesch and Güttinger, 1985; Vallet et al., 1996; Hartley et al., 1997; Ko et al., 2020). Such substantial sex differences in singing behaviour are commonly found in the majority of northern temperate songbird species, even though females of many tropical and southern temperate species sing regularly, and their songs play an important role in inter-sexual communication (Slater and Mann, 2004; Price et al., 2009; Hall et al., 2015; Price, 2019). For example, female blue-capped cordon-bleus (Uraeginthus cyanocephalus) use their song as advertising signals and address their songs to their mates (Immelmann, 1968; Ota et al., 2018), although the female songs appear shorter and less complex than those of males (Geberzahn and Gahr, 2011). In another tropical songbird species, forest weavers (Ploceus bicolor), males and females develop their songs during pair binding and eventually learn to sing identical duets, which they use to defend their territories (Wickler and Seibt, 1980).
Although the extent of sex differences in singing behaviour varies greatly in the songbird clade (Ball, 2016), the song quality and occurrence in males and females of many songbird species are dependent on testosterone (Nottebohm et al., 1987; Leitner et al., 2001b; Fusani et al., 2003; Voigt and Leitner, 2008; Dittrich et al., 2014; Ko et al., 2020). In canaries that breed seasonally, the optimal breeding conditions are tightly associated with an increase in the day length, which initiates gonadal growth and testosterone production as well as various types of breeding activity, including singing (Nottebohm et al., 1987; Leitner et al., 2001b; Voigt and Leitner, 2008). The length and syllable repetition rate of songs during the breeding season are greater than those of songs in non-breeding seasons (Leitner et al., 2001a,b; Voigt and Leitner, 2008). Castrated male canaries sing shorter songs than sham-operated males, and the subcutaneous implantation of testosterone results in the recovery of singing performance (Heid et al., 1985). The local administration of testosterone into the preoptic brain region increases the male canary singing rate by increasing motivation (Alward et al., 2013). Similarly, the systemic administration of testosterone reliably and repeatedly induces singing behaviour in otherwise non-singing female canaries (Leonard, 1939; Shoemaker, 1939; Herrick and Harris, 1957; Nottebohm, 1980; Fusani et al., 2003; Madison et al., 2015; Vellema et al., 2019b). Although female canaries rarely exhibit spontaneous singing (Shoemaker, 1939; Herrick and Harris, 1957; Pesch and Güttinger, 1985; Vallet et al., 1996; Hartley et al., 1997; Ko et al., 2020), its occurrence appears to be associated with the plasma androgen levels (Ko et al., 2020).
A set of interconnected neural circuits called the song control system controls the production and learning of singing behaviour (Nottebohm et al., 1976; Wild, 2004). The premotor nucleus HVC (used as the proper name), which is a sensorimotor integration centre in the song control system, is involved in the frequency and temporal modulation of songs in male and female songbirds (Hahnloser et al., 2002; Hoffmann et al., 2019) and in the sexual preferences regarding conspecific song displays (Brenowitz, 1991; Del Negro et al., 1998). The HVC is the only brain nucleus within the song control system that expresses receptors for both androgens and estrogens (Gahr, 2001; Frankl-Vilches and Gahr, 2018). Intriguingly, the anatomical properties of the HVC, such as volume (Figure 1), neuron number, and dendrite complexity, are male-biased (greater, higher and more complex, respectively, in males than in females) in all songbird species that have been examined, irrespective of the existence of sex differences in singing behaviour (Nottebohm and Arnold, 1976; Gurney and Konishi, 1980; Brenowitz et al., 1985; Nixdorf et al., 1989; Gahr et al., 1998, 2008; MacDougall-Shackleton and Ball, 1999; Hall et al., 2010; Lobato et al., 2015; Schwabl et al., 2015). Testosterone treatment increases the delineable volume of the HVC in both male and female canaries (Nottebohm, 1980; Fusani et al., 2003; Madison et al., 2015) and many other species (Bernard and Ball, 1997; Smith et al., 1997; Gulledge and Deviche, 1999; Van Meir et al., 2004; Dittrich et al., 2014). Nevertheless, the HVC volume of female canaries implanted with testosterone remains markedly smaller than that of males (Nottebohm, 1980) (Figure 1). Testosterone clearly regulates singing behaviour and the anatomy of the HVC in both male and female songbirds. However, an intrinsic limit to the alterations induced by testosterone appears to exist, and this limit prevents female songbirds from reaching the same levels of “maleness” in terms of song characteristics and HVC anatomy.
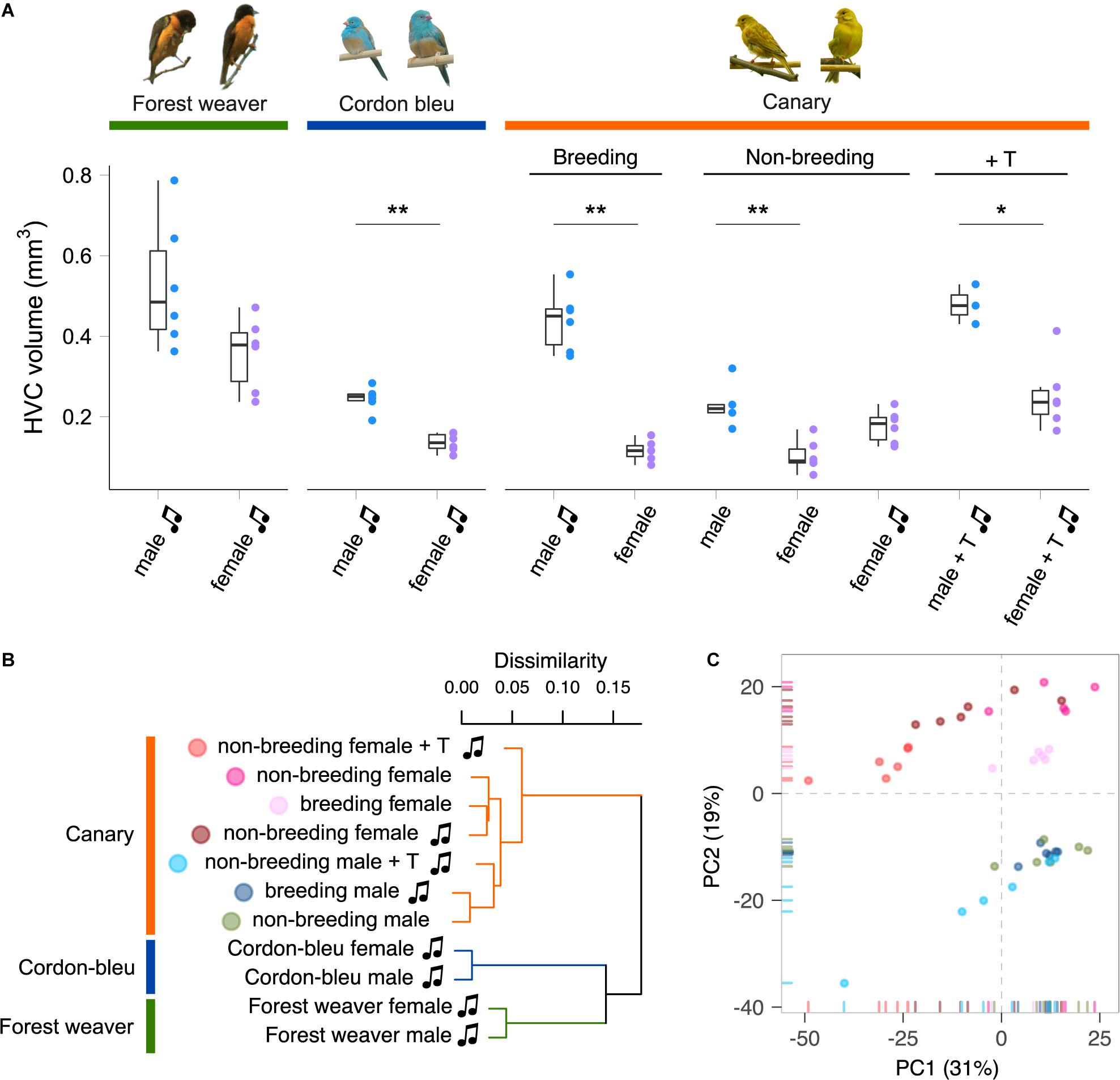
Figure 1. Species, sex, and plasma androgen level are the major determinants of the HVC gene expression patterns in songbirds. (A) HVC volume of birds used in this study. Forest weavers: males 0.528 mm3 (mean), females 0.357 mm3. Mann–Whitney Test, U = 7, P-value = 0.0931. Cordon-bleus: males 0.245 mm3, females 0.136 mm3. Mann–Whitney Test, U = 0, P-value = 0.00217. Breeding canaries: singing males 0.439 mm3, non-singing females 0.116 mm3. Mann–Whitney Test, U = 0, P-value = 0.00217. Non-breeding canaries: non-singing males 0.228 mm3, non-singing females 0.103 mm3, singing females 0.176 mm3. Mann–Whitney Test (non-singing males vs. non-singing females), U = 0, P-value = 0.00492. Mann–Whitney Test (non-singing males vs. singing females), U = 8, P-value = 0.127. Testosterone-implanted canaries: males 0.478 mm3, females 0.253 mm3. Mann–Whitney Test, U = 0, P-value = 0.0238. *P-value < 0.05; **P-value < 0.01. Each colour-coded dot indicates the measurement from one bird. The boxes indicate the 25th/50th/75th percentiles (bottom/middle/top bar), and the extent of the whiskers indicates the most extreme values that are within 1.5 times the IQR (interquartile range) of the hinge. T, testosterone; music note, presence of singing behaviour. (B) Hierarchical clustering showing that phylogenetic relatedness accounts for the most variation in the HVC transcriptomes of females and males of the forest weaver, cordon-bleu, and canary. Among the seven canary groups, the testosterone-treated animals were the least similar to the untreated canaries. (C) A PCA of the HVC transcriptomes of the seven canary groups distinguished male birds (PC2 < 0) from female birds (PC2 > 0). Each point is colour-coded by group (see panel B) and represents an HVC sample.
How are such sex-specific differences in singing and HVC anatomy achieved in different species? We hypothesise the existence of a fundamental difference in gene regulation and expression between male and female conspecific songbirds. Based on this hypothesis, sex differences in the HVC transcriptomes of male and female conspecifics should always be observable, even though both sexes share an almost identical genome. The female-specific W chromosome harbours 30–50 genes, whereas one and two copies of the Z chromosome (harbouring approximately 1,080 genes) are present in female and male birds, respectively (Frankl-Vilches et al., 2015; Smeds et al., 2015; Sayers et al., 2020). We further hypothesise that such intrinsic differences cannot be overwritten by manipulating the testosterone level in females. To test our hypotheses, we compared the transcriptomes of HVCs microdissected from three songbird species—the forest weaver, the blue-capped cordon-bleu, and the canary. These species represent three categories of sex differences in singing behaviour (low, medium, and high). Furthermore, we compared male and female canaries with and without testosterone implantation to evaluate the effects of testosterone (Table 1). The results revealed sex differences in the HVC transcriptome between- and within-species comparisons. Our results indicate that although the extent of sex-biased gene expression is context-dependent, it is persistent regardless of hormonal manipulation, behavioural phenotypes, and distinct genetic backgrounds.
Materials and Methods
Animals
Forest weaver (Ploceus bicolor) pairs were observed in their breeding territories in eastern South Africa; the songs of the pairs were recorded to ensure that both mates were singing. Subsequently, the animals were caught and sacrificed in accordance with permits issued by the local authorities (Chief Professional Officer for Research at the Natal Parks, Game and Fish Preservation Board, P. O. B. 662, Pietermaritzburg 3200). Blue-capped cordon-bleus (Uraeginthus cyanocephalus) and canaries (Serinus canaria) were bred at the animal facilities at the Max Planck Institute for Ornithology in Seewiesen, Germany. The procedures used for animal housing and welfare complied with the European directives for the protection of animals used for scientific purposes (2010/63/EU), and the protocols were approved by the Government of Upper Bavaria (AZ No. 55.2-1-54-2532-181-12). Adult canaries and blue-capped cordon-bleus (aged at least 1 year) were housed in pairs under long-day conditions (light:dark = 14:10 h), and their breeding activities were monitored. The birds (male canaries and cordon-bleus) were sacrificed after singing activity was observed during the breeding season. Non-breeding canaries were housed pairwise with a 9-h light:15-h dark schedule for at least 8 weeks before their singing activity was monitored (generally starting in late September). Non-breeding male and female canaries were maintained alone in sound-attenuated boxes (70 × 50 × 50 cm), recorded continuously during the song monitoring phase (2 weeks) and sacrificed after confirmation of no singing activity. Additional groups of male and female non-breeding and non-singing canaries were implanted with testosterone for 2 weeks and sacrificed after observation of singing activity. Water and food were available ad libitum. The sex was confirmed by PCR using the P2 and P8 primers for CHD genes (Griffiths et al., 1998) and by visual inspection of the reproduction system after sacrifice. We previously found that six non-breeding female canaries exhibited singing behaviour during long-term monitoring, and their songs have been described in detail (Ko et al., 2020). In this study, we included the transcriptomes of these birds. All the birds were sacrificed via an overdose of isoflurane followed by decapitation, the body weights were recorded, and the brains were dissected, weighed, snap-frozen on dry ice and stored at −80°C until further use. Table 1 summarises the information of the experimental groups used in this study.
Testosterone Implantation
A SilasticTM tube (Dow Corning; 1.47-mm inner diameter, 1.96-mm outer diameter, 0.23-mm thickness) was cut to a length of 7 mm and loaded with testosterone (86500, Fluka) as densely as possible. The two ends of the SilasticTM tube were sealed with silicone elastomer (3140, Dow Corning). After closure, the implants were cleaned with 100% ethanol to remove testosterone particles and then immersed in ethanol overnight in a hood to ensure no leakage at either end. Implants with apparent dampness were discarded. One day prior to the implantation, the implants were incubated in 0.1 M phosphate buffered saline (PBS) overnight to enable the immediate release of testosterone upon implantation (Rasika et al., 1994). We started implantation at approximately 8:30 am (immediately after the light was turned on in the morning), which resulted in a 20-min interval between birds based on the scarification time. A small incision was made on the back of the bird over the pectoral musculature, and one testosterone implant was placed subcutaneously. The skin was closed by the application of tissue glue. After 2 weeks, the animals were sacrificed. The testosterone implants were checked, and this inspection revealed that the implants were all in place and were not empty at the end of the experiments.
Radioimmunoassay of Plasma Testosterone
Blood was sampled (<150 μl) at the time sacrifice. All blood samples were taken between 8 and 11 am and were taken within 3 min after caught to avoid the effect of handling (Wingfield et al., 1982). Blood samples were centrifuged (5,000 rpm, 10 min) to separate the plasma from blood cells. Testosterone metabolites were measured with a radioimmunoassay using a commercial antiserum against testosterone (T3-125, Endocrine Sciences, Tarzana, CA, United States) as previously described (Goymann et al., 2002). Standard curves and sample concentrations were calculated with Immunofit 3.0 (Beckman Inc., Fullerton, CA, United States) using a four-parameter logistic curve fit and corrected for individual recoveries.
The testosterone concentrations were assayed in duplicate in five separate assays. The mean extraction efficiency for plasma testosterone was 85.0 ± 3.9% (mean ± SD, N = 42). The lower detection limits of the testosterone assays were 0.34, 0,35, 0.36, 0.38 and 0.35 pg per tube, and all the samples were above the detection limit. The intra-assay coefficients of variation of a chicken plasma pool were 8.7%, 3.4%, 12.8%, 1.9%, and 4.4%. The interassay coefficient of variation as determined by the variation in the chicken plasma pool between all the assays was 5.1%. Because the testosterone antibody used shows significant cross-reactions with 5α-dihydrotestosterone (44%), our measurement might include a fraction of 5α-DHT.
Brain Sectioning
The birds were killed by an overdose of isoflurane, and their brains were snap-frozen on dry ice. The brains were sectioned sagittally into four series of 40-μm sections and two series of 20-μm sections with a cryostat (Jung CM3000 Leica). The 40-μm sections were mounted on glass slides for subsequent tissue dissection for total RNA extraction, whereas the thin sections were mounted on RNase-free Superfrost slides for Nissl staining and measurement of the HVC volume. All sections were stored at −80°C until further processing.
Measurement of the HVC Volume
One series of 20-μm sections mounted on RNase-free Superfrost slides was subjected to Nissl staining with 0.1% thionin (Sigma-Aldrich), dehydrated, immersed in xylene and cover-slipped with Roti-Histokitt II mounting medium (Roth). The HVC areas (typically 8–10 slices) were measured with a Leica DM6000 B microscope connected to a computer-based image-analysis system (IMAtec). All brains were coded such that the observers were blind toward any additional information about the sections they measured during the delineations. The volumes were derived from the summed area measurements multiplied by the section thickness and the intersection distance.
Microarray Procedures and Annotation
For total RNA extraction, the song control nucleus HVC and the visual area of the entopallium were dissected from the abovementioned 40-μm sections under a stereomicroscope (typically 24–32 slices for the HVC and 16–20 slices for the entopallium) and transferred into an Eppendorf tube containing 340 μl of RLT buffer mixture (containing DTT, Qiagen). This dissection procedure using rather thin sections reduces the contamination of HVC tissue with surrounding tissue. RNA was then extracted using the RNeasy® Micro Kit (Qiagen) with the optional DNase digest step. The RNA quality was assessed using the Agilent Model 2100 Bioanalyzer (Agilent Technologies), and the RNA concentrations were assessed using a Nanodrop 1000 spectrometer (Thermo Fisher Scientific). The RNA quality of all samples was good (RIN > 7). The purified total RNA samples (at least 100 ng per sample) were subsequently processed and hybridised using the Ambion WT Expression Kit and the Affymetrix WT Terminal Labelling and Controls Kit. The resulting cDNA was hybridised to the Custom Affymetrix Gene Chip® MPIO-ZF1s520811 Exon Array, which has been used successfully and validated in cross-species hybridisation studies (Dittrich et al., 2014; Frankl-Vilches et al., 2015). The 5.7 million male zebra finch-specific probes spotted on this array correspond to approximately 4,711,133 probe sets and hence to 25,816 transcripts published in public databases until 2010 (NCBI and Ensembl) and mapped to the zebra finch genome published by Warren et al. (2010). We annotated more than 90% of the transcripts to 12,729 human orthologous genes using several publicly available databases [Ensembl, GenBank, UniProt, and DAVID (Benson et al., 2005; Huang et al., 2008, 2009; Flicek et al., 2014; Consortium, 2015; Yates et al., 2016)] and commercial databases (El Dorado, Genomatix, Precigen Bioinformatics Germany GmbH (PBG), Munich, Germany). Hybridisation was performed for 16 h at 45°C and 60 rpm in a GeneChip Hybridization Oven 640 (Affymetrix). The arrays were washed, stained, and scanned using the Affymetrix GeneChip Fluidics Station 450 and the Affymetrix GeneChip scanner 3000 7G. The CEL files were generated using Affymetrix® GeneChip Command Console Software (AGCC), and for quality control, the success of individual hybridisations was assessed using Affymetrix® Expression ConsoleTM software.
Differential expression was calculated using ChipInspector software version 21 [El Dorado Database version E28R1306 (Genomatix, 2021)]. ChipInspector is a single probe-based analysis tool for microarray data that can show increased sensitivity compared with that obtained with conventional probe set-based analyses, such as robust multiarray analysis. ChipInspector consists of four steps: single probe-transcript annotation (ensuring up-to-date annotation), total intensity normalization, SAM (significance analysis of microarrays, adapted to single-probe handling) analysis (Tusher et al., 2001), and transcript identification based on significantly changed probes (Cohen et al., 2008). We set the delta threshold to 0 (to control the false positive rate) and used the groupwise exhaustive comparison tool in ChipInspector. The minimum coverage for each transcript was set to 10 significant probes, and the minimum expression difference was | log2(fold change)| ≥ 0.5. The significantly differentially expressed transcripts obtained were annotated to human orthologous genes as described above. For transcripts belonging to the same genes, the average expression was calculated if all transcripts were regulated in the same manner (e.g., all upregulated or all downregulated). If a gene contains both up- or downregulated transcripts, the transcripts that showed changes in expression in the minority direction were discarded (<40% of the total transcripts), and the average expression was instead calculated from the remaining transcripts (60% or more). Transcripts without human orthologous gene annotation were removed prior to subsequent analyses. We were cautious about possible cross-species bias in hybridisation, and differential expression analyses were only performed between two conspecific groups. The microarray data discussed in this publication have been deposited in NCBI’s Gene Expression Omnibus (Edgar et al., 2002) and are accessible through the GEO Series accession number GSE83674.
Hierarchical Clustering
The normalised gene expression levels (across all transcriptome samples) were calculated using the “justRMA” function of the R package “affy” v1.66.0 (Gautier et al., 2004), and the expression levels were further collapsed to gene levels using the “collapseRows” functions of the R package “WGCNA” v1.69 (Langfelder and Horvath, 2008, 2012). The group-level expression levels were calculated by averaging the gene expression levels. Spearman’s ρ was calculated between samples (or groups) using the “cor” function, and a Euclidean distance matrix was calculated [dist(1-cor)] and used for hierarchical clustering analysis (method: complete) with the “hclust” function. This computation was performed with R v4.0.2 (R Core Team, 2020) using the “dendextend” v1.14.0 package for visualisation (Galili, 2015).
Principal Component Analysis (PCA)
Principal component analysis (PCA) (Ringner, 2008) was performed using the normalised and collapsed HVC gene expression data (described in the hierarchical clustering section) from 41 canaries (Table 1). The computation was performed using the “pca” function of the R package “pcaMethods” [v1.60.0 (Stacklies et al., 2007), method = svd]. The data were centred but not scaled because the expression data had already been normalised.
Fisher’s Exact Testing for Chromosomal Enrichment
Fisher’s exact test was used to test whether sex-biased genes were enriched on a chromosome by comparing the gene lists of interest to the zebra finch annotation (release 103)1. We used the “fisher.test” function in R (R Core Team, 2020) with the alternative set to “greater” for enrichment. The male- and female-biased genes identified from each comparison as well as the male-specific, female-specific, and sex-shared genes were tested separately. The P-values were adjusted using the Bonferroni correction to account for multiple comparisons using “p.adjust” in R (R Core Team, 2020).
Gene Ontology (GO) Term Enrichment Analysis
We used ClueGO v2.2.4, an application built in the Cytoscape environment v3.3.0 (Shannon et al., 2003; Bindea et al., 2009), to predict the putative biological functions of the genes of interest. This software performs GO term enrichment hierarchical analyses and fuses GO terms with similar functions. The enrichment was determined by the right-sided test and corrected using the Bonferroni step-down method considering multiple comparisons.
Results and Discussion
The Species Identity Distinguishes Its HVC Transcriptomes
We quantified the total mRNA from the HVC of male and female birds belonging to three songbird species (forest weavers, blue-capped cordon-bleus, and canaries) during the breeding season (Table 1). For intraspecies sex comparisons, we included male and female canaries during the non-breeding seasons (both non-singing), and subgroups were treated with testosterone to induce singing behaviour in both sexes (Supplementary Figure 1). In addition, we obtained a rare group of non-breeding female canaries that sang spontaneously without exogenous testosterone manipulation. However, their plasma androgen levels were intrinsically higher than those of non-breeding and non-singing females (Ko et al., 2020). To visualise the similarities in the gene expression patterns among and within the three species, we calculated Spearman’s rank correlation coefficient σ for 12,360 gene expression levels and calculated the distance matrix based on the coefficient σ. The resulting cladogram indicated that the HVC transcriptomes of the eleven groups were clustered primarily by phylogenetic relatedness (Figure 1B and Supplementary Figure 2). We obtained a similar result with the transcriptomes of another tissue, the entopallium (Supplementary Figure 3), which is an area of the avian visual system that is functionally equivalent to the primary visual cortex of mammals. The within-species comparison of the canary HVC showed that the individual transcriptomes clustered well by sex, with the exception of the non-breeding canaries implanted with testosterone (Figure 1B and Supplementary Figure 2). The subclusters of forest weaver and cordon-bleu HVC transcriptomes were less sexually differential than those of canaries, i.e., male and female birds were intermittent within the subcluster of the two species (Supplementary Figures 2, 3).
Sex-Biased Gene Expression in the HVC of Songbirds
We defined the degree of sex differences in HVC gene expression levels as the number of sex-biased genes whose expression levels showed significant differences between males and females of the same species (male-biased genes showed higher expression levels in males than in females, log2 (fold change) ≥ 0.5; female-biased genes presented higher expression levels in females than in males, log2 (fold change) ≤ −0.5). For canary, we quantified the sex differences from five distinct male-to-female comparisons (Figure 2A, comparisons 3 to 7).
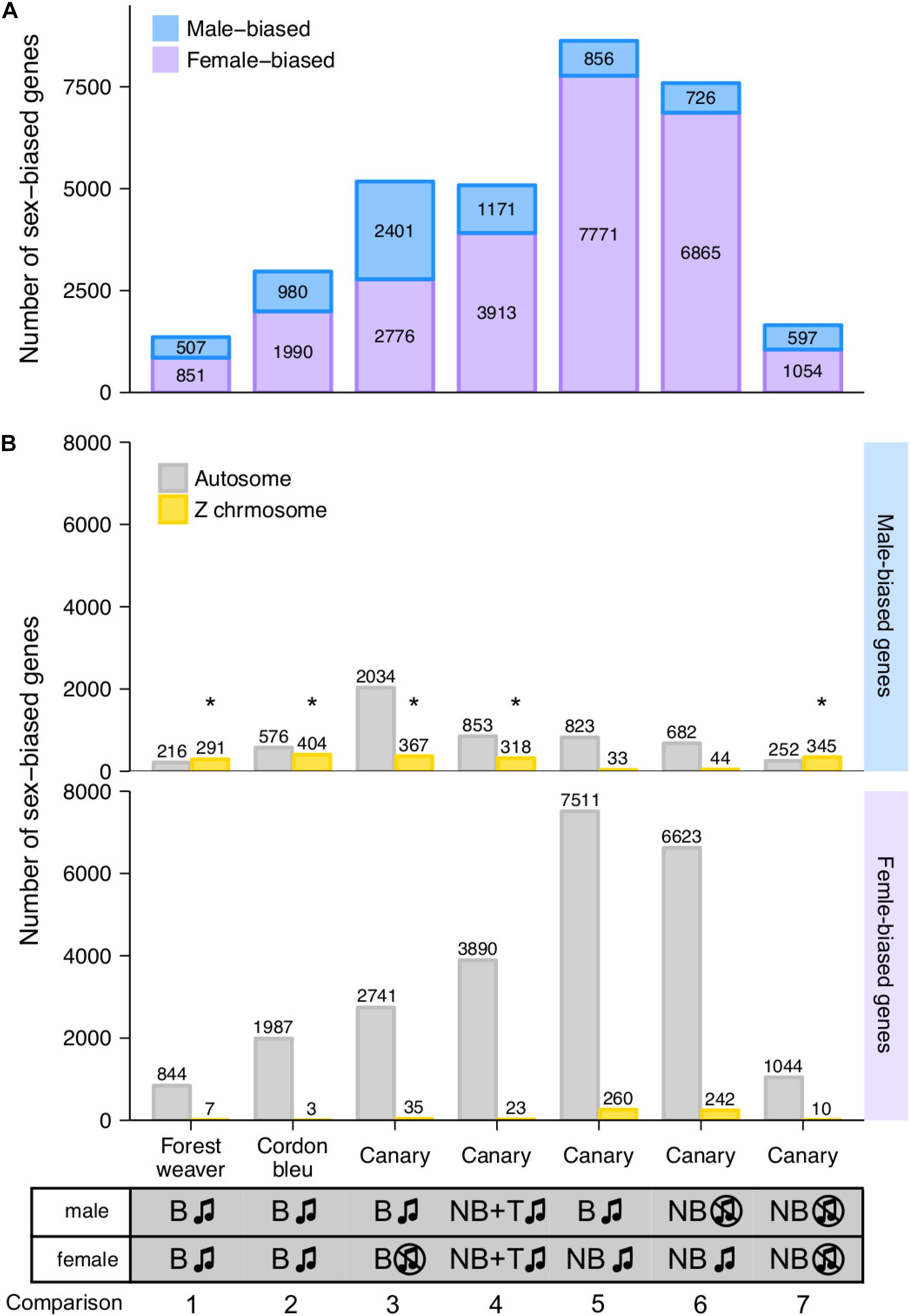
Figure 2. Female- and male-biased sex differences in HVC gene expression levels in songbirds. (A) The bar graph summarises the number of sex-biased genes in the HVC transcriptome identified from each male-to-female comparisons. The phenotypes of the groups being compared in each male-to-female comparison are listed at the bottom of the graph. (B) The bar graph summarises the number of autosomal and Z chromosomal genes classified as male- and female-biased genes in the HVC transcriptome based on each male-to-female comparison. B, breeding; NB, non-breeding; T, testosterone implantation. The music note indicates the presence or absence of singing behaviour; the asterisk (*) indicates enrichment for Z chromosomal genes (adjusted p < 0.05, Fisher’s exact test followed by the Bonferroni correction for multiple testing, see Supplementary Table 1).
Interestingly, we found persistent sex differences in HVC gene expression levels from all the comparisons, regardless of the degree of sex differences in singing behaviour (Figure 2A). The majority (> 90%) of sex-biased genes were lowly biased (0.5 ≤ | log2 (fold change)| < 1, Supplementary Figure 4). The comparison of forest weaver (the female birds can sing identical songs to the males; least differences in the HVC volumes between the sexes; Figure 1A) showed the least prominent sex differences in the HVC transcriptomes across all the comparisons performed in this study. Nevertheless, the extent of sex differences in gene expression levels was extensive (>1,300 sex-biased genes, Figure 2A, comparison 1). The comparison showing the second-lowest degree of sex-differences was the comparison of non-breeding male and non-breeding female canaries (>1,500 sex-biased genes, Figure 2A, comparison 7), both lacking singing behaviour, but HVC volume was male biased (Figure 1A). Testosterone implantation induced singing behaviour and increased the HVC volume of both male and female canaries, although a markedly smaller HVC volume continued to be observed in these females (Figure 1A and Supplementary Figure 1C). Such processes in females are referred to as “masculinisation” of the female brain and behaviour (Arnold and Gorski, 1984; Wade, 2001). However, the gene expression levels of the testosterone-stimulated singing female canaries were very different from those of the male canaries administered the same treatment (>5,000 sex-biased genes, Figure 2A, comparison 4). The comparisons showing the most striking sex differences were the two that included spontaneously singing female canaries. As revealed in these comparisons, many genes showed markedly different regulation in spontaneously singing female canaries, as reflected by expression levels, compared with breeding singing male canaries (>8,600 sex-biased genes, comparison 5) and non-breeding non-singing male canaries (>7,500 sex-biased genes, comparison 6, Figure 2A).
For each comparison, we decomposed the sex-biased genes into two classes, autosomal genes and Z sex chromosomal genes, to assess whether the sex-biased genes were mainly concentrated on the sex chromosomes. Annotation for W chromosomal genes was unfortunately not available in our study. We used Fisher’s exact test to evaluate whether a particular chromosome was enriched in sex-biased genes. We observed enrichments of Z chromosomal genes among the male-biased genes identified in most of the comparisons (Figure 2B and Supplementary Table 1). This observation was not surprising because males are the homogametic sex (have two copies of Z), whereas females are the heterogametic sex (have one copy of Z and one copy of W) in birds, and the dosage compensation for Z-linked genes is less complete in birds than that in mammals (Itoh et al., 2007; Wolf and Bryk, 2011; Mank, 2013; Nätt et al., 2014; Uebbing et al., 2015). However, one canary comparison (breeding singing males and non-breeding singing females, Figure 2B, comparison 5) was an exception (Supplementary Table 1). In this comparison, the spontaneously singing female canaries expressed a high number of Z chromosomal genes (260 genes, approximately 24% of all Z chromosomal genes) at higher levels than the breeding singing male canaries, which eliminated the male enrichment of Z chromosomal genes.
Sex-biased genes were not only Z chromosomal genes; in fact, sizeable numbers of the autosomal genes were found to be sex-biased genes, particularly female-biased genes (male-biased genes: >40%; female-biased genes: >96%, Figure 2B). Many studies have reported sex differences in the gene expression levels in multiple tissues and animal species (Yang et al., 2006; Itoh et al., 2007; Wolf and Bryk, 2011; Frésard et al., 2013; Nätt et al., 2014; Uebbing et al., 2015). Gene expression levels are generally under tight regulation, and perturbed expression levels might result in functional consequences. For example, several genes, including transcription factors, modulate distinct gene sets depending on their expression levels (Birchler et al., 2001; Doghman et al., 2013; Schulz, 2017).
We compared the male-biased genes in a pairwise manner across the comparisons and found that the male-biased autosomal genes showed low similarity to each other (Supplementary Figure 5A), whereas the male-biased Z chromosomal genes showed some similarity across the groups (Supplementary Figure 5B). Pairwise comparison of the female-biased genes across groups showed low similarity, and this finding was obtained for both autosomal genes (Supplementary Figure 5C) and Z chromosomal genes (Supplementary Figure 5D). Fisher’s exact test showed that chromosome 2 (comparisons 1, 4, and, 5) and chromosome 3 (comparisons 1, 2, and 4) are hotspots for female-biased genes; the enrichments of these chromosomes were observed in four out of the seven pairwise comparisons (Supplementary Table 1).
Further examination of the sex-biased genes (comparisons 1, 2, and 5) identified in the groups of naturally singing birds revealed that almost none of the male-biased autosomal genes (Figure 3A) and none of the female-biased Z chromosomal genes (Figure 3B) were shared across the three songbird species. In addition, relatively low numbers of male-biased Z chromosomal genes (Figure 3A) and female-biased autosomal genes (Figure 3B) were shared across the three songbird species. This observation indicates that only a small set of genes were regulated in the same manner (male- or female-biased) between species even though all birds exhibit singing behaviours. Moreover, a Gene Ontology (GO) enrichment analysis suggested that male- and female-biased genes did not functionally converge to similar pathways in the three studied species (Figure 3C).
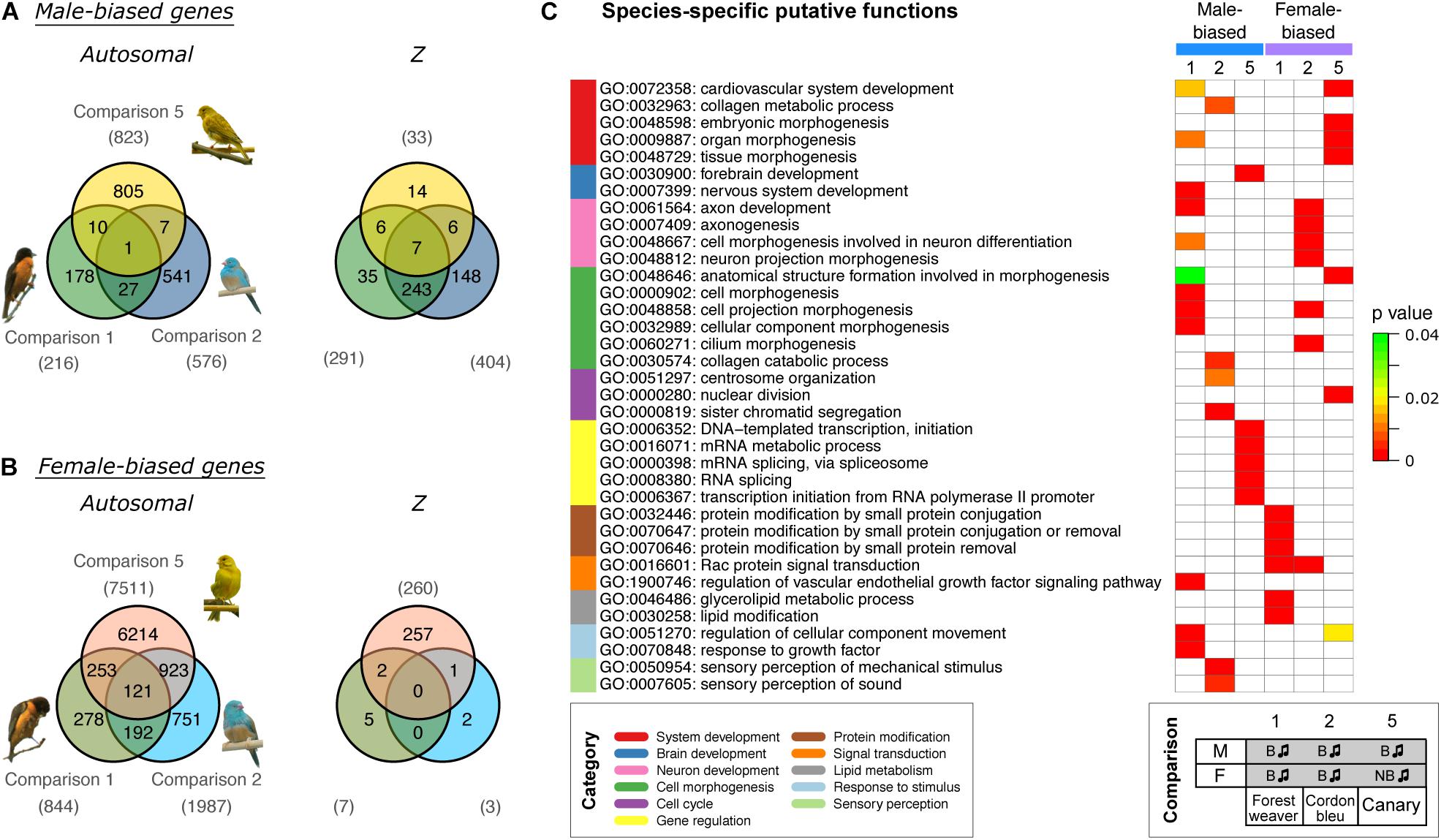
Figure 3. Cross-species comparisons of sex-biased genes expressed in the HVC of birds with singing phenotypes shows high species specificity. Venn diagrams of male-biased (A) and female-biased (B) autosomal and Z chromosomal genes. The numbers of sex-biased genes are indicated in parentheses. (C) GO term enrichment analysis predicting the biological functions of sex-biased (autosomal and Z chromosomal) genes. The GO terms were categorised and are colour coded. Bonferroni-adjusted P-values are shown by colour scales in the heatmap. Only five GO terms with the lowest Bonferroni-adjusted P-values are shown for each set (see Supplementary Table 2 for the complete results). The phenotypes of the groups used for comparisons 1, 2, and 5 are listed at the bottom. B, breeding; NB, non-breeding. The music note indicates the presence or absence of singing behaviour.
The Plasma Androgen Levels Substantially Alter the HVC Transcriptomes of Canaries
With the aim of understanding the features that distinguish the HVC transcriptomes in within-species contexts, we performed a PCA of the HVC transcriptomic data from the seven canary groups to identify variables that would explain the most variation in the data. By calculating the correlation coefficient between the PCs and the variables (plasma testosterone levels (Supplementary Figure 1), HVC volume (Figure 1A), sex, and singing), we identified the variables that were highly correlated with the most important PCs. PC1 explained 32% of the data variance (Figure 1C and Supplementary Figure 6) and was strongly correlated with the blood plasma androgen concentrations and the presence of singing activity (plasma androgen level: Pearson’s r = −0.57, Bonferroni-adjusted p = 1.0 × 10–4; singing: Pearson’s r = −0.51, Bonferroni-adjusted p = 0.001, Supplementary Table 3). Sex and HVC volume were strongly correlated with PC2 (sex: Pearson’s r = −0.91, Bonferroni-adjusted p = 4.4 × 10–16; HVC volume: Pearson’s r = −0.73, Bonferroni-adjusted p = 2.1 × 10–7), which explained 19% of the variance in the data (Supplementary Table 3). Taken together, the hierarchical clustering and PCA results suggest that although phylogenetic relationships dominate the variation between songbird species, the circulating testosterone levels, the presence of singing activity, and sex identity dominate the HVC gene expression patterns within a single species.
The Application of Testosterone Does Not Yield an HVC Transcriptome That Mimics That of Natural Singing Canaries
To investigate the molecular mechanisms underlying the singing phenotype, we performed a differential gene expression analysis of the HVC transcriptomes of the two groups of singing canary females (non-breeding spontaneously singing females and non-breeding testosterone-stimulated singing females) against the non-singing non-breeding female canaries. Similarly, a differential gene expression analysis of the HVC transcriptomes of the two groups of singing male canaries (breeding singing males and non-breeding testosterone-stimulated singing males) with non-singing non-breeding male canaries was performed. A substantial number of genes were differentially expressed between singing and non-singing birds (Figure 4A, non-breeding singing females: 4,125 genes; non-breeding testosterone-stimulated singing females: 7,702 genes; Figure 4B, breeding singing males: 3,359 genes; non-breeding testosterone-stimulated singing males: 5,106 genes). Approximately 65% of the differentially expressed genes found in the naturally singing birds overlapped with those found in the testosterone-treated birds of the same sex (male: 65%; female: 62%). However, both testosterone-treated groups had markedly higher numbers of differentially expressed genes than the naturally singing groups of the same sex (Figures 4A,B). Thus, although testosterone implantation induced singing in females and males, most testosterone-responsive genes (female: 67%; male: 57%) might not be necessary for singing behaviour per se but rather a response to non-physiological levels of testosterone (Supplementary Figure 1). Alternatively, mechanisms that lead to the first song in life might be very different from those that reinduce singing in animals that sang before (Vellema et al., 2019a). Because male canaries sing regularly starting from approximately 50 days of age (Nottebohm et al., 1986) while most female canaries never sing (Ko et al., 2020), the induction of singing in females by testosterone likely activated genes related to first-time singing, whereas in males, this treatment activated genes related to reinduced singing.
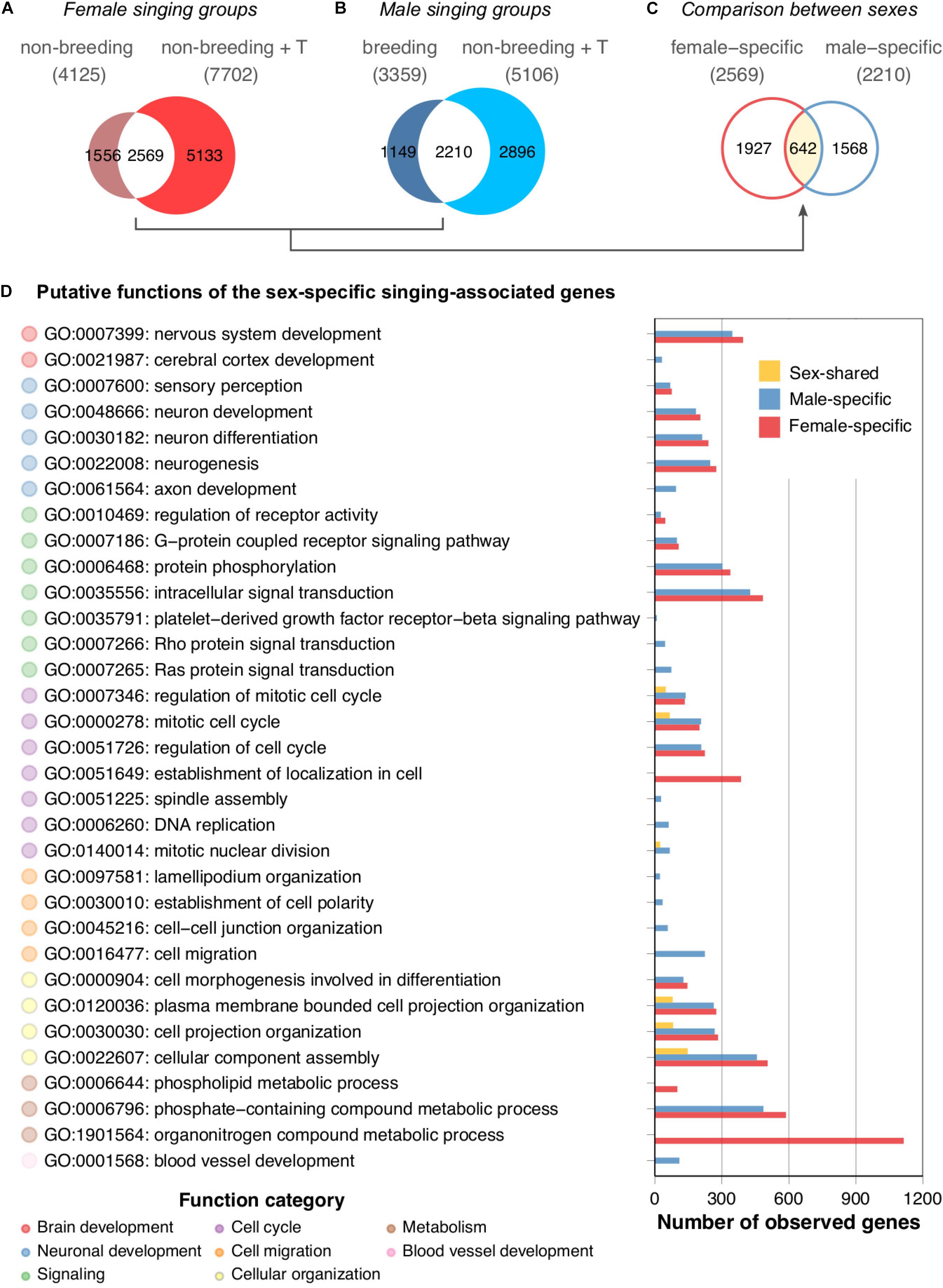
Figure 4. HVC transcriptomes of naturally singing canaries are dissimilar from testosterone-stimulated singing canaries. Venn diagrams comparing (A) the two female singing groups, (B) the two male singing groups, and (C) the female- and male-specific expressed genes resulting from comparisons of panel (A) and panel (B). Note that only approximately 20% of the genes were shared among the singing females and approximately 26% were shared among the singing males. The majority of these overlapping genes were not sex-shared; approximately 75% of female intersections were specific to female groups, whereas 71% of male intersections were specific to male groups. (D) GO term enrichment analysis of female-specific, male-specific, and sex-shared expressed genes derived from comparisons of panels (A) to panel (C). Because many putative functions show similarities between male- and female-specific expressed genes, the results suggest functional intraspecies convergence based on sex-specific gene expression in the HVC. See Supplementary Table 7 for the full results of the GO term enrichment analysis.
The Majority of Differentially Expressed Genes Between Singing and Non-singing Canaries Are Sex-Specific
We examined whether the overlapping gene sets of the female canary signing groups were similar to the overlapping gene sets of the singing males (Figure 4C). The Venn diagram indicated that only approximately 25% (642 of 2569 genes, Supplementary Table 4) of the female-specific expressed genes and 29% (642 of 2210 genes, Supplementary Table 5) of the male-specific expressed genes were shared by both sexes of singing canaries (Supplementary Table 6).
The Sex-Specific Genes Show Functional Overlap
To understand the putative biological functions of the female-specific, male-specific, and sex-shared expressed genes in the HVC, we performed a GO term enrichment analysis. Interestingly, the results suggested that the female- and male-specific expressed genes largely overlapped at the functional level (Figure 4D and Supplementary Table 7). GO terms such as nervous system development (GO:0007399), neuron development (GO:0048666), and intracellular signal transduction (GO:0035556) were shared among the sexes. Female-specific GO terms were mainly related to cellular maintenance, such as organonitrogen compound metabolic process (GO:1901564) and phospholipid metabolic process (GO:0006644). In contrast, the male-specific GO terms were axon development (GO:0061564), DNA replication (GO:0006260), cell migration (GO:0016477), and blood vessel development (GO:0001568). In summary, although only approximately one-fourth to one-third of the sex-specific expressed genes are shared among the sexes in terms of their identities, most of the predicted functions of the sex-specific expressed genes are nevertheless sex-shared in singing canaries.
All four groups of singing canaries had elevated plasma androgen levels compared with non-breeding non-singing canaries of the same sex (Supplementary Figure 1 and Ko et al., 2020). The activation of singing is likely testosterone-dependent in females as in males (Heid et al., 1985; Nottebohm et al., 1987; Hartley and Suthers, 1989; Leitner et al., 2001a). Thus, the potential master regulator for inducing singing behaviour might be testosterone-sensitive and Z-linked. One such candidate is DMRT1 (doublesex and mab-3 related transcription factor 1), which is present in the sex-shared expressed gene list. The Z-linked gene DMRT1 is needed for male sex determination in birds and other animal species (Smith et al., 2009; Lambeth et al., 2014; Herpin and Schartl, 2015). The overexpression of DMRT1 in female chicken embryos reduces aromatase expression in the gonads and triggers development of the testis (Lambeth et al., 2014). The role of DMRT1 in adult avian tissues in general and in the brain in particular is unknown. Thus, whether DMRT1 affects steroid metabolism in the HVC, such as converting testosterone to more active metabolites (see below), or whether it regulates other mechanisms that direct the HVC into a configuration that enables singing needs to be validated by future experiments.
Testosterone can be converted to 5α-dihydrotestosterone (5α-DHT) and 17β-estradiol, which activate androgen receptor (AR) and estrogen receptors (ERα and ERβ), respectively. Both AR (encoded by AR) and ERs (ERα encoded by ESR1 and ERβ encoded by ESR2) are transcription factors that play important roles in the transcription of numerous genes (Bourdeau et al., 2004; Takayama et al., 2007; Pihlajamaa et al., 2015; Wilson et al., 2016). Interestingly, ESR1 was specifically expressed in males, whereas ESR2 was female-specifically expressed in the HVC of canaries, which suggests that estrogen receptor paralogs could provide finer-tuned mechanisms for sex-specific regulation. Empirical results have shown that ERα and ERβ bind to the same estrogen response element motifs (Zhao et al., 2010) and might functionally overlap in some tissues. Moreover, ER paralogs show sex differences in expression levels and tissue specificity (Zhang et al., 2017). In quail, the administration of an agonist specific to ERβ on embryonic day 7 demasculinises male sexual behaviour and midbrain nuclei characteristics in Japanese quails, whereas an agonist specific to ERα does not exert this effect (Court et al., 2020). The specific roles of each ER paralog in the adult HVC of male and female songbirds warrant further investigation. Until now, ERα but not ERβ was expected to regulate the function of HVC neurons of adult songbirds in addition to the AR (Frankl-Vilches and Gahr, 2017).
Conclusion
In this study, we investigated the sex differences in the gene expression patterns in the HVC of three songbird species with different levels of sex-specific singing and several different song-related phenotypes between male and female canaries. Our inter- and intraspecies comparisons yielded large-scale transcriptional sex differences regardless of singing behaviour. Instead, fundamental sex differences in gene expression levels were found in the HVC, and these differences were highly species-specific. By leveraging several experimental groups of canaries, we found that the plasma androgen levels and sex were the major contributors to the variations in the HVC transcriptome. Although testosterone reliably induced singing in both female and male canaries, testosterone treatment did not alter the transcriptome to imitate that of natural singing birds. Our results suggest that female and male canaries rely on different gene networks for singing behaviour, but the sex-specific gene networks might show functional convergence.
Data Availability Statement
The datasets presented in this study can be found in online repositories. The microarray CEL files are available on NCBI’s Gene Expression Omnibus (https://www.ncbi.nlm.nih.gov/geo/query/acc.cgi?acc=GSE83674). The processed microarray data, plasma androgen levels, HVC volume measures, and all scripts used for analysis and visualization are available in GitHub (https://github.com/maggieMCKO/SongbirdSexDiff).
Ethics Statement
The animal study was reviewed and approved by The Government of Upper Bavaria, Germany; Chief Professional Officer for Research at the Natal Parks, Game and Fish Preservation Board, South Africa.
Author Contributions
M-CK collected the non-breeding testosterone-implanted canaries, analysed and visualised the transcriptomic data and was a major contributor to the writing of the manuscript. AB and M-CK performed the RNA extractions and microarray hybridisations. MG and M-CK isolated the tissues for microarray. MG collected the forest weavers and cordon-bleus. MG conceived the study and obtained financial support for the study. M-CK, CF-V, and MG drafted the manuscript. All the authors read and approved the final manuscript.
Funding
This research was supported by Max-Planck-Gesellschaft to MG. The funder had no role in the study design, data collection and analysis, decision to publish, or preparation of the manuscript.
Conflict of Interest
The authors declare that the research was conducted in the absence of any commercial or financial relationships that could be construed as a potential conflict of interest.
Acknowledgments
We thank Dieter Schmidl for catching the forest weavers, Stefan Leitner for providing the breeding canaries, Roswitha Brighton and David Witkowski for maintaining the breeding colonies of the blue-capped cordon-bleus and the canaries, Doris Walcher for providing data from the breeding male and female canaries as well as the non-breeding non-singing male and female canaries, and Wolfgang Goymann and Monika Trappschuh for the RIA of the plasma testosterone. Furthermore, we express our appreciation to Maude Baldwin, Glenn Cockburn, Falk Dittrich, Lisa Gill, Vincent Van Meir, Keren Sadanandan, and Michiel Vellema for their comments on previous versions of this manuscript and to the International Max Planck Research School for Organismal Biology for the training and support provided.
Supplementary Material
The Supplementary Material for this article can be found online at: https://www.frontiersin.org/articles/10.3389/fnins.2021.680530/full#supplementary-material
Supplementary Table 1 | Fisher’s exact tests of sex-biased genes for chromosome enrichment.
Supplementary Table 2 | GO term enrichment analysis of sex-biased genes identified from the forest weaver (comparison 1), cordon-bleu (comparison 2), and canary (comparison 5) comparisons. FWm, forest weaver male-biased genes; CBm, cordon-bleu male-biased genes; Cm, canary male-biased genes; FWf, forest weaver female-biased genes; CBm, cordon-bleu female-biased genes; CfS, canary female-biased genes.
Supplementary Table 3 | Pearson’s correlation analysis of principal components and variables (plasma androgen levels, HVC volume, sex, and singing).
Supplementary Table 4 | Female-specific expressed genes. SDfS, non-breeding spontaneously singing female canaries; SDfT, non-breeding testosterone-stimulated singing female canaries.
Supplementary Table 5 | Male-specific expressed genes. LDm, breeding singing male canaries; SDmT, non-breeding testosterone-stimulated singing male canaries.
Supplementary Table 6 | Sex-shared expressed genes. LDm, breeding singing male canaries; SDfS, non-breeding spontaneously singing female canaries; SDmT, non-breeding testosterone-stimulated singing male canaries; SDfT, non-breeding testosterone-stimulated singing female canaries.
Supplementary Table 7 | GO term enrichment analysis of female-specific, male-specific and sex-shared genes.
Footnotes
References
Alward, B. A., Balthazart, J., and Ball, G. F. (2013). Differential effects of global versus local testosterone on singing behavior and its underlying neural substrate. Proc. Natl. Acad. Sci. U S A. 110, 19573–19578. doi: 10.1073/pnas.1311371110
Arnold, A. P., and Gorski, R. A. (1984). Gonadal steroid induction of structural sex differences in the central nervous system. Annu. Rev. Neurosci. 7, 413–442. doi: 10.1146/annurev.ne.07.030184.002213
Ball, G. F. (2016). Species variation in the degree of sex differences in brain and behaviour related to birdsong: adaptations and constraints. Philos. Trans. R. Soc. Lond. B Biol. Sci. 371:20150117. doi: 10.1098/rstb.2015.0117
Benson, D. A., Karsch-Mizrachi, I., Lipman, D. J., Ostell, J., and Wheeler, D. L. (2005). GenBank. Nucleic Acids Res. 33, D34–D38.
Bernard, D. J., and Ball, G. F. (1997). Photoperiodic Condition Modulates the Effects of Testosterone on Song Control Nuclei Volumes in Male European Starlings. General Comparat. Endocrinol. 105, 276–283. doi: 10.1006/gcen.1996.6829
Bindea, G., Mlecnik, B., Hackl, H., Charoentong, P., Tosolini, M., Kirilovsky, A., et al. (2009). ClueGO: a Cytoscape plug-in to decipher functionally grouped gene ontology and pathway annotation networks. Bioinformatics 25, 1091–1093. doi: 10.1093/bioinformatics/btp101
Birchler, J. A., Bhadra, U., Bhadra, M. P., and Auger, D. L. (2001). Dosage-Dependent Gene Regulation in Multicellular Eukaryotes: Implications for Dosage Compensation, Aneuploid Syndromes, and Quantitative Traits. Dev. Biol. 234, 275–288. doi: 10.1006/dbio.2001.0262
Bourdeau, V. R., Deschenes, J., Meìtivier, R.L., Nagai, Y., Nguyen, D., Bretschneider, N., et al. (2004). Genome-Wide Identification of High-Affinity Estrogen Response Elements in Human and Mouse. Mol. Endocrinol. 18, 1411–1427. doi: 10.1210/me.2003-0441
Brenowitz, E. (1991). Altered perception of species-specific song by female birds after lesions of a forebrain nucleus. Science 251, 303–305. doi: 10.1126/science.1987645
Brenowitz, E. A., Arnold, A. P., and Levin, R. N. (1985). Neural correlates of female song in tropical duetting birds. Brain Res. 343, 104–112. doi: 10.1016/0006-8993(85)91163-1
Cohen, C. D., Lindenmeyer, M. T., Eichinger, F., Hahn, A., Seifert, M., Moll, A. G., et al. (2008). Improved elucidation of biological processes linked to diabetic nephropathy by single probe-based microarray data analysis. PLoS One 3:e2937. doi: 10.1371/journal.pone.0002937
Court, L., Vandries, L., Balthazart, J., and Cornil, C. A. (2020). Key role of estrogen receptor beta in the organization of brain and behavior of the Japanese quail. Horm. Behav. 125:104827. doi: 10.1016/j.yhbeh.2020.104827
Del Negro, C., Gahr, M., Leboucher, G., and Kreutzer, M. (1998). The selectivity of sexual responses to song displays: effects of partial chemical lesion of the HVC in female canaries. Behav. Brain Res. 96, 151–159. doi: 10.1016/s0166-4328(98)00009-6
Dittrich, F., Ramenda, C., Grillitsch, D., Frankl-Vilches, C., Ko, M. C., Hertel, M., et al. (2014). Regulatory mechanisms of testosterone-stimulated song in the sensorimotor nucleus HVC of female songbirds. BMC Neurosci. 15:128. doi: 10.1186/s12868-014-0128-0
Doghman, M., Figueiredo, B. C., Volante, M., Papotti, M., and Lalli, E. (2013). Integrative analysis of SF-1 transcription factor dosage impact on genome-wide binding and gene expression regulation. Nucleic Acids Res. 41, 8896–8907. doi: 10.1093/nar/gkt658
Edgar, R., Domrachev, M., and Lash, A. (2002). Gene Expression Omnibus: NCBI gene expression and hybridization array data repository. Nucleic Acids Res. 30, 207–210. doi: 10.1093/nar/30.1.207
Flicek, P., Amode, M. R., Barrell, D., Beal, K., Billis, K., Brent, S., et al. (2014). Ensembl 2014. Nucleic Acids Res. 42, D749–D755.
Frankl-Vilches, C., and Gahr, M. (2017). Androgen and estrogen sensitivity of bird song: a comparative view on gene regulatory levels. J. Comparat. Physiol. 204, 113–126.
Frankl-Vilches, C., and Gahr, M. (2018). Androgen and estrogen sensitivity of bird song: a comparative view on gene regulatory levels. J. Comparat. Physiol. A 204, 113–126. doi: 10.1007/s00359-017-1236-y
Frankl-Vilches, C., Kuhl, H., Werber, M., Klages, S., Kerick, M., Bakker, A., et al. (2015). Using the canary genome to decipher the evolution of hormone-sensitive gene regulation in seasonal singing birds. Genome Biol. 16:19.
Frésard, L., Morisson, M., Brun, J.-M., Collin, A., Pain, B., Minvielle, F., et al. (2013). Epigenetics and phenotypic variability: some interesting insights from birds. Genet. Select. Evolut. 45:16. doi: 10.1186/1297-9686-45-16
Fusani, L., Metzdorf, R., Hutchison, J., and Gahr, M. (2003). Aromatase inhibition affects testosterone-induced masculinization of song and the neural song system in female canaries. J. Neurobiol. 54, 370–379. doi: 10.1002/neu.10141
Gahr, M. (2001). Distribution of sex steroid hormone receptors in the avian brain: Functional implications for neural sex differences and sexual behaviors. Microscopy Res. Technique 55, 1–11. doi: 10.1002/jemt.1151
Gahr, M., Metzdorf, R., Schmidl, D., and Wickler, W. (2008). Bi-directional sexual dimorphisms of the song control nucleus HVC in a songbird with unison song. PLoS One 3:e3073. doi: 10.1371/journal.pone.0003073
Gahr, M., Sonnenschein, E., and Wickler, W. (1998). Sex difference in the size of the neural song control regions in a dueting songbird with similar song repertoire size of males and females. J. Neurosci. 18, 1124–1131. doi: 10.1523/jneurosci.18-03-01124.1998
Galili, T. (2015). dendextend: an R package for visualizing, adjusting and comparing trees of hierarchical clustering. Bioinformatics 31, 3718–3720. doi: 10.1093/bioinformatics/btv428
Gautier, L., Cope, L., Bolstad, B. M., and Irizarry, R. A. (2004). affy–analysis of Affymetrix GeneChip data at the probe level. Bioinformatics 20, 307–315. doi: 10.1093/bioinformatics/btg405
Geberzahn, N., and Gahr, M. (2011). Undirected (solitary) birdsong in female and male blue-capped cordon-bleus (Uraeginthus cyanocephalus) and its endocrine correlates. PLoS One 6:e26485. doi: 10.1371/journal.pone.0026485
Goymann, W., Möstl, E., and Gwinner, E. (2002). Non-invasive methods to measure androgen metabolites in excrements of European stonechats, Saxicola torquata rubicola. General Comparat. Endocrinol. 129, 80–87. doi: 10.1016/s0016-6480(02)00520-8
Griffiths, R., Double, M. C., Orr, K., and Dawson, R. J. G. (1998). A DNA test to sex most birds. Mol. Ecol. 7, 1071–1075. doi: 10.1046/j.1365-294x.1998.00389.x
Gulledge, C. C., and Deviche, P. (1999). Photoperiod and testosterone independently affect vocal control region volumes in adolescent male songbirds. J. Neurobiol. 36, 550–558. doi: 10.1002/(sici)1097-4695(19980915)36:4<550::aid-neu8>3.0.co;2-v
Gurney, M. E., and Konishi, M. (1980). Hormone-induced sexual differentiation of brain and behavior in zebra finches. Science 208, 1380–1383. doi: 10.1126/science.208.4450.1380
Hahnloser, R. H. R., Kozhevnikov, A. A., and Fee, M. S. (2002). An ultra-sparse code underliesthe generation of neural sequences in a songbird. Nature 419, 65–70. doi: 10.1038/nature00974
Hall, M. L., Rittenbach, M. R. D., and Vehrencamp, S. L. (2015). Female song and vocal interactions with males in a neotropical wren. Front. Ecol. Evolut. 3:12. doi: 10.3389/fevo.2015.00012
Hall, Z. J., MacDougall-Shackleton, S. A., Osorio-Beristain, M., and Murphy, T. G. (2010). Male bias in the song control system despite female bias in song rate in streak-backed orioles (Icterus pustulatus). Brain Behav. Evol. 76, 168–175. doi: 10.1159/000320971
Hartley, R. S., Chinn, M. S., and Ullrich, N. F. E. (1997). Left Syringeal Dominance in Testosterone-Treated Female Canaries. Neurobiol. Learn. Mem. 67, 248–253. doi: 10.1006/nlme.1996.3759
Hartley, R. S., and Suthers, R. A. (1989). Airflow and pressure during canary song: direct evidence for mini-breaths. J. Comparat. Physiol. A 165, 15–26. doi: 10.1007/bf00613795
Heid, P., Güttinger, H. R., and Pröve, E. (1985). The Influence of Castration and Testosterone Replacement on the Song Architecture of Canaries (Serinus canaria). Zeitschrift für Tierpsychologie 69, 224–236. doi: 10.1111/j.1439-0310.1985.tb00148.x
Herpin, A., and Schartl, M. (2015). Plasticity of gene-regulatory networks controlling sex determination: of masters, slaves, usual suspects, newcomers, and usurpators. EMBO Rep. 16, 1260–1274. doi: 10.15252/embr.201540667
Herrick, E. H., and Harris, J. O. (1957). Singing Female Canaries. Science 125, 1299–1300. doi: 10.1126/science.125.3261.1299
Hoffmann, S., Trost, L., Voigt, C., Leitner, S., Lemazina, A., Sagunsky, H., et al. (2019). Duets recorded in the wild reveal that interindividually coordinated motor control enables cooperative behavior. Nat. Commun. 10:2577.
Huang, D. W., Sherman, B. T., and Lempicki, R. A. (2008). Systematic and integrative analysis of large gene lists using DAVID bioinformatics resources. Nat. Protoc. 4, 44–57. doi: 10.1038/nprot.2008.211
Huang, D. W., Sherman, B. T., and Lempicki, R. A. (2009). Bioinformatics enrichment tools: paths toward the comprehensive functional analysis of large gene lists. Nucleic Acids Res. 37, 1–13. doi: 10.1093/nar/gkn923
Immelmann, K. (1968). Zur biologischen Bedeutung des Estrildidengesanges. J. Ornithol. 109, 284–299. doi: 10.1007/bf01678374
Itoh, Y., Melamed, E., Yang, X., Kampf, K., Wang, S., Yehya, N., et al. (2007). Dosage compensation is less effective in birds than in mammals. J. Biol. 6, 1–15. doi: 10.1002/9783527678679.dg01791
Ko, M.-C., Van Meir, V., Vellema, M., and Gahr, M. (2020). Characteristics of song, brain-anatomy and blood androgen levels in spontaneously singing female canaries. Hormones Behav. 117:104614. doi: 10.1016/j.yhbeh.2019.104614
Lambeth, L. S., Raymond, C. S., Roeszler, K. N., Kuroiwa, A., Nakata, T., Zarkower, D., et al. (2014). Over-expression of DMRT1 induces the male pathway in embryonic chicken gonads. Dev. Biol. 389, 160–172. doi: 10.1016/j.ydbio.2014.02.012
Langfelder, P., and Horvath, S. (2008). WGCNA: an R package for weighted correlation network analysis. BMC Bioinform. 9, 1–13. doi: 10.1186/1471-2105-9-559
Langfelder, P., and Horvath, S. (2012). Fast R Functions for Robust Correlations and Hierarchical Clustering. J. Statist. Softw. 46:i11.
Leitner, S., Voigt, C., and Gahr, M. (2001a). Seasonal Changes in the Song Pattern of the Non-Domesticated Island Canary (Serinus Canaria) a Field Study. Behaviour 138, 885–904. doi: 10.1163/156853901753172700
Leitner, S., Voigt, C., Garcia-Segura, L., Van’t Hof, T., and Gahr, M. (2001b). Seasonal activation and inactivation of song motor memories in wild canaries is not reflected in neuroanatomical changes of forebrain song areas. Horm. Behav. 40, 160–168. doi: 10.1006/hbeh.2001.1700
Leonard, S. L. (1939). Induction of Singing in Female Canaries by Injections of Male Hormone. Exp. Biol. Med. 41, 229–230. doi: 10.3181/00379727-41-10631
Lobato, M., Vellema, M., Gahr, C., Leitão, A., de Lima, S. M. A., Geberzahn, N., et al. (2015). Mismatch in sexual dimorphism of developing song and song control system in blue-capped cordon-bleus, a songbird species with singing females and males. Front. Ecol. Evolut. 3:117. doi: 10.3389/fevo.2015.00117
MacDougall-Shackleton, S. A., and Ball, G. F. (1999). Comparative studies of sex differences in the song-control system of songbirds. Trends Neurosci. 22, 432–436. doi: 10.1016/s0166-2236(99)01434-4
Madison, F. N., Rouse, M. L. Jr., Balthazart, J., and Ball, G. F. (2015). Reversing song behavior phenotype: Testosterone driven induction of singing and measures of song quality in adult male and female canaries (Serinus canaria). Gen. Comp. Endocrinol. 215, 61–75. doi: 10.1016/j.ygcen.2014.09.008
Mank, J. E. (2013). Sex chromosome dosage compensation: definitely not for everyone. Trends Genet. 29, 677–683. doi: 10.1016/j.tig.2013.07.005
Nätt, D., Agnvall, B., and Jensen, P. (2014). Large Sex Differences in Chicken Behavior and Brain Gene Expression Coincide with Few Differences in Promoter DNA-Methylation. PLoS One 9:e96376. doi: 10.1371/journal.pone.0096376
Nixdorf, B. E., Davis, S. S., and DeVoogd, T. J. (1989). Morphology of Golgi-impregnated neurons in hyperstriatum ventralis, pars caudalis in adult male and female canaries. J. Comp. Neurol. 284, 337–349. doi: 10.1002/cne.902840302
Nottebohm, F. (1980). Testosterone triggers growth of brain vocal control nuclei in adult female canaries. Brain Res. 189, 429–436. doi: 10.1016/0006-8993(80)90102-x
Nottebohm, F., and Arnold, A. P. (1976). Sexual dimorphism in vocal control areas of the songbird brain. Science 194, 211–213. doi: 10.1126/science.959852
Nottebohm, F., Nottebohm, M., Crane, L., and Wingfield, J. (1987). Seasonal changes in gonadal hormone levels of adult male canaries and their relation to song. Behav. Neural. Biol. 47, 197–211. doi: 10.1016/s0163-1047(87)90327-x
Nottebohm, F., Nottebohm, M. E., and Crane, L. (1986). Developmental and seasonal changes in canary song and their relation to changes in the anatomy of song-control nuclei. Behav. Neural Biol. 46, 445–471. doi: 10.1016/s0163-1047(86)90485-1
Nottebohm, F., Stokes, T. M., and Leonard, C. M. (1976). Central control of song in the canary, Serinus canarius. J. Comparat. Neurol. 165, 457–486. doi: 10.1002/cne.901650405
Ota, N., Gahr, M., and Soma, M. (2018). Couples showing off: Audience promotes both male and female multimodal courtship display in a songbird. Sci. Adv. 4:eaat4779. doi: 10.1126/sciadv.aat4779
Pesch, A., and Güttinger, H.-R. (1985). Der Gesang des weiblichen Kanarienvogels. J. Für Ornithol. 126, 108–110. doi: 10.1007/bf01640450
Pihlajamaa, P., Sahu, B., and Jänne, O. A. (2015). Determinants of Receptor- and Tissue-Specific Actions in Androgen Signaling. Endocr. Rev. 36, 357–384. doi: 10.1210/er.2015-1034
Price, J. J. (2019). Sex differences in song and plumage color do not evolve through sexual selection alone: new insights from recent research. J. Ornithol. 160, 1213–1219. doi: 10.1007/s10336-019-01681-8
Price, J. J., Lanyon, S. M., and Omland, K. E. (2009). Losses of female song with changes from tropical to temperate breeding in the New World blackbirds. Proce. R. Soc. B Biol. Sci. 276, 1971–1980. doi: 10.1098/rspb.2008.1626
R Core Team (2020). R: A language and environment for statistical computing [Internet]. Vienna: R Foundation for Statistical Computing.
Rasika, S., Nottebohm, F., and Alvarez-Buylla, A. (1994). Testosterone increases the recruitment and/or survival of new high vocal center neurons in adult female canaries. Proc. Natl. Acad. Sci. U S A. 91, 7854–7858. doi: 10.1073/pnas.91.17.7854
Sayers, E. W., Beck, J., Brister, J. R., Bolton, E. E., Canese, K., Comeau, D. C., et al. (2020). Database resources of the National Center for Biotechnology Information. Nucleic Acids Res. 48, D9–D16.
Schulz, E. G. (2017). X-chromosome dosage as a modulator of pluripotency, signalling and differentiation? Philosop. Transact. R. Soc. B Biol. Sci. 372:20160366. doi: 10.1098/rstb.2016.0366
Schwabl, H., Dowling, J., Baldassarre, D. T., Gahr, M., Lindsay, W. R., and Webster, M. S. (2015). Variation in song system anatomy and androgen levels does not correspond to song characteristics in a tropical songbird. Anim. Behav. 104, 39–50. doi: 10.1016/j.anbehav.2015.03.006
Shannon, P., Markiel, A., Ozier, O., Baliga, N. S., Wang, J. T., Ramage, D., et al. (2003). Cytoscape: a software environment for integrated models of biomolecular interaction networks. Genome Res. 13, 2498–2504. doi: 10.1101/gr.1239303
Shoemaker, H. H. (1939). Effect of Testosterone Propionate on Behavior of the Female Canary. Proc. Soc. Exp. Biol. Med. 41, 299–302. doi: 10.3181/00379727-41-10652
Slater, P. J. B., and Mann, N. I. (2004). Why do the females of many bird species sing in the tropics? J. Avian Biol. 35, 289–294. doi: 10.1111/j.0908-8857.2004.03392.x
Smeds, L., Warmuth, V., Bolivar, P., Uebbing, S., Burri, R., Suh, A., et al. (2015). Evolutionary analysis of the female-specific avian W chromosome. Nat. Commun. 6:7330.
Smith, C. A., Roeszler, K. N., Ohnesorg, T., Cummins, D. M., Farlie, P. G., Doran, T. J., et al. (2009). The avian Z-linked gene DMRT1 is required for male sex determination in the chicken. Nature 461, 267–271. doi: 10.1038/nature08298
Smith, G. T., Brenowitz, E. A., and Wingfield, J. C. (1997). Roles of photoperiod and testosterone in seasonal plasticity of the avian song control system. J. Neurobiol. 32, 426–442. doi: 10.1002/(sici)1097-4695(199704)32:4<426::aid-neu6>3.0.co;2-8
Stacklies, W., Redestig, H., Scholz, M., Walther, D., and Selbig, J. (2007). pcaMethods—a bioconductor package providing PCA methods for incomplete data. Bioinformatics 23, 1164–1167. doi: 10.1093/bioinformatics/btm069
Takayama, K., Kaneshiro, K., Tsutsumi, S., Horie-Inoue, K., Ikeda, K., Urano, T., et al. (2007). Identification of novel androgen response genes in prostate cancer cells by coupling chromatin immunoprecipitation and genomic microarray analysis. Oncogene 26:4453. doi: 10.1038/sj.onc.1210229
Tusher, V. G., Tibshirani, R., and Chu, G. (2001). Significance analysis of microarrays applied to the ionizing radiation response. Proc. Natl. Acad. Sci. U S A. 98, 5116–5121. doi: 10.1073/pnas.091062498
Uebbing, S., Konzer, A., Xu, L., Backström, N., Brunström, B., Bergquist, J., et al. (2015). Quantitative Mass Spectrometry Reveals Partial Translational Regulation for Dosage Compensation in Chicken. Mol. Biol. Evolut. 32, 2716–2725. doi: 10.1093/molbev/msv147
Vallet, E., Kreutzer, M., and Gahr, M. (1996). Testosterone Induces Sexual Release Quality in the Song of Female Canaries. Ethology 102, 617–628. doi: 10.1111/j.1439-0310.1996.tb01153.x
Van Meir, V., Verhoye, M., Absil, P., Eens, M., Balthazart, J., and Van der Linden, A. (2004). Differential effects of testosterone on neuronal populations and their connections in a sensorimotor brain nucleus controlling song production in songbirds: a manganese enhanced-magnetic resonance imaging study. Neuroimage 21, 914–923. doi: 10.1016/j.neuroimage.2003.10.007
Vellema, M., Diales Rocha, M., Bascones, S., Zsebők, S., Dreier, J., Leitner, S., et al. (2019a). Accelerated redevelopment of vocal skills is preceded by lasting reorganization of the song motor circuitry. eLife 8:e43194.
Vellema, M., Rocha, M., Bascones, S., Zsebõk, S., Dreier, J., Leitner, S., et al. (2019b). Accelerated redevelopment of vocal skills is preceded by lasting reorganization of the song motor circuitry. eLife 8:e43194.
Voigt, C., and Leitner, S. (2008). Seasonality in song behaviour revisited: Seasonal and annual variants and invariants in the song of the domesticated canary (Serinus canaria). Hormones Behav. 54, 373–378. doi: 10.1016/j.yhbeh.2008.05.001
Wade, J. (2001). Zebra finch sexual differentiation: The aromatization hypothesis revisited. Microscopy Res. Techniq. 54, 354–363. doi: 10.1002/jemt.1148
Warren, W., Clayton, D., Ellegren, H., Arnold, A., Hillier, L., and Kunstner, A. (2010). The genome of a songbird. Nature 464, 757–762.
Wickler, W., and Seibt, U. (1980). Vocal Dueting and the Pair Bond II. Unisono dueting of the African forest weaver, Symplectes bicolor. Zeitschrift Für Tierpsychol. 52, 217–226. doi: 10.1111/j.1439-0310.1980.tb00713.x
Wild, J. M. (2004). Functional Neuroanatomy of the Sensorimotor Control of Singing. Ann N. Y. Acad. Sci. 1016, 438–462. doi: 10.1196/annals.1298.016
Wilson, S., Qi, J., and Filipp, F. V. (2016). Refinement of the androgen response element based on ChIP-Seq in androgen-insensitive and androgen-responsive prostate cancer cell lines. Sci. Rep. 6:32611.
Wingfield, J. C., Smith, J. P., and Farner, D. S. (1982). Endocrine Responses of White-Crowned Sparrows to Environmental Stress. Condor 84, 399–409. doi: 10.2307/1367443
Wolf, J. B., and Bryk, J. (2011). General lack of global dosage compensation in ZZ/ZW systems? Broadening the perspective with RNA-seq. BMC Genomics 12:91. doi: 10.1186/1471-2164-12-91
Yang, X., Schadt, E. E., Wang, S., Wang, H., Arnold, A. P., Ingram-Drake, L., et al. (2006). Tissue-specific expression and regulation of sexually dimorphic genes in mice. Genome Res. 16, 995–1004. doi: 10.1101/gr.5217506
Yates, A., Akanni, W., Amode, M. R., Barrell, D., Billis, K., Carvalho-Silva, D., et al. (2016). Ensembl 2016. Nucleic Acids Res. 44, D710–D716.
Zhang, R., Yin, Y., Sun, L., Yan, P., Zhou, Y., Wu, R., et al. (2017). Molecular cloning of ESR2 and gene expression analysis of ESR1 and ESR2 in the pituitary gland of the Chinese alligator (Alligator sinensis) during female reproductive cycle. Gene 623, 15–23. doi: 10.1016/j.gene.2017.04.028
Keywords: sex differences, songbirds, gene expression, brain, HVC, testosterone, singing behaviour
Citation: Ko M-C, Frankl-Vilches C, Bakker A and Gahr M (2021) The Gene Expression Profile of the Song Control Nucleus HVC Shows Sex Specificity, Hormone Responsiveness, and Species Specificity Among Songbirds. Front. Neurosci. 15:680530. doi: 10.3389/fnins.2021.680530
Received: 14 March 2021; Accepted: 28 April 2021;
Published: 31 May 2021.
Edited by:
Heather K. Caldwell, Kent State University, United StatesReviewed by:
Jennifer Marie Barker, University of Liège, BelgiumGregoy Y. Bedecarrats, University of Guelph, Canada
Copyright © 2021 Ko, Frankl-Vilches, Bakker and Gahr. This is an open-access article distributed under the terms of the Creative Commons Attribution License (CC BY). The use, distribution or reproduction in other forums is permitted, provided the original author(s) and the copyright owner(s) are credited and that the original publication in this journal is cited, in accordance with accepted academic practice. No use, distribution or reproduction is permitted which does not comply with these terms.
*Correspondence: Meng-Ching Ko, mcko@orn.mpg.de, orcid.org/0000-0002-2234-9380