- Department of Molecular Biology and Genetics, Canakkale Onsekiz Mart University, Canakkale, Türkiye
Bovine colostrum (BC) is the initial milk an animal produces after giving birth, particularly in the first few days. Numerous bioactive substances found in BC, including proteins, enzymes, growth factors, immunoglobulins, etc., are beneficial to human health. BC has a significant role to play as part of a healthy diet, with well-documented health and nutritional advantages for people. Therefore, the use of BC and its crucial derivatives in the development of functional food and pharmaceuticals for the prevention of several diseases such as gastrointestinal and respiratory system disorders is becoming increasingly popular around the world. A novel coronavirus severe acute respiratory syndrome coronavirus 2 (SARS-CoV-2) was identified as the cause of a cluster of pneumonia cases that is called Coronavirus Disease 2019 (COVID-19) in China. After the first SARS-CoV-2 virus-related fatality was announced, the illness quickly spread throughout China and to other continents, causing a pandemic. Since then, numerous studies have been initiated to develop safe and efficient treatments. To prevent viral infection and potential lingering effects, it is important to investigate alternative treatments for COVID-19. Due to its effective bioactive profile and its immunomodulatory roles in biological processes, BC might be considered a promising approach to assist in combating people affected by the SARS-CoV-2 or prevention from the virus. BC has immunomodulatory effects because to its high concentration of bioactive components such as immunoglobulins, lactoferrin, cytokines, and growth factors, etc., which might help control immunological responses, potentially fostering a balanced immune response. Furthermore, its bioactive components have a potential cross-reactivity against SARS-CoV-2, aiding in virus neutralization and its comprehensive food profile also supplies important vitamins, minerals, and amino acids, fostering a healthy immune system. Hence, the possible contributions of BC to the management of COVID-19 were reviewed in this article based on the most recent research on the subject. Additionally, the key BC components that influence immune system modulation were evaluated. These components may serve as potential mediators or therapeutic advantages in COVID-19.
1 Introduction
Bovine colostrum (BC) is the initial secreted milk from bovine mammary glands during the first few days after calving (1). It contains several bioactive nutrients and immunological compounds that are necessary for the newborn’s nourishment as well as growth and development (2). Colostrum is incredibly rich in bioactive substances such immunoglobulins (IgG, IgA, IgE, IgD, IgM), growth factors, hormones, lysozymes, lactoferrin (Lf), and lactoperoxidase (LPO). Additionally, it has higher levels of lipids, proteins, minerals (phosphates, citrates, etc.), and vitamins (water and fat-soluble) (Table 1) (1, 15, 16). With the exception of lactose content in BC, the concentration of these components is highest in the first few days after postpartum, thereafter rapidly decreasing over the next three days (17).
Both internal and external factors have a considerable impact on the composition and quality of BC (15, 18). Major contributing factors including environment, individuality, season, humidity, breed, dry period, pre-partum nutrition, calving, etc., changes the BC composition and quality. Diseases such as mastitis are other factors that change the BC content and quality (19–24).
Nutritionally and biologically important substances are found in BC at different concentrations and each active substance has a distinct role based on their biological forms as well as types of action shown in Figure 1. Considering the general content of BC, the main nourishing part allows providing energy to the newborn calves, while the biologically active components such as immunoglobulins (Igs), Lf, growth factors, etc., support the immune system and provide passive immunity to protect from several infections from pathogens, viruses, etc. (Table 1) (15, 27).
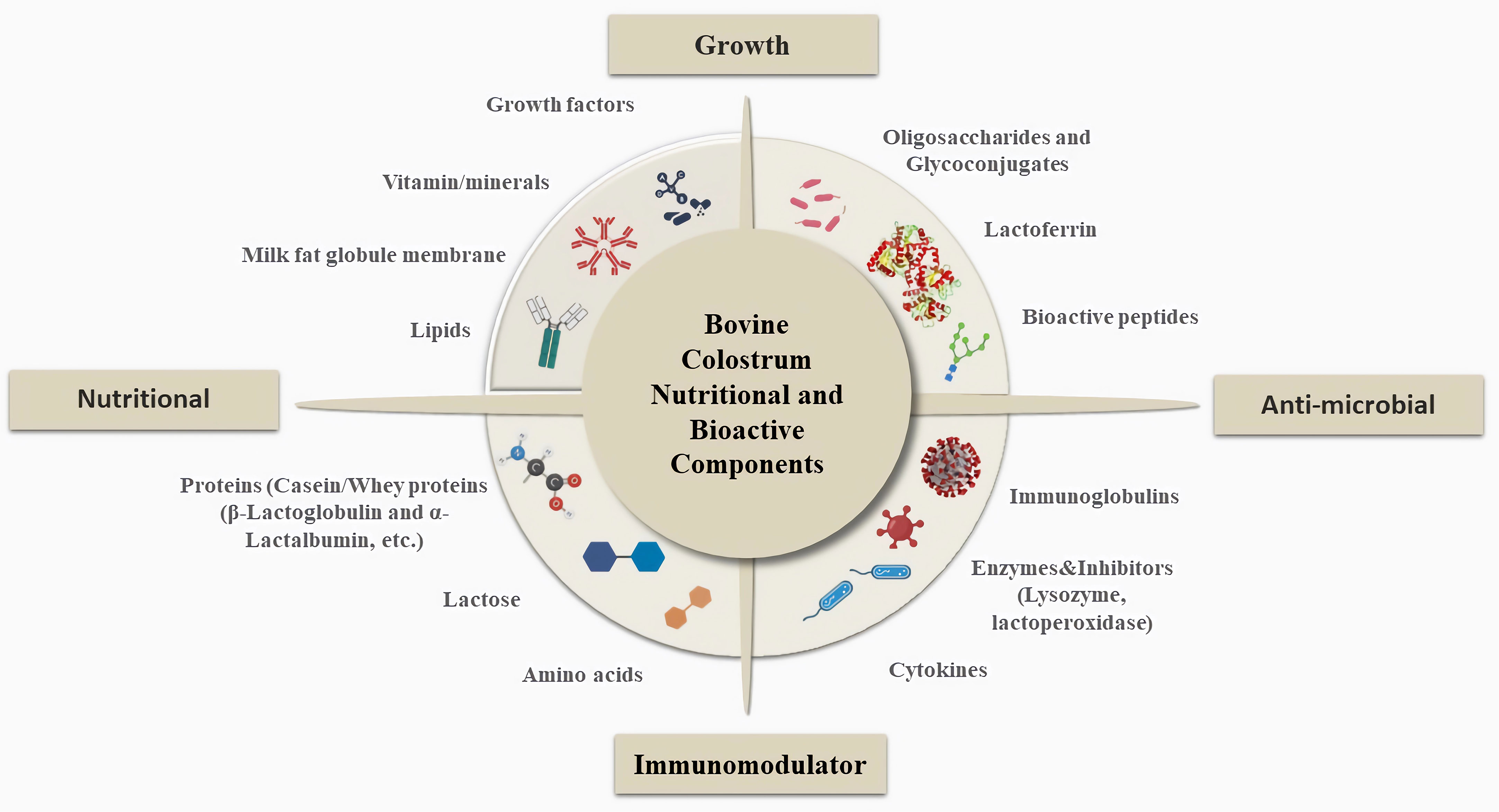
Figure 1 General Overview of Bovine Colostrum Composition and Important Roles. BC contains a variety of nutritionally and physiologically significant compounds and each active substance serves a specific purpose. Considering the general content of BC, the main nourishing part, such as lipids, vitamins-minerals, growth factors, proteins, and lactose, provides energy to newborn calves and supports calf growth, while the biologically active components, such as Igs, Lf, growth factors, etc., support the immune system and provide passive immunity to protect against several infections from pathogens, viruses, etc. In addition to these components, oligosaccharides and glycoconjugates, bioactive proteins, and peptides support calf development and also have antimicrobial properties (25, 26).
A novel coronavirus “severe acute respiratory syndrome coronavirus 2 (SARS-CoV-2)” was identified as the cause of a cluster of pneumonia cases that is called “Coronavirus Disease 2019 (COVID-19) in Wuhan City, China, in late 2019. Within a few months, the disease spread across China and to various continents, causing a global pandemic (28). Since then, several research has been started to perform to find effective and safe treatments. Clinically, manifestations of COVID-19 show a wide heterogeneity, ranging from asymptomatic cases to mild symptoms, including fever, dry cough, headache, myalgia, dyspnea, and, in some cases, diarrhea, nausea, and vomiting. The gradual progression of this disease may lead to difficulty breathing, respiratory distress, pneumonia, cardiac, and neurological damage, and in severe cases, even multiple organ failure and death. It’s worth noting that the severity and duration of COVID-19 repercussions can vary greatly amongst people. While many people recover with no long-term complications, others may face serious health concerns. Additionally, the virus’s influence goes beyond health, influencing society in the form of economic downturns, disruptions in education, mental health issues, and changes in social behavior as a result of public health initiatives (29, 30). The treatment and prevention of the SARS-CoV-2 illness are still being researched, even though there are currently a number of vaccinations available. Additionally, due to the constant evolution of the virus, new viral strains may not be effectively recognized by vaccine-induced protection (31).
To sum up, prior to the development of the vaccine, it is urgently necessary to investigate alternative treatments for COVID-19 clinically advanced conditions without causing side effects in order to decrease viral infection, replication, and spread as well as mortality and to mitigate potential future outbreaks. Due to its effective bioactive profile and its immunomodulatory roles in biological processes, BC can be considered as a possible strategy to assist in treating people affected by SARS-CoV-2 (32).
Based on the most recent research on the topic, the potential contributions of BC to the clinical care of COVID-19 were discussed in this article. Additionally, the primary colostrum components that affect immune system modulation and may function as mediators of possible therapeutic benefits in COVID-19 disease were reviewed.
2 The main immunomodulating and bioactive components of bovine colostrum
2.1 Caseins
The biological characteristics of milk proteins have drawn more attention from scientists and industries such as the food and pharmaceuticals industry (33, 34). Colostrum has a significantly higher protein content than mature milk, mostly because of the higher concentrations of Igs and casein. Whey proteins and caseins, which are two types of milk proteins, can be distinguished based on their solubility in milk. A total of 150 g/L of protein are present in BC, of which 124 g/L are whey protein and 26 g/L are casein In comparison to casein content of milk, colostrum has a higher casein concentration and gets lower with each postpartum milking (34).
Caseins are proteins that control inflammation, and the immune system as well as they have antibacterial activity. As mineral binders’ proteins, they enhance digestion by producing a clot in the stomach, which shortens the time it takes for nutrients to enter the bloodstream and improves digestion (35). The various bioactive peptides derived from casein protein and its sub-forms (α, β, and κ) impart significant physiological properties to them. For example, the therapeutic peptide “RYLGY” was identified by Dash and Jaganmohan (2022) from cold plasma-treated S1-CN of bovine milk. This peptide may interfere with the SARS-CoV-2 spike protein’s ability to bind to the angiotensin-converting enzyme 2 (ACE2) receptors on cell membranes, preventing the virus from entering cells. In-silico docking studies showed that the peptide and ACE2-receptor binding domain complex had increased binding affinity and electrostatic interactions (36).
On the other hand, glycomacropeptides (GMP) which is also known as caseinomacropeptide, is a type of C-terminal glycopeptides isolated from κ-casein. The biological functions of GMP have attracted more attention in recent decades (10). Numerous in-vitro studies have demonstrated that GMP inhibits the adhesion of cariogenic Str. mutans and Str. sobrinus bacteria as well as influenza virus and counteracts the microbial toxins of Escherichia coli (E. coli) and Vibrio cholerae. Furthermore, GMP modifies immunological responses, promotes the growth of beneficial microorganisms such as bifidobacteria, suppresses gastric hormone activities as well as controls blood flow through hypertension and antithrombotic activity (37, 38). The usage of GMP can be a possible strategy to improve the treatment against COVID-19. These results imply that casein and its derivatives may be particularly effective in combating COVID-19, but additional studies are required to properly understand the influence of casein and its peptides as effective targets for COVID-19 (3, 39).
2.2 Lactoferrin (Lf)
Lf is a highly glycosylated whey protein found in bovine milk, with considerably high quantities in BC (Table 1). It has the potential to bind to iron and interact with several pathogens, making it the first line of defense in the mucous membranes of the body (40, 41). It has antibacterial, antiviral, antifungal, antiparasitic, and anti-inflammatory properties, as well as being implicated in innate and adaptive immune modulation (42, 43). Lf has antiviral activity against DNA and RNA viruses (44, 45), and the exact mechanism of its activity against bacterial and viral infections has been highlighted for the prevention of diseases (46).
The underlying mechanism of the antiviral role of Lf in BC is the inhibition of viral entry by attaching to the cell surface and/or viral molecules (47). Up to date, various studies and clinical trials regarding the antiviral activity of Lf derived from milk against different viruses including SARS (48), type I and II herpes simplex virus (HSV) (49, 50), human immunodeficiency virus (HIV) (51), rotavirus, influenza virus, parainfluenza virus, cytomegalovirus, and enterovirus (52–55).
The potential role of BC for the clinical treatment of COVID-19 is based on its an-inflammatory, antibacterial, and antiviral properties as well as on its ability to boost the human innate and adaptive immune systems. Considering the literature, clinical studies suggested that bioactive components of BC, particularly Lf, have considerable antiviral activity, which could assist to delay the progression of COVID-19 (56, 57).
Numerous clinical trials are being conducted to examine the anti-SARS-CoV-2 activity of Lf in-vivo in light of the findings from in-vitro and in-silico research. Lf may be used alone or in conjunction with other medications to treat COVID-19 disease (32). Lf from BC was found to be effective against SARS-CoV-2 viruses, similar to its effectiveness against Zika and Chikungunya viruses (56). In an in-vivo preliminary study, the antiviral effect of liposomal bovine Lf (LLf) was investigated for three months. For this investigation, mild-to-moderate and asymptomatic COVID-19 patients were chosen, and they were classified three main groups including patients (hospitalized and home isolation) who had oral and intranasal LLf; a hospitalized patient who recruited with standard protocol; and remaining patients in home isolation without any medical treatments. Control groups were also specified for the exact analysis. According to study results, LLf-treated patients recovered as compared to other groups. It was concluded that there was a decrease in serum ferritin, interleukin 6 (IL-6), and D-dimer levels. Given the high Lf content of BC, the use of this bioactive component for the effective treatment of COVID-19 and related infections may be a promising approach (58).
In addition to the antiviral activity of Lf, the anti-inflammatory feature of Lf could be effective in moderating the cytokine storm activation seen in severe COVID-19, which causes several health problems such as pulmonary edema and failure, hepatic, cardiac, and renal damage (59). In prospective observational research by Serrano et al. (2020), the impact of LLf supplementation was tested in 75 COVID-19 patients over 10 days. Based on the study, LLf (32 mg of Lf/10 mL together with 12 mg of vitamin C) was given to the patient. 10 mg/mL of zinc solution was also administered twice or three times per day. On the other hand, 12 patients were given only LLf as a control group, while 256 people who had contact with COVID-19 patients were also administered half of the treated dosage. Within the first 4-5 days of treatment, all patients recovered completely and quickly. The reduction of incidence of disease symptoms such as dry cough, headache, fatigue, anosmia, and ageusia was observed. They concluded LLf carries the potential for the prevention and treatment of COVID-19 (44).
The impact of Lf treatment on immune response and infection of SARS-CoV-2 was also investigated in-vitro study. Researchers used real-time quantitative reverse transcription PCR (qRT-PCR) to examine the expression of antiviral immune responses in infected and uninfected Caco-2 intestinal cell lines that had received Lf. As a result of the assessment, the expression of IFNA1, IFNB1, TLR3, TLR7, IRF3, IRF7, and MAVS genes was induced in Lf-treated Caco-2 cells. The virus infection and replication were also inhibited, partially. It was concluded that Lf showed great potential as a an immunomodulator against the infection of SARS-CoV-2 (60). In another in-vitro study from Ward et al. (2022), the human lung cell line H1437 was used to assess the anti-SARS-CoV-2 effectiveness of commercially available bovine Lf and common dairy components. It was shown that bovine Lf demonstrates a broad spectrum of antiviral efficacy in-vitro against SARS-CoV-2 variants, including the South African B.1.351, UK B.1.1.7, Brazilian P.1, and Indian Delta variants (61).
The role of Lf as an iron-binding protein has also been studied. The impact of iron on COVID-19 inflammation was discussed by Dalamaga et al. (2020), as well as the possible benefit of iron chelators in lowering SARS-CoV-2 inflammation brought on by iron overload (62).
When considered collectively, all these characteristics allow us to view Lf as a potentially useful tool capable of addressing multiple facets of the viral development and pathogenesis of COVID-19.
2.3 Immunoglobulins (Igs)
Igs, as the foremost protein part of BC, acting essential roles in transferring and supporting passive immunity in the newborn calves (63). The biological and immunological effect of BC Igs on human health has been extensively researched and is still being studied (64). Based on the properties such as size and charge of the molecule, amino acid, and also carbohydrate content Igs are classified as IgG, IgA, IgE, IgD, and IgM (19, 65, 66). The most abundant antibody present in BC is IgG and the IgG1 and IgG2 are the two subclasses of IgG which account for 80-85% of the total immunoglobulin content of BC (19, 65–67). In comparison to mature milk, the Igs, the main group of immunological components found in BC, are approximately 100-fold greater (68). Based on the reports from several researchers, the higher concentrations of IgG present in BC demonstrate a variety of immuno-protective and immuno-modulatory features (19, 65, 66, 69).
Generally, Igs are widely recognized molecules for immunogenic response-related health benefits in human diseases. The basic mechanism of the immunogenic response of Igs is when foreign molecules (antigens) enter the host organisms, the Igs bind and eliminate these antigens such as bacteria, viruses, toxins, etc. When the host is exposed to the same antigens, they promote the formation of antibodies to eradicate the illness from the body (70, 71). There are a lot of research using this antiviral mechanism in the literature (72).
In the 1970s, Ellens et al. made the initial discovery that normal cow’s milk contains bovine IgG1 antibodies against rotavirus (73). Then, a number of studies on the binding of bovine IgG to numerous human bacterial pathogens and viruses, such as Helicobacter Campylobacter jejuni (C. jejuni), Cryptosporidium, Klebsiella pneumoniae, Salmonella typhimurium, Streptococcus, and Rotavirus (74–76). Bovine Igs are also effective against respiratory pathogens like the human Respiratory Syncytial Virus (RSV), the influenza virus, and Streptococcus pneumoniae in addition to detecting other types of pathogens (77).
Numerous alternative approaches have been employed to combat the viruses and also SARS-CoV-2. Hyperimmune BC (HBC), produced by immunizing cows during gestation, contains a high amount of targeted Igs that can be used for the treatment of several infections. The usage of HBC against viruses is well-documented in the literature. Purcell et. al., 2012 developed the vaccination and production system to produce large-scale neutralizing HIV-1 IgG from recombinant HIV-1 gp140 oligomers vaccinated cows. It was resulted that HBC and also IgG derived from BC exhibited neutralizing properties against the variety of Env-pseudo typed viruses (78). NG et. al., 2010 showed the hemagglutination-inhibitory activity of IgG and F(ab’)2 derived from the HBC of influenza-vaccinated cows (79).
Therefore, the same strategy was employed against COVID-19 infection taking into account prior research on the preventative and therapeutic effects of BC-derived Igs on other pathogenic pathogens (79–83). In COVID-19 patients, the immune system is so important, and BC which has a considerable amount of Igs is crucial for boosting the immune system (84). The published studies regarding the usage of HBC suggest that it is a potential adjuvant for COVID-19 patients because of its strong safety profile (85).
HBC, which has been shown to be effective against different viral infections, was produced for immunization with inactivated SARS-CoV-2 and tested in clinical trials. After measurement of the IgG level found in sera against the virus and the level of neutralizing antibodies in the colostrum and sera using ELISA tests, the product’s safety was assessed in 40 healthy volunteers between the ages of 18 and 65. The BC samples had a titer of the neutralizing antibody that was 69 times higher as compared to sera samples. According to the phase I clinical trial results, there wasn’t any adverse effect or complications in participants and phase II studies are ongoing. HBC was preferred as a treatment strategy of COVID-19 for Nili et. al., 2021 (85).
Considering the activity of BC-derived neutralizing antibodies (NAbs) against the SARS-CoV-2 virus, NAbs have a mechanism that impedes the interaction between the ACE2 and S protein SARS-CoV-2 by blocking the cell entry from viruses. By using this great mechanism, Kangro et. al., 2022 produced NAbs from immunized pregnant cows for the effective blocking of SARS-CoV-2 infection. They also demonstrate the BC-antibody-containing nasal spray for COVID-19 patients. To do so, they concluded the antibodies against the SARS-CoV-2 viruses produced from the BC exhibit significant promise as a preventative medicine and BC Igs have a highly effective neutralizing effect in the in-vitro assay (86). To assess HBC’s capacity to stimulate antiviral interferonγ (IFNγ) T cell responses, Ilan et. al., 2021 performed preclinical and clinical studies. In this research, orally administered HBC during the 5 five days resulted in augmentation of the number of antiviral T cells responses to antigens in healthy participants. Thus, it was suggested that the application of HBC shows great potential for the prevention of COVID-19 (87).
2.4 Lactoperoxidase (LPO)
LPO is one of the peroxidase enzymes and it is considered an important antibacterial enzyme that is present in BC. The concentration of LPO in BC is 0.02 g/L, whereas in mature milk, it is 0.03 g/L. Based on the researchers’ reports, the LPO level in BC reaches the highest level after 3-5 days post-partum, compared with the initial concentration in BC (88). Thus, BC has higher catalyzed activity as compared to mature milk. LPO as a crucial antibacterial enzyme catalyzes thiocyanate oxidation and generates intermediate molecules that have antimicrobial activity (89).
Considering its LPO activity, it generates oxidation products that are toxic for some types of bacterial species including Streptococcus mutans, Salmonella typhimurium, Staphylococcus aureus (S. aureus), Pseudomonas aeruginosa, and Listeria monocytogenes. Furthermore, LPO also has a great mechanism that inactivates certain viral species such as HIV, poliovirus, vaccinia virus, etc. (9, 90–92). Of interest, in-vitro research on cell models showed hypothiocyanite (OSCN−) to be effective at preventing SARS-CoV-2 infection at a micromolar level (93). In another study, Shin et al. (2005) discovered that giving Lf and LPO from bovine milk to BALB/c mice infected with the influenza virus strain reduced inflammatory cell infiltration, suppressed pneumonia, and significantly lowered lung consolidation scores. Furthermore, on Day 6, BLPO dramatically reduced the amount of serum proinflammatory cytokine (IL-6) in mice compared to controls (94). A similar study found that people who did not regularly gargle or wear a face mask but consumed Lf and LPO orally experienced cold and fever symptoms less frequently and for a shorter duration (95). All of these research findings clearly indicate that LPO may also be effective in the treatment of COVID-19.
2.5 β-Lactoglobulin (β-Lg) and α-Lactalbumin (α-La)
β-Lactoglobulin (β-Lg) is one of the whey proteins and it makes up around 50% of these proteins (10). By preventing bacterial adherence to the host surface and hindering pathogen colonization, this protein shows antimicrobial properties (96, 97). Its antimicrobial activity is exhibited against several gram-positive and negative bacteria such as Bacillus subtilis (B. subtilis), S. aureus, E. coli, Bordetella bronchiseptica (97). Furthermore, β-Lg that has been chemically treated with 3-hydroxyphthalic anhydride may prevent Chlamydia trachomatis infection, and both HSV-1 and HSV-2 are susceptible to the 3-HP-lactoglobulin (98).
Peptides derived from α-La serve various biological and physiological functions such as antimicrobial, antiviral, antioxidative, and antihypertensive roles, as well as modulation of the immune system (99, 100). For example, peptides from α-La are mostly active against different microorganisms such as Staphylococcus epidermidis ATCC 12228, Staphylococcus lentus, and B. subtilis BGA (101).
Overall, these features of β-Lg and α-La suggest that it can be considered as the potential bioactive compound in BC for the treatment of COVID-19.
2.6 Lysozyme
A crucial component of the innate immune system, lysozyme has potent antibacterial properties against bacterial, fungal, and viral infections. It boosts the effectiveness of other antibiotics, guards against infections, functions as a natural antibiotic, and fortifies the immune system (102). The concentration of lysozyme differs between the species and BC has 0.14-0.7 mg/L of lysozyme, while mature milk contains 0.07-0.6 mg/L of lysozyme. Age, health, the animals’ parity, and the lactation period are a few of the variables that affect lysozyme concentration in BC (10). There has been a lot of research on lysozymes and their antimicrobial mechanism in literature. By catalyzing the breakdown of the β-1,4 bonds in peptidoglycan in bacterial cell walls, lysozyme exhibits its antibacterial effect (103). Peptidoglycan defines cellular form and provides effective defense against cellular turgor pressure, whereas lysozyme activity results in the loss of its integrity, bacterial cell lysis, and ultimately cell death (104). In addition, rather of acting primarily through its enzymatic function, lysozyme can prevent the growth of viruses and fungus like Aspergillus niger and Penicillium that are connected to its charge (105).
Regarding the antiviral activity of lysozyme, this bioactive enzyme has been shown to be effective against herpes simplex and herpes zoster (106) and to prevent replication of HIV-1 viruses (107), by assembling compounds containing viral DNA. There are notable findings that treatment with lysozyme aerosol is helpful in lowering inflammation and lung tissue damage in animal models of pneumonia and emphysema in addition to its antiviral effect (108). Additionally, lysozyme has neuroprotective properties that may aid to counteract the neurological effects of COVID-19 (109). In the present coronavirus pandemic, a modified form of lysozyme can be utilized to boost the production of interferon, a powerful anti-coronavirus chemical, and so reduce the danger of the life-threatening type of COVID-19 by up to 79% (110, 111). So, it can be a useful component to develop new strategies for the treatment of COVID-19 (112).
2.7 Growth factors
Growth factors are considered signaling proteins that are secreted by various cell and tissue types including neighboring cells, glands, also cancer cells. They can promote cell growth, differentiation, survival, and inflammation, and they are typically regarded as a subgroup of cytokines. For proliferation and viability, growth factors are required for all cell types (113, 114). These bioactive components were first documented in 1997 by Pakkanen and Aalto, and then by Gauther et al. in 2006 (9, 115). Betacellulin GF (BTC), Epidermal GF (EGF), FGF-1, Fibroblast GF (FGF-1, and 2), Insulin-like GF (IGF-1 and 2), Transforming GF (TGF-β1, TGF-β2), and Platelet-derived GF (PDGF) are reported growth factor types that are present in milk and colostrum. EGF, IGF, and TGF growth factors are the most prominent types among the others ones (9).
The transforming growth factor-β (TGF-β) is a type of pleiotropic cytokine that is produced by various cell types such as immune cells and non-hematopoietic cells etc. In the cellular process, it participates in regulating immune responses such as inducing or suppressing immune responses, cell proliferation, and oncogenesis (116–118). TGF-β contributes to the induction of immunological tolerance by suppressing inflammatory responses to luminal bacterial antigens in the intestine (116). Another important activity of TGF-β is the modulation of airway inflammation and effector T helper 2 cell-induced hyper-reactivity. This protein suppresses the airway inflammation that performs a critical regulatory role in asthma (119).
Accumulating evidence demonstrates that this immunomodulatory protein supports the immune development of children and protects them against different types of allergies and possible inflammation (120). Because of such properties, they may be a possible strategy for the prevention of COVID-19 and other types of diseases.
2.8 Oligosaccharides (OS)
OS isolated from bovine milk and colostrum are other types of bioactive components that are gaining commercial interest due to their potential health benefits (121). Highly selective and complex OS are found in BC, and up to date, approximately 50 distinctive OS have been analyzed in this content. Emerging studies on OS have revealed significant differences in the composition profile and relative abundance between cows, and these bioactive components were found to be present in BC at a concentration of 0.7-1.2 mg/mL. Therefore, BC is considered a preferred source of OS as compared to mature milk because of its elevated level of OS and also ease of isolation and identification (122–126). Most of these structures are acidic oligosaccharides that are present in mature bovine milk at lower levels (127).
Bioactive OS from bovine milk and colostrum are similar to human milk OS due to their chemical structures and they have great importance because of their significant roles in biological and physiological mechanisms such as prebiotic activity, protection against various pathogens, etc. (127–133). Their main function appears to be serving as competitive inhibitors for the binding sites on the intestine epithelial surfaces to protect against infections (134). Additionally, there is evidence to support the idea that some of these bioactive components act as colonic microflora genera growth promoters. According to Karav et al. (2016), BMO stimulates Bifidobacterium longum subspecies infantis (B. infantis) development in the infant’s intestine similar to HMO. Complex and hybrid-type glycans from bovine milk and colostrum show prebiotic activity, similar to HMOs/BMOs, selectively utilized by beneficial microorganisms. Promoting brain development, and alleviating metabolic issues are other health benefits of BMO (135).
Considering the biological activity of oligosaccharides, they found in milk and colostrum can compete with pathogenic bacteria and viruses for attachment sites because they are soluble receptor mimics of the carbohydrates found on the surface of epithelial cells. For instance, HMOs were shown by Andersson et al. (1986) to prevent pneumococci or influenza virus from adhering to pharyngeal or buccal epithelial cells (136). Similarly to this, it has been demonstrated that sialylated oligosaccharides prevent pathogenic E. coli strains from adhering to infants (137). In similar studies, OS has been demonstrated to function as receptor analogs for cell surface locations in the digestive tract epithelium, inhibiting the adherence of viruses (138, 139) and bacteria like Helicobacter pylori (140).
In addition, researchers have considered a possible source of anti-infective glycans in BC, which is high in neutral and acidic oligosaccharides. Potential anti-infective glycans in naturally obtained foods were looked into. Anti-infective effectiveness against a highly invasive strain of C. jejuni was investigated for oligosaccharides extracted and purified from the colostrum of Holstein Friesian cows. Because mucins found in BC are highly glycosylated molecules, this discovery would imply that glycan-based substances may function as anti-infectives for C. jejuni. In this context, 37 BC oligosaccharides (BCO) have been structurally characterized by Hydrophilic Interaction Liquid Chromatography-High-Performance Liquid Chromatography (HILIC-HPLC) in conjunction with exoglycosidase digestions and offline mass spectrometry, and it has been shown that C. jejuni can bind to some of these constructions in-vitro. They period-treated the BCO concurrently with the inhibitory test, suggesting a direct bacterial-oligosaccharide interaction that reduced the anti-infective effectiveness of the glycans. This was proven when the BCO entirely eliminated C. jejuni’s ability to bind to chicken intestinal mucus in-vitro. This study provides strong evidence for the anti-infective property of oligosaccharides obtained from BC (141).
Thus, OS may have several uses in the culinary and pharmaceutical industries due to their bioactive properties. In order to comprehend the connection between OS structure and biological function, it is crucial to analyze their structure (128). These qualities make them potentially useful approaches for the SARS-CoV-2 prevention.
2.9 Glycosaminoglycans
Glycosaminoglycans (GAGs), commonly referred to as mucopolysaccharides, are negatively charged, sulfated linear polysaccharide molecules found in milk. Examples include heparin/heparan sulfate (HP/HS), hyaluronic acid (HA), chondroitin sulfate (CS), and dermatan sulfate (DS). In the mammary glands, a particular core protein is connected to the lengthy chains of milk GAGs during production (142).
Milk GAGs may play two crucial roles in the context of COVID-19. These GAGs are used as prebiotics by microorganisms in the intestinal system to promote growth (143). This helps preserve gut health by preventing various enteric bacterial and viral illnesses. Second, although some viruses have been shown to be resistant to the antiviral effects of commercially available GAGs, such as the dengue virus (144), HSV (145), Zika virus (146), and SARS-CoV (147), commercial CS has not been proven effective against all viruses. It is essential to fully describe the antiviral potential of GAGs in bovine milk. Additionally, the fact that several GAGs share similar carbohydrate structures may account for their viral inhibitory effect against various viruses. According to reports by Kwon et al. (2020), free HS prevents Vero cells from becoming infected with SARS-CoV-2. GAGs may have antiviral activity against SARS-CoV-2, the infectious agent responsible for the COVID-19 pandemic (148). Future research may be interesting to further examine and assess the potential of BC and milk GAGs to inhibit this deadly virus.
2.10 Milk fat globule membrane (MFGM)
Mammary epithelial cells release milk fat globules, which are encased in a complex membrane that is called the milk fat globule membrane (MFGM). Polar lipids such as phospholipids and sphingolipids, membrane proteins including glycoproteins, and also enzymes are some of the several substances that make up the thin trilayer structure of MFGM (149–151). The isolation, processing, and analysis methods used as well as the breed of the cow affect the protein and lipid contents of MFGM (12).
Recent research has suggested that MFGM proteins have nutritional and practical benefits for people such as supporting the composition of healthy gut microbiota, protection against infection and inflammation, etc. (152–154). Considering the MFGM proteomes of BC and its importance for the well-being of neonatal calves, it is a rich source of bioactive proteins. Further characterization of these bioactive proteomes can provide important insights into the health benefits of human well-being. Given that several proteins in the bovine MFGM have been demonstrated to have inhibitory effects on a variety of pathogens consuming whey protein concentrate enriched with MFGM may help prevent diarrhea that is both bacterial and viral in origin (155).
Information on the presence of MFGM derived from BC and milk demonstrated that mucoprotein 1 (MUC1), butyrophilin, and lactadherin are a potential bioactive fraction of MFGM. For instance, it was hypothesized that lactadherin might be responsible for the function of the mucin complex based on research with virus-binding properties (156). Another research team carried out in-vitro tests to look for rotavirus-inhibitory elements in bovine milk. One component of bovine whey protein with a high molecular weight showed antiviral activity. Lactadherin, MUC1, and an unknown 80-kDa protein were all present in this fraction. Xanthine oxidase (XO), one of the main MFGM enzyme proteins, has been demonstrated to have antibacterial and immunoprotective effects (157, 158). Butyrophilin, the most prevalent MFGM protein, is known to have immune-modulating properties such as anti-infective or anti-inflammatory actions (159, 160).
Morever, the biological roles of lipid fractions in MFGM have been extensively studied. This bioactivity includes preventing pathogenic and viral infections, reducing cholesterol-induced steatosis, and maintaining gut health (161). It has been shown that MFGM isolates boost resistance to rotaviral infection. In a study looking at MFGM produced from both buttermilk and whey cream, rotaviral infectivity was assessed using a fluorescence focus test. MFGM was shown to exhibit anti-rotaviral effects in all fractions in a dose-dependent manner, while the isolate from cream was marginally more potent (162). This component most likely has a more varied lipid content, which might work in concert with the related viral proteins. In an in-vitro investigation where bovine and ovine MFGM sources were investigated, it was further shown the importance of the lipid fraction in MFGM for preventing rotaviral infection. The cream-derived components in this investigation were discovered to have a rotaviral-neutralizing effect. Nevertheless, heat treatment (denatured proteins) and cream washing (reduced lipid content) both result in reduced effectiveness (163).
As a result of the findings of the possible functional and physiological properties of this component, MFGM can be exploited against viruses in the pharmaceutical industry.
2.11 Vitamin and minerals
Vitamins and minerals are two essential components crucial for a healthy metabolism, growth, and development. They serve as coenzymes throughout the body, and because they are naturally balanced and delivered in colostrum according to the body’s needs, they play a vital role in maintaining health. Specifically, BC contains both fat-soluble vitamins (A, D, and E) and water-soluble vitamins (B series and C), which could have significance for various metabolic functions, such as supporting bone development and acting as antioxidants. Additionally, vitamin D has been linked to improved immune system and mental wellness (14). Compared to mature milk, BC often has higher concentrations of most vitamins, including vitamins B2, B12, E, and D.
In accordance with a different scientific study, vitamin A found in cow’s milk may affect lymphocytes’ homing to the upper respiratory system by inducing the production of tissue homing-linked markers α4β7 (164). Additionally, vitamin A has been suggested as a substitute for CoV therapy and as a strategy to avoid lung infections. Jee et al. (2013) found that calves who received insufficient vitamin A had a reduced ability to produce antibodies in response to the inactivated BCoV vaccine (165). Therefore, vitamin A may play a supporting role in the management of COVID-19, in conjunction with the development of a favorable antibody response in those who have received the CoV vaccination. Numerous studies have shown that vitamin D can lower the incidence of pneumonia and acute viral respiratory tract infections (166, 167). Possible mechanisms of action include the prevention of viral replication, anti-inflammatory effects, and immunomodulatory effects (168). Studies have demonstrated that vitamin D decreases the overexpression of proinflammatory cytokines (TNF-α, IL-1, IL-1β, and IFN-γ), increases the expression of anti-inflammatory cytokines, and releases defensins and cathelicidins that halt viral replication (169). These findings suggest that vitamin D may expedite the recovery of patients with viral infections, particularly COVID-19 (170). The antibacterial and antioxidant properties of vitamin D, along with its role in enhancing the immune system’s resistance to lung infections and airway inflammation, make it a potentially effective treatment for SARS-CoV-2. Free radicals, reactive oxygen species (ROS), and reactive nitrogen species (RNS) are neutralized by the fat-soluble antioxidant vitamin E through the donation of hydrogen ions from its chromanol ring (171). As a result, it reduces oxidative stress, which is primarily responsible for acute respiratory distress syndrome, especially in the case of COVID-19 (172). One of the main causes of death in COVID-19 patients is ferroptosis, which is caused by a deficiency in vitamin E and results in various injuries to the heart, liver, kidneys, intestines, the neurological system, and other organs (173). Vitamin K plays a significant role in the pathogenesis of COVID-19, and its deficiency is linked to numerous organ damages, thrombotic complications, and high mortality (174). Vitamin K insufficiency during the initial stages of COVID-19 infection has been associated with increased IL-6 production and Th2 storm activation, according to research (175).
According to research, vitamin B12 has a high affinity for the SARS-CoV-2 protease (176). Additionally, a deficiency in vitamin B12 results in symptoms comparable to COVID-19, such as increased oxidative stress, elevated homocysteine levels, thrombocytopenia, elevated lactate dehydrogenase, low reticulocyte counts, vasoconstriction, and renal and pulmonary vasculopathies (177). Thus, symptoms of COVID-19 may be alleviated by vitamin B12. Vitamin C has antiviral properties, including reducing endothelial dysfunction, increasing and controlling the generation of IFN and cytokines, reducing inflammation, and restoring mitochondrial function (178).
Besides being rich in a number of vital elements, such as calcium, copper, iron, zinc, magnesium, manganese, and phosphorus, BC is also rich in mature milk (34, 68). Their concentration, however, varies widely and is influenced by numerous factors (179). Minerals might play a crucial role in the treatment and prevention of COVID-19. For instance, normal respiratory function, energy production, immune system strength, nerve conduction, blood coagulation, control of heart rate, hormone and enzyme secretion, and muscular contraction all depend on calcium (180). Numerous researchers have noted that individuals with COVID-19 frequently exhibit a high frequency of hypocalcemia (181). The worst clinical outcomes, including multiple organ dysfunction syndrome, septic shock, and mortality, were observed in COVID-19 patients with low serum calcium levels, particularly below 2.0 mmol/L (182). In addition to regulating fluid and electrolyte balance, sodium is crucial for maintaining normal cellular homeostasis. Studies on rats have shown that a high-sodium diet leads to a decrease in the expression of ACE2 in renal tissue (183). Additionally, it has been found that the COVID-19 disease progresses more severely when serum sodium concentrations are lower (184). This is accompanied by greater IL-6 production. Therefore, salt has a significant influence on the therapeutic outcomes of COVID-19 patients. It is suggested that sodium consumption levels be monitored in patients with severe COVID-19 infections and that low sodium intake be addressed as soon as possible (185). All species require copper as a micromineral, which is important for immune system functioning, protection against free radicals, and respiration (186). Copper improves cell-mediated immunity, the development of certain antibodies, and the functioning of T helper cells, B cells, neutrophils, NK cells, and macrophages in the fight against pathogenic microbes (187). Additionally, copper controls IL-2 levels, NK cell cytotoxicity, the balance between Th1 and Th2 cells, and T helper cell proliferation, all of which are crucial for addressing immunological dysregulation in COVID-19 patients (188). Copper can also downregulate the expression of NF-κB, which is typically stimulated by virus-induced ROS, to reduce the production of inflammatory cytokines, chemokines, and adhesion molecules (189). This could be particularly helpful during the COVID-19 cytokine storm syndrome phase.
In conclusion, in addition to the primary therapy during COVID-19, a number of vitamins and minerals may have symptomatic and supporting functions. Therefore, future research can focus on characterizing these components in BC and exploring their combined use with other bioactive compounds to enhance protection against infections and immune system modulation (190, 191).
3 Bovine colostrum applications in virus infections
3.1 Bovine colostrum for protection against respiratory infection
Upper respiratory tract infections (URTIs) are common types of tract infections caused by respiratory viruses such as SARS-CoV-2 that affect the respiratory system organs including mouth, nose, throat, larynx, and also trachea and can cause nasopharyngitis, sinusitis, pharyngitis, laryngitis and laryngotracheitis (192). The known symptoms of URTIs are considered sore throat, fatigue, headache, runny nose, and watery eyes (193).
Such findings of studies show that BC can decrease the incidence of URTI and symptoms of infection. In a clinical trial study by Brinkworth and Buckley, 2003, the effects of concentrated BC (CBC) protein on the incidence and self-reported symptoms duration of URTI were assessed. After an experimental process, the results of 60g/day CBC supplementation and 60 g/day whey protein supplementation were compared and they demonstrated there weren’t any differences in self-reported symptoms duration, statistically, whereas CBC supplementation reduced the URTI incidence, significantly (194). Patel and Rana, 2006, assessed how effective and tolerable BC is for the prevention of recurrent URTIs and diarrhea in children. According to their findings, 3.0 g of BC supplementation was found to be effective to reduce episodes of URTI and diarrhea (91.19% and 86.60% of patients, respectively). Supplementation was also found to be an option for the improvement of well-being (195). A similar study from Saad et al. resulted that the intake of BC was enabled to reduce URTI and episodes of diarrhea in 1-6 aged children who received BC supplementation (3.0 g to 6.0 g based on the aged) during 4 weeks (196).
To investigate the benefits of BC for the treatment of URT in children with IgA deficiency, patients received BC or placebo randomly three times each day during the one week. During this clinical study, salivary IgA level of children was detected before/after administration, between the doses of BC because salivary IgA and resistance to URT are directly correlated. It was reported that lower severity of the infection score was observed in the BC group than in the placebo group within a week (0.81 ± 0.83, 3.00 ± 1.85; P = 0.000, respectively). On the other hand, no differences between the groups’ salivary IgA levels were observed. Thus, researchers concluded that to validate the effectiveness of BC taken in patients who have IgA deficiency, more research and clinical study are required (197). Alfayoumi et al., 2020 conducted research along with numerous clinical investigations to examine the safety and effectiveness of the BC regimen in preventing URTI recurrence as well as the influence of BC on the nasal flora. In this case report, there were no URTI episodes over the year after starting the BC regimen as opposed to 5 incidents over the year before the BC regimen (p < 0.05). The findings of this case study show that BC was successful in preventing URTIs (198). These outcomes line up with those seen in past research (194, 199).
It is clear from looking at the clinical trials that have been done in this field that researchers have obtained promising results. A randomized, triple blind, placebo-controlled trial study was designed to determine whether the intervention could also help to other young adults who might have been at a higher risk of getting URTIs. For the duration of the study, medical (MED) and health science (HSci) students who were deemed to be at risk received a relatively small daily dose of either BC or a placebo for 45 days. On day 87, a second supplementation phase began and lasted for 7 days. The subjects were only observed for the course of the 107-day experiment by daily online questionnaires. These surveys asked questions regarding the subjects’ overall health, the frequency and severity of URTIs, and any probable gastrointestinal side effects. A significant reduction in the frequency of symptomatic days was seen in the group supplemented with BC compared to the placebo group, particularly among MED students compared to health HSci students, indicating a significant level of protection against URTIs. The intensity of symptoms as well as the general sense of well-being also showed a comparable effect. According to the trial results, BC supplementation can improve defense against URTIs, especially for people at high infectious risk (200).
The information gathered here suggests that BC can function as an adjuvant in preventing lower respiratory tract infections in all ages group. To assess the therapeutic potential of BC supplementation, research on the effects of colostrum supplementation on respiratory tract infections is required. Altogether, BC has gained importance with research based on Exercise Nutrition and Immunology.
3.2 Evidence for protective effects of bovine colostrum in enteric virus infection and SARS-CoV-2
BC has gained importance because of its important features, and it can be employed as a novel approach for the treatment of a variety of diseases in human beings. It is also considered a supportive component in terms of maintenance of different systems such as the gastrointestinal system, local/systemic immunity, etc. Additionally, nonsteroidal anti-inflammatory drugs (NSAIDs)-induced intestinal damage has been treated and prevented with the use of BC. It has been successfully used to treat a variety of joint ailments, including rheumatoid arthritis, and shows immune modulator properties for the treatment of allergic and auto-immune diseases. The use of BC in preventing and treating bacterial and viral infections is supported by several clinical observations (56, 57).
A key feature of BC is that BC and its bioactive components are beneficial to take care of potential upper respiratory tract infections, sinusitis, and pneumonia. In physiological and biological processes, BC is a promising approach for the prevention and treatment of COVID-19 because of its antibacterial/antiviral activity and its capacity to boost both the innate and adaptive immune systems of humans (191).
Systematic reviews, in-vitro and clinical studies reported that supplementation of BC against COVID-19 can reduce the rate of incidence, and disease symptoms and prevent infection of SARS-CoV-2. For the prevention of SARS-CoV-2 infection using a nasal spray strategy, researchers conducted a clinical study. In this trial, the effectiveness of a virus-neutralizing BC supplement delivered intravenously in preventing SARS-CoV-2 infection in household contacts of SARS-CoV-2-positive people was assessed and this trial is still recruiting (NCT number: NCT05552950).
The effect of BC was investigated for evaluation of early recovery in COVID-19 patients by Khartode, 2021. Khartode, 2021 categorized the patients based on their symptoms including mild, moderate, and severe using HRCT Chest Scan results and symptoms’ severity. According to statistical analysis, the early recovery for all symptom categories in the study group was found as significant (p<0.05). Khartode, 2021 resulted that BC intake could be beneficial for COVID-19 patients and their early recovery (84).
A double-blind, randomized control study was conducted to examine the effectiveness of a hen egg white and BC supplement in preventing severe COVID-19. Adults who had mild to moderate COVID-19, risk factors for severe disease, and had experienced symptoms during the last 5 days were split into two groups for this study: the intervention group (n = 77) and the placebo group (n = 79), who randomly assigned. Up to 42 days after enrolment, symptoms were monitored, and viral clearance was assessed 11-13 days after the onset of symptoms. Based on the study records, one participant out of the total number of subjects experienced severe COVID-19. The active arm displayed a decreased severe-type symptom score at 11 to 13 days following the beginning of symptoms in comparison to the placebo group (p = 0.049). In conclusion, the results show that when hen egg white and BC were combined, symptoms in those with mild or moderate COVID-19 were less severe. (Clinical Trial Registration: DOH-27-062021-9191) (201) Table 2.
Thus, a variety of in-vivo, in-vitro, and clinical trial data support the effectiveness of BC for the prevention of COVID-19 as an immune-boosting supplement (Figure 2). Additional efforts are needed to explore new uses and approaches in developing innovative BC-based treatments for COVID-19.
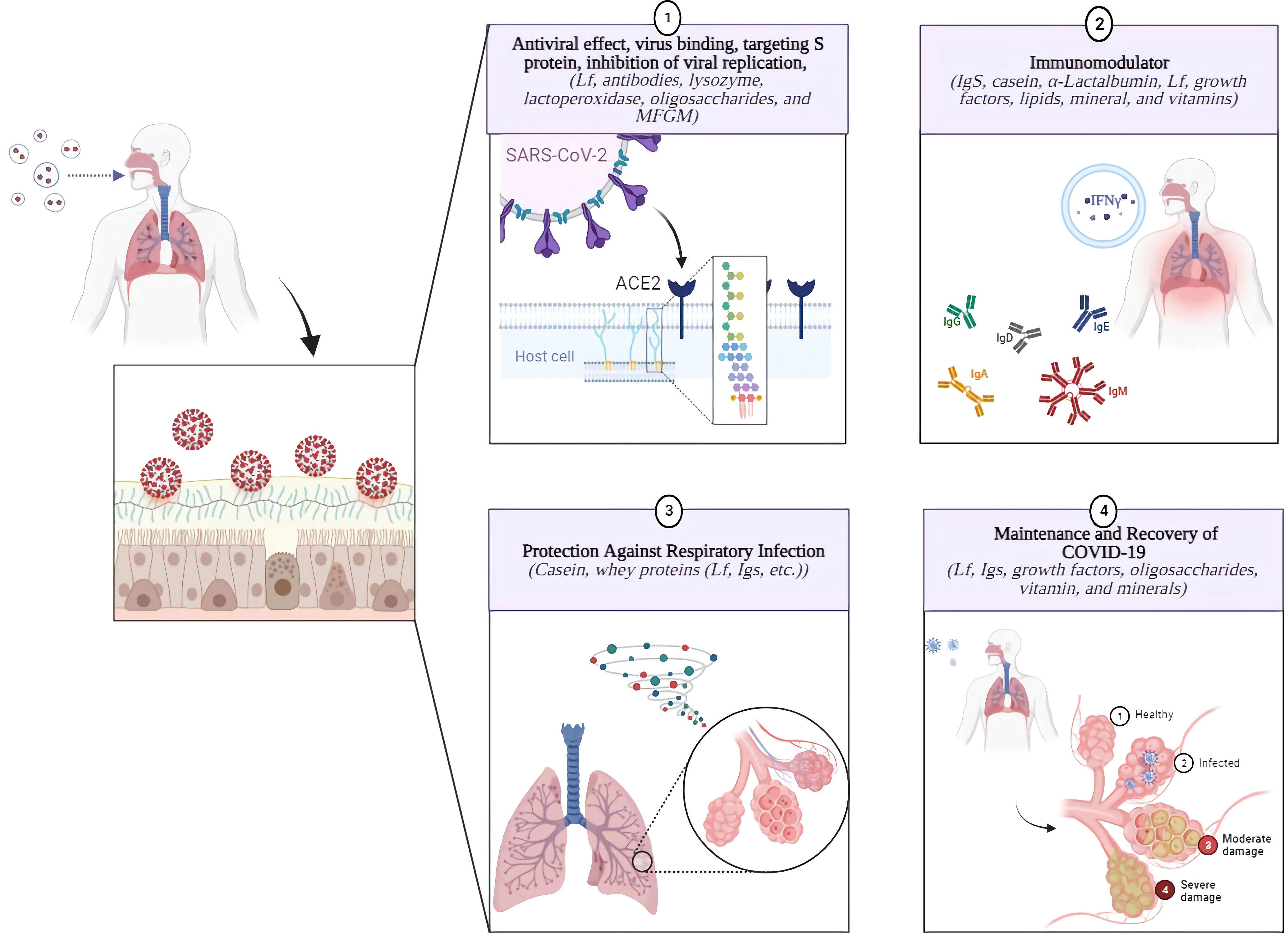
Figure 2 Bioactive Components of Bovine Colostrum and Their Significant Effects on Prevention and Management of COVID-19. BC may be considered a viable method to assisting in the treatment of SARS-CoV-2 patients or protection from the infection. BC has immunomodulatory effects due to its bioactive components, such as Igs, Lf, caseins, growth factors, among others, which may help modulate immune responses. Furthermore, its bioactive components, including Lf, antibodies, lysozyme, LPO, oligosaccharides, MFGM, etc., have a potential antiviral activity against SARS-CoV-2 and respiratory infections. Its comprehensive food profile is also effective in post-illness recovery by supporting a healthy immune system by providing proteins, oligosaccharides, vitamins, minerals, and amino acids (3, 12, 203).
4 Concluding remarks and outlook
The multifunctional effects of BC, including its antiviral, anti-inflammatory, and immunomodulatory properties, have generated a great deal of research over the years. Particularly in relation to viral diseases such as COVID-19, these qualities have garnered a lot of interest. The results of investigations on the emergence and management of COVID-19 have shown that BC and its bioactive components have promise as both non-pharmacological and pharmaceutical treatments for COVID-19.
Inhibiting virus infections and reducing the intensity of illness symptoms are two of BC supplementation’s main benefits. Because of its high concentration of bioactive components such as Igs, several immune boosting factors, BC has immunomodulatory effects, potentially supporting a balanced immune response. Additionally, its bioactive components may cross-react with SARS-CoV-2, assisting in virus neutralization, and its comprehensive dietary profile provides essential vitamins, minerals, and amino acids, promoting a healthy immune system. This shows that BC might be an effective preventative measure against SARS-CoV-2 infection, lowering the chance of infection or lessening its effects if infection does happen. It is crucial to stress that any usage of BC for COVID-19 prevention or management should be done so under the guidance of a qualified medical expert to ensure safety and efficacy.
There is a need for additional research into the creation of BC-based products given the significant medicinal potential of BC and its bioactive components. Infections caused by other viruses as well as COVID-19 may be successfully treated with these products. Investigating the potential of BC becomes even more important in the context of public health emergencies, like as pandemics, where quick and efficient preventive actions are essential.
The use of BC and its bioactive ingredients is not just restricted to the medical field. Products with BC-derived ingredients can be beneficial for a variety of sectors, including the pharmaceutical and food sectors. The adaptability of BC makes it a desirable choice for creating novel products with potential health advantages. Additional scientific study is crucial to advancing the use of BC and its bioactive components. To prove its safety and effectiveness in the prevention and treatment of COVID-19 and other disorders, it is required to investigate its mechanisms of action, optimize dosages, and carry out clinical trials.
In conclusion, BC is a promising choice for further scientific investigation due to its multifunctional qualities and potential as a different strategy to combat COVID-19. Other industries might gain from its implementation, which could go beyond the medical sector. To assure the efficiency and safety of BC-based products in treating public health emergencies and enhancing overall health outcomes, it is required to approach their creation and use with a rigorous scientific research and expert supervision.
Author contributions
HD and SK both contributed significantly to the work’s conception, design, literature searches and interpretation, and HD drafted the article and SK provided critical revision of the article; both HD and SK have read and approved the manuscript. All authors provided comments, direction, and advice in updating manuscript drafts to the final version. All authors contributed to the article and approved the submitted version.
Funding
The authors declare that this study received funding from TUBITAK #118z146, Uluova Süt Ticaret A.S. (Uluova Milk Trading Co.), and Elit Çikolata ve Şekerleme A.S. (Elit Chocolate and Confectionery). The funders were not involved in the study design, collection, analysis, interpretation of data, the writing of this article, or the decision to submit it for publication.
Conflict of interest
The authors declare that the research was conducted in the absence of any commercial or financial relationships that could be construed as a potential conflict of interest.
Publisher’s note
All claims expressed in this article are solely those of the authors and do not necessarily represent those of their affiliated organizations, or those of the publisher, the editors and the reviewers. Any product that may be evaluated in this article, or claim that may be made by its manufacturer, is not guaranteed or endorsed by the publisher.
References
1. Godhia ML, Patel N. Colostrum - Its composition, benefits as a nutraceutical : A review. Curr Res Nutr Food Sci (2013) 1(1):37–47. doi: 10.12944/CRNFSJ.1.1.04
2. Korhonen H, Marnila P, Gill HS. Milk immunoglobulins and complement factors. Br J Nutr (2000) 84:75–80. doi: 10.1017/S0007114500002282
3. Lin T, Meletharayil G, Kapoor R, Abbaspourrad A. Bioactives in bovine milk: Chemistry, technology, and applications. Nutr Rev (2021) 79:48–69. doi: 10.1093/nutrit/nuab099
4. Vegarud GE, Langsrud T, Svenning C. Mineral-binding milk proteins and peptides; Occurrence, biochemical and technological characteristics. Br J Nutr (2000) 84(S1):91–8. doi: 10.1017/s0007114500002300
5. Pihlanto-Leppälä A. Bioactive peptides derived from bovine whey proteins: Opioid and ace-inhibitory peptides. Trends Food Sci Technol (2000) 11(9-10):347–56. doi: 10.1016/S0924-2244(01)00003-6
6. Pihlanto A, Korhonen H. Bioactive peptides and proteins. Adv Food Nutr Res (2003) 47:175–276. doi: 10.1016/S1043-4526(03)47004-6
7. Wheeler TT, Hodgkinson AJ, Prosser CG, Davis SR. Immune components of colostrum and milk - A historical perspective. J Mammary Gland Biol Neoplasia (2007) 12(4):237–47. doi: 10.1007/s10911-007-9051-7
8. Van Hooijdonk ACM, Kussendrager KD, Steijns JM. In vivo antimicrobial and antiviral activity of components in bovine milk and colostrum involved in non-specific defence. Br J Nutr (2000) 84(S1):127–34. doi: 10.1017/s000711450000235x
9. Pakkanen R, Aalto J. Growth factors and antimicrobial factors of bovine colostrum. Int Dairy J (1997) 7(5):285–97. doi: 10.1016/S0958-6946(97)00022-8
10. Kazimierska K, Kalinowska-Lis U. Milk proteins-their biological activities and use in cosmetics and dermatology. Molecules (2021) 26(11):3253. doi: 10.3390/molecules26113253
11. Shah NP. Effects of milk-derived bioactives: An overview. Br J Nutr (2000) 84(S1):3–10. doi: 10.1017/s000711450000218x
12. Korhonen HJ. Bioactive milk proteins, peptides and lipids and other functional components derived from milk and bovine colostrum. Funct Foods (2011) 471–511. doi: 10.1533/9780857092557.3.471
13. Barile D, Marotta M, Chu C, Mehra R, Grimm R, Lebrilla CB, et al. Neutral and acidic oligosaccharides in Holstein-Friesian colostrum during the first 3 days of lactation measured by high performance liquid chromatography on a microfluidic chip and time-of-flight mass spectrometry. J Dairy Sci (2010) 93:3940–9. doi: 10.3168/jds.2010-3156
14. Playford RJ, Weiser MJ. Bovine colostrum: Its constituents and uses. Nutrients (2021) 13(1):265. doi: 10.3390/nu13010265
15. Mehra R, Singh R, Nayan V, Buttar HS, Kumar N, Kumar S, et al. Nutritional attributes of bovine colostrum components in human health and disease: A comprehensive review. Food Biosci (2021) 40:100907. doi: 10.1016/j.fbio.2021.100907
16. Buttar HS, Bagwe SM, Bhullar SK, Kaur G. Health Benefits of Bovine Colostrum in Children and Adults. In: Dairy in Human Health and Disease Across the Lifespan. (Cambridge, MA: Elsevier, Academic Press), (2017) p. 3–20. doi: 10.1016/B978-0-12-809868-4.00001-7
17. McGrath BA, Fox PF, McSweeney PLH, Kelly AL. Composition and properties of bovine colostrum: a review. Dairy Sci Technol (2016) 96:133–58. doi: 10.1007/s13594-015-0258-x
18. Arslan A, Duman H, Kaplan M, Uzkuç H, Bayraktar A, Ertürk M, et al. Determining total protein and bioactive protein concentrations in bovine colostrum. J Vis Exp (2021) 178(e63001). doi: 10.3791/63001
19. Stelwagen K, Carpenter E, Haigh B, Hodgkinson A, Wheeler TT. Immune components of bovine colostrum and milk. J Anim Sci (2009) 87(suppl_13):3–9. doi: 10.2527/jas.2008-1377
20. Moody EG, Wise GH, Parrish DB, Atkeson FW. Properties of the colostrum of the dairy cow. VI. Creaming and rate of flow. J Dairy Sci (1951) 34(2):106–15. doi: 10.3168/jds.S0022-0302(51)91678-5
21. Parrish DB, Wise GH, Hughes JS, Atkeson FW. Properties of the colostrum of the dairy cow. V. Yield, specific gravity and concentrations of total solids and its various components of colostrum and early milk. J Dairy Sci (1950) 33(6):457–65. doi: 10.3168/jds.S0022-0302(50)91921-7
22. Parrish DB, Wise GH, Hughes JS. Properties of the colostrum of the dairy cow. IV. Effect of form of vitamin A and of tocopherol supplements on concentrations of vitamin A and carotenoids. J Dairy Sci (1949) 32(5):458–64. doi: 10.3168/jds.S0022-0302(49)92065-2
23. Parrish DB, Wise GH, Hughes JS, Atkeson FW. Properties of the colostrum of the dairy cow. II. Effect of prepartal rations upon the nitrogenous constituents. J Dairy Sci (1948) 31(10):889–95. doi: 10.3168/jds.S0022-0302(48)92273-5
24. Parrish DB, Wise GH, Hughes JS. Properties of the colostrum of the dairy cow. I. Tocopherol levels in the colostrum and in the early milk. J Dairy Sci (1947) 30(11):849–60. doi: 10.3168/jds.S0022-0302(47)92408-9
25. Sangild PT, Vonderohe C, Melendez Hebib V, Burrin DG. Potential benefits of bovine colostrum in pediatric nutrition and health. Nutrients (2021) 13:1–41. doi: 10.3390/nu13082551
26. Mehra R, Garhwal R, Sangwan K, Guiné RPF, Lemos ET, Buttar HS, et al. Insights into the research trends on bovine colostrum: beneficial health perspectives with special reference to manufacturing of functional foods and feed supplements. Nutrients (2022) 14(3):659. doi: 10.3390/nu14030659
27. Kaplan M, Arslan A, Duman H, Karyelioğlu M, Baydemir B, Günar BB, et al. Production of bovine colostrum for human consumption to improve health. Front Pharmacol (2022) 12:796824. doi: 10.3389/fphar.2021.796824
28. Lai C-C, Shih T-P, Ko W-C, Tang H-J, Hsueh P-R. Severe acute respiratory syndrome coronavirus 2 (SARS-CoV-2) and coronavirus disease-2019 (COVID-19): The epidemic and the challenges. Int J Antimicrob Agents (2020) 55(3):105924. doi: 10.1016/j.ijantimicag.2020.105924
29. Tay MZ, Poh CM, Rénia L, MacAry PA, Ng LFP. The trinity of COVID-19: immunity, inflammation and intervention. Nat Rev Immunol (2020) 20(6):363–74. doi: 10.1038/s41577-020-0311-8
30. Wang D, Hu B, Hu C, Zhu F, Liu X, Zhang J, et al. Clinical characteristics of 138 hospitalized patients with 2019 novel coronavirus-infected pneumonia in Wuhan, China. JAMA - J Am Med Assoc (2020) 323(11):1061–9. doi: 10.1001/jama.2020.1585
31. Gorbalenya AE, Baker SC, Baric RS, de Groot RJ, Drosten C, Gulyaeva AA, et al. The species Severe acute respiratory syndrome-related coronavirus: classifying 2019-nCoV and naming it SARS-CoV-2. Nat Microbiol (2020) 5(4):536–44. doi: 10.1038/s41564-020-0695-z
32. Chang R, Ng TB, Sun WZ. Lactoferrin as potential preventative and adjunct treatment for COVID-19. Int J Antimicrob Agents (2020) 56(3):106118. doi: 10.1016/j.ijantimicag.2020.106118
33. Marnila P, Korhonen H. COLOSTRUM. In: Encyclopedia of Dairy Sciences (Cambridge, MA: Elsevier, Academic Press), (2002) p. 473–8. doi: 10.1016/b0-12-227235-8/00096-1
34. Godden S. Colostrum management for dairy calves. Vet Clin North Am - Food Anim Pract (2008) 24(1):19–39. doi: 10.1016/j.cvfa.2007.10.005
35. Pereira PC. Milk nutritional composition and its role in human health. Nutrition (2014) 30(6):619–27. doi: 10.1016/j.nut.2013.10.011
36. Dash S, Jaganmohan R. Quality of cold plasma treated casein peptide targeting SARS-coV2: an in-silico approach. (2022). 1–19. doi: 10.21203/rs.3.rs-2199614/v1
37. Manso MA, López-Fandiño R. Angiotensin I converting enzyme - Inhibitory activity of bovine, ovine, and caprine κ-casein macropeptides and their tryptic hydrolysates. J Food Prot (2003) 66:1686–92. doi: 10.4315/0362-028X-66.9.1686
38. Brody EP. Biological activities of bovine glycomacropeptide. Br J Nutr (2000) 84(S1):39–46. doi: 10.1017/s0007114500002233
39. Córdova-Dávalos LE, Jiménez M, Salinas E. Glycomacropeptide bioactivity and health: A review highlighting action mechanisms and signaling pathways. Nutrients (2019) 11(3):598. doi: 10.3390/nu11030598
40. Siqueiros-Cendón T, Arévalo-Gallegos S, Iglesias-Figueroa BF, García-Montoya IA, Salazar-Martínez J, Rascón-Cruz Q. Immunomodulatory effects of lactoferrin. Acta Pharmacol Sin (2014) 35(5):557–66. doi: 10.1038/aps.2013.200
41. Korhonen HJ. Production and properties of health-promoting proteins and peptides from bovine colostrum and milk. Cell Mol Biol (2013) 3;59(1):12–24. doi: 10.1170/T943
42. Karav S, German JB, Rouquié C, Le Parc A, Barile D. Studying lactoferrin N-glycosylation. Int J Mol Sci (2017) 18(4):870. doi: 10.3390/ijms18040870
43. Karav S. Selective deglycosylation of lactoferrin to understand glycans’ contribution to antimicrobial activity of lactoferrin. Cell Mol Biol (2018) 64(9):52–7. doi: 10.14715/cmb/2018.64.9.8
44. Serrano G, Kochergina I, Albors A, Diaz E, Oroval M, Hueso G, et al. Liposomal lactoferrin as potential preventative and cure for COVID-19. Int J Res Heal Sci (2020) 8:08–15. doi: 10.5530/ijrhs.8.1.3
45. Bolat E, Eker F, Kaplan M, Duman H. Lactoferrin for COVID- prevention. treatment recovery (2022) 7:992733(9). doi: 10.3389/fnut.2022.992733
46. Peroni DG. Viral infections: Lactoferrin, a further arrow in the quiver of prevention. J Pediatr Neonatal Individ Med (2020) 9:9–11. doi: 10.7363/090142
47. Shen RN. Protective influence of lactoferrin on mice infected with the polycythemia-inducing strain of friend virus complex. Cancer Res (1987) 47(15):4184–8.
48. Lang J, Yang N, Deng J, Liu K, Yang P, Zhang G, et al. Inhibition of SARS pseudovirus cell entry by lactoferrin binding to heparan sulfate proteoglycans. PloS One (2011) 6(8):e23710. doi: 10.1371/journal.pone.0023710
49. Andersen JH, Jenssen H, Gutteberg TJ. Lactoferrin and lactoferricin inhibit Herpes simplex 1 and 2 infection and exhibit synergy when combined with acyclovir. Antiviral Res (2003) 58(3):209–15. doi: 10.1016/S0166-3542(02)00214-0
50. Andersen JH, Jenssen H, Sandvik K, Gutteberg TJ. Anti-HSV activity of lactoferrin and lactoferricin is dependent on the presence of heparan sulphate at the cell surface. J Med Virol (2004) 74(2):262–71. doi: 10.1002/jmv.20171
51. Berkhout B, Floris R, Recio I, Visser S. The antiviral activity of the milk protein lactoferrin against the human immunodeficiency virus type 1. BioMetals (2004) 17(3):291–4. doi: 10.1023/B:BIOM.0000027707.82911.be
52. Van der Strate BWA, Beljaars L, Molema G, Harmsen MC, Meijer DKF. Antiviral activities of lactoferrin. Antiviral Res (2001) 52:225–39. doi: 10.1016/S0166-3542(01)00195-4
53. Superti F, Ammendolia MG, Valenti P, Seganti L. Antirotaviral activity of milk proteins: Lactoferrin prevents rotavirus infection in the enterocyte like cell line HT-29. Med Microbiol Immunol (1997) 186(2-3):83–91. doi: 10.1007/s004300050049
54. Superti F, Berlutti F, Paesano R, Valenti P. Structure and activity of lactoferrin—A multi-functional protective agent for human health. In Iron Metab Dis (2008).
56. Carvalho CAM, Casseb SMM, Gonçalves RB, Silva EVP, Gomes AMO, Vasconcelos PFC. Bovine lactoferrin activity against Chikungunya and Zika viruses. J Gen Virol (2017) 98:1749–54. doi: 10.1099/jgv.0.000849
57. Yadav R, Angolkar T, Kaur G, S. Buttar H. Antibacterial and antiinflammatory properties of bovine colostrum. Recent Pat Inflammation Allergy Drug Discovery (2016) 10(1):49–53. doi: 10.2174/1872214810666160219163118
58. Campione E, Lanna C, Cosio T, Rosa L, Conte MP, Iacovelli F, et al. Lactoferrin as antiviral treatment in COVID-19 management: Preliminary evidence. Int J Environ Res Public Health (2021) 18:1–15. doi: 10.3390/ijerph182010985
59. Kell DB, Heyden EL, Pretorius E. The biology of lactoferrin, an iron-binding protein that can help defend against viruses and bacteria. Front Immunol (2020) 11:1221. doi: 10.3389/fimmu.2020.01221
60. Salaris C, Scarpa M, Elli M, Bertolini A, Guglielmetti S, Pregliasco F, et al. Protective effects of lactoferrin against sars-cov-2 infection in vitro. Nutrients (2021) 13:1–12. doi: 10.3390/nu13020328
61. Wotring JW, Fursmidt R, Ward L, Sexton JZ. Evaluating the in vitro efficacy of bovine lactoferrin products against SARS-CoV-2 variants of concern. J Dairy Sci (2022) 105:2791–802. doi: 10.3168/jds.2021-21247
62. Dalamaga M, Karampela I, Mantzoros CS. Commentary: Could iron chelators prove to be useful as an adjunct to COVID-19 Treatment Regimens? Metabolism (2020) 108:154260. doi: 10.1016/j.metabol.2020.154260
63. Hurley WL, Theil PK. Perspectives on immunoglobulins in colostrum and milk. Nutrients (2011) 3(4):442–74. doi: 10.3390/nu3040442
64. Kusumaningrum CE, Widyasari EM, Sriyani ME, Wongso H. Pharmacological activities and potential use of bovine colostrum for peptide-based radiopharmaceuticals: A review. Pharmacia (2021) 68:471–7. doi: 10.3897/PHARMACIA.68.E65537
65. Mehra R, Marnila P, Korhonen H. Milk immunoglobulins for health promotion. Int Dairy J (2006) 16(11):1262–71. doi: 10.1016/j.idairyj.2006.06.003
66. Tsioulpas A, Grandison AS, Lewis MJ. Changes in physical properties of bovine milk from the colostrum period to early lactation. J Dairy Sci (2007) 90(11):5012–7. doi: 10.3168/jds.2007-0192
67. Madsen BD, Rasmussen MD, Nielsen MO, Wiking L, Larsen LB. Physical properties of mammary secretions in relation to chemical changes during transition from colostrum to milk. J Dairy Res (2004) 71(3):263–72. doi: 10.1017/S0022029904000263
68. Kehoe SI, Jayarao BM, Heinrichs AJ. A survey of bovine colostrum composition and colostrum management practices on Pennsylvania dairy farms. J Dairy Sci (2007) 90(9):4108–16. doi: 10.3168/jds.2007-0040
69. Feeney S, Morrin ST, Joshi L, Hickey RM. The role of immunoglobulins from bovine colostrum and milk in human health promotion. In: Novel proteins for food, pharmaceuticals and agriculture. p. 291–314. doi: 10.1002/9781119385332.ch16
70. Gapper LW, Copestake DEJ, Otter DE, Indyk HE. Analysis of bovine immunoglobulin G in milk, colostrum and dietary supplements: A review. Anal Bioanal Chem (2007) 389(1):93–109. doi: 10.1007/s00216-007-1391-z
71. Korhonen H. Milk-derived bioactive peptides: From science to applications. J Funct Foods (2009) 1(2):177–87. doi: 10.1016/j.jff.2009.01.007
72. Ulfman LH, Leusen JHW, Savelkoul HFJ, Warner JO, van Neerven RJJ. Effects of bovine immunoglobulins on immune function, allergy, and infection. Front Nutr (2018) 5:52. doi: 10.3389/fnut.2018.00052
73. Ellens DJ, de Leeuw PW, Straver PJ. The detection of rotavirus specific antibody in colostrum and milk by ELISA. Ann Rech Vet (1978) 9(2):337–42.
74. Rump JA, Arndt R, Arnold A, Bendick C, Dichtelmüller H, Franke M, et al. Treatment of diarrhoea in human immunodeficiency virus-infected patients with immunoglobulins from bovine colostrum. Clin Investig (1992) 70:588–94. doi: 10.1007/BF00184800
76. Lissner R, Schmidt H, Karch H. A standard immunoglobulin preparation produced from bovine colostra shows antibody reactivity and neutralization activity against Shiga-like toxins and EHEC-hemolysin of Escherichia coli O157:H7. Infection (1996) 24(5):378–83. doi: 10.1007/BF01716084
77. Den Hartog G, Jacobino S, Bont L, Cox L, Ulfman LH, Leusen JHW, et al. Specificity and effector functions of human RSV-specific IgG from bovine milk. PloS One (2014) 9(11):e112047. doi: 10.1371/journal.pone.0112047
78. Kramski M, Center RJ, Wheatley AK, Jacobson JC, Alexander MR, Rawlin G, et al. Hyperimmune bovine colostrum as a low-cost, large-scale source of antibodies with broad neutralizing activity for HIV-1 envelope with potential use in microbicides. Antimicrob Agents Chemother (2012) 56:4310–9. doi: 10.1128/AAC.00453-12
79. Ng WC, Wong V, Muller B, Rawlin G, Brown LE. Prevention and treatment of influenza with hyperimmune bovine colostrum antibody. PloS One (2010) 5:2–11. doi: 10.1371/journal.pone.0013622
80. Xu ML, Kim HJ, Wi GR, Kim HJ. The effect of dietary bovine colostrum on respiratory syncytial virus infection and immune responses following the infection in the mouse. J Microbiol (2015) 53(9):661–6. doi: 10.1007/s12275-015-5353-4
81. Wong EB, Mallet J-F, Duarte J, Matar C, Ritz BW. Bovine colostrum enhances natural killer cell activity and immune response in a mouse model of influenza infection and mediates intestinal immunity through toll-like receptors 2 and 4. Nutr Res (2014) 34:318–25. doi: 10.1016/j.nutres.2014.02.007
82. Sponseller JK, Steele JA, Schmidt DJ, Kim HB, Beamer G, Sun X, et al. Hyperimmune bovine colostrum as a novel therapy to combat clostridium difficile infection. J Infect Dis (2015) 211(8):1334–41. doi: 10.1093/infdis/jiu605
83. Florén CH, Chinenye S, Elfstrand L, Hagman C, Ihse I. ColoPlus, a new product based on bovine colostrum, alleviates HIV-associated diarrhoea. Scand J Gastroenterol (2006) 41(6):682–6. doi: 10.1080/00365520500380817
84. Khartode SS. Early recovery of COVID-19 patients by using immunoglobulins present in cow colostrum food supplement-A clinical study. J Res Med Dent Sci (2021) 9:186–98.
85. Nili H, Bouzari M, Attaran HR, Ghalegolab N, Rabani M, Mahmoudian A. Hyper-immune bovine milk as an immunological and nutritional supplement for COVID-19. Front Nutr (2022) 9:868964. doi: 10.3389/fnut.2022.868964
86. Kangro K, Kurašin M, Gildemann K, Sankovski E, Žusinaite E, Lello LS, et al. Bovine colostrum-derived antibodies against SARS-CoV-2 show great potential to serve as prophylactic agents. PloS One (2022) 17:1–17. doi: 10.1371/journal.pone.0268806
87. Ishay Y, Potruch A, Weksler-Zangen S, Shabat Y, Ilan Y. Augmented antiviral T cell immunity by oral administration of IMM-124E in preclinical models and a phase I/IIa clinical trial: A method for the prevention and treatment of COVID-19. Drug Dev Res (2022) 83:615–21. doi: 10.1002/ddr.21890
88. Hahn R, Schulz PM, Schaupp C, Jungbauer A. Bovine whey fractionation based on cation-exchange chromatography. J Chromatogr A (1998) 795(2):277–87. doi: 10.1016/S0021-9673(97)01030-3
89. Fox PF, Kelly AL. Indigenous enzymes in milk: Overview and historical aspects - Part 1. Int Dairy J (2006) 16(6):500–16. doi: 10.1016/j.idairyj.2005.09.013
90. Fasse S, Alarinta J, Frahm B, Wirtanen G. Bovine colostrum for human consumption—Improving microbial quality and maintaining bioactive characteristics through processing. Dairy (2021) 2(4):556–75. doi: 10.3390/dairy2040044
91. Seifu E, Buys EM, Donkin EF. Significance of the lactoperoxidase system in the dairy industry and its potential applications: A review. Trends Food Sci Technol (2005) 16(4):137–54. doi: 10.1016/j.tifs.2004.11.002
92. EL-Fakharany EM, Abd-Elhamid AI, El-Deeb NM. Preparation and characterization of novel nanocombination of bovine lactoperoxidase with Dye Decolorizing and anti-bacterial activity. Sci Rep (2019) 9(1):8530. doi: 10.1038/s41598-019-44961-2
93. Cegolon L, Javanbakht M, Mastrangelo G. Nasal disinfection for the prevention and control of COVID-19: A scoping review on potential chemo-preventive agents. Int J Hyg Environ Health (2020) 230:113605. doi: 10.1016/j.ijheh.2020.113605
94. Shin K, Wakabayashi H, Yamauchi K, Teraguchi S, Tamura Y, Kurokawa M, et al. Effects of orally administered bovine lactoferrin and lactoperoxidase on influenza virus infection in mice. J Med Microbiol (2005) 54(8):717–23. doi: 10.1099/jmm.0.46018-0
95. Shin K, Wakabayashi H, Sugita C, Yoshida H, Sato K, Sonoda T, et al. Effects of orally administered lactoferrin and lactoperoxidase on symptoms of the common cold. Int J Health Sci (Qassim) (2018) 12:44–50.
96. Pan Y, Shiell B, Wan J, Coventry MJ, Michalski WP, Lee A, et al. The molecular characterisation and antimicrobial properties of amidated bovine β-lactoglobulin. Int Dairy J (2007) 17(12):1450–9. doi: 10.1016/j.idairyj.2007.04.006
97. Chatterton DEW, Smithers G, Roupas P, Brodkorb A. Bioactivity of β-lactoglobulin and α-lactalbumin-Technological implications for processing. Int Dairy J (2006) 16(11):1229–40. doi: 10.1016/j.idairyj.2006.06.001
98. Oevermann A, Engels M, Thomas U, Pellegrini A. The antiviral activity of naturally occurring proteins and their peptide fragments after chemical modification. Antiviral Res (2003) 59:23–33. doi: 10.1016/S0166-3542(03)00010-X
99. Markus CR, Olivier B, De Haan EHF. Whey protein rich in α-lactalbumin increases the ratio of plasma tryptophan to the sum of the other large neutral amino acids and improves cognitive performance in stress-vulnerable subjects. Am J Clin Nutr (2002) 75(6):1051–6. doi: 10.1093/ajcn/75.6.1051
100. Garg S, Nurgali K, Kumar Mishra V. Food proteins as source of opioid peptides-A review. Curr Med Chem (2016) 23(9):893–910. doi: 10.2174/0929867323666160219115226
101. Pellegrini A, Thomas U, Bramaz N, Hunziker P, Von Fellenberg R. Isolation and identification of three bactericidal domains in the bovine α-lactalbumin molecule. Biochim Biophys Acta - Gen Subj (1999) 1426(3):439–48. doi: 10.1016/S0304-4165(98)00165-2
102. Ragland SA, Criss AK. From bacterial killing to immune modulation: Recent insights into the functions of lysozyme. PloS Pathog (2017) 13(9):e1006512. doi: 10.1371/journal.ppat.1006512
103. Mulder AM, Connellan PA, Oliver CJ, Morris CA, Stevenson LM. Bovine lactoferrin supplementation supports immune and antioxidant status in healthy human males. Nutr Res (2008) 28(9):583–9. doi: 10.1016/j.nutres.2008.05.007
104. Primo ED, Otero LH, Ruiz F, Klinke S, Giordano W. The disruptive effect of lysozyme on the bacterial cell wall explored by an in-silico structural outlook. Biochem Mol Biol Educ (2018) 46(1):83–90. doi: 10.1002/bmb.21092
105. Feng K, Wen P, Yang H, Li N, Lou WY, Zong MH, et al. Enhancement of the antimicrobial activity of cinnamon essential oil-loaded electrospun nanofilm by the incorporation of lysozyme. RSC Adv (2017) 7(3):1572–80. doi: 10.1039/c6ra25977d
106. Ferrari R, Callerio C, Podio G. Antiviral activity of lysozyme. Nature (1959) 183(46660):548–8. doi: 10.1038/183548a0
107. Lee-Huang S, Huang PL, Sun Y, Huang PL, Kung HF, Blithe DL, et al. Lysozyme and RNases as anti-HIV components in β-core preparations of human chorionic gonadotropin. Proc Natl Acad Sci U.S.A. (1999) 96(6):2678–81. doi: 10.1073/pnas.96.6.2678
108. Griswold KE, Bement JL, Teneback CC, Scanlon TC, Wargo MJ, Leclair LW. Bioengineered lysozyme in combination therapies for Pseudomonas aeruginosa lung infections. Bioengineered (2014) 5(2):143–7. doi: 10.4161/bioe.28335
109. Helmfors L, Boman A, Civitelli L, Nath S, Sandin L, Janefjord C, et al. Protective properties of lysozyme on β-amyloid pathology: Implications for Alzheimer disease. Neurobiol Dis (2015) 83:122–33. doi: 10.1016/j.nbd.2015.08.024
110. Shalhoub S. Interferon beta-1b for COVID-19. Lancet (2020) 395(10238):1670–1. doi: 10.1016/S0140-6736(20)31101-6
111. Acharya D, Liu GQ, Gack MU. Dysregulation of type I interferon responses in COVID-19. Nat Rev Immunol (2020) 20(7):397–8. doi: 10.1038/s41577-020-0346-x
112. Korhonen H. Antimicrobial factors in bovine colostrum. Agric Food Sci (1977) 49(5):434–47. doi: 10.23986/afsci.71956
113. Fiore M, Chaldakov GN, Aloe L. Nerve growth factor as a signaling molecule for nerve cells and also for the neuroendocrine-immune systems. Rev Neurosci (2009) 20(2)133–45. doi: 10.1515/REVNEURO.2009.20.2.133
114. Galazios G, Papazoglou D, Tsikouras P, Kolios G. Vascular endothelial growth factor gene polymorphisms and pregnancy. J Matern Neonatal Med (2009) 22(5):371–8. doi: 10.1080/14767050802645035
115. Gauthier SF, Pouliot Y, Maubois JL. Growth factors from bovine milk and colostrum: Composition, extraction and biological activities. Lait (2006) 86(2):99–125. doi: 10.1051/lait:2005048
116. Ihara S, Hirata Y, Koike K. TGF-β in inflammatory bowel disease: a key regulator of immune cells, epithelium, and the intestinal microbiota. J Gastroenterol (2017) 52(7):777–87. doi: 10.1007/s00535-017-1350-1
117. Kelly A, Houston SA, Sherwood E, Casulli J, Travis MA. Regulation of innate and adaptive immunity by TGFβ. In: Advances in immunology. (Cambridge, MA: Elsevier, Academic Press), (2017) p. 137–233. doi: 10.1016/bs.ai.2017.01.001
118. Travis MA, Sheppard D. TGF-β activation and function in immunity. Annu Rev Immunol (2014) 32(1):51–82. doi: 10.1146/annurev-immunol-032713-120257
119. Hansen G, McIntire JJ, Yeung VP, Berry G, Thorbecke GJ, Chen L, et al. CD4+ T helper cells engineered to produce latent TGF-β1 reverse allergen-induced airway hyperreactivity and inflammation. J Clin Invest (2000) 105(1):61–70. doi: 10.1172/JCI7589
120. Oddy WH, Rosales F. A systematic review of the importance of milk TGF-β on immunological outcomes in the infant and young child. Pediatr Allergy Immunol (2010) 21(1-Part-I):47–59. doi: 10.1111/j.1399-3038.2009.00913.x
121. Duman H, Kaplan M, Arslan A, Sahutoglu AS, Kayili HM, Frese SA, et al. Potential Applications of Endo-β-N-Acetylglucosaminidases From Bifidobacterium longum Subspecies infantis in Designing Value-Added, Next-Generation Infant Formulas. Front Nutr (2021) 8:646275. doi: 10.3389/fnut.2021.646275
122. Martín-Sosa S, Martín MJ, García-Pardo LA, Hueso P. Sialyloligosaccharides in human and bovine milk and in infant formulas: Variations with the progression of lactation. J Dairy Sci (2003) 86:52–9. doi: 10.3168/jds.S0022-0302(03)73583-8
123. Nakamura T, Kawase H, Kimura K, Watanabe Y, Ohtani M, Arai I, et al. Concentrations of sialyloligosaccharides in bovine colostrum and milk during the prepartum and early lactation. J Dairy Sci (2003) 86:1315–20. doi: 10.3168/jds.S0022-0302(03)73715-1
124. McJarrow P, Van Amelsfort-Schoonbeek J. Bovine sialyl oligosaccharides: Seasonal variations in their concentrations in milk, and a comparison of the colostrums of Jersey and Friesian cows. Int Dairy J (2004) 14:571–9. doi: 10.1016/j.idairyj.2003.11.006
125. Bunyatratchata A, Le Parc A, de Moura Bell JMLN, Cohen JL, Duman H, Arslan A, et al. Release of bifidogenic N-glycans from native bovine colostrum proteins by an endo-β-N-acetylglucosaminidase. Enzyme Microb Technol (2023) 162:110138. doi: 10.1016/j.enzmictec.2022.110138
126. Karav S, Maria J, Nobrega L, Bell DM, Le Parc A, Liu Y, et al. Characterizing the release of bioactive N- glycans from dairy products by a novel endo- b - N- acetylglucosaminidase. Biotechnology Progress (2015) 31:1331–9. doi: 10.1002/btpr.2135
127. Arslan A, Kaplan M, Duman H, Bayraktar A, Ertürk M, Henrick BM, et al. Bovine colostrum and its potential for human health and nutrition. Front Nutr (2021) 8:651721. doi: 10.3389/fnut.2021.651721
128. Gopal PK, Gill HS. Oligosaccharides and glycoconjugates in bovine milk and colostrum. Br J Nutr (2000) 84:69–74. doi: 10.1017/s0007114500002270
129. Coppa GV, Bruni S, Morelli L, Soldi S, Gabrielli O. The first prebiotics in humans: human milk oligosaccharides. J Clin Gastroenterol (2004) 38:S80–3. doi: 10.1097/01.mcg.0000128926.14285.25
130. Boehm G, Stahl B. Oligosaccharides from milk. J Nutr (2007) 137:847S–9S. doi: 10.1093/jn/137.3.847S
131. LoCascio RG, Ninonuevo MR, Freeman SL, Sela DA, Grimm R, Lebrilla CB, et al. Glycoprofiling of bifidobacterial consumption of human milk oligosaccharides demonstrates strain specific, preferential consumption of small chain glycans secreted in early human lactation. J Agric Food Chem (2007) 55:8914–9. doi: 10.1021/jf0710480
132. Karav S. Application of a novel endo-β-N-acetylglucosaminidase to isolate an entirely new class of bioactive compounds: N-glycans. In: Enzymes in food biotechnology. (Cambridge, MA: Elsevier, Academic Press), (2019) p. 389–404. doi: 10.1016/B978-0-12-813280-7.00022-0
133. Karav S, Casaburi G, Arslan A, Kaplan M, Sucu B, Frese S. N-glycans from human milk glycoproteins are selectively released by an infant gut symbiont in vivo. J Funct Foods (2019) 61:103485. doi: 10.1016/j.jff.2019.103485
134. Kaplan M, Şahutoğlu AS, Sarıtaş S, Duman H, Arslan A, Pekdemir B, et al. Role of milk glycome in prevention, treatment, and recovery of COVID-19. Front Nutr (2022) 9:1033779. doi: 10.3389/fnut.2022.1033779
135. Karav S, Le Parc A, de Moura JMLN, Frese SA, Kirmiz N, Block DE, et al. Oligosaccharides released from milk glycoproteins are selective growth substrates for infant-associated bifidobacteria. Appl Environ Microbiol (2016) 82:3622–30. doi: 10.1128/AEM.00547-16
136. Andersson B, Porras O, Hanson L, Lagergård T, Svanborg-Eden C. Inhibition of attachment of streptococcus pneumoniae and haemophilus influenza by human milk and receptor oligosaccharides. J Infect Dis (1986) 153:232–7. doi: 10.1093/infdis/153.2.232
137. Korhonen TK, Valtonen MV, Parkkinen J, Väisänen-Rhen V, Finne J, Ørskov F, et al. Serotypes, hemolysin production, and receptor recognition of Escherichia coli strains associated with neonatal sepsis and meningitis. Infect Immun (1985) 48:486–91. doi: 10.1128/iai.48.2.486-491.1985
138. Rolsma MD, Kuhlenschmidt TB, Gelberg HB, Kuhlenschmidt MS. Structure and function of a ganglioside receptor for porcine rotavirus. J Virol (1998) 72(11):9079–91. doi: 10.1128/jvi.72.11.9079-9091.1998
139. Pacitti AF, Gentsch JR. Inhibition of reovirus type 3 binding to host cells by sialylated glycoproteins is mediated through the viral attachment protein. J Virol (1987) 61(5):1407–15. doi: 10.1128/jvi.61.5.1407-1415.1987
140. Simon PM, Goode PL, Mobasseri A, Zopf D. Inhibition of Helicobacter pylori binding to gastrointestinal epithelial cells by sialic acid-containing oligosaccharides. Infect Immun (1997) 65(2):750–7. doi: 10.1128/iai.65.2.750-757.1997
141. Lane JA, Mariño K, Naughton J, Kavanaugh D, Clyne M, Carrington SD, et al. Anti-infective bovine colostrum oligosaccharides: Campylobacter jejuni as a case study. Int J Food Microbiol (2012) 157:182–8. doi: 10.1016/j.ijfoodmicro.2012.04.027
142. Coppa GV, Gabrielli O, Bertino E, Zampini L, Galeazzi T, Padella L, et al. Human milk glycosaminoglycans: the state of the art and future perspectives. Ital J Pediatr (2013) 39(1):2. doi: 10.1186/1824-7288-39-2
143. Newburg DS, He Y. Neonatal gut microbiota and human milk glycans cooperate to attenuate infection and inflammation. Clin Obstet Gynecol (2015) 58:814–26. doi: 10.1097/GRF.0000000000000156
144. Kato D, Era S, Watanabe I, Arihara M, Sugiura N, Kimata K, et al. Antiviral activity of chondroitin sulphate E targeting dengue virus envelope protein. Antiviral Res (2010) 88(2):236–43. doi: 10.1016/j.antiviral.2010.09.002
145. Laquerre S, Argnani R, Anderson DB, Zucchini S, Manservigi R, Glorioso JC. Heparan sulfate proteoglycan binding by herpes simplex virus type 1 glycoproteins B and C, which differ in their contributions to virus attachment, penetration, and cell-to-cell spread. J Virol (1998) 72(7):6119–30. doi: 10.1128/jvi.72.7.6119-6130.1998
146. Ghezzi S, Cooper L, Rubio A, Pagani I, Capobianchi MR, Ippolito G, et al. Heparin prevents Zika virus induced-cytopathic effects in human neural progenitor cells. Antiviral Res (2017) 140:13–7. doi: 10.1016/j.antiviral.2016.12.023
147. Vicenzi E, Canducci F, Pinna D, Mancini N, Carletti S, Lazzarin A, et al. Coronaviridae and SARS-associated coronavirus strain HSR1. Emerg Infect Dis (2004) 10(3):413–8. doi: 10.3201/eid1003.030683
148. Kwon PS, Oh H, Kwon SJ, Jin W, Zhang F, Fraser K, et al. Sulfated polysaccharides effectively inhibit SARS-CoV-2 in vitro. Cell Discovery (2020) 60(1):50. doi: 10.1038/s41421-020-00192-8
149. Argov N, Lemay DG, German JB. Milk fat globule structure and function: nanoscience comes to milk production. Trends Food Sci Technol (2008) 19:617–23. doi: 10.1016/j.tifs.2008.07.006
150. Fong BY, Norris CS, MacGibbon AKH. Protein and lipid composition of bovine milk-fat-globule membrane. Int Dairy J (2007) 17:275–88. doi: 10.1016/j.idairyj.2006.05.004
151. Ji X, Li X, Ma Y, Li D. Differences in proteomic profiles of milk fat globule membrane in yak and cow milk. Food Chem (2017) 221:1822–7. doi: 10.1016/j.foodchem.2016.10.097
152. Bhinder G, Allaire JM, Garcia C, Lau JT, Chan JM, Ryz NR, et al. Milk fat globule membrane supplementation in formula modulates the neonatal gut microbiome and normalizes intestinal development. Sci Rep (2017) 7:45274. doi: 10.1038/srep45274
153. Rasmussen JT. Bioactivity of milk fat globule membrane proteins. Aust J Dairy Technol (2009) 64:63–7.
154. Salcedo J, Karav S, Le Parc A, Cohen JL, de Moura Bell JMLN, Sun A, et al. Application of industrial treatments to donor human milk: influence of pasteurization treatments, storage temperature, and time on human milk gangliosides. NPJ Sci Food (2018) 2:5. doi: 10.1038/s41538-018-0013-9
155. Honan MC, Fahey MJ, Fischer-Tlustos AJ, Steele MA, Greenwood SL. Shifts in the Holstein dairy cow milk fat globule membrane proteome that occur during the first week of lactation are affected by parity. J Anim Sci Biotechnol (2020) 11:1–15. doi: 10.1186/s40104-020-00478-7
156. Yolken RH, Peterson JA, Vonderfecht SL, Fouts ET, Midthun K, Newburg DS. Human milk mucin inhibits rotavirus replication and prevents experimental gastroenteritis. J Clin Invest (1992) 90:1984. doi: 10.1172/JCI116078
157. Martin HM, Hancock JT, Salisbury V, Harrison R. Role of xanthine oxidoreductase as an antimicrobial agent. Infect Immun (2004) 72:4933–9. doi: 10.1128/IAI.72.9.4933-4939.2004
158. Vorbach C, Harrison R, Capecchi MR. Xanthine oxidoreductase is central to the evolution and function of the innate immune system. Trends Immunol (2003) 24:512–7. doi: 10.1016/S1471-4906(03)00237-0
159. Abeler-Dörner L, Swamy M, Williams G, Hayday AC, Bas A. Butyrophilins: An emerging family of immune regulators. Trends Immunol (2012) 33:34–41. doi: 10.1016/j.it.2011.09.007
160. Arnett HA, Viney JL. Immune modulation by butyrophilins. Nat Rev Immunol (2014) 14:559–69. doi: 10.1038/nri3715
161. Timby N, Domellöf M, Lönnerdal B, Hernell O. Supplementation of infant formula with bovine milk fat globule membranes. Adv Nutr (2017) 8:351–5. doi: 10.3945/an.116.014142
162. Fuller KL, Kuhlenschmidt TB, Kuhlenschmidt MS, Jiménez-Flores R, Donovan SM. Milk fat globule membrane isolated from buttermilk or whey cream and their lipid components inhibit infectivity of rotavirus in vitro. J Dairy Sci (2013) 96:3488–97. doi: 10.3168/jds.2012-6122
163. Parrón JA, Ripollés D, Pérez MD, Calvo M, Rasmussen JT, Sánchez L. Antirotaviral activity of bovine and ovine dairy byproducts. J Agric Food Chem (2017) 65:4280–8. doi: 10.1021/acs.jafc.7b01059
164. Perdijk O, van Splunter M, Savelkoul HFJ, Brugman S, van Neerven RJJ. Cow’s milk and immune function in the respiratory tract: potential mechanisms. Front Immunol (2018) 9:143. doi: 10.3389/fimmu.2018.00143
165. Jee J, Hoet AE, Azevedo MP, Vlasova AN, Loerch SC, Pickworth CL, et al. Effects of dietary vitamin A content on antibody responses of feedlot calves inoculated intramuscularly with an inactivated bovine coronavirus vaccine. Am J Vet Res (2013) 74(10):1353–62. doi: 10.2460/ajvr.74.10.1353
166. Laplana M, Royo JL, Fibla J. Vitamin D Receptor polymorphisms and risk of enveloped virus infection: A meta-analysis. Gene (2018) 678:384–94. doi: 10.1016/j.gene.2018.08.017
167. Teymoori-Rad M, Shokri F, Salimi V, Marashi SM. The interplay between vitamin D and viral infections. Rev Med Virol (2019) 29(2):e2032. doi: 10.1002/rmv.2032
168. Ali N. Role of vitamin D in preventing of COVID-19 infection, progression and severity. J Infect Public Health (2020) 13:1373–80. doi: 10.1016/j.jiph.2020.06.021
169. Grant WB, Lahore H, McDonnell SL, Baggerly CA, French CB, Aliano JL, et al. Evidence that vitamin d supplementation could reduce risk of influenza and covid-19 infections and deaths. Nutrients (2020) 12:1–19. doi: 10.3390/nu12040988
170. Balla M, Merugu GP, Konala VM, Sangani V, Kondakindi H, Pokal M, et al. Back to basics: review on vitamin D and respiratory viral infections including COVID-19. J Community Hosp Intern Med Perspect (2020) 10(6):529–36. doi: 10.1080/20009666.2020.1811074
171. Gulcin İ. Antioxidants and antioxidant methods: an updated overview. Arch Toxicol (2020) 94(3):651–715. doi: 10.1007/s00204-020-02689-3
172. Chernyak BV, Popova EN, Prikhodko AS, Grebenchikov OA, Zinovkina LA, Zinovkin RA. COVID-19 and oxidative stress. Biochem (2020) 85(12-13):1543–53. doi: 10.1134/S0006297920120068
173. Ashrafizadeh M, Mohammadinejad R, Tavakol S, Ahmadi Z, Roomiani S, Katebi M. Autophagy, anoikis, ferroptosis, necroptosis, and endoplasmic reticulum stress: Potential applications in melanoma therapy. J Cell Physiol (2019) 234(11):19471–9. doi: 10.1002/jcp.28740
174. Ali AM, Kunugi H, Abdelmageed HA, Mandour AS, Ahmed ME, Ahmad S, et al. Vitamin k in covid-19—potential anti-covid-19 properties of fermented milk fortified with bee honey as a natural source of vitamin k and probiotics. Fermentation (2021) 7(4):202. doi: 10.3390/fermentation7040202
175. Anastasi E, Ialongo C, Labriola R, Ferraguti G, Lucarelli M, Angeloni A. Vitamin K deficiency and covid-19. Scand J Clin Lab Invest (2020) 80:525–7. doi: 10.1080/00365513.2020.1805122
176. Kandeel M, Al-Nazawi M. Virtual screening and repurposing of FDA approved drugs against COVID-19 main protease. Life Sci (2020) 251:117627. doi: 10.1016/j.lfs.2020.117627
177. dos Santos LMJ. Can vitamin B12 be an adjuvant to COVID-19 treatment? GSC Biol Pharm Sci (2020) 11(3):001–5. doi: 10.30574/gscbps.2020.11.3.0155
178. Carr A, Maggini S. Vitamin C and immune function. Nutrients (2017) 9:1211. doi: 10.3390/nu9111211
179. Singh P, Hernandez-Rauda R, Peña-Rodas O. Preventative and therapeutic potential of animal milk components against COVID-19: A comprehensive review. Food Sci Nutr (2023) 11:2547–79. doi: 10.1002/fsn3.3314
180. Bailey RL, Gahche JJ, Lentino CV, Dwyer JT, Engel JS, Thomas PR, et al. Dietary supplement use in the United States, 2003-2006. J Nutr (2011) 141(2):261–6. doi: 10.3945/jn.110.133025
181. di Filippo L, Doga M, Giustina A. Hypocalcemia in COVID-19: Prevalence, clinical significance and therapeutic implications. Rev Endocr Metab Disord (2022) 23(2):299–308. doi: 10.1007/s11154-021-09655-z
182. Sun JK, Zhang WH, Zou L, Liu Y, Li JJ, Kan XH, et al. Serum calcium as a biomarker of clinical severity and prognosis in patients with coronavirus disease 2019. Aging (Albany NY) (2020) 12(12):11287–95. doi: 10.18632/aging.103526
183. Berger RCM, Vassallo PF, De Oliveira Crajoinas R, Oliveira ML, Martins FL, Nogueira BV, et al. Renal effects and underlying molecular mechanisms of long-term salt content diets in spontaneously hypertensive rats. PloS One (2015) 10(10):e0141288. doi: 10.1371/journal.pone.0141288
184. Berni A, Malandrino D, Parenti G, Maggi M, Poggesi L, Peri A. Hyponatremia, IL-6, and SARS-CoV-2 (COVID-19) infection: may all fit together? J Endocrinol Invest (2020) 43:1137–9. doi: 10.1007/s40618-020-01301-w
185. Post A, Dullaart RPF, Bakker SJL. Is low sodium intake a risk factor for severe and fatal COVID-19 infection? Eur J Intern Med (2020) 75:109. doi: 10.1016/j.ejim.2020.04.003
186. Fooladi S, Matin S, Mahmoodpoor A. Copper as a potential adjunct therapy for critically ill COVID-19 patients. Clin Nutr ESPEN (2020) 40:90–1. doi: 10.1016/j.clnesp.2020.09.022
187. Raha S, Mallick R, Basak S, Duttaroy AK. Is copper beneficial for COVID-19 patients? Med Hypotheses (2020) 142:109814. doi: 10.1016/j.mehy.2020.109814
188. Hopkins RG, Failla ML. Copper deficiency reduces interleukin-2 (IL-2) production and IL-2 mRNA in human T-lymphocytes. J Nutr (1997) 127(2):257–62. doi: 10.1093/jn/127.2.257
189. Rani I, Goyal A, Bhatnagar M, Manhas S, Goel P, Pal A, et al. Potential molecular mechanisms of zinc- and copper-mediated antiviral activity on COVID-19. Nutr Res (2021) 92:109–28. doi: 10.1016/j.nutres.2021.05.008
190. Cleminson JS, Zalewski SP, Embleton ND. Nutrition in the preterm infant: What’s new? Curr Opin Clin Nutr Metab Care (2016) 19(3):220–5. doi: 10.1097/MCO.0000000000000270
191. Thapa BR. Health factors in colostrum. Indian J Pediatr (2005) 72:579–81. doi: 10.1007/BF02724182
192. Grief SN. Upper respiratory infections. Prim Care - Clin Off Pract (2013) 40:757–70. doi: 10.1016/j.pop.2013.06.004
193. Gleeson M, Pyne DB. Respiratory inflammation and infections in high-performance athletes. Immunol Cell Biol (2016) 94:124–31. doi: 10.1038/icb.2015.100
194. Brinkworth GD, Buckley JD. Concentrated bovine colostrum protein supplementation reduces the incidence of self-reported symptoms of upper respiratory tract infection in adult males. Eur J Nutr (2003) 42:228–32. doi: 10.1007/s00394-003-0410-x
195. Patel K, Rana R. Pedimune in recurrent respiratory infection and diarrhoea- The Indian experience-the pride study. Indian J Pediatr (2006) 73:585–91. doi: 10.1007/BF02759923
196. Saad K, Abo-Elela MGM, El-Baseer KAA, Ahmed AE, Ahmad FA, Tawfeek MSK, et al. Effects of bovine colostrum on recurrent respiratory tract infections and diarrhea in children. Med (United States) (2016) 95:4–8. doi: 10.1097/MD.0000000000004560
197. Patiroğlu T, Kondolot M. The effect of bovine colostrum on viral upper respiratory tract infections in children with immunoglobulin A deficiency. Clin Respir J (2013) 7:21–6. doi: 10.1111/j.1752-699X.2011.00268.x
198. Alsayed A, Al-Doori A, Al-Dulaimi A, Alnaseri A, Abuhashish J, Aliasin K, et al. Influences of bovine colostrum on nasal swab microbiome and viral upper respiratory tract infections – A case report. Respir Med Case Rep (2020) 31:101189. doi: 10.1016/j.rmcr.2020.101189
199. Crooks C, Cross ML, Wall C, Ali A. Effect of bovine colostrum supplementation on respiratory tract mucosal defenses in swimmers. Int J Sport Nutr Exerc Metab (2010) 20:224–35. doi: 10.1123/ijsnem.20.3.224
200. Baśkiewicz-Hałasa M, Stachowska E, Grochans E, Maciejewska-Markiewicz D, Bühner L, Skonieczna-Żydecka K, et al. Moderate dose bovine colostrum supplementation in prevention of upper respiratory tract infections in medical university students: A randomized, triple blind, placebo-controlled trial. Nutrients (2023) 15(8):1925. doi: 10.3390/nu15081925
201. Mann JK, Reddy T, Van Der Stok M, Ngubane A, Mchunu N, Nevhungoni P, et al. Hen egg white bovine colostrum supplement reduces symptoms of mild/moderate COVID-19 : a randomized control trial. Future Sci OA (2023) 9(8). doi: 10.2144/fsoa-2023-0024
202. Kaducu FO, Okia SA, Upenytho G, Elfstrand L, Florén CH. Effect of bovine colostrum-based food supplement in the treatment of HIV-associated diarrhea in Northern Uganda: A randomized controlled trial. Indian J Gastroenterol (2011) 30(6):270–6. doi: 10.1007/s12664-011-0146-0
Keywords: bovine colostrum, bioactive compound, immunomodulation, SARS-CoV-2, COVID-19, antiviral activity, health benefits
Citation: Duman H and Karav S (2023) Bovine colostrum and its potential contributions for treatment and prevention of COVID-19. Front. Immunol. 14:1214514. doi: 10.3389/fimmu.2023.1214514
Received: 29 April 2023; Accepted: 27 September 2023;
Published: 16 October 2023.
Edited by:
Ning Wang, The University of Hong Kong, ChinaReviewed by:
Keith R. Martin, University of Memphis, United StatesPriyanka Verma, University of Michigan, United States
Engy Elekhnawy, Tanta University, Egypt
Copyright © 2023 Duman and Karav. This is an open-access article distributed under the terms of the Creative Commons Attribution License (CC BY). The use, distribution or reproduction in other forums is permitted, provided the original author(s) and the copyright owner(s) are credited and that the original publication in this journal is cited, in accordance with accepted academic practice. No use, distribution or reproduction is permitted which does not comply with these terms.
*Correspondence: Sercan Karav, sercankarav@comu.edu.tr