Clusterin/apolipoprotein J, its isoforms and Alzheimer's disease
- 1Institute for Memory Impairment and Neurological Disorders, University of California, Irvine, Irvine, CA, United States
- 2Department of Neurobiology and Behavior, School of Biological Sciences, University of California, Irvine, Irvine, CA, United States
Introduction
Late-Onset Alzheimer's Disease (LOAD) is the most common form of Alzheimer's disease, accounting for ~95% of all AD cases (Reitz and Mayeux, 2014). It is believed to be caused by a complex interplay of genetic and environmental factors. The Apolipoprotein E (ApoE) ε4 allele is the best-known genetic risk factor for LOAD (Jiang et al., 2013), but various genome-wide association studies (GWAS) have identified many other low-penetrance alleles that also influence the risk of LOAD. One such genetic factor is the clusterin (CLU) gene, also known as apolipoprotein J (ApoJ). This gene has been found to be one of the most important genetic factors associated with an increased risk of LOAD in multiple GWAS studies (Harold et al., 2009; Lambert et al., 2009; Seshadri et al., 2010; Wightman et al., 2021).
The CLU protein is found body wide, including peripheral organs (Ahuja et al., 1996; Guo et al., 2016; Park et al., 2020), the brain (Pasinetti et al., 1994; Thambisetty et al., 2013; Moon et al., 2021), and in bodily fluids (Trougakos and Gonos, 2002) such as plasma (De Silva et al., 1990b; Martinez-Bujidos et al., 2015; Hsu et al., 2017; Liu et al., 2021), urine (Solichova et al., 2007), cerebrospinal fluid (Nilselid et al., 2006), seminal fluid (Atlas-White et al., 2000; Saewu et al., 2017), and tears (Yu et al., 2018). The functions of CLU in peripheral tissues have been well studied and include the clearance of misfolded proteins (Humphreys et al., 1999), lipid transport (Calero et al., 1999), inhibition of the complement system (Jenne and Tschopp, 1989), and the regulation of oxidative stress and cell death (Foster et al., 2019). In the brain, CLU expression is found in astrocytes (Pasinetti et al., 1994; Morgan et al., 1995; Demattos et al., 2001; Charnay et al., 2008; John Lin et al., 2017; Chen et al., 2021) and in cortical and hippocampal neurons (Figure 1; O'bryan et al., 1993; Pasinetti et al., 1994; Herring et al., 2019). The specific functions of CLU in the brain, however, are not as well-understood. Studies have shown that CLU expression is upregulated in degenerative conditions, such as AD (Calero et al., 2005; Nuutinen et al., 2009), due to cellular and oxidative stress or dysregulation of specific signaling pathways (Wong et al., 1994; Gutacker et al., 1999; Schepeler et al., 2007; Trougakos and Gonos, 2009). However, the literature provides conflicting results as to whether CLU expression improves or exacerbates cellular stress (Schreiber et al., 1993; Han et al., 2001; Imhof et al., 2006; Kim et al., 2012; Trindade et al., 2016; Troakes et al., 2017).
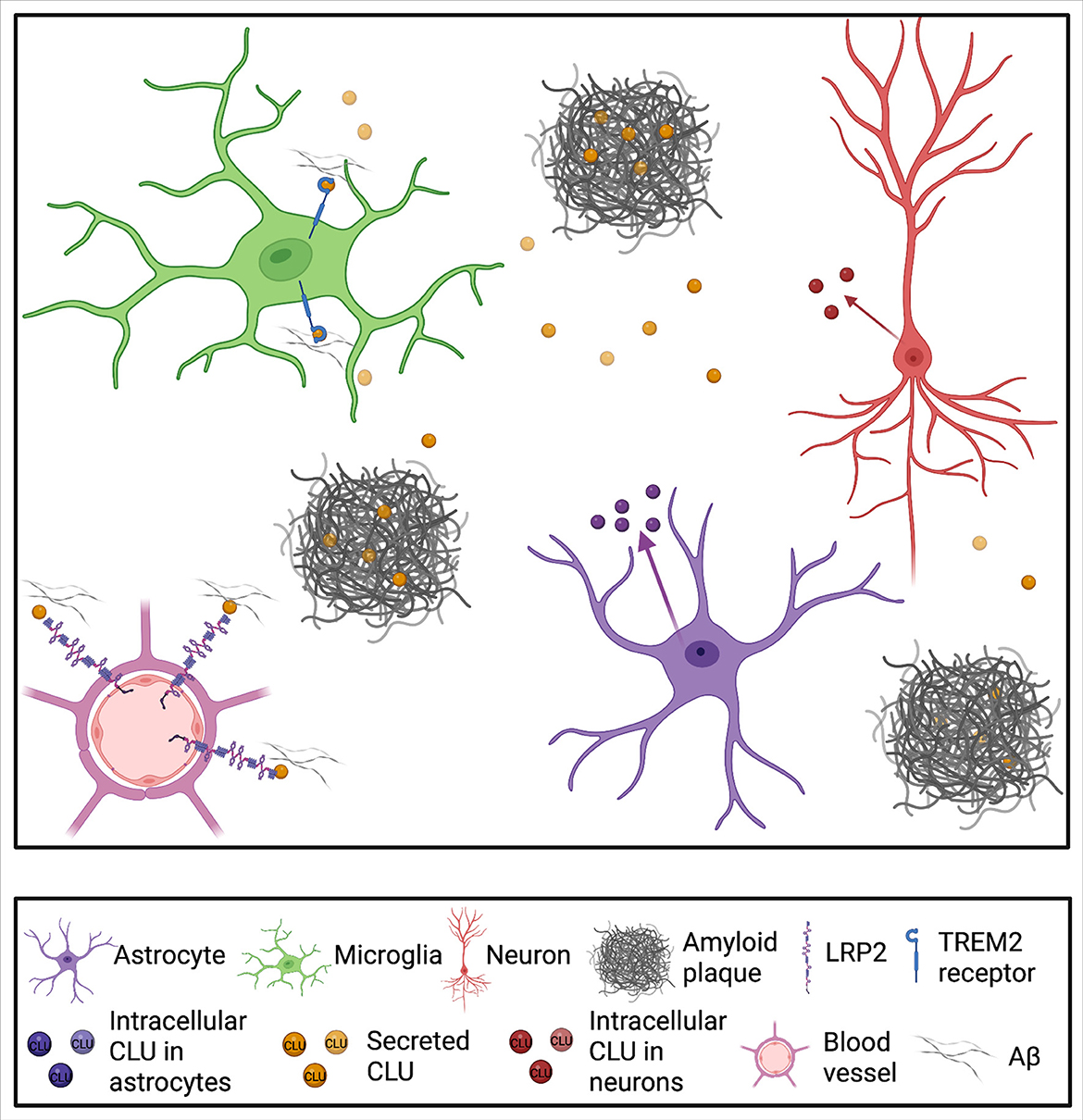
Figure 1. Clusterin (CLU) in the brain. CLU expression in the brain is found in astrocytes and neurons. CLU has multiple isoforms, including secreted and non-secreted. Secreted CLU is known to bind Aβ plaques as well as to participate in Aβ uptake by microglia via the TREM2 receptor and Aβ clearance via the brain vasculature. The figure was created with BioRender.com.
In AD, levels of CLU are increased in the brain (Mcgeer et al., 1992; Lidstrom et al., 1998) and in cerebrospinal fluid (CSF) (May et al., 1990; Bertrand et al., 1995; Miners et al., 2017). CLU has been found to bind to amyloid-beta (Aβ) and play a role in Aβ deposition as well as its clearance (Wilson and Easterbrook-Smith, 1992; Narayan et al., 2011). CLU has also been found in Aβ plaques, vessels of cerebral amyloid angiopathy (CAA; Figure 1) (Mcgeer et al., 1992; Craggs et al., 2016; Camacho et al., 2019), associated with neurofibrillary
tangles (Mcgeer et al., 1992), and to interact with modified Tau species in human AD brain tissue (Zhou et al., 2014). However, CLU may also be involved in non-Aβ pathways that could alter susceptibility to AD (Braskie et al., 2011; Erk et al., 2011; Thambisetty et al., 2013). Importantly, different single nucleotide polymorphisms (SNPs) in the CLU gene may exert their effects in combination with other genetic risk factors, such as APOE4 (Roussotte et al., 2014; Jackson et al., 2019), TREM2 (Yeh et al., 2016) and BIN1 (Zhou et al., 2014). Additionally, at least three different mRNA isoforms are produced from the CLU gene (Calero et al., 1999) and recent research suggests that different variants in the CLU gene may lead to alterations in the ratios of isoforms being produced, which in turn could affect the outcome of the disease and play a role in the development and progression of AD.
CLU isoforms
Alternative splicing has been implicated in the susceptibility of Alzheimer's disease (AD) (Raj et al., 2018) and many genes associated with AD undergo alternative splicing (Rockenstein et al., 1995; Zhou et al., 2014; Koch, 2018), CLU being one of them (Szymanski et al., 2011; Foster et al., 2019; Herring et al., 2019; Han et al., 2020). CLU consists of 11 exons (two exons are untranslated) that give rise to secreted and cytosolic isoforms through alternative splicing. Research challenges exist due to the limited characterization of CLU mRNA transcript variants present in different sexes, at different developmental timepoints, cell types, and in different brain areas in a healthy brain (De Silva et al., 1990a; Herring et al., 2019).
CLU protein exists in multiple forms, including secreted (sCLU) and non-secreted/intracellular isoforms which are targeted to at least three different compartments within the cell (cytosol, ER/mitochondria, nucleus; Herring et al., 2019). Unless specified, here we will call all non-secreted isoforms intracellular CLU (iCLU). The different forms of CLU may have distinct functions, depending on where and when they are found, as well as how long they are produced. The sCLU is produced from Exon 2 (Rizzi and Bettuzzi, 2010; Ling et al., 2012) and contains an endoplasmic reticulum (ER) signaling peptide. Similar to the small heat-shock proteins, sCLU can function as a chaperone and bind to partially unfolded proteins preventing their aggregation (Humphreys et al., 1999; Wojtas et al., 2020). (Yeh et al., 2016; Wojtas et al., 2017; Yuste-Checa et al., 2021). iCLU forms have been suggested to play a role in apoptosis (Yang et al., 2000; Debure et al., 2003; Zhang et al., 2005), DNA repair (Yang et al., 2000), transcription (Santilli et al., 2003) and microtubule organization (Kang et al., 2005). One of the iCLU is produced from Exon 3 and lacks Exon 2 (Leskov et al., 2003; Kim et al., 2012; Prochnow et al., 2013), while another iCLU isoform lacks exon 5 (Kimura and Yamamoto, 1996; Kimura et al., 1997; Leskov et al., 2003). sCLU has been suggested to promote cell survival (Trougakos and Gonos, 2009), while iCLU is associated with decreased cell growth and apoptosis (Yang et al., 2000; Scaltriti et al., 2004; Kim and Choi, 2011). The iCLU that lacks exon 2 has been termed a nuclear CLU (nCLU) and described as a cell death protein that can be found in the cytoplasm and nuclei of cells (Kimura and Yamamoto, 1996; Yang et al., 2000; Leskov et al., 2003; Prochnow et al., 2013). However, this isoform is present at very low levels (Yang et al., 2000; Prochnow et al., 2013) or, as some state, is not produced at all (Andersen et al., 2007). It is worth noting that many studies on the role of CLU isoforms have been conducted in vitro, in cancer cells, and not in the healthy brain (Leskov et al., 2003; Rodriguez-Pineiro et al., 2006; Moretti et al., 2007; Rizzi and Bettuzzi, 2010).
In the most comprehensive study of CLU isoforms so far, CLU mRNA transcripts have been characterized in the rodent brain, primary cultures of rodent and human neurons and astrocytes, and in rodent and human brain-derived cell lines (Herring et al., 2019). In the cortex, iCLU could be found in the nuclear, organelle, and cytosolic compartments of neurons, but only low levels were detected in astrocytes. Six different CLU immunoreactive bands (5 in cytosolic and 1 in nuclear fraction) were identified in primary cultures of rodent cortical neurons. Five immunoreactive bands (4 in cytosolic and 1 in nuclear fraction) were detected in astrocytes. It was concluded that two isoforms were exclusive to neurons. Three different Exon 1 variants were discovered—Exon 1A, Exon 1B, and Exon 1C. Exon 1A and Exon 1C are expressed in astrocytes and neurons, respectively, while Exon 1B mRNA transcript was detected in both cell types and, thus, two neuron-specific CLU isoforms likely originate from a neuron-specific Exon 1C variant. A novel mitochondrial CLU (mitoCLU) was identified in female adult cortical tissue, which is translated from a non-canonical start site CUG (Leucine) in Exon 3. mitoCLU is also found in human cells but is generated from an AUG and a CUG start sites located in Exon 3 (Herring et al., 2019). In light of these findings, there may be a need to re-evaluate some of the historical results.
CLU isoforms in AD
Multiple CLU SNPs, both intronic and exonic, have been associated with LOAD (Harold et al., 2009; Lambert et al., 2009; Moon et al., 2021). Interestingly, while some confer protection against AD, others have been linked to an increased AD risk [reviewed in Woody and Zhao (2016)]. Both sCLU and nCLU are upregulated in response to stress (Nizard et al., 2007) and inflammation, and CLU and CLU mRNA is altered in neurodegenerative disorders (Grewal et al., 1999; Sasaki et al., 2002; Ingram et al., 2014; Labadorf et al., 2015; Das Gupta et al., 2019; Yuste-Checa et al., 2022), including AD (Calero et al., 2005; Zhou et al., 2014; Bettens et al., 2015; Foster et al., 2019; Jackson et al., 2019). However, while sCLU is thought to be protective, iCLU isoforms were linked to cytotoxicity (Nizard et al., 2007; Prochnow et al., 2013; Yeh et al., 2016; Wojtas et al., 2017; Yuste-Checa et al., 2021). Therefore, CLU variants and other factors that modify the ratio between different isoforms could also alter the risk for LOAD and associated brain pathology through the multiple functions attributed to CLU.
The most well-known SNP is rs11136000, which is located in intron 3 and is carried by about 36% of the Caucasian population (Bertram et al., 2007; Braskie et al., 2011). The major allele, rs1113600C, is associated with reduced expression of CLU and an increased risk of AD (Ling et al., 2012; Roussotte et al., 2014; Tan et al., 2016). This allele has also been linked to faster cognitive decline (Thambisetty et al., 2013) and poorer memory scores (Pedraza et al., 2014). Additionally, research has shown that the C allele can change brain structure and network activity in young adults, suggesting that brain circuitry in early life may contribute to cognitive effects later in life (Braskie et al., 2011; Lancaster et al., 2015). In contrast, the minor allele, rs11136000T, is associated with increased CLU expression and a reduced risk of AD (Ling et al., 2012; Roussotte et al., 2014; Tan et al., 2016). Studies have also found that this allele is associated with increased nCLU expression, but not sCLU. The ratio of expressed isoforms may change depending on the CLU genotype, with both nCLU and sCLU levels increasing with AD (Ling et al., 2012).
Other SNPs have also been linked to changes in CLU protein localization, for example, rare SNPs located in exons 5 and 6 have been identified and linked to alterations in CLU isoform production in AD, such as a reduction in sCLU (Bettens et al., 2012, 2015; Han et al., 2020). Studies with Tg4510 mice, which overexpress the human mutant P301L Tau (Ramsden et al., 2005), have also shown changes in CLU expression. In these mice, sCLU was upregulated in the hippocampus at 5.5 months, but a truncated version of iCLU was increased in the hippocampus as early as 2 months (pre-tangle time point). This truncated version of iCLU was found to interact directly with Tau protein, but outside of the microtubule binding region. Interestingly, the Tg2576 mouse model of amyloidosis did not show any age-related changes in CLU isoform expression (Zhou et al., 2014) suggesting that in this study Tau rather than amyloid was driving changes in CLU isoform expression.
CLU function in AD
The availability of CLU mouse models is currently limited to two models from the Jackson Laboratory. The first model is a CLU−/− model (JAX:005642) that was developed over 20 years ago (Mclaughlin et al., 2000). The second model contains a 2kb region of human DNA sequence that spans from intron 7 to exon 9, including a human LOAD CLU risk SNP rs2279590 (JAX:037496), that we have produced as part of the MODEL-AD consortium that is currently undergoing phenotyping. Recently, it was discovered that the existing CLU−/− model is not a complete KO as the mitoCLU isoform is still present (Herring et al., 2019). In addition, a mouse with the deletion of exon 3 was created in 2021, but, except for the effect on the auditory function, has not been characterized yet or validated (Zhao et al., 2021). Given the association between CLU risk alleles and altered CLU isoform production (Foster et al., 2019; Han et al., 2020), there is a significant urgency to validate CLU functions identified with the currently available CLU−/− model in the Jackson Laboratory, and possibly to create the new CLU−/−.
The function of CLU in AD has been studied using mouse models, including the CLU−/− model and its crosses with other known AD models. Studies have shown that loss of CLU in PDAPP transgenic mice leads to a reduction in dense core plaques and neuritic dystrophy (Demattos et al., 2002), while crossing of the same CLU−/− with APP/PS1 mice increased incidence of cerebral amyloid angiopathy while also reducing dense core plaques (Wojtas et al., 2017). Notably, CLU−/− mice show impaired presynaptic function, and reduced spine density (Chen et al., 2021). CLU−/− crossed with the 5xFAD mouse model of amyloidosis led to decreased levels of soluble Aβ oligomers and amyloid plaques and an increase in synaptic proteins as well as improved scores of behavioral tests. However, these results were only seen in younger mice, suggesting CLU's role in the early stages of AD (Oh et al., 2019). However, due to incomplete KO of all CLU isoforms in the CLU−/− mice used in all of these studies, these findings need to be re-explored. Additionally, some data on CLU function is available through CLU overexpression studies, where overexpression in astrocytes of 5xFAD mice reduced amyloid pathology, neuronal toxicity, and rescued synaptic deficits (Chen et al., 2021). A recent publication also describes the effects of removing Exon 2 from CLU and shows downregulation of extracellular matrix pathways in cultured neurons (Foster et al., 2022). However, no AD-related CLU mutations have been identified in Exon 2, which harbors the ER-targeting sequence (Moon et al., 2021; Foster et al., 2022).
There are two ways that CLU has been suggested to be involved in the Aβ clearance – transvascular and microglial. Transvascular pathway encompasses Aβ clearance across the blood-brain barrier (BBB) as a free peptide and/or bound to APOE or CLU. While APOE2 and APOE3 bound Aβ is removed via endothelial low-density lipoprotein receptor-related protein 1 (LRP1), CLU facilitates Aβ clearance via endothelial low-density lipoprotein receptor-related protein 2 (LRP2) (Bell et al., 2007; Zlokovic, 2011; Zhao et al., 2015b). APOE4 cannot bind LRP1 and, thus, together with aging, leads to enhanced risk of cerebral amyloid angiopathy (CAA) (Zhao et al., 2015a,b). In 12 month APP/PS1;CLU−/− mice, significant increase in dense core Aβ plaques was observed in leptomeningeal vessels and penetrating arterioles. Accordingly, plaques were reduced in cortical and hippocampal regions indicating a shift to Aβ accumulation in the perivascular drainage pathways leading to increased CAA in the absence of CLU. This study also showed an increase in Aβ40:42 ratio due to a longer Aβ40 clearance time in mice lacking CLU (Wojtas et al., 2017). Similarly, in 12 month PDAPP;CLU−/− mice, a reduction in Aβ plaques was reported, but no changes in the total Aβ levels in the cortex or hippocampus or effect on CAA. Interestingly, authors did observe an increase in soluble Aβ in the brain (Demattos et al., 2004). It was suggested that the differences in effect on CAA may be due to the younger age that the mice develop CAA at, which is 6 and 12 months for the APP/PS1, PDAPP mice, respectively (Nelson et al., 2017). Overall, CLU plays a role in the Aβ clearance via the brain vasculature, thus, SNPs in CLU leading to changes in the levels of secreted CLU, may affect the severity of CAA observed in AD. Given that Aβ40 is the predominant Aβ species which accumulates in the vessel walls and presents as CAA (Yamada, 2015; Robert et al., 2017), lack of CLU may be responsible for the altered clearance of Aβ40 specifically and, in turn, the enhanced risk of CAA.
CLU and APOE have been identified as ligands of the triggering receptor expressed on myeloid cells 2 (TREM2) (Yeh et al., 2016). TREM2 is a receptor that is selectively expressed on microglia in the brain (Wang et al., 2015; Ulland et al., 2017; Nugent et al., 2020) and macrophages in the periphery (Chung et al., 2002), and is known to play a role in inflammatory signaling (Kobayashi et al., 2016), microglial metabolism (Ulland et al., 2017), phagocytosis (Poliani et al., 2015; Wang et al., 2015), activation (Jay et al., 2015; Wang et al., 2015), survival (Wang et al., 2015; Ulland et al., 2017; Zheng et al., 2017), and proliferation (Poliani et al., 2015). TREM2 was identified as a lipid receptor and was shown to control cholesterol and phospholipid metabolism in the brain (Wang et al., 2015; Andreone et al., 2020; Nugent et al., 2020; Li et al., 2022). Lipidated CLU can bind to Aβ, and CLU-Aβ complexes can then be taken up by microglia through binding to TREM2 (Yeh et al., 2016). This suggests that CLU may be facilitating microglial Aβ uptake through TREM2 (Figure 1), and a lack of sCLU due to changes in CLU isoform expression may affect Aβ uptake and clearance. In addition, given the role that lipid metabolism plays in AD (Zhu et al., 2019; Kao et al., 2020; Paasila et al., 2021; Turri et al., 2022), TREM2 function as a lipid metabolism regulator (Wang et al., 2015; Nugent et al., 2020; Li et al., 2022), and CLU binding lipids and cholesterol to influence their trafficking (Matukumalli et al., 2017; Foster et al., 2019), the interaction between TREM2 and CLU should be further explored as it could have a great impact on AD pathogenesis.
Conclusions
In conclusion, the role of CLU in Alzheimer's disease is complex and not fully understood. Multiple CLU isoforms have been identified, but future research is needed to understand their transcription patterns in different cell types, brain regions, during development and aging, in healthy brain and disease as well as different sexes. Moreover, it is still unclear how each identified AD-linked CLU SNP alters the ratios of translated CLU isoforms. Studies utilizing a single CLU−/− model and crossing it with other known mouse AD models have shown a range of CLU functions in the AD brain. However, these studies may need to be re-evaluated due to incomplete KO of all the CLU isoforms in this model. New and improved CLU models focusing on different CLU isoforms are needed to elucidate the specific role of CLU in healthy brain, the development and progression of AD.
Author contributions
GM wrote the first draft of the manuscript. KNG edited the manuscript. Both authors have read and approved the final manuscript for publication.
Funding
This study was supported by the Model Organism Development and Evaluation for Late-onset Alzheimer's Disease (MODEL-AD) consortium funded by the National Institute on Aging (U54 AG054349).
Conflict of interest
The authors declare that the research was conducted in the absence of any commercial or financial relationships that could be construed as a potential conflict of interest.
Publisher's note
All claims expressed in this article are solely those of the authors and do not necessarily represent those of their affiliated organizations, or those of the publisher, the editors and the reviewers. Any product that may be evaluated in this article, or claim that may be made by its manufacturer, is not guaranteed or endorsed by the publisher.
References
Ahuja, H. S., Tenniswood, M., and Zakeri, Z. F. (1996). Differential expression of clusterin in the testis and epididymis of postnatal and germ cell deficient mice. J. Androl. 17, 491–501.
Andersen, C. L., Schepeler, T., Thorsen, K., Birkenkamp-Demtroder, K., Mansilla, F., Aaltonen, L. A., et al. (2007). Clusterin expression in normal mucosa and colorectal cancer. Mol. Cell. Proteomics 6, 1039–1048. doi: 10.1074/mcp.M600261-MCP200
Andreone, B. J., Przybyla, L., Llapashtica, C., Rana, A., Davis, S. S., Van Lengerich, B., et al. (2020). Alzheimer's-associated PLCgamma2 is a signaling node required for both TREM2 function and the inflammatory response in human microglia. Nat. Neurosci. 23, 927–938. doi: 10.1038/s41593-020-0650-6
Atlas-White, M., Murphy, B. F., and Baker, H. W. (2000). Localisation of clusterin in normal human sperm by immunogold electron microscopy. Pathology 32, 258–261. doi: 10.1080/713688946
Bell, R. D., Sagare, A. P., Friedman, A. E., Bedi, G. S., Holtzman, D. M., Deane, R., et al. (2007). Transport pathways for clearance of human Alzheimer's amyloid beta-peptide and apolipoproteins E and J in the mouse central nervous system. J. Cereb. Blood Flow Metab. 27, 909–918. doi: 10.1038/sj.jcbfm.9600419
Bertram, L., Mcqueen, M. B., Mullin, K., Blacker, D., and Tanzi, R. E. (2007). Systematic meta-analyses of Alzheimer disease genetic association studies: the AlzGene database. Nat. Genet. 39, 17–23. doi: 10.1038/ng1934
Bertrand, P., Poirier, J., Oda, T., Finch, C. E., and Pasinetti, G. M. (1995). Association of apolipoprotein E genotype with brain levels of apolipoprotein E and apolipoprotein J (clusterin) in Alzheimer disease. Brain Res. Mol. Brain Res. 33, 174–178. doi: 10.1016/0169-328X(95)00097-C
Bettens, K., Brouwers, N., Engelborghs, S., Lambert, J. C., Rogaeva, E., Vandenberghe, R., et al. (2012). Both common variations and rare non-synonymous substitutions and small insertion/deletions in CLU are associated with increased Alzheimer risk. Mol. Neurodegener. 7, 3. doi: 10.1186/1750-1326-7-3
Bettens, K., Vermeulen, S., Van Cauwenberghe, C., Heeman, B., Asselbergh, B., Robberecht, C., et al. (2015). Reduced secreted clusterin as a mechanism for Alzheimer-associated CLU mutations. Mol. Neurodegener. 10, 30. doi: 10.1186/s13024-015-0024-9
Braskie, M. N., Jahanshad, N., Stein, J. L., Barysheva, M., Mcmahon, K. L., De Zubicaray, G. I., et al. (2011). Common Alzheimer's disease risk variant within the CLU gene affects white matter microstructure in young adults. J. Neurosci. 31, 6764–6770. doi: 10.1523/JNEUROSCI.5794-10.2011
Calero, M., Rostagno, A., Frangione, B., and Ghiso, J. (2005). Clusterin and Alzheimer's disease. Subcell. Biochem. 38, 273–298. doi: 10.1007/0-387-23226-5_14
Calero, M., Tokuda, T., Rostagno, A., Kumar, A., Zlokovic, B., Frangione, B., et al. (1999). Functional and structural properties of lipid-associated apolipoprotein J (clusterin). Biochem. J. 344(Pt 2), 375–383. doi: 10.1042/bj3440375
Camacho, J., Moline, T., Bonaterra-Pastra, A., Ramon, Y. C. S., Martinez-Saez, E., and Hernandez-Guillamon, M. (2019). Brain ApoA-I, ApoJ and ApoE immunodetection in cerebral amyloid angiopathy. Front. Neurol. 10, 187. doi: 10.3389/fneur.2019.00187
Charnay, Y., Imhof, A., Vallet, P. G., Hakkoum, D., Lathuiliere, A., Poku, N., et al. (2008). Clusterin expression during fetal and postnatal CNS development in mouse. Neuroscience 155, 714–724. doi: 10.1016/j.neuroscience.2008.06.022
Chen, F., Swartzlander, D. B., Ghosh, A., Fryer, J. D., Wang, B., and Zheng, H. (2021). Clusterin secreted from astrocyte promotes excitatory synaptic transmission and ameliorates Alzheimer's disease neuropathology. Mol. Neurodegener. 16, 5. doi: 10.1186/s13024-021-00426-7
Chung, D. H., Seaman, W. E., and Daws, M. R. (2002). Characterization of TREM-3, an activating receptor on mouse macrophages: definition of a family of single Ig domain receptors on mouse chromosome 17. Eur. J. Immunol. 3, 2. doi: 10.1002/1521-4141(200201)32:1andlt;59::AID-IMMU59andgt;3.0.CO;2-U
Craggs, L., Taylor, J., Slade, J. Y., Chen, A., Hagel, C., Kuhlenbaeumer, G., et al. (2016). Clusterin/Apolipoprotein J immunoreactivity is associated with white matter damage in cerebral small vessel diseases. Neuropathol. Appl. Neurobiol. 42, 194–209. doi: 10.1111/nan.12248
Das Gupta, S., Lipponen, A., Paldanius, K. M. A., Puhakka, N., and Pitkanen, A. (2019). Dynamics of clusterin protein expression in the brain and plasma following experimental traumatic brain injury. Sci. Rep. 9, 20208. doi: 10.1038/s41598-019-56683-6
De Silva, H. V., Harmony, J. A., Stuart, W. D., Gil, C. M., and Robbins, J. (1990a). Apolipoprotein J: structure and tissue distribution. Biochemistry 29, 5380–5389. doi: 10.1021/bi00474a025
De Silva, H. V., Stuart, W. D., Duvic, C. R., Wetterau, J. R., Ray, M. J., Ferguson, D. G., et al. (1990b). A 70-kDa apolipoprotein designated ApoJ is a marker for subclasses of human plasma high density lipoproteins. J. Biol. Chem. 265, 13240–13247. doi: 10.1016/S0021-9258(19)38290-0
Debure, L., Vayssiere, J. L., Rincheval, V., Loison, F., Le Drean, Y., and Michel, D. (2003). Intracellular clusterin causes juxtanuclear aggregate formation and mitochondrial alteration. J. Cell Sci. 116, 3109–3121. doi: 10.1242/jcs.00619
Demattos, R. B., Brendza, R. P., Heuser, J. E., Kierson, M., Cirrito, J. R., Fryer, J., et al. (2001). Purification and characterization of astrocyte-secreted apolipoprotein E and J-containing lipoproteins from wild-type and human apoE transgenic mice. Neurochem. Int. 39, 415–425. doi: 10.1016/S0197-0186(01)00049-3
Demattos, R. B., Cirrito, J. R., Parsadanian, M., May, P. C., O'dell, M. A., Taylor, J. W., et al. (2004). ApoE and clusterin cooperatively suppress Abeta levels and deposition: evidence that ApoE regulates extracellular Abeta metabolism in vivo. Neuron 41, 193–202. doi: 10.1016/S0896-6273(03)00850-X
Demattos, R. B., O'dell, M. A., Parsadanian, M., Taylor, J. W., Harmony, J. A., et al. (2002). Clusterin promotes amyloid plaque formation and is critical for neuritic toxicity in a mouse model of Alzheimer's disease. Proc. Natl. Acad. Sci. USA. 99, 10843–10848. doi: 10.1073/pnas.162228299
Erk, S., Meyer-Lindenberg, A., Opitz Von Boberfeld, C., Esslinger, C., Schnell, K., Kirsch, P., et al. (2011). Hippocampal function in healthy carriers of the CLU Alzheimer's disease risk variant. J. Neurosci. 31, 18180–18184. doi: 10.1523/JNEUROSCI.4960-11.2011
Foster, E. M., Dangla-Valls, A., Lovestone, S., Ribe, E. M., and Buckley, N. J. (2019). Clusterin in Alzheimer's disease: mechanisms, genetics, and lessons from other pathologies. Front. Neurosci. 13, 164. doi: 10.3389/fnins.2019.00164
Foster, E. M., Fernandes, M., Dangla-Valls, A., Hublitz, P., Pangalos, M., Lovestone, S., et al. (2022). Glycosylated clusterin species facilitate Abeta toxicity in human neurons. Sci. Rep. 12, 18639. doi: 10.1038/s41598-022-23167-z
Grewal, R. P., Morgan, T. E., and Finch, C. E. (1999). C1qB and clusterin mRNA increase in association with neurodegeneration in sporadic amyotrophic lateral sclerosis. Neurosci. Lett. 271, 65–67. doi: 10.1016/S0304-3940(99)00496-6
Guo, J., Guan, Q., Liu, X., Wang, H., Gleave, M. E., Nguan, C. Y., et al. (2016). Relationship of clusterin with renal inflammation and fibrosis after the recovery phase of ischemia-reperfusion injury. BMC Nephrol. 17, 133. doi: 10.1186/s12882-016-0348-x
Gutacker, C., Klock, G., Diel, P., and Koch-Brandt, C. (1999). Nerve growth factor and epidermal growth factor stimulate clusterin gene expression in PC12 cells. Biochem. J. 339(Pt 3), 759–766. doi: 10.1042/bj3390759
Han, B. H., Demattos, R. B., Dugan, L. L., Kim-Han, J. S., Brendza, R. P., Fryer, J. D., et al. (2001). Clusterin contributes to caspase-3-independent brain injury following neonatal hypoxia-ischemia. Nat. Med. 7, 338–343. doi: 10.1038/85487
Han, S., Nho, K., and Lee, Y. (2020). Alternative splicing regulation of an Alzheimer's risk variant in CLU. Int. J. Mol. Sci. 21. doi: 10.3390/ijms21197079
Harold, D., Abraham, R., Hollingworth, P., Sims, R., Gerrish, A., Hamshere, M. L., et al. (2009). Genome-wide association study identifies variants at CLU and PICALM associated with Alzheimer's disease. Nat. Genet. 41, 1088–1093. doi: 10.1038/ng.440
Herring, S. K., Moon, H. J., Rawal, P., Chhibber, A., and Zhao, L. (2019). Brain clusterin protein isoforms and mitochondrial localization. Elife 8, 17. doi: 10.7554/eLife.48255.017
Hsu, J. L., Lee, W. J., Liao, Y. C., Wang, S. J., and Fuh, J. L. (2017). The clinical significance of plasma clusterin and Abeta in the longitudinal follow-up of patients with Alzheimer's disease. Alzheimers. Res. Ther. 9, 91. doi: 10.1186/s13195-017-0319-x
Humphreys, D. T., Carver, J. A., Easterbrook-Smith, S. B., and Wilson, M. R. (1999). Clusterin has chaperone-like activity similar to that of small heat shock proteins. J. Biol. Chem. 274, 6875–6881. doi: 10.1074/jbc.274.11.6875
Imhof, A., Charnay, Y., Vallet, P. G., Aronow, B., Kovari, E., French, L. E., et al. (2006). Sustained astrocytic clusterin expression improves remodeling after brain ischemia. Neurobiol. Dis. 22, 274–283. doi: 10.1016/j.nbd.2005.11.009
Ingram, G., Loveless, S., Howell, O. W., Hakobyan, S., Dancey, B., Harris, C. L., et al. (2014). Complement activation in multiple sclerosis plaques: an immunohistochemical analysis. Acta Neuropathol. Commun. 2, 53. doi: 10.1186/2051-5960-2-53
Jackson, R. J., Rose, J., Tulloch, J., Henstridge, C., Smith, C., and Spires-Jones, T. L. (2019). Clusterin accumulates in synapses in Alzheimer's disease and is increased in apolipoprotein E4 carriers. Brain Commun. 1, fcz003. doi: 10.1093/braincomms/fcz003
Jay, T. R., Miller, C. M., Cheng, P. J., Graham, L. C., Bemiller, S., Broihier, M. L., et al. (2015). TREM2 deficiency eliminates TREM2+ inflammatory macrophages and ameliorates pathology in Alzheimer's disease mouse models. J. Exp. Med. 212, 287–295. doi: 10.1084/jem.20142322
Jenne, D. E., and Tschopp, J. (1989). Molecular structure and functional characterization of a human complement cytolysis inhibitor found in blood and seminal plasma: identity to sulfated glycoprotein 2, a constituent of rat testis fluid. Proc. Natl. Acad. Sci. USA. 86, 7123–7127. doi: 10.1073/pnas.86.18.7123
Jiang, T., Yu, J. T., Tian, Y., and Tan, L. (2013). Epidemiology and etiology of Alzheimer's disease: from genetic to non-genetic factors. Curr. Alzheimer Res. 10, 852–867. doi: 10.2174/15672050113109990155
John Lin, C. C., Yu, K., Hatcher, A., Huang, T. W., Lee, H. K., Carlson, J., et al. (2017). Identification of diverse astrocyte populations and their malignant analogs. Nat. Neurosci. 20, 396–405. doi: 10.1038/nn.4493
Kang, S. W., Shin, Y. J., Shim, Y. J., Jeong, S. Y., Park, I. S., and Min, B. H. (2005). Clusterin interacts with SCLIP (SCG10-like protein) and promotes neurite outgrowth of PC12 cells. Exp. Cell Res. 309, 305–315. doi: 10.1016/j.yexcr.2005.06.012
Kao, Y. C., Ho, P. C., Tu, Y. K., Jou, I. M., and Tsai, K. J. (2020). Lipids and Alzheimer's disease. Int. J. Mol. Sci. 21. doi: 10.3390/ijms21041505
Kim, N., and Choi, W. S. (2011). Proapoptotic role of nuclear clusterin in brain. Anat. Cell Biol. 44, 169–175. doi: 10.5115/acb.2011.44.3.169
Kim, N., Han, J. Y., Roh, G. S., Kim, H. J., Kang, S. S., Cho, G. J., et al. (2012). Nuclear clusterin is associated with neuronal apoptosis in the developing rat brain upon ethanol exposure. Alcohol. Clin. Exp. Res. 36, 72–82. doi: 10.1111/j.1530-0277.2011.01588.x
Kimura, K., Asami, K., and Yamamoto, M. (1997). Effect of heat shock treatment on the production of variant testosterone-repressed prostate message-2 (TRPM-2) mRNA in culture cells. Cell Biochem. Funct. 15, 251-7. doi: 10.1002/(SICI)1099-0844(199712)15:4andlt;251::AID-CBF748andgt;3.0.CO;2-3
Kimura, K., and Yamamoto, M. (1996). Modification of the alternative splicing process of testosterone-repressed prostate message-2 (TRPM-2) gene by protein synthesis inhibitors and heat shock treatment. Biochim. Biophys. Acta 1307, 83–88. doi: 10.1016/0167-4781(96)00017-6
Kobayashi, M., Konishi, H., Sayo, A., Takai, T., and Kiyama, H. (2016). TREM2/DAP12 signal elicits proinflammatory response in microglia and exacerbates neuropathic pain. J. Neurosci. 36, 11138–11150. doi: 10.1523/JNEUROSCI.1238-16.2016
Koch, L. (2018). Altered splicing in Alzheimer transcriptomes. Nat. Rev. Genet. 19, 738–739. doi: 10.1038/s41576-018-0064-4
Labadorf, A., Hoss, A. G., Lagomarsino, V., Latourelle, J. C., Hadzi, T. C., Bregu, J., et al. (2015). RNA sequence analysis of human huntington disease brain reveals an extensive increase in inflammatory and developmental gene expression. PLoS ONE 10, e0143563. doi: 10.1371/journal.pone.0143563
Lambert, J. C., Heath, S., Even, G., Campion, D., Sleegers, K., Hiltunen, M., et al. (2009). Genome-wide association study identifies variants at CLU and CR1 associated with Alzheimer's disease. Nat. Genet. 41, 1094–1099. doi: 10.1038/ng.439
Lancaster, T. M., Brindley, L. M., Tansey, K. E., Sims, R. C., Mantripragada, K., Owen, M. J., et al. (2015). Alzheimer's disease risk variant in CLU is associated with neural inefficiency in healthy individuals. Alzheimers. Dement. 11, 1144–1152. doi: 10.1016/j.jalz.2014.10.012
Leskov, K. S., Klokov, D. Y., Li, J., Kinsella, T. J., and Boothman, D. A. (2003). Synthesis and functional analyses of nuclear clusterin, a cell death protein. J. Biol. Chem. 278, 11590–11600. doi: 10.1074/jbc.M209233200
Li, R. Y., Qin, Q., Yang, H. C., Wang, Y. Y., Mi, Y. X., Yin, Y. S., et al. (2022). TREM2 in the pathogenesis of AD: a lipid metabolism regulator and potential metabolic therapeutic target. Mol. Neurodegener. 17, 40. doi: 10.1186/s13024-022-00542-y
Lidstrom, A. M., Bogdanovic, N., Hesse, C., Volkman, I., Davidsson, P., and Blennow, K. (1998). Clusterin (apolipoprotein J) protein levels are increased in hippocampus and in frontal cortex in Alzheimer's disease. Exp. Neurol. 154, 511–521. doi: 10.1006/exnr.1998.6892
Ling, I. F., Bhongsatiern, J., Simpson, J. F., Fardo, D. W., and Estus, S. (2012). Genetics of clusterin isoform expression and Alzheimer's disease risk. PLoS ONE 7, e33923. doi: 10.1371/journal.pone.0033923
Liu, Y., Thalamuthu, A., Mather, K. A., Crawford, J., Ulanova, M., Wong, M. W. K., et al. (2021). Plasma lipidome is dysregulated in Alzheimer's disease and is associated with disease risk genes. Transl. Psychiatry 11, 344. doi: 10.1038/s41398-021-01362-2
Martinez-Bujidos, M., Rull, A., Gonzalez-Cura, B., Perez-Cuellar, M., Montoliu-Gaya, L., Villegas, S., et al. (2015). Clusterin/apolipoprotein J binds to aggregated LDL in human plasma and plays a protective role against LDL aggregation. FASEB J. 29, 1688–1700. doi: 10.1096/fj.14-264036
Matukumalli, S. R., Tangirala, R., and Rao, C. M. (2017). Clusterin: full-length protein and one of its chains show opposing effects on cellular lipid accumulation. Sci. Rep. 7, 41235. doi: 10.1038/srep41235
May, P. C., Lampert-Etchells, M., Johnson, S. A., Poirier, J., Masters, J. N., and Finch, C. E. (1990). Dynamics of gene expression for a hippocampal glycoprotein elevated in Alzheimer's disease and in response to experimental lesions in rat. Neuron 5, 831–839. doi: 10.1016/0896-6273(90)90342-D
Mcgeer, P. L., Kawamata, T., and Walker, D. G. (1992). Distribution of clusterin in Alzheimer brain tissue. Brain Res. 579, 337–341. doi: 10.1016/0006-8993(92)90071-G
Mclaughlin, L., Zhu, G., Mistry, M., Ley-Ebert, C., Stuart, W. D., Florio, C. J., et al. (2000). Apolipoprotein J/clusterin limits the severity of murine autoimmune myocarditis. J. Clin. Invest. 106, 1105–1113. doi: 10.1172/JCI9037
Miners, J. S., Clarke, P., and Love, S. (2017). Clusterin levels are increased in Alzheimer's disease and influence the regional distribution of Abeta. Brain Pathol. 27, 305–313. doi: 10.1111/bpa.12392
Moon, H. J., Herring, S. K., and Zhao, L. (2021). Clusterin: a multifaceted protein in the brain. Neural. Regen. Res. 16, 1438–1439. doi: 10.4103/1673-5374.301013
Moretti, R. M., Montagnani Marelli, M., Mai, S., Cariboni, A., Scaltriti, M., Bettuzzi, S., et al. (2007). Clusterin isoforms differentially affect growth and motility of prostate cells: possible implications in prostate tumorigenesis. Cancer Res. 67, 10325–10333. doi: 10.1158/0008-5472.CAN-07-0516
Morgan, T. E., Laping, N. J., Rozovsky, I., Oda, T., Hogan, T. H., Finch, C. E., et al. (1995). Clusterin expression by astrocytes is influenced by transforming growth factor beta 1 and heterotypic cell interactions. J. Neuroimmunol. 58, 101–110. doi: 10.1016/0165-5728(94)00194-S
Narayan, P., Orte, A., Clarke, R. W., Bolognesi, B., Hook, S., Ganzinger, K. A., et al. (2011). The extracellular chaperone clusterin sequesters oligomeric forms of the amyloid-beta(1-40) peptide. Nat. Struct. Mol. Biol. 19, 79–83. doi: 10.1038/nsmb.2191
Nelson, A. R., Sagare, A. P., and Zlokovic, B. V. (2017). Role of clusterin in the brain vascular clearance of amyloid-beta. Proc. Natl. Acad. Sci. USA. 114, 8681–8682. doi: 10.1073/pnas.1711357114
Nilselid, A. M., Davidsson, P., Nagga, K., Andreasen, N., Fredman, P., and Blennow, K. (2006). Clusterin in cerebrospinal fluid: analysis of carbohydrates and quantification of native and glycosylated forms. Neurochem. Int. 48, 718–728. doi: 10.1016/j.neuint.2005.12.005
Nizard, P., Tetley, S., Le Drean, Y., Watrin, T., Le Goff, P., Wilson, M. R., et al. (2007). Stress-induced retrotranslocation of clusterin/ApoJ into the cytosol. Traffic 8, 554–565. doi: 10.1111/j.1600-0854.2007.00549.x
Nugent, A. A., Lin, K., Van Lengerich, B., Lianoglou, S., Przybyla, L., Davis, S. S., et al. (2020). TREM2 regulates microglial cholesterol metabolism upon chronic phagocytic challenge. Neuron 105, 837-854 e9. doi: 10.1016/j.neuron.2019.12.007
Nuutinen, T., Suuronen, T., Kauppinen, A., and Salminen, A. (2009). Clusterin: a forgotten player in Alzheimer's disease. Brain Res. Rev. 61, 89–104. doi: 10.1016/j.brainresrev.2009.05.007
O'bryan, M. K., Cheema, S. S., Bartlett, P. F., Murphy, B. F., and Pearse, M. J. (1993). Clusterin levels increase during neuronal development. J. Neurobiol. 24, 421–432. doi: 10.1002/neu.480240402
Oh, S. B., Kim, M. S., Park, S., Son, H., Kim, S. Y., Kim, M. S., et al. (2019). Clusterin contributes to early stage of Alzheimer's disease pathogenesis. Brain Pathol. 29, 217–231. doi: 10.1111/bpa.12660
Paasila, P. J., Aramideh, J. A., Sutherland, G. T., and Graeber, M. B. (2021). Synapses, microglia, and lipids in Alzheimer's disease. Front. Neurosci. 15, 778822. doi: 10.3389/fnins.2021.778822
Park, J. S., Lee, W. K., Kim, H. S., Seo, J. A., Kim, D. H., Han, H. C., et al. (2020). Clusterin overexpression protects against western diet-induced obesity and NAFLD. Sci. Rep. 10, 17484. doi: 10.1038/s41598-020-73927-y
Pasinetti, G. M., Johnson, S. A., Oda, T., Rozovsky, I., and Finch, C. E. (1994). Clusterin (SGP-2): a multifunctional glycoprotein with regional expression in astrocytes and neurons of the adult rat brain. J. Comp. Neurol. 339, 387–400. doi: 10.1002/cne.903390307
Pedraza, O., Allen, M., Jennette, K., Carrasquillo, M., Crook, J., Serie, D., et al. (2014). Evaluation of memory endophenotypes for association with CLU, CR1, and PICALM variants in black and white subjects. Alzheimers. Dement. 10, 205–213. doi: 10.1016/j.jalz.2013.01.016
Poliani, P. L., Wang, Y., Fontana, E., Robinette, M. L., Yamanishi, Y., Gilfillan, S., et al. (2015). TREM2 sustains microglial expansion during aging and response to demyelination. J. Clin. Invest. 125, 2161–2170. doi: 10.1172/JCI77983
Prochnow, H., Gollan, R., Rohne, P., Hassemer, M., Koch-Brandt, C., and Baiersdorfer, M. (2013). Non-secreted clusterin isoforms are translated in rare amounts from distinct human mRNA variants and do not affect Bax-mediated apoptosis or the NF-kappaB signaling pathway. PLoS ONE 8, e75303. doi: 10.1371/annotation/48cea21c-d035-4757-bf42-c97028306f11
Raj, T., Li, Y. I., Wong, G., Humphrey, J., Wang, M., Ramdhani, S., et al. (2018). Integrative transcriptome analyses of the aging brain implicate altered splicing in Alzheimer's disease susceptibility. Nat. Genet. 50, 1584–1592. doi: 10.1038/s41588-018-0238-1
Ramsden, M., Kotilinek, L., Forster, C., Paulson, J., Mcgowan, E., Santacruz, K., et al. (2005). Age-dependent neurofibrillary tangle formation, neuron loss, and memory impairment in a mouse model of human tauopathy (P301L). J. Neurosci. 25, 10637–10647. doi: 10.1523/JNEUROSCI.3279-05.2005
Reitz, C., and Mayeux, R. (2014). Alzheimer disease: epidemiology, diagnostic criteria, risk factors and biomarkers. Biochem. Pharmacol. 88, 640–651. doi: 10.1016/j.bcp.2013.12.024
Rizzi, F., and Bettuzzi, S. (2010). The clusterin paradigm in prostate and breast carcinogenesis. Endocr. Relat. Cancer 17, R1–17. doi: 10.1677/ERC-09-0140
Robert, J., Button, E. B., Yuen, B., Gilmour, M., Kang, K., Bahrabadi, A., et al. (2017). Clearance of beta-amyloid is facilitated by apolipoprotein E and circulating high-density lipoproteins in bioengineered human vessels. Elife 6, 18. doi: 10.7554/eLife.29595.018
Rockenstein, E. M., Mcconlogue, L., Tan, H., Power, M., Masliah, E., and Mucke, L. (1995). Levels and alternative splicing of amyloid beta protein precursor (APP) transcripts in brains of APP transgenic mice and humans with Alzheimer's disease. J. Biol. Chem. 270, 28257–28267. doi: 10.1074/jbc.270.47.28257
Rodriguez-Pineiro, A. M., De La Cadena, M. P., Lopez-Saco, A., and Rodriguez-Berrocal, F. J. (2006). Differential expression of serum clusterin isoforms in colorectal cancer. Mol. Cell. Proteomics 5, 1647–1657. doi: 10.1074/mcp.M600143-MCP200
Roussotte, F. F., Gutman, B. A., Madsen, S. K., Colby, J. B., Thompson, P. M., and Alzheimer's Disease Neuroimaging, I. (2014). Combined effects of Alzheimer risk variants in the CLU and ApoE genes on ventricular expansion patterns in the elderly. J. Neurosci. 34, 6537–6545. doi: 10.1523/JNEUROSCI.5236-13.2014
Saewu, A., Kadunganattil, S., Raghupathy, R., Kongmanas, K., Diaz-Astudillo, P., Hermo, L., et al. (2017). Clusterin in the mouse epididymis: possible roles in sperm maturation and capacitation. Reproduction 154, 867–880. doi: 10.1530/REP-17-0518
Santilli, G., Aronow, B. J., and Sala, A. (2003). Essential requirement of apolipoprotein J (clusterin) signaling for IkappaB expression and regulation of NF-kappaB activity. J. Biol. Chem. 278, 38214–38219. doi: 10.1074/jbc.C300252200
Sasaki, K., Doh-Ura, K., Ironside, J. W., and Iwaki, T. (2002). Increased clusterin (apolipoprotein J) expression in human and mouse brains infected with transmissible spongiform encephalopathies. Acta Neuropathol. 103, 199–208. doi: 10.1007/s004010100456
Scaltriti, M., Santamaria, A., Paciucci, R., and Bettuzzi, S. (2004). Intracellular clusterin induces G2-M phase arrest and cell death in PC-3 prostate cancer cells1. Cancer Res. 64, 6174–6182. doi: 10.1158/0008-5472.CAN-04-0920
Schepeler, T., Mansilla, F., Christensen, L. L., Orntoft, T. F., and Andersen, C. L. (2007). Clusterin expression can be modulated by changes in TCF1-mediated Wnt signaling. J. Mol. Signal. 2, 6. doi: 10.1186/1750-2187-2-6
Schreiber, S. S., Tocco, G., Najm, I., and Baudry, M. (1993). Seizure activity causes a rapid increase in sulfated glycoprotein-2 messenger RNA in the adult but not the neonatal rat brain. Neurosci. Lett. 153, 17–20. doi: 10.1016/0304-3940(93)90066-T
Seshadri, S., Fitzpatrick, A. L., Ikram, M. A., Destefano, A. L., Gudnason, V., Boada, M., et al. (2010). Genome-wide analysis of genetic loci associated with Alzheimer disease. JAMA 303, 1832–1840. doi: 10.1001/jama.2010.574
Solichova, P., Karpisek, M., Ochmanova, R., Hanulova, Z., Humenanska, V., Stejskal, D., et al. (2007). Urinary clusterin concentrations–a possible marker of nephropathy? Pilot study. Biomed. Pap Med. Fac. Univ. Palacky Olomouc Czech Repub. 151, 233–236. doi: 10.5507/bp.2007.039
Szymanski, M., Wang, R., Bassett, S. S., and Avramopoulos, D. (2011). Alzheimer's risk variants in the clusterin gene are associated with alternative splicing. Transl. Psychiatry 1, e18. doi: 10.1038/tp.2011.17
Tan, L., Wang, H. F., Tan, M. S., Tan, C. C., Zhu, X. C., Miao, D., et al. (2016). Effect of CLU genetic variants on cerebrospinal fluid and neuroimaging markers in healthy, mild cognitive impairment and Alzheimer's disease cohorts. Sci. Rep. 6, 26027. doi: 10.1038/srep26027
Thambisetty, M., Beason-Held, L. L., An, Y., Kraut, M., Nalls, M., Hernandez, D. G., et al. (2013). Alzheimer risk variant CLU and brain function during aging. Biol. Psychiatry 73, 399–405. doi: 10.1016/j.biopsych.2012.05.026
Trindade, P., Hampton, B., Manhaes, A. C., and Medina, A. E. (2016). Developmental alcohol exposure leads to a persistent change on astrocyte secretome. J. Neurochem. 137, 730–743. doi: 10.1111/jnc.13542
Troakes, C., Smyth, R., Noor, F., Maekawa, S., Killick, R., King, A., et al. (2017). Clusterin expression is upregulated following acute head injury and localizes to astrocytes in old head injury. Neuropathology 37, 12–24. doi: 10.1111/neup.12320
Trougakos, I. P., and Gonos, E. S. (2002). Clusterin/apolipoprotein J in human aging and cancer. Int. J. Biochem. Cell Biol. 34, 1430–1448. doi: 10.1016/S1357-2725(02)00041-9
Trougakos, I. P., and Gonos, E. S. (2009). Chapter 9: Oxidative stress in malignant progression: The role of Clusterin, a sensitive cellular biosensor of free radicals. Adv. Cancer Res. 104, 171–210. doi: 10.1016/S0065-230X(09)04009-3
Turri, M., Marchi, C., Adorni, M. P., Calabresi, L., and Zimetti, F. (2022). Emerging role of HDL in brain cholesterol metabolism and neurodegenerative disorders. Biochim. Biophys. Acta Mol. Cell. Biol. Lipids 1867, 159123. doi: 10.1016/j.bbalip.2022.159123
Ulland, T. K., Song, W. M., Huang, S. C., Ulrich, J. D., Sergushichev, A., Beatty, W. L., et al. (2017). TREM2 maintains microglial metabolic fitness in Alzheimer's disease. Cell 170, 649–663 e13. doi: 10.1016/j.cell.2017.07.023
Wang, Y., Cella, M., Mallinson, K., Ulrich, J. D., Young, K. L., Robinette, M. L., et al. (2015). TREM2 lipid sensing sustains the microglial response in an Alzheimer's disease model. Cell 160, 1061–1071. doi: 10.1016/j.cell.2015.01.049
Wightman, D. P., Jansen, I. E., Savage, J. E., Shadrin, A. A., Bahrami, S., Holland, D., et al. (2021). A genome-wide association study with 1,126,563 individuals identifies new risk loci for Alzheimer's disease. Nat. Genet. 53, 1276–1282. doi: 10.1038/s41588-021-00921-z
Wilson, M. R., and Easterbrook-Smith, S. B. (1992). Clusterin binds by a multivalent mechanism to the Fc and Fab regions of IgG. Biochim. Biophys. Acta 1159, 319–326. doi: 10.1016/0167-4838(92)90062-I
Wojtas, A. M., Kang, S. S., Olley, B. M., Gatherer, M., Shinohara, M., Lozano, P. A., et al. (2017). Loss of clusterin shifts amyloid deposition to the cerebrovasculature via disruption of perivascular drainage pathways. Proc. Natl. Acad. Sci. USA. 114, E6962–E6971. doi: 10.1073/pnas.1701137114
Wojtas, A. M., Sens, J. P., Kang, S. S., Baker, K. E., Berry, T. J., Kurti, A., et al. (2020). Astrocyte-derived clusterin suppresses amyloid formation in vivo. Mol. Neurodegener. 15, 71. doi: 10.1186/s13024-020-00416-1
Wong, P., Taillefer, D., Lakins, J., Pineault, J., Chader, G., and Tenniswood, M. (1994). Molecular characterization of human TRPM-2/clusterin, a gene associated with sperm maturation, apoptosis and neurodegeneration. Eur. J. Biochem. 221, 917–925. doi: 10.1111/j.1432-1033.1994.tb18807.x
Woody, S. K., and Zhao, L. (2016). :Clusterin (APOJ) in Alzheimer's disease: an old molecule with a new role.” in Update on Dementia. eBook, ed Moretti, D. V., doi: 10.5772/64233
Yamada, M. (2015). Cerebral amyloid angiopathy: emerging concepts. J. Stroke 17, 17–30. doi: 10.5853/jos.2015.17.1.17
Yang, C. R., Leskov, K., Hosley-Eberlein, K., Criswell, T., Pink, J. J., Kinsella, T. J., et al. (2000). Nuclear clusterin/XIP8, an x-ray-induced Ku70-binding protein that signals cell death. Proc. Natl. Acad. Sci. USA. 97, 5907–5912. doi: 10.1073/pnas.97.11.5907
Yeh, F. L., Wang, Y., Tom, I., Gonzalez, L. C., and Sheng, M. (2016). TREM2 binds to apolipoproteins, including APOE and CLU/APOJ, and thereby facilitates uptake of amyloid-beta by microglia. Neuron 91, 328–340. doi: 10.1016/j.neuron.2016.06.015
Yu, V., Bhattacharya, D., Webster, A., Bauskar, A., Flowers, C., Heur, M., et al. (2018). Clusterin from human clinical tear samples: positive correlation between tear concentration and Schirmer strip test results. Ocul. Surf. 16, 478–486. doi: 10.1016/j.jtos.2018.08.001
Yuste-Checa, P., Bracher, A., and Hartl, F. U. (2022). The chaperone clusterin in neurodegeneration-friend or foe? Bioessays 44, e2100287. doi: 10.1002/bies.202100287
Yuste-Checa, P., Trinkaus, V. A., Riera-Tur, I., Imamoglu, R., Schaller, T. F., Wang, H., et al. (2021). The extracellular chaperone clusterin enhances Tau aggregate seeding in a cellular model. Nat. Commun. 12, 4863. doi: 10.1038/s41467-021-25060-1
Zhang, H., Kim, J. K., Edwards, C. A., Xu, Z., Taichman, R., and Wang, C. Y. (2005). Clusterin inhibits apoptosis by interacting with activated Bax. Nat. Cell Biol. 7, 909–915. doi: 10.1038/ncb1291
Zhao, X., Henderson, H. J., Wang, T., Liu, B., and Li, Y. (2021). Deletion of clusterin protects cochlear hair cells against hair cell aging and ototoxicity. Neural Plast. 2021, 9979157. doi: 10.1155/2021/9979157
Zhao, Z., Nelson, A. R., Betsholtz, C., and Zlokovic, B. V. (2015a). Establishment and dysfunction of the blood-brain barrier. Cell 163, 1064–1078. doi: 10.1016/j.cell.2015.10.067
Zhao, Z., Sagare, A. P., Ma, Q., Halliday, M. R., Kong, P., Kisler, K., et al. (2015b). Central role for PICALM in amyloid-beta blood-brain barrier transcytosis and clearance. Nat. Neurosci. 18, 978–987. doi: 10.1038/nn.4025
Zheng, H., Jia, L., Liu, C. C., Rong, Z., Zhong, L., Yang, L., et al. (2017). TREM2 promotes microglial survival by activating Wnt/beta-catenin pathway. J. Neurosci. 37, 1772–1784. doi: 10.1523/JNEUROSCI.2459-16.2017
Zhou, Y., Hayashi, I., Wong, J., Tugusheva, K., Renger, J. J., and Zerbinatti, C. (2014). Intracellular clusterin interacts with brain isoforms of the bridging integrator 1 and with the microtubule-associated protein Tau in Alzheimer's disease. PLoS ONE 9, e103187. doi: 10.1371/journal.pone.0103187
Zhu, T. B., Zhang, Z., Luo, P., Wang, S. S., Peng, Y., Chu, S. F., et al. (2019). Lipid metabolism in Alzheimer's disease. Brain Res. Bull. 144, 68–74. doi: 10.1016/j.brainresbull.2018.11.012
Keywords: clusterin, apolipoprotein J, Alzheimer's disease, isoforms, mouse models
Citation: Milinkeviciute G and Green KN (2023) Clusterin/apolipoprotein J, its isoforms and Alzheimer's disease. Front. Aging Neurosci. 15:1167886. doi: 10.3389/fnagi.2023.1167886
Received: 16 February 2023; Accepted: 27 March 2023;
Published: 13 April 2023.
Edited by:
Helene Eliane Hirbec, INSERM U1191 Institut de Génomique Fonctionnelle (IGF), FranceReviewed by:
Fabrizio Piazza, University of Milano-Bicocca, ItalyCopyright © 2023 Milinkeviciute and Green. This is an open-access article distributed under the terms of the Creative Commons Attribution License (CC BY). The use, distribution or reproduction in other forums is permitted, provided the original author(s) and the copyright owner(s) are credited and that the original publication in this journal is cited, in accordance with accepted academic practice. No use, distribution or reproduction is permitted which does not comply with these terms.
*Correspondence: Giedre Milinkeviciute, giedre.milin@gmail.com