The role of long noncoding RNAs as regulators of the epithelial–Mesenchymal transition process in oral squamous cell carcinoma cells
- 1Xiangya School of Stomatology, Central South University, Changsha, Hunan, China
- 2Hunan Clinical Research Center of Oral Major Diseases and Oral Health and Xiangya Stomatological Hospital, Changsha, China
Oral squamous cell carcinoma (OSCC) is a highly invasive and relatively prevalent cancer, accounting for around 3% of all cancers diagnosed. OSCC is associated with bad outcomes, with only 50% overall survival (OS) after five years. The ability of OSCC to invade local and distant tissues relies on the induction of the epithelial–mesenchymal transition (EMT), wherein epithelial cells shed their polarity and cell-to-cell contacts and acquire mesenchymal characteristics. Consequently, a comprehensive understanding of how tumor cell EMT induction is regulated has the potential of direct attempts to prevent tumor progression and metastasis, resulting in better patient outcomes. Several recent studies have established the significance of particular long noncoding RNAs (lncRNAs) in the context of EMT induction. Moreover, lncRNAs regulate a vast array of oncogenic pathways. With a focus on the mechanisms by which the underlined lncRNAs shape the metastatic process and a discussion of their potential utility as clinical biomarkers or targets for therapeutic intervention in patients with OSCC, the present review thus provides an overview of the EMT-related lncRNAs that are dysregulated in OSCC.
Introduction
In both developed and developing countries, cancer is by far the leading cause of mortality. Cancer remains a chronic risk to public health worldwide, with an estimated 19.3 million diagnoses and 9.9 million cancer-associated deaths in 2020 alone (Sung et al., 2021). Oral cancer is the 18th most prevalent tumor subtype, with approximately 370,000 patients diagnosed in 2020 (Sung et al., 2021) and over 300,000 new diagnoses annually, together with 145,000 cases of oral cancer-related mortality (Thomson, 2018). Oral squamous cell carcinoma (OSCC) represents more than 90% of all oral tumors (Ali et al., 2017). Mid-southern Asia has extremely high OSCC incidence rates; however, in northern and eastern Europe, an elevated OSCC incidence rate was also recorded (Johnson et al., 2020). The majority of OSCC treatment options involve a combination of surgery, radiotherapy, and chemotherapy. The 5-year OS of affected individuals is ∼50% due to the rapid advancement of the metastatic disease. In this view, the mechanism underlying OSCC tumor metastasis must be elucidated in more detail to improve the prognostic assessment of affected individuals (Chi et al., 2015).
Numerous microarray- and RNA-seq-based studies have revealed the dysregulation of lncRNA expression in various types of carcinomas (Huarte, 2015). Notably, many of these lncRNAs have been shown to play critical regulatory roles in the induction of EMT in tumor cells, thus supporting metastatic growth (Liu et al., 2020). The present review thus provides an overview of the molecular mechanisms linking lncRNAs and EMT induction in OSCC.
Role of epithelial–Mesenchymal transition induction in tumor invasion and metastasis
EMT is a complex process by which the epithelial-derived cells lose their polarity and associated features and adopt mesenchymal-like properties. The EMT is broadly classified into three types that are involved in embryogenesis, wound healing/tissue fibrosis, and the malignant progression of epithelial cell-derived tumors (Kalluri and Weinberg, 2009). During embryo development, tight junctions and other structures, including desmosomes, gap junctions, and adheren junctions, link epithelial cells to the basement membrane (Yang and Weinberg, 2008). Eventually, however, these cells lose their polarity and, when developmentally mature, become more motile and invasive (Lim and Thiery, 2012). This same process can also occur in tumor cells of epithelial origin. They detach from the basement membrane and enter the systemic circulation, where they can start metastatic tumor growth in other parts of the body (Figure 1) (Horejs, 2016). This process is characterized by a decrease in the expression levels of marker genes such as E-cadherin. It is transcriptionally controlled by proteins including Zeb, Twist, Snail, and FoxC2. This increases or suppresses the E-cadherin expression while activating various related signaling pathways such as the Notch, Wnt, NF-κB, and TGF-β pathways (Lamouille et al., 2014; Dongre and Weinberg, 2019).
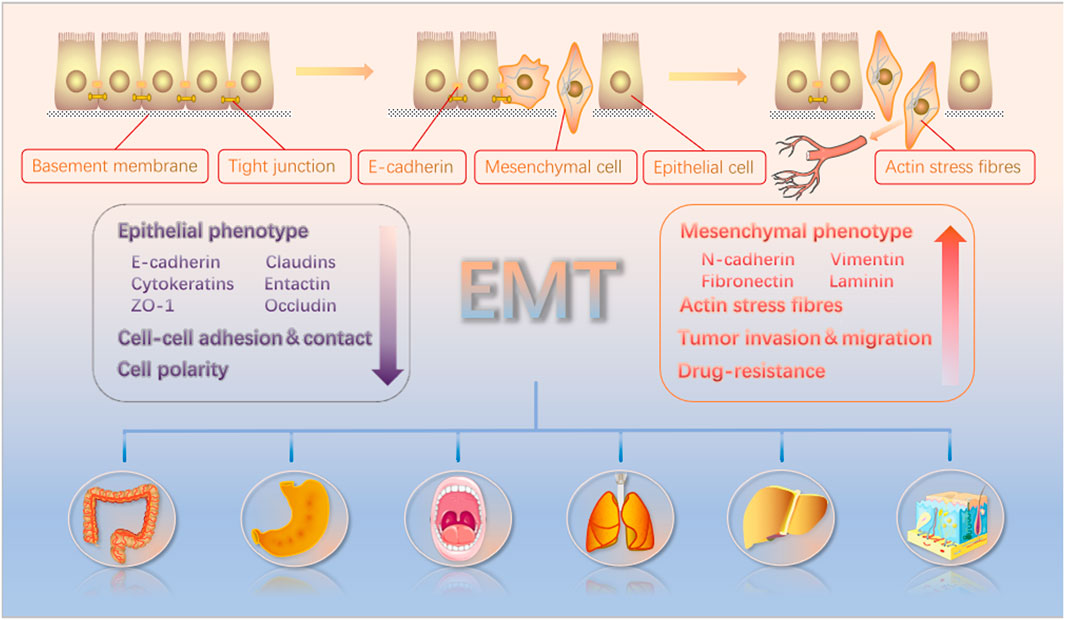
FIGURE 1. EMT causes polarized epithelial cells to acquire characteristics similar to motile mesenchymal cells. These cells can then detach from the basement membrane and disseminate from the whole body through the bloodstream. The EMT induction results in enhanced tumor cell invasion, migration, and resistance to drug treatment. Furthermore, epithelial and mesenchymal biomarkers and their changes in the context of EMT induction are noted in the figure.
Function-based significance of lncRNAs as regulators of the epithelia–Mesenchymal transition induction in oral squamous cell carcinoma cells
Noncoding RNAs (ncRNAs) are separated into different length-based categories (Esteller, 2011), with short (18–25 nt) microRNAs (miRNAs) being capable of regulating over half of all of the genes expressed in mammals (Simonson and Das, 2015). In contrast, lncRNAs comprised of >200 nucleotides can control biological processes through multiple distinct mechanisms. For instance, some lncRNAs interact with antisense mRNAs or bind to sequester target miRNAs to regulate the post-transcriptional gene regulation. In contrast, others recruit chromatin-modifying proteins or transcription factors to particular regions by binding them, while some engage in cis protein interactions to facilitate transcriptional regulation (Engreitz et al., 2016). The underlined mechanisms enable lncRNAs to shape key malignant processes in diseases such as renal, bladder, and prostate cancers (Cheng et al., 2019). The importance of lncRNAs as regulators of OSCC cell’s invasion and metastasis has been a focus of recent interest. Moreover, the EMT induction and associated signaling cascades are necessary for metastatic tumor progression. Specific lncRNAs have the potential to influence OSCC cell EMT induction by interacting with a range of targets in the Wnt, TGF-β, Notch, and NF-κB pathways either directly or indirectly by interacting with the target miRNAs (Figure 2; Table 1).
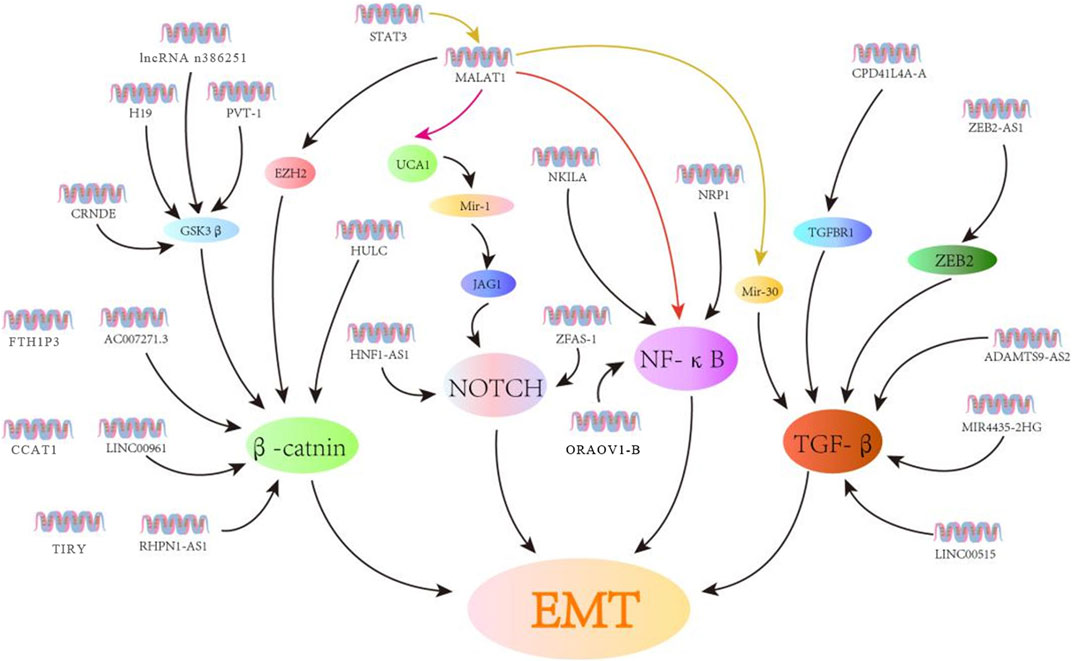
FIGURE 2. LncRNAs control EMT induction in OSCC cells through various signaling cascades. Herein, the lncRNAs associated with EMT are related to the Wnt, Notch, NF-κB, and TGF-β signaling cascades.
Significance of lncRNAs as epithelial–Mesenchymal transition induction regulators in oral squamous cell carcinoma cells
Wnt/β-catenin signaling pathway
The Wnt signaling pathway is a highly conserved regulator of numerous cellular processes. The dysregulation of Wnt contributes to the advancement of a variety of malignancies. For instance, in melanoma, Wnt5a can increase protein kinase C (PKC)-mediated EMT induction, hence facilitating metastasis (Dissanayake et al., 2007). In contrast, WNT974 can interfere with the Wnt signaling and suppress the growth of head and neck squamous cell carcinoma (HNSCC) cells (Giefing et al., 2016). The deregulation of critical Wnt pathway-associated proteins such as catenin β1 (CTNNB1), axis inhibition protein 1 (AXIN1), casein kinase 1α (CSNK1A1), glycogen synthase kinase 3 β (GSK3β), and T-cell factor 4 (TCF4) can eventually affect tumor cell invasion and pathways correlated with malignancies (Qiu et al., 2019).
The lncRNA RHPN1-AS1 is overexpressed in many OSCC cancers, which is associated with histological tumor grade. When RPHN-AS1 is silenced in HNSCC cells, this hampers their ability to grow and participate in invasion and metastasis. This is accompanied by the decrease in the expression of vimentin, β-catenin, and claudin-1 (Qiu et al., 2019). Similarly, the knockdown of the lncRNA CRNDE (colorectal neoplasia differentially expressed) can decrease the N-cadherin, vimentin, Snail, p-GSK-3β, and β-catenin expression (Dai et al., 2019). The β-catenin/GSK3β signaling can be inactivated via downregulating the H19 lncRNA in tongue squamous cell carcinoma (TSCC) cells (Zhang et al., 2017) or through overexpressing the PVT1 (plasmacytoma variant translocation 1) lncRNA in OSCC and HNSCC cells (Wang and Zhang, 2019). Moreover, the LINC00961 overexpression can reduce the β-catenin expression, and silencing the underlined lncRNA can increase tumor cell invasion and proliferation via the Wnt/β-catenin pathway activation (Zhang et al., 2019a). MALAT1 (metastasis-associated lung adenocarcinoma transcript 1) can activate Wnt/β-catenin signaling and induce the EMT induction in TSCC, with the Wnt pathway activator, that is, EZH2 (enhancer of zeste homolog 2), mediating the underlying mechanisms. Specifically, EZH2 interacts with MALAT1 and thus facilitates the nuclear translocation of β-catenin, promoting the EMT and protecting cells from apoptosis (Liang et al., 2017). The AC007271.3 lncRNA has been correlated with the regulation of Wnt/β-catenin signaling in OSCC cells to prevent apoptosis while augmenting proliferative, invasive, and metastatic activity (Shao et al., 2019). Similar to how the FTH1P3 lncRNA modulates PI3K/Akt/GSK3β/Wnt/β-catenin signaling to promote oncogenesis, LOLA1 (lncRNA oral leukoplakia progressed associated 1) can influence AKT/GSK‐3β signaling to assist the EMT induction (Liu et al., 2018a). The ability of the CCAT1 lncRNA to control OSCC cell proliferative, invasive, and migratory activity is tied to its inhibition of miR-181a and consequent activation of the Wnt/β-catenin pathway activity (Li et al., 2019a). The overexpression of the lncRNA TIRY in cancer-associated fibroblasts (CAFs) can also contribute to OSCC cell metastasis through the Wnt/β-catenin pathway activity mediated by serving as a molecular sponge for miR-14 (Jin et al., 2020).
TGF-β signaling pathway
TGF-β is a cytokine secreted by cells and controls recipient cells’ proliferative activity, invasion, and differentiation. TGF-β can suppress tumor growth and promote apoptotic death in precancerous cells during early tumorigenesis. However, this suppressive activity is lost during the later stages of tumor growth such that TGF-β can stimulate the EMT induction.
According to the Diao et al. (2019a) reported study, the lncRNA ZEB2-AS1 is an essential mediator of in vitro EMT induction in response to TGF-β1, with the silencing of ZEB2-AS1 resulting in the ZEB2 mRNA stabilization that ultimately suppresses the ability of HNSCC cells to proliferate, invade, and metastasize. Consistently, the loss of ZEB2-AS1 expression is associated with reduced N-cadherin expression and increased E-cadherin expression, consistent with the ability of the lncRNA to support the EMT induction within HNSCC cells. The ADAMTS9-AS2 lncRNA can suppress AKT signaling activity and control the expression of associated EMT biomarker genes in a conducive manner to inhibit the progression of precancerous oral submucous fibrosis (OSF) lesion to OSCC onset (Zhou et al., 2021). The STAT3 (signal transducer and activator of transcription 3) transcriptional regulator contributes to the EMT induction in many malignancies (Agarwal et al., 2015). Furthermore, TGF-β can promote STAT3 activation and the corresponding EMT induction in HNSCC cells, and knocking out STAT3 influences the TGF-β/MALAT1/miR-30 regulatory axis (Wang et al., 2018a). Based on the study by Teng et al., the TGF-β pathway-related lncRNAs EPB41L4A-AS2, LINC00515, and miR4435-2HG are dysregulated in the head and neck carcinoma, with EPB41L4A-AS2 serving to repress TGFBR1 and limit TGF-β's ability to drive the EMT progression. However, the regulatory roles of the remaining lncRNAs have not yet been fully explored. The lnRNA PAPAS (promoter and pre-rRNA antisense) can enhance TGFβ1 and thus promote the invasion and metastasis of OSCC. However, this process may or may not be related to the EMT induction (Huang et al., 2018a).
Notch signaling pathway
The Notch signaling pathway is an evolutionarily conserved correlated pathway with successful intercellular communication and proper tissue, cell, and organ development. It is hypothesized that the lncRNA-mediated modulation of Notch signaling influences crucial oncogenic processes in a variety of tumor types with the dysregulated Notch pathway activation.
According to Zhang et al. (2019b), urothelial cancer associated 1 (UCA1) can influence tongue cancer cell malignancy via the miR-124/JAG1 signaling axis. Knocking down UCA1 reduces the TGFβ1‐induced EMT induction and invasion in these cells through miR-124-mediated JAG1 regulation and Notch signaling, whereas the miR-124 knockdown has the opposite effect. The STAT3-induced upregulation of HNF1A antisense RNA 1lncRNA (HNF1A-AS1) can promote the Notch pathway activation and OSCC progression via HES-1, with HNF1A-AS1 also offering significance as a prognostic biomarker in patients with this carcinoma (Liu et al., 2019). Knocking down the ZNFX1 antisense, RNA1 (ZFAS1) lncRNA can enhance the Notch-related HES-1 and the Notch intracellular domain (NICD) expression in cells (Gao et al., 2017), thereby promoting the Notch1 upregulation in HNSCC cells in a manner conducive to enhanced metastatic progression (Kolenda et al., 2019).
NF-κB signaling pathway
In mammalian cells, the NF-κB transcription factor is assembled through the heterodimerization of five different subunit transcription factors: NF-κB/p105, NF-κB/p100, RelA/p65, RelB, and c-Rel (Gupta et al., 2020). These multiple NF-BNF-κB conformations can modulate the target gene expression depending on the situation after dimerization. NF-κB signaling has been shown to play a central role in many human diseases, including neurodegenerative disease, cardiovascular disease, arthritis, and various cancers, including OSCC.
In a variety of disease situations, studies have revealed interactions between NF-κB (Wu et al., 2018) and the transcription factors NKILA42, MALAT142, and HOX transcript antisense RNA (HOTAIR) and the host gene (Özeş et al., 2016) of miR-31 (miR31HG) (Jin et al., 2016) and Gm4419 (Wen et al., 2017). However, relatively few studies have examined these interactions or their clinical significance in the context of OSCC.
The activation of the NF-κB pathway is critical to the EMT induction and maintenance and supports the overall metastatic process. Lower levels of NKILA expression are associated with higher rates of metastasis and poor prognostic outcomes in TSCC patients, which is consistent with its role as a suppressor of tumor growth and with its known capacity to block NF-κB activation by reducing IκBα phosphorylation (Gupta et al., 2020). In one report, Zhou et al. (2015) demonstrated that MALAT1 knockdown suppressed nuclear NF-κB p65, β-catenin, and p-β-catenin accumulation, suggesting that MALAT1 may coordinate signaling between the NF-κB, Wnt, and the Notch pathways to shape the EMT process. Major NF-κB target gene TNF-α is downregulated by the NKILA overexpression, which is consistent with the transcription factor’s capacity to inhibit NF-κB signaling. The siRNA-mediated knockdown of MALAT1, conversely, suppresses OSCC cell NF-κB activation (Zhou et al., 2015). The transmembrane NRP1 glycoprotein is commonly expressed in bladder cancer (Saban et al., 2010), pancreatic cancer (Dallas et al., 2008), and oral cancer.
Moreover, its upregulation is associated with enhanced IκB phosphorylation and nuclear p65 translocation with E-cadherin and β-catenin downregulation and vimentin and N-cadherin upregulation (Raimondi et al., 2016). This suggests that NRP1 may activate NF-κB signaling to induce the EMT induction (Chu et al., 2014). By binding Hsp90, ORAOV1-B can increase its stability and thereby modulate the function of specific client proteins, including IκB, ultimately promoting NF-κB signaling and TNF-α expression, which further enhance NF-κB activation and EMT induction in OSCC (Luo et al., 2021).
Significance of lncRNAs as regulators of other oral squamous cell carcinoma-associated processes
In addition to the mechanisms and pathways described above, it has been found that several lncRNAs indirectly affect EMT induction and the progression of metastases in OSCC, as shown in Table 2.
Function of lncRNAs in regulating the invasion and metastasis of tumor cells
Various lncRNAs have been found to promote metastasis and tumor cell invasion. For instance, the lncRNA AC132217.4 can upregulate the expression of IGF2 to increase OSCC invasion, while Krüppel-like factor 8 (KLF8) regulates the AC132217.4 expression through upstream binding (Li et al., 2017). In HNSCC cells, LINC00460 can promote nuclear PRDX1 translocation, thereby facilitating the EMT activation (Jiang et al., 2019). Alternatively, MIR31HG can promote HNSCC cell progression through the cell cycle and associated proliferation by targeting HIF1A and P21 (Wang et al., 2018b). The HULC (highly upregulated in liver cancer) lncRNA can influence the EMT activity and the expression of other essential proteins such as cyclin D1, MMP-9, Bax, and Bcl-2. These proteins have a key role in tumor cell proliferation and invasion (Su et al., 2019). In OSCC, the PRKG1-AS1 lncRNA also regulates the EMT to shape essential malignant processes such as growth and invasion (Wu et al., 2020). At the same time, the MYOSLID lncRNA modulates a partial EMT program in OSCC cells to promote invasion and metastasis (Xiong et al., 2019). BANCR (BRAF-activated lncRNA) can enhance OSCC cell migration and proliferation by the MAPK pathway (Yao et al., 2021).
Many lncRNAs have been shown to influence the metastatic progression of OSCC through their ability to interact with specific miRNAs or other factors. For example, the NEAT1 (nuclear paraspeckle assembly transcript 1) lncRNA can control invasion and proliferation in OSCC cells via the miR-365/RGS20 pathway (Liu et al., 2018b). OSCC cells proliferate more aggressively while HOXA11-AS binds to miR-98-5p and encourages YBX2 (Y-box binding protein 2) overexpression. HOXC13 is upregulated by its antisense lncRNA transcript (HOXC13-AS), which can bind to sequester miR-378g, suppressing OSCC cell malignancy (Li et al., 2020). By acting as a molecular sponge for miR-411-5p, LINC0664 can enhance the expression of KLF9 and associated OSCC cell EMT induction, proliferation, migration, and invasion, whereas KLF9 binds to the LINC00664 promoter region, establishing a LINC00664/miR-411-5p/KLF9 regulatory feedback loop that governs the ability of OSCC tumors to progress (Wang et al., 2021a). Through a LINC00319/miR-199a-5p/FZD4 regulatory axis, the CCL18-induced LINC00319-mediated sequestration of miR-199a-5p promotes angiogenic, proliferative, and metastatic activities in OSCC cells that stimulate the EMT induction (Jiang et al., 2020). In OSCC, the ability of ZEB1-AS1 to bind miR-23a underlines its prooncogenic activity (Wang et al., 2021b), with PSMA3-AS1 sequestering miR-136-5p, and thus promotes the upregulation of the downstream target gene FN1, with the lncRNA thus contributing to more invasive tumor cell growth through the miR-136-5p/FN1 axis (Cao et al., 2021). Due to its capacity to sequester miR-34a-5p, increased LINC01123 expression in OSCC has been associated with poor patient outcomes and increased malignancy at the cellular level (Qin et al., 2022). Higher LINC00958 levels are also present in OSCC tumors wherein they can promote oncogenic processes via the miR-185-5p/YWHAZ axis (Wang et al., 2020a). PRM1A is indirectly upregulated by TSPEAR-AS2 via its ability to bind and sequester miR-487a-3p during OSCC progression (Xia et al., 2021).
Functions of lncRNAs as suppressors of tumor metastasis and invasion
The USP17-SNAI1 pathway is regulated by the deubiquitinating enzyme USP17, and LINC02487’s overexpression is sufficient to prevent proliferation, migration, and invasive growth (Feng et al., 2020a). Through its ability to target ROCK1, LOC441178 can constrain squamous carcinoma cell invasion and migration. (Xu et al., 2018). Similarly, TINCR suppresses OSCC tumor growth by regulating JAK2/STAT3 signaling cascades (Zhuang et al., 2020). Malignant cells exhibit high levels of expression of the let-7 miRNA cluster, which is essential as a regulator of the EMT induction and other malignant processes (Shimono et al., 2015). When H19 is silenced, let-7a-mediated HMGA2 expression is impaired, and the HMGA2-mediated EMT process is disrupted (Kou et al., 2019). Furthermore, GAS5 can suppress proliferation, metastasis, invasion, and EMT within OSCC cells through the miR-21/PTEN axis (Zeng et al., 2019). At the same time, MEG3 is a lncRNA that regulates miR-548d-3p/SOCS5/SOCS6 signaling to control the JAK-STAT signaling cascade, ultimately suppressing OSCC cell migration and enhancing apoptotic death (Tan et al., 2019). Functionally, miR-17-5p sequestration and cyclin D1 overexpression are mediated by the SNHG16 lncRNA and can affect OSCC cell malignancy and EMT induction. Cyclin D1 overexpression and miR-17-5p downregulation abolish the prevention of OSCC development caused by the underlined lncRNA (Wang et al., 2021c).
Future perspectives of LncRNAs in oral squamous cell carcinoma therapy
There is substantial evidence that the EMT plays a crucial role in OSCC’s chemoresistance and disease progression (Chandra Gupta and Nandan Tripathi, 2017), with multiple signaling molecules participating in mutually reinforcing functions in these pathways. Notably, lncRNAs have emerged as crucial regulators of such EMT induction and chemoresistance, contributing to establishing key malignant feedback loops within tumor cells that induce OSCC progression. Cisplatin is generally used as a first-line chemotherapeutic agent to treat OSCC patients. Wang et al. (2020b) determined that MALAT1 can contribute to resistance against this drug, owing to its ability to regulate the EMT induction, regulate the P-glycoprotein expression, and promote the PI3K/AKT/mTOR signaling. The transcription of the lncRNA CYTOR (cytoskeleton regulator RNA) is stimulated by the FOXD1 transcription factor’s interaction with the region upstream of the lncRNA’s promoter. It has the potential to act as a competitive endogenous RNA (ceRNA) that interferes with miR-1252-5p and miR-3148 activity, thereby facilitating LPP upregulation and serving as a considerable mediator of FOXD1-associated EMT induction and chemoresistance in OSCC progression (Meng et al., 2021).
OSCC patients experience high tumor metastasis and recurrence rates, contributing to the high mortality. However, there are currently no biomarkers available that can reliably guide early-stage OSCC diagnosis and treatment. Given the diversity and disease-specific expression patterns of lncRNAs, they demonstrate great promise as targets for diagnosing or treating OSCC. Indeed, several reports have shown that 2+ specific lncRNAs can, when analyzed in combination with tumor biomarkers, enable the more reliable detection of these tumors compared to single biomarker analyses. As such, investigations of lncRNAs and tumor biomarkers offer the opportunity to improve the sensitivity and accuracy of cancer diagnoses (Chen et al., 2021a), protecting patients from the harm associated with misdiagnosis.
Several reports have emphasized the prognostic relevance of lncRNAs in OSCC (Table 3). For example, the upregulation of PSMA3-AS1 is associated with poor patient outcomes (Cao et al., 2021). According to the reported study by Feng et al., the lncRNA SLC16A1-AS1 has the potential to act as a key mediator of OSCC cell proliferation, acting through modulating the cell cycle. The knockdown of SLC16A1-AS1 in SCC25 and CAL27 cell lines contributes to impaired growth and colony-forming activity, and this lncRNA is an attractive prognostic biomarker (Feng et al., 2020b). In another study, the lncRNA CEBPA-AS1 was associated with poor predictive outcomes. It enhanced OSCC progression through its ability to target the CEBPA/Bcl2 axis82. At the same time, LEF-AS1 interacts with LATS1 to suppress Hippo signaling in OSCC cells, indicating that this lncRNA may offer value as a prognostic and therapeutic target against the underlined carcinoma (Zhang et al., 2019c). The FOXD2-AS1 lncRNA is generally upregulated in OSCC patient tissues and correlated with poor predictive outcomes and a poor pathological grade (Liang et al., 2020). According to Tu et al., the intronic regions of the MIR31HG lncRNA were found to contain miR-31. They revealed that there was a correlation between the greater levels of MIR31HG and poor survival in stage-IV OSCC patients.
In contrast, higher miR-31 levels predicted nodal metastasis among stage I–III patients (Tu et al., 2022). A close association between HOTAIRM1 and OSCC tumor development has been reported, with this lncRNA serving as a cell cycle regulator and candidate prognostic/therapeutic target in OSCC (Yu et al., 2021). Higher LINC01793 levels are associated with a poorer OSCC prognosis for patients and poor clinicopathological features, showing that this lncRNA is an oncogenic mediator in cancer (Yuan et al., 2021). Serum Samson levels exhibit a high diagnostic sensitivity and specificity relative to other serum biomarkers (i.e., CEA, SCCA, and TSGF), indicating that this lncRNA may be an essential regulator of progression in OSCC to serve as a diagnostic and prognostic biomarker (Zheng et al., 2020). Moreover, an elevated level of H19 lncRNA expression is evident in OSCC cells. Compared to healthy control cells, this overexpression frequently coexists with H19 promoter hypomethylation, increasing the possibility that this hypomethylation could serve as a candidate of oral cancer prognostic biomarker (Lee et al., 2021).
Prognostic lncRNA profiles for OSCC cancer have been revealed by bioinformatics analyses in a substantial way (Table 3). Based on the Zhao et al. (2018) study, a panel of three lncRNAs was associated with OSCC patient prognosis. Similarly, Diao et al. (2019b) developed a risk score model based on four lncRNAs that has predictive implications for several clinicopathological parameters. Chen et al. (2021b) identified seven lncRNAs that together make up an immune-related lncRNA prognostic signature (IRLPS) capable of classifying patients with HNSCC into IRLPS-high and -low subgroups by analyzing their associations with immune function. In this view, these predictive methods could distinguish between the distinct tumor microenvironmental characteristics of OSCC patients. Moreover, Huang et al. (2019) detected seven prognosis-associated lncRNAs, while Jiang et al. (2021) defined a prognostic marker for OSCC patients consisting of autophagy-associated lncRNAs. Li et al. (2022) developed a prediction model using eight ferroptosis-associated lncRNAs and found that while high-risk patients had greater levels of AC099850.3, AC090246.1, ALMS1-IT1, AC021087.4, and HOTARM1, low-risk patients had higher levels of STARD4-AS1, MIAT, and AL512274.1 expression. Miao et al. (2020) identified seven lncRNAs with potential clinical and prognostic relevance in OSCC. Wu et al. (2022) reported a prognostic signature consisting of four glycolysis-associated lncRNAs capable of differentiating between low- and high-risk patients. Xu et al. (2022) found that a signature composed of 14 lncRNAs was able to predict OSCC patient prognosis and offer insight regarding immune cell status that may support immunotherapeutic intervention efforts. Yang et al. (2022) found several m6A-associated lncRNAs that could be used to predict the outcome of OSCC, and they used the underlined lncRNAs to make a prediction model.
The TME is a primary focus of recent cancer-related research, consisting of the stromal cells, immune cells, and extracellular matrix (ECM) proteins that form the local tumor niche (Quail and Joyce, 2013). The TME in OSCC tumors is typically hypoxic and has immunosuppressive and proinflammatory properties. The complex biomolecular signaling processes within the TME ultimately shape oncogenesis, metastatic progression, and treatment-related outcomes (Tang et al., 2021). Hypoxia can promote enhanced EMT induction in epithelial-like OSCC cells (Huang et al., 2018b). In patients with high-grade invasive OSCC, programmed-death 1 (PD-1) receptor/programmed-death ligand (PD-L1) overexpression has been seen in macrophages and dendritic cells. Similar findings have been observed for mesenchymal OSCC cells in vitro (Hirai et al., 2017; Wu et al., 2021). Tumor-associated macrophages (TAMs) with an M1 phenotype can drive EMT progression and promote cancer-stem cell (CSC) development in OSCC through the upregulation of MMP-14 and MME (You et al., 2022). In OSCC, stromal CAFs express high levels of lysyl oxidase (LOX), which promotes matrix collagen remodeling and stiffens the matrix in a way that promotes OSCC progression via the FAK phosphorylation pathway (Zhang et al., 2021). Hu et al. (2022) determined that neutrophils can control EMT and JAK2/STAT3 signaling in OSCC via chemerin, thus promoting progression. Interactions between the malignant cells and the TME are essential for EMT induction and multidrug resistance (MDR) onset (Erin et al., 2020). EMT induction consumes large amounts of energy, compromises T cell activation, and contributes to immune evasion (Bai et al., 2021).
The development of new therapeutic regimens that are more effective and maintain individualized treatment prognostic evaluation and diagnosis can be greatly helped by extensive studies to reveal the molecular mechanism underlying the role of specific lncRNAs in the context of tumorigenesis, metastatic progression, and production functions. Several clinical trials have explored the use of lncRNA-targeting therapeutics such as siRNAs and antisense oligonucleotides. These therapeutic modalities are particularly promising given that many lncRNAs exhibit tissue- or tumor-specific expression patterns that make them superior pharmacological targets, given that traditional small molecule inhibitor drugs show low target protein specificity levels. In this view, the lncRNAs have considerable potential as candidate clinical biomarkers and therapeutic targets. However, they will need to be tested in more clinical trials to make sure they are important to patient outcomes before they can be used more widely in clinics.
Author contributions
ZS, XW, YL, YH, and KL designed the concept. ZS researched the literature, wrote the manuscript, and made figures and tables. ZS, XW, YL, YH, and KL revised the manuscript. All authors have read and approved the final manuscript.
Funding
This work has been supported by the National Natural Science Foundation of China (81800952); the Natural Science Foundation of Hunan Province, China (2021JJ30987); and Hunan Provincial Science and Technology Department (2018SK51002).
Acknowledgments
The authors thank all the reviewers who participated in the review and MJ Editor (www.mjeditor.com) for providing English editing services during the preparation of this manuscript.
Conflict of interest
The authors declare that the research was conducted in the absence of any commercial or financial relationships that could be construed as a potential conflict of interest.
Publisher’s note
All claims expressed in this article are solely those of the authors and do not necessarily represent those of their affiliated organizations, or those of the publisher, the editors, and the reviewers. Any product that may be evaluated in this article, or claim that may be made by its manufacturer, is not guaranteed or endorsed by the publisher.
References
Agarwal, S., Lakoma, A., Chen, Z., Hicks, J., Metelitsa, L. S., Kim, E. S., et al. (2015). G-CSF promotes neuroblastoma tumorigenicity and metastasis via STAT3-dependent cancer stem cell activation. Cancer Res. 75, 2566–2579. doi:10.1158/0008-5472.CAN-14-2946
Ali, J., Sabiha, B., Jan, H. U., Haider, S. A., Khan, A. A., and Ali, S. S. (2017). Genetic etiology of oral cancer. Oral Oncol. 70, 23–28. doi:10.1016/j.oraloncology.2017.05.004
Bai, Y., Sha, J., Okui, T., Moriyama, I., Ngo, H. X., Tatsumi, H., et al. (2021). The epithelial-mesenchymal transition influences the resistance of oral squamous cell carcinoma to monoclonal antibodies via its effect on energy homeostasis and the tumor microenvironment. Cancers 13, 5905. doi:10.3390/cancers13235905
Cao, X., Luan, K., Yang, J., and Huang, Y. (2021). Targeting lncRNA PSMA3-AS1, a prognostic marker, suppresses malignant progression of oral squamous cell carcinoma. Dis. Markers 2021, 3138046. doi:10.1155/2021/3138046
Chandra Gupta, S., and Nandan Tripathi, Y. (2017). Potential of long non-coding RNAs in cancer patients: From biomarkers to therapeutic targets. Int. J. Cancer 140, 1955–1967. doi:10.1002/ijc.30546
Chen, S., Yang, M., Wang, C., Ouyang, Y., Chen, X., Bai, J., et al. (2021). Forkhead box D1 promotes EMT and chemoresistance by upregulating lncRNA CYTOR in oral squamous cell carcinoma. Cancer Lett. 503, 43–53. doi:10.1016/j.canlet.2020.11.046
Chen, Y., Luo, T. Q., Xu, S. S., Chen, C. Y., Sun, Y., Lin, L., et al. (2021). An immune-related seven-lncRNA signature for head and neck squamous cell carcinoma. Cancer Med. 10, 2268–2285. doi:10.1002/cam4.3756
Cheng, J. T., Wang, L., Wang, H., Tang, F. R., Cai, W. Q., Sethi, G., et al. (2019). Insights into biological role of LncRNAs in epithelial-mesenchymal transition. Cells 8, E1178. doi:10.3390/cells8101178
Chi, A. C., Day, T. A., and Neville, B. W. (2015). Oral cavity and oropharyngeal squamous cell carcinoma--an update. Ca. Cancer J. Clin. 65, 401–421. doi:10.3322/caac.21293
Chu, W., Song, X., Yang, X., Ma, L., Zhu, J., He, M., et al. (2014). Neuropilin-1 promotes epithelial-to-mesenchymal transition by stimulating nuclear factor-kappa B and is associated with poor prognosis in human oral squamous cell carcinoma. PLoS One 9, e101931. doi:10.1371/journal.pone.0101931
Dai, J., Mu, J-W., and Mu, H. (2019). Long non-coding RNA CRNDE regulates cell proliferation, migration, invasion, epithelial-mesenchymal transition, and apoptosis in oral squamous cell carcinoma. Oncol. Lett. 17, 3330–3340. doi:10.3892/ol.2019.9978
Dallas, N. A., Gray, M. J., Xia, L., Fan, F., van Buren, G., Gaur, P., et al. (2008). Neuropilin-2-mediated tumor growth and angiogenesis in pancreatic adenocarcinoma. Clin. Cancer Res. 14, 8052–8060. doi:10.1158/1078-0432.CCR-08-1520
Diao, P., Ge, H., Song, Y., Wu, Y., Li, J., Li, Z., et al. (2019). Overexpression of ZEB2-AS1 promotes epithelial-to-mesenchymal transition and metastasis by stabilizing ZEB2 mRNA in head neck squamous cell carcinoma. J. Cell. Mol. Med. 23, 4269–4280. doi:10.1111/jcmm.14318
Diao, P., Song, Y., Ge, H., Wu, Y., Li, J., Zhang, W., et al. (2019). Identification of 4-lncRNA prognostic signature in head and neck squamous cell carcinoma. J. Cell. Biochem. 120, 10010–10020. doi:10.1002/jcb.28284
Dissanayake, S. K., Wade, M., Johnson, C. E., O'Connell, M. P., Leotlela, P. D., French, A. D., et al. (2007). The Wnt5A/protein kinase C pathway mediates motility in melanoma cells via the inhibition of metastasis suppressors and initiation of an epithelial to mesenchymal transition. J. Biol. Chem. 282, 17259–17271. doi:10.1074/jbc.M700075200
Dongre, A., and Weinberg, R. A. (2019). New insights into the mechanisms of epithelial-mesenchymal transition and implications for cancer. Nat. Rev. Mol. Cell Biol. 20, 69–84. doi:10.1038/s41580-018-0080-4
Engreitz, J. M., Haines, J. E., Perez, E. M., Munson, G., Chen, J., Kane, M., et al. (2016). Local regulation of gene expression by lncRNA promoters, transcription, and splicing. Nature 539, 452–455. doi:10.1038/nature20149
Erin, N., Grahovac, J., Brozovic, A., and Efferth, T. (2020). Tumor microenvironment and epithelial-mesenchymal transition as targets to overcome tumor multidrug resistance. Drug Resist. Updat. 53, 100715. doi:10.1016/j.drup.2020.100715
Esteller, M. (2011). Non-coding RNAs in human disease. Nat. Rev. Genet. 12, 861–874. doi:10.1038/nrg3074
Feng, H., Zhang, X., Lai, W., and Wang, J. (2020). Long non-coding RNA slc16a1-AS1: Its multiple tumorigenesis features and regulatory role in cell cycle in oral squamous cell carcinoma. Cell 19, 1641–1653. doi:10.1080/15384101.2020.1762048
Feng, L., Zhang, J., Sun, M., Qiu, F., Chen, W., and Qiu, W. (2020). Tumor suppressor LINC02487 inhibits oral squamous cell carcinoma cell migration and invasion through the USP17-SNAI1 Axis. Front. Oncol. 10, 559808. doi:10.3389/fonc.2020.559808
Gao, K., Ji, Z., She, K., Yang, Q., and Shao, L. (2017). Long non-coding RNA ZFAS1 is an unfavourable prognostic factor and promotes glioma cell progression by activation of the Notch signaling pathway. Biomed. Pharmacother. 87, 555–560. doi:10.1016/j.biopha.2017.01.014
Giefing, M., Wierzbicka, M., Szyfter, K., Brenner, J. C., Braakhuis, B. J., Brakenhoff, R. H., et al. (2016). Moving towards personalised therapy in head and neck squamous cell carcinoma through analysis of next generation sequencing data. Eur. J. Cancer. 55. Oxford, England, 147–157. doi:10.1016/j.ejca.2015.10.070
Guo, Y., Ma, Y., Hu, X., Song, R., Zhu, L., and Zhong, M. (2018). Long non-coding RNA CEBPA-AS1 correlates with poor prognosis and promotes tumorigenesis via CEBPA/Bcl2 in oral squamous cell carcinoma. Cancer Biol. Ther. 19, 205–213. doi:10.1080/15384047.2017.1416276
Gupta, S. C., Awasthee, N., Rai, V., Chava, S., Gunda, V., and Challagundla, K. B. (2020). Long non-coding RNAs and nuclear factor-κB crosstalk in cancer and other human diseases. Biochim. Biophys. Acta. Rev. Cancer 1873, 188316. doi:10.1016/j.bbcan.2019.188316
Hirai, M., Kitahara, H., Kobayashi, Y., Kato, K., Bou-Gharios, G., Nakamura, H., et al. (2017). Regulation of PD-L1 expression in a high-grade invasive human oral squamous cell carcinoma microenvironment. Int. J. Oncol. 50, 41–48. doi:10.3892/ijo.2016.3785
Horejs, C. M. (2016). Basement membrane fragments in the context of the epithelial-to-mesenchymal transition. Eur. J. Cell Biol. 95, 427–440. doi:10.1016/j.ejcb.2016.06.002
Hu, X., Xiang, F., Feng, Y., Gao, F., Ge, S., Wang, C., et al. (2022). Neutrophils promote tumor progression in oral squamous cell carcinoma by regulating EMT and JAK2/STAT3 signaling through chemerin. Front. Oncol. 12, 812044. doi:10.3389/fonc.2022.812044
Huang, C. M., Yan, T. L., Xu, Z., Wang, M., Zhou, X. C., Jiang, E. H., et al. (2018). Hypoxia enhances fusion of oral squamous carcinoma cells and epithelial cells partly via the epithelial-mesenchymal transition of epithelial cells. Biomed. Res. Int. 2018, 5015203. doi:10.1155/2018/5015203
Huang, G. Z., Wu, Q. Q., Zheng, Z. N., Shao, T. R., and Lv, X. Z. (2019). Identification of candidate biomarkers and analysis of prognostic values in oral squamous cell carcinoma. Front. Oncol. 9, 1054. doi:10.3389/fonc.2019.01054
Huang, T., Huang, W., Lu, H., Zhang, B. Y., Ma, J., Zhao, D., et al. (2018). Identification and validation a TGF-β-associated long non-coding RNA of head and neck squamous cell carcinoma by bioinformatics method. J. Transl. Med. 16, 46. doi:10.1186/s12967-018-1418-6
Huarte, M. (2015). The emerging role of lncRNAs in cancer. Nat. Med. 21, 1253–1261. doi:10.1038/nm.3981
Jiang, Q., Xue, D., Shi, F., and Qiu, J. (2021). Prognostic significance of an autophagy-related long non-coding RNA signature in patients with oral and oropharyngeal squamous cell carcinoma. Oncol. Lett. 21, 29. doi:10.3892/ol.2020.12290
Jiang, X., Liu, J., Li, S., Jia, B., Huang, Z., Shen, J., et al. (2020). CCL18-induced LINC00319 promotes proliferation and metastasis in oral squamous cell carcinoma via the miR-199a-5p/FZD4 axis. Cell Death Dis. 11, 777. doi:10.1038/s41419-020-02978-w
Jiang, Y., Cao, W., Wu, K., Qin, X., Wang, X., Li, Y., et al. (2019). LncRNA LINC00460 promotes EMT in head and neck squamous cell carcinoma by facilitating peroxiredoxin-1 into the nucleus. J. Exp. Clin. Cancer Res. 38, 365. doi:10.1186/s13046-019-1364-z
Jin, C., Jia, L., Huang, Y., Zheng, Y., Du, N., Liu, Y., et al. (2016). Inhibition of lncRNA MIR31HG promotes osteogenic differentiation of human adipose-derived stem cells. Stem Cells 34, 2707–2720. doi:10.1002/stem.2439
Jin, N., Jin, N., Bu, W., Li, X., Liu, L., Wang, Z., et al. (2020). Long non-coding RNA TIRY promotes tumor metastasis by enhancing epithelial-to-mesenchymal transition in oral cancer. Exp. Biol. Med. 245, 585–596. doi:10.1177/1535370220903673
Johnson, D. E., Burtness, B., Leemans, C. R., Lui, V. W. Y., Bauman, J. E., and Grandis, J. R. (2020). Head and neck squamous cell carcinoma. Nat. Rev. Dis. Prim. 6, 92. doi:10.1038/s41572-020-00224-3
Kalluri, R., and Weinberg, R. A. (2009). The basics of epithelial-mesenchymal transition. J. Clin. Invest. 119, 1420–1428. doi:10.1172/JCI39104
Kolenda, T., Guglas, K., Kopczyńska, M., Teresiak, A., Bliźniak, R., Mackiewicz, A., et al. (2019). Oncogenic role of ZFAS1 lncRNA in head and neck squamous cell carcinomas. Cells 8, E366. doi:10.3390/cells8040366
Kou, N., Liu, S., Li, X., Li, W., Zhong, W., Gui, L., et al. (2019). H19 facilitates tongue squamous cell carcinoma migration and invasion via sponging miR-let-7. Oncol. Res. 27, 173–182. doi:10.3727/096504018X15202945197589
Lamouille, S., Xu, J., and Derynck, R. (2014). Molecular mechanisms of epithelial-mesenchymal transition. Nat. Rev. Mol. Cell Biol. 15, 178–196. doi:10.1038/nrm3758
Lee, E. Y., Song, J. M., Kim, H. J., and Park, H. R. (2021). Hypomethylation of lncRNA H19 as a potential prognostic biomarker for oral squamous cell carcinoma. Arch. Oral Biol. 129, 105214. doi:10.1016/j.archoralbio.2021.105214
Li, G. H., Ma, Z. H., and Wang, X. (2019). Long non-coding RNA CCAT1 is a prognostic biomarker for the progression of oral squamous cell carcinoma via miR-181a-mediated Wnt/β-catenin signaling pathway. Cell cycleGeorget. Tex) 18, 2902–2913. doi:10.1080/15384101.2019.1662257
Li, T., Wang, Y., Xiang, X., and Chen, C. (2022). Development and validation of a ferroptosis-related lncRNAs prognosis model in oral squamous cell carcinoma. Front. Genet. 13, 847940. doi:10.3389/fgene.2022.847940
Li, W., Zhu, Q., Zhang, S., Liu, L., Zhang, H., and Zhu, D. (2020). HOXC13-AS accelerates cell proliferation and migration in oral squamous cell carcinoma via miR-378g/HOXC13 axis. Oral Oncol. 111, 104946. doi:10.1016/j.oraloncology.2020.104946
Li, X., Ma, C., Zhang, L., Li, N., Zhang, X., He, J., et al. (2017). LncRNAAC132217.4, a KLF8-regulated long non-coding RNA, facilitates oral squamous cell carcinoma metastasis by upregulating IGF2 expression. Cancer Lett. 407, 45–56. doi:10.1016/j.canlet.2017.08.007
Li, Y., Wan, Q., Wang, W., Mai, L., Sha, L., Mashrah, M., et al. (2019). LncRNA ADAMTS9-AS2 promotes tongue squamous cell carcinoma proliferation, migration, and EMT via the miR-600/EZH2 axis. Biomed. Pharmacother. 112, 108719. doi:10.1016/j.biopha.2019.108719
Liang, J., Liang, L., Ouyang, K., Li, Z., and Yi, X. (2017). MALAT1 induces tongue cancer cells' EMT and inhibits apoptosis through the Wnt/β-catenin signaling pathway. J. Oral Pathology Med. 46, 98–105. doi:10.1111/jop.12466
Liang, X., Chen, Z., and Wu, G. (2020). FOXD2-AS1 predicts dismal prognosis for oral squamous cell carcinoma and regulates cell proliferation. Cell Transpl. 29, 1. doi:10.1177/0963689720964411
Lim, J., and Thiery, J. P. (2012). Epithelial-mesenchymal transitions: Insights from development. Development 139, 3471–3486. doi:10.1242/dev.071209
Liu, K., Gao, L., Ma, X., Huang, J. J., Chen, J., Zeng, L., et al. (2020). Long non-coding RNAs regulate drug resistance in cancer. Mol. Cancer 19, 54. doi:10.1186/s12943-020-01162-0
Liu, M., Gao, X., and Liu, C. L. (2018). Increased expression of lncRNA FTH1P3 promotes oral squamous cell carcinoma cells migration and invasion by enhancing PI3K/Akt/GSK3b/Wnt/β-catenin signaling. Eur. Rev. Med. Pharmacol. Sci. 22, 8306–8314. doi:10.26355/eurrev_201812_16528
Liu, W., Yao, Y., Shi, L., Tang, G., and Wu, L. (2021). A novel lncRNA LOLA1 may predict malignant progression and promote migration, invasion, and EMT of oral leukoplakia via the AKT/GSK-3β pathway. J. Cell. Biochem. 122, 1302–1312. doi:10.1002/jcb.29951
Liu, X., Shang, W., and Zheng, F. (2018). Long non-coding RNA NEAT1 promotes migration and invasion of oral squamous cell carcinoma cells by sponging microRNA-365. Exp. Ther. Med. 16, 2243–2250. doi:10.3892/etm.2018.6493
Liu, Z., Li, H., Fan, S., Lin, H., and Lian, W. (2019). STAT3-induced upregulation of long non-coding RNA HNF1A-AS1 promotes the progression of oral squamous cell carcinoma via activating Notch signaling pathway. Cancer Biol. Ther. 20, 444–453. doi:10.1080/15384047.2018.1529119
Luo, X., Jiang, Y., Chen, F., Wei, Z., Qiu, Y., Xu, H., et al. (2021). ORAOV1-B promotes OSCC metastasis via the NF-κB-TNFα loop. J. Dent. Res. 100, 858–867. doi:10.1177/0022034521996339
Meng, X., Lou, Q-Y., Yang, W-Y., Wang, Y-R., Chen, R., Wang, L., et al. (2021). The role of non-coding RNAs in drug resistance of oral squamous cell carcinoma and therapeutic potential. Cancer Commun. 41, 981–1006. doi:10.1002/cac2.12194
Miao, T., Si, Q., Wei, Y., Fan, R., Wang, J., and An, X. (2020). Identification and validation of seven prognostic long non-coding RNAs in oral squamous cell carcinoma. Oncol. Lett. 20, 939–946. doi:10.3892/ol.2020.11603
Niu, X., Yang, B., Liu, F., and Fang, Q. (2020). LncRNA HOXA11-AS promotes OSCC progression by sponging miR-98-5p to upregulate YBX2 expression. Biomed. Pharmacother. 121, 109623. doi:10.1016/j.biopha.2019.109623
Özeş, A. R., Miller, D. F., Özeş, O. N., Fang, F., Liu, Y., Matei, D., et al. (2016). NF-κB-HOTAIR axis links DNA damage response, chemoresistance and cellular senescence in ovarian cancer. Oncogene 35, 5350–5361. doi:10.1038/onc.2016.75
Qin, H., Wang, C., and Hua, Y. (2022). LINC01123 is associated with prognosis of oral squamous cell carcinoma and involved in tumor progression by sponging miR-34a-5p. Oral Surg. Oral Med. Oral Pathol. Oral Radiol. 133, 50–59. doi:10.1016/j.oooo.2021.07.013
Qiu, X., Lei, Z., Wang, Z., Xu, Y., Liu, C., Li, P., et al. (2019). Knockdown of LncRNA RHPN1-AS1 inhibits cell migration, invasion and proliferation in head and neck squamous cell carcinoma. J. Cancer 10, 4000–4008. doi:10.7150/jca.29029
Quail, D. F., and Joyce, J. A. (2013). Microenvironmental regulation of tumor progression and metastasis. Nat. Med. 19, 1423–1437. doi:10.1038/nm.3394
Raimondi, C., Brash, J. T., Fantin, A., and Ruhrberg, C. (2016). NRP1 function and targeting in neurovascular development and eye disease. Prog. Retin. Eye Res. 52, 64–83. doi:10.1016/j.preteyeres.2016.02.003
Saban, M. R., Sferra, T. J., Davis, C. A., Simpson, C., Allen, A., Maier, J., et al. (2010). Neuropilin-VEGF signaling pathway acts as a key modulator of vascular, lymphatic, and inflammatory cell responses of the bladder to intravesical BCG treatment. Am. J. Physiol. Ren. Physiol. 299, F1245–F1256. doi:10.1152/ajprenal.00352.2010
Shao, T-R., Zheng, Z-N., Chen, Y-C., Wu, Q-Q., Huang, G-Z., Li, F., et al. (2019). LncRNA AC007271.3 promotes cell proliferation, invasion, migration and inhibits cell apoptosis of OSCC via the Wnt/β-catenin signaling pathway. Life Sci. 239, 117087. doi:10.1016/j.lfs.2019.117087
Shimono, Y., Mukohyama, J., Nakamura, S-I., and Minami, H. (2015). MicroRNA regulation of human breast cancer stem cells. J. Clin. Med. 5, E2. doi:10.3390/jcm5010002
Simonson, B., and Das, S. (2015). MicroRNA therapeutics: The next magic bullet? Mini Rev. Med. Chem. 15, 467–474. doi:10.2174/1389557515666150324123208
Su, W., Tang, J., Wang, Y., Sun, S., Shen, Y., and Yang, H. (2019). Long non-coding RNA highly up-regulated in liver cancer promotes epithelial-to-mesenchymal transition process in oral squamous cell carcinoma. J. Cell. Mol. Med. 23, 2645–2655. doi:10.1111/jcmm.14160
Sung, H., Ferlay, J., Siegel, R. L., Laversanne, M., Soerjomataram, I., Jemal, A., et al. (2021). Global cancer statistics 2020: GLOBOCAN estimates of incidence and mortality worldwide for 36 cancers in 185 countries. Ca. Cancer J. Clin. 71, 209–249. doi:10.3322/caac.21660
Tan, J., Xiang, L., and Xu, G. (2019). LncRNA MEG3 suppresses migration and promotes apoptosis by sponging miR-548d-3p to modulate JAK-STAT pathway in oral squamous cell carcinoma. IUBMB Life 71, 882–890. doi:10.1002/iub.2012
Tang, J., Fang, X., Chen, J., Zhang, H., and Tang, Z. (2021). Long non-coding RNA (lncRNA) in oral squamous cell carcinoma: Biological function and clinical application. Cancers 13, 5944. doi:10.3390/cancers13235944
Thomson, P. J. (2018). Perspectives on oral squamous cell carcinoma prevention-proliferation, position, progression, and prediction. J. Oral Pathol. Med. 47, 803–807. doi:10.1111/jop.12733
Tu, H. F., Liu, C. J., Hung, W. W., and Shieh, T. M. (2022). Co-upregulation of miR-31 and its host gene lncRNA MIR31HG in oral squamous cell carcinoma. J. Dent. Sci. 17, 696–706. doi:10.1016/j.jds.2021.11.006
Wang, C., Wang, Q., and Weng, Z. (2021). LINC00664/miR-411-5p/KLF9 feedback loop contributes to the progression of human oral squamous cell carcinoma. Oral Dis. 1, 1. doi:10.1111/odi.14033
Wang, Q., Han, J., Xu, P., Jian, X., Huang, X., and Liu, D. (2021). Silencing of LncRNA SNHG16 downregulates cyclin D1 (CCND1) to abrogate malignant phenotypes in oral squamous cell carcinoma (OSCC) through upregulating miR-17-5p. Cancer Manag. Res. 13, 1831–1841. doi:10.2147/CMAR.S298236
Wang, Q., and Zhang, W. (2019). LncRNA PVT1 participates in developing oral squamous cell carcinomas through accelerating EMT and serves as a diagnostic biomarker. Panminerva Med. 63, 1. doi:10.23736/S0031-0808.19.03703-0
Wang, R., Lu, X., and Yu, R. (2020). lncRNA MALAT1 promotes EMT process and cisplatin resistance of oral squamous cell carcinoma via PI3K/AKT/mTOR signal pathway. Onco. Targets. Ther. 13, 4049–4061. doi:10.2147/OTT.S251518
Wang, R., Ma, Z., Feng, L., Yang, Y., Tan, C., Shi, Q., et al. (2018). LncRNA MIR31HG targets HIF1A and P21 to facilitate head and neck cancer cell proliferation and tumorigenesis by promoting cell-cycle progression. Mol. Cancer 17, 162. doi:10.1186/s12943-018-0916-8
Wang, X., Guo, Y., Wang, C., Wang, Q., and Yan, G. (2021). Long noncoding RNA ZEB1-AS1 downregulates miR-23a, promotes tumor progression, and predicts the survival of oral squamous cell carcinoma patients. Onco. Targets. Ther. 14, 2699–2710. doi:10.2147/OTT.S297209
Wang, Y., Wu, C., Zhang, C., Li, Z., Zhu, T., Chen, J., et al. (2018). TGF-β-induced STAT3 overexpression promotes human head and neck squamous cell carcinoma invasion and metastasis through malat1/miR-30a interactions. Cancer Lett. 436, 52–62. doi:10.1016/j.canlet.2018.08.009
Wang, Z., Zhu, X., Dong, P., and Cai, J. (2020). Long non-coding RNA LINC00958 promotes the oral squamous cell carcinoma by sponging miR-185-5p/YWHAZ. Life Sci. 242, 116782. doi:10.1016/j.lfs.2019.116782
Wen, Y., Yu, Y., and Fu, X. (2017). LncRNA Gm4419 contributes to OGD/R injury of cerebral microglial cells via IκB phosphorylation and NF-κB activation. Biochem. Biophys. Res. Commun. 487, 923–929. doi:10.1016/j.bbrc.2017.05.005
Wu, L., Liu, J., and Li, W. (2022). Prognostic significance of a 4-lncRNA glycolysis-related signature in oral squamous cell carcinoma. J. Dent. Sci. 17, 991–1000. doi:10.1016/j.jds.2021.11.002
Wu, T., Tang, C., Tao, R., Yong, X., Jiang, Q., and Feng, C. (2021). PD-L1-Mediated immunosuppression in oral squamous cell carcinoma: Relationship with macrophage infiltration and epithelial to mesenchymal transition markers. Front. Immunol. 12, 693881. doi:10.3389/fimmu.2021.693881
Wu, T., Zhang, S. Y., Dong, W. J., Wang, M., and Sun, Y. B. (2020). The potential influence of long non-coding RNA PRKG1-AS1 on oral squamous cell carcinoma: A comprehensive study based on bioinformatics and in vitro validation. J. Oral Pathol. Med. 49, 409–416. doi:10.1111/jop.12980
Wu, W., Chen, F., Cui, X., Yang, L., Chen, J., Zhao, J., et al. (2018). LncRNA NKILA suppresses TGF-β-induced epithelial-mesenchymal transition by blocking NF-κB signaling in breast cancer. Int. J. Cancer 143, 2213–2224. doi:10.1002/ijc.31605
Xia, Y. C., Cao, J., Yang, J., Zhang, Y., and Li, Y. S. (2021). lncRNA TSPEAR-AS2, a novel prognostic biomarker, promotes oral squamous cell carcinoma progression by upregulating PPM1A via sponging miR-487a-3p. Dis. Markers 2021, 2217663. doi:10.1155/2021/2217663
Xiong, H. G., Li, H., Xiao, Y., Yang, Q. C., Yang, L. L., Chen, L., et al. (2019). Long non-coding RNA MYOSLID promotes invasion and metastasis by modulating the partial epithelial-mesenchymal transition program in head and neck squamous cell carcinoma. J. Exp. Clin. Cancer Res. 38, 278. doi:10.1186/s13046-019-1254-4
Xu, K., Tian, H., Zhao, S., Yuan, D., Jiang, L., Liu, X., et al. (2018). Long noncoding RNA LOC441178 reduces the invasion and migration of squamous carcinoma cells by targeting ROCK1. Biomed. Res. Int. 2018, 4357647. doi:10.1155/2018/4357647
Xu, Z., Li, X., Pan, L., Tan, R., Ji, P., and Tang, H. (2022). Development of a lncRNA-based prognostic signature for oral squamous cell carcinoma. J. Oral Pathol. Med. 51, 358–368. doi:10.1111/jop.13281
Yang, J., and Weinberg, R. A. (2008). Epithelial-mesenchymal transition: At the crossroads of development and tumor metastasis. Dev. Cell 14, 818–829. doi:10.1016/j.devcel.2008.05.009
Yang, Q., Cheng, C., Zhu, R., Guo, F., Lai, R., Liu, X., et al. (2022). A N6-methyladenosine-related long non-coding RNAs model for predicting prognosis in oral squamous cell carcinoma: Association with immune cell infiltration and tumor metastasis. Oral Oncol. 127, 105771. doi:10.1016/j.oraloncology.2022.105771
Yao, C., Kong, F., Zhang, S., Wang, G., She, P., and Zhang, Q. (2021). Long non-coding RNA BANCR promotes proliferation and migration in oral squamous cell carcinoma via MAPK signaling pathway. J. Oral Pathol. Med. 50, 308–315. doi:10.1111/jop.12968
You, Y., Tian, Z., Du, Z., Wu, K., Xu, G., Dai, M., et al. (2022). M1-like tumor-associated macrophages cascade a mesenchymal/stem-like phenotype of oral squamous cell carcinoma via the IL6/Stat3/THBS1 feedback loop. J. Exp. Clin. Cancer Res. 41, 10. doi:10.1186/s13046-021-02222-z
Yu, Y., Niu, J., Zhang, X., Wang, X., Song, H., Liu, Y., et al. (2021). Identification and validation of HOTAIRM1 as a novel biomarker for oral squamous cell carcinoma. Front. Bioeng. Biotechnol. 9, 798584. doi:10.3389/fbioe.2021.798584
Yuan, S. J., Li, S. Y., Wang, Y. H., Zhang, H. F., Hua, Y., and Wang, T. (2021). The clinical significance, prognostic value and biological role of lncRNA LINC01793 in oral squamous cell carcinoma. Arch. Oral Biol. 125, 105105. doi:10.1016/j.archoralbio.2021.105105
Zeng, B., Li, Y., Jiang, F., Wei, C., Chen, G., Zhang, W., et al. (2019). LncRNA GAS5 suppresses proliferation, migration, invasion, and epithelial-mesenchymal transition in oral squamous cell carcinoma by regulating the miR-21/PTEN axis. Exp. Cell Res. 374, 365–373. doi:10.1016/j.yexcr.2018.12.014
Zhang, C., Bao, C., Zhang, X., Lin, X., Pan, D., and Chen, Y. (2019). Knockdown of lncRNA LEF1-AS1 inhibited the progression of oral squamous cell carcinoma (OSCC) via Hippo signaling pathway. Cancer Biol. Ther. 20, 1213–1222. doi:10.1080/15384047.2019.1599671
Zhang, D-M., Lin, Z-Y., Yang, Z-H., Wang, Y-Y., Wan, D., Zhong, J-L., et al. (2017). IncRNA H19 promotes tongue squamous cell carcinoma progression through β-catenin/GSK3β/EMT signaling via association with EZH2. Am. J. Transl. Res. 9, 3474–3486.
Zhang, J. Y., Zhu, W. W., Wang, M. Y., Zhai, R. D., Wang, Q., Shen, W. L., et al. (2021). Cancer-associated fibroblasts promote oral squamous cell carcinoma progression through LOX-mediated matrix stiffness. J. Transl. Med. 19, 513. doi:10.1186/s12967-021-03181-x
Zhang, L., Shao, L., and Hu, Y. (2019). Long non-coding RNA LINC00961 inhibited cell proliferation and invasion through regulating the Wnt/β-catenin signaling pathway in tongue squamous cell carcinoma. J. Cell. Biochem. 120, 12429–12435. doi:10.1002/jcb.28509
Zhang, T. H., Liang, L. Z., Liu, X. L., Wu, J. N., Su, K., Chen, J. Y., et al. (2019). LncRNA UCA1/miR-124 axis modulates TGFβ1-induced epithelial-mesenchymal transition and invasion of tongue cancer cells through JAG1/Notch signaling. J. Cell. Biochem. 120, 10495–10504. doi:10.1002/jcb.28334
Zhao, C., Zou, H., Wang, J., Shen, J., and Liu, H. (2018). A three long noncoding RNA-based signature for oral squamous cell carcinoma prognosis prediction. DNA Cell Biol. 37, 888–895. doi:10.1089/dna.2018.4317
Zheng, X., Tian, X., Zhang, Q., Shi, P., and Li, S. (2020). Long non-coding RNA SAMMSON as a novel potential diagnostic and prognostic biomarker for oral squamous cell carcinoma. J. Dent. Sci. 15, 329–335. doi:10.1016/j.jds.2019.11.008
Zhou, S., Zhu, Y., Li, Z., Zhu, Y., He, Z., and Zhang, C. (2021). Exosome-derived long non-coding RNA ADAMTS9-AS2 suppresses progression of oral submucous fibrosis via AKT signalling pathway. J. Cell. Mol. Med. 25, 2262–2273. doi:10.1111/jcmm.16219
Zhou, X., Liu, S., Cai, G., Kong, L., Zhang, T., Ren, Y., et al. (2015). Long non-coding RNA MALAT1 promotes tumor growth and metastasis by inducing epithelial-mesenchymal transition in oral squamous cell carcinoma. Sci. Rep. 5, 15972. doi:10.1038/srep15972
Keywords: lncRNAs, OSCC, EMT, mechanism, signaling pathways
Citation: Shao Z, Wang X, Li Y, Hu Y and Li K (2022) The role of long noncoding RNAs as regulators of the epithelial–Mesenchymal transition process in oral squamous cell carcinoma cells. Front. Mol. Biosci. 9:942636. doi: 10.3389/fmolb.2022.942636
Received: 12 May 2022; Accepted: 01 August 2022;
Published: 29 August 2022.
Edited by:
Wei Zhao, Chengdu Medical College, ChinaReviewed by:
Erhui Jiang, Wuhan University, ChinaRishi Kumar Jaiswal, Loyola University Chicago, United States
Copyright © 2022 Shao, Wang, Li, Hu and Li. This is an open-access article distributed under the terms of the Creative Commons Attribution License (CC BY). The use, distribution or reproduction in other forums is permitted, provided the original author(s) and the copyright owner(s) are credited and that the original publication in this journal is cited, in accordance with accepted academic practice. No use, distribution or reproduction is permitted which does not comply with these terms.
*Correspondence: Yanjia Hu, 17027301@qq.com; Kun Li, 406889138@qq.com