- 1Department of Neuroscience and Pharmacology, University of Iowa Carver College of Medicine, Iowa City, IA, United States
- 2F.O.E. Diabetes Research Center, University of Iowa Carver College of Medicine, Iowa City, IA, United States
- 3Obesity Research and Educational Initiative, University of Iowa Carver College of Medicine, Iowa City, IA, United States
- 4Iowa Neuroscience Institute, University of Iowa Carver College of Medicine, Iowa City, IA, United States
- 5Eating Recovery Center of San Antonio, San Antonio, TX, United States
Eating disorders (EDs) are serious mental illnesses thought to arise from the complex gene-environment interactions. DNA methylation patterns in histone deacetylase 4 (HDAC4) locus have been associated with EDs and we have previously identified a missense mutation in the HDAC4 gene (HDAC4A786T) that increases the risk of developing an ED. In order to evaluate the biological consequences of this variant and establish a useful mouse model of EDs, here we performed behavioral characterization of mice homozygous for Hdac4A778T (corresponding to human HDAC4A786T) that were further backcrossed onto C57BL/6 background. When fed high-fat diet, male, but not female, homozygous mice showed a trend toward decreased weight gain compared to their wild-type littermates. Behaviorally, male, but not female, homozygous mice spent less time in eating and exhibited reduced motivation to work for palatable food and light phase-specific decrease in locomotor activity. Additionally, homozygous Hdac4A778T female, but not male, mice display social subordination when subjected to a tube dominance test. Collectively, these results reveal a complex sex- and circadian-dependent role of ED-associated Hdac4A778T mutation in affecting mouse behaviors. Homozygous Hdac4A778T mice could therefore be a useful animal model to gain insight into the neurobiological basis of EDs.
Introduction
Eating disorders (EDs), such as anorexia nervosa (AN) and bulimia nervosa (BN), are serious mental illnesses thought to develop as a result of complex gene-environment interaction (Hinney et al., 2010; Carmichael and Lockhart, 2012; Lutter et al., 2016; Baker et al., 2017). In spite of EDs having the highest mortality rate of all mental disorders, available treatments are extremely limited, mainly due to the poor understanding of the underlying neurobiology of EDs and lack of useful animal models that can be used to develop novel treatment strategies (Lutter et al., 2016; Lutter, 2017). Among the proposed factors contributing to EDs, genetics plays a major role in the development of an ED, as family and twin studies indicate that the risk of developing an ED is 60–80% heritable (Lilenfeld et al., 1998; Strober et al., 2000; Karwautz et al., 2002; Baker et al., 2017).
Recent advancement in large scale genetic approaches provide some clues in understanding the genetic basis of EDs (Wang et al., 2011; Boraska et al., 2014; Duncan et al., 2017; Huckins et al., 2018; Watson et al., 2019), but replicable and definitive genetic links to EDs have not yet been established. By combining genetic linkage analysis and whole exome sequencing in two independent families in which multiple members are affected by EDs, we have previously identified two missense mutations in the estrogen-related receptor alpha (ESRRAR188Q) and histone deacetylase 4 (HDAC4A786T) genes, respectively (Cui et al., 2013), that increase the risk of developing an ED. While the family in which ESRRAR188Q mutation was found has a high rate of AN and comorbidity of obsessive-compulsive disorder (OCD), another family in which HDAC4A786T mutation was found has higher rates of BN but significantly lower rates of comorbid OCD. Multiple independent studies have shown that many CpG sites located in genomic region around 5′ upstream of HDAC4 gene are differentially methylated in the peripheral tissues of patients with AN (Booij et al., 2015; Kesselmeier et al., 2018; Subramanian et al., 2018; Sild and Booij, 2019; Steiger et al., 2019), further supporting the role of HDAC4 in the pathophysiology of EDs. In the followup mouse studies, we found that Esrra knockout mice display behavioral deficits relevant to EDs, including reduced food intake, decreased motivation to work for high-fat diet (HFD), and social subordination (Cui et al., 2015). Furthermore, mice heterozygous for Hdac4A778T [corresponding to the human HDAC4A786T mutation which we previously reported to be a gain-of-function mutation in the catalytic domain of HDAC4 to increase gene suppression in cultured cells (Cui et al., 2013)] on a mixed C57BL/6 and 129/Sv genetic background display altered feeding behaviors depending on housing condition (Lutter et al., 2017). In order to definitively evaluate the biological consequence of Hdac4A778T mutation, here we performed a comprehensive behavioral characterization in homozygous Hdac4A778T mice that we further backcrossed onto C57BL/6 background till reach over 98% of purity. We report that homozygous Hdac4A778T mice, which are raised by heterozygous Hdac4A778T dams on a C57BL/6 background, display behavioral alterations that are distinct from heterozygous Hdac4A778T mice previously reported. Our results may indicate a complex role for Hdac4A778T mutation in affecting mouse behaviors in different genetic background and growth environment with sex-dependent manner.
Materials and Methods
Animal Usage
All animal procedures were performed in accordance with University of Iowa Institutional Animal Care and Use Committee guidelines. Mice were handled in accordance with the Guideline for the Care and Use of Laboratory Animals as adopted by the United States National Institutes of Health. Specific protocols were approved by the Institutional Animal Care and Use Committee. Mice were housed in the University of Iowa vivarium in a temperature-controlled environment (lights on: 06:00–18:00) with ad libitum access to water and regular chow (7913 NIH-31 modified open formula mouse sterilized diet, Harlan-Teklad) or 60% HFD (Research Diet, #D12492) as noted.
Generation of HDAC4A778T Mice
Hdac4A778T point mutant knock-in mouse was generated by introducing Hdac4A778T mutation that correspond to human HDAC4A786T mutation (Figure 1A) by CRISPR technology as previously reported (Lutter et al., 2017). Heterozygous mice with mixed C57BL/6 and 129/Sv genetic background were further backcrossed to C57BL/6 female mice until reach over 98% of C57BL/6 background. Male and female heterozygous Hdac4A778T mutant mice were bred to generate wild-type (WT) and homozygous Hdac4A778T mice for experimental use. Mice were weaned at 3 weeks and genotyped by PCR amplification of tail genomic DNA encompassing HDAC4A778T mutation (primer pairs used: 5′-TCAGCCACTGGGAAGGTTAG-3′ and 5′-AGCCCAGGTCTGTTAGAGCA-3′) followed by restriction enzyme digestion (BstEII-HF, New England Biolab) at 37°C for 2 h. Genotypes were determined by running BStEII-digested PCR product in agarose gel as shown Figure 1B.
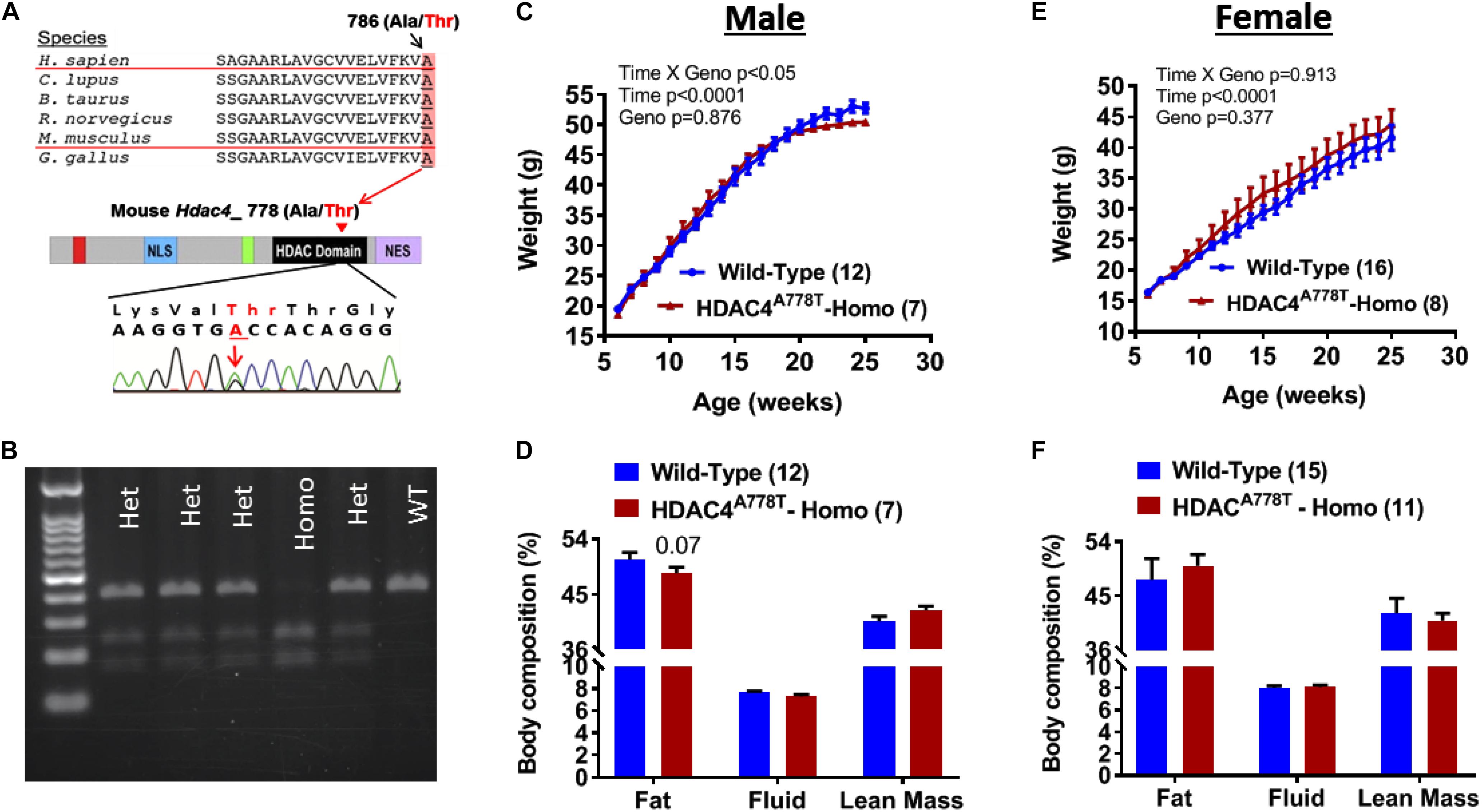
Figure 1. The effects of Hdac4A778T mutation on body weight and body composition. (A) Schematic drawing of conserved human HDAC4A786T mutation across the species and corresponding mouse Hdac4A778T mutation in the catalytic domain of HDAC4. (B) Representative genotyping image of Hdac4A778T mutation by agarose gel electrophoresis of BStEII-digested PCR product. (C–F) Male mice body weight (C) and body composition, and female mice body weight (E) and body composition (F) on HFD. Data are presented as mean ± SEM.
Body Weight Gain and Body Composition on High-Fat Diet (HFD)
An independent cohort of mice was produced for metabolic phenotyping on HFD. Mice were weaned and genotyped by tail snip and PCR at 3 weeks of age. Group-housed mice were fed 60% HFD (Research Diet, #D12492) for 20 weeks beginning at 6 weeks of age and body weight was monitored weekly. At the end of the study, body composition for some of mice was determined by the University of Iowa Metabolic Phenotyping Core using a Bruker Minispec LF50 (Bruker, Billerica, MA, United States).
Behavioral Studies
Two different cohorts of seven to 12-week-old mice kept on a normal chow diet were used for behavioral studies. The first large cohort of male and female mice were sequentially (in order of least to most stressful) subjected to rotarod, elevated plus maze test, open field test, light/dark box test, LABORAS test, social interaction test, social dominance test, sucrose preference test, tail suspension test, forced swim test, and operant responding test. A second independent cohort of male and female mice were produced and subjected to binge-like eating behavioral paradigm.
Rotarod
Mice were placed on a rotarod apparatus (IITC Life Science, Woodland Hills, CA, United States) and the rotational speed was ramped up from an initial 4 rpm to 40 rpm in 4 min. Mice were recorded in sets of five mice at a time. The time for each mouse to fall off the rotarod was measured for three trials consecutively before moving on to the next set of mice. Mice were then placed back into their original home cages for ∼24 h. Data for each trial was averaged to generate an average daily score for each mouse. The test was repeated over the next 2 days for a total of 3 days of testing.
Elevated Plus Maze
Mice were subjected to the elevated plus maze (EPM) test to test for anxiety-like behavior as previously reported (Cui et al., 2015; Lutter et al., 2017). Mice were brought to the testing room approximately 1 h prior to testing to allow time for mice to acclimate to the testing environment. Mice were placed in the center of the apparatus and allowed to explore for 5 min during which the mice were tracked using ANY-Maze Behavioral tracking software (Stoelting, Wood Dale, IL, United States). The time spent in the center, the open or closed arms were measured and analyzed.
Open Field Test
Mice were subjected to the open field test to evaluate for novel environment exploration and anxiety-related behavior as previously reported (Cui et al., 2015; Lutter et al., 2017). Mice were placed in the testing room approximately 1 h prior to testing to allow time for the mice to acclimate to the testing environment. Each mouse was placed in the center of an open box (42 cm × 42 cm) and allowed to explore for 5 min. Subjects were recorded and time spent in the center of the box (20 cm × 20 cm2) and time spent in the edges of the field was recorded. Data was collected using ANY-Maze behavioral tracking software (Stoelting, Wood Dale, IL, United States).
Light-Dark Box Test
The Light-Dark box test was performed under dimmed light conditions as reported previously (Cui et al., 2015; Lutter et al., 2017). During 10 min of testing, the time spent on the light side of the box and distance traveled was calculated using ANY-maze behavioral tracking software (Stoelting).
Laboratory Animal Behavior Observation Registration and Analysis System (LABORAS)
Mice were placed in an automated LABORAS system (Metris, Netherlands), to quantify the locomotion and the time spent on eating, drinking, climbing, grooming, and rearing in a home cage-like environment as previously reported (Cui et al., 2015; Lutter et al., 2017). Mice were placed in the cage shortly after the beginning of the light cycle and the system was left to collect data for up to 24 h. To allow the system to accurately track and measure the animal’s behavior, mice were singly-housed in these cages for the duration of the test. The data were analyzed and compared separately for light and dark cycles.
Three-Chamber Sociability and Social Novelty Test
The social interaction test was performed as previously described (Cui et al., 2015; Lutter et al., 2017). Briefly, two novel female and male mice were selected as novel mice and allowed to acclimate for 5 min each in a small cage within a three-chambered apparatus. Each test mouse was then given 5 min to explore the three chambers. The test mouse was then moved to the middle chamber and trapped by closing the doors to the left and right chambers. The novel mouse was then placed in a side chamber, the doors were removed and the test mouse was allowed to explore the apparatus for 10 min. The test mouse was then moved back and trapped in the middle chamber, and the second novel mouse was then placed in the chamber opposite the initial novel mouse. The doors were removed and the mouse was again allowed another 10 min to explore the apparatus. The side that the novel mouse is placed on was alternated in between each test mouse. Behavioral activity was recorded and measured using ANY-Maze behavioral tracking software (Stoelting).
Social Dominance Test
Tube dominance test was performed as previously reported (Cui et al., 2015; Lutter et al., 2017). Each mouse under went 3 days of training prior to the test day. During the first training day, each mouse was guided into a three foot-long tube with an inner-diameter of 3/8-inches. A dark enclosure (the goal box) was fixed at the other end of the tube and the mouse was given 180 s to reach the goal box. This process was repeated for each mouse on the first day of training. On the subsequent training days, each mouse performed the same task twice as done on first day training, but each subject was only given 30 s to reach the goal box. During training, the subject was forced to the goal box after the time expired. On test day, wild-type and mutant mice were set up in pairs in a round-robin tournament ensuring that mice in each pair had never previously interacted. A partition was placed in the middle of the tubing and each mouse of each pair was placed at opposite ends of the tubing. Once mice reached the middle of the tube the partition was removed. The inner-diameter of the tube was such that mice were unable to turn around. The first mouse to retreat from the tubing was categorized as the loser, and the other as the winner.
Sucrose Preference Test
The sucrose preference test can assay for anhedonia in mice, which can be used as an associative measurement of depression. Mice were singly-housed and given free access to either a 2% sucrose solution or water. The solutions were placed in 50 mL conical tubes that were fitted with an animal drinking nozzle in a rubber stopper. Amounts of solution taken were measured over 4 days, during which the location of the bottles was switched every day to avoid place preference. Amounts were taken by change in weight. The data were only analyzed for the last 2 days were used for the data analysis. Sucrose preference was calculated by the ratio of amount of sucrose taken over total solution consumed and compared between the groups.
Tail Suspension Test
Tail suspension behavioral despair test was performed to evaluate depression-like symptoms as previously reported (Castagne et al., 2010). Each mouse had its tail taped to a hook and was left hanging for 6 min. Video of the subject’s movements and behavior was recorded using ANY-maze behavioral tracking software. The videos were then manually scored for time that each mouse spent immobile and compared between the groups.
Forced Swim Test
Test was performed as described previously (Castagne et al., 2010; Cui et al., 2015; Lutter et al., 2017). ANY-Maze behavioral tracking settings were identical to the previous study.
Operant Responding Test
Mice were tested for motivation for high-fat diet through operant responding as previously described (Cui and Lutter, 2013; Cui et al., 2015; Lutter et al., 2017). Briefly, mice were trained to press a lever for a high-fat diet pellet reward. Mice were trained to pass one fixed-ratio (FR) 1 program, two FR 3 programs, and three FR 5 programs prior to testing. Mice were fasted for ∼20 h prior to each training session. Testing consisted of 6 days. During the first three test days, mice continued to be fasted for ∼20 h and were placed on progressive ratio schedules. Mice were given ad lib feeding on days 4–6. The operant conditioning apparatus was cleaned with Windex after uses.
Binge Eating Test
Finally, binge eating behavior was assessed in mice. Another cohort of mice were singly-housed and given free access to both a high-sugar high-fat diet (Research Diets, New Brunswick, NJ, United States), and regular chow. The test was performed in a similar manner to methods previously performed (Czyzyk et al., 2010). Diets dispensed in two separate food hoppers on either the left or right side of the cage top. The side that the food was placed on was randomized. The testing consisted of seven cycles lasting a week at a time. Food intake data was collected each week 2.5 and 24 h after reintroducing the mice to HFD each week. After each 24 h recording, HFD was removed but mice were still kept on regular chow during the remainder of the week, until the cycle would start again and mice were reintroduced.
Statistical Analyses
Statistical analyses were performed using GraphPad Prism (GraphPad Software, La Jolla, CA, United States) software. Comparisons between groups were made by Student’s t-test (anxiety-like behavior, depression-like behaviors, and social dominance test), multiple t-test (body composition and LABORAS), one-way (three-chamber sociability test) or repeated measure two-way ANOVA (Rotarod, body weight, operant responding test, and binge-like eating with genotype and time as independent variables) with Sidak post hoc analysis as needed. P < 0.05 was considered statistically significant. Data are presented as mean ± SEM.
Results
The Effects of Hdac4A778T Mutation on Body Weight and Body Composition
Male and female mice heterozygous for Hdac4A778T mutation (>98% of C57BL/6 genetic background) were bred together to generate experimental cohorts of mice. Pups were then weaned and genotyped at 3 weeks of age (Figure 1B). At 6 weeks of age, group-housed mice were given access to 60% high-fat diet (HFD) and body weights were recorded until 26 weeks of age. Group-housed homozygous male mice showed slight but significant reduction in body weight gain compared to their WT littermates (Genotype × Time interaction with repeated measure two-way ANOVA, F19,323 = 1.853, p = 0.017, Figure 1C) without significant change of body composition (Figure 1D), whereas female homozygous mice did not show statistical significance (Genotype × Time interaction with repeated measure two-way ANOVA, F19,399 = 0.591, p = 0.913, Figure 1E). No significant change in body composition was observed for female mice (Figure 1F).
The Effects of Hdac4A778T Mutation on Motor Coordination and Anxiety- and Depression-Like Behaviors
To first determine whether mice homozygous for Hdac4A778T mutation have impaired motor coordination, we performed the rotarod test and found no difference in motor performance between WT and homozygous mice for both sexes (Supplementary Figures S1A,B). Mice were then subjected to three different behavioral paradigms frequently used to determine levels of anxiety in mice: elevated plus maze test (EPM), open field test (OF), and light/dark box test (LDB). For EPM, homozygous male mice spend less time on open arms compared to their WT littermates (by Student t-test, t(29) = 2.27, p = 0.0154, Figure 2A), while homozygous female mice tend to spend more time on open arms though it did not reach statistical significance (Figure 1B). Distance traveled during 5 min of EPM test was comparable between the groups for both male and female mice (Figures 2A,B). For OF test, the time spent in center zone and the distance traveled during the test was comparable between the groups for both sexes (Figures 2C,D). Likewise, no difference was observed for the time spent in light box or the number of entries to light box between the groups for both sexes (Figures 2E,F).
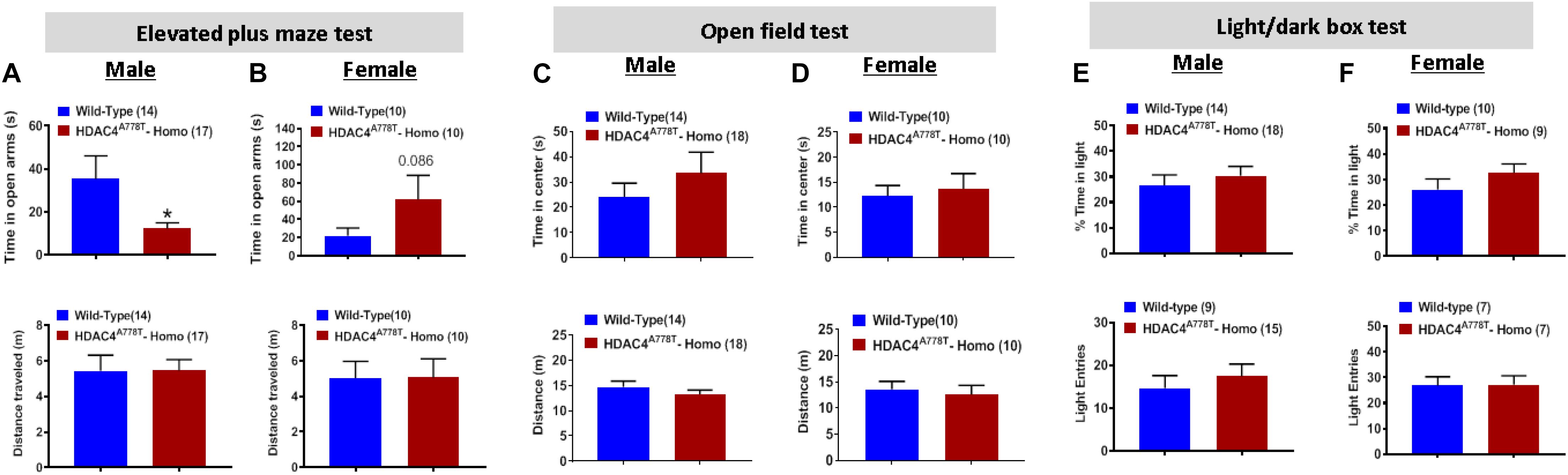
Figure 2. The effects of Hdac4A778T mutation on anxiety-like behaviors. (A,B) The time spent in open arms and total distance traveled during EPM test for both male (A) and female (B) mice. (C,D) The time spent in center zone and total distance traveled during OF test for both male (C) and female (D) mice. (E,F) The% of time spent in light box and the number of entries to light box during L/D test for both male (E) and female (F) mice (*p < 0.05). Data are presented as mean ± SEM.
We also subjected mice to three well-established behavioral paradigms used to evaluate the levels of depression-like symptoms: tail suspension test (TST), forced swim test (FST), and sucrose preference test (SPT). For all three tests, we did not observe any significant differences between the groups for both sexes (Figures 3A–F), except female homozygous mice showed a non-statistically significant increase in immobile time during FST compared to their WT littermates (Figure 3D).
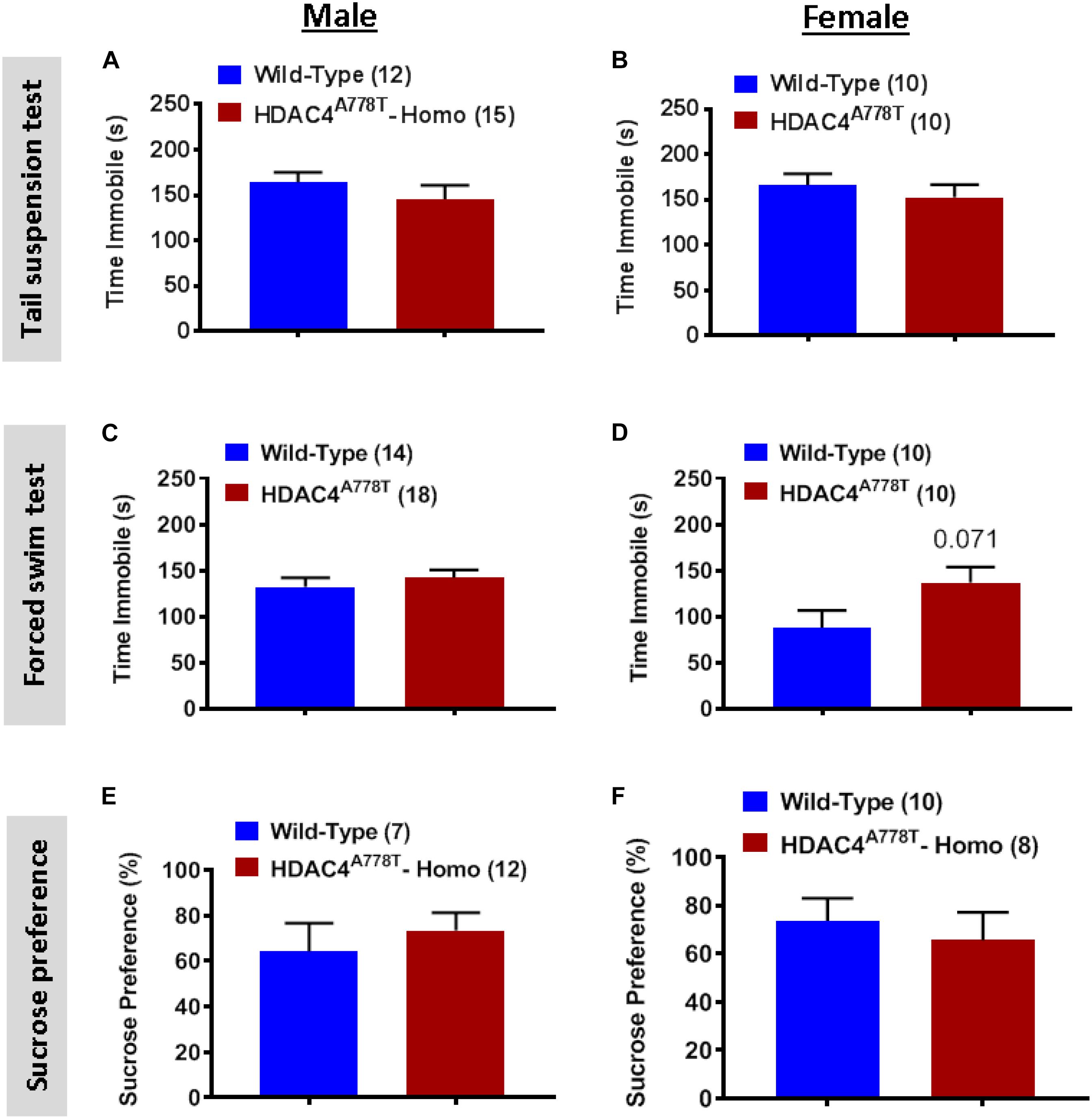
Figure 3. The effects of homozygous Hdac4A778T mutation on depression-like behaviors. (A,B) Immobile time during tail suspension test for both male (A) and female (B) mice. (C,D) Immobile time during tail suspension test for both male (C) and female (D) mice. (E,F) % of sucrose consumed during sucrose preference test for both male (E) and female (F) mice. Data are presented as mean ± SEM.
The Effects of Hdac4A778T Mutation on Behaviors in Home Cage-Like Environment (LABORAS)
Laboratory Animal Behavior Observation Registration and Analysis System has been used to analyze mouse behaviors in home cage-like environment for extended period of time as we have previously reported (Cui et al., 2015; Lutter et al., 2017). Singly housed male and female mice were allowed to spend overnight (∼24 h) in LABORAS cage and the data were collected and analyzed separately for light and dark phase. Male homozygous mice overall spend significantly less time in eating (by multiple t-test, t(30) = 2.19, p = 0.036, Figure 4A), but not drinking, during both light and dark phases, while they display light cycle-specific reduction in locomotor activity (by multiple t-test, t(30) = 3.898, p = 0.0005, Figure 4A) and rearing behavior (by multiple t-test, t(30) = 3.083, p = 0.004, Figure 4A). No differences were observed in female homozygous mice, except a trend toward increased grooming in light cycle (Figures 4C,D).
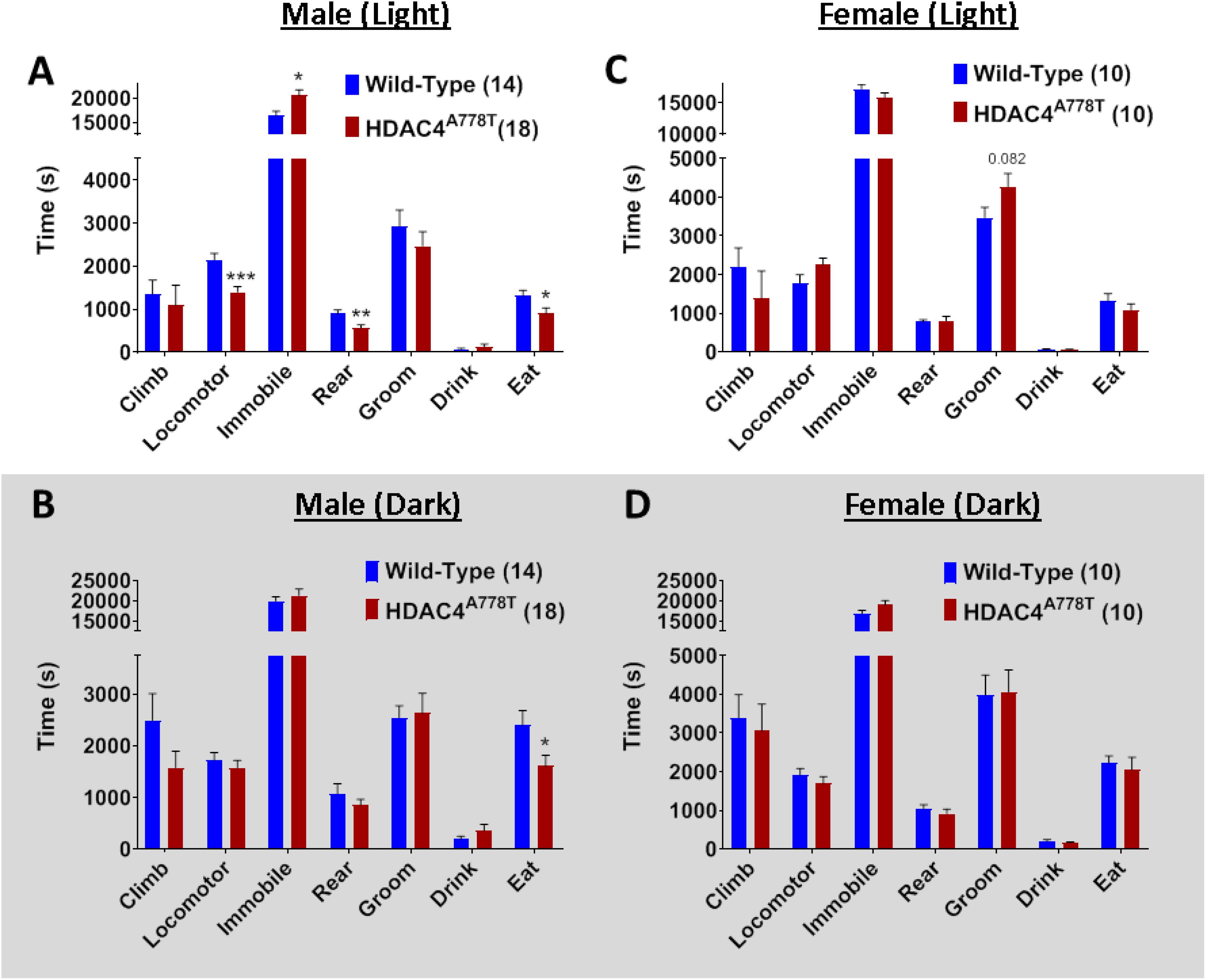
Figure 4. The effects of homozygous Hdac4A778T mutation on general behaviors in home cage-like environment. (A,B) Vibration-based LABORAS behavioral quantification for male mice during light (A) and dark (B) cycles. (C,D) Vibration-based LABORAS behavioral quantification for female mice during light (C) and dark DB) cycles (*p < 0.05, **p < 0.01, ***p < 0.001). Data are presented as mean ± SEM.
The Effects of Hdac4A778T Mutation on Social Behaviors
Impaired social behaviors have been repeatedly reported in patients with EDs. To determine whether Hdac4A778T mutation has an impact on social functions, we subjected the mice to three-chamber sociability and social novelty test as well as social dominance tube test as previously reported (Cui et al., 2015; Lutter et al., 2017). Both male and female WT and mutant mice exhibited no significant difference for both social interaction and social novelty test during 3-chamber sociability test (Figures 5A,B). However, for social dominance tube test, female homozygous mice exhibited a fewer number of wins or a greater number of retreats from the tube (by Student t-test, t(22) = 3.318, p = 0.003, Figure 5C), which was not observed in males (Figure 5D). This result indicates social subordination in female homozygous mice compared to their WT littermates.
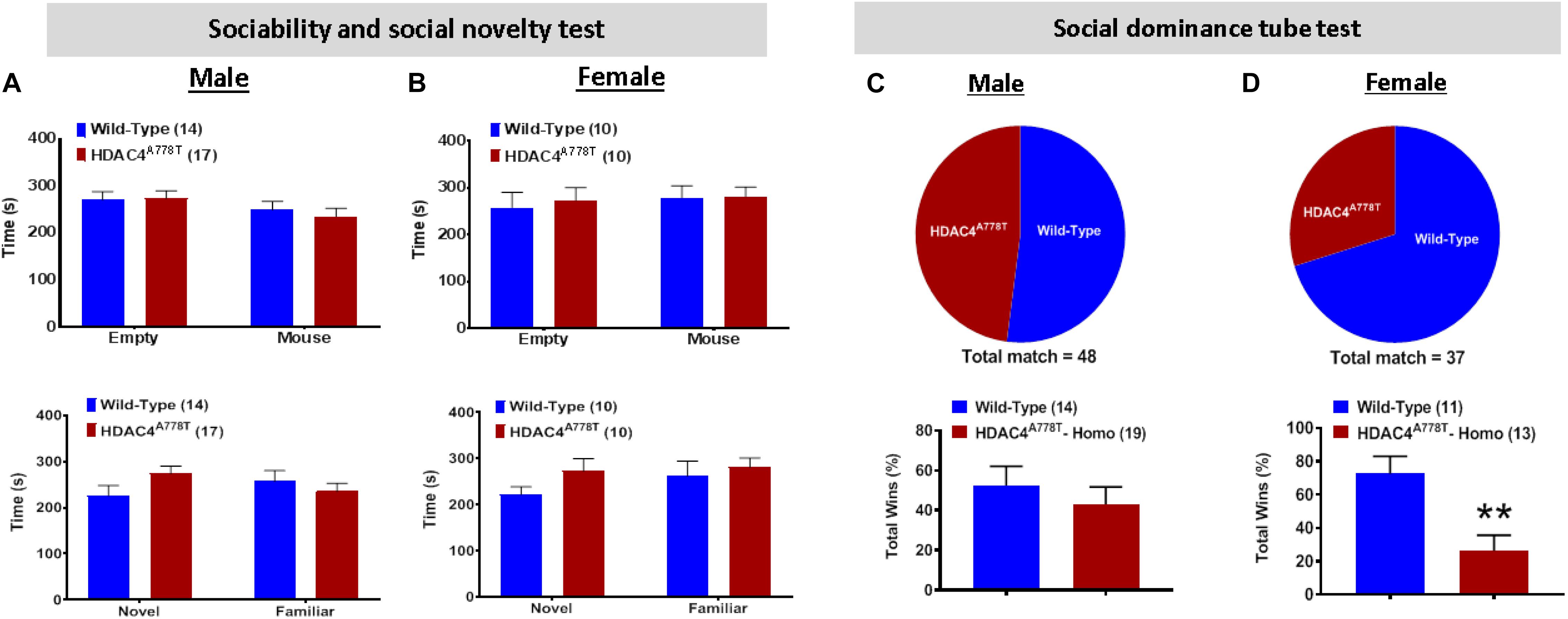
Figure 5. The effects of homozygous Hdac4A778T mutation on socia behaviors. (A,B) Time either spent in empty box or interacted with familiar or novel mice during Immobile time during three-chamber sociability and social novelty test for both male (A) and female (B) mice. (C,D) % of total win during a round-robin tournament of tube dominance test for both male (C) and female (D) mice (**p < 0.01). Data are presented as mean ± SEM.
The Effects of Hdac4A778T Mutation on Food Reward Behavior
Food-associated reward dysfunction is one of core features of patients with EDs (Fladung et al., 2010; Frank et al., 2016; Foldi et al., 2017). To determine whether mice carrying homozygous Hdac4A778T mutation have impaired food-associated reward behavior, we performed effortful operant responding test using a progressive ratio schedule; a well-established behavioral paradigm often used to determine the desire and the motivation for an animal to obtain palatable food. As described in the method section, the mice first had to learn and pass all required training (FR1 × 1, FR3 × 2, and FR5 × 3) before they enter PR schedule for motivation. On average, both male and female homozygous mice took a similar amount of time as WT to pass all required training, indicating that procedural learning ability in homozygous mice is intact (Figures 6A,E). Subsequent measurement for food motivation with PR schedule reveal that male, but not female, homozygous mice tend to less motivated to press lever (by multiple t-test, t(119) = 2.027, p = 0.044, Figure 6B; t(19) = 2.28, p = 0.034, Figure 6D) to obtain high-fat pellets (by multiple t-test, t(19) = 2.188, p = 0.041, Figure 6C) after overnight fasting. No significant differences were observed when mice were fed ad lib (Figures 6B–D, F–H).
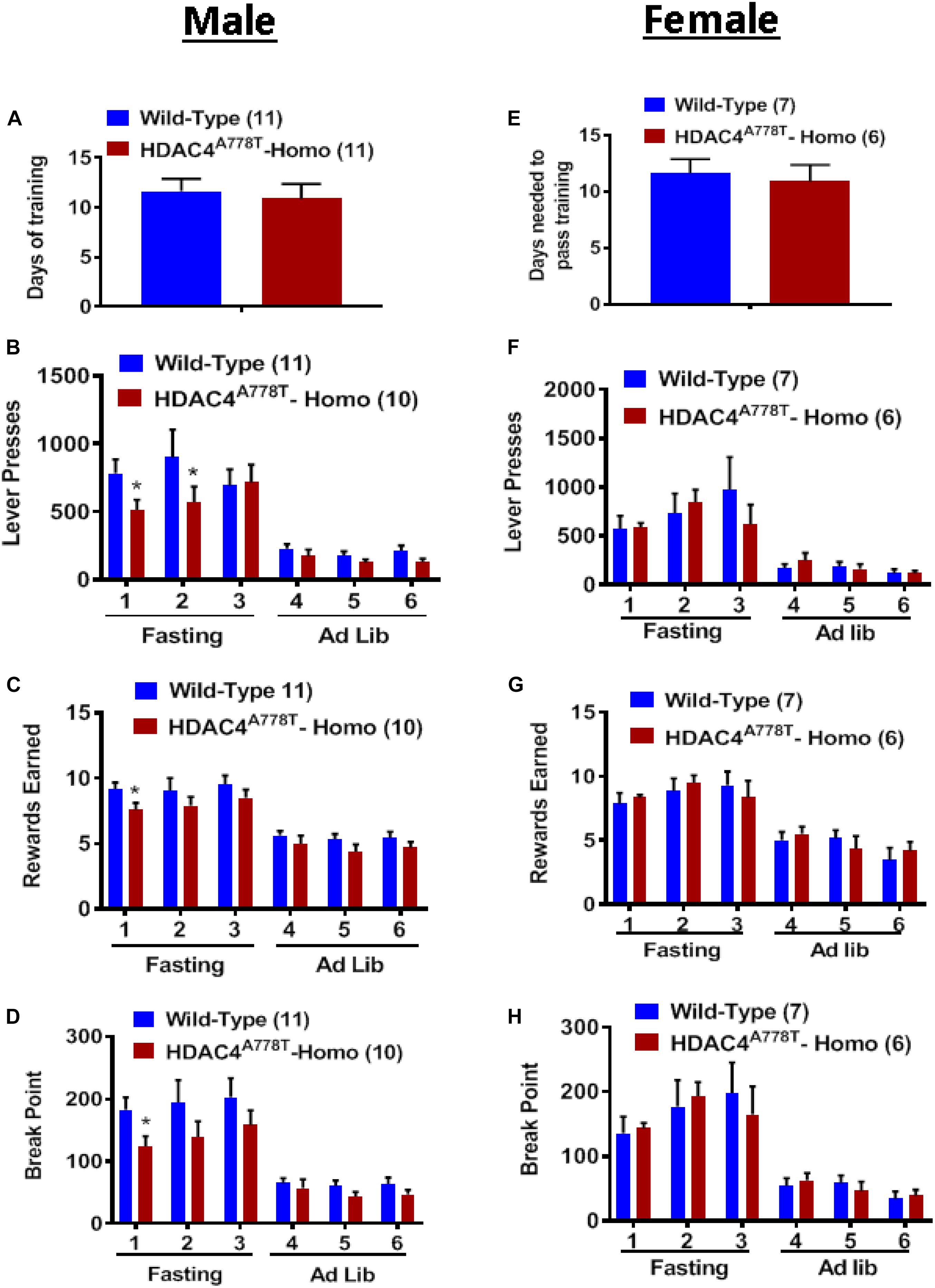
Figure 6. The effect of homozygous Hdac4A778T mutation on food motivation. (A) Days needed to pass all required FR training in male mice. (B–D) Total numbers of lever press (B), total numbers of reward earned (C), and calculated break point (D) during operant responding test with PR schedule for male mice. (E) Days needed to pass all required FR training in female mice. (F–H) Total numbers of lever press (F), total numbers of reward earned (G), and calculated break point HD) during operant responding test with PR schedule for female mice (*p < 0.05). Data are presented as mean ± SEM.
The Effects of Hdac4A778T Mutation on Binge-Like Eating
Binge-like eating behavior assessed by the intake of HFD during first 2.5 h of intermittent access to HFD revealed that female, but not male, homozygous mice tend to have reduced binge-like eating behavior compared to their WT littermates (Figures 7A,C). However, 24-hour intake of HFD during weekly intermittent access reveal that male, but not female, homozygous mice tend to consume less amount of HFD compared to their WT littermates (Figures 7B,D).
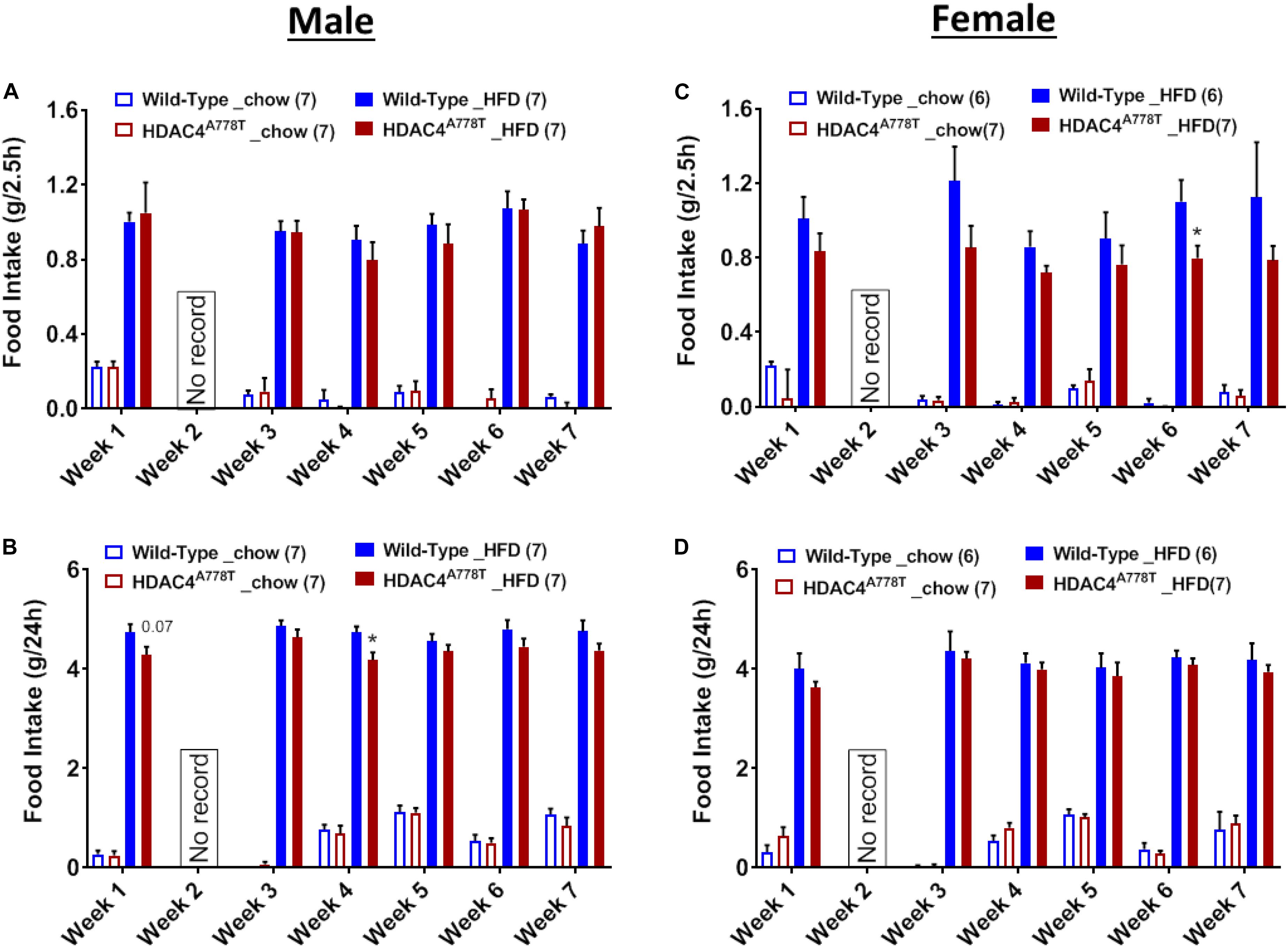
Figure 7. The effects of homozygous Hdac4A778T mutation on binge-like eating. (A,B) 2.5-hour (A) and 24-hour (B) food intake during 7 weeks of binge-like eating test for male mice. (C,D) 2.5-hour (C) and 24-hour (D) food intake during 7 weeks of binge-like eating test for male mice (*p < 0.05). Data are presented as mean ± SEM.
The major metabolic and behavioral differences observed in homozygous mice of current study and heterozygous mice of our previous report are summarized in Table 1.
Discussion
Eating disorders are severe but inadequately treated mental illnesses, in part due to our limited understanding of underlying neurobiology of the illness as well as lack of reliable animal models that can be used for rigorous testing and development of treatment options (Lutter, 2017). As an attempt to establish a more reliable animal model of EDs, in the present study we performed extensive behavioral characterization of homozygous mice for the Hdac4A778T missense mutation, which we previously found to be associated with the risk of developing an ED (Cui et al., 2013). We observed distinct behavioral phenotypes compared to previously reported phenotypes for heterozygous Hdac4A778T mice (Lutter et al., 2017). One notable difference is the gender for which the phenotypes were observed. In contrast to the majority of phenotypes observed in female heterozygous Hdac4A778T mice, in the present study behavioral abnormalities were mainly observed in male homozygous mice (Table 1). While it is puzzling how differences in genotype (homo vs. het) and genetic background (C57BL/6 vs. mixed) affect behavioral phenotypes in different sexes, links between estrogenic signaling and HDAC4 have been well documented. For instance, it has been shown that HDAC4 can be recruited by the N-terminal region of estrogen receptor alpha (ERα) to the promoter of endogenous estrogen responsive genes and suppresses transcriptional activity of ERα in a cell type-dependent manner (Leong et al., 2005); HDAC2 and HDAC4 have been reported to involve in epigenetic histone modifications of ERα, which affect the developmental process of brain masculinization (Matsuda et al., 2011); and more recently, it was shown that HDAC4 is associated with female post-traumatic stress disorder (PTSD) likely through a direct effect of estrogen on HDAC4 expression during traumatic stress (Maddox et al., 2018). Future studies focusing on the bidirectional association between HDAC4 and estrogenic signaling may advance our understanding of neurobiological basis of EDs.
Another factor that may have an impact on the different behavioral phenotypes observed is the “environment” in which all experimental cohorts of mice were produced. It is noteworthy that, compared to WT dams from which all experimental cohort of WT and heterozygous Hdac4A778T mutant mice were produced in our previous report (Lutter et al., 2017), all experimental cohorts of WT and homozygous Hdac4A778T mice in the present study were produced and raised by Hdac4A778T heterozygous dams, which showed notable social deficits in the previous study (Lutter et al., 2017). Therefore, the maternal environments in which the experimental mice grew up, including in utero and pre-weanling postnatal growth, are different between the two studies. While it is known that gene-environmental interactions and epigenetics play an important role for the development of an ED (Campbell et al., 2011; Strober et al., 2014; Steiger and Thaler, 2016; Thaler and Steiger, 2017), it is impossible to distinguish whether the behavioral alterations observed in the present study are due to either genetics or environmentally mediated epigenetic regulations (or could be a combination of both). Future studies with a cross-fostering design may help to evaluate the effect of pre-weanling postnatal growth environment on behavioral manifestations associated with Hdac4A778T mutation.
One interesting behavioral phenotype that we observed for homozygous Hdac4A778T mutant mice, but not for heterozygous mice, is social subordination. Compared to WT littermates, most female, but not male, homozygous mice are submissive in a tube dominance test, which is consistent with absolute social subordination we previously observed in female Esrra-null mice – another mutation that was also found to be associated with EDs (Cui et al., 2013). These observations, along with clinical findings showing that many patients with EDs have social deficits (Troop et al., 2003, 2014; Connan et al., 2007; Troop and Baker, 2008; Veronese et al., 2010), suggest that the trait of social subordination may represent a useful endophenotype of EDs to guide and advance future preclinical studies. Investigating neural circuits and molecular pathways that impact social subordination might therefore open up new avenues for developing a targeted treatment option for EDs.
In summary, we report that homozygous Hdac4A778T mice on a nearly isogenic C57BL/6 background exhibit certain metabolic and behavioral phenotypes relevant to EDs in a sex-dependent manner, including altered feeding and body weight, reduced motivation for palatable food and social subordination, which may be influenced by complicated environmental factors. Since HDAC4 has been implicated in many different brain functions, including learning and memory (Kim et al., 2012; Sando et al., 2012; Fitzsimons et al., 2013; Makinistoglu and Karsenty, 2015; Wu et al., 2016), neurodegeneration (Majdzadeh et al., 2008; Li et al., 2012; Mielcarek et al., 2013, 2015; Whitehouse et al., 2015; Wu et al., 2017; Federspiel et al., 2019), drug addiction (Griffin et al., 2017; Penrod et al., 2018; Gonzalez et al., 2019), PTSD (Maddox et al., 2018; Saha et al., 2019), and major depressive disorder (Hobara et al., 2010; Sarkar et al., 2014; Higuchi et al., 2016), future mechanistic investigations in homozygous Hdac4A778T mice at molecular, cellular and circuit levels will not only provide novel insight into the neurobiological basis of an ED, but also advance our knowledge about the underlying mechanisms of other neuropsychiatric disorders.
Data Availability Statement
All datasets generated for this study are included in the article/Supplementary Material.
Ethics Statement
The animal study was reviewed and approved by University of Iowa Animal Care and Use Committee.
Author Contributions
KD, ML, and HC conceived and designed the experiments and wrote the manuscript. KD, KS, SR, and BT performed the experiments. KD, KS, and HC analyzed the data. HC contributed to reagents, materials, and analysis tools.
Funding
This work was supported by grants from National Institutes of Health (HL127673, MH109920, and HL084207 to HC). The funders had no role in study design, data collection and analysis, decision to publish, or preparation of the manuscript.
Conflict of Interest
The authors declare that the research was conducted in the absence of any commercial or financial relationships that could be construed as a potential conflict of interest.
Acknowledgments
We thank Dr. Andrew A. Pieper and Dr. John A. Wemmie for kindly sharing their laboratory equipment for some behavioral testing.
Supplementary Material
The Supplementary Material for this article can be found online at: https://www.frontiersin.org/articles/10.3389/fnins.2020.00139/full#supplementary-material
References
Baker, J. H., Schaumberg, K., and Munn-Chernoff, M. A. (2017). Genetics of Anorexia Nervosa. Curr. Psychiatry Rep. 19:84. doi: 10.1007/s11920-017-0842-2
Booij, L., Casey, K. F., Antunes, J. M., Szyf, M., Joober, R., Israel, M., et al. (2015). DNA methylation in individuals with anorexia nervosa and in matched normal-eater controls: a genome-wide study. Int. J. Eat. Disord. 48, 874–882. doi: 10.1002/eat.22374
Boraska, V., Franklin, C. S., Floyd, J. A., Thornton, L. M., Huckins, L. M., Southam, L., et al. (2014). A genome-wide association study of anorexia nervosa. Mol. Psychiatry 19, 1085–1094. doi: 10.1038/mp.2013.187
Campbell, I. C., Mill, J., Uher, R., and Schmidt, U. (2011). Eating disorders, gene-environment interactions and epigenetics. Neurosci. Biobehav. Rev. 35, 784–793. doi: 10.1016/j.neubiorev.2010.09.012
Carmichael, O., and Lockhart, S. (2012). The role of diffusion tensor imaging in the study of cognitive aging. Curr. Top. Behav. Neurosci. 11, 289–320. doi: 10.1007/7854_2011_176
Castagne, V., Moser, P., Roux, S., and Porsolt, R. D. (2010). Rodent models of depression: forced swim and tail suspension behavioral despair tests in rats and mice. Curr. Protoc. Pharmacol. Chapter 5:Unit58. doi: 10.1002/0471141755.ph0508s49
Connan, F., Troop, N., Landau, S., Campbell, I. C., and Treasure, J. (2007). Poor social comparison and the tendency to submissive behavior in anorexia nervosa. Int. J. Eat. Disord. 40, 733–739. doi: 10.1002/eat.20427
Cui, H., Lu, Y., Khan, M. Z., Anderson, R. M., McDaniel, L., Wilson, H. E., et al. (2015). Behavioral disturbances in estrogen-related receptor alpha-null mice. Cell Rep. 11, 344–350. doi: 10.1016/j.celrep.2015.03.032
Cui, H., and Lutter, M. (2013). The expression of MC4Rs in D1R neurons regulates food intake and locomotor sensitization to cocaine. Genes Brain Behav. 12, 658–665. doi: 10.1111/gbb.12057
Cui, H., Moore, J., Ashimi, S. S., Mason, B. L., Drawbridge, J. N., Han, S., et al. (2013). Eating disorder predisposition is associated with ESRRA and HDAC4 mutations. J. Clin. Invest. 123, 4706–4713. doi: 10.1172/JCI71400
Czyzyk, T. A., Sahr, A. E., and Statnick, M. A. (2010). A model of binge-like eating behavior in mice that does not require food deprivation or stress. Obesity 18, 1710–1717. doi: 10.1038/oby.2010.46
Duncan, L., Yilmaz, Z., Gaspar, H., Walters, R., Goldstein, J., Anttila, V., et al. (2017). Significant locus and metabolic genetic correlations revealed in genome-wide association study of Anorexia Nervosa. Am. J. Psychiatry 174, 850–858. doi: 10.1176/appi.ajp.2017.16121402
Federspiel, J. D., Greco, T. M., Lum, K. K., and Cristea, I. M. (2019). Hdac4 interactions in Huntington’s Disease viewed through the prism of multiomics. Mol. Cell Proteomics 18(8 Suppl. 1), S92–S113. doi: 10.1074/mcp.RA118.001253
Fitzsimons, H. L., Schwartz, S., Given, F. M., and Scott, M. J. (2013). The histone deacetylase HDAC4 regulates long-term memory in Drosophila. PLoS One 8:e83903. doi: 10.1371/journal.pone.0083903
Fladung, A. K., Gron, G., Grammer, K., Herrnberger, B., Schilly, E., Grasteit, S., et al. (2010). A neural signature of anorexia nervosa in the ventral striatal reward system. Am. J. Psychiatry 167, 206–212. doi: 10.1176/appi.ajp.2009.09010071
Foldi, C. J., Milton, L. K., and Oldfield, B. J. (2017). A focus on reward in anorexia nervosa through the lens of the activity-based anorexia rodent model. J. Neuroendocrinol. 29:e12479. doi: 10.1111/jne.12479
Frank, G. K., Shott, M. E., Riederer, J., and Pryor, T. L. (2016). Altered structural and effective connectivity in anorexia and bulimia nervosa in circuits that regulate energy and reward homeostasis. Transl. Psychiatry 6, e932. doi: 10.1038/tp.2016.199
Gonzalez, B., Bernardi, A., Torres, O. V., Jayanthi, S., Gomez, N., Sosa, M. H., et al. (2019). HDAC superfamily promoters acetylation is differentially regulated by modafinil and methamphetamine in the mouse medial prefrontal cortex. Addict. Biol. doi: 10.1111/adb.12737 [Epub ahead of print],
Griffin, E. A. Jr., Melas, P. A., Zhou, R., Li, Y., Mercado, P., Kempadoo, K. A., et al. (2017). Prior alcohol use enhances vulnerability to compulsive cocaine self-administration by promoting degradation of HDAC4 and HDAC5. Sci. Adv. 3:e1701682. doi: 10.1126/sciadv.1701682
Higuchi, F., Uchida, S., Yamagata, H., Abe-Higuchi, N., Hobara, T., Hara, K., et al. (2016). Hippocampal MicroRNA-124 enhances chronic stress resilience in mice. J. Neurosci. 36, 7253–7267. doi: 10.1523/JNEUROSCI.0319-16.2016
Hinney, A., Scherag, S., and Hebebrand, J. (2010). Genetic findings in anorexia and bulimia nervosa. Prog. Mol. Biol. Transl. Sci. 94, 241–270. doi: 10.1016/S1877-1173(10)94009-X
Hobara, T., Uchida, S., Otsuki, K., Matsubara, T., Funato, H., Matsuo, K., et al. (2010). Altered gene expression of histone deacetylases in mood disorder patients. J. Psychiatr. Res. 44, 263–270. doi: 10.1016/j.jpsychires.2009.08.015
Huckins, L. M., Hatzikotoulas, K., Southam, L., Thornton, L. M., Steinberg, J., Aguilera-McKay, F., et al. (2018). Investigation of common, low-frequency and rare genome-wide variation in anorexia nervosa. Mol. Psychiatry 23, 1169–1180. doi: 10.1038/mp.2017.88
Karwautz, A., Haidvogl, M., Wagner, G., Nobis, G., Wober-Bingol, C., and Friedrich, M. H. (2002). [Subjective family image in anorexia nervosa and bulimia nervosa in adolescence: a controlled study]. Z Kinder Jugendpsychiatr. Psychother. 30, 251–259. doi: 10.1024/1422-4917.30.4.251
Kesselmeier, M., Putter, C., Volckmar, A. L., Baurecht, H., Grallert, H., Illig, T., et al. (2018). High-throughput DNA methylation analysis in anorexia nervosa confirms TNXB hypermethylation. World J. Biol. Psychiatry 19, 187–199. doi: 10.1080/15622975.2016.1190033
Kim, M. S., Akhtar, M. W., Adachi, M., Mahgoub, M., Bassel-Duby, R., Kavalali, E. T., et al. (2012). An essential role for histone deacetylase 4 in synaptic plasticity and memory formation. J. Neurosci. 32, 10879–10886. doi: 10.1523/JNEUROSCI.2089-12.2012
Leong, H., Sloan, J. R., Nash, P. D., and Greene, G. L. (2005). Recruitment of histone deacetylase 4 to the N-terminal region of estrogen receptor alpha. Mol. Endocrinol. 19, 2930–2942. doi: 10.1210/me.2005-0178
Li, J., Chen, J., Ricupero, C. L., Hart, R. P., Schwartz, M. S., Kusnecov, A., et al. (2012). Nuclear accumulation of HDAC4 in ATM deficiency promotes neurodegeneration in ataxia telangiectasia. Nat. Med. 18, 783–790. doi: 10.1038/nm.2709
Lilenfeld, L. R., Kaye, W. H., Greeno, C. G., Merikangas, K. R., Plotnicov, K., Pollice, C., et al. (1998). A controlled family study of anorexia nervosa and bulimia nervosa: psychiatric disorders in first-degree relatives and effects of proband comorbidity. Arch. Gen. Psychiatry 55, 603–610.
Lutter, M. (2017). Emerging treatments in eating disorders. Neurotherapeutics 14, 614–622. doi: 10.1007/s13311-017-0535-x
Lutter, M., Croghan, A. E., and Cui, H. (2016). Escaping the golden cage: animal models of eating disorders in the post-diagnostic and statistical manual era. Biol. Psychiatry 79, 17–24. doi: 10.1016/j.biopsych.2015.02.006
Lutter, M., Khan, M. Z., Satio, K., Davis, K. C., Kidder, I. J., McDaniel, L., et al. (2017). The eating-disorder associated HDAC4(A778T) mutation alters feeding behaviors in female mice. Biol. Psychiatry 81, 770–777. doi: 10.1016/j.biopsych.2016.09.024
Maddox, S. A., Kilaru, V., Shin, J., Jovanovic, T., Almli, L. M., Dias, B. G., et al. (2018). Estrogen-dependent association of HDAC4 with fear in female mice and women with PTSD. Mol. Psychiatry 23, 658–665. doi: 10.1038/mp.2016.250
Majdzadeh, N., Wang, L., Morrison, B. E., Bassel-Duby, R., Olson, E. N., and D’Mello, S. R. (2008). HDAC4 inhibits cell-cycle progression and protects neurons from cell death. Dev. Neurobiol. 68, 1076–1092. doi: 10.1002/dneu.20637
Makinistoglu, M. P., and Karsenty, G. (2015). The class II histone deacetylase HDAC4 regulates cognitive, metabolic and endocrine functions through its expression in osteoblasts. Mol. Metab. 4, 64–69. doi: 10.1016/j.molmet.2014.10.004
Matsuda, K. I., Mori, H., Nugent, B. M., Pfaff, D. W., McCarthy, M. M., and Kawata, M. (2011). Histone deacetylation during brain development is essential for permanent masculinization of sexual behavior. Endocrinology 152, 2760–2767. doi: 10.1210/en.2011-0193
Mielcarek, M., Landles, C., Weiss, A., Bradaia, A., Seredenina, T., Inuabasi, L., et al. (2013). HDAC4 reduction: a novel therapeutic strategy to target cytoplasmic huntingtin and ameliorate neurodegeneration. PLoS Biol. 11:e1001717. doi: 10.1371/journal.pbio.1001717
Mielcarek, M., Zielonka, D., Carnemolla, A., Marcinkowski, J. T., and Guidez, F. (2015). HDAC4 as a potential therapeutic target in neurodegenerative diseases: a summary of recent achievements. Front. Cell Neurosci. 9:42. doi: 10.3389/fncel.2015.00042
Penrod, R. D., Carreira, M. B., Taniguchi, M., Kumar, J., Maddox, S. A., and Cowan, C. W. (2018). Novel role and regulation of HDAC4 in cocaine-related behaviors. Addict. Biol. 23, 653–664. doi: 10.1111/adb.12522
Saha, P., Gupta, R. K., Sen, T., and Sen, N. (2019). Histone deacetylase 4 downregulation elicits post-traumatic psychiatric disorders through impairment of neurogenesis. J. Neurotrauma doi: 10.1089/neu.2019.6373 [Epub ahead of print],
Sando, R. III, Gounko, N., Pieraut, S., Liao, L., Yates, J. III, and Maximov, A. (2012). HDAC4 governs a transcriptional program essential for synaptic plasticity and memory. Cell 151, 821–834. doi: 10.1016/j.cell.2012.09.037
Sarkar, A., Chachra, P., Kennedy, P., Pena, C. J., Desouza, L. A., Nestler, E. J., et al. (2014). Hippocampal HDAC4 contributes to postnatal fluoxetine-evoked depression-like behavior. Neuropsychopharmacology 39, 2221–2232. doi: 10.1038/npp.2014.73
Sild, M., and Booij, L. (2019). Histone deacetylase 4 (HDAC4): a new player in anorexia nervosa? Mol. Psychiatry 24, 1425–1434. doi: 10.1038/s41380-019-0366-8
Steiger, H., Booij, L. K., McGregor, K., Thaler, L., Fletcher, E., Rossi, E., et al. (2019). A longitudinal, epigenome-wide study of DNA methylation in anorexia nervosa: results in actively ill, partially weight-restored, long-term remitted and non-eating-disordered women. J. Psychiatry Neurosci. 44, 205–213. doi: 10.1503/jpn.170242
Steiger, H., and Thaler, L. (2016). Eating disorders, gene-environment interactions and the epigenome: roles of stress exposures and nutritional status. Physiol. Behav. 162, 181–185. doi: 10.1016/j.physbeh.2016.01.041
Strober, M., Freeman, R., Lampert, C., Diamond, J., and Kaye, W. (2000). Controlled family study of anorexia nervosa and bulimia nervosa: evidence of shared liability and transmission of partial syndromes. Am. J. Psychiatry 157, 393–401. doi: 10.1176/appi.ajp.157.3.393
Strober, M., Peris, T., and Steiger, H. (2014). The plasticity of development: how knowledge of epigenetics may advance understanding of eating disorders. Int. J. Eat. Disord. 47, 696–704. doi: 10.1002/eat.22322
Subramanian, S., Braun, P. R., Han, S., and Potash, J. B. (2018). Investigation of differential HDAC4 methylation patterns in eating disorders. Psychiatr. Genet. 28, 12–15. doi: 10.1097/YPG.0000000000000189
Thaler, L., and Steiger, H. (2017). Eating disorders and epigenetics. Adv. Exp. Med. Biol. 978, 93–103. doi: 10.1007/978-3-319-53889-1_5
Troop, N. A., Allan, S., Treasure, J. L., and Katzman, M. (2003). Social comparison and submissive behaviour in eating disorder patients. Psychol. Psychother. 76(Pt 3), 237–249. doi: 10.1348/147608303322362479
Troop, N. A., Andrews, L., Hiskey, S., and Treasure, J. L. (2014). Social rank and symptom change in eating disorders: a 6-month longitudinal study. Clin. Psychol. Psychother. 21, 115–122. doi: 10.1002/cpp.1830
Troop, N. A., and Baker, A. H. (2008). The specificity of social rank in eating disorder versus depressive symptoms. Eat. Disord. 16, 331–341. doi: 10.1080/10640260802115993
Veronese, A., Zanon, S., Zanetti, T., Santonastaso, P., and Favaro, A. (2010). [Perception of social rank and submissive behavior in eating disorders]. Riv. Psichiatr. 45, 41–48.
Wang, K., Zhang, H., Bloss, C. S., Duvvuri, V., Kaye, W., Schork, N. J., et al. (2011). A genome-wide association study on common SNPs and rare CNVs in anorexia nervosa. Mol. Psychiatry 16, 949–959. doi: 10.1038/mp.2010.107
Watson, H. J., Yilmaz, Z., Thornton, L. M., Hubel, C., Coleman, J. R. I., Gaspar, H. A., et al. (2019). Genome-wide association study identifies eight risk loci and implicates metabo-psychiatric origins for anorexia nervosa. Nat. Genet. 51, 1207–1214. doi: 10.1038/s41588-019-0439-2
Whitehouse, A., Doherty, K., Yeh, H. H., Robinson, A. C., Rollinson, S., Pickering-Brown, S., et al. (2015). Histone deacetylases (HDACs) in frontotemporal lobar degeneration. Neuropathol. Appl. Neurobiol. 41, 245–257. doi: 10.1111/nan.12153
Wu, K. L., Wu, C. W., Tain, Y. L., Huang, L. T., Chao, Y. M., Hung, C. Y., et al. (2016). Environmental stimulation rescues maternal high fructose intake-impaired learning and memory in female offspring: its correlation with redistribution of histone deacetylase 4. Neurobiol. Learn. Mem. 130, 105–117. doi: 10.1016/j.nlm.2016.02.004
Keywords: eating disorders, histone deacetylase 4, mouse behaviors, social subordination, body weight, food intake, genetic mutation, humanized mouse model
Citation: Davis KC, Saito K, Rodeghiero SR, Toth BA, Lutter M and Cui H (2020) Behavioral Alterations in Mice Carrying Homozygous HDAC4A778T Missense Mutation Associated With Eating Disorder. Front. Neurosci. 14:139. doi: 10.3389/fnins.2020.00139
Received: 10 December 2019; Accepted: 04 February 2020;
Published: 21 February 2020.
Edited by:
Julia Maria Schulze-Hentrich, Tübingen University Hospital, GermanyReviewed by:
Camron D. Bryant, Boston University School of Medicine, United StatesMichael John Gambello, Emory University, United States
Copyright © 2020 Davis, Saito, Rodeghiero, Toth, Lutter and Cui. This is an open-access article distributed under the terms of the Creative Commons Attribution License (CC BY). The use, distribution or reproduction in other forums is permitted, provided the original author(s) and the copyright owner(s) are credited and that the original publication in this journal is cited, in accordance with accepted academic practice. No use, distribution or reproduction is permitted which does not comply with these terms.
*Correspondence: Huxing Cui, huxing-cui@uiowa.edu