Environmental Quality and Ecological Risk Assessment of Heavy Metals in the Zhuhai Coast, China
- 1Faculty of Architecture, Civil and Transportation Engineering, Beijing University of Technology, Beijing, China
- 2South China Sea Marine Survey Technology Center, State Oceanic Administration, Guangzhou, China
The rapid urbanization and industrialization in the coastal Zhuhai have resulted in severe heavy metal contamination. Heavy metals are extensively used as indicators of coastal contamination, but the comparability among different geographic environments needs to be further explored and scrutinized. In this study, heavy metals (i.e., As, Zn, Cd, Cr, Hg, Cu, and Pb) in surface seawater (20 sites), sediments (10 sites), and organisms (13 sites, shellfish, algae, fish, and crustaceans) were analyzed to assess the distribution, enrichment, and ecological risk in the coastal regions of Zhuhai (2017 and 2018). The results showed that Cu, Zn, and Pb exceeded the standards of seawater, indicating the presence of anthropogenic pollution. Meanwhile, the ecological risk results showed that Cd and Cu indicated moderately high environmental risks. The quantity and quality of heavy metals in organisms showed different trends in the sea, which affected marine organisms in the northeastern area. The main factors contributing to the ecological risk of these two heavy metals are the emissions from chemical companies. Therefore, preserving the high biological diversity in this area is important to ensure the health and productivity of the seawater ecosystem in the coastal area. These results may play a key role in promoting decision makers to manage the sustainability of the Zhuhai coast.
1 Introduction
Heavy metals are one of the most serious pollutants in the environment and have attracted widespread attention worldwide due to their inherent toxicity, persistence, and bioaccumulation properties (Abaya et al., 2018; Huang et al., 2019; Kong et al., 2021). With industrial, agricultural, and urban development, heavy metals discharged into the coastal marine environment through surface runoff are increasing and enriching in marine sediments and aquatic organisms (Sruthi et al., 2018). When the concentration of heavy metals in the coastal ecosystem reaches a certain level, it will pose a great danger to the marine ecosystem and human health (Wang et al., 2018). The coastal ecosystem is a very important link in the ecological protection of coastal areas and plays an important role in the marine environment, social development, and population growth of coastal areas (Majithiya et al., 2018; Rao et al., 2021). Coastal ecosystems can also provide ecological buffers to the coastal areas and protect them from external intrusion and hazards (Sruthi et al., 2018). Changes in coastal zone ecosystems are partly due to sea-level rise, climate change, and partly human activities, such as changing land properties for coastal development, aquaculture, agricultural, and industrial activities (Wang et al., 2018; Wang L. et al., 2019).
Ecological risk assessment is developed from risk assessment, and in recent decades, its evaluation content, scope, and research scale have been greatly expanded. In general, the assessment tools can be used to qualitatively evaluate the degree to which the seawater- or sediment-associated chemical status might adversely affect aquatic organisms and are designed to assist in the interpretation of water/sediment quality (Kaikkonen et al., 2018; Quyen et al., 2021). The research scale of ecological risk assessment has been extended from single species to ecosystems, and the research objects have been extended from terrestrial to marine ecosystems (Wang Q. et al., 2019). The species sensitivity distribution (SSD) method, on the basis of differences in the sensitivity of different species to contaminations, was employed to develop environmental criteria for the conservation. The SSD calculates the toxicological data of different species for the whole ecosystem so as to understand the hazards of pollutants at the ecosystem level (Xia et al., 2020). The SSD method has become one of the advantageous methods of risk assessment internationally in recent years due to its advantages of conciseness and clear ecological significance (Xu J. et al., 2018; Xu et al., 2021). However, only a few studies on this method have been reported in China and mainly focused on freshwater organisms and assessed the ecological risk of various organisms such as DDT, lindane, polycyclic aromatic hydrocarbons, chlorophenols, and diisooctyl phthalate to freshwater organisms (Li et al., 2018a; Cui et al., 2021; Xu et al., 2022). Ecological risk assessment based on species sensitivity differences has obvious advantages for solving complex ecosystems (Sruthi et al., 2018), such as integrated ecological risk classification in coastal seas (Kumar et al., 2019; Luo et al., 2019).
The Pearl River is the second most important river system in South China, ranking among the seven major rivers in China in terms of annual runoff. The stability and health of the ecological environment of the Zhuhai coast, which is one of the most important sea outlets in the Zhuhai watershed, are related to the healthy development of this special economic zone. In previous studies, large-scale data on the heavy metal concentration and ecological risk of coastal seawater and sediments along the coast of Guangdong province have been collected, especially focusing on the economically developed Pearl River estuary (Birch, 2018; Wang R. et al., 2020). Previous studies found that the characterization of the distribution of heavy metals in these coastal marine areas has certain regularity. From far to near the coast, the concentration of heavy metals is gradually increasing, and the resulting risk is also gradually increasing (Table S1). Usually, the coastal special economic zones are experiencing high economic development, which inevitably leads to the discharge of industrial pollution. Analyzed from the environmental-geochemical point of view, the accumulation of heavy metals is gradually weakened with the extension of migration paths, which is the main reason for the serious excess of heavy metals on the coast of this study area. The ecological risk studies based on SSD have been carried out less for the sea area of the Zhuhai coast. Therefore, in this study, the seawater, sediment, biological species, and productivity were mainly sampled and investigated by selecting the sea area of Gaolan on the Pearl River coast as the study area.
In China, the marine areas with the most serious heavy metal pollution are the bays and estuaries where industrial and mining industries are concentrated, some of them are contaminated with Cd, Cu, Pb, and Hg, and some of them are seriously contaminated with Cd and As (Fang et al., 2018; Hu et al., 2021). Heavy metal pollution in the ocean is bound to bring a great threat to the local ecological diversity (Reddy et al., 2016; Satheeswaran et al., 2019; Pandey et al., 2021; Pandey et al., 2022). Therefore, it is urgent to study the ecological risk of heavy metal pollution in China’s oceans. Hence, the target of this research is to assess the potential impact of heavy metals on seawater, sediment, and marine organisms and provide ecological risk evaluation and risk management of heavy metal pollution in China’s marine environment. Thus the SSD curves of heavy metals, the 5% hazard concentration (HC5) of marine organisms at different trophic levels, and the potential impact ratio (PAF) were calculated to support the completion of the above goals.
2 Material and Methods
2.1 Description of Study Area
Zhuhai (21°45′–22°40′N, 113°03′–113°35′E) is an economic functional area with a total area of 380 km2 and is the main coastal hub port in South China (Figure 1). Zhuhai and its coastal sea areas have a typical subtropical monsoon climate with an obvious winter monsoon. From 1962 to 2010, the linear warming rate of the annual average temperature in Zhuhai was 0.14°C/10a, the average temperature was 22.61°C (Li and Chen, 2014), and the SD was 0.44. The depth of seawater is about −8 to 10 m, which provides excellent conditions for industrial and agricultural production. As a national-level economic and technological development zone in China, Zhuhai is the gateway to the world from the South China Sea, and the Zhuhai coast is one of the most dynamic and potential areas of Guangdong’s marine economy, with the largest berths of liquid chemicals and bulk cargo in the Pearl River Delta. The industrial types in Zhuhai are mainly large equipment manufacturing, petrochemical industry, and fine chemical industry, and aquaculture is also an important contributor to economic development.
2.2 Sampling Collection and Analysis
The location of the study area and the layout of the survey stations are shown in Figure 1. A total of 20 water quality survey sites, 10 sediment survey sites, and 13 ecological survey sites (partially overlapping, totaling 20 sampling sites) were studied in this survey. Sample collection and survey studies were conducted in November 2017 (Autumn) and March 2018 (Spring). Samples were collected only once per season. On each of the sampling sites, four subsamples were taken by randomly collecting fresh surfaces around the center sampling site (5 m). Combined with the center sample, five samples are collected and mixed thoroughly into one composite sample for post-processing analysis. Following the collection, all samples were stored in a refrigerator and transported to the laboratory by a professional transporter. The People’s Republic of China for Marine Survey Specifications (GB/T 12763-2007) and Marine Monitoring Specifications (GB17378-2007) were chosen as the references for steps and tools in the sample collection process (Li et al., 2018a; Liu et al., 2018; Wang X. et al., 2020). The concentration of heavy metals was determined by inductively coupled plasma mass spectrometry and inductively coupled plasma atomic emission spectrometry. To ensure the quality of analysis, the control methods and laboratory quality assurance were implemented, including the use of standard operating procedures, analysis of reagent blanks, calibration with standards, and analysis of replicates. The relative SD indicating the precision of analytical procedures ranged from 3.5% to 8.5%. The percent recoveries varied between 86% and 95% for all metals.
The seawater depth, transparency, and color were observed on site. Surface and bottom samples were collected at 0.1–1 m from the seawater surface and 2 m from the bottom. Sediment samples were collected using a 0.05-m2 grab mud collector to collect sediment samples at 0–1 cm from the surface layer of seafloor sediments and were stored in sealed packages. After stones and other debris were removed, samples were air-dried at room temperature (20°C to 23°C). Then the samples were ground with an agate mortar and passed through 160 mesh nylon screens. Four categories of organisms, including locally common and representative shellfish, algae, fish, and crustaceans, were selected among intertidal organisms and benthic organisms for the quality survey, and the samples were collected, preserved, and transported according to the relevant regulations of GB/T 12763.6-2007 for the biological survey (Kim et al., 2018; Wu et al., 2018). A trawling survey was carried out on the sampling site, the net was set at a distance of 2 nautical miles from the station, and the towing speed was controlled at 2–3 knots. A sufficient number (about 1.5 kg) of intact organisms samples were selected and placed in a clean polyethylene bag for sealing and preservation. This bag along with the sample label in another polyethylene bag was sealed and refrigerated. The collected water samples were filtered using a 0.45-μm or 60-mm microporous membrane with HNO3/H2SO4 to pH < 2 for cryogenic refrigeration, the collected sediment samples were sealed and stored away from light, and the samples of various organisms were sorted and stored in polyethylene bags for freezing. As and Hg were determined by atomic fluorescence, while Cu, Cd, Cr, Pb, and Zn were determined by flameless atomic absorption spectrophotometry (Yang et al., 2019; Anusuya et al., 2021).
2.3 Data Analysis
Several statistical tools, mainly including Pearson’s correlation analysis, non-metric multidimensional scaling analysis (NMDS), Adonis test, Procrustes analysis, and constrained ordination principal coordinate analysis (CPCOA), were used to investigate the correlations or differences between heavy metals and samples. Network analysis was employed to explore the co-occurrence of heavy metals and organisms in marine based on their strong and significant correlation matrixes (p-value <0.05; Spearman’s r > 0.8). Network visualization was performed employing Gephi (v0.9.2). Statistical analyses were conducted in R (v3.4.2) by utilizing several R packages, including gunifrac, car, and vegan. Kriging interpolation method of ArcGIS (v10.8, ESRI, Redlands, CA, USA) was employed for the spatial distribution characteristics of heavy metals in the sediment.
2.4 Partitioning and Bioaccumulation
The distribution coefficient between sediment and water (Kd), biota-sediment accumulation factor (BSAF), and bioaccumulation factor (BAF) were employed to explore the accumulation and adsorption of heavy metals between different phases. The specific formulas were calculated as follows (Ashayeri and Keshavarzi, 2019; Liu et al., 2022):
where Csed, Csea, and Corg are the concentration of target heavy metal in sediments, seawaters, and marine organisms, respectively. The value of Kd shows the possibilities of heavy metals preferentially retrained through sediments, and a higher Kd value indicates greater liquidity. Both BSAF and BAF are employed to describe the capability of organisms accumulating heavy metals from other mediums in the surrounding. BSAF < 1 or BAF > 100 demonstrates a low accumulation effect between the surrounding environment and organisms (Liu et al., 2022).
2.5 Pollution Assessment
The standard index method (single water quality index (WQI) method) was used to assess the degree of pollution of each heavy metal in seawater, and the characteristic pollution factors were identified based on the ranking of the calculated results (Zhao et al., 2019). In order to assess the regional water quality condition, the WQI method was used for comprehensive water quality assessment (Xiao et al., 2021; Xu Y. et al., 2018). The calculation formula was as follows:
where Ai is the standard index of i pollutants; Ci is the measured concentration of i pollutants; Csi is the evaluation standard of i pollutants. The evaluation is carried out according to the corresponding category standards in the Seawater Quality Standard (GB3097-1997). WQI is a comprehensive index of water quality, Ai is a single factor of the standard index, and n is the number of items of all the water quality parameters involved in the assessment. WQI < 1 means that the water quality is excellent; 1 < WQI < 2, good; 2 < WQI < 3, normal; 3 < WQI < 5, poor; and WQI > 5, very poor (Xu Y. et al., 2018).
2.6 Biological Quality Assessment
In this study, primary productivity and diversity index were used to characterize the biological quality in the study sea, where primary productivity characterizes the richness of the marine environment and diversity index characterizes the dominance of marine organism populations. The primary productivity was estimated by the chlorophyll a method according to the simplified formula proposed by Cadée and Hegeman (1974). The diversity index was calculated using the Shannon–Wiener index formula, as follows:
where P is the primary productivity, mg·C/m2·d; Ca is the surface chlorophyll a concentration, mg/m3; Q is the assimilation coefficient, mg·C/(mgChl-a·h), based on the results of the South China Sea survey, which was taken here as 3.70 (Wang X. et al., 2020). H′ is the species diversity index, S is the total number of species in the sample, and Pi is the number of individuals and the total number of species in the ith sample. Pi is the ratio of the number of individuals in the ith to the total number. Uniformity J is used to characterize the integrity of the marine ecosystem, and Pielou’s uniformity formula is J = H′/log2S, where J represents uniformity, H′ characterizes the species diversity index, and S is the total number of species in the sample.
2.7 Species Sensitivity Distribution Assessment
The SSD method was used to evaluate the ecological effects of heavy metal pollutants in seawater, which is an advanced statistical extrapolation method with high confidence based on the differences in the sensitivity of different species to pollutants, and has the advantages of conciseness and clear ecological significance. The Burr type III distribution was chosen to fit the exposure concentration accumulation curve, and the Burr type III distribution covers a large range in both skewness and kurtosis, which makes it more flexible to fit the parameters (Li et al., 2018b; Liu et al., 2020). The parametric equation of the Burr type III function F(x) is as follows:
In the equations, b, c, and k are the three parameters of Burr type III distribution; HC5 is the cumulative concentration corresponding to the proportion of hazardous species on the SSD curve when it reaches 5%; and the smaller HC5 means that it represents a more toxic heavy metal. In equation (9), q is the corresponding protection level, and PAF is the proportion of hazardous species corresponding to each measured concentration on the SSD curve. In equation (10), x is the measured concentration of pollutants. In equation (11), msPAF is the proportion of hazardous species produced by the combination of multiple pollutants, which can reflect the joint pollution of multiple pollutants in the water body.
3 Results and Discussion
3.1 Characteristics of Heavy Metals in Seawater, Sediment, and Marine Organism
3.1.1 Contamination Characteristics of Heavy Metals in the Seawater
The water quality evaluation results of the sea area calculated by using the factor evaluation method are shown in Table 1 (Liu et al., 2022). It can be seen from Table 1 that in the autumn of 2017, the main exceedance factors are Cu and Zn, and the exceedance rates are 11.1% and 22.2%, respectively. The exceedance factors in the main discharge areas of industrial towns were inorganic nitrogen and reactive phosphate, with 100% and 50% exceedance rates, respectively. Similarly, it can be seen from Table 2 that in the spring of 2018, the main exceedance factors of seawater in the agricultural farming area were inorganic nitrogen, Pb, reactive phosphate, and Cu, with exceedance rates of 100%, 66.7%, 33.3%, and 11.1%, respectively. From the overall analysis, the different pollution factors in Autumn 2017 and Spring 2018 are mainly caused by the uneven distribution of seawater caused by seasonal monsoon. On the other hand, the water quality in the lower layer of seawater (B layer) is significantly better than that in the upper layer (A layer), mainly since the upper layer is the main area of influence of farming, industrial discharges, and other activities. The main source of inorganic nitrogen and phosphate, as the main pollutants, is the marine agricultural farming activities near the sea area, and the release of large amounts of inorganic nitrogen and phosphate in this process is the main reason for the exceedance of these two pollutants in the overall sea area.
The analysis results of a heavy metal evaluation in the coastal seawater of Zhuhai are shown in Figures 2, 3. From Figures 2, 3, it can be seen that the concentration of Cu, Zn, Cd, Cr, Hg, and As in the seawater in Autumn 2017 are in line with the first-class standard of seawater quality, and only one site has Pb concentration exceeding the second class standard of seawater quality. The concentration of Cu, Pb, Cd, Cr, Hg, and As in the seawater in spring 2018 is in line with the first-class standard of seawater quality, and only one site has a Zn concentration exceeding the second class standard of seawater quality. Zn concentration at one site exceeded the second standard for seawater quality. At the same time, the concentration of heavy metals in the seawater of the Gaolan Sea has a significant seasonal trend, and the concentrations of As, Cd, and Cr have significant seasonality. The concentrations of As, Cd, and Cr in autumn are higher than those in spring, and this result may be due to the higher rainfall in spring (569.6 mm) than in autumn (288.9 mm) (Cui et al., 2021) and the seasonal variation of river flow in Guangdong Province. This result suggests that dilution leads to lower concentrations of heavy metals in seawater; the seasonal distribution of precipitation in Guangdong is affected by the recession of cold high pressure. The rise and fall of summer winds leading to higher rainfall in spring were also reported in the studies of Zhang et al. (2017) and Liu et al. (2018). As, Cd, Cr, Cu, Hg, and Zn were detected at the highest concentrations at stations closer to the coastline, with a decreasing trend in the direction toward the ocean, while the highest concentrations of Pb were found at 4–5 km south of the coastline. The comparison of the distribution of heavy metal concentration in the sea area with other similar areas is shown in Table S1, which shows that the surface heavy metal hazard in the sea area of coastal Zhuhai is not very serious and is at a relatively acceptable level, but the proliferation of heavy metals should still be strictly prevented. The water quality evaluation results of Zhuhai coastal waters are shown in Figure 4. It can be found that in 2017, the water quality in the southwestern sea is worse than that in the northeast, while the two are opposite in 2018. This may be due to the seasonal industrial production and agricultural fishing activities. In 2017, there were more factory discharges along the southwestern coast, which led to the accumulation of heavy metals in seawater (Wang R. et al., 2020).
3.1.2 Contamination Characteristics of Heavy Metals in Sediments
The results of the heavy metals in sediments of coastal Zhuhai are shown in Table 1. It can be seen from Table 1 that the main exceedance factors of the marine protected area within the study area are Cu, Cr, and Cd, and the exceedance rates are 80%, 80%, and 40%, respectively. In contrast, the main exceedance factor in the industrial town discharge area is Cd, and the exceedance rate is 50%. The spatial distribution characteristics of heavy metals in the sediment of the Zhuhai coast were simulated by using the Kriging spatial interpolation method. From Figures 2, 3, it can be found that As in this study area is concentrated in the recreational tourism area in the southeast direction; Zn, Pb, Hg, and Cd are mainly concentrated in the discharge area of industrial towns in the east; and Cu and Cr are concentrated in the discharge area of industrial towns in the southwest direction. The highly concentrated sediment heavy metal distribution indicates that the main distribution of heavy metals in the sediment of this sea area is still influenced by the discharge of industrial towns under the influence of the oceanic monsoon climate. Although there will be oceanic monsoon currents driving the migration of heavy metals with seawater, in general, sedimentation activities are still dominant (Han et al., 2020). This requires the environmental and ecological management department to focus on the impact of industrial towns on the marine sediment environment in the later planning and management.
3.1.3 Contamination Characteristics of Heavy Metals in Marine Organism
The quality standards of shellfish in marine organisms refer to Marine Biological Quality (GB18421-2001), and the quality evaluation standards of other fish, crustaceans, mollusks, and other marine organisms adopt the standards in the Concise Regulations for the Comprehensive Survey of National Coastal Zone and Sea-coat Resources. The single index method was used to calculate the index of marine biological quality, and the results are shown in Table 1. The heavy metal Cu has the greatest impact on marine organisms, especially invertebrates and shellfish, followed by the heavy metal Pb. According to Pandey’s study, organisms in the ocean near the coast are mostly affected by external factors (Pandey et al., 2021).
Cu, Pb, Zn, and Cd in shellfish in the fall of 2017 survey area exceeded the first category of biological quality standards, and all other factors met the first category of biological quality standards. In addition, the Cd in the shrimp mayflies exceeded the standards stipulated in the Concise Regulations for the Comprehensive Survey of National Coastal Zone and Sea-coat Resources and the Technical Regulations for the Second National Marine Pollution Baseline Survey (Second Branch), while the quality of fish, shrimp, and other marine organisms in other stations met the Concise Regulations for the Comprehensive Survey of National Coastal Zone and Sea-coat Resources and the Technical Regulations for the Second National Marine Pollution Baseline Survey (Second Branch). The quality of marine organisms in the survey area is good.
In the spring of 2018, there were no shellfish samples in the survey area, and only zinc in the nudibranchs exceeded the standard stipulated in the Concise Regulations for the Comprehensive Survey of National Coastal Zone and Sea-coat Resources, while the quality of other fish, shrimps, and other marine organisms all met the relevant index standards in the Concise Regulations for the Comprehensive Survey of National Coastal Zone and Sea-coat Resources and the Technical Regulations for the Second National Baseline Survey of Marine Pollution (the second volume), indicating that the quality of marine organisms in the survey area was good.
3.2 Marine Organism Quality Assessment
The results of the marine ecological characteristics survey in the sea area of coastal Zhuhai are shown in Figure 5. In terms of chlorophyll a and primary productivity, the variation of chlorophyll a concentration in the surface water column of the sea area surveyed in Autumn 2017 ranged from 0.74 to 5.66 mg/m3, with a mean value of 1.58 mg/m3, and the variation of primary productivity ranged from 5.99 to 75.62 mg·C/(m2·d), with a mean value of 23.55 mg·C/(m2·d). The variation of chlorophyll a concentration in the surface waters of the sea area in Spring 2018 ranged from 1.42 to 11.42 mg/m3 with a mean value of 4.70 mg/m3, and the variation of primary productivity ranged from 25.97 to 464.00 mg·C/(m2·d) with a mean value of 201.89 mg·C/(m2·d). Overall, the productivity index in the northeast is higher than in other regions, indicating that this region is the priority production fishing area in the study area.
The variation in the number of phytoplankton species at each station in Autumn 2017 ranged from 4 to 22 species, with an average of 12 species (Figure 6). The Shannon–Wiener diversity index ranged from 0.009 to 1.486, with an average of 0.366, and the distribution of biomass among species at each station was very heterogeneous. In contrast, the results of Spring 2018 survey showed that the number of phytoplankton species varied from 15 to 35 species at each station, with an average of 23 species. The Shannon–Wiener diversity index ranged from 1.836 to 2.487, with an average of 2.175, and Pielou’s evenness index ranged from 0.601 to 0.878, with an average of 0.701. The distribution of biomass among species was relatively uniform, and the planar distribution trends of diversity index and evenness index were basically consistent.
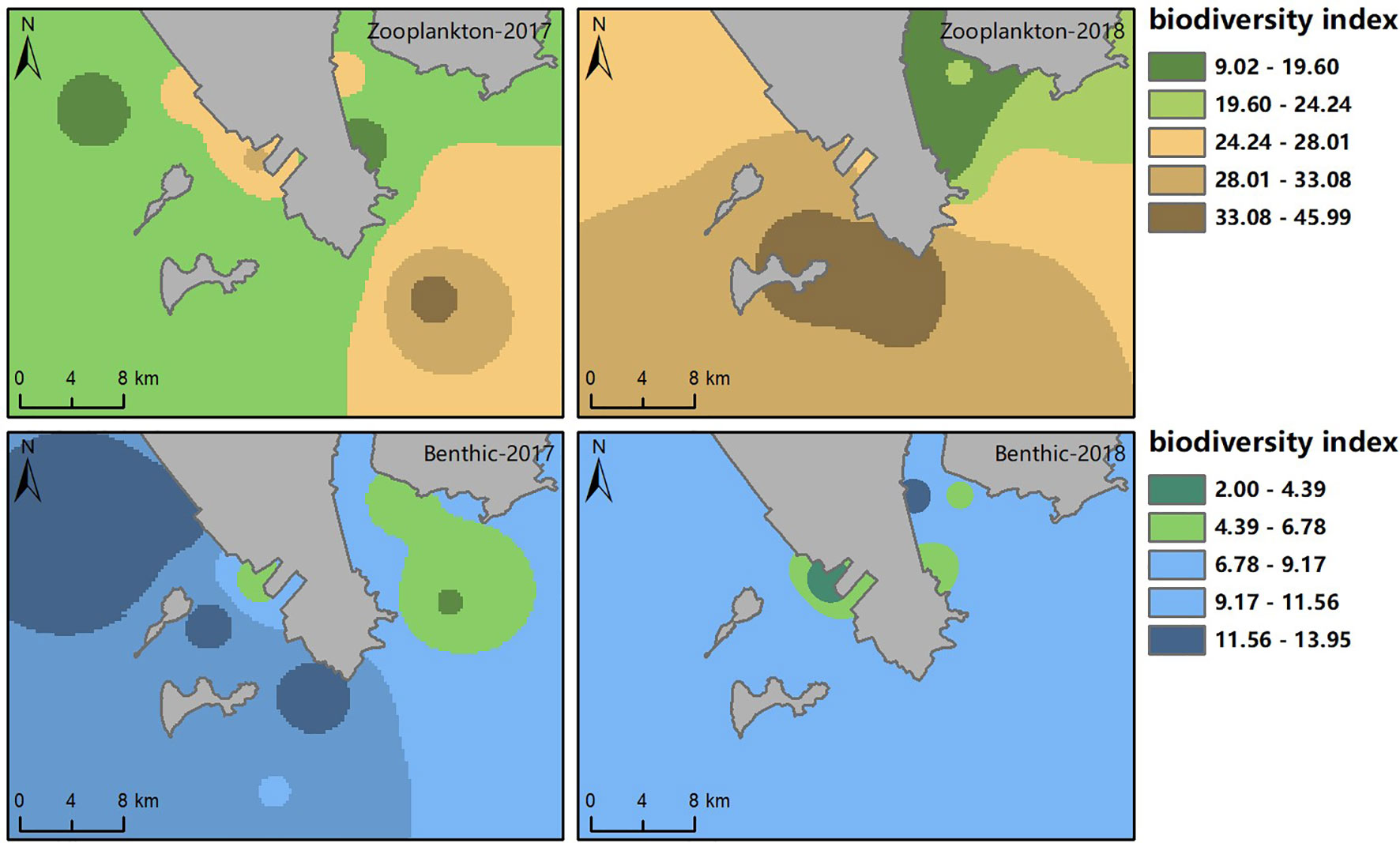
Figure 6 Evaluation results of zooplankton diversity index and benthic biodiversity index in coastal of Zhuhai.
Compared with plankton, benthic organisms showed less variation. The range of species variation of macro-benthic organisms at each quantitative sampling station in the Fall 2017 survey area was from 4 to 14 species/station, with an average of 9 species/station. The variation of the diversity index (H′) ranged from 0.21 to 2.39, with a mean value of 1.56 (Table S2), and the benthic biodiversity index in the surveyed sea area was moderately low. The range of uniformity was from 0.15 to 1.00, with an average of 0.72, reflecting the uneven distribution of species, and the variation in the number of macro-benthic species at each quantitative sampling station in the spring of 2018 ranged from 2 to 14 species/s tation, with an average of 9 species/station. The variation of diversity index (H′) ranged from 0.451 to 2.199, with a mean value of 1.421 (Table S2). The benthic biodiversity index of the surveyed sea area was moderately low. The evenness ranged from 0.268 to 0.945, with a mean value of 0.691, reflecting a relatively even distribution of species among the stations.
3.3 Interrelation and Source Analysis of Heavy Metals
The results of heavy metal and other particle correlation analyses in this study area are shown in Figure 7. The correlation matrix table shows that salinity was negatively correlated with As and Cr concentrations, indicating that salinity may hinder seawater mixing and maintain higher concentrations of As in surface seawater. Pearson’s correlation analysis further showed that the concentration of Cu, Pb, and Zn in coastal sediments of the high bar sea area was significantly and positively correlated with the Gross Domestic Product (GDP) and wastewater discharge of each province (p = 0.01), suggesting that these three metals are more influenced by seasonal changes and fishing periods. Pb, Zn, Cd, and As were all significantly and positively correlated with seasons (p = 0.01), suggesting that the concentration of these four heavy metals in sediments is more influenced by local seasonal changes. Cu concentration was significantly and positively correlated with the gross industrial product (p = 0.005), indicating that Cu concentration was mainly influenced by the discharge of local industrial wastewater.
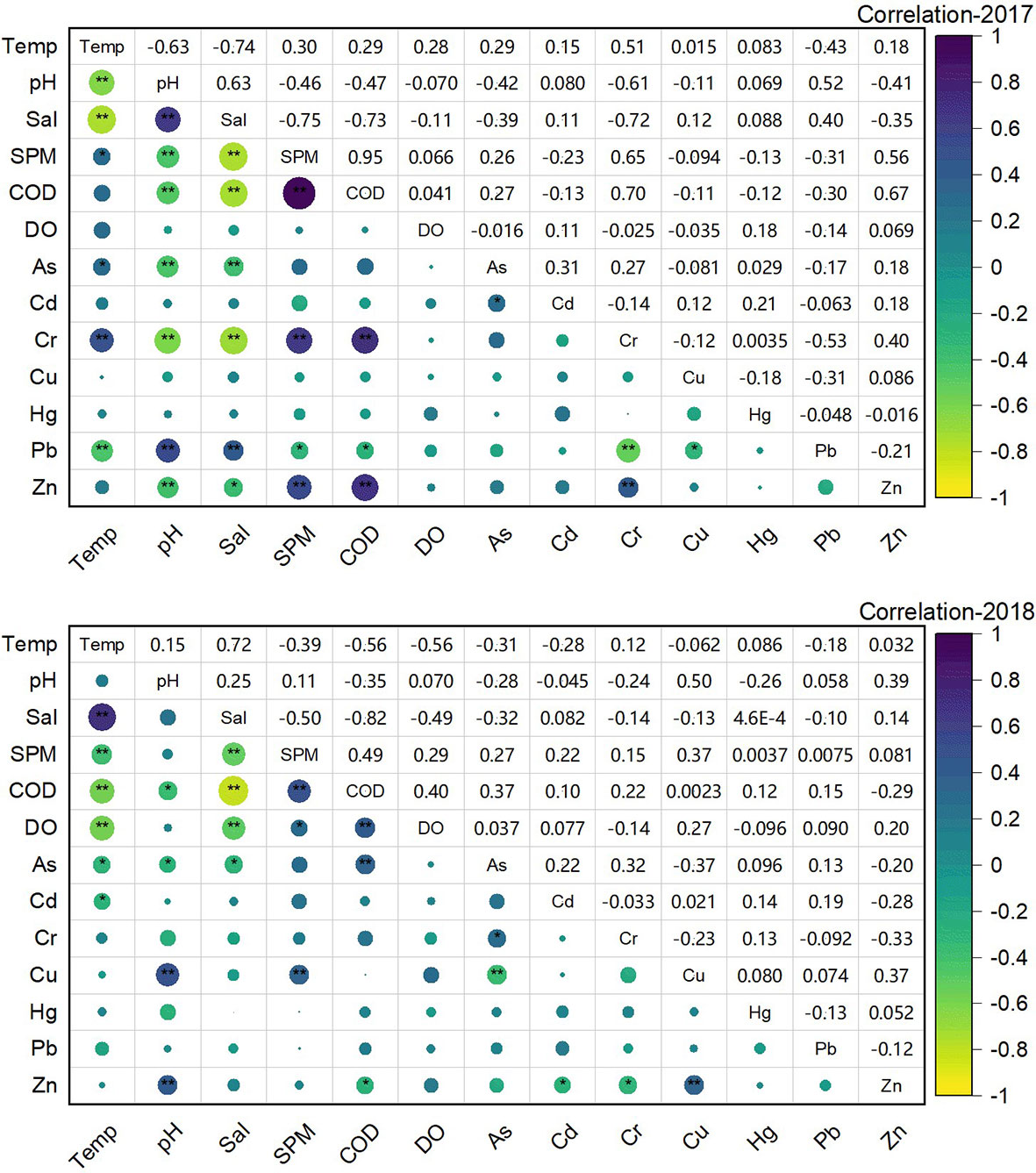
Figure 7 The correlation analysis of heavy metals in the seawater of coastal Zhuhai in 2017 and 2018, respectively (*P < 0.05; **P <0.01).
Kd is the specific coefficient employed to quantify the partition and transport of heavy metals between different phases such as soluble and solid. It can be affected by both the characteristics of heavy metals and the nature of sediment and seawater. The results of heavy metal enrichment in coastal Zhuhai are shown in Table 2. In this study, the order of value Kd in both 2017 and 2018 was Pb > Cr > Cu > As > Zn > Hg > Cd, and the value of Pb was much larger than the value of other heavy metals, which suggested that the heavy metal Pb was most easily transported and enriched from seawater to the nearby sediment environment. From the temporal analysis, the overall Kd values of each heavy metal in 2017 were smaller than the Kd values obtained in 2018, which indicated that the lower seawater temperature was not conducive to the enrichment of heavy metals in the sediment environment. In terms of orientation, the distribution of Kd values of heavy metals was clearly characterized. The Kd values of points near the southwest and southeast coasts are much higher than those in the sea area far from the coast, which indicated that the more submerged the seawater depth was, the easier the heavy metals were enriched.
From Table 3, we can see that the pollution of heavy metals in the sediments along the coast of Zhuhai generally showed a decreasing trend; the concentration of Hg, As, and Cr remained unchanged on the whole; and the concentration of Cu, Pb, Cd, and Zn decreased year by year except for the reasons of data errors in individual years. In general, due to the increased national attention to marine heavy metal pollution in recent years, marine heavy metal concentration may decrease in the next few years.
The BSAF values of each heavy metal except Pb in 2017 and 2018 were less than 1, indicating that only the heavy metal Pb is easily enriched in organisms from seawater or sediment environment. Other heavy metals are not easily enriched in organisms due to their metal properties. This required local managers to pay special attention to the management of heavy metal Pb to prevent elevated biological health risks. Geographically, Pb was more likely to be enriched in organisms in the inner bay of the northeastern part of the Zhuhai-Gaolan Peninsula compared to other locations, indicating that the mobility of seawater also had an influence on the bioconcentration of Pb. The highly mobile offshore area was less prone to Pb enrichment and was suitable for mariculture production activities in this area. The above results were also verified by BAF.
From the perspective of heavy metal classification, the main source of As was aquaculture in the sea area of coastal Zhuhai. In the southern sea area, aquaculture was the main source of the local economy, the large area of aquaculture caused the disorderly placement of aquaculture feed, and the discharged seawater will then cause the accumulation of As in the seawater flowing through the sea area (Xu Y. et al., 2018). Pb, Zn, Cu, and other heavy metals were mainly from the local fine processing industries such as precision electronic instruments, chips, electronic product parts processing, and other industries. Coastal Zhuhai is a national industrial park established by the Chinese government in Zhuhai, equipped with a large number of precision electronic processing industries, which inevitably affect the surrounding seawater environment in the process of continuous development and growth, thus causing the accumulation of heavy metals in the sediment.
3.4 Ecological Risk Assessment
The potential biohazard effects of heavy metals in this sea area, calculated according to the species sensitivity analysis function, are shown in Figure 8 and Table S3. It can be seen that the biohazard coefficient of heavy metals in the study area was significantly higher in Autumn 2017 than in Spring 2018, which may be due to the weakened seawater activity and elevated heavy metal concentration due to low temperatures in autumn. From the analysis of heavy metal species, it was found that Cd, Cu, and Zn were the main heavy metals causing biohazard, while As, Hg, and Pb did not produce heavy metal biohazard. Taken together, the potential biohazard coefficients and ratios (more than 6%) in the northeastern sea area are significantly higher than those in other sea areas. Based on the heavy metal characterization of ocean dynamics, this was caused by seawater currents and coastal topography (Jahan and Strezov, 2018; Khalid et al., 2021). This requires the local ecological and environmental protection department to pay close attention to the protection of marine ecology in the northeast.
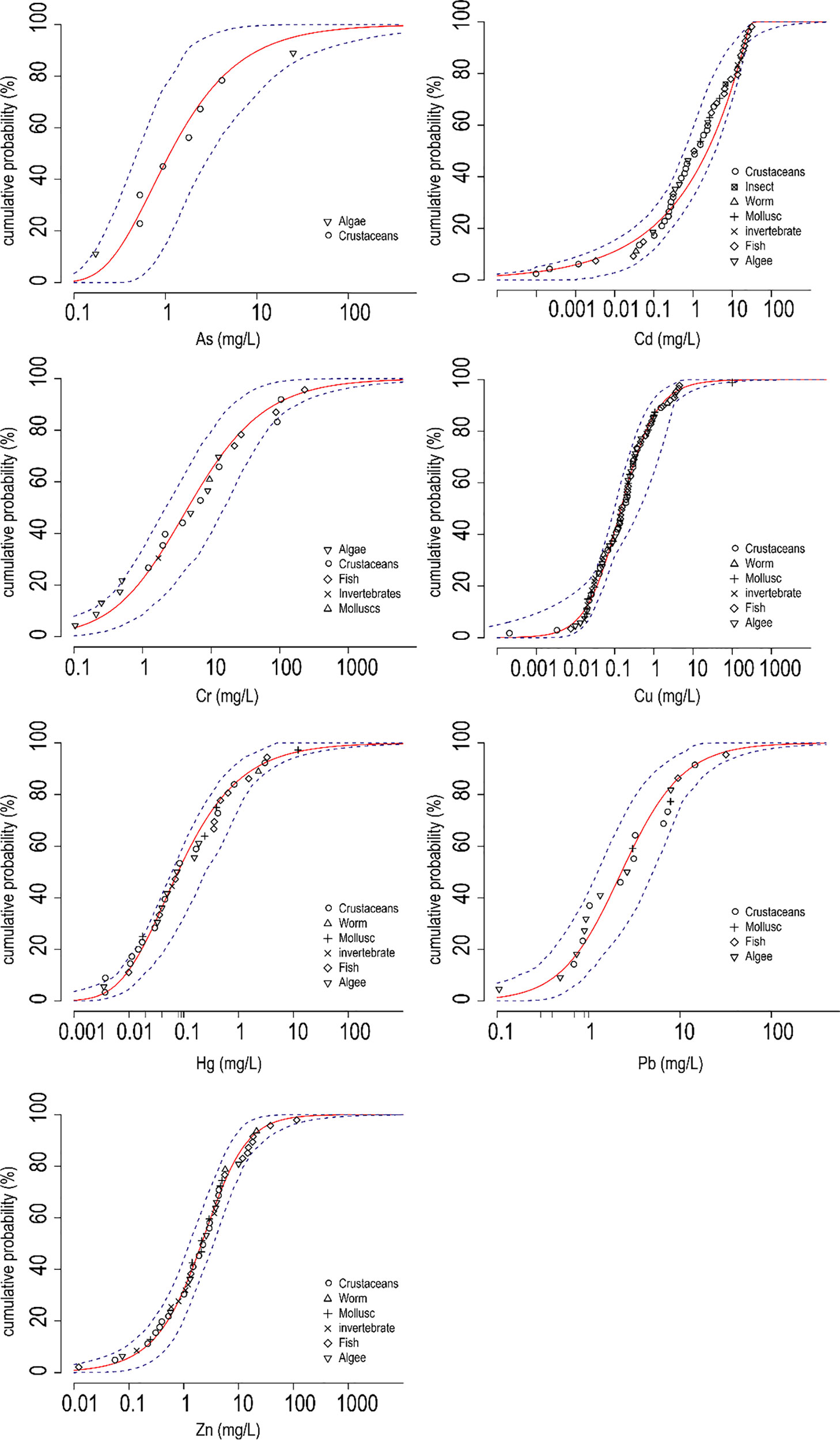
Figure 8 The correlation analysis of heavy metals in the seawater of coastal Zhuhai in 2017 and 2018, respectively.
In addition, the spatial distribution of bio-sensitivity of heavy metals in the sea area of coastal Zhuhai has obvious directionality. From Figure 9, it was found that the bio-sensitivity survey points were all located in the southeast and southwest areas of coastal Zhuhai, and these two areas were also the main precision instrument processing areas in coastal Zhuhai. A large number of metals processing several times the heavy metals discharged from the production of processing industries were accumulated in the sediment, which was the main reason for the significantly higher risk of heavy metal bio-sensitivity in these areas than in other areas. The biological species and biological quality in the sea area were greatly affected by the heavy metal concentration, and the number of biological populations and biological quality that suffer from the risk crisis can also seriously affect the ecological and environmental safety of the sea area in this region (Jawad et al., 2019; Han et al., 2020). So there is a need to carry out wastewater disposal for precision instrument processing enterprises in the southeast and southwest, which can cause the bio-sensitivity crisis collection to reduce the bioecological risk in the region.
3.5 Priority Control and Recommendations
In general terms, few previous studies showed spatial differences in heavy metal contamination on the Zhuhai coast, but while comparing the data with seasonal responses in this study, Cu, Pb, and Zn demonstrated higher values in winter in seawater and sediment, especially near the coast, and this might indicate that organisms near the coast suffered pro-oxidant heavy metal enrichment pressures during the cold season. This explained why organisms, especially invertebrates’ biochemical responses, would be higher in winter, which is the beginning of the reserve accumulation period on the Zhuhai coast. From the perspective of aquaculture production, the survey of heavy metals distribution on the Zhuhai coast can further improve the quality of marine products and effectively evade the pollution areas. From the perspective of environmental management, the data of HM distribution and risk characteristics can provide accurate information for precise heavy metal pollution control and avoid wasting government resources for ecological protection.
Heavy metals are widespread in aquatic environments on the Zhuhai coast, and understanding their distribution at the local scare is necessary to advise marine environmental protection policymakers. The key issue for heavy metal contamination on the Zhuhai coast is the scarcity of emission control, and it is linked to coastal areas of industrial production, which do not have any option other than polluted rivers as sources for sewage discharge. Industrial wastewater treatment needs massive expenditure and reduces local economic revenue due to the installation of costly treatment installations. For better safeguarding and management of the marine environment including organisms, it is paramount that regional heavy metal polluting enterprises implement the most stringent wastewater treatment and discharge control measures in water bodies. Consequently, provisions and specialized supervision departments and supervisors should be introduced, consisting of GIS online monitoring in order to tap into local contamination information, as well as to recognize pollution sources and information requirements.
Our research exhibited that the cumulative effects of different heavy metals in seawater and sediment were different, indicating that a single evaluation method was not useful to evaluate heavy metal contamination on the Zhuhai coast. The heavy metal distribution characteristics and ecological risk curve evaluation system established in this study can effectively assess the cumulative effects of heavy metals in seawater, sediment, and organisms and can provide scientific and effective information support for the next phase of ecological restoration work. In addition, advance planning is needed based on Zhuhai’s special geographical environment and industrial layout. This requires the local government to plan in advance the special disposal institutions and control areas for heavy metal wastewater in order to centralize the management and purification of heavy metal wastewater within the industrial park and to achieve zero pollution discharge. Therefore, the governmental environmental protection department, enterprise regulatory agencies, and local resident representatives need to participate in the development of the regional heavy metal control plan.
4 Conclusions
In the present study, multi-evaluation methods have been employed to investigate the heavy metal pollution on the Zhuhai coast. The results showed that the study area was heavily polluted by heavy metals, especially on the north coast of Gaolan Island. Comparative studies revealed that the main source of heavy metals was the discharge of local industrial wastewater, especially in offshore waters. The SSD evaluation of organisms showed that the northern part of the Zhuhai coast was in medium risk conditions, with high pollution levels of Cd and Cu.
Overall risk distribution patterns of heavy metals and zonation of SSD obtained from this study could support policymakers to take coherent action based on pollution control measures and marine aquaculture. Our research suggested that chemical fractionation should be considered to provide a more accurate assessment of the ecological risks of heavy metals in marine ecosystems. In addition, marine resources in the northern part of the Zhuhai coast may be more vulnerable to anthropogenic pollution. Our research results provided important information on the ecological response of organisms experiencing heavy metal contamination on the Zhuhai coast. Future studies should focus on integrated prevention and control strategies to ensure better conservation of the coastal and estuarine ecosystem.
Data Availability Statement
The original contributions presented in the study are included in the article/Supplementary Material. Further inquiries can be directed to the corresponding author.
Author Contributions
JW and SR developed the methodology. JW, SR, and MW wrote the original manuscript. JW and JL revised the manuscript. RL is responsible for contacting the editorial department and collecting data. All authors contributed to the article and approved the submitted version.
Funding
This study was supported by the South China Sea Marine Survey Technology Center, Key Laboratory of Marine Environmental Detection Technology and Application, Ministry of Natural Resources of China, Project Number: MESTA-2020-C002.
Conflict of Interest
The authors declare that the research was conducted in the absence of any commercial or financial relationships that could be construed as a potential conflict of interest.
Publisher’s Note
All claims expressed in this article are solely those of the authors and do not necessarily represent those of their affiliated organizations, or those of the publisher, the editors and the reviewers. Any product that may be evaluated in this article, or claim that may be made by its manufacturer, is not guaranteed or endorsed by the publisher.
Supplementary Material
The Supplementary Material for this article can be found online at: https://www.frontiersin.org/articles/10.3389/fmars.2022.898423/full#supplementary-material
References
Abaya L. M., Wiegner T. N., Colbert S. L., Beets J. P., Carlson K. M., Kramer K. L., et al. (2018). A Multi-Indicator Approach for Identifying Shoreline Sewage Pollution Hotspots Adjacent to Coral Reefs. Mar. Pollut. Bullet. 129 (1), 70–80. doi: 10.1016/j.marpolbul.2018.02.005
Anusuya T., Kumar V., Kumar V. (2021). Hydrophilic Graphene Quantum Dots as Turn-Off Fluorescent Nanoprobes for Toxic Heavy Metal Ions Detection in Aqueous Media. Chemosphere 282, 131019. doi: 10.1016/j.chemosphere.2021.131019
Ashayeri N. Y., Keshavarzi B. (2019). Geochemical Characteristics, Partitioning, Quantitative Source Apportionment, and Ecological and Health Risk of Heavy Metals in Sediments and Water: A Case Study in Shadegan Wetland, Iran. Mar. Pollut. Bullet. 149, 110495. doi: 10.1016/j.marpolbul.2019.110495
Birch G. F. A. (2018). Review of Chemical-Based Sediment Quality Assessment Methodologies for the Marine Environment. Mar. Pollut. Bullet. 133, 218–232. doi: 10.1016/j.marpolbul.2018.05.039
Cadée G. C., Hegeman J. (1974). Primary Production of Phytoplankton in the Dutch Wadden Sea. Neth. J. Sea Res. 8 (2-3), 240–259. doi: 10.1016/0077-7579(74)90019-2
Cui L., Wang X., Li J., Gao X., Zhang J., Liu Z. (2021). Ecological and Health Risk Assessments and Water Quality Criteria of Heavy Metals in the Haihe River. Environ. Pollut. 290, 117971. doi: 10.1016/j.envpol.2021.117971
Fang L., Li L., Qu Z., Xu H., Xu J., Yan N. (2018). A Novel Method for the Sequential Removal and Separation of Multiple Heavy Metals From Wastewater. J. Haz. Mat. 342, 617–624. doi: 10.1016/j.jhazmat.2017.08.072
Fei Y., Wang X., Liang G., Gu Y., Lin Q., Ning J., et al. (2016). Fuzzy Comprehensive Assessment of Heavy Metals and Pb Isotopic Signature in Surface Sediments From a Bay Under Serious Anthropogenic Influences: Daya Bay, China. Ecotoxicol. Environ. Safe. 126, 38–44. doi: 10.1016/j.ecoenv.2015.12.011
Han R., Zhou B., Huang Y., Lu X., Li N. (2020). Bibliometric Overview of Research Trends on Heavy Metal Health Risks and Impacts in 1989–2018. J. Clean. Product. 276, 123249. doi: 10.1016/j.jclepro.2020.123249
Huang Y., Zhou B., Li N., Li Y., Han R., Qi J., et al. (2019). Spatial-Temporal Analysis of Selected Industrial Aquatic Heavy Metal Pollution in China. J. Clean. Product. 238, 117944. doi: 10.1016/j.jclepro.2019.117944
Hu C., Shui B., Yang X., Wang L., Zhang X. (2021). Trophic Transfer of Heavy Metals Through Aquatic Food Web in a Seagrass Ecosystem of Swan Lagoon, China. Sci. Tot. Environ. 762, 143139. doi: 10.1016/j.scitotenv.2020.143139
Jahan S., Strezov V. (2018). Comparison of Pollution Indices for the Assessment of Heavy Metals in the Sediments of Seaports of NSW, Australia. Mar. Pollut. Bullet. 128, 295–306. doi: 10.1016/j.marpolbul.2018.01.036
Jawad A., Peng L., Liao Z., Zhou Z., Shahzad A., Ifthikar J., et al. (2019). Selective Removal of Heavy Metals by Hydrotalcites as Adsorbents in Diverse Wastewater: Different Intercalated Anions With Different Mechanisms. J. Clean. Product. 211, 1112–1126. doi: 10.1016/j.jclepro.2018.11.234
Kaikkonen L., Venesjärvi R., Nygård H., Kuikka S. (2018). Assessing the Impacts of Seabed Mineral Extraction in the Deep Sea and Coastal Marine Environments: Current Methods and Recommendations for Environmental Risk Assessment. Mar. Pollut. Bullet. 135, 1183–1197. doi: 10.1016/j.marpolbul.2018.08.055
Khalid N., Aqeel M., Noman A., Khan S. M., Akhter N. (2021). Interactions and Effects of Microplastics With Heavy Metals in Aquatic and Terrestrial Environments. Environ. Pollut. 290, 118104. doi: 10.1016/j.envpol.2021.118104
Kim B. S. M., Angeli J. L. F., Ferreira P. A. L., Mahiques M. D., Figueira R. L. (2018). Critical Evaluation of Different Methods to Calculate the Geoaccumulation Index for Environmental Studies: A New Approach for Baixada Santista – Southeastern Brazil. Mar. Pollut. Bullet. 127, 548–552. doi: 10.1016/j.marpolbul.2017.12.049
Kong Q., Shi X., Ma W., Zhang F., Wei C. (2021). Strategies to Improve the Adsorption Properties of Graphene-Based Adsorbent Towards Heavy Metal Ions and Their Compound Pollutants: A Review. J. Haz. Mat. 415, 125690. doi: 10.1016/j.jhazmat.2021.125690
Kumar V., Parihar R. D., Sharma A., Bakshi P., Rodrigo-Comino J. (2019). Global Evaluation of Heavy Metal Content in Surface Water Bodies: A Meta-Analysis Using Heavy Metal Pollution Indices and Multivariate Statistical Analyses. Chemosphere 236, 124364. doi: 10.1016/j.chemosphere.2019.124364
Li S., Chen J. (2014). Discussion on Air Temperature Change in Zhuhai in Recent 49 Years and the Effects of Urbanization. J. Meteorol. Res. Appl. 35, 02, 40–45.
Li H., Gao X., Gu Y., Wang R., Xie P., Liang M., et al. (2018a). Comprehensive Large-Scale Investigation and Assessment of Trace Metal in the Coastal Sediments of Bohai Sea. Mar. Pollut. Bullet. 129 (1), 126–134. doi: 10.1016/j.marpolbul.2018.02.022
Li H., Sun Z., Qiu Y., Yu X., Han X., Ma Y. (2018b). Integrating Bioavailability and Soil Aging in the Derivation of DDT Criteria for Agricultural Soils Using Crop Species Sensitivity Distributions. Ecotoxicol. Environ. Safe. 165, 527–532. doi: 10.1016/j.ecoenv.2018.09.035
Liu Y., Kuang W., Xu J., Chen J., Sun X., Lin C., et al. (2022). Distribution, Source and Risk Assessment of Heavy Metals in the Seawater, Sediments, and Organisms of the Daya Bay, China. Mar. Pollut. Bullet. 174, 113297. doi: 10.1016/j.marpolbul.2021.113297
Liu Q., Wang F., Meng F., Jiang L., Li G., Zhou R. (2018). Assessment of Metal Contamination in Estuarine Surface Sediments From Dongying City, China; Use of a Modified Ecological Risk Index. Mar. Pollut. Bullet. 126, 293–303. doi: 10.1016/j.marpolbul.2017.11.017
Liu J., Wu J., Feng W., Li X. (2020). Ecological Risk Assessment of Heavy Metals in Water Bodies Around Typical Copper Mines in China. Int. J. Environ. Res. Public Health 17 (12), 4315. doi: 10.3390/ijerph17124315
Luo L., Mei K., Qu L., Zhang C., Chen H., Wang S., et al. (2019). Assessment of the Geographical Detector Method for Investigating Heavy Metal Source Apportionment in an Urban Watershed of Eastern China. Sci. Tot. Environ. 653, 714–722. doi: 10.1016/j.scitotenv.2018.10.424
Majithiya D., Yadav A., Ram A. (2018). Behaviour of Trace Metals in the Anoxic Environment of Veraval Harbour, India. Mar. Pollut. Bullet. 129 (2), 645–654. doi: 10.1016/j.marpolbul.2017.10.052
Pandey V., Jha D. K., Kumar P. S., Santhanakumar J., Venkatnarayanan S., Jebakumar J., et al. (2022). Effect of Multiple Stressors on the Functional Traits of Sub-Tidal Macrobenthic Fauna: A Case Study of the Southeast Coast of India. Mar. Pollut. Bullet. 175, 113355. doi: 10.1016/j.marpolbul.2022.113355
Pandey V., Venkatnarayanan S., Kumar P. S., Ratnam K., Dharani G. (2021). Assessment of Ecological Health of Swarnamukhi River Estuary, Southeast Coast of India, Through AMBI Indices and Multivariate Tools. Mar. Pollut. Bullet. 164, 112031. doi: 10.1016/j.marpolbul.2021.112031
Quyen V. T., Pham T. H., Kim J., Dang M. T., Kim T. (2021). Biosorbent Derived From Coffee Husk for Efficient Removal of Toxic Heavy Metals From Wastewater. Chemosphere 284, 131312. doi: 10.1016/j.chemosphere.2021.131312
Rao K., Tang T., Zhang X., Mo W., Ji E., Bi W., et al. (2021). Spatial-Temporal Dynamics, Ecological Risk Assessment, Source Identification and Interactions With Internal Nutrients Release of Heavy Metals in Surface Sediments From a Large Chinese Shallow Lake. Chemosphere 282, 131041. doi: 10.1016/j.chemosphere.2021.131041
Reddy B. C., Jayaraju N., Sreenivasulu G., Suresh U., Reddy A. N. (2016). Heavy Metal Pollution Monitoring With Foraminifera in the Estuaries of Nellore Coast, East Coast of India. Mar. Pollut. Bullet. 113, 542–551. doi: 10.1016/j.marpolbul.2016.08.051
Satheeswaran A., Yuvaraj P., Damotharan P., Karthikeyan V., Jha D. K., Dharani G., et al. (2019). Assessment of Trace Metal Contamination in the Marine Sediment, Seawater, and Bivalves of Parangipettai, Southeast Coast of India. Mar. Pollut. Bullet. 149, 110499. doi: 10.1016/j.marpolbul.2019.110499
Sruthi S. N., Shyleshchandran M. S., Mohan M., Ramasamy E. V. (2018). Distribution of Priority Pollutants in the Sediment of Vembanad Estuary, Peninsular India. Mar. Pollut. Bullet. 133, 294–303. doi: 10.1016/j.marpolbul.2018.05.033
Wang X., Fu R., Li H., Zhang Y., Xiong Y. (2020). Heavy Metal Contamination in Surface Sediments: A Comprehensive, Large-Scale Evaluation for the Bohai Sea, China. Environ. Pollut. 260, 113986. doi: 10.1016/j.envpol.2020.113986
Wang Q., Li H., Zhang Y., Wang X. J., Zhang C., Xiao K., et al. (2019). Evaluations of Submarine Groundwater Discharge and Associated Heavy Metal Fluxes in Bohai Bay, China. Sci. Tot. Environ. 695, 133873. doi: 10.1016/j.scitotenv.2019.133873
Wang L., Wang Y., Ma F., Tankpa V., Bai S., Guo X., et al. (2019). Mechanisms and Reutilization of Modified Biochar Used for Removal of Heavy Metals From Wastewater: A Review. Sci. Tot. Environ. 668, 1298–1309. doi: 10.1016/j.scitotenv.2019.03.011
Wang R., Zhang C., Huang X., Zhao L., Yin D. (2020). Distribution and Source of Heavy Metals in the Sediments of the Coastal East China Sea: Geochemical Controls and Typhoon Impact. Environ. Pollut. 260, 113936. doi: 10.1016/j.envpol.2020.113936
Wang C., Zou X., Feng Z., Hao Z., Gao J. (2018). Distribution and Transport of Heavy Metals in Estuarine–Inner Shelf Regions of the East China Sea. Sci. Tot. Environ. 644, 298–305. doi: 10.1016/j.scitotenv.2018.06.383
Wu W., Wu P., Yang F., Sun D., Zhang D., Zhou Y., et al. (2018). Assessment of Heavy Metal Pollution and Human Health Risks in Urban Soils Around an Electronics Manufacturing Facility. Sci. Tot. Environ. 630, 53–61. doi: 10.1016/j.scitotenv.2018.02.183
Xia P., Ma L., Sun R., Yang Y., Yi Y. (2020). Evaluation of Potential Ecological Risk, Possible Sources and Controlling Factors of Heavy Metals in Surface Sediment of Caohai Wetland, China. Sci. Tot. Environ. 740, 140231. doi: 10.1016/j.scitotenv.2020.140231
Xiao J., Han X., Sun S., Wang L., Rinklebe J. (2021). Heavy Metals in Different Moss Species in Alpine Ecosystems of Mountain Gongga, China: Geochemical Characteristics and Controlling Factors. Environ. Pollut. 272, 115991. doi: 10.1016/j.envpol.2020.115991
Xu J., Cao Z., Zhang Y., Yuan Z., Lou Z., Xu X., et al. (2018). A Review of Functionalized Carbon Nanotubes and Graphene for Heavy Metal Adsorption From Water: Preparation, Application, and Mechanism. Chemosphere 195, 351–364. doi: 10.1016/j.chemosphere.2017.12.061
Xu X., Hu X., Wang T., Sun M., Wang L., Zhang L. (2021). Non-Inverted U-Shaped Challenges to Regional Sustainability: The Health Risk of Soil Heavy Metals in Coastal China. J. Clean. Product. 279, 123746. doi: 10.1016/j.jclepro.2020.123746
Xu Y., Xie Y., Zhao P., Zhang T., Zhou Q., Hu B. (2018). Heavy Metal Pollution of Seawater in Weizhou Island, Beibu Gulf of Guangxi. Ecol. Environ. Sci. 27 (05), 908–915. doi: 10.16258/j.cnki.1674-5906.2018.05.016
Xu Z., Zhang Q., Li X., Huang X. (2022). A Critical Review on Chemical Analysis of Heavy Metal Complexes in Water/Wastewater and the Mechanism of Treatment Methods. Chem. Eng. J. 429, 131688. doi: 10.1016/j.cej.2021.131688
Yang T., Cheng H., Wang H., Drews M., Li S., Huang W., et al. (2019). Comparative Study of Polycyclic Aromatic Hydrocarbons (PAHs) and Heavy Metals (HMs) in Corals, Surrounding Sediments and Surface Water at the Dazhou Island, China. Chemosphere 218, 157–168. doi: 10.1016/j.chemosphere.2018.11.063
Ye Z., Chen J., Gao L., Liang Z., Gnanachandrasamy G. (2020). 210Pb Dating to Investigate the Historical Variations and Identification of Different Sources of Heavy Metal Pollution in Sediments of the Pearl River Estuary, Southern China. Mar. Pollut. Bull. 150, 110670. doi: 10.1016/j.marpolbul.2019.110670
Zhang G., Bai J., Xiao R., Zhao Q., Jia J., Cui B. (2017). Heavy Metal Fractions and Ecological Risk Assessment in Sediments From Urban, Rural and Reclamation-Affected Rivers of The Pearl River Estuary, China. Chemosphere 184, 278–288. doi: 10.1016/j.chemosphere.2017.05.155
Zhao M., Wang S., Wang H., Qin P., Yang D., Sun Y., et al. (2019). Application of Sodium Titanate Nanofibers as Constructed Wetland Fillers for Efficient Removal of Heavy Metal Ions From Wastewater. Environ. Pollut. 248, 938–946. doi: 10.1016/j.envpol.2019.02.040
Zhao G., Ye S., Yuan H., Ding X., Wang J. (2017). Surface Sediment Properties and Heavy Metal Pollution Assessment in the Pearl River Estuary, China. Environ. Sci. Pollut. 24, 2966–2979. doi: 10.1007/s11356-016-8003-4
Zhao G., Ye S., Yuan H., Ding X., Wan J., Laws E. A. (2018). Surface Sediment Properties and Heavy Metal Contamination Assessment in River Sediments of the Pearl River Delta, China. Mar. Pollut. Bullet. 136, 300–308. doi: 10.1016/j.marpolbul.2018.09.035
Keywords: heavy metals, water quality assessment, ecological risk assessment, species sensitivity distribution, marine
Citation: Wu J, Rong S, Wang M, Lu R and Liu J (2022) Environmental Quality and Ecological Risk Assessment of Heavy Metals in the Zhuhai Coast, China. Front. Mar. Sci. 9:898423. doi: 10.3389/fmars.2022.898423
Received: 18 March 2022; Accepted: 18 April 2022;
Published: 20 May 2022.
Edited by:
Dilip Kumar Jha, National Institute of Ocean Technology, IndiaReviewed by:
Satheeswaran Thangaraj, Incheon National University, South KoreaThilagam Harikrishnan, Pachaiyappa’s College for Men, India
Biraja Kumar Sahu, Council of Scientific and Industrial Research (CSIR), India
Copyright © 2022 Wu, Rong, Wang, Lu and Liu. This is an open-access article distributed under the terms of the Creative Commons Attribution License (CC BY). The use, distribution or reproduction in other forums is permitted, provided the original author(s) and the copyright owner(s) are credited and that the original publication in this journal is cited, in accordance with accepted academic practice. No use, distribution or reproduction is permitted which does not comply with these terms.
*Correspondence: Rong Lu, lurong@smst.gz.cn