Neurobiology of Loneliness, Isolation, and Loss: Integrating Human and Animal Perspectives
- Department of Pharmacology and Toxicology, School of Pharmacy, University of Kansas, Lawrence, KS, United States
In social species such as humans, non-human primates, and even many rodent species, social interaction and the maintenance of social bonds are necessary for mental and physical health and wellbeing. In humans, perceived isolation, or loneliness, is not only characterized by physical isolation from peers or loved ones, but also involves negative perceptions about social interactions and connectedness that reinforce the feelings of isolation and anxiety. As a complex behavioral state, it is no surprise that loneliness and isolation are associated with dysfunction within the ventral striatum and the limbic system – brain regions that regulate motivation and stress responsiveness, respectively. Accompanying these neural changes are physiological symptoms such as increased plasma and urinary cortisol levels and an increase in stress responsivity. Although studies using animal models are not perfectly analogous to the uniquely human state of loneliness, studies on the effects of social isolation in animals have observed similar physiological symptoms such as increased corticosterone, the rodent analog to human cortisol, and also display altered motivation, increased stress responsiveness, and dysregulation of the mesocortical dopamine and limbic systems. This review will discuss behavioral and neuropsychological components of loneliness in humans, social isolation in rodent models, and the neurochemical regulators of these behavioral phenotypes with a neuroanatomical focus on the corticostriatal and limbic systems. We will also discuss social loss as a unique form of social isolation, and the consequences of bond disruption on stress-related behavior and neurophysiology.
Introduction
Social relationships are critical for health and wellbeing of the individual and for survival of social species. Thus, social behaviors are valued and motivating, while social isolation and ostracization are aversive and stressful. While different species exhibit a variety of behaviors that facilitate social bonding and maintain social relationships, many of the neural mechanisms that govern the display of these behaviors are conserved across species. For example, neuropeptides such as oxytocin (OT) and vasopressin, and neurotransmitters such as dopamine (DA) and endorphins, are critically involved in promoting affiliative and social-seeking behaviors. In addition, stress-related neuropeptides such as glucocorticoids and corticotrophin releasing hormone (CRH) are increased during social isolation and social ostracization, leading to anxiety- and depressive-like behaviors and altering sociality in various ways. This review will synthesize what we currently know about the neurobiological foundations of loneliness in humans and the use of animal models of social isolation to provide a more mechanistic approach to study how the brain adapts to being alone. Additionally, we will discuss social loss as a unique form of loneliness and isolation in both humans and animal models and how the neurochemical underpinnings of loss overlap with and diverge from those that influence loneliness in the absence of loss.
Social Connectedness Is Rewarding and Stress Buffering in Social Species
From an ecological standpoint, species that have evolved to rely on sociality and community for survival should certainly have neurobiological mechanisms in place that drive individual members of the species to seek out and form relationships with others. In such species, social groups provide greater protection from predators (i.e., shared vigilance, mob defense), enhances success in locating and maintaining access to resources including food and mates, and increases survival of offspring by increasing the number of caregivers and protectors (Lee, 1994; Burkart and van Schaik, 2016; van Schaik, 2016). Socialization in these species begins early in development, and the social environment (and thus, the definition of what it means to be appropriately social at that time point) change throughout the lifespan (Box 1). Social interaction is driven neurobiologically by activation of reward circuitry involving the neurotransmitter DA being synthesized from the midbrain ventral tegmental area (VTA) and released into the ventral striatum (i.e., nucleus accumbens, NAc) and higher-order cortical regions (i.e., prefrontal cortex, PFC; insular cortex, IC; anterior cingulate cortex, ACC). Through this mechanism and via coordination with the neuropeptides OT and vasopressin that are released during social contact and intimacy, forming and maintaining social relationships is gratifying and valuable. Many social mammals show affiliation and affection through the physical touch of social grooming (Carter et al., 1999). In non-human mammals, social grooming causes a release of endogenous opioids (i.e., endorphins; Keverne et al., 1989), neurochemicals that promote reward and reduce pain sensitivity. This form of tactile stimulation is encoded by mechanoreceptive C-tactile (CT) fibers at the base of most hair follicles in many species (rodents, Leem et al., 1993; primates, Kumazawa and Perl, 1977; humans, Vallbo et al., 1999; Löken et al., 2009; Olausson et al., 2010). These axons are unmyelinated, respond specifically to slow and gentle stroking (∼2.5 cm per second), and directly trigger endorphin release (Nummenmaa et al., 2016). As humans no longer have fur to socially groom, these receptors respond instead to stroking, caressing, touching, and hugging which we use as a means of expressing and strengthening intimate relationships (Dunbar, 2010; Löken and Olausson, 2010; Suvilehto et al., 2015). Even non-tactile behaviors such as laughing (Caruana, 2017; Manninen et al., 2017), singing (Pearce et al., 2015), dancing (Tarr et al., 2015, 2016), and emotional story telling (Dunbar et al., 2016) in social contexts can facilitate the release of endogenous opioids. Thus, showing and receiving affection and intimacy activates neurobiological processes that reward and promote continued display of such behaviors.
BOX 1. Social development and loneliness across the lifespan.
Social mammals experience a wide variety of social environments throughout their lifespan and navigate these social spaces with the skills they currently have at that developmental period. During infancy, the brain undergoes extensive plasticity including the making and breaking of connections via the growth of dendritic arborizations, synaptogenesis, and myelination to strengthen existing connections (Gao et al., 2009; Tau and Peterson, 2010). During this time, the social environment, which mainly consists of caregivers and siblings, can have drastic impacts on the development of early social behavior. For instance, rodent studies have shown that the amount and quality of maternal care influences adult maternal behavior, sexual behavior, and peer interactions (Bosch and Neumann, 2012). Additionally, when parental behavior is stable and predictable, infants (humans, non-human primates, rats) develop optimally (Johnson et al., 1996; Davis et al., 2017). Furthermore, developmental settings that are associated with unstable and unpredictable caregiving, such as postpartum psychopathology, loss of a caregiver, and orphanhood, interfere with social development. In support, infants of mothers with postpartum depression show disrupted attachment to their mother (McMahon et al., 2006; Lanzi et al., 2009) and lower social engagement (Feldman et al., 2009). Loss of one or more parental caregivers disrupts later social behavior and bonding in prairie voles (Rogers and Bales, 2020; Valera-Marín et al., 2021) and in non-human primates (Harlow and Suomi, 1971; Winslow et al., 2003), and it is estimated that between one half and two thirds of children entering the foster care system demonstrate emotional or behavioral problems (Chernoff et al., 1994). Parental insensitivity also predicts higher sympathetic nervous system activity in adult children during conflict discussions with their romantic partner (Raby et al., 2015), indicating that childhood social environment can have long-lasting impacts on future social behavior. As an extension, the parent–infant dyad helps infants learn the behavioral repertoire of sociality, including bonding, cooperation, and competition to promote a long-term framework for social affiliation. The social environment expands drastically once infants reach childhood and begin interacting with others of their age. Lack of high-quality peer relationships are also one of the first sources of loneliness, especially as children transition from forming and maintaining relationships based on proximity and shared interest to desiring relationships that have more positive qualities. Forming high-quality friendships with peers can positively impact social confidence and success navigating the social world (see Collins and Laursen, 1999; Hartup and Stevens, 1999); however, friendships with negative qualities, such as dominance and rivalry, can negatively impact children’s social skills, increase disruptive and disagreeable behaviors, and increase feelings of loneliness (Keefe and Berndt, 1996; Ladd et al., 1996). Exclusion from peer groups in general is also linked to loneliness in kindergarteners (Kochenderfer-Ladd and Wardrop, 2001). Adolescence is characterized by a further shift in prioritizing quality within social relationships, a desire to be liked by the entire peer group, and a new focus on intimacy within social relationships. Indeed, lack of friendship, low friendship quality, and peer rejection are all predictors of loneliness in adolescence (Vanhalst et al., 2015). Interestingly, stability of loneliness across childhood and adolescence is variable, with some individuals remaining high from age 7 to 17, some starting low and increasing over time, some starting high and decreasing over time, and some with low, stable levels of loneliness (Qualter et al., 2013). Additionally, negative reactivity, low levels of social engagement, and low social preference increased the risk of following the high loneliness trajectory. Romantic relationships also emerge as a new source of loneliness during this time (Collins et al., 2009) and quality of romantic relationships has a lasting impact on loneliness. For instance, relational disappointment is associated with higher levels of loneliness in college students (Flora and Segrin, 2000), and marriage quality explains individual differences in loneliness in adulthood (Hawkley et al., 2008). In the socially monogamous prairie vole, being isolated from a pair-bonded partner also increases anxiety-like behavior, but this separation does not affect prairie voles that did not have a strong bond with their mate (Osako et al., 2018). At older ages, the social environment begins to decrease in size and quality, resulting in increased risk for feelings of loneliness. Such social challenges that older adults face include losing a partner, reduced engagement in social activities due to limited mobility or poor health, and being confronted with their own or their partner’s increasing frailty (Dykstra et al., 2005). Additionally, the length of time spent in a relationship and age at which a partner is lost are both positively correlated with stress-related hormone release during isolation in prairie voles (Grippo et al., 2021). Thus, aging is related to increased social experience, and therefore, increased susceptibility to negative outcomes when either the relationship itself, or one’s ability to actively participate in such relationships are lost.
Being close to other individuals can also be stress buffering and protective against the consequences of hardship and adversity. Emotionally, social support is related to more positive moods (Williamson et al., 2019), better mood regulation (Tabatabaei et al., 2018), and lower psychological distress (Lepore et al., 1991; Lee C. Y. S. et al., 2018). Positive social experiences shape our self-perceptions, and thus have a great impact on how we view ourselves, our merit, and our ability to overcome pain or failure. Central to this idea is self-compassion, which has three main components: (1) self-kindness; (2) common humanity, or the view of oneself as part of a broader human experience; and (3) mindfulness of one’s own thoughts and feelings (Neff, 2003). Because our own self-esteem and self-view depends on our perceived feelings of social value and inclusion (Leary and Baumeister, 2000), social support and positive social interactions encourage a more balanced, self-forgiving perspective that may facilitate better emotional well-being. In support, people with high perceived social support have more positive and fewer negative self-perceptions and beliefs about others’ views of themselves (Sarason et al., 1991). Social support is also positively associated with mindfulness, self-compassion, and savoring (the use of thoughts and actions to increase appreciation of positive experiences and emotions), and these three factors significantly mediate the relationship between perceived social support and increased psychological well-being (Wilson et al., 2020). By promoting a more positive view of our ability to navigate hardship, social support may also affect stress-responsivity when experiencing distress. Indeed, social support suppresses cortisol release in humans given a social stress test or experiencing stressful events (Heinrichs et al., 2003; Raymond and Sheppard, 2017), predicts lower cardiovascular reactivity to stress (Howard et al., 2017), and increases pain tolerance (Roberts et al., 2015). Studies in non-human primates also support the stress-buffering role of social affiliation. Introducing a number of aversive stimuli that would typically increase stress responsivity does not do so when animals are presented the stimuli in social groups (Coe et al., 1982; Stanton et al., 1985; Winslow et al., 2003). Stress-buffering can even occur in the absence of physical contact, as exposure to vocalizations of a pair-mate was sufficient to blunt the increase in urinary cortisol of isolated male marmosets (Rukstalis and French, 2005). Studies in rodents have also shown a protective effect of social connectedness against the development of adverse behavioral and physiological consequences. For instance, socially housed rats born to a lineage of stressed mothers (multigenerational ancestral stress) exhibited blunted HPA axis response to chronic stress compared to those that were isolated from peers during stress (Faraji et al., 2017). In the socially monogamous prairie vole (Microtus ochrogaster), stress responsiveness is similarly buffered when an animal experiences a stressor in the presence of his or her pair-bonded partner (Smith and Wang, 2014; McNeal et al., 2017; Donovan et al., 2018). Taken together, research from human and animal studies highlight the cognitive, behavioral, and physiological benefits of social affiliation, which implies that removal of such support could have negative repercussions for individual health and well-being.
Loneliness in Humans Is Associated With Altered Social Behavior
While social affiliation is rewarding and valued, social isolation and ostracization are stressful and aversive. Thus, individuals that perceive being socially isolated, regardless of actual social connectedness, experience feelings of loneliness that are severely distressing. Because loneliness is characterized by the perceived state of isolation, it is unsurprising that lonely individuals have more negative perceptions of everyday interpersonal interactions (Hawkley et al., 2007). This tendency to view social interactions through a negative lens may be driven by previous experience with social relationships. For example, poor-quality social relationships (Mullins and Dugan, 1990; Hawkley et al., 2008) and conflict in current marital or family relationships (Jones D. C., 1992; Burke et al., 2012) are risk factors that predict feelings of loneliness and isolation (Hawkley et al., 2008). As a result, loneliness is also related to stronger expectations of, and motivation to avoid, negative social outcomes and weaker expectations of positive social outcomes (Gable, 2006) as well as increased comorbidity with symptoms of social anxiety (Lieberz et al., 2021a). Because of this fear of social rejection, loneliness is also associated with increased hypervigilance to social threats and, therefore, lonely individuals are more likely to be less trusting and more hostile in uncomfortable social situations (Duck et al., 1994; Anderson and Martin, 1995; Cacioppo and Patrick, 2008; Lieberz et al., 2021b). Despite the tendency to anticipate social rejection, lonely individuals still desire social interaction, behave in ways to promote social connectedness (DeWall and Richman, 2011), and show increased sensitivity to social information (Pickett et al., 2004; Gardner et al., 2005). However, this heightened sensitivity during social interactions, driven by the need to feel socially connected and biased by experience with “failed” relationships, may ultimately prime the expectation of negative outcomes once attempts at social connection are initiated, leading to reduced fulfillment and positive affect following positive social interactions (Lieberz et al., 2021b). Furthermore, this series of events produces anxiety surrounding the social environment, resulting in physiological and health-related consequences that will be summarized in the following section.
Loneliness in Humans Is Associated With Anxiety and Altered Stress Responsivity
The experience of loneliness is extremely distressing, leading to changes in stress physiology and reactivity in lonely individuals. Stress reactivity is governed by the body’s hypothalamus-pituitary-adrenal (HPA) axis, where stress-related neuropeptides are released from the hypothalamus, causing excretion of “releasing factors” from the pituitary to signal glucocorticoid (cortisol in humans and non-human primates, corticosterone in rodents) release from the adrenal glands. This process is regulated by a negative feedback loop – cortisol released by the adrenal gland will elicit a behavioral response (in concert with the autonomic fight or flight) toward environmental stimuli, but it will also bind to receptors in the brain that turn off the HPA axis which dampens continued cortisol release. Disruptions in both the basal functioning of the HPA axis as well as its ability to appropriately activate and deactivate under stressful conditions have been shown to underlie a variety of psychopathologies (reviewed in Buitelaar, 2013), and similar disruptions can be found in lonely individuals. For instance, lonely people have higher salivary (Steptoe et al., 2004; Doane and Adam, 2010), plasma (Pressman et al., 2005), and excretory (Kiecolt-Glaser et al., 1984) cortisol levels. Trait loneliness is also associated with altered diurnal pattern of cortisol release characterized by lower levels in the morning and afternoon and much higher levels in the evening (Cacioppo et al., 2000). Interestingly, lonely students in this study were just as likely to engage in restorative behaviors (sleep, exercise, spending time with others) as non-lonely students; yet these activities did not seem to blunt stress reactivity as it did in the socially embedded individuals. Basal heart rate and heart rate reactivity after reciting both a social and a non-social speech was also lower in lonely individuals compared to non-lonely individuals, and this suggests blunted autonomic nervous system functioning (Cacioppo et al., 2000). Research in socially deprived chimpanzees has also shown increased HPA and stress reactivity, and resocialization caused a reversal of isolation/deprivation-induced increase in glucocorticoid secretion (Reimers et al., 2007).
Loneliness in Humans Is Associated With Altered Brain Activation
Loneliness can increase sensitivity to social disconnection, impacting the emotional response to social isolation and the expectations about others. Thus, the perception of isolation may reflect functional changes to brain regions involved in processing social information, regulating and expressing emotion, and assigning reward value to social interactions. In support, the reward-related ventral striatum showed reduced activation in response to viewing images of unfamiliar people in lonely compared to non-lonely individuals (Cacioppo et al., 2009). Furthermore, Inagaki et al. (2016a) found that lonely people had higher activity in the ventral striatum when viewing pictures of familiar people compared to strangers. Thus, it is possible that, due to increased negative expectations and higher anxiety of social outcomes, lonely individuals have a bias against novel social encounters and toward interactions with people they know will respond positively to their attempts for social connection. Moreover, the insula and anterior cingulate cortex are typically activated during presentation of social-related words (i.e. fight, glory) compared to individual words (i.e. talent, pain; Arioli et al., 2021). However, activity in both regions is dampened in lonely individuals during resting state (Morr et al., 2021) while activity in just the insula is blunted in response to viewing pleasant non-social stimuli (Cacioppo et al., 2009) and during participation in a social trust task (Lieberz et al., 2021b). Gray and white matter volume is also lower in the insula and PFC of individuals scoring high on loneliness, which could indicate reduced myelination and/or synapse number that would reduce signal efficacy (Nakagawa et al., 2015; Düzel et al., 2019). While activity is dampened in lonely individuals at rest and during a variety of positive social and non-social stimuli, the insula, ACC, PFC, and ventral striatum are more active in non-lonely individuals that reported feeling excluded during a virtual game (Eisenberger et al., 2003, 2007), and this activity pattern in response to exclusion predicts loneliness scores (Oh, 2020). Thus, it could be that these regions are sensitive to particular information in lonely individuals, i.e., less activation during pleasant stimuli but more activation during aversive or unpleasant stimuli. Furthermore, activation of visual attention regions following presentation of negative social words was greater for lonely compared to non-lonely individuals (Cacioppo S. et al., 2015), suggesting increased sensitivity to negative social stimuli. Altered emotion perception may also be a risk factor for feeling lonely and isolated, as alexithymia (a personality trait characterized by impaired emotional awareness and interpersonal relating) modulates the correlation between insular activity and loneliness (Morr et al., 2021). The insular cortex also integrates sensory input and links these cues with emotional state of both the self and others (Davidson and Irwin, 1999; Ebisch et al., 2011; Zaki et al., 2012; Gu et al., 2013) and is a critical hub for coordinating network switching (Sridharan et al., 2008). Because attention to social cues is heightened in lonely individuals, loneliness may be characterized by disrupted ability to process and relate such social cues to emotional state of social conspecifics, leading to a feeling of unfulfillment after attempts to socially connect (Box 2).
BOX 2. The insular cortex as a neural hub for processing social encounters.
Recent reviews have postulated a role for the insular cortex in maintaining internal homeostasis through the lens of the inference framework (reviewed in Barrett and Simmons, 2015). This view hypothesizes that the brain forms representations constructed from previous experience while simultaneously using sensory information gathered during current experiences to anticipate a reaction. Thus, perception (based on the integration of past and current experiences) and action are tightly coupled. Extending on theories proposed by Barrett and colleagues who have used predictive processing to describe the development (Atzil et al., 2018) and regulation of social behavior via internal allostatic mechanisms involving the insular cortex (Atzil and Barrett, 2017), we propose that loneliness can be described as a breakdown of these processes. With loneliness being characterized by a strong prediction that attempts at social connection will end poorly, the individual anticipates this response regardless of sensory information currently being gathered that might suggest a different outcome. Furthermore, the insular cortex may play a key role in this process because of its integration of exteroception (perception of external sensory stimuli) and interoception (perception of the internal body) (Kleckner et al., 2017; Koeppel et al., 2020; Lefranc et al., 2020). For example, the agranular insular cortex is thought to contain “prediction neurons” that are ready to respond to an expected outcome (Barrett and Simmons, 2015). These neurons send their predictions in the form of chemical signals to the dysgranular and granular insular layers, altering the firing rate of these neurons in anticipation of sensory information arriving from the thalamus and visceromotor regions in the brain and body (Figure 1). The received sensory signal is compared to the prediction signal and “prediction error” is generated based on how much the dysgranular and granular neurons must change their firing to accommodate the sensory information. The goal of the brain and body is to minimize prediction error and maintain homeostasis, resulting in one of three options: the prediction error signal can be sent back to the agranular layer to update the internal prediction, behavior can be changed to physically modulate the incoming sensory input to match the prediction, or the neurons receiving the sensory signals can bias the influence of particular signals that match the prediction. Appropriate functioning of the insular cortex may be crucial for generating predictions about the social environment. Thus, reduced activation of the insular cortex in lonely individuals at rest or during presentation of social stimuli may reflect an inability to adjust the predicted outcome based on the received sensory stimuli. Furthermore, loneliness is associated with reduced white matter connectivity of the insular cortex to nodes in the attention network (Tian et al., 2014), suggesting that delivery of these signals to the insular cortex could be interrupted or weakened. Some neurons (termed “precision neurons”) in the insular cortex are also responsible for tuning the gain on prediction errors, thereby amplifying some signals and reducing others in an attempt to make sense of the overall input (Shipp et al., 2013; Quattrocki and Friston, 2014). These precision neurons, in particular, could be altered during the loneliness condition, thereby biasing signals that match the prediction (i.e., hypervigilance for social threat) and failing to incorporate others that would update the prediction. Indeed, social exclusion is associated with decreased interoceptive accuracy, which could be explained by altered functionality of the insular cortex. One neurochemical candidate that could be working in the insula to alter appropriate functioning is oxytocin (OT). In rats, OT in the rostral agranular insula is necessary for recognizing affective state of social conspecifics and drives social avoidance of stressed adult rats (Rogers-Carter et al., 2018). Additionally, OT is increased in isolated animals, which could cause over-attention to affective state and ultimately lead to reduced social interaction. Together, this information suggests hyperactivity of attentional networks, but confirmation bias and fear of rejection may prevent such information from being processed and transformed into the desired behavioral output (i.e., social contact).
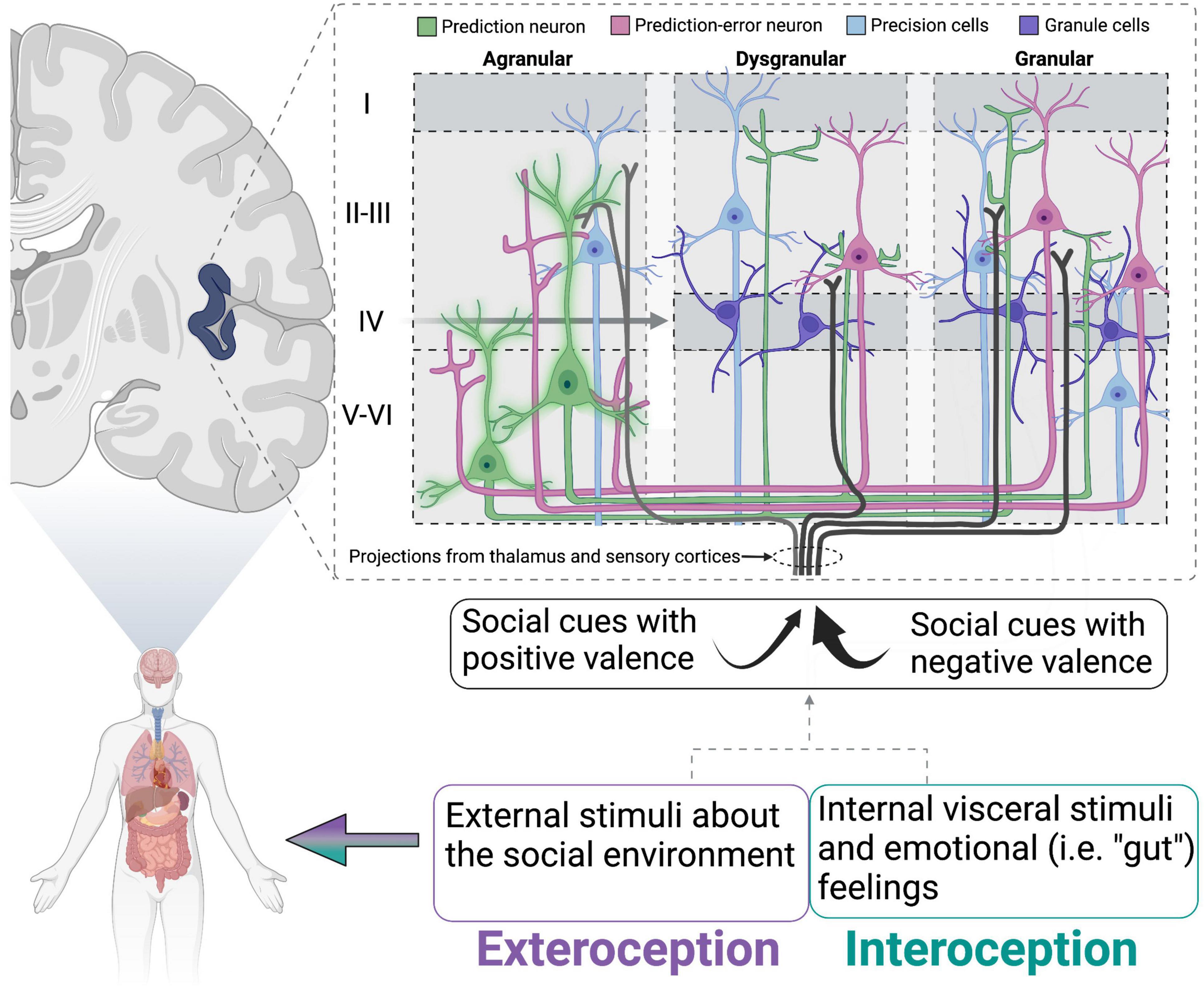
Figure 1. Schematic of proposed insular cortex activity in lonely individuals. Schematic of proposed insular cortex activity in lonely individuals (modeled from Barrett and Simmons, 2015). Prediction neurons (green) are “inflexible” or sending strong prediction signals to dysgranular and granular layers. Simultaneously, social cues/stimuli with negative valence (that match the prediction) are attended to more strongly than those that would generate a prediction error. This would lead to insufficient activation of prediction-error neurons, and therefore no error signal being relayed to prediction neurons to update the simulation. Oxytocin is particularly well situated to modulate the activity of precision neurons and has been hypothesized to do so in a similar model for autism spectrum disorders (reviewed in Quattrocki and Friston, 2014). Adapted from Barrett and Simmons (2015), with permission from Springer Nature.
As an acute response, social isolation should drive an individual to seek out social relationships analogous to the way hunger drives an individual to seek out and consume food. However, the findings synthesized above describe a dampening of motivation-related brain regions. Importantly, recent work has begun exploring the effects of acute social isolation in humans to better understand the shift in brain function and resulting behavioral output from acute to chronic isolation and loneliness. Interestingly, Tomova et al. (2020) found that acute social isolation induces midbrain activation that they likened to craving, as similar responses occurred in people that had fasted. Additionally, social and food craving led to activation of different subregions of the striatum, with social cues evoking responses in the caudate and food cues evoking responses in the nucleus accumbens (Tomova et al., 2020). Finally, they found that the increase in VTA activity and self-reported craving in the socially isolated group was blunted in individuals that scored high on chronic loneliness, suggesting that there is indeed a functional and behavioral shift that occurs when acute social isolation becomes chronic loneliness.
With regards to stress-related brain regions, findings generally indicate increased activation of the amygdala and hippocampus. For example, there is a positive correlation between amygdala volume and social distress score, and this effect was significantly mediated by loneliness (Tian, 2016). Social exclusion also increases activation of the amygdala, and this effect is greater in lonely individuals (Oh, 2020). Increased amygdala activation may be related to attention to socially salient stimuli. For example, socially embedded individuals display stronger coupling of the limbic system with the cortical salience network (which includes the ACC and IC; Bressler and Menon, 2010), while lonely individuals show reduced functional connectivity between these networks (Layden et al., 2017). Oxytocin may be involved in strengthening this connectivity in socially enriched individuals, as OT administration enhances the functional coupling of the amygdala with the cortical salience network during positive social interactions (Rilling et al., 2018). Furthermore, social network size and complexity is positively associated with amygdala volume and functional connectivity of the amygdala to both the affiliation network and the perception network (Bickart et al., 2012). This may reflect an increase in perceptual ability, particularly to affect-laden stimuli, required to foster and maintain a high number of social contacts (for reviews, see Adolphs and Spezio, 2006; Bickart et al., 2014). Because lonely individuals have heightened attenuation to emotional stimuli (Cacioppo et al., 2009), this could explain the increased amygdala activation. The amygdala also responds to threatening stimuli, which may be how lonely individuals perceive social cues based on their hypervigilance and heightened attention during social interaction (Pickett et al., 2004; Gardner et al., 2005). Moreover, reduced connectivity of the amygdala to higher-order cortical regions of the cingulo-opercular and salience networks could indicate an inability of these regions to influence or override the hypervigilance and threat sensitivity that accompanies loneliness. Hippocampal activity is also heightened during a social exclusion task (Eisenberger et al., 2007), and this region hosts a large density of glucocorticoid receptors that act as a negative feedback loop to prevent excess cortisol release during a stressful event (van Haarst et al., 1997). Together, amygdalar and hippocampal sensitivity in lonely persons may reflect their hypervigilance and stress-reactive state.
Summary of Brain Regions Implicated in Human Loneliness
The hallmark features of loneliness are an increase in desire to engage socially, increased hypervigilance and attention to social stimuli, and disrupted stress-reactivity. As such, loneliness is associated with alterations in resting and stimuli-induced activity in brain regions that are known to regulate these behaviors (Figure 2). For instance, activity and volume of attention and emotion-related regions (i.e., salience and frontoparietal networks) such as the IC, ACC, and PFC are significantly influenced by loneliness, as is activity of limbic regions including the amygdala and hippocampus. Functional connectivity between individual nodes within these networks and among the different networks also appears to be influenced by perceived isolation and loneliness, such that there is generally greater connectivity within regions in the salience and emotion networks and lower connectivity between regions of the salience network to regions of the limbic network. Taken together, the outlined activation and connectivity patterns within the brain of lonely individuals suggests reduced reaction to positive social stimuli, heightened reaction to negative social stimuli, reduced ability of the salience network to override limbic hyperactivity/hypervigilance, and elevated alertness/attention.
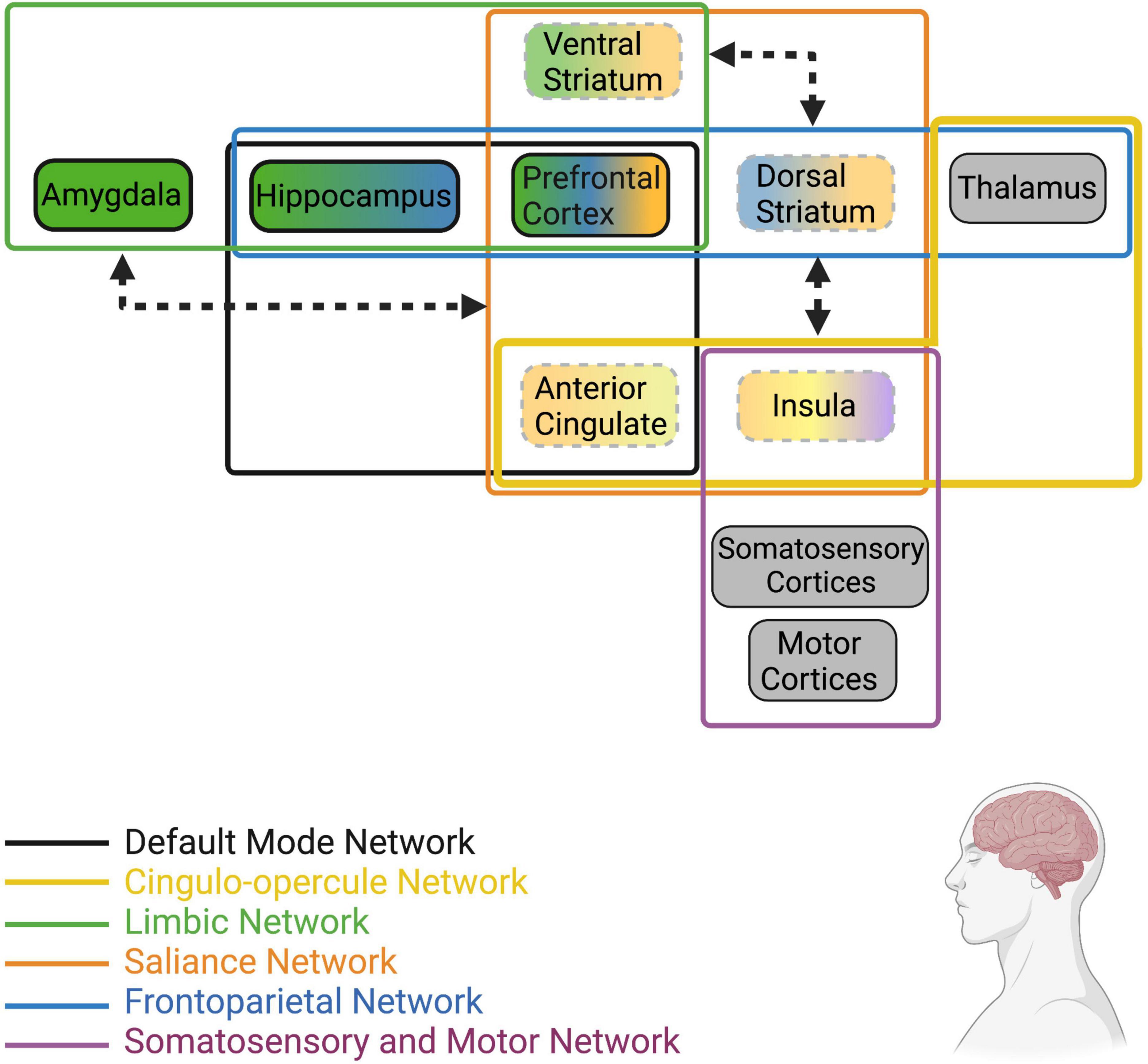
Figure 2. Human brain networks and brain regions within networks that have been implicated in loneliness. Colors represent the network that each region or group of regions belongs to (Note: this is not an extensive list of all known neural networks, only those that are most implicated in human loneliness). Dotted lines represent reduced resting state activity (for individual brain regions) or reduced functional connectivity (arrows between regions/networks) in lonely individuals compared to non-lonely individuals. Bolded lines represent increased resting state activity (for individual brain regions) or increased functional connectivity (arrows between regions/networks) in lonely individuals. Arrowheads touching network boxes denote altered connectivity between networks, while arrowheads touching brain regions denote altered connectivity between regions. Gray boxes represent regions receiving direct stimuli about the environment (i.e., exteroception, interoception, proprioception) and relay such information to other regions defined in these networks; however, information about their resting state activity or connectivity in lonely individuals is unknown at this time. For a recent review of the structural and functional alterations in brain regions and networks associated with loneliness, see Lam et al. (2021).
Loneliness Modeling in Animals
Animal Behavior Mimics the Human Loneliness Condition
Animal research is a valuable aid for understanding the mechanisms involved during the experience of social isolation; however, there will inevitably be gaps due to the perceptive component to human loneliness that may not be possible to accurately model using animals. So far, we know that social isolation causes a similar behavioral phenotype to human loneliness, characterized by increased desire for social contact (Panksepp and Beatty, 1980; Ikemoto and Panksepp, 1992; Panksepp and Burgdorf, 2000) followed by a deficiency in social interaction once it’s been obtained (Zelikowsky et al., 2018; de Moura Oliveira et al., 2019; Kaneda et al., 2021), increased stress- and depression-related behaviors (Parker and Morinan, 1986; Hellemans et al., 2004; Grippo et al., 2007b; Yorgason et al., 2013; Lander et al., 2017; Zorzo et al., 2019), cognitive deficits such as poor spatial learning and memory (Hellemans et al., 2004; Lander et al., 2017; Zorzo et al., 2019) and impaired novel object discrimination (Bianchi et al., 2006), increased threat responsiveness (Zelikowsky et al., 2018), and avoidance of (and hyper-activity when exposed to) new experiences/stimuli (Parker and Morinan, 1986; Gentsch et al., 1988; Varty et al., 2000). This behavioral phenotype is driven by alterations in a host of neurochemicals in a variety of brain sites, detailed below.
Alterations in Neurotransmitter and Neuropeptide Systems
Neurobiologically, the display of social behaviors in non-human animals is governed by similar brain regions and neurochemicals as they are in humans. For instance, DA in the ventral striatum, specifically the NAc, is the “end point” that drives motivated behaviors including social affiliation (reviewed in Floresco, 2015). The NAc is also modulated by OT, endogenous opioids (i.e., endorphins), and stress-related neuropeptides, the integration of which adjusts the salience of social stimuli depending on context and physiological state (Mannella et al., 2013). Using rodents to assess the effects of social isolation on the brain and behavior, interactions between DA, OT, stress neuropeptides, and endorphins both widely and in the NAc specifically have been identified and implicated in the behavioral adaptations that occur during isolation. It should be noted that the effects of social isolation on rodent social and emotional behavior and neurophysiology do vary based on the age at which the isolation occurs and the length of time that isolation is experienced. However, this review will mainly focus on isolation during adulthood unless otherwise specified. For a detailed review of the effects of social isolation during adolescence, see Walker et al. (2019).
Oxytocin
Because of its role in promoting affiliation, it is unsurprising that OT is protective against the negative behavioral and physiological consequences of social isolation. For instance, isolation-induced increased basal heart rate and reduced vagal regulation of the heart was reversed by systemic OT administration (Grippo et al., 2009). OT treatment also prevented isolation-induced cellular aging, characterized by oxidative damage and telomere degradation (Stevenson et al., 2019). Interestingly, social isolation is associated with an increase in the density of OT cells in the paraventricular nucleus of the hypothalamus (PVN), and an increase in the number of these cells that are activated via co-expression of the immediate early gene, cFos (Grippo et al., 2007a). Similarly, male and female hamsters that were socially isolated at weaning had higher OT mRNA in the PVN and lower oxytocin receptor (OTR) binding in the NAc in adulthood (de Moura Oliveira et al., 2019). Stress-induced serum OT was also heightened in animals that have been socially isolated (Grippo et al., 2007a). On the surface, these findings could be considered contradictory to the traditional anxiolytic view of OT (i.e., high OT = low anxiety). However, this heightening of endogenous OT activity during social isolation could indicate abnormal homeostatic processes, as acutely, OT is released from the PVN to facilitate appropriate behavioral responses to unpredictable threats (Dabrowska et al., 2011; Knobloch et al., 2012). Building on the concept of abnormal homeostatic mechanisms, Musardo et al. (2021) found that acute social isolation resulted in increased social interaction and a disruption in social habituation and social novelty preference. While these behaviors could be viewed as negative outcomes, it could be the result of brain mechanisms promoting social contact regardless of familiarity or length of contact (i.e., fewer “restrictions” on who to interact with as long as social contact is achieved). Importantly, inhibiting OT neurons in the PVN abolished the above behavioral effects, suggesting that increased OT release during acute isolation may be at least partially responsible for increased social-seeking behavior aimed at achieving social homeostasis. Oxytocin in the insular cortex, a region known to be less active in lonely humans, is also necessary for recognizing social affective stimuli. Adult male rats have a natural tendency to prefer interacting with stressed juvenile rats and avoid stressed adults, and blocking OTRs in the rostral agranular IC (RAIC) reduces interaction with stressed juveniles and increases interaction with stressed adults (Rogers-Carter et al., 2018). Moreover, OT injected into the RAIC further reduced social interaction with a stressed adult. Thus, increased OT release into the RAIC, presumed via upregulated PVN OT release during these conditions, could contribute to social avoidance.
Oxytocin also influences the HPA axis differently depending on the stress state of the animal. For example, blocking OT receptors in the PVN under basal conditions resulted in enhanced secretion of adrenocorticotrophic hormone (ACTH) from the pituitary gland, while under stress conditions OTR blockade reduced ACTH secretion (Neumann et al., 2000a,b). Additionally, OT administration prior to an elevated platform stress inhibits stress-induced CRH release via activation of GABA neurons, while post-stress OT administration has no effect on CRH release (Smith et al., 2016). These findings suggest that OT may suppress HPA axis function normally, but during stress experience facilitates HPA activity. Furthermore, these divergent roles of OT may involve differences in OT neuron population, receptor distribution, or receptor sensitivity under various conditions. OTRs are widely distributed throughout the brain and once bound to OT, can have varying effects on social behavior depending on the brain region being influenced. For instance, activating OT neurons in the bed nucleus of the stria terminalis (BNST) causes increased vigilance behavior in socially defeated female California mice (Peromyscus californicus) (Duque-Wilckens et al., 2018), while activating OTRs in the NAc promotes pair bonding in prairie voles. OTR distribution and sensitivity during isolation conditions could underly the ability of OT to promote or inhibit social interaction. Therefore, a disruption in the balance of OTRs and OT release in isolated animals may contribute to the altered social and stress-related behavior observed during isolation. To date, no studies exist that directly manipulate OTR activation in specific brain sites under isolation conditions; however, OTR expression does indeed vary by brain region in response to social isolation. OTR mRNA is increased in BNST (Perkins et al., 2019) following chronic social isolation, while OTR binding was lower in the DR of isolated female Syrian hamsters (Ross et al., 2019) and in the CeA of isolated male rats (Han et al., 2018). Thus, manipulating OTR activity in particular brain regions during social isolation would likely produce varying effects on behavior.
To add another layer of complexity, other neurotransmitters and neuropeptides interact with the OTergic system to modulate its regulation of social and stress-related behavior, and in turn, OT can do the same to those other systems. For instance, serotonin (5-HT) directly influences OT release via 5-HT1A and 2A receptors in the PVN, and this interaction has been shown to affect social behavior (Edwards et al., 2018). Similarly, DA modulates OT release and influences socio-sexual behavior via direct projections of DA fibers from the incertohypothalamic system to the mPOA, SON, and PVN (Buijs et al., 1984; Decavel et al., 1987; Holstege et al., 1996; Baskerville et al., 2009). The influence of OT on other systems appears to be widespread, as it not only interacts with 5-HT and DA, but also directly influences CRH and opioid activity. To list a few examples: OTRs expressed on serotonin (5-HT) terminals in the NAc are critical for social motivation (Dölen et al., 2013), OTR activation in the VTA is necessary for social reward in male and female Syrian hamsters (Borland et al., 2019), OT modulates HPA activity and CRH release via interaction with GABA-A receptors in the PVN (Smith et al., 2016), and OT binds to Mu opioid receptors as a positive allosteric modulator and directly influences anxiety-like behavior associated with chronic pain (Meguro et al., 2018; Miyano et al., 2021). Finally, the social salience hypothesis of OT proposes that OT regulates the salience of social stimuli and increases sensitivity to social cues depending on context and individual factors (reviewed in Shamay-Tsoory and Abu-Akel, 2016). The mechanism underlying this is also thought to involve interaction with the DArgic system since it governs the processing of aversive and rewarding events. Thus, OT interacting with other neurotransmitters and peptides expands its potential involvement in social isolation and the resulting stress-related behavior in a complex manner and further emphasizes the need for continued research on the nuanced neural mechanisms underlying these behaviors.
Dopamine
As stated above, DA is critically involved in the ability to receive, assign valence, and respond appropriately to a variety of rewarding and aversive stimuli. OT may also boost DAs role in social attention by increasing the valence of social stimuli, as both DA and OT are required for pair bond formation in prairie voles (Liu and Wang, 2003; Romero-Fernandez et al., 2013), and OT further activates the DA system during times in which social affiliation is increased (such as when maternal rats lick their pups; Shahrokh et al., 2010). In addition to its general role in social motivation, DA signaling has been shown to affect social-seeking behavior under isolation conditions. In fact, Matthews et al. (2016) propose that DA neurons in the midbrain dorsal raphe nucleus encode the experience of loneliness. They elegantly showed that activating these DA neurons in a social context promotes social interaction, while activating these neurons in the absence of a social conspecific is aversive. These DA neurons from the dorsal raphe almost exclusively innervate the amygdala and extended amygdala (i.e., central amygdala and bed nucleus of the stria terminalis; Hasue and Shammah-Lagnado, 2002) and regulate different behaviors depending on their downstream target. For instance, DRN DA neurons projecting to the CeA promote sociality, while those projecting to the posterior basolateral amygdala (BLP) regulate negative affective state (Tye et al., 2021). DA DRN neurons are involved in the perception of salient stimuli promoting vigilance and arousal (Cho et al., 2017). Because isolation increases glutamatergic tone on DA DRN neurons, the basal activity of these neurons in socially isolated animals creates an aversive experience that causes seeking of social contact to ameliorate the unpleasant state of being isolated. Another distinct DA circuit that is influenced by social isolation is the DA afferent projections from the VTA to corticostriatal regions including the prefrontal cortex and nucleus accumbens, though much of this work has been done in adolescent rodents. Specifically, DA neurons in the VTA are more sensitive in socially isolated rats, as stimulating them causes greater DA release in the NAc compared to group-housed rats (Karkhanis et al., 2018). This increased DA release in socially isolated rats can be evoked by both rewarding and aversive stimuli (Lapiz et al., 2003; Yorgason et al., 2013, 2016; Karkhanis et al., 2016). Basal extracellular DA level is not affected by social isolation (Jones G. H., 1992; Karkhanis et al., 2014), though some studies report an increase in DA turnover in the NAc (Hall et al., 1998). Postpartum rats that have had their pups removed and were socially isolated for 3 weeks showed reduced basal activity of DA neurons in the VTA accompanied by increased passive coping and reduced social motivation (Rincón-Cortés and Grace, 2021). Interestingly, when dams were housed with another postpartum rat that had her litter removed as well, passive coping behavior was partially ameliorated and VTA DA activity was restored.
The balance of basal and stimuli-induced DA release is critical for the appropriate processing of salient stimuli. For example, maternal rats experience a reduction in baseline DA and increased sensitivity and response of DA neurons to pup-related cues (Robinson et al., 2011) that allows them to respond with the appropriate behavior quickly. In the case of social isolation, it appears that the ability to discern between various stimuli may be impaired since high DA responsiveness occurs regardless of the stimulus. Interestingly, depleting DA in the NAc reverses the increase in pre-pulse inhibition response of socially isolated rats (Powell et al., 2003). This suggests that the signal to noise ratio might be low following social isolation, creating an inability to discern between salient stimuli and reducing the noise allows for more appropriate gating of behavioral responses.
Acutely and via the DArgic system, social isolation may drive social-seeking behavior as a mechanism to restore social homeostasis (reviewed in Matthews and Tye, 2019). However, as the isolation becomes more chronic and/or more distressing, the experience of social contact becomes more stressful due to increased hypervigilance and over-attention to social cues, and also less rewarding. Still, there is evidence that these long-term, isolation-induced alterations in DA signaling can be reversed by successful social reintroduction, suggesting that this shift from adaptive mechanism to maladaptive behavioral inflexibility can be undone. For example, non-human primates that were socially isolated for up to one and a half years were then reintroduced into social groups consisting of four animals. Repeated positron emission tomography (PET) scanning showed an increase in D2 receptors in the basal ganglia after as little as 3 months of social re-housing (Morgan et al., 2002). The neurobiological underpinnings governing the shift from homeostatic resolution to chronic reactivity and hypervigilance have not been clearly identified. Efforts to investigate this phenomenon could prove to be invaluable for developing therapeutic treatments for loneliness and isolation in humans.
Opioids
Endogenous opioids consist of three main groups: endorphins, enkephalins, and dynorphin. These peptides vary in both structure and binding affinity to the three main opioid receptors. Endorphin primarily binds to the mu opioid receptor (μ; MOR) and delta opioid receptor (δ; DOR), enkephalins have an affinity for DOR, and dynorphin binds primarily to kappa opioid receptor (κ; KOR). The opioid system is closely linked to the DArgic system as it is highly involved in motivated behavior. Additionally, the opioid system is a key regulator of pain sensation via both mu opioid and kappa opioid receptors. The sensation of pain can be viewed as an alert signal that tells the organism when something is not right. In the case of physical pain, humans and non-human animals respond quickly with a reflexive movement to alleviate the pain accompanied by release of endogenous opioids that ease the pain response (Mayer and Saper, 2000). Since pain is aversive, it is possible that the displeasure of being socially isolated could be processed by pain-sensing brain regions and include the involvement of endogenous opioids. In fact, “emotional pain” is an increasingly more common term used to describe feelings of social exclusion in humans (Mee et al., 2006; Eisenberger, 2008). In humans, involvement in intimate relationships elevates pain thresholds (Younger et al., 2010; Che et al., 2018), while in prairie voles, physical pain sensitivity is positively correlated with the anxiety-like symptoms produced by social isolation via partner loss (Osako et al., 2018). Because of its role in both reward and pain signaling, the opioid system is uniquely situated to modulate the interception of these states during social isolation. Indeed, opioids are broadly involved in social behavior, as opioid receptor agonists have been shown to reduce time spent in close proximity to social conspecifics (Herman and Panksepp, 1978; Panksepp et al., 1979), while opioid antagonists increase attempts to engage socially through allogrooming (Fabre-Nys et al., 1982; Martel et al., 1995). These behavioral responses are thought to be driven by interaction of opioids with the reward system, as activating opioid receptors would provide positive reward state and thus suppress the need to seek out pleasurable experiences via social contact. Similarly, blocking these receptors would produce an aversive experience and drive behaviors to ameliorate those feelings by engaging in affiliative behavior. Opioid administration also reduces the distress experienced by separation/isolation, as evidenced by reduced vocalizations of rat pups separated from their mother (Panksepp et al., 1978), and social affiliation increases endogenous opioid release (Keverne et al., 1989). Social isolation in rodents is also accompanied by reduced mRNA expression of mu and kappa opioid receptors in the hippocampus and amygdala, while systemically injecting a DOR agonist reverses isolation-induced anxiety-like behavior (Haj-Mirzaian et al., 2019). In humans, blocking opioid receptors reduces feelings of social connectedness, reduces perceptions of emotional and physical warmth, and reduces activity in the ventral striatum (Inagaki et al., 2015, 2016b,2020). Variation in human attachment is also associated with the opioid system, as humans with more avoidant attachment styles have lower mu opioid receptor availability, particularly in the anterior cingulate and insular cortices, while secure attachment was associated with higher MOR availability (Nummenmaa et al., 2015; Turtonen et al., 2021).
While mu opioid receptors have gained the most attention regarding the positive and rewarding aspects of social behavior, kappa opioid receptors (KORs) may mediate the aversive experience of stress, particularly via coordination with DA circuits (Margolis and Karkhanis, 2019). Forced swimming and foot shocks both produce aversive responses that are reversed by blocking KORs or deleting the KOR gene (i.e., KOR knockout mice; Land et al., 2008). This study also localized KORs involved in stress responsiveness to the BLA, NAc, DR, and hippocampus by showing their activity both during stress and during administration of corticotrophin releasing hormone (CRH). KOR blockade or deletion from DA transporter (DAT)-expressing cells in the NAc also prevents defeat-induced anhedonia when the social defeat is acute, but has no effect when defeat is chronic (Donahue et al., 2015), suggesting a shift in the functionality of KORs the longer a stressor is experienced. Thus, the opioid system may integrate motivation and stress-related circuits to assess threat, social stimuli, and motivational state to initiate a behavioral response. The opioid system may further modulate the connection between stress and social affiliation by acting directly on the OT and CRH systems. For instance, administering an exogenous opioid receptor agonist (i.e., morphine) enhances HPA axis responses to acute stress, while blocking opioid receptors reduces stress responsiveness (Buckingham and Cooper, 1986; Buller et al., 2005). Additionally, opioid receptor activation via morphine (exogenous), met-enkephalin (endogenous), and leu-enkephalin (endogenous) all directly stimulate CRH secretion from the hypothalamus (Buckingham and Cooper, 1984). Interestingly, the influence of endogenous opioid on HPA activity may change depending on physiological state. In support, blocking opioid receptors in pregnant rats leads to an increase in stress-induced CRH and OT release, indicating a tonic inhibitory effect of opioids during pregnancy (Brunton et al., 2005).
Comparing opioid involvement in social behavior to its involvement in drug addiction, it has been implicated that, acutely, social isolation may mimic a withdrawal state characterized by reduced endorphin release (via reduced social affiliation) in order to drive motivation for seeking out social contact (reviewed in Machin and Dunbar, 2011; Burkett and Young, 2012). Additionally, endorphin withdrawal coupled with a stress-induced increase in the dysphoria-inducing PDYN/KOR system could compound to create a strong desire to achieve social homeostasis. However, as social isolation becomes chronic, leading to alterations in physiological state characterized by high basal glucocorticoid secretion and exaggerated HPA activity, it’s possible that constant hypervigilance and stress-reactivity overrides the ability of the opioid system to respond during subsequent social interactions.
Neuropeptides (Corticotrophic Releasing Hormone and Tachykinin Peptides)
As has been outlined in this review, it is stressful to be alone and isolated from kin and peers. Both chronic and acute social isolation are characterized by altered responsiveness of the HPA axis, and thus a disruption in the production and release of the neuropeptides that regulate it. Acute (7 h) isolation from a partner significantly increases cortisol in marmosets, while blocking CRH-1 receptors during separation blunted this effect and increased sociosexual behavior without affecting alarm calls (French et al., 2007). Thus, CRH receptor activation appears to be involved in HPA responsiveness and anxiety-like behavior during acute isolation. In prairie voles, a single isolation event or repeated isolation, but not chronic isolation, result in higher expression of CRH mRNA in the hypothalamus and hippocampus of both males and females (Pournajafi-Nazarloo et al., 2009, 2011). All three isolation conditions result in reduced mRNA expression of CRH-R2 receptors in the hypothalamus, while chronic isolation was also associated with higher expression of this receptor subtype in the hippocampus (Pournajafi-Nazarloo et al., 2009) and reduced expression in cardiovascular tissue (Pournajafi-Nazarloo et al., 2007). Interestingly, one day of re-pairing with a sibling does not reverse the altered mRNA expression in repeated or chronically isolated animals (Pournajafi-Nazarloo et al., 2007, 2009). Additional studies have found that CRH binding protein (CRH-BP) is significantly influenced by social isolation. CRH-BP was originally thought to sequester CRH by binding to it and making it less available to bind to its receptors. However, more recent studies suggest that it may actually enhance CRH signaling through trafficking of CRH receptors to the cell surface (Ketchesin et al., 2017). Crhbp mRNA expression is significantly reduced following 24 h of isolation (Leser and Wagner, 2015; Lavenda-Grosberg, 2019), while the protein product of this gene is significantly more abundant in acutely isolated animals (Lavenda-Grosberg, 2019).
Although there are currently no studies that have directly manipulated the CRH system in socially isolated animals, this system has been the target of other studies utilizing various social stress paradigms. CRH-R1 receptor blockade reduces defeat-induced immobility in rats (Wood et al., 2010), while non-selective CRH blockade injected directly into the DR of Syrian hamsters reduced the acquisition and expression of conditioned defeat by reducing submissive and defensive behavior (Cooper and Huhman, 2007). Since chronic social defeat and chronic social isolation both upregulate the HPA system, including an increase in CRH-related gene and protein expression (Marini et al., 2006; Pournajafi-Nazarloo et al., 2009), it could be expected that directly manipulating the CRH system in isolated animals would impact stress-related behaviors. CRH may also affect social behavior during isolation, as central injection of CRH reduces the number of social interactions in rats (Dunn and File, 1987) and mice (Mele et al., 1987). Site-specifically, infusing CRH or urocortin-1 into the basolateral amygdala reduces social interactions (Rainnie et al., 2004; Spiga et al., 2006), while infusing urocortin-1 into the BSNT induces anxiety-like behavior during a social interaction test (Lee et al., 2008). Thus, the general effect of central CRH release, at least during a stress-naïve state, appears to be anti-social. Importantly, while increased CRH is typically indicative of an anxiogenic state, pair bonding in male prairie voles is accompanied by an increase in CRH mRNA in the BNST (Bosch et al., 2009) and CRH facilitates social bonding in males (DeVries et al., 1996, 2002). In females, however, glucocorticoid injection prevents pair bond formation while adrenalectomy facilitates it (DeVries et al., 1995). This sex difference could play a role during social isolation as well, because female prairie voles that were isolated for 6 weeks, a time point that has been shown to increase CRH immunoreactivity in the hypothalamus (Grippo et al., 2007a) showed increased affiliative behavior (Lieberwirth et al., 2012). Furthermore, CRH infused directly into the insular cortex suppresses presynaptic GABAergic neurotransmission, leading to greater excitatory synaptic efficacy, and increases social investigation in male rats but not female rats. The insular cortex is also responsible for detecting stress state of other animals, as inactivating the IC prevents the preference that male rats have for interacting with stressed juveniles and their avoidance of stressed adults (Rogers-Carter et al., 2018). CRH-R1 receptors in the IC appear to be necessary for this social decision-making process, but only in male rats (Rieger et al., 2021). These findings describe a complex role for CRH activity in regulating social and affiliative behavior that varies based on sex and stress experience.
While CRH has received much attention due to its widely known role in stress and anxiety-related behaviors, other work has shown that social isolation may affect social behavior via tachykinin peptides. Specifically, tachykinin 2 (Tac2) mRNA and neurokinin B (NkB) immunostaining are upregulated in the dorsal BNST, central amygdala (CeA), dorsomedial hypothalamus (DMH), and ACC following social isolation (Zelikowsky et al., 2018). Additionally, blocking Nk3 receptors, which show the highest affinity for NkB, reverses social isolation-induced aggression and heightened threat response. Tac2 knockdown in the DMH attenuates isolation-induced aggression, while Tac2 knockdown in the CeA attenuates threat and fear responsiveness, showing that Tac2 expression in specific brain regions differentially mediates isolation-induced social and stress-related behaviors.
Summary of Neurobiology Underlying Social Isolation
Animal models of social isolation reveal a number of alterations in neurobiological systems that regulate social behavior, motivation, and stress reactivity (see Figure 3 for circuit summary). Although this review focused only on OT, DA, opioids, and CRH, it is known that other neurotransmitter and neuropeptide systems are involved in the physiological and behavioral effects of social isolation in both humans and non-human animals. Still, it is likely that at least these four systems influence isolation-induced behavior in humans in a similar manner to their influence in animals. For instance, OT has been consistently shown to affect social and affection-related behavior in humans, even in lonely or excluded individuals (Lucht et al., 2009; Norman et al., 2011). DA and endogenous opioids are also known to underly the pleasurable experiences of social touch and other rewarding aspects of social connection (Inagaki et al., 2015, 2016b,2020), and activity and connectivity of DA- and opioid-rich brain regions such as the ventral striatum, insula, and anterior cingulate cortex is also disrupted in lonely individuals (Cacioppo et al., 2009; Nakagawa et al., 2015; Morr et al., 2021). Finally, HPA responsivity is disrupted in lonely individuals, and downstream targets of HPA activity including the amygdala and hippocampus show altered activation in lonely people (Tian, 2016).
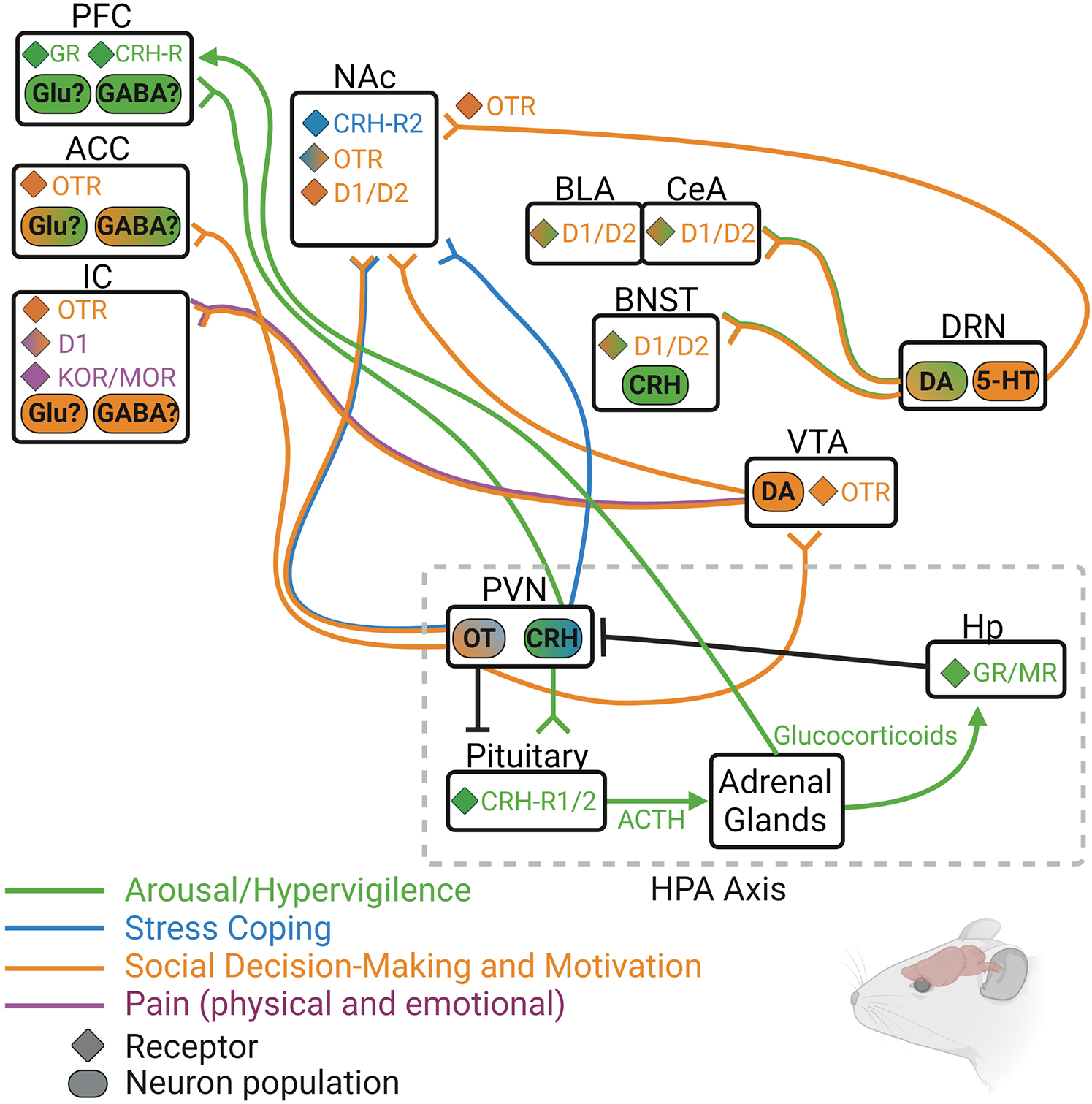
Figure 3. Rodent model depicting some of the known neurochemical circuits that regulate hypervigilance (green), social motivation (orange), passive coping (blue), and pain (purple), with particular focus on the four main neurochemical systems discussed in this review: oxytocin (OT/OTR), dopamine (DA/D1/D2), endogenous opioids (DOR/KOR/MOR), and corticotrophic releasing hormone (CRH/CRH-R1/2). 5-HT, serotonin; ACC, anterior cingulate cortex; ACTH, adrenocorticotrophic hormone; BLA, basolateral amygdala; BNST, bed nucleus of the stria terminalis; CeA, central amygdala; DRN, dorsal raphe nucleus; GR, glucocorticoid receptor; Hp, hippocampus; IC, insular cortex; MR, mineralocorticoid receptor; NAc, nucleus accumbens; PFC, prefrontal cortex; PVN, paraventricular nucleus of the hypothalamus; VTA, ventral tegmental area.
Areas of Mutual Interest for Human and Animal Researchers Studying Loneliness and Isolation
Several reviews have been published identifying the neurobiological correlates for loneliness in humans and the behavioral and neurophysiological effects associated with social isolation in rodent models (Cacioppo J. T. et al., 2015; Mumtaz et al., 2018; Tomova et al., 2021). As mentioned previously, it is difficult to directly compare the two literatures because of the largely subjective nature of loneliness in humans. However, here we will highlight some similarities in the neural correlates and brain regions affected by both experiences and will draw from the more detailed knowledge of the neurochemical underpinnings of isolation garnered from the animal research to make hypotheses about their contribution in the human loneliness condition.
One important aspect of human loneliness that is conserved in animal models using social isolation is the high-stress state that is produced as a result of being either subjectively or objectively isolated. In humans, loneliness is associated with increased social anxiety (Lieberz et al., 2021a), heightened attention to social cues (Pickett et al., 2004; Gardner et al., 2005) and increased amygdala activity and gray matter volume (Tian, 2016). In adult prairie voles, chronic social isolation reduced the total number and the overall length of dendritic branches while increasing the spine density in interneurons within the BLA (Hylin et al., 2022). These results suggest a possible reduction in the number of neuronal signals received via reduced dendritic matter and a simultaneous increase in synaptic efficacy of the signals that are received via increased number of synapses. This could indicate a biasing or “tuning in” of particular BLA neurons to specific signals being received from certain brain regions. One projection that could be biased is that of DA neurons coming from the DR, as they are especially active during isolation. In fact, DR DA neurons almost exclusively project to limbic regions, and those that terminate in the amygdala drive social behavior and negative affective state in isolated rodents. OT may also be involved, as plasma levels are increased during chronic social isolation in rodents (Hylin et al., 2022), and in humans OT modulates attention to both positively and negatively valenced social cues (Domes et al., 2007; Gamer et al., 2010). OT neurons also project to dopaminergic neurons in both the VTA (Hung et al., 2017) and, although to a lesser extent, the DR (Otero-García et al., 2016; Fujisaki et al., 2020). Silencing PVN OT neurons in juvenile rats that have been socially isolated for 1 week restores social novelty preference, indicating that over-activation of these neurons during isolation may drive input to DA neurons to increase their activity and alter social behavior (Musardo et al., 2021).
In lonely humans, reward-related brain regions show reduced activation, which may seem counter to the above findings in rodents indicating heightened activity of DAergic regions; however, acute isolation in humans does increase VTA activity in response to social cues, although when loneliness was factored into these analyses the increase in VTA responsiveness to social cues was blunted in lonely individuals (Tomova et al., 2020). Thus, it is unclear whether DA activity remains heightened as acute social isolation becomes chronic or as objective isolation becomes subjective (i.e., loneliness). Excessive DA release in the dorsal striatum does reduce social behavior and social exploration in adult non-isolated mice (Lee Y. et al., 2018). Additionally, continuous over-activation of DAergic system over a long period of time would likely cause alterations in receptor expression or responsiveness, which could affect the strength and efficiency of the signal being received by downstream DAergic target regions. To this point, some downstream targets of DA are more active in lonely humans (amygdala and hippocampus) while others are less active (ACC, insula). If overall DA activity is heightened during loneliness and social isolation, this could simultaneously increase the desire to interact socially while promoting increased attention and hypervigilance during said social encounters, which may alter the social experience to provide less reward value. Furthermore, while human loneliness is associated with reduced activation of reward regions in the brain both at resting state and during presentation of social stimuli, activity in these regions is increased during presentation of familiar faces (Inagaki et al., 2016a). An interesting animal correlate to this behavior is one that researchers typically interpret as a social learning deficit, characterized by the inability of a rodent that has been socially isolated to spend more time with a novel animal over an animal they have already interacted with (Shahar-Gold et al., 2013; Lavenda-Grosberg et al., 2021). One could alternatively interpret this behavior as a familiarity preference rather than a lack of novelty preference. If an individual is anxious about social encounters, they may be more likely to interact with the social stimulus that they already know rather than a novel one that they have not encountered before. Alternatively, the social homeostasis model (reviewed in Lee et al., 2021) postulates that after continued attempts to engage in social behavior during acute isolation, animals will eventually switch from an active coping strategy to a passive one, leading to an adjustment in homeostatic set-point for social contact. In other words, the neural circuits that promote social behavior during acute isolation (driving an animal to get back to its social set point by restoring social connections) are the same ones that promote antisocial behavior during chronic social isolation (driving an animal to maintain it’s new social set-point by avoiding social conspecifics). Thus, reduced social novelty preference during chronic isolation may instead be the result of homeostatic mechanisms geared toward maintaining the new social set-point that is now characterized by a social deficit.
In mice, one brain region that drives novelty preference is the interpeduncular nucleus (IPN), as photoactivating this region reduces exploration of a novel social stimulus, mimicking familiarity, while reducing activation of the IPN during social conditioned place preference increases the rewarding properties of a familiar social encounter (Molas et al., 2017). As a novel social stimulus becomes familiar, activity within the IPN increases via cholinergic-glutamatergic projections from the medial habenula (mHb) to the IPN, whereas during an encounter with a novel stimulus, VTA-IPN projections activate D1 receptors to increase the salience of the novel stimulus to promote exploration. Post-weaning social isolation blunts activation of the Hb in response to a novel social encounter (Ahern et al., 2016); thus, reduced Hb activity resulting from isolation may prevent the encoding of a novel stimulus as familiar in order to bias interaction with familiar social conspecifics. The Hb and IPN have been understudied in lonely humans; however, novelty is also detected by higher order brain regions within the cognitive control networks, many of which are blunted in activity in lonely individuals (Cacioppo et al., 2009; Inagaki et al., 2015; Wong et al., 2016). These cognitive control networks, including the frontoparietal and cingulo-opercule networks, are also involved in conflict-detection, goal-oriented behavior, and behavioral flexibility, and disruptions in the ability to perform such tasks could underly some of the behavioral phenotype featured during loneliness.
Finally, the human and animal literatures could benefit from a more cohesive research trajectory. Although methodologically difficult, future research in humans could attempt to identify neurotransmitter and/or neuropeptide systems that are affected by perceived social isolation, either by measuring plasma or CSF concentrations of these neurochemicals or using PET ligands. Meanwhile, animal studies can begin further characterizing the neural circuits that regulate the behavioral phenotypes resulting from various lengths of isolation, particularly related to regions that show altered activity or connectivity in lonely humans. Additionally, a more specific time course could be identified characterizing the shift from normal, sporadic feelings of loneliness (and isolation) to pathological and chronic loneliness. Lee et al. (2021) make the point that the behavioral shift that occurs between acute and chronic isolation may be mechanistically linked to one or more variables other than time, including effort expended or number of rejected attempts. Assessing more specific factors that contribute to or influence feelings of loneliness (or the behavioral correlates in animals) is an area that would be interesting for both human and animal researchers to explore.
Social Loss as a Unique Form of Social Isolation
Losing a relationship happens naturally in social species. A relationship may dissolve due to conflict, when one individual leaves to join a new social group, or even from death of a loved one. We know that the response of an individual to social loss depends on the strength of the relationship and the type of relationship (Smigelsky et al., 2019; Sekowski and Prigerson, 2021); however, the mechanisms involved in the behavioral and physiological response to loss is poorly understood. Additionally, examining how the brain adapts to loss and overcomes the negative consequences of loss to allow an individual to form new relationships could provide valuable insights for recovery during grief and loss in humans.
Grief and Bereavement in Humans
Each year in the US, approximately 8 million individuals suffer from the loss of a friend or loved one, leaving over 800,000 new widows and widowers who are at increased risk of mortality during the first 6 months following their loss (Rees and Lutkins, 1967; Hensley et al., 2009). Similar to social isolation, social loss is distressing and leads to increased stress, anxiety, and feelings of loneliness (Zisook and Shear, 2009; Fried et al., 2015). However, one key difference is that increased social connectedness does not reverse the feelings of loneliness and isolation in bereaving individuals. In support, married women with low perceived social support report feeling lonelier than those with high perceived social support, while widowed women report high emotional and social loneliness regardless of their perceived social support (Stroebe et al., 1996). Additionally, while loneliness decreases over time in bereaving individuals, so does social support, suggesting that the two are not inversely related (Utz et al., 2014). Bereavement and social isolation are also characterized by alterations in brain activity in the same brain regions, such as the insula and anterior cingulate cortex (Gündel et al., 2003; O’Connor et al., 2007). However, lonely individuals display reduced activity in these regions both at baseline and in response to presentation of social-related cues (Nakagawa et al., 2015; Morr et al., 2021), while those experiencing loss have significantly increased activity in the insula and anterior cingulate cortex following presentation of a picture of the deceased individual (Gündel et al., 2003; O’Connor et al., 2007). Interestingly, lonely individuals that view photos of a familiar social conspecific do display higher activation of the insula compared to when they view photos of a stranger (Inagaki et al., 2016a). These results suggest that the insula may be more sensitive to familiar social cues than to unfamiliar social cues during loneliness. The insula is involved in emotional processing and empathy, which both may be increased when viewing pictures of someone familiar and especially in someone who is craving social interaction with a particular person. Interestingly, increased activity of the insula in bereaving individuals is reduced as time since loss increases, assuming that the individual is coping well. However, one distinct brain region that remains highly activated in persons having trouble coping with loss (termed complicated grief) is the ventral striatum (i.e., NAc; O’Connor et al., 2008). O’Connor et al. (2008) propose that over time, and in individuals with non-complicated grief, the NAc becomes less activated by reminders of the lost loved one. Meanwhile, those experiencing complicated grief continue yearning for the deceased individual through increased responsiveness of the NAc. The NAc receives connections from both the insula and anterior cingulate cortex. Activation of the insula during reminders of the deceased loved one could cause excitation of the NAc, leading to a yearning response. Another study found that complicated grief was associated with reduced rostral anterior cingulate cortex activation compared to the non-complicated grief group during an emotional Stroop task where individuals were tasked with ignoring deceased-related words (Arizmendi et al., 2016). The authors postulate that individuals experiencing complicated grief may be unable to emotionally regulate during reminders of the deceased. Additionally, nodes in the attention network are more active in participants with high avoidance of deceased-related thoughts during a mind-wandering task and were less likely to report thinking about the deceased (Schneck et al., 2019a,b). Avoiding reminders of the lost individual may be a maladaptive coping strategy for those who experience intense yearning and emotional pain resulting from such reminding. This may ultimately prolong the time it takes to heal from the loss as the individual has fewer opportunities to learn how to appropriately cope with these thoughts.
Modeling Grief Using Social Loss in Pair-Bonded Animals
Several non-human mammal species form socially monogamous mating pairs, and such species have been used in a laboratory setting to study the formation and maintenance of strong social bonds. The prairie vole, in particular, has become a valuable tool for understanding the neural mechanisms underlying social relationships. Unsurprisingly, the OT and DA systems are involved in the pair bonding process, though additional work has described roles for vasopressin, the opioid system, and the serotonergic system (reviewed in McGraw et al., 2010). Recently, several vole research labs have begun to study the neural and behavioral effects of losing a pair bonded partner with the hopes of understanding more about how loss is processed in the brain. Bosch and colleagues found that male prairie voles that lost a female partner displayed increased stress responsivity compared to those that remained in an intact pair and those that were separated from a same-sex sibling (Bosch et al., 2009). This behavior also corresponded with increased corticosterone levels. Interestingly, they also found that pair-bonding regardless of loss resulted in increased CRH mRNA in the medial bed nucleus of the stria terminalis (mBST) and blocking central CRH receptors during loss reversed the increase in passive coping behavior of males separated from their partner. Other studies have repeatedly found that partner loss adversely affects stress-coping behavior and potentially does so through alterations in the physiological stress response (governed by the HPA axis). For example, separated males and females display higher corticosterone and ACTH circulation, higher resting heart rate, and an imbalance of autonomic nervous system function (McNeal et al., 2014). Sun and colleagues also found increased CRH, OT, and AVP immunoreactivity in the paraventricular nucleus (Sun et al., 2014). Moving downstream to assess receptor binding sites for the neuropeptides that appear to be affected by loss, Bosch et al. (2016) saw reduced OTR binding in the NAc shell of animals experiencing loss of a female partner compared to those experiencing loss of a male sibling. They then showed a direct influence of OT on stress-coping behavior by infusing either OT or an OTR antagonist into the NAc and saw a reduction and an increase, respectively, in passive coping behavior. Relationship quality also has a significant impact on distress following loss of a partner. Although the majority of prairie voles will form a partner preference with an opposite sex animal, a small percentage (∼25%) will not form a pair bond. These animals, termed non-bonded, do not show increased anxiety-like behavior following loss of their partner while pair bonded males are more anxious and more sensitive to pain after loss (Osako et al., 2018). This suggests that forming a strong, high-quality bond with another individual leads to more distress when that relationship is lost and corroborates findings in humans that suggest relationship quality and type influence grief outcome (Smigelsky et al., 2019; Sekowski and Prigerson, 2021).
A study in titi monkeys found that pair-bonding was associated with higher overall glucose uptake in the entire brain, and short-term separation from a pair-mate reduced glucose uptake in the ventral pallidum, lateral septum, PVN, and periaqueductal gray (PAG). Interestingly, long-term separation significantly reduced whole brain glucose uptake, suggesting reversal of the brain-wide changes in activity associated with a pair-bonded state (Hinde et al., 2016). Physiologically, short-term separation resulted in increased plasma cortisol concentrations, while long-term separated animals no longer showed heightened cortisol and instead had higher plasma OT concentrations. Elevated OT concentrations may be necessary for preserving social motivation for partner interaction during separation, as animals treated with an OT antagonist spent less time in proximity with their pair-mate upon reunion following a long separation (Cavanaugh et al., 2018).
Current research is attempting to determine a timeline for the degradation of bond-related neurochemistry. Male prairie voles that lose their partner will continue to display a preference for their partner for up to 2 weeks following loss (Sun et al., 2014). However, by 4 weeks post-loss this partner preference is no longer present, and these males are able to form new partnerships. In fact, 4 weeks of separation from an old partner is required to replace the preference for the first partner with the second partner (Harbert et al., 2020), supporting the theory that the brain adapts over time to the loss of a specific relationship. This research group followed these results with a study showing that pair bonding is associated with drastic changes in gene transcription in the NAc, many of which were degraded after 4 weeks of separation from a partner (Sadino et al., 2021). Future studies should assess whether variation in this process predicts individual differences in loss outcome as this could be a particularly valuable finding that is translational to humans coping with grief after losing a loved one.
While rats and mice do not display attachment to mates in the same way as socially monogamous animals, they do experience social attachment in the form of maternal behavior toward offspring. The maternal brain undergoes a host of neurochemical changes that enable high motivation, aggression toward intruders, and blunted emotional reactivity which all serve to create a behavioral phenotype that ensures survival of the infants (Lonstein et al., 2015). However, permanently removing pups from the mother reverses her low anxiety state (Kalyani et al., 2017), increases behavioral despair (Pawluski et al., 2009), and reduces social motivation and responsiveness of VTA DA neurons (Rincón-Cortés and Grace, 2021). Thus, postpartum social isolation, loss of the mother-infant social relationship, or some combination of both lead to similar behavioral and neurochemical phenotypes that are associated with isolation in other models. Additionally, maternal rats and mice are highly motivated to reunite with their offspring, as they will learn to bar press for access to their pups (Wilsoncroft, 1969; Lee et al., 1999) and even find them more rewarding than cocaine during early lactation (Mattson et al., 2003); thus, separation from infants results in motivation to reunite with them. Furthermore, infant loss in non-human primates can be devastating (Watson and Matsuzawa, 2018; Sharma et al., 2020), while infant loss in humans is associated with post-traumatic stress disorder (Jind et al., 2010), anxiety and depression (Kreicbergs et al., 2004; Hunter et al., 2017), and prolonged grief disorder (Lundorff et al., 2017). Therefore, utilizing rodent models of maternal separation to identify the possible neural underpinnings of loss and stress reactions during infant removal may prove to be beneficial for understanding parental grief reactions following infant death (for a recent review, see Demarchi et al., 2021).
Summary and Conclusion
This review comes at a particularly salient moment where many individuals across the globe are experiencing isolation from peers and loved ones due to the COVID-19 pandemic. As such, loneliness has been prevalent over the last two years as people have been self-isolating or quarantining due to stay at home orders (Killgore et al., 2020; Li and Wang, 2020; van Tilburg et al., 2021), and even populations that are not typically at risk for feeling lonely (i.e., students) are suffering from consequences of isolation (Bu et al., 2020). While many individuals are experiencing a deficit in the quality and quantity of social relationships during the COVID-19 pandemic, a recent meta-analysis indicates that a variety of psychological interventions are successful in reducing feelings of loneliness (Hickin et al., 2021). However, another meta-analysis revealed that interventions that simply attempted to increase social support or social contact were less effective than those that attempted to target the maladaptive cognitions that foster loneliness (Masi et al., 2011), suggesting that individuals may need to take a more active role in breaking the cycle of loneliness. Thus far, no studies have examined brain activity or connectivity in lonely individuals that have received successful interventions. This would certainly be an interesting research topic, and we speculate that since interventions that are aimed at interrupting the negative thought cycle are more successful, this may be associated with increased cognitive control and thus an increase in activity within frontoparietal and/or cingulo-opercule regions.
Not only are we physically separated from much of our social support, but many people are also dealing with the loss of a loved one due to COVID-19. This shared global experience has emphasized the need for continued research on the neural underpinnings not only of loneliness, but of loss and grief processing as well. Like loneliness, loss of a loved one is associated with altered activity in similar brain regions, leading to alterations in motivation and stress reactivity. Although loneliness is a common experience for grieving individuals, research using humans and animal models suggest that the loneliness state during this period is unique from the experience of loneliness in the absence of loss. This is likely due to the structural and neurochemical changes that occur during the formation (and subsequent maintenance) of a strong bond/relationship that appears to be more rewarding, and therefore harder to get over, than isolation from peers. Further research using the prairie vole model of social loss appears to be aimed toward understanding the natural degradation of relationship-related neural pathways, while additional work should assess the features of an individual or a relationship that makes it harder to move on from some compared to others. Investigating loneliness, isolation, and loss through various lenses could allow us to find a new approach to improving the quality of life for those experiencing such conditions.
Author Contributions
EV and AS wrote and edited all versions of this manuscript. Both authors contributed to the article and approved the submitted version.
Funding
AS was supported by NIH grant R01-NS113104-01.
Conflict of Interest
The authors declare that the research was conducted in the absence of any commercial or financial relationships that could be construed as a potential conflict of interest.
Publisher’s Note
All claims expressed in this article are solely those of the authors and do not necessarily represent those of their affiliated organizations, or those of the publisher, the editors and the reviewers. Any product that may be evaluated in this article, or claim that may be made by its manufacturer, is not guaranteed or endorsed by the publisher.
Acknowledgments
All figures were created with BioRender.com.
References
Adolphs, R., and Spezio, M. (2006). Role of the amygdala in processing visual social stimuli. Prog. Brain Res. 156, 363–378. doi: 10.1016/S0079-6123(06)56020-0
Ahern, M., Goodell, D. J., Adams, J., and Bland, S. T. (2016). Brain regional differences in social encounter-induced Fos expression in male and female rats after post-weaning social isolation. Brain Res. 1630, 120–133. doi: 10.1016/j.brainres.2015.11.006
Anderson, C. M., and Martin, M. M. (1995). The effects of communication motives, interaction involvement, and loneliness on satisfaction: a model of small groups. Small Group Res. 26, 118–137. doi: 10.1177/1046496495261007
Arioli, M., Gianelli, C., and Canessa, N. (2021). Neural representation of social concepts: a coordinate-based meta-analysis of fMRI studies. Brain Imaging Behav. 15, 1912–1921. doi: 10.1007/s11682-020-00384-6
Arizmendi, B., Kaszniak, A. W., and O’Connor, M. F. (2016). Disrupted prefrontal activity during emotion processing in complicated grief: an fMRI investigation. Neuroimage 124, 968–976. doi: 10.1016/j.neuroimage.2015.09.054
Atzil, S., and Barrett, L. F. (2017). Social regulation of allostasis: commentary on “Mentalizing homeostasis: the social origins of interoceptive inference” by Fotopoulou and Tsakiris. Neuropsychoanalysis 19, 29–33. doi: 10.1080/15294145.2017.1295214
Atzil, S., Gao, W., Fradkin, I., and Barrett, L. F. (2018). Growing a social brain. Nat. Hum. Behav. 2, 624–636.
Barrett, L. F., and Simmons, W. K. (2015). Interoceptive predictions in the brain. Nat. Rev. Neurosci. 16, 419–429. doi: 10.1038/nrn3950
Baskerville, T. A., Allard, J., Wayman, C., and Douglas, A. J. (2009). Dopamine–oxytocin interactions in penile erection. Eur. J. Neurosci. 30, 2151–2164. doi: 10.1111/j.1460-9568.2009.06999.x
Bianchi, M., Fone, K. F. C., Azmi, N., Heidbreder, C. A., Hagan, J. J., and Marsden, C. A. (2006). Isolation rearing induces recognition memory deficits accompanied by cytoskeletal alterations in rat hippocampus. Eur. J. Neurosci. 24, 2894–2902. doi: 10.1111/j.1460-9568.2006.05170.x
Bickart, K. C., Dickerson, B. C., and Barrett, L. F. (2014). The amygdala as a hub in brain networks that support social life. Neuropsychologia 63, 235–248. doi: 10.1016/j.neuropsychologia.2014.08.013
Bickart, K. C., Hollenbeck, M. C., Barrett, L. F., and Dickerson, B. C. (2012). Intrinsic amygdala–cortical functional connectivity predicts social network size in humans. J. Neurosci. 32, 14729–14741. doi: 10.1523/JNEUROSCI.1599-12.2012
Borland, J. M., Aiani, L. M., Norvelle, A., Grantham, K. N., O’Laughlin, K., Terranova, J. I., et al. (2019). Sex-dependent regulation of social reward by oxytocin receptors in the ventral tegmental area. Neuropsychopharmacology 44, 785–792. doi: 10.1038/s41386-018-0262-y
Bosch, O. J., Dabrowska, J., Modi, M. E., Johnson, Z. V., Keebaugh, A. C., Barrett, C. E., et al. (2016). Oxytocin in the nucleus accumbens shell reverses CRFR2-evoked passive stress-coping after partner loss in monogamous male prairie voles. Psychoneuroendocrinology 64, 66–78. doi: 10.1016/j.psyneuen.2015.11.011
Bosch, O. J., Nair, H. P., Ahern, T. H., Neumann, I. D., and Young, L. J. (2009). The CRF system mediates increased passive stress-coping behavior following the loss of a bonded partner in a monogamous rodent. Neuropsychopharmacology 34, 1406–1415. doi: 10.1038/npp.2008.154
Bosch, O. J., and Neumann, I. D. (2012). Both oxytocin and vasopressin are mediators of maternal care and aggression in rodents: from central release to sites of action. Horm. Behav. 61, 293–303. doi: 10.1016/j.yhbeh.2011.11.002
Bressler, S. L., and Menon, V. (2010). Large-scale brain networks in cognition: emerging methods and principles. Trends Cogn. Sci. 14, 277–290. doi: 10.1016/j.tics.2010.04.004
Brunton, P. J., Meddle, S. L., Ma, S., Ochedalski, T., Douglas, A. J., and Russell, J. A. (2005). Endogenous opioids and attenuated hypothalamic-pituitary-adrenal axis responses to immune challenge in pregnant rats. J. Neurosci. 25, 5117–5126. doi: 10.1523/JNEUROSCI.0866-05.2005
Bu, F., Steptoe, A., and Fancourt, D. (2020). Who is lonely in lockdown? Cross-cohort analyses of predictors of loneliness before and during the COVID-19 pandemic. Public Health 186, 31–34. doi: 10.1016/j.puhe.2020.06.036
Buckingham, J. C., and Cooper, T. A. (1984). Differences in hypothalamo-pituitary-adrenocortical activity in the rat after acute and prolonged treatment with morphine. Neuroendocrinology 38, 411–417. doi: 10.1159/000123927
Buckingham, J. C., and Cooper, T. A. (1986). Pharmacological characterization of opioid receptors influencing the secretion of corticotrophin releasing factor in the rat. Neuroendocrinology 44, 36–40. doi: 10.1159/000124618
Buijs, R. M., Geffard, M., Pool, C. W., and Hoorneman, E. M. D. (1984). The dopaminergic innervation of the supraoptic and paraventricular nucleus. A light and electron microscopical study. Brain Res. 323, 65–72. doi: 10.1016/0006-8993(84)90265-8
Buitelaar, J. K. (2013). The role of the HPA-axis in understanding psychopathology: cause, consequence, mediator, or moderator? Eur. Child Adolesc. Psychiatry 22, 387–389. doi: 10.1007/s00787-013-0441-7
Buller, K. M., Hamlin, A. S., and Osborne, P. B. (2005). Dissection of peripheral and central endogenous opioid modulation of systemic interleukin-1β responses using c-fos expression in the rat brain. Neuropharmacology 49, 230–242. doi: 10.1016/j.neuropharm.2005.03.014
Burkart, J. M., and van Schaik, C. P. (2016). Revisiting the consequences of cooperative breeding. J. Zool. 299, 77–83. doi: 10.1111/jzo.12351
Burke, T. J., Woszidlo, A., and Segrin, C. (2012). Social skills, family conflict, and loneliness in families. Commun. Rep. 25, 75–87.
Burkett, J. P., and Young, L. J. (2012). The behavioral, anatomical and pharmacological parallels between social attachment, love and addiction. Psychopharmacology 224, 1–26. doi: 10.1007/s00213-012-2794-x
Cacioppo, J. T., Cacioppo, S., Capitanio, J. P., and Cole, S. W. (2015). The neuroendocrinology of social isolation. Annu. Rev. Psychol. 66, 733–767. doi: 10.1146/annurev-psych-010814-015240
Cacioppo, J. T., Ernst, J. M., Burleson, M. H., McClintock, M. K., Malarkey, W. B., Hawkley, L. C., et al. (2000). Lonely traits and concomitant physiological processes: the MacArthur social neuroscience studies. Int. J. Psychophysiol. 35, 143–154. doi: 10.1016/s0167-8760(99)00049-5
Cacioppo, J. T., Norris, C. J., Decety, J., Monteleone, G., and Nusbaum, H. (2009). In the eye of the beholder: individual differences in perceived social isolation predict regional brain activation to social stimuli. J. Cogn. Neurosci. 21, 83–92. doi: 10.1162/jocn.2009.21007
Cacioppo, J. T., and Patrick, W. (2008). Loneliness: Human Nature and the Need for Social Connection. New York, NY: WW Norton & Company.
Cacioppo, S., Balogh, S., and Cacioppo, J. T. (2015). Implicit attention to negative social, in contrast to nonsocial, words in the Stroop task differs between individuals high and low in loneliness: evidence from event-related brain microstates. Cortex 70, 213–233. doi: 10.1016/j.cortex.2015.05.032
Carter, C. S., Lederhendler, I. I., and Kirkpatrick, B. (eds) (1999). The Integrative Neurobiology of Affiliation. Cambridge, MA: MIT Press.
Caruana, F. (2017). Laughter as a neurochemical mechanism aimed at reinforcing social bonds: integrating evidence from opioidergic activity and brain stimulation. J. Neurosci. 37, 8581–8582. doi: 10.1523/JNEUROSCI.1589-17.2017
Cavanaugh, J., Mustoe, A., and French, J. A. (2018). Oxytocin regulates reunion affiliation with a pairmate following social separation in marmosets. Am. J. Primatol. 80:e22750. doi: 10.1002/ajp.22750
Che, X., Cash, R., Chung, S., Fitzgerald, P. B., and Fitzgibbon, B. M. (2018). Investigating the influence of social support on experimental pain and related physiological arousal: a systematic review and meta-analysis. Neurosci. Biobehav. Rev. 92, 437–452. doi: 10.1016/j.neubiorev.2018.07.005
Chernoff, R., Combs-Orme, T., Risley-Curtiss, C., and Heisler, A. (1994). Assessing the health status of children entering foster care. Pediatrics 93, 594–601. doi: 10.1542/peds.93.4.594
Cho, J. R., Treweek, J. B., Robinson, J. E., Xiao, C., Bremner, L. R., Greenbaum, A., et al. (2017). Dorsal raphe dopamine neurons modulate arousal and promote wakefulness by salient stimuli. Neuron 94, 1205–1219. doi: 10.1016/j.neuron.2017.05.020
Coe, C. L., Franklin, D., Smith, E. R., and Levine, S. (1982). Hormonal responses accompanying fear and agitation in the squirrel monkey. Physiol. Behav. 29, 1051–1057. doi: 10.1016/0031-9384(82)90297-9
Collins, W. A., and Laursen, B. (eds) (1999). Relationships as Developmental Contexts: The Minnesota Symposia on Child Psychology, Vol. 30. Hove: Psychology Press.
Collins, W. A., Welsh, D. P., and Furman, W. (2009). Adolescent romantic relationships. Annu. Rev. Psychol. 60, 631–652.
Cooper, M. A., and Huhman, K. L. (2007). Corticotropin-releasing factor receptors in the dorsal raphe nucleus modulate social behavior in Syrian hamsters. Psychopharmacology 194, 297–307. doi: 10.1007/s00213-007-0849-1
Dabrowska, J., Hazra, R., Ahern, T. H., Guo, J. D., McDonald, A. J., Mascagni, F., et al. (2011). Neuroanatomical evidence for reciprocal regulation of the corticotrophin-releasing factor and oxytocin systems in the hypothalamus and the bed nucleus of the stria terminalis of the rat: implications for balancing stress and affect. Psychoneuroendocrinology 36, 1312–1326. doi: 10.1016/j.psyneuen.2011.03.003
Davidson, R. J., and Irwin, W. (1999). The functional neuroanatomy of emotion and affective style. Trends Cogn. Sci. 3, 11–21. doi: 10.1016/s1364-6613(98)01265-0
Davis, E. P., Stout, S. A., Molet, J., Vegetabile, B., Glynn, L. M., Sandman, C. A., et al. (2017). Exposure to unpredictable maternal sensory signals influences cognitive development across species. Proc. Natl. Acad. Sci. U.S.A. 114, 10390–10395. doi: 10.1073/pnas.1703444114
de Moura Oliveira, V. E., Neumann, I. D., and de Jong, T. R. (2019). Post-weaning social isolation exacerbates aggression in both sexes and affects the vasopressin and oxytocin system in a sex-specific manner. Neuropharmacology 156:107504. doi: 10.1016/j.neuropharm.2019.01.019
Decavel, C., Geffard, M., and Calas, A. (1987). Comparative study of dopamine-and noradrenaline-immunoreactive terminals in the paraventricular and supraoptic nuclei of the rat. Neurosci. Lett. 77, 149–154. doi: 10.1016/0304-3940(87)90577-5
Demarchi, L., Pawluski, J. L., and Bosch, O. J. (2021). The brain oxytocin and corticotropin-releasing factor systems in grieving mothers: what we know and what we need to learn. Peptides 143:170593. doi: 10.1016/j.peptides.2021.170593
DeVries, A. C., DeVries, M. B., Taymans, S., and Carter, C. S. (1995). Modulation of pair bonding in female prairie voles (Microtus ochrogaster) by corticosterone. Proc. Natl. Acad. Sci. U.S.A. 92, 7744–7748. doi: 10.1073/pnas.92.17.7744
DeVries, A. C., DeVries, M. B., Taymans, S. E., and Carter, C. S. (1996). The effects of stress on social preferences are sexually dimorphic in prairie voles. Proc. Natl. Acad. Sci. U.S.A. 93, 11980–11984. doi: 10.1073/pnas.93.21.11980
DeVries, A. C., Guptaa, T., Cardillo, S., Cho, M., and Carter, C. S. (2002). Corticotropin-releasing factor induces social preferences in male prairie voles. Psychoneuroendocrinology 27, 705–714. doi: 10.1016/s0306-4530(01)00073-7
DeWall, C. N., and Richman, S. B. (2011). Social exclusion and the desire to reconnect. Soc. Pers. Psychol. Compass 5, 919–932.
Doane, L. D., and Adam, E. K. (2010). Loneliness and cortisol: momentary, day-to-day, and trait associations. Psychoneuroendocrinology 35, 430–441. doi: 10.1016/j.psyneuen.2009.08.005
Dölen, G., Darvishzadeh, A., Huang, K. W., and Malenka, R. C. (2013). Social reward requires coordinated activity of nucleus accumbens oxytocin and serotonin. Nature 501, 179–184. doi: 10.1038/nature12518
Domes, G., Heinrichs, M., Gläscher, J., Büchel, C., Braus, D. F., and Herpertz, S. C. (2007). Oxytocin attenuates amygdala responses to emotional faces regardless of valence. Biol. Psychiatry 62, 1187–1190. doi: 10.1016/j.biopsych.2007.03.025
Donahue, R. J., Landino, S. M., Golden, S. A., Carroll, F. I., Russo, S. J., and Carlezon, W. A. Jr. (2015). Effects of acute and chronic social defeat stress are differentially mediated by the dynorphin/kappa-opioid receptor system. Behav. Pharmacol. 26, 654–663. doi: 10.1097/FBP.0000000000000155
Donovan, M., Liu, Y., and Wang, Z. (2018). Anxiety-like behavior and neuropeptide receptor expression in male and female prairie voles: the effects of stress and social buffering. Behav. Brain Res. 342, 70–78. doi: 10.1016/j.bbr.2018.01.015
Duck, S., Pond, K., and Leatham, G. (1994). Loneliness and the evaluation of relational events. J. Soc. Pers. Relat. 11, 253–276. doi: 10.1177/0265407594112006
Dunbar, R. I. (2010). The social role of touch in humans and primates: behavioural function and neurobiological mechanisms. Neurosci. Biobehav. Rev. 34, 260–268. doi: 10.1016/j.neubiorev.2008.07.001
Dunbar, R. I., Teasdale, B., Thompson, J., Budelmann, F., Duncan, S., van Emde Boas, E., et al. (2016). Emotional arousal when watching drama increases pain threshold and social bonding. R. Soc. Open Sci. 3:160288. doi: 10.1098/rsos.160288
Dunn, A. J., and File, S. E. (1987). Corticotropin-releasing factor has an anxiogenic action in the social interaction test. Horm. Behav. 21, 193–202. doi: 10.1016/0018-506x(87)90044-4
Duque-Wilckens, N., Steinman, M. Q., Busnelli, M., Chini, B., Yokoyama, S., Pham, M., et al. (2018). Oxytocin receptors in the anteromedial bed nucleus of the stria terminalis promote stress-induced social avoidance in female California mice. Biol. Psychiatry 83, 203–213. doi: 10.1016/j.biopsych.2017.08.024
Düzel, S., Drewelies, J., Gerstorf, D., Demuth, I., Steinhagen-Thiessen, E., Lindenberger, U., et al. (2019). Structural brain correlates of loneliness among older adults. Sci. Rep. 9:13569. doi: 10.1038/s41598-019-49888-2
Dykstra, P. A., Van Tilburg, T. G., and Gierveld, J. D. J. (2005). Changes in older adult loneliness: results from a seven-year longitudinal study. Res. Aging 27, 725–747.
Ebisch, S. J., Ferri, F., Salone, A., Perrucci, M. G., D’Amico, L., Ferro, F. M., et al. (2011). Differential involvement of somatosensory and interoceptive cortices during the observation of affective touch. J. Cogn. Neurosci. 23, 1808–1822. doi: 10.1162/jocn.2010.21551
Edwards, K. A., Madden, A. M., and Zup, S. L. (2018). Serotonin receptor regulation as a potential mechanism for sexually dimorphic oxytocin dysregulation in a model of autism. Brain Res. 1701, 85–92. doi: 10.1016/j.brainres.2018.07.020
Eisenberger, N. (2008). Understanding the moderators of physical and emotional pain: a neural systems-based approach. Psychol. Inq. 19, 189–195. doi: 10.1080/10478400802608855
Eisenberger, N. I., Lieberman, M. D., and Williams, K. D. (2003). Does rejection hurt? An fMRI study of social exclusion. Science 302, 290–292. doi: 10.1126/science.1089134
Eisenberger, N. I., Way, B. M., Taylor, S. E., Welch, W. T., and Lieberman, M. D. (2007). Understanding genetic risk for aggression: clues from the brain’s response to social exclusion. Biol. Psychiatry 61, 1100–1108. doi: 10.1016/j.biopsych.2006.08.007
Fabre-Nys, C., Meller, R. E., and Keverne, E. B. (1982). Opiate antagonists stimulate affiliative behaviour in monkeys. Pharmacol. Biochem. Behav. 16, 653–659. doi: 10.1016/0091-3057(82)90432-4
Faraji, J., Soltanpour, N., Lotfi, H., Moeeini, R., Moharreri, A. R., Roudaki, S., et al. (2017). Lack of social support raises stress vulnerability in rats with a history of ancestral stress. Sci. Rep. 7:5277. doi: 10.1038/s41598-017-05440-8
Feldman, R., Granat, A. D. I., Pariente, C., Kanety, H., Kuint, J., and Gilboa-Schechtman, E. (2009). Maternal depression and anxiety across the postpartum year and infant social engagement, fear regulation, and stress reactivity. J. Am. Acad. Child Adolesc. Psychiatry 48, 919–927. doi: 10.1097/CHI.0b013e3181b21651
Flora, J., and Segrin, C. (2000). Relationship development in dating couples: implications for relational satisfaction and loneliness. J. Soc. Pers. Relat. 17, 811–825. doi: 10.1177/0265407500176006
Floresco, S. B. (2015). The nucleus accumbens: an interface between cognition, emotion, and action. Annu. Rev. Psychol. 66, 25–52. doi: 10.1146/annurev-psych-010213-115159
French, J. A., Fite, J. E., Jensen, H., Oparowski, K., Rukstalis, M. R., Fix, H., et al. (2007). Treatment with CRH-1 antagonist antalarmin reduces behavioral and endocrine responses to social stressors in marmosets (Callithrix kuhlii). Am. J. Primatol. 69, 877–889. doi: 10.1002/ajp.20385
Fried, E. I., Bockting, C., Arjadi, R., Borsboom, D., Amshoff, M., Cramer, A. O., et al. (2015). From loss to loneliness: the relationship between bereavement and depressive symptoms. J. Abnorm. Psychol. 124, 256–265. doi: 10.1037/abn0000028
Fujisaki, M., Nakamura, A., Muroi, Y., and Ishii, T. (2020). Oxytocin in the dorsal raphe nucleus antagonizes the inhibition of maternal care induced by food deprivation. Horm. Behav. 124:104773. doi: 10.1016/j.yhbeh.2020.104773
Gable, S. L. (2006). Approach and avoidance social motives and goals. J. Pers. 74, 175–222. doi: 10.1111/j.1467-6494.2005.00373.x
Gamer, M., Zurowski, B., and Büchel, C. (2010). Different amygdala subregions mediate valence-related and attentional effects of oxytocin in humans. Proc. Natl. Acad. Sci. U.S.A. 107, 9400–9405. doi: 10.1073/pnas.1000985107
Gao, W., Lin, W., Chen, Y., Gerig, G., Smith, J. K., Jewells, V., et al. (2009). Temporal and spatial development of axonal maturation and myelination of white matter in the developing brain. Am. J. Neuroradiol. 30, 290–296. doi: 10.3174/ajnr.A1363
Gardner, W. L., Pickett, C. L., Jefferis, V., and Knowles, M. (2005). On the outside looking in: loneliness and social monitoring. Pers. Soc. Psychol. Bull. 31, 1549–1560. doi: 10.1177/0146167205277208
Gentsch, C., Lichtsteiner, M., Frischknecht, H. R., Feer, H., and Siegfried, B. (1988). Isolation-induced locomotor hyperactivity and hypoalgesia in rats are prevented by handling and reversed by resocialization. Physiol. Behav. 43, 13–16. doi: 10.1016/0031-9384(88)90091-1
Grippo, A. J., Gerena, D., Huang, J., Kumar, N., Shah, M., Ughreja, R., et al. (2007a). Social isolation induces behavioral and neuroendocrine disturbances relevant to depression in female and male prairie voles. Psychoneuroendocrinology 32, 966–980. doi: 10.1016/j.psyneuen.2007.07.004
Grippo, A. J., Lamb, D. G., Carter, C. S., and Porges, S. W. (2007b). Social isolation disrupts autonomic regulation of the heart and influences negative affective behaviors. Biol. Psychiatry 62, 1162–1170. doi: 10.1016/j.biopsych.2007.04.011
Grippo, A. J., McNeal, N., Normann, M. C., Colburn, W., Dagner, A., and Woodbury, M. (2021). Behavioral and neuroendocrine consequences of disrupting a long-term monogamous social bond in aging prairie voles. Stress 24, 239–250. doi: 10.1080/10253890.2020.1812058
Grippo, A. J., Trahanas, D. M., Zimmerman, R. R. II, Porges, S. W., and Carter, C. S. (2009). Oxytocin protects against negative behavioral and autonomic consequences of long-term social isolation. Psychoneuroendocrinology 34, 1542–1553. doi: 10.1016/j.psyneuen.2009.05.017
Gu, X., Liu, X., Van Dam, N. T., Hof, P. R., and Fan, J. (2013). Cognition–emotion integration in the anterior insular cortex. Cereb. Cortex 23, 20–27. doi: 10.1093/cercor/bhr367
Gündel, H., O’Connor, M. F., Littrell, L., Fort, C., and Lane, R. D. (2003). Functional neuroanatomy of grief: an FMRI study. Am. J. Psychiatry 160, 1946–1953. doi: 10.1176/appi.ajp.160.11.1946
Haj-Mirzaian, A., Nikbakhsh, R., Ramezanzadeh, K., Rezaee, M., Amini-Khoei, H., Haj-Mirzaian, A., et al. (2019). Involvement of opioid system in behavioral despair induced by social isolation stress in mice. Biomed. Pharmacother. 109, 938–944. doi: 10.1016/j.biopha.2018.10.144
Hall, F. S., Wilkinson, L. S., Humby, T., Inglis, W., Kendall, D. A., Marsden, C. A., et al. (1998). Isolation rearing in rats: pre-and postsynaptic changes in striatal dopaminergic systems. Pharmacol. Biochem. Behav. 59, 859–872. doi: 10.1016/s0091-3057(97)00510-8
Han, R. T., Kim, Y. B., Park, E. H., Kim, J. Y., Ryu, C., Kim, H. Y., et al. (2018). Long-term isolation elicits depression and anxiety-related behaviors by reducing oxytocin-induced GABAergic transmission in central amygdala. Front. Mol. Neurosci. 11:246. doi: 10.3389/fnmol.2018.00246
Harbert, K. J., Pellegrini, M., Gordon, K. M., and Donaldson, Z. R. (2020). How prior pair-bonding experience affects future bonding behavior in monogamous prairie voles. Horm. Behav. 126:104847. doi: 10.1016/j.yhbeh.2020.104847
Harlow, H. F., and Suomi, S. J. (1971). Social recovery by isolation-reared monkeys. Proc. Natl. Acad. Sci. U.S.A. 68, 1534–1538. doi: 10.1073/pnas.68.7.1534
Hartup, W. W., and Stevens, N. (1999). Friendships and adaptation across the life span. Curr. Dir. Psychol. Sci. 8, 76–79.
Hasue, R. H., and Shammah-Lagnado, S. J. (2002). Origin of the dopaminergic innervation of the central extended amygdala and accumbens shell: a combined retrograde tracing and immunohistochemical study in the rat. J. Comp. Neurol. 454, 15–33. doi: 10.1002/cne.10420
Hawkley, L. C., Hughes, M. E., Waite, L. J., Masi, C. M., Thisted, R. A., and Cacioppo, J. T. (2008). From social structural factors to perceptions of relationship quality and loneliness: the Chicago health, aging, and social relations study. J. Gerontol. Ser. B Psychol. Sci. Soc. Sci. 63, S375–S384. doi: 10.1093/geronb/63.6.s375
Hawkley, L. C., Preacher, K. J., and Cacioppo, J. T. (2007). “Multilevel modeling of social interactions and mood in lonely and socially connected individuals: the MacArthur social neuroscience studies,” in Oxford Handbook of Methods in Positive Psychology, eds A. D. Ong and M. H. M. van Dulmen (New York, NY: Oxford University Press), 559–575.
Heinrichs, M., Baumgartner, T., Kirschbaum, C., and Ehlert, U. (2003). Social support and oxytocin interact to suppress cortisol and subjective responses to psychosocial stress. Biol. Psychiatry 54, 1389–1398. doi: 10.1016/s0006-3223(03)00465-7
Hellemans, K. G., Benge, L. C., and Olmstead, M. C. (2004). Adolescent enrichment partially reverses the social isolation syndrome. Dev. Brain Res. 150, 103–115. doi: 10.1016/j.devbrainres.2004.03.003
Hensley, P. L., Slonimski, C. K., Uhlenhuth, E. H., and Clayton, P. J. (2009). Escitalopram: an open-label study of bereavement-related depression and grief. J. Affect. Disord. 113, 142–149. doi: 10.1016/j.jad.2008.05.016
Herman, B. H., and Panksepp, J. (1978). Effects of morphine and naloxone on separation distress and approach attachment: evidence for opiate mediation of social affect. Pharmacol. Biochem. Behav. 9, 213–220. doi: 10.1016/0091-3057(78)90167-3
Hickin, N., Käll, A., Shafran, R., Sutcliffe, S., Manzotti, G., and Langan, D. (2021). The effectiveness of psychological interventions for loneliness: a systematic review and meta-analysis. Clin. Psychol. Rev. 88:102066. doi: 10.1016/j.cpr.2021.102066
Hinde, K., Muth, C., Maninger, N., Ragen, B. J., Larke, R. H., Jarcho, M. R., et al. (2016). Challenges to the pair bond: neural and hormonal effects of separation and reunion in a monogamous primate. Front. Behav. Neurosci. 10:221. doi: 10.3389/fnbeh.2016.00221
Holstege, J. C., Dijken, H. V., Buijs, R. M., Goedknegt, H., Gosens, T., and Bongers, C. M. H. (1996). Distribution of dopamine immunoreactivity in the rat, cat, and monkey spinal cord. J. Comp. Neurol. 376, 631–652. doi: 10.1002/(SICI)1096-9861(19961223)376:4<631::AID-CNE10>3.0.CO;2-P
Howard, S., Creaven, A. M., Hughes, B. M., O’Leary, ÉD., and James, J. E. (2017). Perceived social support predicts lower cardiovascular reactivity to stress in older adults. Biol. Psychol. 125, 70–75. doi: 10.1016/j.biopsycho.2017.02.006
Hung, L. W., Neuner, S., Polepalli, J. S., Beier, K. T., Wright, M., Walsh, J. J., et al. (2017). Gating of social reward by oxytocin in the ventral tegmental area. Science 357, 1406–1411. doi: 10.1126/science.aan4994
Hunter, A., Tussis, L., and MacBeth, A. (2017). The presence of anxiety, depression and stress in women and their partners during pregnancies following perinatal loss: a meta-analysis. J. Affect. Disord. 223, 153–164. doi: 10.1016/j.jad.2017.07.004
Hylin, M. J., Watanasriyakul, W. T., Hite, N., McNeal, N., and Grippo, A. J. (2022). Morphological changes in the basolateral amygdala and behavioral disruptions associated with social isolation. Behav. Brain Res. 416:113572. doi: 10.1016/j.bbr.2021.113572
Ikemoto, S., and Panksepp, J. (1992). The effects of early social isolation on the motivation for social play in juvenile rats. Dev. Psychobiol. 25, 261–274. doi: 10.1002/dev.420250404
Inagaki, T. K., Hazlett, L. I., and Andreescu, C. (2020). Opioids and social bonding: effect of naltrexone on feelings of social connection and ventral striatum activity to close others. J. Exp. Psychol. Gen. 149, 732–745. doi: 10.1037/xge0000674
Inagaki, T. K., Irwin, M. R., and Eisenberger, N. I. (2015). Blocking opioids attenuates physical warmth-induced feelings of social connection. Emotion 15, 494–500. doi: 10.1037/emo0000088
Inagaki, T. K., Muscatell, K. A., Moieni, M., Dutcher, J. M., Jevtic, I., Irwin, M. R., et al. (2016a). Yearning for connection? Loneliness is associated with increased ventral striatum activity to close others. Soc. Cogn. Affect. Neurosci. 11, 1096–1101. doi: 10.1093/scan/nsv076
Inagaki, T. K., Ray, L. A., Irwin, M. R., Way, B. M., and Eisenberger, N. I. (2016b). Opioids and social bonding: naltrexone reduces feelings of social connection. Soc. Cogn. Affect. Neurosci. 11, 728–735. doi: 10.1093/scan/nsw006
Jind, L., Elklit, A., and Christiansen, D. (2010). Cognitive schemata and processing among parents bereaved by infant death. J. Clin. Psychol. Med. Settings 17, 366–377. doi: 10.1007/s10880-010-9216-1
Johnson, E. O., Kamilaris, T. C., Calogero, A. E., Gold, P. W., and Chrousos, G. P. (1996). Effects of early parenting on growth and development in a small primate. Pediatr. Res. 39, 999–1005. doi: 10.1203/00006450-199606000-00012
Jones, D. C. (1992). Parental divorce, family conflict and friendship networks. J. Soc. Pers. Relat. 9, 219–235.
Jones, G. H. (1992). Social isolation and individual differences: behavioural and dopaminergic responses to psychomotor stimulants. Clin. Neuropharmacol. 15(Pt A), 253A–254A. doi: 10.1097/00002826-199201001-00132
Kalyani, M., Callahan, P., Janik, J. M., and Shi, H. (2017). Effects of pup separation on stress response in postpartum female rats. Int. J. Mol. Sci. 18:1370. doi: 10.3390/ijms18071370
Kaneda, Y., Kawata, A., Suzuki, K., Matsunaga, D., Yasumatsu, M., and Ishiwata, T. (2021). Comparison of neurotransmitter levels, physiological conditions, and emotional behavior between isolation-housed rats with group-housed rats. Dev. Psychobiol. 63, 452–460. doi: 10.1002/dev.22036
Karkhanis, A. N., Leach, A. C., Yorgason, J. T., Uneri, A., Barth, S., Niere, F., et al. (2018). Chronic social isolation stress during peri-adolescence alters presynaptic dopamine terminal dynamics via augmentation in accumbal dopamine availability. ACS Chem. Neurosci. 10, 2033–2044. doi: 10.1021/acschemneuro.8b00360
Karkhanis, A. N., Locke, J. L., McCool, B. A., Weiner, J. L., and Jones, S. R. (2014). Social isolation rearing increases nucleus accumbens dopamine and norepinephrine responses to acute ethanol in adulthood. Alcohol. Clin. Exp. Res. 38, 2770–2779. doi: 10.1111/acer.12555
Karkhanis, A. N., Rose, J. H., Weiner, J. L., and Jones, S. R. (2016). Early-life social isolation stress increases kappa opioid receptor responsiveness and downregulates the dopamine system. Neuropsychopharmacology 41, 2263–2274. doi: 10.1038/npp.2016.21
Keefe, K., and Berndt, T. J. (1996). Relations of friendship quality to self-esteem in early adolescence. J. Early Adolesc. 16, 110–129.
Ketchesin, K. D., Stinnett, G. S., and Seasholtz, A. F. (2017). Corticotropin-releasing hormone-binding protein and stress: from invertebrates to humans. Stress 20, 449–464. doi: 10.1080/10253890.2017.1322575
Keverne, E. B., Martensz, N. D., and Tuite, B. (1989). Beta-endorphin concentrations in cerebrospinal fluid of monkeys are influenced by grooming relationships. Psychoneuroendocrinology 14, 155–161. doi: 10.1016/0306-4530(89)90065-6
Kiecolt-Glaser, J. K., Ricker, D., George, J., Messick, G., Speicher, C. E., Garner, W., et al. (1984). Urinary cortisol levels, cellular immunocompetency, and loneliness in psychiatric inpatients. Psychosom. Med. 46, 15–23. doi: 10.1097/00006842-198401000-00004
Killgore, W. D., Cloonan, S. A., Taylor, E. C., and Dailey, N. S. (2020). Loneliness: a signature mental health concern in the era of COVID-19. Psychiatry Res. 290:113117.
Kleckner, I. R., Zhang, J., Touroutoglou, A., Chanes, L., Xia, C., Simmons, W. K., et al. (2017). Evidence for a large-scale brain system supporting allostasis and interoception in humans. Nat. Hum. Behav. 1:69. doi: 10.1038/s41562-017-0069
Knobloch, H. S., Charlet, A., Hoffmann, L. C., Eliava, M., Khrulev, S., Cetin, A. H., et al. (2012). Evoked axonal oxytocin release in the central amygdala attenuates fear response. Neuron 73, 553–566. doi: 10.1016/j.neuron.2011.11.030
Kochenderfer-Ladd, B., and Wardrop, J. L. (2001). Chronicity and instability of children’s peer victimization experiences as predictors of loneliness and social satisfaction trajectories. Child Dev. 72, 134–151. doi: 10.1111/1467-8624.00270
Koeppel, C. J., Ruser, P., Kitzler, H., Hummel, T., and Croy, I. (2020). Interoceptive accuracy and its impact on neuronal responses to olfactory stimulation in the insular cortex. Hum. Brain Mapp. 41, 2898–2908. doi: 10.1002/hbm.24985
Kreicbergs, U., Valdimarsdóttir, U., Onelöv, E., Henter, J. I., and Steineck, G. (2004). Anxiety and depression in parents 4–9 years after the loss of a child owing to a malignancy: a population-based follow-up. Psychol. Med. 34, 1431–1441. doi: 10.1017/s0033291704002740
Kumazawa, T., and Perl, E. R. (1977). Primate cutaneous sensory units with unmyelinated (C) afferent fibers. J. Neurophysiol. 40, 1325–1338. doi: 10.1152/jn.1977.40.6.1325
Ladd, G. W., Kochenderfer, B. J., and Coleman, C. C. (1996). Friendship quality as a predictor of young children’s early school adjustment. Child Dev. 67, 1103–1118. doi: 10.1111/j.1467-8624.1996.tb01785.x
Lam, J. A., Murray, E. R., Yu, K. E., Ramsey, M., Nguyen, T. T., Mishra, J., et al. (2021). Neurobiology of loneliness: a systematic review. Neuropsychopharmacol. 46, 1873–1887. doi: 10.1038/s41386-021-01058-7
Land, B. B., Bruchas, M. R., Lemos, J. C., Xu, M., Melief, E. J., and Chavkin, C. (2008). The dysphoric component of stress is encoded by activation of the dynorphin κ-opioid system. J. Neurosci. 28, 407–414. doi: 10.1523/JNEUROSCI.4458-07.2008
Lander, S. S., Linder-Shacham, D., and Gaisler-Salomon, I. (2017). Differential effects of social isolation in adolescent and adult mice on behavior and cortical gene expression. Behav. Brain Res. 316, 245–254. doi: 10.1016/j.bbr.2016.09.005
Lanzi, R. G., Bert, S. C., Jacobs, B. K., and Centers for the Prevention of Child Neglect (2009). Depression among a sample of first-time adolescent and adult mothers. J. Child Adolesc. Psychiatr. Nurs. 22, 194–202. doi: 10.1111/j.1744-6171.2009.00199.x
Lapiz, M. D. S., Fulford, A., Muchimapura, S., Mason, R., Parker, T., and Marsden, C. A. (2003). Influence of postweaning social isolation in the rat on brain development, conditioned behavior, and neurotransmission. Neurosci. Behav. Physiol. 33, 13–29. doi: 10.1023/a:1021171129766
Lavenda-Grosberg, D. (2019). Brain Transcriptomic Changes Induced by Acute Social Isolation and Regrouping. Ann Arbor, MI: PQDT-Global.
Lavenda-Grosberg, D., Lalzar, M., Leser, N., Yaseen, A., Malik, A., Maroun, M., et al. (2021). Acute social isolation and regrouping cause short-and long-term molecular changes in the rat medial amygdala. Mol. Psychiatry 1–10. doi: 10.1038/s41380-021-01342-4
Layden, E. A., Cacioppo, J. T., Cacioppo, S., Cappa, S. F., Dodich, A., Falini, A., et al. (2017). Perceived social isolation is associated with altered functional connectivity in neural networks associated with tonic alertness and executive control. Neuroimage 145, 58–73. doi: 10.1016/j.neuroimage.2016.09.050
Leary, M. R., and Baumeister, R. F. (2000). “The nature and function of self-esteem: Sociometer theory,” in Advances in Experimental Social Psychology, Vol. 32, ed. M. P. Zanna (San Diego, CA: Academic Press), 1–62. doi: 10.1016/s0065-2601(00)80003-9
Lee, A., Clancy, S., and Fleming, A. S. (1999). Mother rats bar-press for pups: effects of lesions of the mpoa and limbic sites on maternal behavior and operant responding for pup-reinforcement. Behav. Brain Res. 100, 15–31. doi: 10.1016/s0166-4328(98)00109-0
Lee, C. R., Chen, A., and Tye, K. M. (2021). The neural circuitry of social homeostasis: consequences of acute versus chronic social isolation. Cell 184, 1500–1516. doi: 10.1016/j.cell.2021.02.028
Lee, C. Y. S., Goldstein, S. E., and Dik, B. J. (2018). The relational context of social support in young adults: links with stress and well-being. J. Adult Dev. 25, 25–36. doi: 10.1007/s10804-017-9271-z
Lee, P. C. (1994). “Social structure and evolution,” in Behaviour and Evolution, eds P. J. B. Slater and T. R. Halliday (Cambridge: Cambridge University Press), 266–303. doi: 10.1038/nature10149
Lee, Y., Fitz, S., Johnson, P. L., and Shekhar, A. (2008). Repeated stimulation of CRF receptors in the BNST of rats selectively induces social but not panic-like anxiety. Neuropsychopharmacology 33, 2586–2594. doi: 10.1038/sj.npp.1301674
Lee, Y., Kim, H., Kim, J. E., Park, J. Y., Choi, J., Lee, J. E., et al. (2018). Excessive D1 dopamine receptor activation in the dorsal striatum promotes autistic-like behaviors. Mol. Neurobiol. 55, 5658–5671. doi: 10.1007/s12035-017-0770-5
Leem, J. W., Willis, W. D., Weller, S. C., and Chung, J. M. (1993). Differential activation and classification of cutaneous afferents in the rat. J. Neurophysiol. 70, 2411–2424. doi: 10.1152/jn.1993.70.6.2411
Lefranc, B., Martin-Krumm, C., Aufauvre-Poupon, C., Berthail, B., and Trousselard, M. (2020). Mindfulness, interoception, and olfaction: a network approach. Brain Sci. 10:921. doi: 10.3390/brainsci10120921
Lepore, S. J., Evans, G. W., and Schneider, M. L. (1991). Dynamic role of social support in the link between chronic stress and psychological distress. J. Pers. Soc. Psychol. 61, 899–909. doi: 10.1037//0022-3514.61.6.899
Leser, N., and Wagner, S. (2015). The effects of acute social isolation on long-term social recognition memory. Neurobiol. Learn. Mem. 124, 97–103. doi: 10.1016/j.nlm.2015.07.002
Li, L. Z., and Wang, S. (2020). Prevalence and predictors of general psychiatric disorders and loneliness during COVID-19 in the United Kingdom. Psychiatry Res. 291:113267. doi: 10.1016/j.psychres.2020.113267
Lieberwirth, C., Liu, Y., Jia, X., and Wang, Z. (2012). Social isolation impairs adult neurogenesis in the limbic system and alters behaviors in female prairie voles. Horm. Behav. 62, 357–366. doi: 10.1016/j.yhbeh.2012.03.005
Lieberz, J., Shamay-Tsoory, S. G., Saporta, N., Esser, T., Kuskova, E., Stoffel-Wagner, B., et al. (2021a). Loneliness and the social brain: how perceived social isolation impairs human interactions. bioRxiv [Preprint]. doi: 10.1101/2021.03.03.433569
Lieberz, J., Shamay-Tsoory, S. G., Saporta, N., Kanterman, A., Esser, T., Kuskova, E., et al. (2021b). Behavioral and neural dissociation of social anxiety and loneliness. medRxiv [Preprint]. doi: 10.1101/2021.08.25.21262544
Liu, Y., and Wang, Z. X. (2003). Nucleus accumbens oxytocin and dopamine interact to regulate pair bond formation in female prairie voles. Neuroscience 121, 537–544. doi: 10.1016/s0306-4522(03)00555-4
Löken, L. S., Wessberg, J., McGlone, F., and Olausson, H. (2009). Coding of pleasant touch by unmyelinated afferents in humans. Nat. Neurosci. 12, 547–548. doi: 10.1038/nn.2312
Lonstein, J. S., Lévy, F., and Fleming, A. S. (2015). Common and divergent psychobiological mechanisms underlying maternal behaviors in non-human and human mammals. Horm. Behav. 73, 156–185. doi: 10.1016/j.yhbeh.2015.06.011
Lucht, M. J., Barnow, S., Sonnenfeld, C., Rosenberger, A., Grabe, H. J., Schroeder, W., et al. (2009). Associations between the oxytocin receptor gene (OXTR) and affect, loneliness and intelligence in normal subjects. Prog. Neuropsychopharmacol. Biol. Psychiatry 33, 860–866. doi: 10.1016/j.pnpbp.2009.04.004
Lundorff, M., Holmgren, H., Zachariae, R., Farver-Vestergaard, I., and O’Connor, M. (2017). Prevalence of prolonged grief disorder in adult bereavement: a systematic review and meta-analysis. J. Affect. Disord. 212, 138–149. doi: 10.1016/j.jad.2017.01.030
Machin, A. J., and Dunbar, R. I. (2011). The brain opioid theory of social attachment: a review of the evidence. Behaviour 148, 985–1025.
Mannella, F., Gurney, K., and Baldassarre, G. (2013). The nucleus accumbens as a nexus between values and goals in goal-directed behavior: a review and a new hypothesis. Front. Behav. Neurosci. 7:135. doi: 10.3389/fnbeh.2013.00135
Manninen, S., Tuominen, L., Dunbar, R. I., Karjalainen, T., Hirvonen, J., Arponen, E., et al. (2017). Social laughter triggers endogenous opioid release in humans. J. Neurosci. 37, 6125–6131. doi: 10.1523/JNEUROSCI.0688-16.2017
Margolis, E. B., and Karkhanis, A. N. (2019). Dopaminergic cellular and circuit contributions to kappa opioid receptor mediated aversion. Neurochem. Int. 129:104504. doi: 10.1016/j.neuint.2019.104504
Marini, F., Pozzato, C., Andreetta, V., Jansson, B., Arban, R., Domenici, E., et al. (2006). Single exposure to social defeat increases corticotropin-releasing factor and glucocorticoid receptor mRNA expression in rat hippocampus. Brain Res. 1067, 25–35. doi: 10.1016/j.brainres.2005.10.002
Martel, F. L., Nevison, C. M., Simpson, M. J., and Keverne, E. B. (1995). Effects of opioid receptor blockade on the social behavior of rhesus monkeys living in large family groups. Dev. Psychobiol. 28, 71–84. doi: 10.1002/dev.420280202
Masi, C. M., Chen, H. Y., Hawkley, L. C., and Cacioppo, J. T. (2011). A meta-analysis of interventions to reduce loneliness. Pers. Soc. Psychol. Rev. 15, 219–266.
Matthews, G. A., Nieh, E. H., Vander Weele, C. M., Halbert, S. A., Pradhan, R. V., Yosafat, A. S., et al. (2016). Dorsal raphe dopamine neurons represent the experience of social isolation. Cell 164, 617–631. doi: 10.1016/j.cell.2015.12.040
Matthews, G. A., and Tye, K. M. (2019). Neural mechanisms of social homeostasis. Ann. N. Y. Acad. Sci. 1457, 5–25. doi: 10.1111/nyas.14016
Mattson, B. J., Williams, S. E., Rosenblatt, J. S., and Morrell, J. I. (2003). Preferences for cocaine-or pup-associated chambers differentiates otherwise behaviorally identical postpartum maternal rats. Psychopharmacology 167, 1–8. doi: 10.1007/s00213-002-1351-4
Mayer, E. A., and Saper, C. B. (2000). Pain modulation: expectation, opioid analgesia and virtual pain. Biol. Basis Mind Body Interact. 122, 245–253. doi: 10.1016/s0079-6123(08)62143-3
McGraw, L., Székely, T., and Young, L. J. (2010). “Pair bonds and parental behaviour,” in Social Behaviour: Genes, Ecology and Evolution, eds T. Szekely, A. J. Moore, and J. Komdeur (Cambridge: Cambridge University Press), 271–301. doi: 10.1017/cbo9780511781360.023
McMahon, C. A., Barnett, B., Kowalenko, N. M., and Tennant, C. C. (2006). Maternal attachment state of mind moderates the impact of postnatal depression on infant attachment. J. Child Psychol. Psychiatry 47, 660–669. doi: 10.1111/j.1469-7610.2005.01547.x
McNeal, N., Appleton, K. M., Johnson, A. K., Scotti, M. A. L., Wardwell, J., Murphy, R., et al. (2017). The protective effects of social bonding on behavioral and pituitary-adrenal axis reactivity to chronic mild stress in prairie voles. Stress 20, 175–182. doi: 10.1080/10253890.2017.1295444
McNeal, N., Scotti, M. A. L., Wardwell, J., Chandler, D. L., Bates, S. L., LaRocca, M., et al. (2014). Disruption of social bonds induces behavioral and physiological dysregulation in male and female prairie voles. Auton. Neurosci. 180, 9–16. doi: 10.1016/j.autneu.2013.10.001
Mee, S., Bunney, B. G., Reist, C., Potkin, S. G., and Bunney, W. E. (2006). Psychological pain: a review of evidence. J. Psychiatr. Res. 40, 680–690.
Meguro, Y., Miyano, K., Hirayama, S., Yoshida, Y., Ishibashi, N., Ogino, T., et al. (2018). Neuropeptide oxytocin enhances μ opioid receptor signaling as a positive allosteric modulator. J. Pharmacol. Sci. 137, 67–75. doi: 10.1016/j.jphs.2018.04.002
Mele, A., Cabib, S., Oliverio, A., Melchiorri, P., and Puglisi-Allegra, S. (1987). Effects of corticotropin releasing factor and sauvagine on social behavior of isolated mice. Peptides 8, 935–938. doi: 10.1016/0196-9781(87)90083-0
Miyano, K., Yoshida, Y., Hirayama, S., Takahashi, H., Ono, H., Meguro, Y., et al. (2021). Oxytocin is a positive allosteric modulator of κ-opioid receptors but not δ-opioid receptors in the G protein signaling pathway. Cells 10:2651. doi: 10.3390/cells10102651
Molas, S., Zhao-Shea, R., Liu, L., DeGroot, S. R., Gardner, P. D., and Tapper, A. R. (2017). A circuit-based mechanism underlying familiarity signaling and the preference for novelty. Nat. Neurosci. 20, 1260–1268. doi: 10.1038/nn.4607
Morgan, D., Grant, K. A., Gage, H. D., Mach, R. H., Kaplan, J. R., Prioleau, O., et al. (2002). Social dominance in monkeys: dopamine D 2 receptors and cocaine self-administration. Nat. Neurosci. 5, 169–174. doi: 10.1038/nn798
Morr, M., Lieberz, J., Dobbelstein, M., Philipsen, A., Hurlemann, R., and Scheele, D. (2021). Insula reactivity mediates subjective isolation stress in alexithymia. Sci. Rep. 11:15326. doi: 10.1038/s41598-021-94799-w
Mullins, L. C., and Dugan, E. (1990). The influence of depression, and family and friendship relations, on residents’ loneliness in congregate housing. Gerontologist 30, 377–384. doi: 10.1093/geront/30.3.377
Mumtaz, F., Khan, M. I., Zubair, M., and Dehpour, A. R. (2018). Neurobiology and consequences of social isolation stress in animal model—a comprehensive review. Biomed. Pharmacother. 105, 1205–1222. doi: 10.1016/j.biopha.2018.05.086
Musardo, S., Contestabile, A., Mairesse, J., Baud, O., and Bellone, C. (2021). Oxytocin neurons mediates the effect of social isolation via the VTA circuits. bioRxiv [Preprint]. doi: 10.1101/2021.09.06.459105
Nakagawa, S., Takeuchi, H., Taki, Y., Nouchi, R., Sekiguchi, A., Kotozaki, Y., et al. (2015). White matter structures associated with loneliness in young adults. Sci. Rep. 5:17001. doi: 10.1038/srep17001
Neff, K. (2003). Self-compassion: an alternative conceptualization of a healthy attitude toward oneself. Self Identity 2, 85–101.
Neumann, I. D., Krömer, S. A., Toschi, N., and Ebner, K. (2000a). Brain oxytocin inhibits the (re) activity of the hypothalamo–pituitary–adrenal axis in male rats: involvement of hypothalamic and limbic brain regions. Regul. Pept. 96, 31–38. doi: 10.1016/s0167-0115(00)00197-x
Neumann, I. D., Wigger, A., Torner, L., Holsboer, F., and Landgraf, R. (2000b). Brain oxytocin inhibits basal and stress-induced activity of the hypothalamo-pituitary-adrenal axis in male and female rats: partial action within the paraventricular nucleus. J. Neuroendocrinol. 12, 235–243. doi: 10.1046/j.1365-2826.2000.00442.x
Norman, G. J., Cacioppo, J. T., Morris, J. S., Malarkey, W. B., Berntson, G. G., and DeVries, A. C. (2011). Oxytocin increases autonomic cardiac control: moderation by loneliness. Biol. Psychol. 86, 174–180. doi: 10.1016/j.biopsycho.2010.11.006
Nummenmaa, L., Manninen, S., Tuominen, L., Hirvonen, J., Kalliokoski, K. K., Nuutila, P., et al. (2015). Adult attachment style is associated with cerebral μ−opioid receptor availability in humans. Hum. Brain Mapp. 36, 3621–3628. doi: 10.1002/hbm.22866
Nummenmaa, L., Tuominen, L., Dunbar, R., Hirvonen, J., Manninen, S., Arponen, E., et al. (2016). Social touch modulates endogenous μ-opioid system activity in humans. Neuroimage 138, 242–247. doi: 10.1016/j.neuroimage.2016.05.063
O’Connor, M. F., Gündel, H., McRae, K., and Lane, R. D. (2007). Baseline vagal tone predicts BOLD response during elicitation of grief. Neuropsychopharmacology 32, 2184–2189. doi: 10.1038/sj.npp.1301342
O’Connor, M. F., Wellisch, D. K., Stanton, A. L., Eisenberger, N. I., Irwin, M. R., and Lieberman, M. D. (2008). Craving love? Enduring grief activates brain’s reward center. Neuroimage 42, 969–972. doi: 10.1016/j.neuroimage.2008.04.256
Oh, N. (2020). Neural Pattern Signature of Social Exclusion Predicts Loneliness in Older Adults. Master’s thesis. Seoul: Seoul National University.
Olausson, H., Wessberg, J., McGlone, F., and Vallbo, Å. (2010). The neurophysiology of unmyelinated tactile afferents. Neurosci. Biobehav. Rev. 34, 185–191. doi: 10.1016/j.neubiorev.2008.09.011
Osako, Y., Nobuhara, R., Arai, Y. C. P., Tanaka, K., Young, L. J., Nishihara, M., et al. (2018). Partner loss in monogamous rodents: modulation of pain and emotional behavior in male prairie voles. Psychosom. Med. 80, 62–68. doi: 10.1097/PSY.0000000000000524
Otero-García, M., Agustín-Pavón, C., Lanuza, E., and Martínez-García, F. (2016). Distribution of oxytocin and co-localization with arginine vasopressin in the brain of mice. Brain Struct. Funct. 221, 3445–3473. doi: 10.1007/s00429-015-1111-y
Panksepp, J., and Beatty, W. W. (1980). Social deprivation and play in rats. Behav. Neural Biol. 30, 197–206.
Panksepp, J., and Burgdorf, J. (2000). 50-kHz chirping (laughter?) in response to conditioned and unconditioned tickle-induced reward in rats: effects of social housing and genetic variables. Behav. Brain Res. 115, 25–38. doi: 10.1016/s0166-4328(00)00238-2
Panksepp, J., Herman, B., Conner, R., Bishop, P., and Scott, J. P. (1978). The biology of social attachments: opiates alleviate separation distress. Biol. Psychiatry 13, 607–618.
Panksepp, J., Najam, N., and Soares, F. (1979). Morphine reduces social cohesion in rats. Pharmacol. Biochem. Behav. 11, 131–134. doi: 10.1016/0091-3057(79)90002-9
Parker, V., and Morinan, A. (1986). The socially-isolated rat as a model for anxiety. Neuropharmacology 25, 663–664. doi: 10.1016/0028-3908(86)90224-8
Pawluski, J. L., Lieblich, S. E., and Galea, L. A. (2009). Offspring-exposure reduces depressive-like behaviour in the parturient female rat. Behav. Brain Res. 197, 55–61. doi: 10.1016/j.bbr.2008.08.001
Pearce, E., Launay, J., and Dunbar, R. I. (2015). The ice-breaker effect: singing mediates fast social bonding. R. Soc. Open Sci. 2:150221. doi: 10.1098/rsos.150221
Perkins, A. E., Varlinskaya, E. I., and Deak, T. (2019). Impact of housing conditions on social behavior, neuroimmune markers, and oxytocin receptor expression in aged male and female Fischer 344 rats. Exp. Gerontol. 123, 24–33. doi: 10.1016/j.exger.2019.05.005
Pickett, C. L., Gardner, W. L., and Knowles, M. (2004). Getting a cue: the need to belong and enhanced sensitivity to social cues. Pers. Soc. Psychol. Bull. 30, 1095–1107. doi: 10.1177/0146167203262085
Pournajafi-Nazarloo, H., Partoo, L., Sanzenbacher, L., Azizi, F., and Carter, C. S. (2007). Modulation of corticotropin-releasing hormone type 2 receptor and urocortin 1 and urocortin 2 mRNA expression in the cardiovascular system of prairie voles following acute or chronic stress. Neuroendocrinology 86, 17–25. doi: 10.1159/000103587
Pournajafi-Nazarloo, H., Partoo, L., Sanzenbacher, L., Paredes, J., Hashimoto, K., Azizi, F., et al. (2009). Stress differentially modulates mRNA expression for corticotrophin-releasing hormone receptors in hypothalamus, hippocampus and pituitary of prairie voles. Neuropeptides 43, 113–123. doi: 10.1016/j.npep.2008.12.002
Pournajafi-Nazarloo, H., Partoo, L., Yee, J., Stevenson, J., Sanzenbacher, L., Kenkel, W., et al. (2011). Effects of social isolation on mRNA expression for corticotrophin-releasing hormone receptors in prairie voles. Psychoneuroendocrinology 36, 780–789. doi: 10.1016/j.psyneuen.2010.10.015
Powell, S. B., Geyer, M. A., Preece, M. A., Pitcher, L. K., Reynolds, G. P., and Swerdlow, N. R. (2003). Dopamine depletion of the nucleus accumbens reverses isolation-induced deficits in prepulse inhibition in rats. Neuroscience 119, 233–240. doi: 10.1016/s0306-4522(03)00122-2
Pressman, S. D., Cohen, S., Miller, G. E., Barkin, A., Rabin, B. S., and Treanor, J. J. (2005). Loneliness, social network size, and immune response to influenza vaccination in college freshmen. Health Psychol. 24, 297–306. doi: 10.1037/0278-6133.24.3.297
Qualter, P., Brown, S. L., Rotenberg, K. J., Vanhalst, J., Harris, R. A., Goossens, L., et al. (2013). Trajectories of loneliness during childhood and adolescence: predictors and health outcomes. J. Adolesc. 36, 1283–1293. doi: 10.1016/j.adolescence.2013.01.005
Quattrocki, E., and Friston, K. (2014). Autism, oxytocin and interoception. Neurosci. Biobehav. Rev. 47, 410–430.
Raby, K. L., Roisman, G. I., Simpson, J. A., Collins, W. A., and Steele, R. D. (2015). Greater maternal insensitivity in childhood predicts greater electrodermal reactivity during conflict discussions with romantic partners in adulthood. Psychol. Sci. 26, 348–353. doi: 10.1177/0956797614563340
Rainnie, D. G., Bergeron, R., Sajdyk, T. J., Patil, M., Gehlert, D. R., and Shekhar, A. (2004). Corticotrophin releasing factor-induced synaptic plasticity in the amygdala translates stress into emotional disorders. J. Neurosci. 24, 3471–3479. doi: 10.1523/JNEUROSCI.5740-03.2004
Raymond, J. M., and Sheppard, K. (2017). Effects of peer mentoring on nursing students’ perceived stress, sense of belonging, self-efficacy and loneliness. J. Nurs. Educ. Pract. 8:16. doi: 10.5430/jnep.v8n1p16
Rees, W. D., and Lutkins, S. G. (1967). Mortality of bereavement. Br. Med. J. 4, 13–16. doi: 10.4324/9781410606143-6
Reimers, M., Schwarzenberger, F., and Preuschoft, S. (2007). Rehabilitation of research chimpanzees: stress and coping after long-term isolation. Horm. Behav. 51, 428–435. doi: 10.1016/j.yhbeh.2006.12.011
Rieger, N. S., Varela, J. A., Ng, A., Granata, L., Djerdjaj, A., Brenhouse, H. C., et al. (2021). Insular cortex corticotropin-releasing factor integrates stress signaling with social decision making. bioRxiv [Preprint]. doi: 10.1101/2021.03.23.436680
Rilling, J. K., Chen, X., Chen, X., and Haroon, E. (2018). Intranasal oxytocin modulates neural functional connectivity during human social interaction. Am. J. Primatol. 80:e22740. doi: 10.1002/ajp.22740
Rincón-Cortés, M., and Grace, A. A. (2021). Early pup removal leads to social dysfunction and dopamine deficit in late postpartum rats: prevention by social support. Front. Glob. Womens Health 2:694808. doi: 10.3389/fgwh.2021.694808
Roberts, M. H., Klatzkin, R. R., and Mechlin, B. (2015). Social support attenuates physiological stress responses and experimental pain sensitivity to cold pressor pain. Ann. Behav. Med. 49, 557–569. doi: 10.1007/s12160-015-9686-3
Robinson, D. L., Zitzman, D. L., and Williams, S. K. (2011). Mesolimbic dopamine transients in motivated behaviors: focus on maternal behavior. Front. Psychiatry 2:23. doi: 10.3389/fpsyt.2011.00023
Rogers, F. D., and Bales, K. L. (2020). Revisiting paternal absence: female alloparental replacement of fathers recovers partner preference formation in female, but not male prairie voles (Microtus ochrogaster). Dev. Psychobiol. 62, 573–590. doi: 10.1002/dev.21943
Rogers-Carter, M. M., Varela, J. A., Gribbons, K. B., Pierce, A. F., McGoey, M. T., Ritchey, M., et al. (2018). Insular cortex mediates approach and avoidance responses to social affective stimuli. Nat. Neurosci. 21, 404–414. doi: 10.1038/s41593-018-0071-y
Romero-Fernandez, W., Borroto-Escuela, D. O., Agnati, L. F., and Fuxe, K. (2013). Evidence for the existence of dopamine d2-oxytocin receptor heteromers in the ventral and dorsal striatum with facilitatory receptor–receptor interactions. Mol. Psychiatry 18, 849–850. doi: 10.1038/mp.2012.103
Ross, A. P., McCann, K. E., Larkin, T. E., Song, Z., Grieb, Z. A., Huhman, K. L., et al. (2019). Sex-dependent effects of social isolation on the regulation of arginine-vasopressin (AVP) V1a, oxytocin (OT) and serotonin (5HT) 1a receptor binding and aggression. Horm. Behav. 116:104578. doi: 10.1016/j.yhbeh.2019.104578
Rukstalis, M., and French, J. A. (2005). Vocal buffering of the stress response: exposure to conspecific vocalizations moderates urinary cortisol excretion in isolated marmosets. Horm. Behav. 47, 1–7. doi: 10.1016/j.yhbeh.2004.09.004
Sadino, J. M., Bradeen, X. G., Kelly, C. J., Walker, D. M., and Donaldson, Z. R. (2021). Prolonged partner separation erodes nucleus accumbens transcriptional signatures of pair bonding in male prairie voles. bioRxiv [Preprint]. doi: 10.1101/2021.07.14.452355
Sarason, B. R., Pierce, G. R., Shearin, E. N., Sarason, I. G., Waltz, J. A., and Poppe, L. (1991). Perceived social support and working models of self and actual others. J. Pers. Soc. Psychol. 60, 273–287.
Schneck, N., Tu, T., Bonanno, G. A., Shear, M. K., Sajda, P., and Mann, J. J. (2019a). Self-generated unconscious processing of loss linked to less severe grieving. Biol. Psychiatry 4, 271–279. doi: 10.1016/j.bpsc.2018.08.003
Schneck, N., Tu, T., Haufe, S., Bonanno, G. A., GalfaIvy, H., Ochsner, K. N., et al. (2019b). Ongoing monitoring of mindwandering in avoidant grief through cortico-basal-ganglia interactions. Soc. Cogn. Affect. Neurosci. 14, 163–172. doi: 10.1093/scan/nsy114
Sekowski, M., and Prigerson, H. G. (2021). Conflicted or close: which relationships to the deceased are associated with loss-related psychopathology? Br. J. Clin. Psychol. 1–17. doi: 10.1111/bjc.12344
Shahar-Gold, H., Gur, R., and Wagner, S. (2013). Rapid and reversible impairments of short-and long-term social recognition memory are caused by acute isolation of adult rats via distinct mechanisms. PLoS One 8:e65085. doi: 10.1371/journal.pone.0065085
Shahrokh, D. K., Zhang, T. Y., Diorio, J., Gratton, A., and Meaney, M. J. (2010). Oxytocin-dopamine interactions mediate variations in maternal behavior in the rat. Endocrinology 151, 2276–2286. doi: 10.1210/en.2009-1271
Shamay-Tsoory, S. G., and Abu-Akel, A. (2016). The social salience hypothesis of oxytocin. Biol. Psychiatry 79, 194–202.
Sharma, N., Pokharel, S. S., Kohshima, S., and Sukumar, R. (2020). Behavioural responses of free-ranging Asian elephants (Elephas maximus) towards dying and dead conspecifics. Primates 61, 129–138. doi: 10.1007/s10329-019-00739-8
Shipp, S., Adams, R. A., and Friston, K. J. (2013). Reflections on agranular architecture: predictive coding in the motor cortex. Trends Neurosci. 36, 706–716. doi: 10.1016/j.tins.2013.09.004
Smigelsky, M. A., Bottomley, J. S., Relyea, G., and Neimeyer, R. A. (2019). Investigating risk for grief severity: attachment to the deceased and relationship quality. Death Stud. 44, 402–411. doi: 10.1080/07481187.2018.1548539
Smith, A. S., Tabbaa, M., Lei, K., Eastham, P., Butler, M. J., Linton, L., et al. (2016). Local oxytocin tempers anxiety by activating GABAA receptors in the hypothalamic paraventricular nucleus. Psychoneuroendocrinology 63, 50–58. doi: 10.1016/j.psyneuen.2015.09.017
Smith, A. S., and Wang, Z. (2014). Hypothalamic oxytocin mediates social buffering of the stress response. Biol. Psychiatry 76, 281–288. doi: 10.1016/j.biopsych.2013.09.017
Spiga, F., Lightman, S. L., Shekhar, A., and Lowry, C. A. (2006). Injections of urocortin 1 into the basolateral amygdala induce anxiety-like behavior and c-Fos expression in brainstem serotonergic neurons. Neuroscience 138, 1265–1276. doi: 10.1016/j.neuroscience.2005.12.051
Sridharan, D., Levitin, D. J., and Menon, V. (2008). A critical role for the right fronto-insular cortex in switching between central-executive and default-mode networks. Proc. Natl. Acad. Sci. U.S.A. 105, 12569–12574. doi: 10.1073/pnas.0800005105
Stanton, M. E., Patterson, J. M., and Levine, S. (1985). Social influences on conditioned cortisol secretion in the squirrel monkey. Psychoneuroendocrinology 10, 125–134. doi: 10.1016/0306-4530(85)90050-2
Steptoe, A., Owen, N., Kunz-Ebrecht, S. R., and Brydon, L. (2004). Loneliness and neuroendocrine, cardiovascular, and inflammatory stress responses in middle-aged men and women. Psychoneuroendocrinology 29, 593–611. doi: 10.1016/S0306-4530(03)00086-6
Stevenson, J. R., McMahon, E. K., Boner, W., and Haussmann, M. F. (2019). Oxytocin administration prevents cellular aging caused by social isolation. Psychoneuroendocrinology 103, 52–60. doi: 10.1016/j.psyneuen.2019.01.006
Stroebe, W., Stroebe, M., Abakoumkin, G., and Schut, H. (1996). The role of loneliness and social support in adjustment to loss: a test of attachment versus stress theory. J. Pers. Soc. Psychol. 70, 1241–1249. doi: 10.1037//0022-3514.70.6.1241
Sun, P., Smith, A. S., Lei, K., Liu, Y., and Wang, Z. (2014). Breaking bonds in male prairie vole: long-term effects on emotional and social behavior, physiology, and neurochemistry. Behav. Brain Res. 265, 22–31. doi: 10.1016/j.bbr.2014.02.016
Suvilehto, J. T., Glerean, E., Dunbar, R. I., Hari, R., and Nummenmaa, L. (2015). Topography of social touching depends on emotional bonds between humans. Proc. Natl. Acad. Sci. U.S.A. 112, 13811–13816.
Tabatabaei, S. A., Abro, A. H., and Klein, M. (2018). With a little help from my friends: a computational model for the role of social support in mood regulation. Cogn. Syst. Res. 47, 133–146.
Tarr, B., Launay, J., Cohen, E., and Dunbar, R. (2015). Synchrony and exertion during dance independently raise pain threshold and encourage social bonding. Biol. Lett. 11:20150767. doi: 10.1098/rsbl.2015.0767
Tarr, B., Launay, J., and Dunbar, R. I. (2016). Silent disco: dancing in synchrony leads to elevated pain thresholds and social closeness. Evol. Hum. Behav. 37, 343–349. doi: 10.1016/j.evolhumbehav.2016.02.004
Tau, G. Z., and Peterson, B. S. (2010). Normal development of brain circuits. Neuropsychopharmacology 35, 147–168.
Tian, Q. (2016). Intergeneration social support affects the subjective well-being of the elderly: mediator roles of self-esteem and loneliness. J. Health Psychol. 21, 1137–1144. doi: 10.1177/1359105314547245
Tian, Y., Liang, S., Yuan, Z., Chen, S., Xu, P., and Yao, D. (2014). White matter structure in loneliness: preliminary findings from diffusion tensor imaging. NeuroReport 25, 843–847. doi: 10.1097/WNR.0000000000000197
Tomova, L., Tye, K., and Saxe, R. (2021). The neuroscience of unmet social needs. Soc. Neurosci. 16, 221–231. doi: 10.1080/17470919.2019.1694580
Tomova, L., Wang, K. L., Thompson, T., Matthews, G. A., Takahashi, A., Tye, K. M., et al. (2020). Acute social isolation evokes midbrain craving responses similar to hunger. Nat. Neurosci. 23, 1597–1605. doi: 10.1038/s41593-020-00742-z
Turtonen, O., Saarinen, A., Nummenmaa, L., Tuominen, L., Tikka, M., Armio, R. L., et al. (2021). Adult attachment system links with brain mu opioid receptor availability in vivo. Biol. Psychiatry 6, 360–369. doi: 10.1016/j.bpsc.2020.10.013
Tye, K., Matthews, G., Lemieux, M., Brewer, E., Miranda, R., Borio, M., et al. (2021). Separable dorsal raphe dopamine projections mediate sociability and valence. Res. Square [Preprint]. doi: 10.21203/rs.3.rs-1025403/v1
Utz, R. L., Swenson, K. L., Caserta, M., Lund, D., and deVries, B. (2014). Feeling lonely versus being alone: loneliness and social support among recently bereaved persons. J. Gerontol. Ser. B Psychol. Sci. Soc. Sci. 69, 85–94. doi: 10.1093/geronb/gbt075
Valera-Marín, G., Young, L. J., Camacho, F., Paredes, R. G., Rodríguez, V. M., Díaz, N. F., et al. (2021). Raised without a father: monoparental care effects over development, sexual behavior, sexual reward, and pair bonding in prairie voles. Behav. Brain Res. 408:113264. doi: 10.1016/j.bbr.2021.113264
Vallbo, Å. B., Olausson, H., and Wessberg, J. (1999). Unmyelinated afferents constitute a second system coding tactile stimuli of the human hairy skin. J. Neurophysiol. 81, 2753–2763. doi: 10.1152/jn.1999.81.6.2753
van Haarst, A. D., Oitzl, M. S., and De Kloet, E. R. (1997). Facilitation of feedback inhibition through blockade of glucocorticoid receptors in the hippocampus. Neurochem. Res. 22, 1323–1328. doi: 10.1023/a:1022010904600
van Tilburg, T. G., Steinmetz, S., Stolte, E., van der Roest, H., and de Vries, D. H. (2021). Loneliness and mental health during the COVID-19 pandemic: a study among Dutch older adults. J. Gerontol. Ser. B 76, e249–e255. doi: 10.1093/geronb/gbaa111
Vanhalst, J., Soenens, B., Luyckx, K., Van Petegem, S., Weeks, M. S., and Asher, S. R. (2015). Why do the lonely stay lonely? Chronically lonely adolescents’ attributions and emotions in situations of social inclusion and exclusion. J. Pers. Soc. Psychol. 109, 932–948. doi: 10.1037/pspp0000051
Varty, G. B., Paulus, M. P., Braff, D. L., and Geyer, M. A. (2000). Environmental enrichment and isolation rearing in the rat: effects on locomotor behavior and startle response plasticity. Biol. Psychiatry 47, 864–873. doi: 10.1016/s0006-3223(99)00269-3
Walker, D. M., Cunningham, A. M., Gregory, J. K., and Nestler, E. J. (2019). Long-term behavioral effects of post-weaning social isolation in males and females. Front. Behav. Neurosci. 13:66. doi: 10.3389/fnbeh.2019.00066
Watson, C. F., and Matsuzawa, T. (2018). Behaviour of nonhuman primate mothers toward their dead infants: uncovering mechanisms. Philos. Trans. R. Soc. B Biol. Sci. 373:20170261.
Williamson, J. A., Oliger, C., Wheeler, A., and O’Hara, M. W. (2019). More social support is associated with more positive mood but excess support is associated with more negative mood. J. Soc. Pers. Relat. 36, 3588–3610.
Wilson, J. M., Weiss, A., and Shook, N. J. (2020). Mindfulness, self-compassion, and savoring: factors that explain the relation between perceived social support and well-being. Pers. Individ. Differ. 152:109568.
Wilsoncroft, W. E. (1969). Babies by bar-press: maternal behavior in the rat. Behav. Res. Methods Instrum. 1, 229–230.
Winslow, J. T., Noble, P. L., Lyons, C. K., Sterk, S. M., and Insel, T. R. (2003). Rearing effects on cerebrospinal fluid oxytocin concentration and social buffering in rhesus monkeys. Neuropsychopharmacology 28, 910–918. doi: 10.1038/sj.npp.1300128
Wong, N. M., Liu, H. L., Lin, C., Huang, C. M., Wai, Y. Y., Lee, S. H., et al. (2016). Loneliness in late-life depression: structural and functional connectivity during affective processing. Psychol. Med. 46, 2485–2499. doi: 10.1017/S0033291716001033
Wood, S. K., Walker, H. E., Valentino, R. J., and Bhatnagar, S. (2010). Individual differences in reactivity to social stress predict susceptibility and resilience to a depressive phenotype: role of corticotropin-releasing factor. Endocrinology 151, 1795–1805. doi: 10.1210/en.2009-1026
Yorgason, J. T., Calipari, E. S., Ferris, M. J., Karkhanis, A. N., Fordahl, S. C., Weiner, J. L., et al. (2016). Social isolation rearing increases dopamine uptake and psychostimulant potency in the striatum. Neuropharmacology 101, 471–479. doi: 10.1016/j.neuropharm.2015.10.025
Yorgason, J. T., Espana, R. A., Konstantopoulos, J. K., Weiner, J. L., and Jones, S. R. (2013). Enduring increases in anxiety-like behavior and rapid nucleus accumbens dopamine signaling in socially isolated rats. Eur. J. Neurosci. 37, 1022–1031. doi: 10.1111/ejn.12113
Younger, J., Aron, A., Parke, S., Chatterjee, N., and Mackey, S. (2010). Viewing pictures of a romantic partner reduces experimental pain: involvement of neural reward systems. PLoS One 5:e13309. doi: 10.1371/journal.pone.0013309
Zaki, J., Davis, J. I., and Ochsner, K. N. (2012). Overlapping activity in anterior insula during interoception and emotional experience. Neuroimage 62, 493–499. doi: 10.1016/j.neuroimage.2012.05.012
Zelikowsky, M., Hui, M., Karigo, T., Choe, A., Yang, B., Blanco, M. R., et al. (2018). The neuropeptide Tac2 controls a distributed brain state induced by chronic social isolation stress. Cell 173, 1265–1279. doi: 10.1016/j.cell.2018.03.037
Zisook, S., and Shear, K. (2009). Grief and bereavement: what psychiatrists need to know. World Psychiatry 8, 67–74. doi: 10.1002/j.2051-5545.2009.tb00217.x
Keywords: loneliness, isolation, loss, oxytocin, dopamine, CRH, opioids
Citation: Vitale EM and Smith AS (2022) Neurobiology of Loneliness, Isolation, and Loss: Integrating Human and Animal Perspectives. Front. Behav. Neurosci. 16:846315. doi: 10.3389/fnbeh.2022.846315
Received: 31 December 2021; Accepted: 21 February 2022;
Published: 08 April 2022.
Edited by:
Angela J. Grippo, Northern Illinois University, United StatesReviewed by:
Edward Narayan, The University of Queensland, AustraliaMillie Rincón-Cortés, The University of Texas at Dallas, United States
Copyright © 2022 Vitale and Smith. This is an open-access article distributed under the terms of the Creative Commons Attribution License (CC BY). The use, distribution or reproduction in other forums is permitted, provided the original author(s) and the copyright owner(s) are credited and that the original publication in this journal is cited, in accordance with accepted academic practice. No use, distribution or reproduction is permitted which does not comply with these terms.
*Correspondence: Erika M. Vitale, evitale@ku.edu; Adam S. Smith, adamsmith@ku.edu