Association Between Plasma Apolipoprotein M With Alzheimer’s Disease: A Cross-Sectional Pilot Study From China
- 1Department of Clinical Medicine, North Sichuan Medical College, Nanchong, China
- 2Department of Neurology, General Hospital of Western Theater Command, Chengdu, China
- 3Department of Geriatrics, Sichuan Provincial People’s Hospital, University of Electronic Science and Technology of China, Chengdu, China
- 4Chinese Academy of Sciences Sichuan Translational Medicine Research Hospital, Chengdu, China
- 5Department of Neurology, Sichuan Provincial People’s Hospital, University of Electronic Science and Technology of China, Chengdu, China
- 6Department of Neurology, Chengdu Eighth People’s Hospital, Chengdu, China
Background: Recent evidence of genetics and metabonomics indicated a potential role of apolipoprotein M (ApoM) in the pathogenesis of Alzheimer’s disease (AD). Here, we aimed to investigate the association between plasma ApoM with AD.
Methods: A multicenter, cross-sectional study recruited patients with AD (n = 67), age- and sex-matched cognitively normal (CN) controls (n = 73). After the data collection of demographic characteristics, lifestyle risk factors, and medical history, we examined and compared the plasma levels of ApoM, tau phosphorylated at threonine 217 (p-tau217) and neurofilament light (NfL). Multivariate logistic regression analysis was applied to determine the association of plasma ApoM with the presence of AD. The correlation analysis was used to explore the correlations between plasma ApoM with cognitive function [Mini–Mental State Examination (MMSE) and Montreal Cognitive Assessment (MoCA)], activities of daily living (ADL), and the representative blood-based biomarkers (plasma p-tau217 and NfL). Receiver operating characteristic (ROC) analysis and Delong’s test were used to determine the diagnostic power of plasma ApoM.
Results: Plasma ApoM and its derived indicators (ratios of ApoM/TC, ApoM/TG, ApoM/HDL-C, and ApoM/LDL-C) were significantly higher in AD group than those in CN group (each p < 0.0001). After adjusted for the risk factors of AD, the plasma ApoM and its derived indicators were significantly associated with the presence of AD, respectively. ApoM (OR = 1.058, 95% CI: 1.027–1.090, p < 0.0001), ApoM/TC ratio (OR = 1.239, 95% CI: 1.120–1.372, p < 0.0001), ApoM/TG ratio (OR = 1.064, 95% CI: 1.035–1.095, p < 0.0001), ApoM/HDL-C ratio (OR = 1.069, 95% CI: 1.037–1.102, p < 0.0001), and ApoM/LDL-C ratio (OR = 1.064, 95% CI:1.023–1.106, p = 0.002). In total participants, plasma ApoM was significantly positively correlated with plasma p-tau217, plasma NfL, and ADL (each p < 0.0001) and significantly negatively correlated with MMSE and MoCA (each p < 0.0001), respectively. In further subgroup analyses, these associations remained in different APOEϵ 4 status participants and sex subgroups. ApoM/TC ratio (ΔAUC = 0.056, p = 0.044) and ApoM/TG ratio (ΔAUC = 0.097, p = 0.011) had a statistically remarkably larger AUC than ApoM, respectively. The independent addition of ApoM and its derived indicators to the basic model [combining age, sex, APOEϵ 4, and body mass index (BMI)] led to the significant improvement in diagnostic power, respectively (each p < 0.05).
Conclusion: All the findings preliminarily uncovered the association between plasma ApoM and AD and provided more evidence of the potential of ApoM as a candidate biomarker of AD.
Introduction
As characterized by the progressive decline of cognitive function, activities of daily living (ADL), and social function (Knopman et al., 2021), Alzheimer’s disease (AD) is an age-related neurodegenerative disorder, which accounts for about 60–80% of all dementias (Alzheimer’s Association, 2021). It is generally believed that the sporadic AD (which accounts for more than 99% of all cases) is comprehensively caused by age, genetics, metabolism, inflammation, and environmental and other factors (Livingston et al., 2020); however, the exact pathogenesis has not been fully elucidated and the treatment that can block or reverse the disease progress has not been developed so far (Levey, 2021). Thus, the significance of the early diagnosis of AD grows to be increasingly prominent.
In the past decades, the great progress of biomarkers has promoted an early diagnosis of AD to a large extent. The AD research framework proposed by NIA-AA in 2018 categorized AD biomarkers into A/T(N) system (Jack et al., 2018), in which the core biomarkers were mainly based on cerebrospinal fluid (CSF) test [e.g., CSF-amyloid β (Aβ) and CSF-phosphorylated-tau (p-tau)] or positron emission tomography (PET) test (e.g., 11C-Pittsburgh compound B (PiB)-PET and tau-PET) (Jack et al., 2018). Nevertheless, the potential risk of invasive deep puncture in CSF collection and the high cost of PET test have hindered the applications of these biomarkers. Therefore, the development of blood-based biomarkers of AD is promising currently (Thijssen and Rabinovici, 2021).
Apolipoprotein M (ApoM) is a newly discovered apolipoprotein with a molecular weight of 26 kDa (Xu and Dahlbäck, 1999), which is mainly expressed in liver, kidney, adipose tissue, and cerebrovascular endothelial cells of human beings (Xu and Dahlbäck, 1999; Kober et al., 2017; Sramkova et al., 2019). To date, the studies of ApoM mainly focused on lipid metabolism (Wolfrum et al., 2005), cardiovascular disease (Versmissen et al., 2016), and diabetes (Kurano et al., 2020). Of note, a genome-wide association study (GWAS) found that the APOM gene could drive the association of lipid metabolism pathway with AD risk (Kunkle et al., 2019; Chernick et al., 2020). Besides, the CSF level of ApoM in the patients with AD was significantly lower than that in the cognitively normal (CN) controls (Khoonsari et al., 2016). But, the relationship between blood ApoM and AD has not been revealed so far.
In addition, there are often obviously individual differences in the absolute values of certain blood-based biomarkers in clinical practice, which limits their interpretation and applications. Thus, standardization or normalization by calculating with other related clinical indicators, such as the international normalized ratio (INR) generated from the prothrombin time (PT), may lead to better stability and comparability (Deitcher, 2002). Actually, the combination of biomarkers has also been a common strategy in AD research, such as Aβ42/Aβ40 ratio, p-tau/total-tau ratio, and total-tau/α-synuclein ratio (Shim et al., 2020), etc. Considering that ApoM is closely related to lipid metabolism and based on our previous research experience (Yang et al., 2021), the ratios of blood ApoM level to that of different blood lipids including total cholesterol (TC), triglyceride (TG), high-density lipoprotein cholesterol (HDL-C), and low-density lipoprotein cholesterol (LDL-C), may be the more optimized indicators than ApoM itself (Yang et al., 2021).
Therefore, here, we reported a multicenter, cross-sectional pilot clinical study, in which AD group and CN control group were recruited. The demographic data, clinical characteristics, and some of the common indicators in clinical laboratories were collected. Then, we detected and compared the plasma ApoM levels of both groups. Further, we investigated the correlation between plasma ApoM with the presence, main clinical characteristics, and the representative biomarkers of AD, respectively. Finally, we explored the diagnostic power of plasma ApoM for AD. We expected that this study could provide new evidence for evaluating plasma ApoM as a new candidate biomarker of AD.
Materials and Methods
Study Design and Participant Selection
Both groups were recruited from the Department of Neurology and the Department of Gerontology of Sichuan Provincial People’s Hospital, Western Theater Command General Hospital, and the Eighth People’s Hospital of Chengdu from March to September, 2021. The diagnosis of AD was in accordance with the criteria for “Probable AD” of the National Institute of Neurological and Speech Disorders and Stroke/Alzheimer’s Disease and Related Disorders Association criteria (NINCDS-ADRDA) (McKhann et al., 1984). These procedures were administered by two trained interviewers who were experienced neurologists. The participants were excluded if they had (Zhuang et al., 2018; Zhang et al., 2021) (1) a family history of dementia; (2) severe cardiac, pulmonary, hepatic, renal diseases, or any kinds of tumor; (3) enduring mental illness (e.g., schizophrenia); and (4) other kinds of cognitive deficits, such as vascular dementia, dementia with Lewy bodies, frontotemporal dementia, dementia of Parkinson’s disease, and normal pressure hydrocephalus. We first screened the cognitive state of participants using the China version of the Mini–Mental State Examination (MMSE) and Montreal Cognitive Assessment (MoCA). The cutoff values after correcting the years of education were used in the MMSE (≤24 for 6 + years of education, ≤20 for 6 years of education, and ≤17 for 0 year of education) and MoCA (<26 for 12 + years of education and <25 for 12 years of education) (Hu et al., 2020). ADL scale was used to evaluate the daily living ability of all the participants. The full score was 56 points, and the higher the score was, the more severe the impairment of daily living ability was. The vascular factors were assessed by the Hachinski Ischemic Score (HIS). Participants with abnormal cognitive function assessment were further assessed with the Clinical Dementia Rating (CDR) in which patients with AD had a CDR score ≥0.5 (Morris, 1993).
Baseline data on demographic characteristics (age, sex, and years of education), body mass index (BMI), lifestyle risk factors (cigarette smoking and alcohol consumption), and medical history (hypertension and diabetes mellitus) were collected within 24 h of hospital admission.
Biochemical and Molecular Investigations
Following our previously published protocols (Yang et al., 2021), fasting blood samples were collected between 06:00 and 07:00 a.m. within 24 h of admission to avoid variations related to possible circadian rhythm effects, and aliquots were made for routine blood tests [i.e., hemoglobin A1C (HbA1C), white blood cell (WBC) count, C-reactive protein (CRP), TC, TG, HDL-C, LDL-C], and APOE genotype [determined using a Sanger sequencing assay for single-nucleotide polymorphism (SNP) rs7412 and rs429358 (Belloy et al., 2019)] in the Department of Clinical Laboratory.
The plasma was separated and frozen at –80°C until ApoM, p-tau217, and NfL measurement. In accordance with our previous published studies (Sun et al., 2020), the plasma p-tau217 and NfL were quantified using enzyme-linked immunosorbent assay (ELISA) kits (Enzyme Biosystems). The specimen, standard, and HRP-labeled detection antibodies corresponding to two specific proteins were sequentially added to microwells precoated with human p-tau217 and NfL capture antibody, respectively. The microwells were incubated and washed thoroughly. The substrate TMB was used to develop color. TMB was first converted to a blue color under the catalysis of peroxidase and converted to the final yellow color by the action of an acid. The color depth was positively correlated with p-tau217 and NfL in the sample. Besides, we employed a commercial ELISA kit (SEC299Hu, Cloud-Clone Corp., Houston, TX, United States) to measure the plasma ApoM concentrations in accordance with the previous studies (Zhang et al., 2017). Samples and standards were measured in duplicate, and the means of the duplicates were used for statistical analysis.
Statistical Analyses
All statistical analyses were performed using IBM SPSS Statistics version 25 (IBM, Armonk, NY, United States) and GraphPad Prism 6 (GraphPad Software, La Jolla, CA, United States). For continuous variables, data were expressed as mean ± standard deviation (SD) or median and interquartile range (IQR), which depended on the data distribution, and the Mann–Whitney U test or the t-test were used to test the differences between the two groups. Categorical variables were expressed as proportions, and a chi-square test was applied in the comparison between groups. The influence of plasma ApoM and ApoM-derived indicators on the presence of AD was determined by multivariate logistic regression analysis, which allowed adjustment for the confounding factors. Clinical variables with p < 0.05 on univariate analysis and the general AD risk factors (age, sex, and APOE4 carriage status) were incorporated into multivariate logistic regression analysis models. The results were expressed as odds ratio (OR) with corresponding 95% confidence interval (CI). Correlations between plasma ApoM and its derived indicators with clinical characteristics and the representative blood-based biomarkers of AD were analyzed using Spearman’s rank correlation or Pearson’s correlation. The clinical value of adding ApoM and ApoM-derived indicators to the general risk factors for identifying or predicting the presence of AD was calculated with receiver operating characteristic (ROC) curves. Using the DeLong’s test, we compared the area under the curve (AUC) between ApoM with ApoM-derived indicators and between the basic model (sex, age, APOE4, and BMI) with basic model combined with ApoM and its derived indicators, respectively. All tests were two-sided, and p < 0.05 was considered statistically significant. Goodness-of-fit of logistic regression models was assessed using the Hosmer–Lemeshow test. None of the above models displayed a Hosmer–Lemeshow chi-squared value yielding a p < 0.05, and therefore, none were rejected.
Results
Comparison of Baseline Data Between Two Groups
Eventually, a total of 140 participants were recruited including CN group (n = 73) and AD group (n = 67), and the detailed enrollment process for participants is shown in Supplementary Figure 1. The demographic characteristics, BMI, lifestyle risk factors, medical history, cognitive function, ADL, and blood tests of all participants are shown in Table 1. No differences in age, sex, years of education, cigarette smoking, alcohol consumption, hypertension, diabetes mellitus, WBC count, HbA1c, and CRP between two groups were found (each p > 0.05) (Table 1). Compared with CN group, the percentage of APOE4-positive was significantly higher in AD group (12.3 vs. 38.3%, p < 0.0001), whereas BMI (23.86 ± 2.99 vs. 20.72 ± 2.19, p < 0.0001), TC (4.20 ± 0.86 vs. 3.76 ± 0.72, p = 0.001), TG (1.68 ± 0.54 vs. 1.24 ± 0.41, p < 0.0001), and HDL-C (1.33 ± 0.29 vs. 1.18 ± 0.24, p = 0.001) were markedly lower, respectively.
As for cognitive function and ADL, AD group had significantly lower MMSE scores [28 (27–29) vs. 10 (8–14), p < 0.0001] and MoCA scores [25 (25–26) vs. 6 (4–8), p < 0.0001], and remarkably higher ADL scores [14 (14–14) vs. 43 (31–52), p < 0.0001] than CN group, respectively (Table 1). Regarding the representative blood biomarkers, plasma levels of p-tau 217 (174.14 ± 89.20 vs. 276.31 ± 128.37, p < 0.0001) and NfL (532.64 ± 189.28 vs. 851.93 ± 344.70, p < 0.0001) in AD group were significantly higher than those in CN group, respectively (Table 1 and Figure 1).
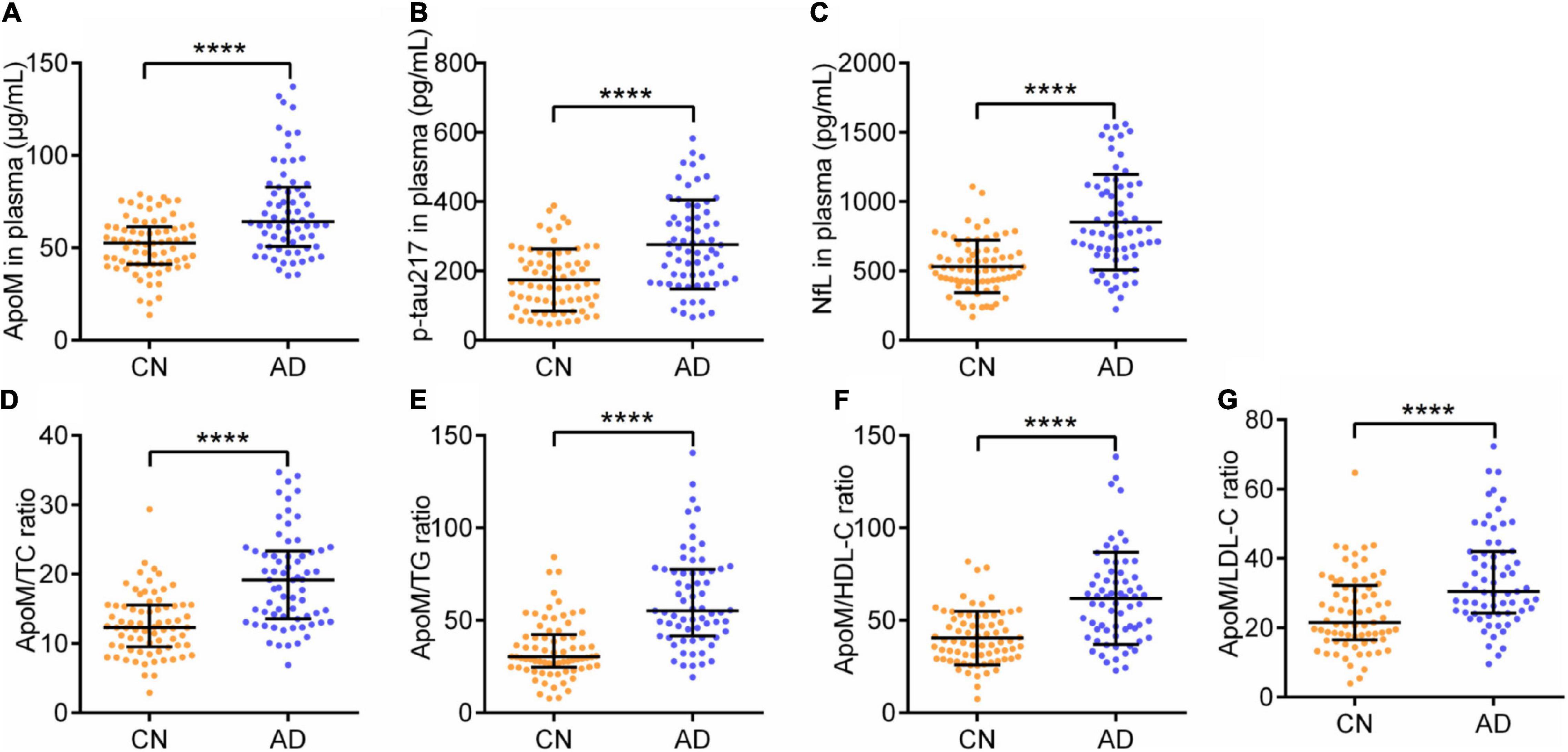
Figure 1. Plasma levels of ApoM, p-tau217, NfL, and ApoM-derived indicators between two groups. ApoM, apolipoprotein M; p-tau217, tau phosphorylated at threonine 217; NfL, neurofilament light chain; CN, cognitively normal; AD, Alzheimer’s disease; TC, total cholesterol; TG, triglyceride; HDL-C, high-density lipoprotein cholesterol; LDL-C, low-density lipoprotein cholesterol; SD, standard deviation; IQR, interquartile range. Median with IQR (A,D,E,G) and mean ± SD (B,C,F). Statistical comparison was performed using Mann–Whitney U test (A,D,E,G) or Student’s t-test (B,C,F). ****p ≤ 0.0001. p < 0.05 was considered the statistical significance.
Alzheimer’s disease group had a significantly higher plasma ApoM level [52.66 (41.20–61.44) vs. p < 0.0001] than CN group (Table 1 and Figure 1). Besides, each of the ApoM-derived indicators that include the ratios of ApoM/TC [12.29 (9.49–15.52) vs. 19.17(13.55–23.34), p < 0.0001], ApoM/TG [30.26 (24.62–42.24) vs. 55.20 (41.61–77.64), p < 0.0001], ApoM/HDL-C (40.43 ± 14.46 vs. 61.85 ± 24.97, p < 0.0001), and ApoM/LDL-C [21.50 (16.52–32.19) vs. 30.48 (24.24–42.01), p < 0.0001] was significantly higher in AD group than that in CN group (Table 1 and Figure 1).
Association Between Plasma Apolipoprotein M With the Presence of Alzheimer’s Disease
The associations of plasma ApoM level and its derived indicators with the presence of AD were investigated by multivariate logistic regression analysis. When AD risk factors (age, sex, and APOE4 carriage status) and BMI were adjusted, the plasma ApoM level (OR = 1.058, 95% CI: 1.027–1.090, p < 0.0001) and the ratios of ApoM/TC (OR = 1.239, 95% CI: 1.120–1.372, p < 0.0001), ApoM/TG (OR = 1.064, 95% CI: 1.035–1.095, p < 0.0001), ApoM/HDL-C (OR = 1.069, 95% CI: 1.037–1.102, p < 0.0001), and ApoM/LDL-C (OR = 1.064, 95% CI: 1.023–1.106, p = 0.002) were significantly associated with the presence of AD, respectively (Table 2).
Correlations Between Plasma Apolipoprotein M With Clinical Characteristics of Alzheimer’s Disease
Among all participants, ApoM and four derived indicators were significantly negatively correlated with MMSE scores and MoCA scores and significantly positively correlated with ADL scores, respectively (each p < 0.0001). Thereinto, ApoM/TG ratio had the highest absolute value of correlation coefficient in each analysis (rs = –0.551 with MMSE scores, rs = –0.573 with MoCA scores, rs = 0.619 with ADL scores) (Figure 2).
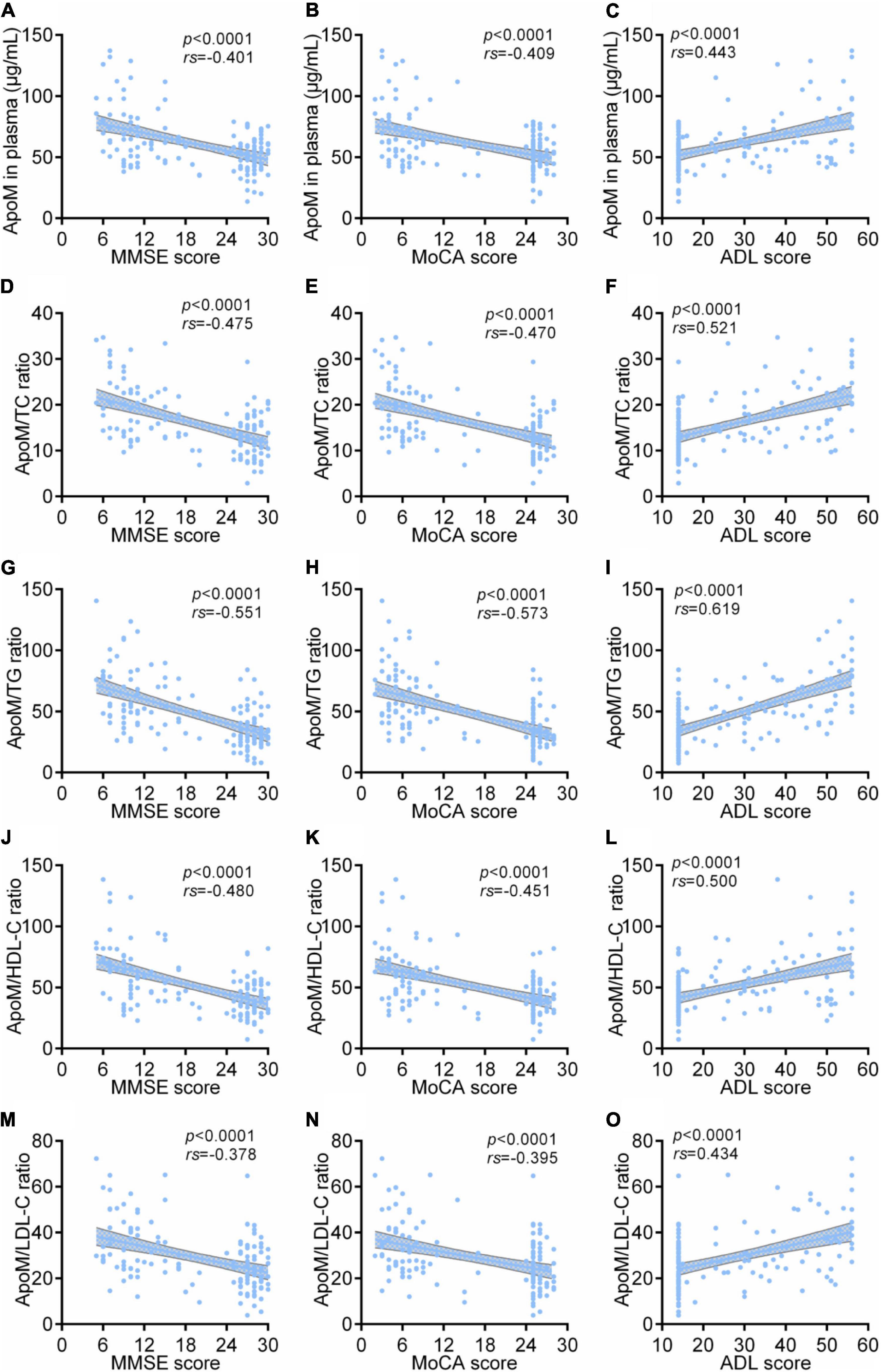
Figure 2. Correlations between plasma ApoM and its derived indicators with clinical characteristics of AD among all participants. The correlations between plasma ApoM with MMSE score (A), MoCA score (B) and ADL score (C). The correlations between ApoM/TC ratio with MMSE score (D), MoCA score (E) and ADL score (F). The correlations between ApoM/TG ratio with MMSE score (G), MoCA score (H) and ADL score (I). The correlations between ApoM/HDL-C ratio with MMSE score (J), MoCA score (K) and ADL score (L). The correlations between ApoM/LDL-C ratio with MMSE score (M), MoCA score (N) and ADL score (O). ApoM, apolipoprotein M; AD, Alzheimer’s disease; MMSE, Mini–Mental State Examination; MoCA, Montreal Cognitive Assessment; ADL, activities of daily living; TC, total cholesterol; TG, triglyceride; HDL-C, high-density lipoprotein cholesterol; LDL-C, low-density lipoprotein cholesterol. Correlation analyses were performed using Spearman’s rank correlation. p < 0.05 was considered the statistical significance.
In AD group, ApoM and all the derived indicators were significantly negatively correlated with MMSE scores and MoCA scores, respectively (each p < 0.05), but each absolute value of correlation coefficient was low (<0.5). Except for ApoM/HDL-C ratio, ApoM and the other indicators were significantly positively correlated with ADL scores (each p < 0.01), in which ApoM/TG ratio had the highest correlation coefficient (rs = 0.540). Furthermore, we found that plasma ApoM and ApoM/HDL-C ratio were significantly positively correlated with the CDR sum of boxes (CDR-SB) total scores (Figure 3).
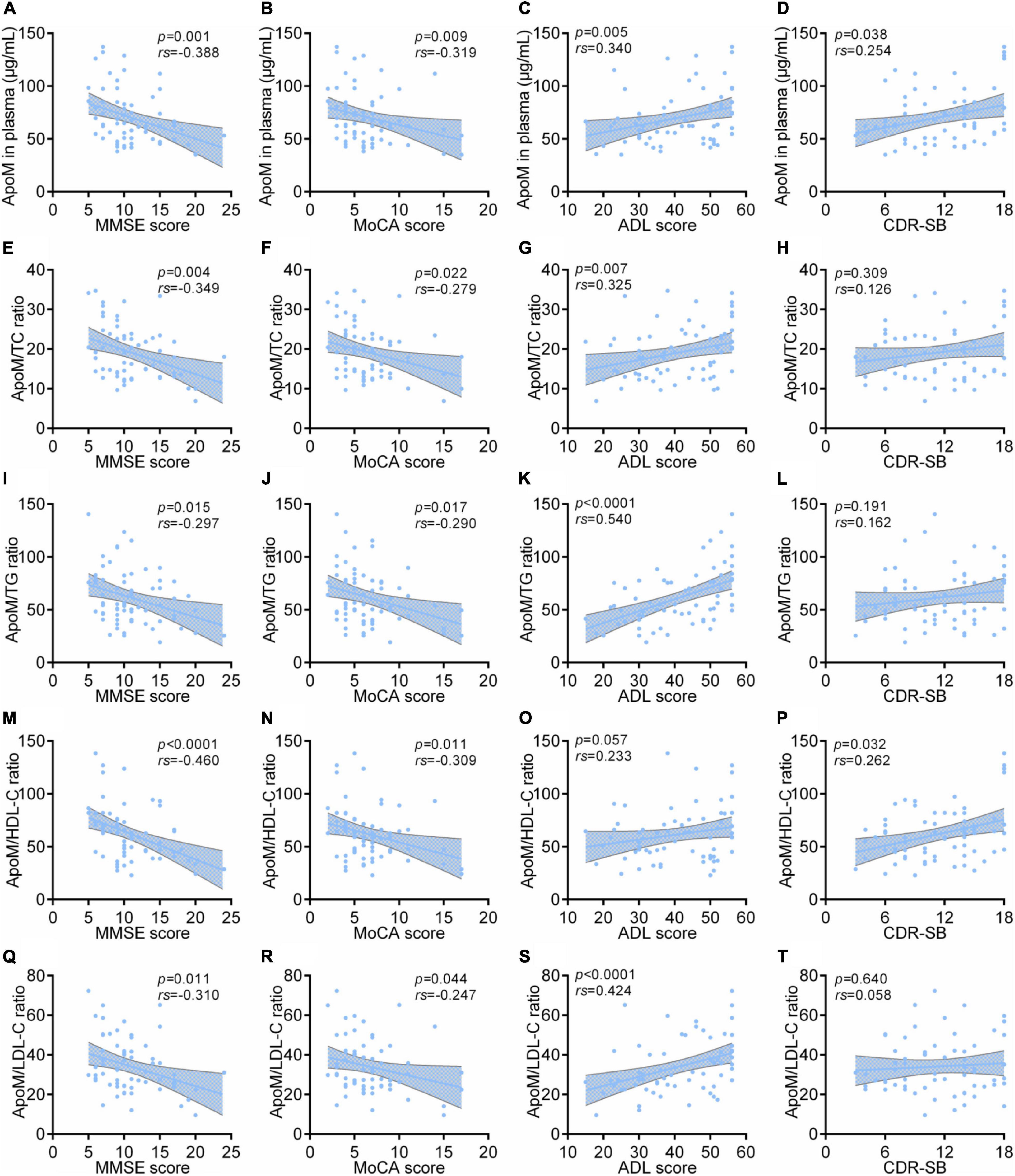
Figure 3. Correlations between plasma ApoM and its derived indicators with clinical characteristics of AD in AD group. The correlations between plasma ApoM with MMSE score (A), MoCA score (B), ADL score (C) and CDR-SB (D). The correlations between ApoM/TC ratio with MMSE score (E), MoCA score (F), ADL score (G) and CDR-SB (H). The correlations between ApoM/TG ratio with MMSE score (I), MoCA score (J), ADL score (K) and CDR-SB (L). The correlations between ApoM/HDL-C ratio with MMSE score (M), MoCA score (N), ADL score (O) and CDR-SB (P). The correlations between ApoM/LDL-C ratio with MMSE score (Q), MoCA score (R), ADL score (S) and CDR-SB (T). ApoM, apolipoprotein M; AD, Alzheimer’s disease; MMSE, Mini–Mental State Examination; MoCA, Montreal Cognitive Assessment; ADL, activities of daily living; CDR-SB, Clinical Dementia Rating Sum of Boxes; TC, total cholesterol; TG, triglyceride; HDL-C, high-density lipoprotein cholesterol; LDL-C, low-density lipoprotein cholesterol. Correlation analyses were performed using Spearman’s rank correlation. p < 0.05 was considered the statistical significance.
We performed the subgroup analyses of all participants according to their APOE 4 carriage status and sex, respectively. Among APOE 4-negative participants (n = 105), ApoM and all the derived indicators were significantly negatively correlated with MMSE scores (each p < 0.01) and MoCA scores (each p < 0.0001), and markedly positively correlated with ADL scores (each p < 0.0001), respectively (Supplementary Figure 2). ApoM/TG ratio performed best in the analysis with MMSE scores (rs = –0.540) and with MoCA scores (rs = –0.569). Both ApoM/TG ratio (rs = 0.585) and ApoM/TC ratio (rs = 0.507) performed better in the analysis with ADL scores (Supplementary Figure 2). Moreover, the trend of each correlation analysis results in APOE 4-positive participants (n = 35) was consistent with that of APOE 4-negative of statistical significance (each p < 0.05) (Supplementary Figure 2). In detail, the ratios of ApoM/TC (rs = –0.553), ApoM/HDL-C (rs = –0.534), and ApoM/LDL-C (rs = –0.529) performed better in the analysis with MMSE scores, the ApoM/TC ratio got the best correlation coefficient in the analysis with MoCA scores (rs = –0.522), and the ratios of ApoM/TC (rs = 0.537), ApoM/TG (rs = 0.572), and ApoM/LDL-C (rs = 0.578) had better correlations with ADL scores (Supplementary Figure 2). In addition, we conducted subgroup analyses by sex. Interestingly, the correlation between ApoM and four derived indicators with clinical characteristics of AD still remained (Supplementary Figure 3).
Correlations Between Plasma Apolipoprotein M With Representative Blood Biomarkers of Alzheimer’s Disease
Among all participants, ApoM and four derived indicators were significantly positively correlated with plasma levels of p-tau217 and NfL, respectively (each p < 0.0001), in which ApoM had the highest correlation coefficient in the analysis with p-tau217 (rs = 0.540), but each of the other correlation coefficient was low (<0.5) (Figure 4). In AD group, ApoM and all the derived indicators were significantly positively correlated with plasma p-tau217 (each p ≤ 0.01) and NfL (each p ≤ 0.001), but all the correlation coefficients were low (<0.5) except that of ApoM with NfL (rs = 0.507) (Figure 5).
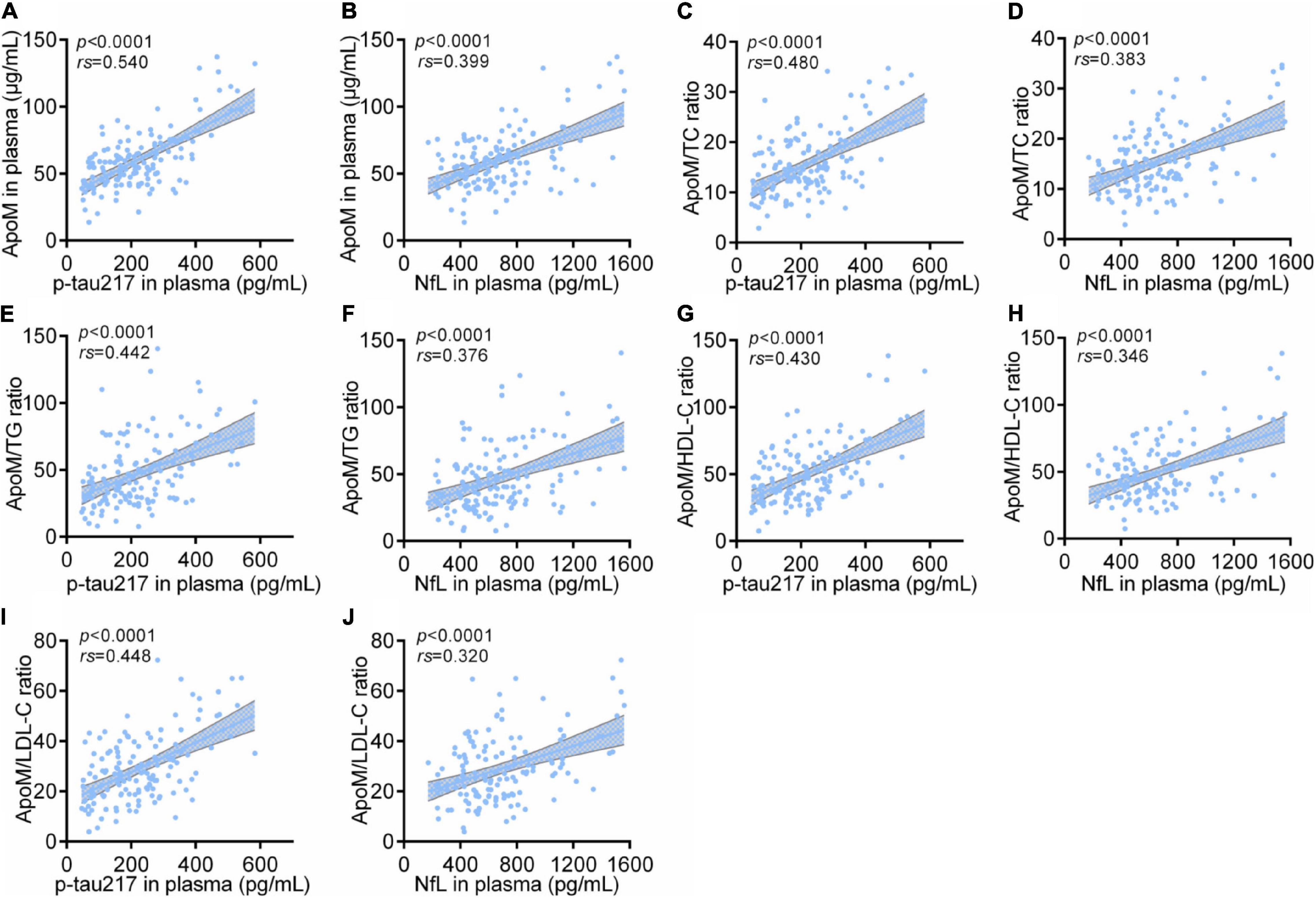
Figure 4. Correlations between plasma ApoM and its derived indicators with representative blood biomarkers of AD among all participants. The correlations between plasma ApoM with plasma p-tau217 (A) and NfL (B). The correlations between ApoM/TC ratio with plasma p-tau217 (C) and NfL (D). The correlations between ApoM/TG ratio with plasma p-tau217 (E) and NfL (F). The correlations between ApoM/HDL-C ratio with plasma p-tau217 (G) and NfL (H). The correlations between ApoM/LDL-C ratio with plasma p-tau217 (I) and NfL (J). ApoM, apolipoprotein M; p-tau217, tau phosphorylated at threonine 217; NfL, neurofilament light chain; CN, cognitively normal; AD, Alzheimer’s disease; TC, total cholesterol; TG, triglyceride; HDL-C, high-density lipoprotein cholesterol; LDL-C, low-density lipoprotein cholesterol. Correlation analysis was performed with Spearman’s rank correlation. P < 0.05 was considered the statistical significance.
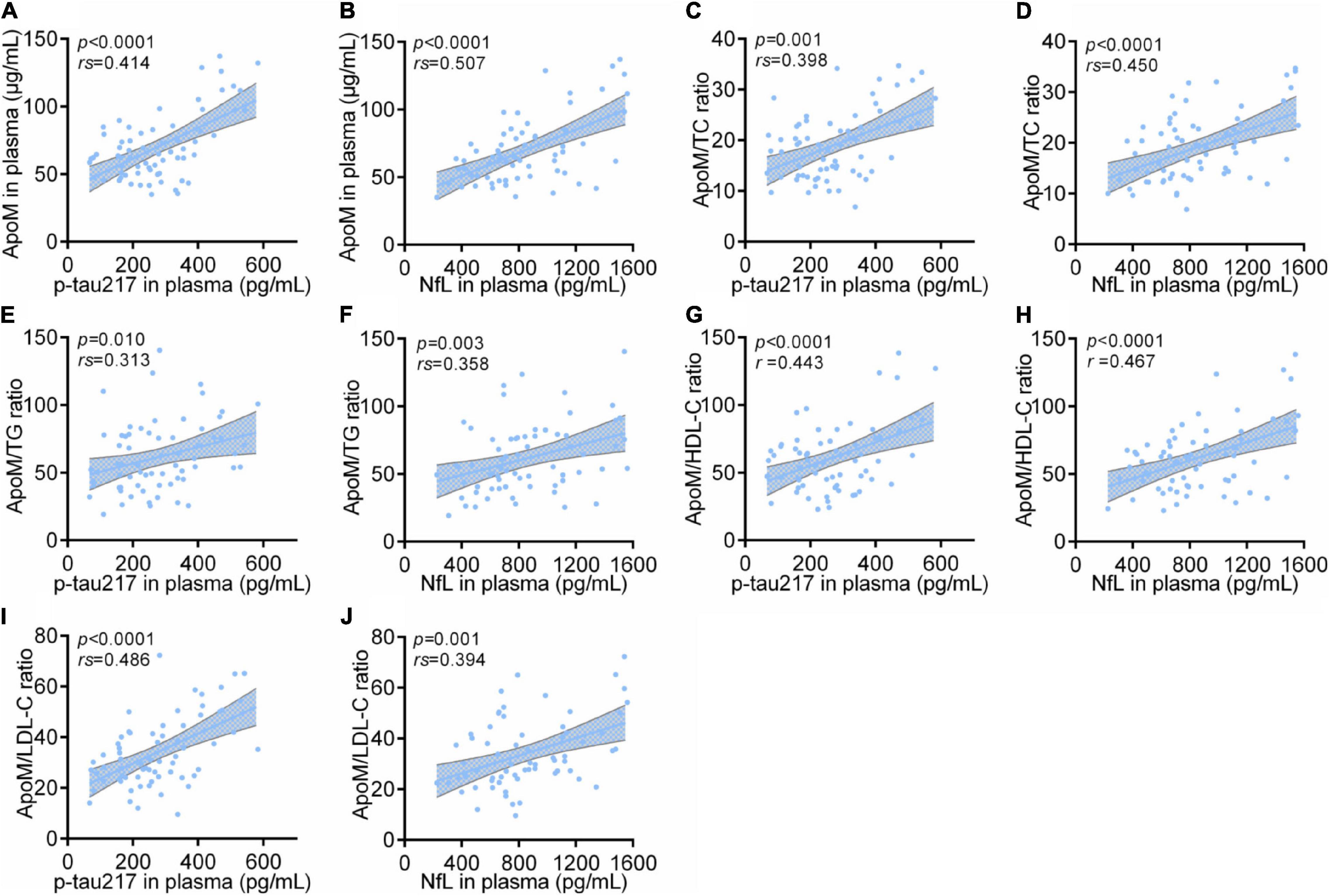
Figure 5. Correlations between plasma ApoM and its derived indicators with representative blood biomarkers of AD in AD group. ApoM, apolipoprotein M; p-tau217, tau phosphorylated at threonine 217; NfL, neurofilament light chain; CN, cognitively normal; AD, Alzheimer’s disease; TC, total cholesterol; TG, triglyceride; HDL-C, high-density lipoprotein cholesterol; LDL-C, low-density lipoprotein cholesterol. Correlation analyses were performed using Spearman’s rank correlation (A–F,I,J) or Pearson’s correlation (G,H) as appropriate. P < 0.05 was considered the statistical significance.
The trend of each correlation analysis results among APOE 4-negative participants (n = 105) was consistent with that in AD group of statistical significance (each p < 0.0001 in analysis with p-tau217 and each p < 0.05 in analysis with NfL) (Supplementary Figure 4). In detail, all the correlation coefficients were low (<0.5) except that of ApoM with p-tau217 (rs = 0.535) (Supplementary Figure 4). Except for ApoM/HDL-C ratio, the other indicators had the same statistical trend among APOE 4-positive participants (n = 35) with p-tau217 (each p ≤ 0.01) and NfL (each p ≤ 0.05), respectively (Supplementary Figure 4). Specifically, the ratios of ApoM/TG (rs = 0.500) and ApoM/LDL-C (rs = 0.525) performed better in the analysis with p-tau217, and ApoM/LDL-C ratio got the highest correlation coefficient in the analysis with NfL (rs = 0.525) (Supplementary Figure 4). Moreover, we conducted subgroup analyses by sex. The correlations between ApoM and four derived indicators representative blood biomarkers of AD still remained (Supplementary Figure 5).
The Diagnostic Power of Plasma Apolipoprotein M for Alzheimer’s Disease
To analyze the performance of plasma ApoM and its derived indicators to distinguish patients with AD from CN controls, we performed a ROC analysis. Each indicator featured significantly high AUC, which far exceeded the random chance (AUC of 50%) (each p < 0.0001, AUCs ranged from 0.712 to 0.825) (Figure 6). DeLong’s test was used to compare the ROC curve of these five indicators mutually. The ApoM/TC ratio (ΔAUC = 0.056, p = 0.044) and ApoM/TG ratio (ΔAUC = 0.097, p = 0.011) had a statistically remarkably larger AUC than ApoM, respectively (Table 3).
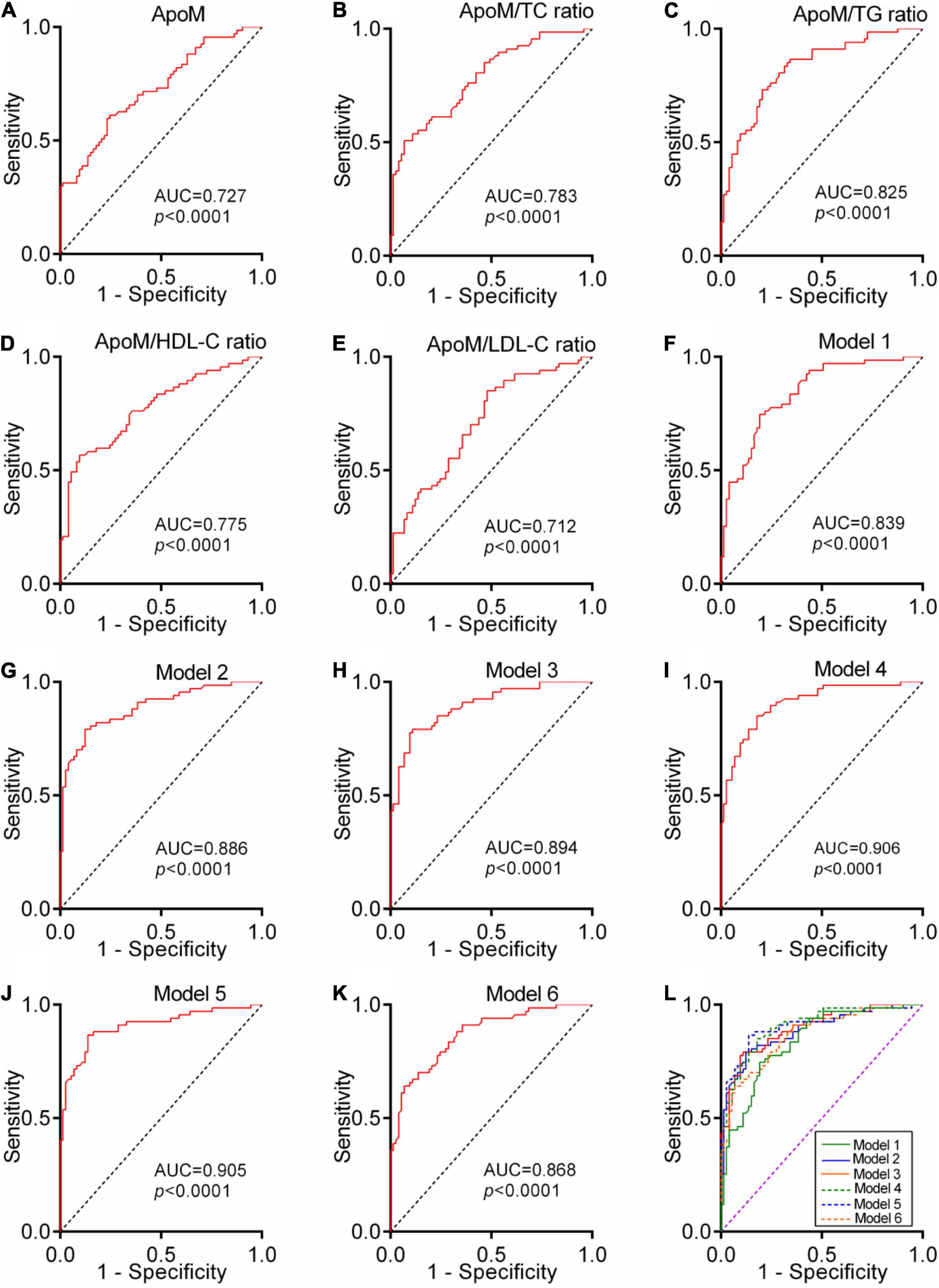
Figure 6. Diagnostic power of plasma ApoM and its derived indicators for AD. ROC analyses of ApoM (A), ApoM/TC ratio (B), ApoM/TG ratio (C), ApoM/HDL-C ratio (D) and ApoM/LDL-C ratio (E). Model 1: age, sex, APOE ε4, and BMI (F). Model 2: age, sex, APOE ε4, BMI, and ApoM (G). Model 3: age, sex, APOE ε4, BMI, and ApoM/TC ratio (H). Model 4: age, sex, APOE ε4, BMI, and ApoM/TG ratio (I). Model 5: age, sex, APOE ε4, BMI, and ApoM/HDL-C ratio (J). Model 6: age, sex, APOE ε4, BMI, and ApoM/LDL-C ratio (K). Comparison of ROC-AUCs of all the models using DeLong’s test (L). ApoM, apolipoprotein M; AD, Alzheimer’s disease; TC, total cholesterol; TG, triglyceride; HDL-C, high-density lipoprotein cholesterol; LDL-C, low-density lipoprotein cholesterol, BMI, body mass index; APOE, apolipoprotein E; ROC, receiver operating characteristic; AUC, area under the curve. P < 0.05 was considered the statistical significance.
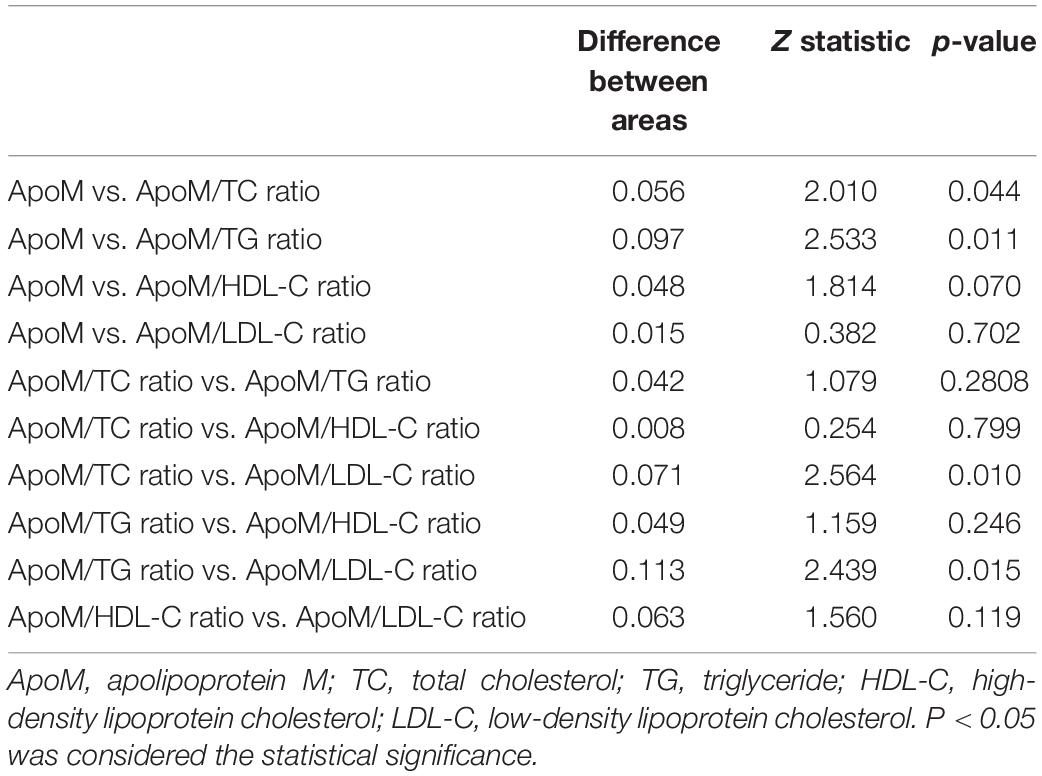
Table 3. Pairwise comparison of the area under the receiver operator characteristic curves by the DeLong’s test.
A ROC curve constructed by combining age, sex, APOE 4, and BMI got a significantly larger AUC than the random chance (Model 1, p < 0.0001, AUC = 0.839). The independent addition of ApoM and its derived indicators to Model 1 established Model 2–6 with a significantly larger AUC than the random chance, respectively (each p < 0.0001, AUCs ranged from 0.868 to 0.906) (Figure 6). DeLong’s test showed that Model 2–5 had statistically higher diagnostic power than Model 1, respectively (each p < 0.05) (Supplementary Table 1).
Discussion
In this multicenter, cross-sectional study, we found for the first time that plasma level of ApoM and its derived indicators (i.e., ratios of ApoM/TC, ApoM/TG, ApoM/HDL-C, and ApoM/LDL-C) were significantly higher in AD group than those in CN group, respectively. Then, we confirmed that plasma ApoM and its derived indicators were remarkably associated with the presence of AD, respectively. Subsequently, the correlations between plasma ApoM and its derived indicators with clinical characteristics (i.e., cognitive impairment and ADL) and representative blood biomarkers (i.e., p-tau217 and NfL) of AD were analyzed, respectively. Finally, we assessed the diagnostic power of plasma ApoM for AD and revealed that the majority of the four derived indicators performed individually better than ApoM. Meanwhile, the plasma ApoM and all the derived indicators could significantly enhance the diagnostic efficiency of the basic model of combining age, sex, APOE4 carriage status, and BMI, respectively. To our knowledge, this is the first time to reveal the association between plasma ApoM with AD so far.
In the past decades, the early diagnosis and the exploration of biomarkers have been the frontiers of AD research. The research framework proposed by NIA-AA in 2018 categorized the biomarkers of AD as A/T(N), where A represents Aβ deposition, T represents tau hyperphosphorylation and neurofibrillary tangle (NFT) formation, and N represents neuronal loss and neurodegeneration (Jack et al., 2018). This framework requires that the establishment of AD definitive diagnosis must be based on the evidence of brain Aβ aggregation (cerebrospinal fluid or PET test) (Jack et al., 2018). However, the high cost of invasive lumbar puncture and molecular imaging greatly limit their applications. Thus, blood-derived biomarkers are attracting increasing attention from clinicians and researchers (Thijssen and Rabinovici, 2021).
At present, both plasma p-tau217 and NfL are representative among all blood-based biomarkers of AD (Janelidze et al., 2021b). Additionally, a series of novel blood biomarkers and combinations of different biomarkers were also being developed and validated taking plasma p-tau217 and NfL as reference (Cullen et al., 2021; Palmqvist et al., 2021a). Thus, in this study, we measured the plasma levels of p-tau217 and NfL and further analyzed their correlations with ApoM and its derived indicators, respectively. All the results from total participants and subgroup analyses (sex and APOE4 carriage status) indicated that plasma ApoM had the potential to evaluate the severity of neurodegeneration of AD. The extensive studies on plasma p-tau217 have shown an excellent accuracy of p-tau217 in differentiating patients with AD and non-AD (Palmqvist et al., 2020; Palmqvist et al., 2021b; Thijssen et al., 2021). However, in this study, we found an overlap of plasma p-tau217 between the AD and CN groups. The previous studies have shown that biomarkers may vary with the stage and severity of AD, and the CSF p-tau as an early biomarker of downstream pathological changes was triggered by Aβ deposition (Sato et al., 2018). Research has shown different patterns of changes in CSF p-tau and tau-PET measures across the AD continuum. CSF levels of p-tau increased in the earliest disease stages (i.e., in asymptomatic individuals with CSF or PET evidence of abnormal Aβ accumulation) and appeared to reach a plateau or even decrease in later symptomatic stages of AD (Fagan et al., 2014; Mattsson-Carlgren et al., 2020a; Janelidze et al., 2021a). Considering the consistency of CSF and plasma p-tau (Barthélemy et al., 2020; Palmqvist et al., 2020; Janelidze et al., 2021a), it is speculated that the plasma p-tau217 may have a similar trajectory to CSF p-tau217. Most of the patients with AD in previous clinical studies involving plasma p-tau217 were at the stage of mild or moderate AD (Mattsson-Carlgren et al., 2020b,2021). However, many patients with AD in this study were moderate to severe AD (CDR scored 2–3), which indicates that the plasma p-tau217 might have entered a plateau or even declined, which could result in an overlap with CN group.
However, the role and related mechanism of ApoM in the pathogenesis of AD is uncovered. Reviewing the previously published literature, we proposed that ApoM might influence on Aβ metabolism and AD pathogenesis through the pathways as followings. First, sphingosine 1-phosphate (S1P), a metabolite of membrane sphingosine lipid, binds to the extracellular partners, is enriched in circulating fluid, and binds to G protein-coupled S1P receptors (S1PRs), which regulates the embryonic development and organ function (Cartier and Hla, 2019). It showed that immunoregulatory S1P had the potential as a biomarker for plasma diagnosis and differential diagnosis of AD (Chua et al., 2020). Postmortem study found that the level of brain S1P was reduced in patients with AD and significantly negatively correlated with the level of brain Aβ and hyperphosphorylated tau protein (He et al., 2010). Cell experiments showed that S1P was specifically bound to full-length BACE1 and increased its proteolytic activity. Reducing S1P generation in mouse neurons could reduce BACE1 activity level and Aβ production (Takasugi et al., 2011). It was also found that the accumulation of intracellular S1P disrupted the amyloid precursor protein (APP) metabolism. Inhibition of S1P lyase led to the accumulation of S1P, which impeded lysosomal degradation of APP, that leads to the accumulation of APP in cells and increase of APP amyloid fragment, and also reduced γ-secretase activity (Karaca et al., 2014). Nevertheless, in some neurodegenerative models, S1P has been shown to counteract the pro-apoptotic effect of neuramide by reducing oxidative stress and regulating the expression of pro-apoptotic and anti-apoptotic proteins in the Bcl-2 family (Czubowicz and Strosznajder, 2014). It was found that ApoM-bound S1P maintained the low level of permeability of paracellular blood–brain barrier (BBB) in all cerebral microvessels and low level of vesicle-mediated transport in the penetrating arterioles (Mathiesen Janiurek et al., 2019). In addition, S1P receptor 1 of cerebral endothelial cells maintained the BBB permeability in a size-selective manner by regulating the correct localization of tight junction protein (Yanagida et al., 2017). In addition, accumulation of S1P in neurons activated the microglia through S1P receptor 2, which leads to impaired autophagy and neuroinflammation (Karunakaran et al., 2019). The S1P axis in the nucleus might promote the transformation of microglia from M1 to M2 phenotype, thus regulating the neuroinflammation (Ji et al., 2019). Therefore, considering that ApoM is an important molecular chaperone of S1P, it stably binds and transports S1P in circulation and interstitial fluid, promoting the activation of receptor S1PRs and forming an important signal axis (Christoffersen et al., 2011), and ApoM is also involved in regulating the metabolism and transport of S1P (Christensen et al., 2017). These studies suggested that ApoM could regulate Aβ metabolism and AD pathogenesis through S1P and its receptors.
Second, the scavenger receptor class B type I (SR-BI) has been found in astrocytes and vascular smooth muscle cells and has been shown to mediate the adhesion of microglia to fibrillary Aβ. Microglia can directly uptake the aggregates of Aβ via scavenger receptors (Paresce et al., 1996). It showed that the reduction of SR-BI enhanced the deposition of fibrillary Aβ and cerebral amyloid angiopathy, impaired the response of perivascular macrophages to Aβ, and exacerbated the deficits of learning and memory (Thanopoulou et al., 2010). SR-BI keeps Aβ in a soluble state by blocking the entry of Aβ into endothelial cells, thus mediating the inhibition of Aβ-mediated monocyte adhesion to cerebrovascular endothelial cells by HDL (Robert et al., 2017). In addition, synaptic plasticity (long-term potentiation) in the CA1 region of hippocampus was found to be defective in very old SR-BI knockout mice, which also showed selective impairment in recognition and spatial memory (Chang et al., 2009). Thus, considering that ApoM is highly bound to SR-BI (Yao et al., 2020), it suggested that ApoM might regulate Aβ metabolism and AD pathogenesis through SR-BI.
Third, ApoM is widely distributed in lipoprotein in plasma, and most of it (about 95%) mainly binds to HDL, which plays a crucial role in the formation of pre-β-HDL and cholesterol efflux (Wolfrum et al., 2005). Although it is currently considered that low-level dysfunction of HDL is both risk factors for AD, the relationship and mechanism between HDL with Aβ metabolism and AD pathogenesis are still not elucidated (Stukas et al., 2014). A recent GWAS study preliminarily found that APOM gene was the main regulation gene of intracellular cholesterol that drove lipid metabolism pathway and thus increased the risk of AD, which suggests that ApoM may play an important role in AD (Kunkle et al., 2019). These findings suggested that ApoM could be a key in unlocking the link between HDL and AD.
Currently, ApoM is not a clinical blood lipid indicator. To improve the application value of ApoM in the clinical practice, we adopted the ratio of ApoM to clinically common blood lipid indicators based on our previous experience (Yang et al., 2021) and sought the more optimized blood biomarkers related to ApoM. The results showed that some of the four ApoM-derived indicators could perform better in their correlation analysis with cognitive function, abilities of daily living, and the representative blood biomarkers and also performed better in the diagnosis of AD compared with ApoM itself.
This study still has the following limitations: (1) As a cross-sectional clinical study, it only revealed the association between plasma ApoM and AD, but could not determine the causal relationship between them. (2) Due to the fact that most patients with AD were hospitalized for a long time and their families were not willing to undergo lumbar puncture and PET examination, the inclusion criteria for AD group in this study were based on the clinical criteria of NINCDS-ADRDA rather than the most popular biomarker-based criteria of NIA-AA. (3) The Eighth People’s Hospital of Chengdu is a chronic disease hospital and municipal sanatorium for cadres. Many patients with AD are long-term hospitalized, and a majority of them had only underwent the head CT scanning. In addition, the brain MRI data often lacked the coronal images to assess hippocampal atrophy. Thus, we did not determine the association between ApoM and neuroimaging. (4) Considering the sample size of this study, we did not carry out the external validation after diagnostic power evaluation.
Taken together, this study preliminarily uncovered that the plasma ApoM is correlated with the presence, clinical characteristics, and representative blood biomarkers of AD and has certain diagnostic power of AD. The role and related mechanism of ApoM on the pathogenesis of AD is worthy of further study in the future.
Data Availability Statement
The raw data supporting the conclusions of this article will be made available by the authors, without undue reservation.
Ethics Statement
The studies involving human participants were reviewed and approved by the Ethics Review Committee of Sichuan Provincial People’s Hospital. The patients/participants provided their written informed consent to participate in this study.
Author Contributions
W-dL, S-JY, and YX carried out the study conception and design. J-YX, XH, YS, H-SJ, JF, N-wY, F-QG, FY, and JX performed the material preparation, data collection, and analysis. J-YX and YX wrote the first draft of the manuscript. All authors commented on the previous versions of the manuscript and read and approved the final manuscript.
Funding
This study was supported by the National Natural Science Foundation of China (grant numbers: 81601112 and 81801090), Top Project of Youth Incubation Program of Military Medical Science and Technology (grant number: 19QNP065), and Sichuan Department of Science and Technology Fund (grant numbers: 2020YFS0436 and 2019YFS0213).
Conflict of Interest
The authors declare that the research was conducted in the absence of any commercial or financial relationships that could be construed as a potential conflict of interest.
Publisher’s Note
All claims expressed in this article are solely those of the authors and do not necessarily represent those of their affiliated organizations, or those of the publisher, the editors and the reviewers. Any product that may be evaluated in this article, or claim that may be made by its manufacturer, is not guaranteed or endorsed by the publisher.
Acknowledgments
We thank all the participants and their families for their commitments and statements to help advance research into clinical diagnosis of AD.
Supplementary Material
The Supplementary Material for this article can be found online at: https://www.frontiersin.org/articles/10.3389/fnagi.2022.838223/full#supplementary-material
Supplementary Figure 1 | The flow diagram for the enrollment of the two groups. MMSE, mini-mental state examination; MoCA, Montreal cognitive assessment; ADL, activities of daily living; CDR-SB, Clinical Dementia Rating; CT, Computed tomography; MRI, Magnetic resonance imaging; AD, Alzheimer’s disease; CN, cognitively normal.
Supplementary Figure 2 | Correlations between plasma ApoM and its-derived indicators with clinical characteristics of AD in subgroup analysis based on APOEε4 status. ApoM, apolipoprotein M; AD, Alzheimer’s disease; APOE, apolipoprotein E; MMSE, mini-mental state examination; MoCA, Montreal cognitive assessment; ADL, activities of daily living; TC, total cholesterol; TG, triglyceride; HDL-C, high-density lipoprotein cholesterol; LDL-C, low-density lipoprotein cholesterol. Correlation analyses were performed using Spearman’s rank correlation. P < 0.05 was considered the statistical significance.
Supplementary Figure 3 | Correlations between plasma ApoM and its-derived indicators with clinical characteristics of AD in subgroup analysis based on sex. ApoM, apolipoprotein M; AD, Alzheimer’s disease; MMSE, mini-mental state examination; MoCA, Montreal cognitive assessment; ADL, activities of daily living; TC, total cholesterol; TG, triglyceride; HDL-C, high-density lipoprotein cholesterol; LDL-C, low-density lipoprotein cholesterol. Correlation analyses were performed using Spearman’s rank correlation. P < 0.05 was considered the statistical significance.
Supplementary Figure 4 | Correlations between plasma ApoM and its-derived indicators with representative blood biomarkers of AD in subgroup analysis based on APOEε4 status. ApoM, apolipoprotein M; p-tau217, tau phosphorylated at threonine 217; NfL, neurofilament light chain; CN, cognitively normal; AD, Alzheimer’s disease; TC, total cholesterol; TG, triglyceride; HDL-C, high-density lipoprotein cholesterol; LDL-C, low-density lipoprotein cholesterol. Correlation analyses were performed using Spearman’s rank correlation or Pearson’s correlation as appropriate. P < 0.05 was considered the statistical significance.
Supplementary Figure 5 | Correlations between plasma ApoM and its-derived indicators with representative blood biomarkers of AD in subgroup analysis based on sex. ApoM, apolipoprotein M; p-tau217, tau phosphorylated at threonine 217; NfL, neurofilament light chain; CN, cognitively normal; AD, Alzheimer’s disease; TC, total cholesterol; TG, triglyceride; HDL-C, high-density lipoprotein cholesterol; LDL-C, low-density lipoprotein cholesterol. Correlation analyses were performed using Spearman’s rank correlation or Pearson’s correlation as appropriate. P < 0.05 was considered the statistical significance.
References
Alzheimer’s Association (2021). Alzheimer’s disease facts and figures. Alzheimers. Dement. 17, 327–406. doi: 10.1002/alz.12328
Barthélemy, N. R., Horie, K., Sato, C., and Bateman, R. J. (2020). Blood plasma phosphorylated-tau isoforms track CNS change in Alzheimer’s disease. J Exp Med. 217:e20200861. doi: 10.1084/jem.20200861
Belloy, M. E., Napolioni, V., and Greicius, M. D. (2019). A Quarter Century of APOE and Alzheimer’s Disease: progress to Date and the Path Forward. Neuron 101, 820–838. doi: 10.1016/j.neuron.2019.01.056
Cartier, A., and Hla, T. (2019). Sphingosine 1-phosphate: lipid signaling in pathology and therapy. Science 366:eaar5551. doi: 10.1126/science.aar5551
Chang, E. H., Rigotti, A., and Huerta, P. T. (2009). Age-related influence of the HDL receptor SR-BI on synaptic plasticity and cognition. Neurobiol. Aging 30, 407–419. doi: 10.1016/j.neurobiolaging.2007.07.006
Chernick, D., Zhong, R., and Li, L. (2020). The Role of HDL and HDL Mimetic Peptides as Potential Therapeutics for Alzheimer’s Disease. Biomolecules 10:1276. doi: 10.3390/biom10091276
Christensen, P. M., Bosteen, M. H., Hajny, S., Nielsen, L. B., and Christoffersen, C. (2017). Apolipoprotein M mediates sphingosine-1-phosphate efflux from erythrocytes. Sci. Rep. 7:14983. doi: 10.1038/s41598-017-15043-y
Christoffersen, C., Obinata, H., Kumaraswamy, S. B., Galvani, S., Ahnstrom, J., Sevvana, M., et al. (2011). Endothelium-protective sphingosine-1-phosphate provided by HDL-associated apolipoprotein M. Proc. Natl. Acad. Sci. U. S. A. 108, 9613–9618. doi: 10.1073/pnas.1103187108
Chua, X. Y., Chai, Y. L., Chew, W. S., Chong, J. R., Ang, H. L., Xiang, P., et al. (2020). Immunomodulatory sphingosine-1-phosphates as plasma biomarkers of Alzheimer’s disease and vascular cognitive impairment. Alzheimers. Res. Ther. 12:122. doi: 10.1186/s13195-020-00694-3
Cullen, N. C., Leuzy, A., Janelidze, S., Palmqvist, S., Svenningsson, A. L., Stomrud, E., et al. (2021). Plasma biomarkers of Alzheimer’s disease improve prediction of cognitive decline in cognitively unimpaired elderly populations. Nat. Commun. 12:3555. doi: 10.1038/s41467-021-23746-0
Czubowicz, K., and Strosznajder, R. (2014). Ceramide in the molecular mechanisms of neuronal cell death. The role of sphingosine-1-phosphate. Mol. Neurobiol. 50, 26–37. doi: 10.1007/s12035-013-8606-4
Deitcher, S. R. (2002). Interpretation of the international normalised ratio in patients with liver disease. Lancet 359, 47–48. doi: 10.1016/S0140-6736(02)07282-3
Fagan, A. M., Xiong, C., Jasielec, M. S., Bateman, R. J., Goate, A. M., Benzinger, T. L. S., et al. (2014). Longitudinal change in CSF biomarkers in autosomal-dominant Alzheimer’s disease. Sci. Transl. Med. 6:226ra230. doi: 10.1126/scitranslmed.3007901
He, X., Huang, Y., Li, B., Gong, C. X., and Schuchman, E. H. (2010). Deregulation of sphingolipid metabolism in Alzheimer’s disease. Neurobiol. Aging. 31, 398–408. doi: 10.1016/j.neurobiolaging.2008.05.010
Hu, H., Tan, L., Bi, Y. L., Xu, W., Tan, L., Shen, X. N., et al. (2020). Association of serum Apolipoprotein B with cerebrospinal fluid biomarkers of Alzheimer’s pathology. Ann. Clin. Transl. Neurol. 7, 1766–1778. doi: 10.1002/acn3.51153
Jack, C. R., Bennett, D. A., Blennow, K., Carrillo, M. C., Dunn, B., Haeberlein, S. B., et al. (2018). NIA-AA Research Framework: toward a biological definition of Alzheimer’s disease. Alzheimers. Dement. 14, 535–562. doi: 10.1016/j.jalz.2018.02.018
Janelidze, S., Palmqvist, S., Leuzy, A., Stomrud, E., Verberk, I. M. W., Zetterberg, H., et al. (2021b). Detecting amyloid positivity in early Alzheimer’s disease using combinations of plasma Abeta42/Abeta40 and p-tau. Alzheimers. Dement. Epub online ahead of print. doi: 10.1002/alz.12395
Janelidze, S., Berron, D., Smith, R., Strandberg, O., Proctor, N. K., Dage, J. L., et al. (2021a). Associations of Plasma Phospho-Tau217 Levels With Tau Positron Emission Tomography in Early Alzheimer Disease. JAMA Neurol. 78, 149–156. doi: 10.1001/jamaneurol.2020.4201
Ji, J., Wang, J., Yang, J., Wang, X. P., Huang, J. J., Xue, T. F., et al. (2019). The Intra-nuclear SphK2-S1P Axis Facilitates M1-to-M2 Shift of Microglia via Suppressing HDAC1-Mediated KLF4 Deacetylation. Front. Immunol. 10:1241. doi: 10.3389/fimmu.2019.01241
Karaca, I., Tamboli, I. Y., Glebov, K., Richter, J., Fell, L. H., Grimm, M. O., et al. (2014). Deficiency of sphingosine-1-phosphate lyase impairs lysosomal metabolism of the amyloid precursor protein. J. Biol. Chem. 289, 16761–16772. doi: 10.1074/jbc.M113.535500
Karunakaran, I., Alam, S., Jayagopi, S., Frohberger, S. J., Hansen, J. N., Kuehlwein, J., et al. (2019). Neural sphingosine 1-phosphate accumulation activates microglia and links impaired autophagy and inflammation. Glia 67, 1859–1872. doi: 10.1002/glia.23663
Khoonsari, P. E., Haggmark, A., Lonnberg, M., Mikus, M., Kilander, L., Lannfelt, L., et al. (2016). Analysis of the Cerebrospinal Fluid Proteome in Alzheimer’s Disease. PLoS One 11:e0150672. doi: 10.1371/journal.pone.0150672
Knopman, D. S., Amieva, H., Petersen, R. C., Chetelat, G., Holtzman, D. M., Hyman, B. T., et al. (2021). Alzheimer disease. Nat. Rev. Dis. Primers. 7:33. doi: 10.1038/s41572-021-00269-y
Kober, A. C., Manavalan, A. P. C., Tam-Amersdorfer, C., Holmer, A., Saeed, A., Fanaee-Danesh, E., et al. (2017). Implications of cerebrovascular ATP-binding cassette transporter G1 (ABCG1) and apolipoprotein M in cholesterol transport at the blood-brain barrier. Biochim. Biophys. Acta Mol. Cell. Biol. Lipids 1862, 573–588. doi: 10.1016/j.bbalip.2017.03.003
Kunkle, B. W., Grenier-Boley, B., Sims, R., Bis, J. C., Damotte, V., Naj, A. C., et al. (2019). Genetic meta-analysis of diagnosed Alzheimer’s disease identifies new risk loci and implicates Abeta, tau, immunity and lipid processing. Nat. Genet. 51, 414–430. doi: 10.1038/s41588-019-0358-2
Kurano, M., Tsukamoto, K., Shimizu, T., Kassai, H., Nakao, K., Aiba, A., et al. (2020). Protection Against Insulin Resistance by Apolipoprotein M/Sphingosine-1-Phosphate. Diabetes 69, 867–881. doi: 10.2337/db19-0811
Levey, A. I. (2021). Progress with Treatments for Alzheimer’s Disease. N. Engl. J. Med. 384, 1762–1763. doi: 10.1056/NEJMe2103722
Livingston, G., Huntley, J., Sommerlad, A., Ames, D., Ballard, C., Banerjee, S., et al. (2020). Dementia prevention, intervention, and care: 2020 report of the Lancet Commission. Lancet 396, 413–446. doi: 10.1016/s0140-6736(20)30367-6
Mathiesen Janiurek, M., Soylu-Kucharz, R., Christoffersen, C., Kucharz, K., and Lauritzen, M. (2019). Apolipoprotein M-bound sphingosine-1-phosphate regulates blood-brain barrier paracellular permeability and transcytosis. Elife 8:e49405. doi: 10.7554/eLife.49405
Mattsson-Carlgren, N., Andersson, E., Janelidze, S., Ossenkoppele, R., Insel, P., Strandberg, O., et al. (2020a). Aβ deposition is associated with increases in soluble and phosphorylated tau that precede a positive Tau PET in Alzheimer’s disease. Sci. Adv. 6:eaaz2387. doi: 10.1126/sciadv.aaz2387
Mattsson-Carlgren, N., Janelidze, S., Palmqvist, S., Cullen, N., Svenningsson, A. L., Strandberg, O., et al. (2020b). Longitudinal plasma p-tau217 is increased in early stages of Alzheimer’s disease. Brain 143, 3234–3241. doi: 10.1093/brain/awaa286
Mattsson-Carlgren, N., Janelidze, S., Bateman, R. J., Smith, R., Stomrud, E., Serrano, G. E., et al. (2021). Soluble P-tau217 reflects amyloid and tau pathology and mediates the association of amyloid with tau. EMBO Mol. Med. 13:e14022. doi: 10.15252/emmm.202114022
McKhann, G., Drachman, D., Folstein, M., Katzman, R., Price, D., and Stadlan, E. M. (1984). Clinical diagnosis of Alzheimer’s disease: report of the NINCDS-ADRDA Work Group under the auspices of Department of Health and Human Services Task Force on Alzheimer’s Disease. Neurology 34, 939–944. doi: 10.1212/wnl.34.7.939
Morris, J. C. (1993). The Clinical Dementia Rating (CDR): current version and scoring rules. Neurology 43, 2412–2414. doi: 10.1212/wnl.43.11.2412-a
Palmqvist, S., Janelidze, S., Quiroz, Y. T., Zetterberg, H., Lopera, F., Stomrud, E., et al. (2020). Discriminative Accuracy of Plasma Phospho-tau217 for Alzheimer Disease vs Other Neurodegenerative Disorders. JAMA 324, 772–781. doi: 10.1001/jama.2020.12134
Palmqvist, S., Tideman, P., Cullen, N., Zetterberg, H., Blennow, K., Alzheimer’s Disease Neuroimaging, I., et al. (2021a). Prediction of future Alzheimer’s disease dementia using plasma phospho-tau combined with other accessible measures. Nat. Med. 27, 1034–1042. doi: 10.1038/s41591-021-01348-z
Palmqvist, S., Tideman, P., Cullen, N., Zetterberg, H., Blennow, K., Dage, J. L., et al. (2021b). Prediction of future Alzheimer’s disease dementia using plasma phospho-tau combined with other accessible measures. Nat. Med. 27, 1034–1042.
Paresce, D. M., Ghosh, R. N., and Maxfield, F. R. (1996). Microglial cells internalize aggregates of the Alzheimer’s disease amyloid beta-protein via a scavenger receptor. Neuron 17, 553–565. doi: 10.1016/s0896-6273(00)80187-7
Robert, J., Button, E. B., Stukas, S., Boyce, G. K., Gibbs, E., Cowan, C. M., et al. (2017). High-density lipoproteins suppress Abeta-induced PBMC adhesion to human endothelial cells in bioengineered vessels and in monoculture. Mol. Neurodegener. 12:60. doi: 10.1186/s13024-017-0201-0
Sato, C., Barthélemy, N. R., Mawuenyega, K. G., Patterson, B. W., Gordon, B. A., Jockel-Balsarotti, J., et al. (2018). Tau Kinetics in Neurons and the Human Central Nervous System. Neuron 97, 1284–1298.e7. doi: 10.1016/j.neuron.2018.02.015
Shim, K. H., Kang, M. J., Suh, J. W., Pyun, J. M., Ryoo, N., Park, Y. H., et al. (2020). CSF total tau/alpha-synuclein ratio improved the diagnostic performance for Alzheimer’s disease as an indicator of tau phosphorylation. Alzheimers. Res. Ther. 12:83. doi: 10.1186/s13195-020-00648-9
Sramkova, V., Berend, S., Siklova, M., Caspar-Bauguil, S., Carayol, J., Bonnel, S., et al. (2019). Apolipoprotein M: a novel adipokine decreasing with obesity and upregulated by calorie restriction. Am. J. Clin. Nutr. 109, 1499–1510. doi: 10.1093/ajcn/nqy331
Stukas, S., Robert, J., and Wellington, C. L. (2014). High-density lipoproteins and cerebrovascular integrity in Alzheimer’s disease. Cell. Metab. 19, 574–591. doi: 10.1016/j.cmet.2014.01.003
Sun, R., Wang, H., Shi, Y., Sun, Z., Jiang, H., and Zhang, J. (2020). Changes in the Morphology, Number, and Pathological Protein Levels of Plasma Exosomes May Help Diagnose Alzheimer’s Disease. J. Alzheimers. Dis. 73, 909–917. doi: 10.3233/JAD-190497
Takasugi, N., Sasaki, T., Suzuki, K., Osawa, S., Isshiki, H., Hori, Y., et al. (2011). BACE1 activity is modulated by cell-associated sphingosine-1-phosphate. J. Neurosci. 31, 6850–6857. doi: 10.1523/JNEUROSCI.6467-10.2011
Thanopoulou, K., Fragkouli, A., Stylianopoulou, F., and Georgopoulos, S. (2010). Scavenger receptor class B type I (SR-BI) regulates perivascular macrophages and modifies amyloid pathology in an Alzheimer mouse model. Proc. Natl. Acad. Sci. U. S. A. 107, 20816–20821. doi: 10.1073/pnas.1005888107
Thijssen, E. H., La Joie, R., Strom, A., Fonseca, C., Iaccarino, L., Wolf, A., et al. (2021). Plasma phosphorylated tau 217 and phosphorylated tau 181 as biomarkers in Alzheimer’s disease and frontotemporal lobar degeneration: a retrospective diagnostic performance study. Lancet Neurol. 20, 739–752. doi: 10.1016/s1474-4422(21)00214-3
Thijssen, E. H., and Rabinovici, G. D. (2021). Rapid Progress Toward Reliable Blood Tests for Alzheimer Disease. JAMA Neurol. 78, 143–145. doi: 10.1001/jamaneurol.2020.4200
Versmissen, J., Vongpromek, R., Yahya, R., van der Net, J. B., van Vark-van der Zee, L., Blommesteijn-Touw, J., et al. (2016). Familial hypercholesterolaemia: cholesterol efflux and coronary disease. Eur. J. Clin. Invest. 46, 643–650. doi: 10.1111/eci.12643
Wolfrum, C., Poy, M. N., and Stoffel, M. (2005). Apolipoprotein M is required for prebeta-HDL formation and cholesterol efflux to HDL and protects against atherosclerosis. Nat. Med. 11, 418–422. doi: 10.1038/nm1211
Xu, N., and Dahlbäck, B. (1999). A novel human apolipoprotein (apoM). J. Biol. Chem. 274, 31286–31290. doi: 10.1074/jbc.274.44.31286
Yanagida, K., Liu, C. H., Faraco, G., Galvani, S., Smith, H. K., Burg, N., et al. (2017). Size-selective opening of the blood-brain barrier by targeting endothelial sphingosine 1-phosphate receptor 1. Proc. Natl. Acad. Sci. U. S. A. 114, 4531–4536. doi: 10.1073/pnas.1618659114
Yang, C., Xin, J. Y., Liu, Z. L., Fan, F., Li, Y. M., Jin, F., et al. (2021). Association Between Serum C1q Tumor Necrosis Factor-Related Protein 9 and the Clinical Characteristics and Prognosis of Ischemic Stroke. Neurol Ther. 11, 87–101. doi: 10.1007/s40120-021-00296-7
Yao, S., Luo, G., Liu, H., Zhang, J., Zhan, Y., Xu, N., et al. (2020). Apolipoprotein M promotes the anti-inflammatory effect of high-density lipoprotein by binding to scavenger receptor BI. Ann. Transl. Med. 8:1676. doi: 10.21037/atm-20-7008
Zhang, B., Yu, H. X., Zhi, N., Cui, C., Han, Y. Y., Hu, M., et al. (2021). Association of HMOX-1 with sporadic Alzheimer’s disease in southern Han Chinese. Eur. J. Neurol. 28, 2922–2926. doi: 10.1111/ene.14870
Zhang, P., Gao, J., Pu, C., Feng, G., Wang, L., Huang, L., et al. (2017). ApoM/HDL-C and apoM/apoA-I ratios are indicators of diabetic nephropathy in healthy controls and type 2 diabetes mellitus. Clin. Chim. Acta 466, 31–37. doi: 10.1016/j.cca.2017.01.006
Keywords: Alzheimer’s disease, apolipoprotein M, neurofilament light chain, tau, biomarker
Citation: Xin J-Y, Huang X, Sun Y, Jiang H-S, Fan J, Yu N-w, Guo F-Q, Ye F, Xiao J, Le W-d, Yang S-J and Xiang Y (2022) Association Between Plasma Apolipoprotein M With Alzheimer’s Disease: A Cross-Sectional Pilot Study From China. Front. Aging Neurosci. 14:838223. doi: 10.3389/fnagi.2022.838223
Received: 17 December 2021; Accepted: 17 February 2022;
Published: 18 March 2022.
Edited by:
Yanxing Chen, Zhejiang University, ChinaCopyright © 2022 Xin, Huang, Sun, Jiang, Fan, Yu, Guo, Ye, Xiao, Le, Yang and Xiang. This is an open-access article distributed under the terms of the Creative Commons Attribution License (CC BY). The use, distribution or reproduction in other forums is permitted, provided the original author(s) and the copyright owner(s) are credited and that the original publication in this journal is cited, in accordance with accepted academic practice. No use, distribution or reproduction is permitted which does not comply with these terms.
*Correspondence: Shao-Jie Yang, yldbwdx2005@hotmail.com; Yang Xiang, xiangyangttt@126.com