Effects of Environmental Factors on the Abundance, Biomass, and Individual Weight of Juvenile Red King Crabs in the Barents Sea
- Murmansk Marine Biological Institute (MMBI), Murmansk, Russia
We examined the effects of environmental factors (water temperature, the North Atlantic Oscillation winter index – NAOw, and cod biomass) on the abundance (population number), biomass and mean individual weight of juvenile red king crab (Paralithodes camtschaticus) at a typical coastal site of the Barents Sea, Dalnezelenetskaya Bay, a place of red king crab introduction. Current-year abundance and stock data for 3–5-year-old crabs were positively related to water temperature in September and October. The biomass of crabs at age 0–2 year was positively correlated with lag 1 water temperature in August, while the abundance of age 0–5 year was positively correlated to the NAOw indicating more favorable habitat conditions in warmer periods. The abundance of crabs at age 3–5 year was negatively correlated to December temperature and mean water temperature and temperature anomaly with a 1-year lag. These temperature relationships, together with negative correlations between stock indices of crabs and cod (Gadus morhua) and positive relationships between mean crab weight and cod biomass, suggests that the fish predation, which is higher in warmer years, has a more important role in mortality of 3–5 year crabs than 0–2 year crabs. Our findings may be useful for modeling recruitment processes and management of red king crab in the Barents Sea.
Introduction
In the marine environment, many species of bottom organisms have long life spans. These characteristics provide the potential for benthic fauna to integrate environmental fluctuations over long and short time scales (Denisenko, 2007; Frolova et al., 2007; Johnson et al., 2020).
The red king crab, Paralithodes camtschaticus (Tilesius, 1815) is a native species of the North Pacific region. It was introduced into the Barents Sea by soviet scientists during the 1960s to increase the economic potential of the local fishery. In the Barents Sea, the crab has formed a new self-sustaining population. In Russia, the commercial fishery of the Barents Sea red king crab started in 2004 (Dvoretsky and Dvoretsky, 2015, 2018).
Recently, we analyzed the effects of environmental factors on the stock characteristics P. camtschaticus in the open sea beyond the 12 nautical mile zone (Dvoretsky and Dvoretsky, 2016). We found that red king crab abundance indices were negatively correlated to the North Atlantic Oscillation index (NAO, an integrated index reflecting atmospheric pattern of seasonal and long-term fluctuations in the North Atlantic) in August and positively related to water temperature in late winter and early summer. Both total and commercial stocks were negatively related to the Arctic Oscillation index in August and the winter NAO index at lags 1 and 2, respectively. NAO indices in February and August (lags 9 and 10) were positively associated with the commercial stock of red king crab (Dvoretsky and Dvoretsky, 2016).
However, the response of commercial fish and crustaceans to changing climate conditions may vary widely due to fishery activities and other direct and indirect effects of environmental and anthropogenic factors (Glantz, 1992). Taking into account that fishing is one of the factors contributing to the decline of many exploited stocks, a deteriorating environment related to climate change together with overfishing may reduce the stocks below the levels of minimum viable abundances (Orensanz et al., 1998). For this reason, abundance indices of juveniles of commercial species that have no or negligible fishery impact, low migration activity, and high local concentrations, may be more appropriate for analyzing the linkages between population processes and environmental factors such as climate shifts and predation pressure (Sanchez-Rubio et al., 2011). Such studies can provide significant insight into demographic traits and recruitment patterns of commercial species.
Estimates suggest that, in 2003–2006, the number of juvenile crabs in the coastal Barents Sea varied from 28–163 million individuals (Sokolov and Milyutin, 2006, 2007). P. camtschaticus juveniles have an important ecological significance at shallow water sites and provides the basis of recruitment of the commercial stock (Dvoretsky and Dvoretsky, 2013), but there are no complete studies describing links between stock indices of immature crabs and temperature conditions and predator concentrations. The main predators of red king crab in the Barents Sea are cod, wolffish and skates (Kuzmin and Gudimova, 2002).
The purpose of the present study is to examine the relationship between the environmental variables and the abundance indices of immature red king crabs. We hypothesized that the crab stock indices are positively correlated with climatic conditions and negatively with cod biomass.
Materials and Methods
Study Area
Dalnezelenetskaya Bay is a shallow-water semi-closed small bay with five islands separating the area of the bay from the open sea (Figure 1). Dalnezelenetskaya Bay is almost square with a size ca. 1 × 1 nautical miles (total area 2.23 km2). The maximum depths are registered in the western part of the bay. Mean depth is 7 m. Depth range in adjacent sea areas considered in this study is 30–40 m. High tidal levels (3–4 m) ensure intensive water exchange between the inner part of Dalnezelenetskaya Bay and the open sea. According to 2002–2012 data, temperature minimum in the upper water layer (surface) occurs in February (0.7°C) and the maximum in August (9.7°C) although in some years the maximum could be observed in July (Il’in et al., 2016). The lowest salinity level (32.2 psu) is usually registered in May and associated with high input of melt water. In autumn and winter, salinity is stabilized at a level of 34 psu (Il’in et al., 2016). The minimum level of dissolve oxygen is registered in December (94%), the maximum in May (124%) (Dvoretsky and Dvoretsky, in press).
Crabs
Red king crabs were collected in Dalnezelenetskaya Bay in July–August 2002–2013 by divers at depths 5–40 m. Each crab was sexed and the carapace length (CL, mm) was measured. In this work we considered juvenile crabs to have a CL < 90 mm (Dvoretsky and Dvoretsky, 2018). The crabs were divided into two age groups: 0–2 year-old (1–45 mm CL) and 3–5 year-old (46–90 mm CL) according to Dvoretsky and Dvoretsky (2014). Dalnezelenetskaya Bay is a nursery area for red king crabs. In spring, after mating, most of mature males migrate to deeper water, while female crabs tend to stay at this site until autumn. All juveniles at age 0–2 stay in the bay, while 3–5-year-old crabs can migrate at adjacent shallow-water sites (Dvoretsky and Dvoretsky, 2013).
The area of the bay was divided into several parts with the same types of biological communities (benthic organisms living on hard and soft grounds, kelps and sand). A standard transect grid, which consisted of 10–25 transects covering a wide range of depths and different types of benthic communities, was established (Dvoretsky and Dvoretsky, 2013). The number of crabs and their biomass on each transect line as well as the transect length and depth and observation width (15 m) were used as primary data to estimate the total abundance (the total number of crabs) and the total biomass by a spline approximation method (the approximate representation of a function in a given class from incomplete information using splines, Stolyarenko, 1986) with the Chartmaster Software Tool (Bizikov et al., 2007). In addition to the stock indices, the analyses were also applied for averaged individual weights (g) of different aged crabs. During the study period, the total number of juvenile crabs sampled by divers varied from 5 (in 2008) to 199 (in 2005) individuals. The majority of crabs at age 0–2 (52%) was collected at 8–10 m depths, whereas 3–5-year old crabs were the most abundant (53%) at 18–22 m depths.
Abiotic and Biotic Factors
We used mean sea temperatures and information on temperature and salinity anomalies from a long-term data set collected by Polar Institute of Fisheries and Oceanography (PINRO, now Polar branch of Russian Federal Research Institute of Fisheries and Oceanography)1. As climatic conditions of the southern Barents Sea are determined by temperature characteristics of Murmansk coastal waters, we used averaged values in different layers obtained at stations in the standard transect called “Kola Section.” The water temperature variables considered in the analysis were obtained from stations 1–3 of the Kola Section in the 50–200 m layer because these values quite adequately correspond to near bottom temperature variables. These variables consisted of mean water temperature in January–December, mean yearly temperatures, and temperature anomalies. Salinity variables were taken from the 0–200 m layer of the Kola Section; these variables included mean yearly salinities and salinity anomalies at stations. In addition, we used the North Atlantic Oscillation Winter Index (NAOw), which was calculated as the average of the monthly indices between December and March inclusive (Hurrell et al., 2003) and was obtained from the CDG site2. The NAOw for year x included the December value in year x–1. Positive NAOw indicates a dominance of southwesterly winds that leads to lower Polar surface air pressure, large transport of Atlantic Water into the Arctic Ocean and higher water temperatures in the Barents Sea including the Kola Section (Sakshaug et al., 2009).
The stock indices of Northeast Arctic cod (Gadus morhua), i.e., recruit biomass, total biomass, and total spawning biomass, were included in the correlation analysis owing to predation on crabs from cod in the bay and similarity in trends of cod dynamics observed in the Barents Sea and in Dalnezelenetskaya Bay (Karamushko et al., 2014). Cod stock indices were taken from ICES (2016).
Data Analysis
To assess potential environmental drivers of the system, the Pearson’s product-moment correlations (alpha = 0.05) among environmental variables and the crab stock indices were investigated, including lag periods of up to 5 years according to the maximum age of a juvenile crab. This is reasonable considering the impact on recruitment depending on growth from juvenile to adult. Normality and homogeneity of variances were tested prior to performing the statistical analyses. The assumption of homogeneity of variance was verified with a Levene’s test and the assumption of normality with a Shapiro-Wilk’s test. The variables that did not meet the criteria for the parametric tests were log-transformed. The Benjamini-Hochberg procedure was used to correct p-values for multiple testing (McDonald, 2009). In each case, the total number of tests was 20 and a false discovery rate was set at 0.25.
Additionally, we used canonical correspondence analysis (CCA). CCA is a technique that has the advantage of directly relating biological data to explanatory sets of variables (environmental descriptors) (Leps and Smilauer, 2003). CCA was used because preliminary tests indicated that the length of gradient was >4 (ter Braak and Smilauer, 2002). Forward selection was performed in order to test the statistical significance of environmental variables that contributed most strongly to the canonical model. Selected environmental variables were included to the model when their F-ratios were >5% of the simulated F-values generated by 999 Monte Carlo permutations (i.e., p < 0.05) (ter Braak and Smilauer, 2002).
Statistical tests were performed in CANOCO 4.5 and Statistica 6.0.
Results
Abiotic and Biotic Factors
In 1997–2013, climatic conditions varied strongly in the Barents Sea. Fluctuations of mean temperature are presented in Figures 2A–D. The warm temperature anomalies reached their maximum levels in the period of 2001–2006, followed by a smooth decrease in recent years with a new peak in 2012 (Figure 2F). In 2013, water temperature was close to the long-term mean.
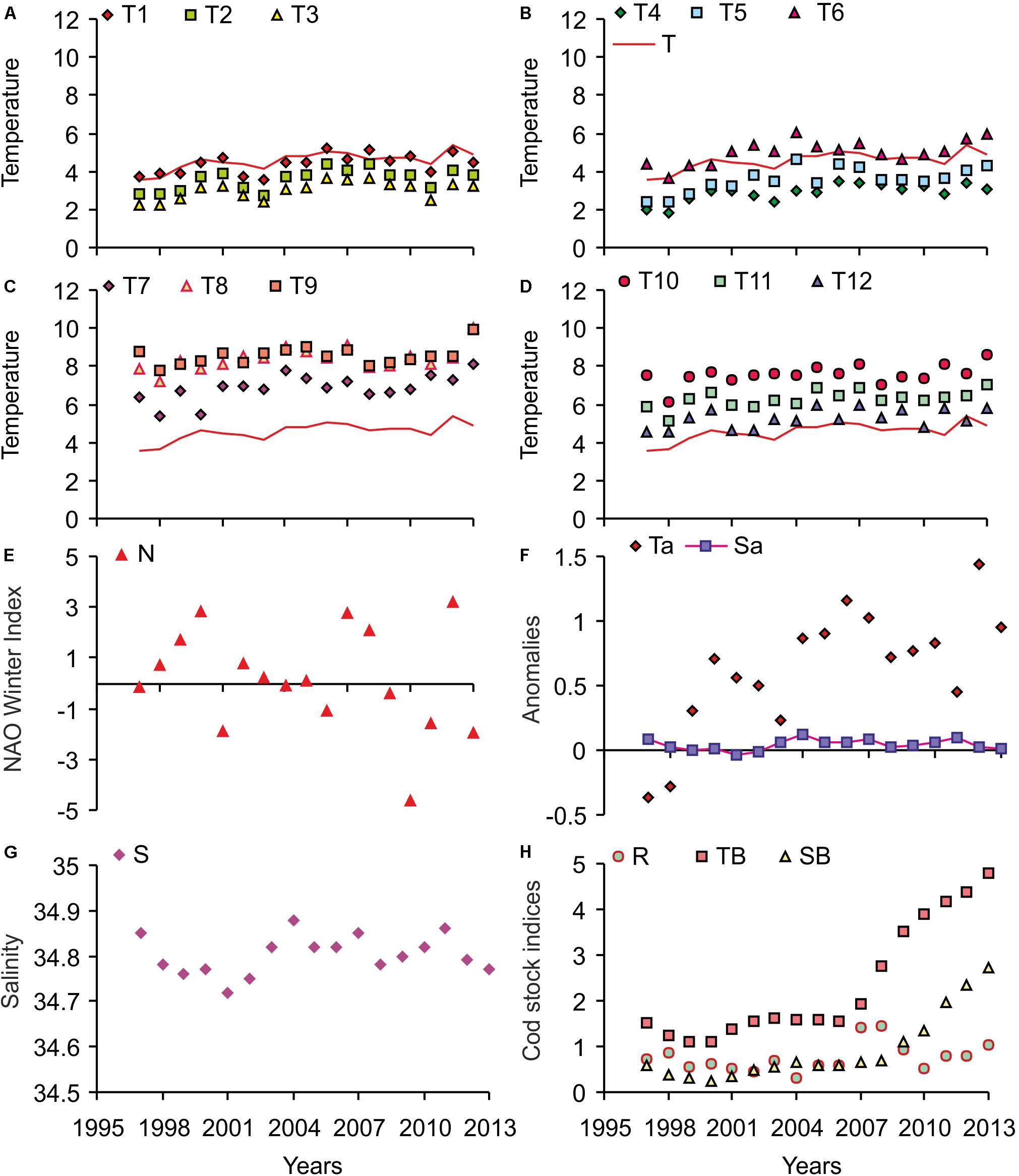
Figure 2. Variations in abiotic and biotic factors in 1997–2013. T1–T12 – mean monthly water temperature at stations 1–3 of the Kola section in January (T1)–December (T12), T – averaged year water temperature (A–D), N – North Atlantic Oscillation Winter Index (E), Ta – temperature anomaly (F), S – salinity (G), Sa – salinity anomaly (F), R – cod recruit biomass, TB – cod total biomass, SB – cod spawning biomass (H). Cod stock indices are expressed in million metric tons.
Figure 2E shows variations of the NAOw in 1997–2013. Since 1997, positive values of the NAOw were recorded, which correspond to warm bottom temperatures in the southern Barents Sea. Since 2007 a cooling trend was registered, and in 2009–2011, the NAOw indices were negative. The minimum value of the NAOw for the whole study period (–4.64) occurred in 2010. Both salinity and salinity anomalies had low-level variations during the study period (Figures 2F,G).
Cod recruit biomass changed relatively little in 1997–2006 (Figure 2H). In the subsequent two years, this parameter dramatically increased, peaking in 2008 at 1,500,000 t. In the next years, cod recruit biomass generally stayed above the long-term mean. Total spawning biomass of cod varied only slightly from 1997–2008. Subsequently, this parameter increased sharply from 1,107,000 t in 2009 to 2,737,000 t in 2013. A similar pattern was registered for total cod biomass (Figure 2H).
Crab Abundance, Biomass, and Weight
The abundance of 0–2 year old crabs oscillated around 1,000 crabs, with the exception of 2006 and 2007, which had over 4,000 crabs (Figure 3A). High abundances of 3–5-year-old crabs were registered in 2002, 2004, 2006, and 2009 (Figure 3B). The maximum level (5,360 crabs) was observed in 2010. Peaks in the total crab number were found at each fourth year during the study period (Figure 3B). After 2010, a sharp decrease in the abundance of crabs was registered at the study site. Biomass dynamics had the same patterns as the abundance indices (Figures 3C,D).
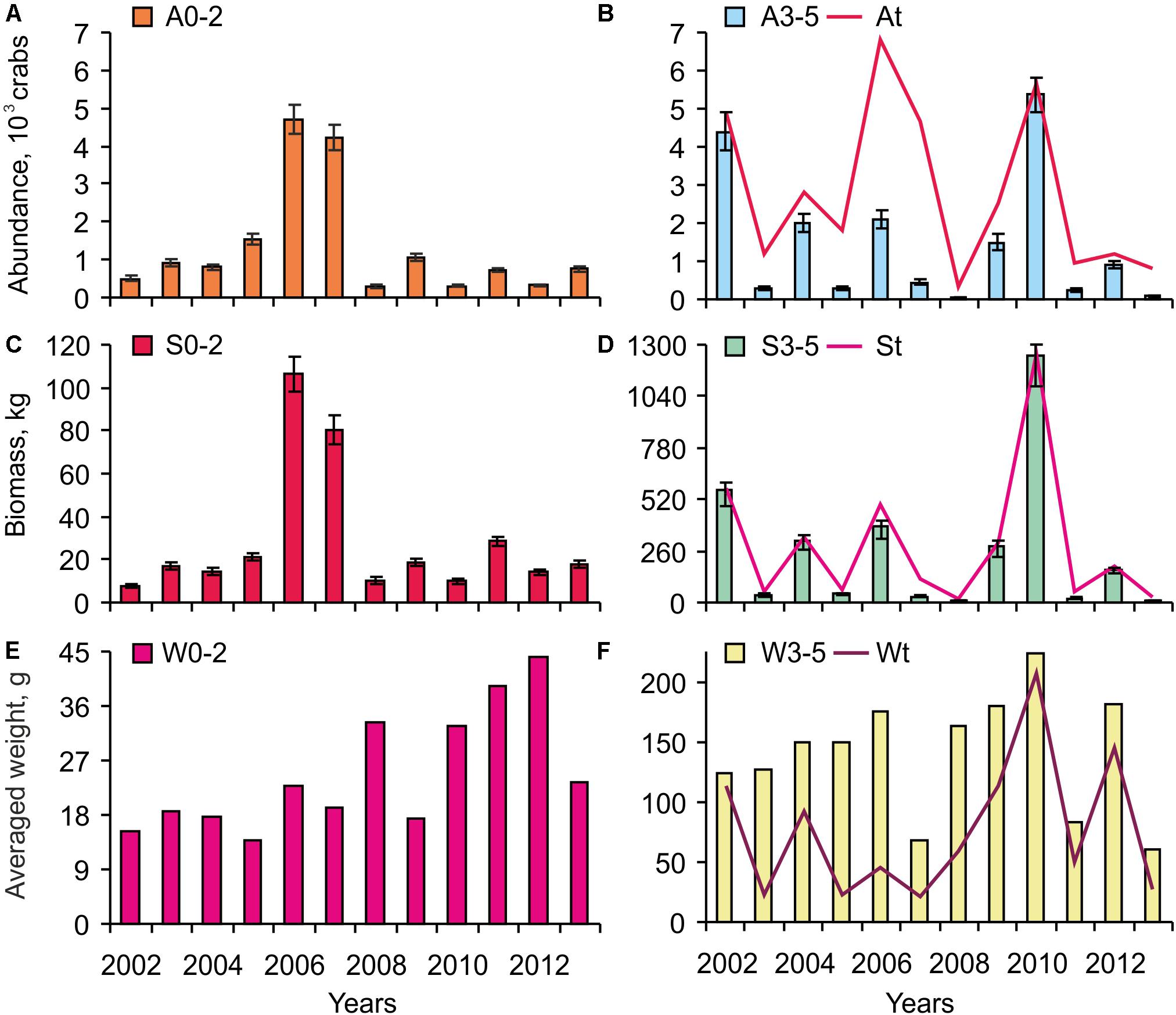
Figure 3. Variations in red king crab indices over 2002–2013. A – abundance (A,B), S – stock (C,D), W – weight (E,F). 0–2 – Age 0–2 year, 3–5 – Age 3–5 year, t – Age 0–5 year. Vertical bars show standard errors.
In general, the mean individual weight of a 0–2-year-old crab had low variations in 2002–2009 (15–20 g), while it gradually increased from 2010–2012 (Figure 3E). In 2013, weight decreased again to a level close to the multiannual average. For crabs at age 3–5, a smooth increase in the mean weight occurred in 2002–2006 and in 2008–2010 (Figure 4F). In 2007, 2011, and 2013, these parameters were as low as 60–80 g. For the entire juvenile stage (0–5 year), the lowest levels were found in 2003, 2005, 2007 and 2013 (21–27 g), and the maximum occurred in 2010 (207.5 g) (Figure 3F).
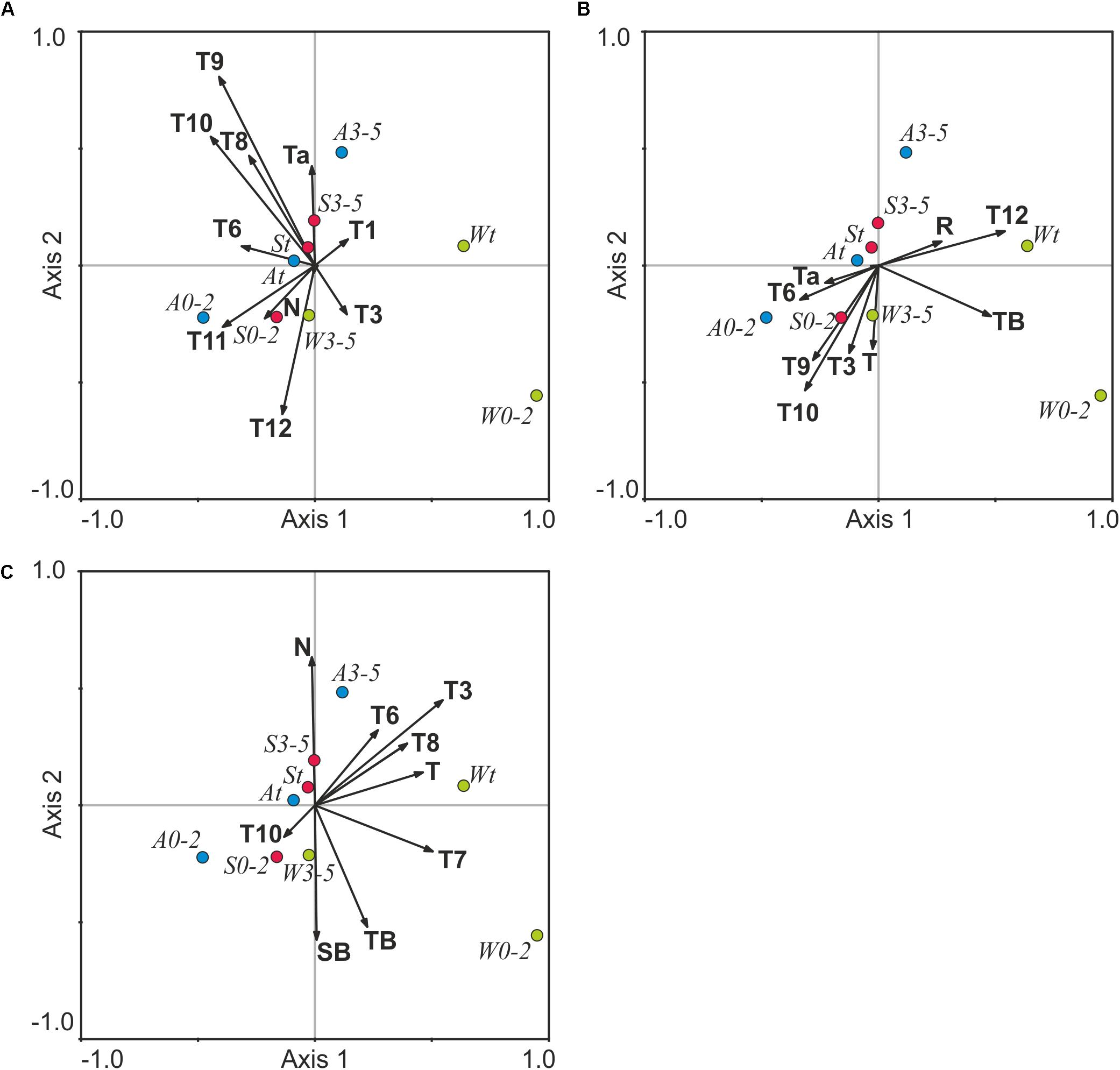
Figure 4. Canonical Correspondence Analysis showing red king crab characteristics in relation to environmental variables in Dalnezelenetskaya Bay at lag 0 (A), lag 1 (B), and lag 2 (C). For abbreviations see Figures 2, 3.
Relationships Between Crab Indices and Environmental Variables
Using the analysis of simple correlations between pairs of target parameters and environmental variables we found that the current-year abundance and biomass data for 3–5-year-old crabs were positively related to water temperature in September and October, and negatively to December values and overall water temperature and the temperature anomaly at lag 1. The biomass of crabs at age 0–2 year was positively correlated with lag 1 water temperature in August. In addition, the abundance of 0–5-year-old crabs was positively correlated to the NAOw with a 2 year lag (Supplementary Tables S1, S2).
Both abundance and biomass of the crabs at age 3–5 as well as the biomass of all juveniles were negatively associated with the stock of cod recruits in the current year. Mean individual weights of 0–2-year-old-crabs had positive correlation with the total biomass of cod at lags 0, 1, and 2, and the spawning cod biomass at lags 0 and 1 (Supplementary Tables S1, S2). There were no significant associations among the red king crab target parameters and environmental factors at lags 3–5.
In general, multivariate CCA confirmed the results obtained by simple correlation analyses. For the current year (lag 0 dataset), the first two CCA axes explained 76% of the total variance in the crab indices and environmental factors. The CCA showed that September-October temperature contributed significantly to the data variation with negative correlations (p = 0.015–0.020) between the species abundance and autumn temperature (Figure 4A and Supplementary Table S3).
For the previous year (lag 1 data set), the majority of environmental variables were negatively associated with the first CCA axis explaining 46.7% of the total variation in the crab indices. December temperature and the total cod biomass had strong correlations with Axis 1 (r = 0.48–0.54, p < 0.05). Along Axis 2, the king crab indices were negatively scaled with environmental variables except for the cod recruit biomass and December temperature (Figure 4B and Supplementary Table S3). This axis explained only 30% of the total variation.
CCA applied to the lag 2 dataset showed that Axes 1 and 2 had eigenvalues of 0.01 and 0.006 and explained 46.8 and 29.2% of the data variation, respectively. The first axis was the only significant component. Along Axis 1, March and July temperature demonstrated a positive significant relationship (p < 0.05) with some crab indices (Figure 4C and Supplementary Table S3).
Discussion
We found that abundant size classes of larger juveniles appeared with lags 2 or 3 after abundant size classes of smaller crabs. This pattern was expressed in a few cases (for example years 2006–2007 and 2009–2010) probably owing to migrations of 3–5-year-old crabs to adjacent areas. The total number and biomass of juvenile P. camtschaticus was most strongly associated with water temperature in late summer and early autumn (Figure 4A) and with the NAOw (Figure 4C and Supplementary Tables). As shown in Figure 2E, in 2010, the NAOw reached its minimum during the study period suggesting the extreme temperature conditions in winter 2010. This also corresponds to the freeze-over of Kola Bay in winter 2010/2011 (Matishov et al., 2012). As a result of extremely low water temperatures at coastal sites, after the period with high abundance indices of red king crabs in the summer 2010 we observed a significant decrease of these parameters during the next 3 years. In 2006–2010, the proportions of immature: mature crabs were 60–100% while in 2011–2013 these levels did not exceed 30% at each year (Dvoretsky and Dvoretsky, 2013).
Temperature of the current and previous year seems to affect natural mortality of both larvae and immature red king crabs (Stevens, 2014a). In the Barents Sea zooplankton, red king crab larvae usually occur in April–June (Dvoretsky and Dvoretsky, 2018). In periods with colder than average water temperatures, king crab embryo development and the molt-mate cycle was found to be delayed (Stevens, 2014b). This delay likely impacts the migration of adult females of P. camtschaticus to spawning areas and their ability to extrude uneyed embryos and also leads to extended embryo development and, therefore, decreases the abundance of red king crabs. Thus, the positive relationships between temperature and other climatic indices and total number of crabs are not surprising (Supplementary Tables).
Another factor that is directly correlated with water temperature is the total biomass of benthic organisms. Studies conducted by Frolova et al. (2007) and Denisenko (2007) have shown that in the Barents Sea, total biomass of boreal biota is increased in warm conditions with 3–7 year lags for some species. The crab diet includes many of the common benthic organisms, and the increase of their biomass is favorable for P. camtschaticus (Dvoretsky and Dvoretsky, 2015).
More interesting findings are the negative correlations found between abundance of 3–5-year-old crabs and temperature conditions of current and previous years (Figure 4B and Supplementary Tables). We suppose that in the case of this age group the impact of temperature conditions is masked by the impact of biotic factors, especially predation by cod on juvenile crabs. In the Barents Sea, P. camtschaticus at age 3–5 demonstrate higher mobility, higher aggression level in response to predation attacks and, therefore, much higher vulnerability to potential predators than smaller crabs which prefer spend their time in refuges and demonstrate pronounced escape actions as a response to dangerous factors (Pereladov, 2003).
Previous studies have shown that the abundance of recruits (age 3 year, body size 40–44 cm), spawning stock biomass, and survival of cod, are related to climate variability (Dippner and Ottersen, 2001). In addition to higher cod stocks, higher migration activity of this species in warmer years leads to its wider distribution in the Barents Sea. For example, over the abnormally warm period of 2004–2006, cod migrations were directed both east and north (Matishov et al., 2012). During September-October, in the east of the Barents Sea, cod distributions reached the coastal sites of Novaya Zemlya (70–74°C) (Matishov et al., 2012). Cod abundance at coastal sites of the Kola Peninsula, including Dalnezelenetskaya Bay, has been shown to increase in warm periods (Karamushko et al., 2014). Higher concentrations of predators in warmer years likely lead to higher red king crab mortality (Kuzmin and Gudimova, 2002). Taking into account cannibalistic behavior in red king crabs and food competition between adult and juvenile crabs (Pavlova, 2008) we can propose a higher impact of large specimens on small crabs in warmer than in colder periods (Dvoretsky and Dvoretsky, 2016). Cannibalism between cohorts could vary seasonally because the major portion of adult crabs migrates at deeper sites in autumn. In periods with high biomass of benthic animals, large king crabs can stay at shallow-water sites and directly or indirectly affect the number of small conspecifics (Kuzmin and Gudimova, 2002). A similar pattern was reported for cod: in warm years, these predators were found in high concentrations at coastal sites over a longer period than in cold or normal years (Karamushko et al., 2014). Vulnerability of small king crabs to predators also depends on season because only recently molted crabs can be consumed by cod and adult crabs (Pavlova, 2008). Usually, mass molting of juveniles at age 3–5 occurs in January-February, but in warmer conditions, they can molt much earlier, in December or even November (Dvoretsky and Dvoretsky, 2012). This pattern can explain negative associations between the stock indices of crabs at age 3–5 and climatic variables in December in warm periods when soft-shell young crabs meet high concentrations of their predators. Similar negative associations between climatic factors and abundance indices were found for mature red king crabs in their native areas. For example, in Gulf of Alaska, a quantitative retrospective analysis has shown negative correlations of P. camtschaticus recruitment with Kodiak air temperature at lags 6–8 and with water temperature at lag 7, and with the Southern Oscillation Index in spring (lags 6–8), and for the annual level at 8–9-year lags (Bechtol and Kruse, 2009). The authors suggested that predation was a likely reason for higher natural mortality of P. camtschaticus (Bechtol and Kruse, 2009).
The high presumed importance of cod predation in red king crab mortality is confirmed by negative correlations between biomasses of these species and positive correlations between cod abundance and averaged weights of juvenile crabs (Figures 4B,C and Supplementary Tables). A similar trend was found during underwater observations on red king crab predation by Pacific fishes at Yankee Cove, Alaska, i.e., small crabs were consumed sooner than large crabs (Daly et al., 2013). The same patterns were registered for the Atlantic cod–snow crab interactions in the Gulf of St. Lawrence, Canada (Chabot et al., 2008).
In conclusion, we suggest that smaller red king crabs (age 0–2) that had lower vulnerability to predators are less affected by climatic factors than older crabs (age 3–5). The associations among abiotic and biotic factors found in our study may be useful for modeling recruitment processes and management of the non-native crab in the coastal Barents Sea.
Data Availability Statement
The raw data supporting the conclusions of this article will be made available by the authors, without undue reservation.
Author Contributions
AD made the data collection and wrote the manuscript. VD made the analysis and reviewed the manuscript. All authors contributed to the article and approved the submitted version.
Funding
This work was supported by the Ministry of Science and Higher Education of the Russian Federation.
Conflict of Interest
The authors declare that the research was conducted in the absence of any commercial or financial relationships that could be construed as a potential conflict of interest.
Acknowledgments
We thank Dr. Anett Trebitz for correcting the English. We thank the reviewers for their thoughtful comments.
Supplementary Material
The Supplementary Material for this article can be found online at: https://www.frontiersin.org/articles/10.3389/fmars.2020.00726/full#supplementary-material
Footnotes
References
Bechtol, W. R., and Kruse, G. H. (2009). Reconstruction of historical abundance and recruitment of red king crab during 1960–2004 around Kodiak, Alaska. Fish. Res. 100, 86–98. doi: 10.1016/j.fishres.2008.09.003
Bizikov, V. A., Goncharov, S. M., and Polyakov, A. V. (2007). The geographical informational system CardMaster. Rybn. Khoz. 1, 96–99. (in Russian)
Chabot, D., Sainte-Marie, B., Briand, K., and Hanson, J. M. (2008). Atlantic cod and snow crab predator–prey size relationship in the Gulf of St. Lawrence, Canada. Mar. Ecol. Prog. Ser. 363, 227–240. doi: 10.3354/meps07384
Daly, B., Eckert, G. L., and White, T. D. (2013). Predation of hatchery-cultured juvenile red king crabs (Paralithodes camtschaticus) in the wild. Can. J. Fish. Aquat. Sci. 70, 358–366. doi: 10.1139/cjfas-2012-0377
Denisenko, S. G. (2007). “The Barents Sea zoobenthos under the conditions of changing climate and anthropogenic impact,” in Dynamics of Marine Ecosystems and Current Preservation Problems of Biological Potential of the Seas of Russia, ed. V. G. Tarasov (Vladivostok: Dalnauka), 418–511. (in Russian).
Dippner, J. W., and Ottersen, G. (2001). Cod and climate variability in the Barents Sea. Clim. Res. 17, 73–82. doi: 10.3354/cr017073
Dvoretsky, A. G., and Dvoretsky, V. G. (2012). Does spine removal affect molting process in the king red crab (Paralithodes camtschaticus) in the Barents Sea? Aquaculture 32, 173–177. doi: 10.1016/j.aquaculture.2011.10.028
Dvoretsky, A. G., and Dvoretsky, V. G. (2013). Population dynamics of the invasive lithodid crab, Paralithodes camtschaticus, in a typical bay of the Barents Sea. ICES J. Mar. Sci. 70, 1255–1262. doi: 10.1093/icesjms/fst037
Dvoretsky, A. G., and Dvoretsky, V. G. (2014). Size-at-age of juvenile red king crab (Paralithodes camtschaticus) in the coastal Barents Sea. Cah. Biol. Mar. 55, 43–48.
Dvoretsky, A. G., and Dvoretsky, V. G. (2015). Commercial fish and shellfish in the Barents Sea: have introduced crab species affected the population trajectories of commercial fish? Rev. Fish Biol. Fisheries 25, 297–322. doi: 10.1007/s11160-015-9382-1
Dvoretsky, A. G., and Dvoretsky, V. G. (2016). Inter-annual dynamics of the Barents Sea red king crab (Paralithodes camtschaticus) stock indices in relation to environmental factors. Polar Sci. 10, 541–552. doi: 10.1016/j.polar.2016.08.002
Dvoretsky, A. G., and Dvoretsky, V. G. (2018). Red king crab (Paralithodes camtschaticus) fisheries in Russian waters: historical review and present status. Rev. Fish Biol. Fisheries 28, 331–353. doi: 10.1007/s11160-017-9510-1
Dvoretsky, A. G., and Dvoretsky, V. G. (in press). Aquaculture of green sea urchin in the Barents Sea: a brief review of Russian studies. Rev. Aquac. doi: 10.1111/raq.12423
Frolova, E. A., Lyubina, O. S., Dikayeva, D. R., Ahmetchina, O. Y., and Frolov, A. A. (2007). Effect of climatic changes on the zoobenthos of the Barents Sea (on the example of several abundant species). Dokl. Biol. Sci. 416, 349–351. doi: 10.1134/s0012496607050079
Glantz, M. (1992). Climate Variability, Climate Change, and Fisheries. Cambridge: Cambridge University Press.
Hurrell, J., Kushnir, Y., Ottersen, G., and Visbeck, M. (eds). (2003). The North Atlantic Oscillation: Climatic Significance and Environmental Impact. Washington, DC: American Geophysical Union.
ICES (2016). Report of the Arctic Fisheries Working Group (AFWG). ICES CM 2016/ACOM:06. Copenhagen: ICES.
Il’in, G. V., Moiseev, D. V., Shirokolobov, D. V., Deryabin, A. A., and Pavlova, L. G. (2016). Long-term dynamics of hydrological conditions of the Zelenetskaya Bay, East Murman. Vestn. MSTU 19, 268–277. (in Russian with English abstract), doi: 10.21443/1560-9278-2016-1/2-268-277
Johnson, C., Inall, M., Gary, S., and Cunningham, S. (2020). Significance of climate indices to benthic conditions across the Northern North Atlantic and adjacent shelf seas. Front. Mar. Sci. 7:2. doi: 10.3389/fmars.2020.00350
Karamushko, O. V., Zhuravleva, N. G., Karamushko, L. I., Kudryavtseva, O. Y., Raskhozheva, E. V., et al. (2014). “Modern research of the Barents Sea and adjacent waters ichthyofauna,” in Marine Ecosystems and Communities in the Conditions of Current Climate Change, ed. G. G. Matishov (Saint Petersburg: Renome), 223–243. (in Russian).
Kuzmin, S. A., and Gudimova, E. N. (2002). Introduction of the Kamchatka (red king) crab in the Barents Sea. Pecularities of biology, perspectives of fishery. Apatity: KSC RAS Press. (in Russian).
Leps, J., and Smilauer, P. (2003). Multivariate Analysis of Ecological Data Using CANOCO. Cambridge: Cambridge University Press.
Matishov, G., Moiseev, D., Lyubina, O., Zhichkin, A., Dzhenyuk, S., Karamushko, O., et al. (2012). Climate and cyclic hydrobiological changes of the Barents Sea from the twentieth to twenty-first centuries. Polar Biol. 35, 1773–1790. doi: 10.1007/s00300-012-1237-9
McDonald, J. H. (2009). Handbook of Biological Statistics, Vol. 2. Baltimore: Sparky House Publishing.
Orensanz, J. I. M., Armstrong, J., Armstrong, D., and Hilborn, K. (1998). Crustacean resources are vulnerable to serial depletion – the multifaceted decline of crab and shrimp fisheries in the Greater Gulf of Alaska. Rev. Fish Biol. Fisheries 8, 117–176.
Pavlova, L. V. (2008). “Red king crab trophic relations and its influence on bottom biocenoses,” in Biology and Physiology of the Red King Crab from the Coastal Zone of the Barents Sea, ed. G. G. Matishov (Apatity: KSC RAS Press), 77–104. (in Russian).
Pereladov, M. V. (2003). Some aspects of distribution and behavior of red king crab (Paralithodes camtschaticus), on the Barents Sea shallow coastal water. Tr. VNIRO 142, 103–119. (in Russian),Google Scholar
Sakshaug, E., Johnsen, G., and Kovacs, K. (eds) (2009). Ecosystem Barents Sea. Trondheim: Tapir Academic Press.
Sanchez-Rubio, G., Perry, H. M., Biesiot, P. M., Johnson, D. R., and Lipcius, R. N. (2011). Climate-related hydrological regimes and their effects on abundance of juvenile blue crabs (Callinectes sapidus) in the northcentral Gulf of Mexico. Fish. Bull. 396, 139–146.
Sokolov, V. I., and Milyutin, D. M. (2006). Distribution, size-sexcomposition, and reserves of the red king crab (Paralithodes camtschaticus) in the upper sublittoral of the Kola Peninsula (the Barents Sea). Zool. Zhurn. 85, 158–171. (in Russian with English abstract),
Sokolov, V. I., and Milyutin, D. M. (2007). Changes in population and distribution of the red king crab in the coastal area of the Barents Sea. Tr. VNIRO 147, 158–172. (in Russian with English abstract),Google Scholar
Stevens, B. G. (2014a). “Biology and ecology of juvenile king crabs,” in King Crabs of the World: Biology and Fisheries Management, ed. B. G. Stevens (Boca Raton: CRC Press), 261–401. doi: 10.1201/b16664-10
Stevens, B. G. (2014b). “Development and biology of king crab larvae,” in King Crabs of the World: Biology and Fisheries Management, ed. B. G. Stevens (Boca Raton, FL: CRC Press), 233–260. doi: 10.1201/b16664-9
Stolyarenko, D. A. (1986). Data analysis of trawl shrimp survey with spline approximation of stock density. ICES C.M. Doc. K:25, 16.
Keywords: Paralithodes camtschaticus, juveniles, Barents Sea, stock dynamics, climatic indices
Citation: Dvoretsky AG and Dvoretsky VG (2020) Effects of Environmental Factors on the Abundance, Biomass, and Individual Weight of Juvenile Red King Crabs in the Barents Sea. Front. Mar. Sci. 7:726. doi: 10.3389/fmars.2020.00726
Received: 20 March 2020; Accepted: 11 August 2020;
Published: 01 September 2020.
Edited by:
Morten Omholt Alver, Norwegian University of Science and Technology, NorwayReviewed by:
Torstein Pedersen, The Arctic University of Norway, NorwayHarald Gjøsaeter, Norwegian Institute of Marine Research (IMR), Norway
Copyright © 2020 Dvoretsky and Dvoretsky. This is an open-access article distributed under the terms of the Creative Commons Attribution License (CC BY). The use, distribution or reproduction in other forums is permitted, provided the original author(s) and the copyright owner(s) are credited and that the original publication in this journal is cited, in accordance with accepted academic practice. No use, distribution or reproduction is permitted which does not comply with these terms.
*Correspondence: Alexander G. Dvoretsky, ag-dvoretsky@yandex.ru