- 1Institute of Preventive Veterinary Medicine, Sichuan Agricultural University, Chengdu, Sichuan, China
- 2Key Laboratory of Animal Disease and Human Health of Sichuan Province, Sichuan Agricultural University, Chengdu, Sichuan, China
- 3Avian Disease Research Center, College of Veterinary Medicine, Sichuan Agricultural University, Chengdu, Sichuan, China
Upon infection, the herpes viruses create a cellular environment suitable for survival, but innate immunity plays a vital role in cellular resistance to viral infection. The UL13 protein of herpesviruses is conserved among all herpesviruses and is a serine/threonine protein kinase, which plays a vital role in escaping innate immunity and promoting viral replication. On the one hand, it can target various immune signaling pathways in vivo, such as the cGAS-STING pathway and the NF-κB pathway. On the other hand, it phosphorylates regulatory many cellular and viral proteins for promoting the lytic cycle. This paper reviews the research progress of the conserved herpesvirus protein kinase UL13 in immune escape and viral replication to provide a basis for elucidating the pathogenic mechanism of herpesviruses, as well as providing insights into the potential means of immune escape and viral replication of other herpesviruses that have not yet resolved the function of it.
Introduction
Herpes virus is a virus of double-stranded DNA that can be divided into three subfamilies: α-, β-, and γ-herpesvirus. For instance, Herpes simplex virus type 1/2 (HSV1/2), Varicella-zoster virus (VZV), Pseudorabies virus (PRV), Epstein-Barr virus (EBV), Human cytomegalovirus (HCMV), Kaposi’s sarcoma-associated herpesvirus (KSHV), Murine gamma-herpesvirus 68(MHV-68), Marek’s disease virus (MDV) and Duck plague virus (DPV) (1–3). Herpes virus infections severely impact the health of humans and animals. The host’s innate immune system is the first line of defense against invading pathogens, it relies on the mutual recognition of various pathogen recognition receptors (PRR) and pathogen-associated molecular patterns (PAMP) on the surface of the pathogenic organism. The interaction between PRR and PAMP on the surface of pathogenic organisms induces the production of Type I interferon (IFN-I) and other antiviral factors, promoting cellular antiviral immunity and activating the corresponding immune system (4). cyclic GMP-AMP synthase (cGAS) is a nucleotidyltransferase, as a member of the PRR family, which is activated by binding viral double-stranded DNA to induce the production of IFN-ß (5, 6). The nuclear factor kappa-B (NF-κB) regulates the production of inflammatory and immune responses to protect the host from pathogens (7, 8). Similarly, the JAK-STAT signaling pathway, the PKR-eIF2α signaling pathway, the Sterile alpha motif and HD domain-containing protein 1 (SAMHD1), and the CD8+ T cell play a critical role in antiviral response.
The HSV pUL13 and its homologs (e.g., EBV pBGLF4, HCMV pUL97, KSHV pORF36, MHV-68 pORF36, and VZV pORF47) are serine/threonine protein kinase belonging to the conserved herpesvirus protein kinase family (CHPK), which is a tegument protein of herpes virions (9–11). Their catalytic core consists of 12 conserved subdomains (12–15) (Table 1), which can catalyze the transfer of the γ-phosphate of a nucleoside triphosphate to amino acid residues of protein substrates to affect their function. CHPK from different herpesvirus subfamilies has considerable amino acid variation, and there is no consensus phosphorylation sequence for all CHPKs (16–18). Moreover, the herpesvirus protein kinases have very low homology with known cell kinases.
With the continuous discovery of UL13 protein kinase substrates (Table 2), pUL13 has been shown to play an important role in the physiological activity of the herpes virus. For example, VZV pORF47 and KSHV pORF36 are essential for virus proliferation in T and B cells (19–21); PRV pUL13 affects IFN- β by inhibiting zinc finger CCHC-type containing protein 3 (ZCCHC3) expression (22); EBV pBGLF4 is a regulator of the EBV immune genes BCRF1 and BPLF1 (23). Moreover, HSV-2 pUL13 Ser18 was significantly crucial for the HSV-2 capacity of replication and cell-to-cell spread in U2OS cells (24); the deletion of pUL13 reduced the size and number of Viral plaques of DPV (25); CHPK of β and γ herpes viruses promotes DNA virus replication by mimicking cyclin-dependent kinases1/2 (CDK1/2) phosphorylation of cyclin (26, 27). These suggest that pUL13 plays an essential role in immune escape and viral replication of herpes viruses.
The role of pUL13 in viral evasion of innate immunity
Inhibition of the cGAS/STING pathway
The type I interferon pathway is a significant component of innate immunity and plays an essential role in the control and clearance of pathogens. Upon infection, inhibition of interferon regulatory factor 3 (IRF3) by viral infection is a critical link for the termination of the type I interferon pathway. Here, IRF3 is an essential target for pUL13 action during herpes virus infection because many studies have shown that they can phosphorylate IRF3 and inhibit IRF3 dimerization, binding to the positive regulatory domains III-I (PRDIII-I), and interaction with the CREB-binding protein and P300 protein (CBP/P300) (28–32). Meanwhile, Lin Lv et al. showed that PRV UL13 relies on its kinase active sites of Lys49 and Lys387 to target IRF3 and promote its ubiquitination for degradation by the proteasome (33) (Figure 1).
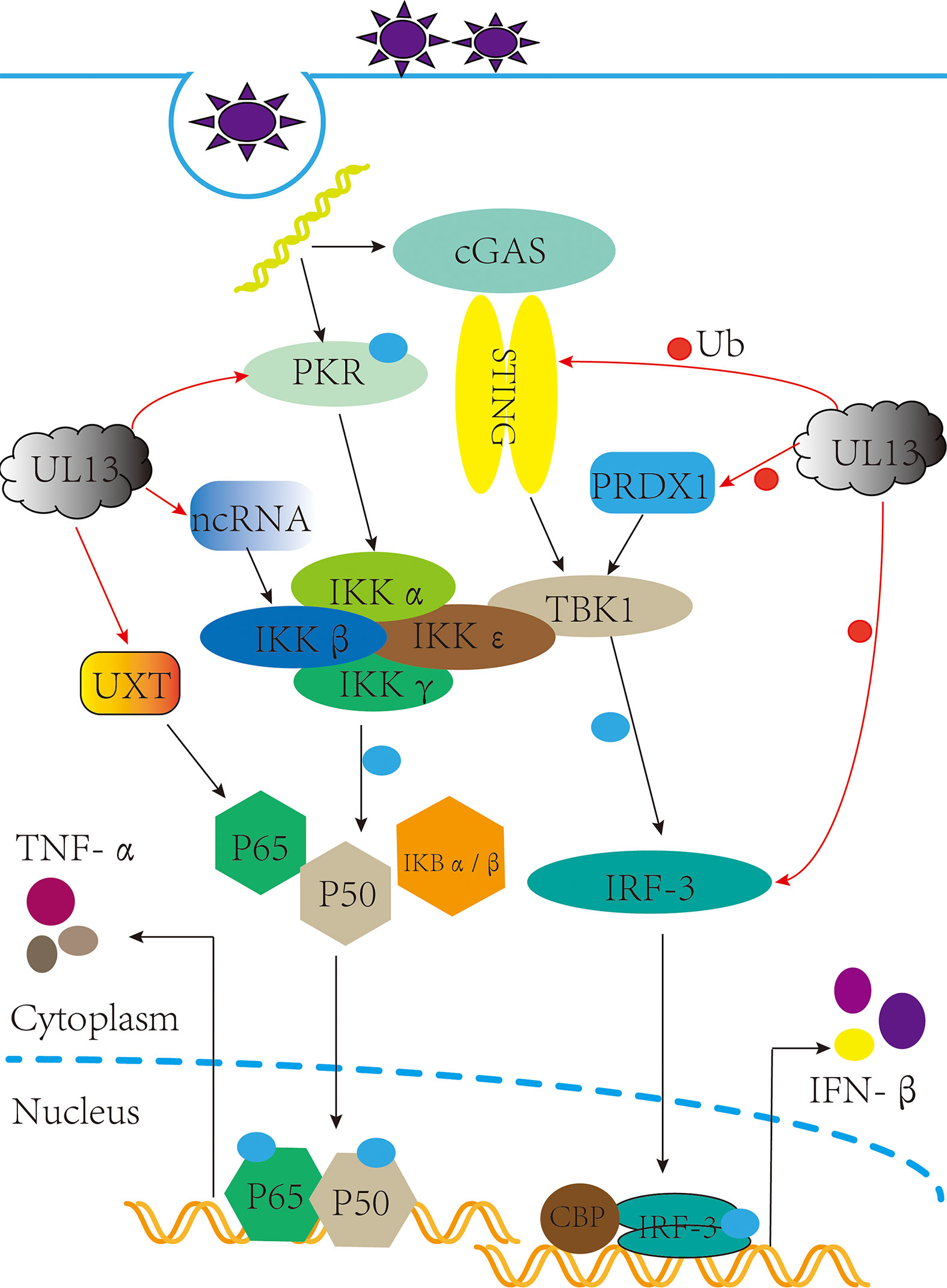
Figure 1 pUL13 inhibits the cGAS-STING signaling pathway and the NF-κB signaling pathway. pUL13 can inhibit the dimerization, nuclear translocation, CBP binding, and binding to IFN-β promoter elements of interferon regulatory factor 3 (IRF3); meanwhile, it can ubiquitinally degrade stimulator of interferon genes (STING), IRF-3, and peroxidase 1 (PRDX1) to inhibit the production of IFN-β. pUL13 indirectly inhibits the NF-κB pathway by regulating ubiquitously expressed transcript (UXT), Protein kinase R (PKR), and B2 SINE ncRNA.
In addition, pUL13 promotes the ubiquitinated degradation of immunomodulatory proteins as a necessary action affecting innate immunity. For example, PRV pUL13 recruits the E3 ligase RING-finger protein 5 (RNF5) to degrade the stimulator of interferon genes protein (STING) indirectly and also participates in ubiquitination degradation of the host protein peroxidase 1 (PRDX1) to inhibit innate immunity (34, 35) (Figure 1). Tripartite motif (TRIM) proteins play a critical role in the antiviral host response. Based on E3 ubiquitin ligases RNF5, TRIM29 and TRIM30α are responsible for the ubiquitination degradation of STING protein; TRIM18 recruit protein phosphatase 1A (PPM1A) to dephosphorylate TANK binding kinase 1 (TBK1) to suppress the innate immune response (36, 37). We believe that the TRIM family members (such as TRIM29 and TRIM18) may be essential partners of herpesvirus pUL13 in promoting the ubiquitination degradation of host immune proteins. However, there are no reports about the interaction between TRIM family members and pUL13.
Inhibition of NF-κB pathway
After virus infection, NF-κB is activated and translocated into the nucleus, which induces an inflammatory and immune response to protect the host from the pathogen (38, 39). As a vital component of the immune system, which can be regulated by the ubiquitously expressed transcript (UXT). In 2012, Chang et al. found that EBV pBGLF4 phosphorylated UXT at the Thr3, weakening interaction with p65 to inhibit NF-κB activity (40) (Figure 1). Not only did it reveal the role of the conserved herpesvirus protein kinases in evading immune clearance by NF-κB, but it also revealed its essential for promoting the lytic cycle.
Furthermore, short interspersed elements (SINEs) are non-coding retrotransposons transcribed by RNA polymerase III (RNA Pol III), which activate antiviral NF-κB signaling through a mitochondrial antiviral signaling protein (MAVS)-dependent and independent mechanism pathways (41). However, MHV-68 infection can sustainably induce transcription of SINE ncRNA, which is explained by Xiaonan Dong et al.: Inducing phosphorylation degradation of the RelA/p65 subunit of NF-κB in the pre-MHV-68 infection period to blunt the NF-κB transcription response, it is associated with IKKβ kinase (42). In 2020, Aaron M Schaller et al. reported that CHPKs-mediated chromatin modification changes contribute to activating B2 SINEs during MHV68 infection; hijacking uses B2 SINE RNA signal to activate IKKβ kinase and phosphorylates transcription initiation factor Rta to promote viral replication (43) (Figure 1). Much more interesting is that the activated SINE ncRNA can directly interact with RNA pol II to participate in the transcriptional suppression of genes (44, 45). By and large, the B2 SINEs seem to do more harm than good for viral replication. Nevertheless, the herpes virus pUL13 chose it, demonstrating that B2 SINEs have many potential mechanisms to be developed in the life cycle of herpes viruses.
Inhibition of the JAK/STAT signaling pathway
JAK/STAT acts as an inflammatory signaling pathway for stress and has immunomodulatory effects, receiving multiple cytokine signals from cells, such as IFN-α and IFN-γ (46, 47). In 2017, Yuka Sato et al. reported that pUL13 could phosphorylate the associated constitutive transcription factor SP1 (SP1) to induce suppressor of cytokine signaling 3(SOCS3) production, which regulates the JAK/STAT signaling pathway negatively (48) (Figure 2). That is SP1 can combine with GC-rich regions of the SOCS3 promoter to facilitate transcription and translation of SOCS3 (49, 50), and then curb the JAK/STAT signal pathway (51–53). Moreover, the phosphorylation of Sp1 by pUL13 could specifically induce the transcription of the immediate-early and early genes expression of the herpes virus (54–57), which reveals the importance of pUL13 for transcriptional regulation of herpesvirus genes.
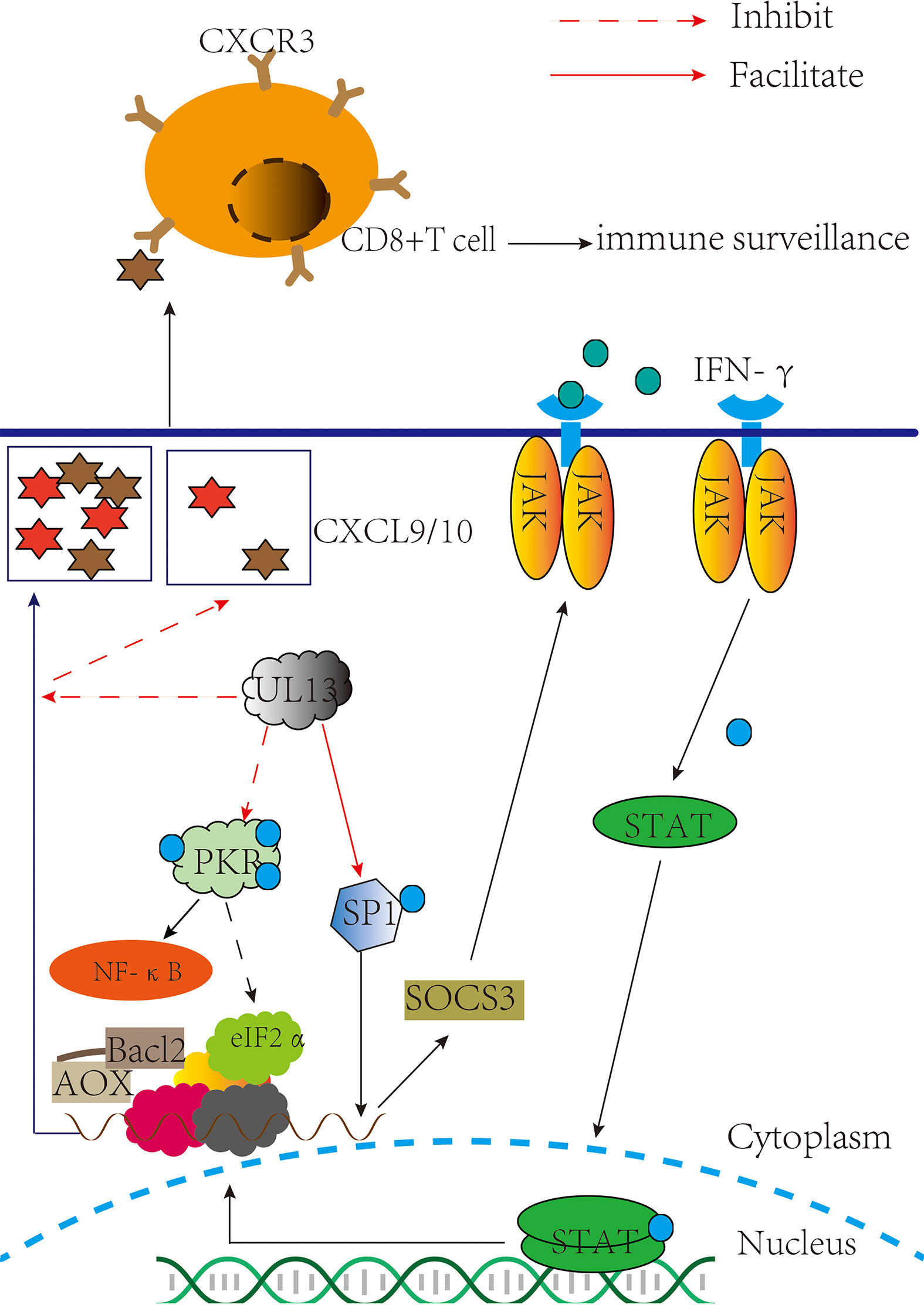
Figure 2 pUL13 inhibits PKR, CD8+ T cells, and the JAK-STAT signaling pathway to evade innate immunity. pUL13 inhibits PKR phosphorylation to evade innate immunity and promotes viral protein translation with eukaryotic initiation factor 2α (eIF2α); pUL13 phosphorylates the associated constitutive transcription factor SP1 (SP1), which induces suppressor of cytokine signaling 3 (SOCS3) expression to inhibit the JAK-STAT signaling pathway; and pUL13 can down-regulate the C-X-C motif chemokine ligand 9 (CXCL9) signaling molecules to prevent cd8+ T cell molecules from clustering at the site of infection.
SOCS3 plays a significant role in modulating the outcome of infections and autoimmune diseases. And many viruses, such as HSV- 1, EBV, and VZV (58–61), can activate the expression of SOCS3 because of the close relationship between SOCS3 and JAK kinase with STAT signaling factors (62–65). It was suggested that SOCS3 is induced that not only inhibits the antiviral response of the JAK-STAT signal pathway but also maintains immune homeostasis in the body under pathological conditions and physiological conditions (66), such as the expression of SOCS3 inhibits several NF-κB-regulated proapoptotic pathways to protect β-cells from IL- 1 β-mediated apoptosis (67). Perhaps this is more important for the production of SOCS3 induced by pUL13 during herpesvirus infection.
Effect on PKR-eIF2α-mediated antiviral effects
Protein kinase R (PKR) in host cells exerts antiviral effects by inhibiting viral mRNA translation and inducing apoptosis. Many data indicate PKR promotes NF-κB activation (68–73), promotes mRNA stability of IFN-β (74), and is involved in the tumor suppressor function of p53 protein (75–77). When dsRNA binds to the Conserved double-stranded RNA binding motif (dsRBMs) of PKR, it is activated by autophosphorylation at Thr446 (78). Next, it phosphorylates Ser 51 of eukaryotic initiation factor 2α (eIF2α) and inhibits the translation initiation activity of mRNAs which encode antiviral factors and mediate stress responses (79, 80). In the PKR-eIF2a pathway, PKR inhibition and eIF2a dephosphorylation must be used to achieve massive replication of the virus, so the virus has evolved a variety of strategies in regulating the PKR-eIF2a pathway: controlling dsRNA masking and degradation (81–84), PKR degradation (85), inhibiting PKR dimerization and autophosphorylation (86–89), dephosphorylation of eIF2α (90–92), and PKR desensitization (93, 94). In 2020, Rosamaria Pennisi et al. demonstrated that HSV-1 pUL13 inhibits the phosphorylation of cellular PKR. Although the specific pathway by which pUL13 inhibits PKR phosphorylation cannot be demonstrated (95) (Figure 2). These suggest that pUL13 inhibition of PKR can not only evade innate immunity and prevent PKR-mediated apoptosis but also use eIF2α to promote viral mRNA translation.
Especially, PKR is one of four kinases that integrate stress responses. It regulates the protein homeostasis of the cell to maintain the body’s homeostasis; conversely, its abnormal activation can cause severe damage to the body, such as systemic lupus erythematosus (96, 97). Based on these results, whether the molecular mechanism of pUL13 inhibition of PKR can inspire treating diseases associated with abnormal activation of PKR remains to be further studied.
Effect on CD8+ T cells mediated antiviral effects
Compared with pICP47 and pUS3 recognizing the main histocompatibility complex (MHC I) that are distributed on the cell surface and presentation of antigen peptides to T cells to exert cellular immune clearance regulation, the effect of pUL13 on it is not apparent (98–101). However, HSV- 1 pUL13 triggered viral encephalitis in mice by downregulating CXCL-9 and inhibiting the infiltration of CD8+ T cell molecules at the site of infection (102) (Figure 2). The author also points out that the HSV-1 pUL13- mediated immune evasion mechanism might be specific to the CNS. Maybe it associated with CXCL-9/10 and CD8+ T cells inhibiting the reactivation of HSV within nerve cells, further suggesting the role of pUL13 in the latent reactivation of the herpes virus (103–106). Although the molecular mechanisms underlying the downregulation of CXCL-9 by pUL13 are unclear, it is suggested that inhibition of pUL13 has a potential effect in treating encephalitis of the central nervous system caused by HSV-1 infection (107–110).
Inhibition of SAMHD1
SAMHD1 is an antiviral host limiting factor (111–116), and the virus has adopted a variety of strategies to inhibit its dNTP enzyme activity, such as HIV-2 and SIV virus-encoded Vpx proteins, to induce SAMHD1 degradation and promote self-replication (117–120); Ribonucleotide reductase (RNR) (121) and thymidine kinase (TK) (122, 123) encoded by DNA viruses can antagonize SAMHD1’s dNTP enzyme activity, providing the necessary substrate for viral DNA polymerase; The intracellular CyclinA2/CDK1/CDK2 complex regulates phosphorylation of SAMHD1 Thr592 (124), and phosphorylation of Thr592 has been shown to reduce SAMHD1 antiviral activity (125), echoing IFN-I-induced dephosphorylation of SAMHD1 Thr592 (126). It has been also reported that pUL13 of the β and γ herpes virus participates in phosphorylation of SAMHD1 T592, inhibiting the dNTP enzyme activity of SAMHD1 from ensuring adequate intracellular levels of dNTPs for viral replication (127, 128) (Figure 3).
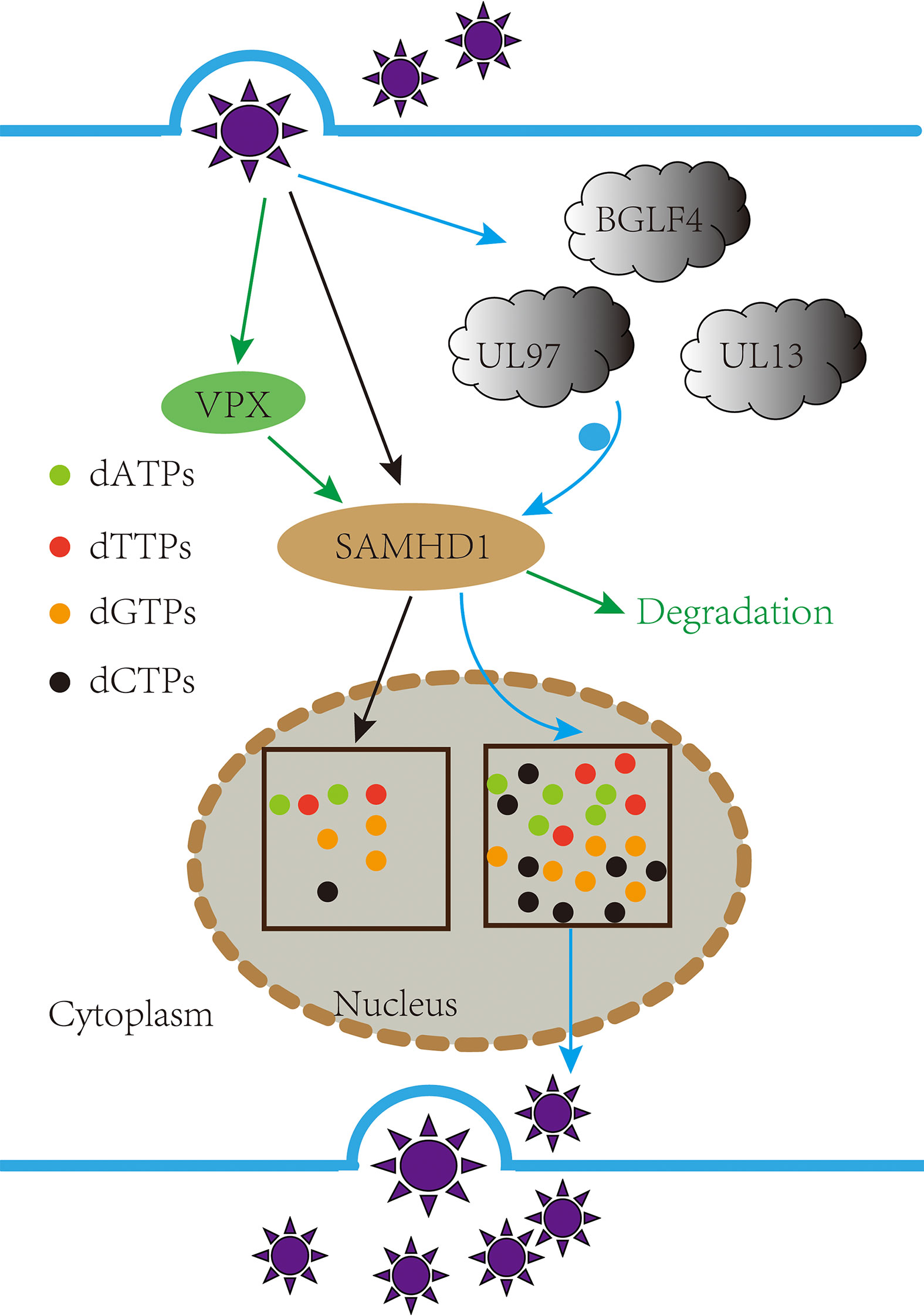
Figure 3 pUL13 phosphorylated the antiviral factor SAMDH1 Thr592 to promote viral replication. Inhibiting the dNTP enzyme activity of the sterile alpha motif and HD domain-containing protein 1 (SAMHD1) from ensuring adequate intracellular levels of dNTPs for viral replication.
SAMHD1 can inhibit the excessive immune and inflammatory response, possibly proving why VZV and KSHV proliferate in lymphocytes requiring pORF47 and pORF36 (129). However, whether and how pUL13 can phosphorylate SMADH1 to coordinate the immune and inflammatory response remains to be studied.
It is revealed here that pUL13 plays an essential role in inhibiting various antiviral factors from escaping innate immunity. Additionally, pUL13 also plays an important role in viral replication, latent infection, and other critical physiological activities.
The role of pUL13 in promoting viral replication
pUL13 phosphorylates H2AX to promote viral replication
DNA-damage response (DDR) is a mechanism by which cells protect themselves through DNA damage repair and apoptosis to resist DNA damage induced by various factors (130, 131). Micah A. Luftig has discussed the interrelationship between viruses and DDR, noting that DNA viruses require DDR activation for replication (132). The research shows that the viral infection process acts on the different nodes of the DNA damage response pathway. For example, HSV-1 infections activate ataxia telangiectasia mutated (ATM) kinase activity but inhibit the role of ataxia telangiectasia- and Rad3-related protein (ATR); EBV virus infection activates upstream regulators of the DDR pathway in the DDR pathway-histone acetyltransferase TIP60 (133–135).
H2A histone family member X (H2AX) is a substrate of ATM, ATR, and DNA-dependent protein kinase catalytic subunits in phosphatidylinositol 3-kinase-like protein kinase family (PIKKs) (136–140); it is also a substrate for pUL13 (141, 142). In H2AX knockdown cells, the replication capacity of MHV-68 and KSHV are significantly abating (143, 144), and the date of EBV pBGLF4, PRV pUL13 what suggesting that pUL13 phosphorylate H2AX to activate DDR for viral replication (145, 146). Still, VZV pORF47 cannot phosphorylate H2AX and indicates the difference in the members of the CHPKs (147). An attractive hypothesis is that replication of viral DNA requires or is enhanced by the cellular DNA damage machinery (133, 148–150) (Figure 4). Generally, more evidence is needed to support whether pUL13 of the herpes virus plays a vital role in this matter.
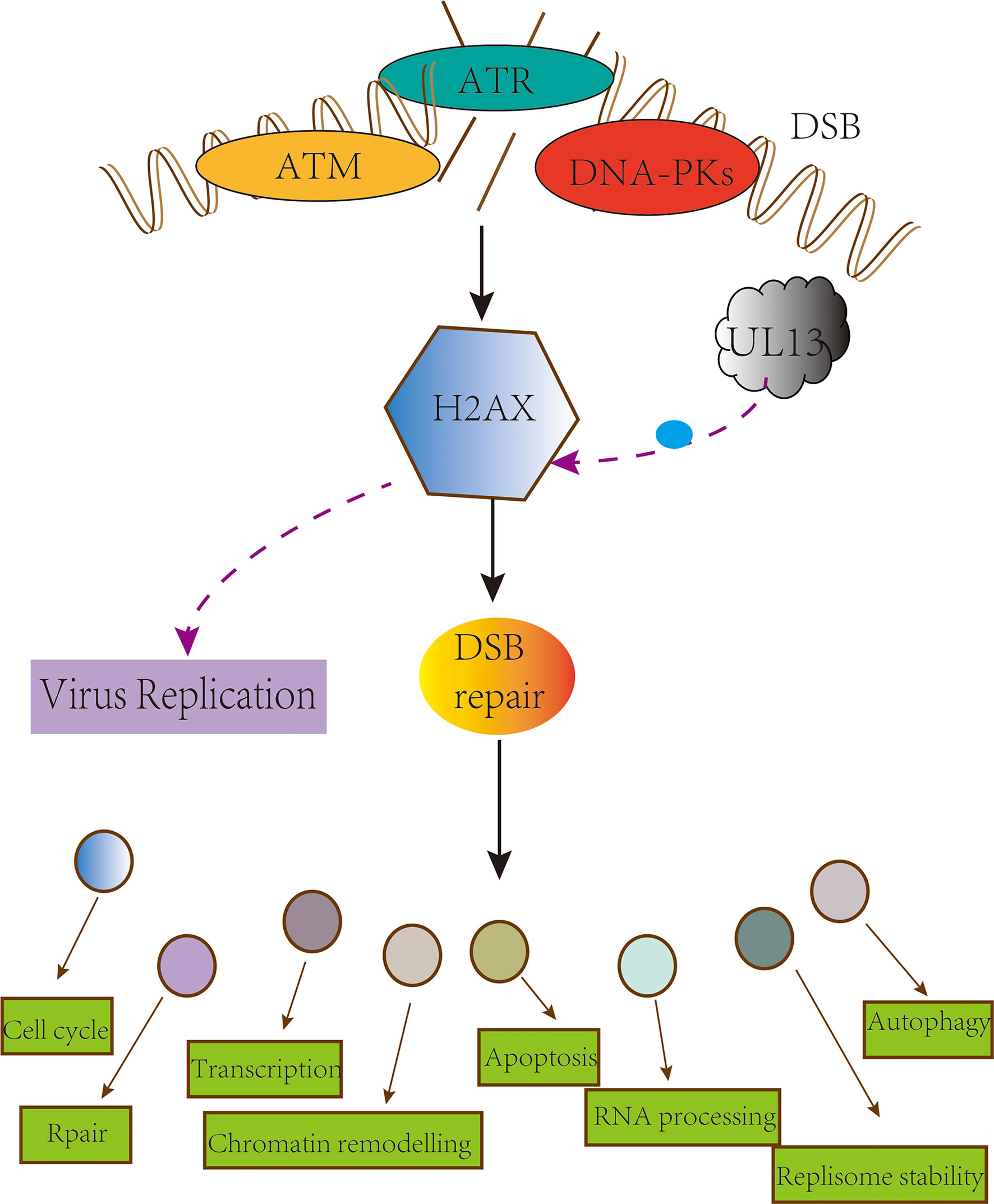
Figure 4 pUL13 phosphorylates H2AX to promote viral replication. The conserved herpesvirus Protein kinase pUL13 regulates DNA damage marker H2A histone family member X (H2AX), and pUL13-mediated H2AX phosphorylation plays a pivotal role in efficient virus replication and progeny production.
pUL13 phosphorylates EF-1б to promote viral replication
Herpesvirus pUL13 can promote host cell synthesis of proteins, such as the KSHV pORF36 mimicking cellular protein S6 kinase (S6KB1) to promote cell proliferation (151). Similarly, as a substrate of pUL13, the translation extension factor -1б (EF-1б) exists in two forms in the normal state of hypophosphorylation and hyperphosphorylation, involved in the process of mRNA translation into peptide chain extension. EF-1б is mainly present in the hyperphosphorylated form in HSV-1-infected cells. Because HSV-1 pUL13, HCMV pUL97, EBV pBGLF4, and intracellular cycle-dependent kinase cdc2 are involved in EF-1б’s hyperphosphorylation and work together on its Ser 133 (152–154). It shows that UL13 can synthesize its viral protein using EF-1б.
pUL13 works with SUMO proteins to promote viral replication
Small Ubiquitin-related Modifier (SUMO) is a post-translational modifier protein. The SUMO system is essential in herpes virus replication, such as KSHV replication and transcription activator (K-Rta) and HSV-1 ICP0 degrade SUMO-modified promyelocytic leukemia-nuclear bodies (PML-NBs) (155, 156), inhibition of the NF-κB signaling pathway (157) and participation in degradation of IRF-3 and IRF-7 (158–160). KSHV basic region-leucine zipper (K-bZIP) is a potent transcriptional repressor that binds directly to K-Rta and attenuates K-Rta-mediated trans-activation activity, relying on SUMO modifications to regulate viral and host gene expression (161, 162). Studies have shown that KSHV ORF36 phosphorylates Thr111 of K-bZIP and inhibits the SUMO level of bZIP, causing a decrease in transcriptional inhibition activity (163) (Figure 5), and appears to cooperate with K-Rta inhibition of K-bZIP to promote viral transcriptional expression (164); Also involved in the phosphorylation of the cell chromatin remodeling molecule KAP-1 inhibits SUMO level and thus inhibits chromosomal remodeling capacity (165). It is also reflected in the EBV pBGLF4 negatively regulating SUMO-modified Zta to promote the establishment of viral latency (166, 167). It suggests that although the protein kinase of the γ-herpes virus cannot be modified by SUMO, its phosphorylation and SUMO can cooperate to promote viral replication.
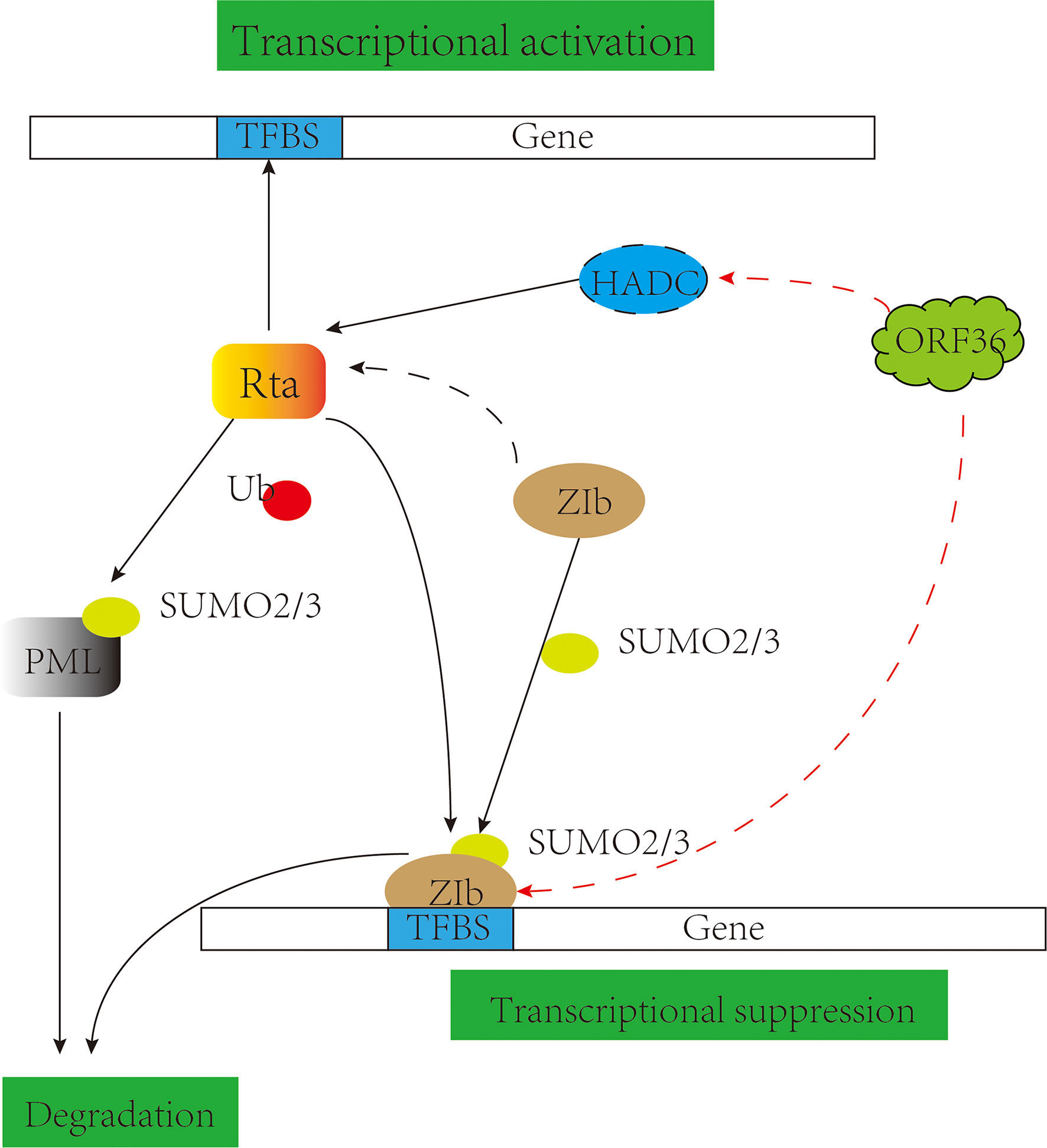
Figure 5 pUL13 works with SUMO proteins to promote viral replication. Gammaherpesvirus protein kinase pORF36 interacts with histone deacetylase 1 and 2 (HADC1/2) and prevents the association of these HDACs with the viral promoter driving expression of KSHV replication and transcription activator (K-Rta). pORF36 phosphorylates Thr111 of KSHV basic region-leucine zipper (K-bZIP) and inhibits the SUMO level of K-bZIP to repress the transcriptional inhibition activity.
pUL13 promotes viral replication in conjunction with ICP22 and VP22
The interaction between herpesvirus protein kinase and viral proteins to promote its replication is a complex network, such as the interaction of KSHV pORF36 and pORF45 (168), HSV-1 pUL13 and pUL41 (169). As early as 1993, Purves reported that pUL13 phosphorylation modulated ICP22 to stabilize to increase transcription of specific subpopulations of viral RNA and accumulate corresponding viral proteins (170). Subsequently, it was found that ICP22 and pUL13 were jointly involved in phosphorylation of RNA Pol II, mediating the degradation of cyclins A and B1 and activating cdc2, in which activated cdc2 and viral DNA synthesis factor pUL42 formed a complex to recruit topoisomerase II to promote the expression of advanced genes (171–179), indicating that ICP22 and pUL13 were necessary for early gene expression of herpes virus.
In HSV-1-infected cells, UL13 protein kinase promotes the dissociation of VP22 from virions and phosphorylate VP22 (169, 180). VP22 released into cells can interact with Template-activating factor I (TAF-1) proteins and histone H4 (Histone H4), inhibit the assembly of nucleosomes on DNA and H4 histone acetylation and participate in chromatin recombination, cell cycle control, and gene regulation (181, 182). The expression of VP22 can also inhibit cGAS activity and affect natural immunity (183). It can be seen that pUL13 can promote viral replication by regulating the ICP22 and VP22 proteins and collaborating.
pUL13 is involved in multiple processes of herpes virus replication, including gene replication, transcription, and translation of viruses (184); pUL13 in herpesvirus can destroy LaminA/C to promote capsid exodus from the nucleus (185–187); assembly, maturation, and release of virions (188). It is meaningful to construct pUL13 protein interaction networks to understand better the function of UL13 protein kinases in the life cycle of the herpes virus.
The role of pUL13 in latent infection
Induction and escape of herpesvirus genomic silencing is a biological marker of the herpes virus. Many reports suggest that pUL13 may play an essential role in the latent infection of the herpes virus. Firstly, Jolien Van Cleemput’s study found that pUL13 may be indirectly involved in the latent infection reactivation of α herpesvirus by phosphorylating other cortical proteins (189); Secondly, in γ herpes virus, EBV pBGLF4 and KSHV pORF36 are closely associated with latent infection-related proteins as such Rta, Zta, the latency-associated nuclear antigen (LANA), and TAT interacting protein 60 kD (TIP60) (190–195); Lastly, MHV-68 pORF36 inhibits the antiviral effects of bone marrow-specific STAT1 expression and promotes the establishment of latent infection of MHV-68 in spleen B cells (196). In addition, herpesvirus CHPKs can also use CD8+ T cells and many host proteins (UXT, H2AX, small ubiquitin-related modification regulatory proteins) to promote the establishment of latent infections (197–199). Although the complex mechanism of establishment and reactivation of herpes virus latent infection is unknown, UL13 protein kinase will be an essential breakthrough for the follow-up study of latent infection of herpes virus.
Summary and prospect
pUL13 acts as a serine/threonine kinase encoded by the herpes virus. It is retained in the continuous natural screening of the virus and plays a vital role in the physiological activity of the herpes virus.
In terms of immune escape, to evade innate immune defense line and persist in host cells, pUL13 and its homologs directly or indirectly play a role in signaling pathways, which acts on different immunoregulatory proteins and many antiviral factors. Then pUL13 use varieties transcription factors and translation factors in host cells to assist the lytic cycle, such as EF-1б, H2AX, SP1, embodied in lacking pUL13 will lead to the weakening of the replication ability and virulence of the virus. At the same time, herpesvirus can use pUL13 to assist in the establishment and reactivation of its latent infection.
pUL13 can phosphorylate many protein targets and participate in the activation and inhibition of related protein functions. It is similar to a switch in the life cycle of the herpes virus. It is committed to building a systematic protein interaction network diagram of pUL13, which is conducive to unveiling pUL13 in the life cycle of the herpes virus.
Herpesvirus pUL13 is an important target for developing anti-herpesvirus drugs. With the initial clinical application of GCV (200), followed by the anti-herpesvirus trials of compounds such as Maribavir (201), K252A (202), ISIS 1082 (203, 204), and 17-DMAG (205), as well as the continuous innovation of UL13 gene deletion vaccine (206, 207) and immunotherapy (208–210). However, given that low homology among different CHPK members complicates the development of compounds targeting an entire group, further development of more broad-spectrum, efficient and safe herpesvirus protein kinase inhibitors for the treatment of herpesvirus is needed.
pUL13 undertakes a variety of functions in the life cycle of herpes virus, and exploring the mechanism of action of pUL13 can not only solve the problem of infection, transmission, and immune escape mechanism of herpes virus but also provide a theoretical basis for the research and development of clinical drugs for the anti-herpes virus.
Author contributions
LZ and AC contributed to the design of the manuscript. XO, DS, SM, JH, QY, YW, SC, SZ, and DZ provided ideas contributing to the conception of this manuscript. RJ, ML, X-XZ, QG, and BT helped to create the figures. MW modified the manuscript. All the authors reviewed the manuscript. All authors contributed to the article and approved the submitted version.
Funding
This work was supported by the China Agricultural Research System of MOF and MARA and Sichuan Veterinary Medicine and Drug Innovation Group of China Agricultural Research System (SCCXTD-2020-18).
Conflict of interest
The authors declare that the research was conducted in the absence of any commercial or financial relationships that could be construed as a potential conflict of interest.
Publisher’s note
All claims expressed in this article are solely those of the authors and do not necessarily represent those of their affiliated organizations, or those of the publisher, the editors and the reviewers. Any product that may be evaluated in this article, or claim that may be made by its manufacturer, is not guaranteed or endorsed by the publisher.
References
1. Qi X, Yang X, Cheng A, Wang M, Zhu D, Jia R. Quantitative analysis of virulent duck enteritis virus loads in experimentally infected ducklings. Avian Dis (2008) 52(2):338–44. doi: 10.1637/8120-100207-ResNote.1
2. Wu Y, Cheng A, Wang M, Yang Q, Zhu D, Jia R, et al. Complete genomic sequence of Chinese virulent duck enteritis virus. J Virol (2012) 86(10):5965. doi: 10.1128/jvi.00529-12
3. Jia R, Cheng A, Wang M, Qi X, Zhu D, Ge H, et al. Development and evaluation of an antigen-capture Elisa for detection of the Ul24 antigen of the duck enteritis virus, based on a polyclonal antibody against the Ul24 expression protein. J Virol Methods (2009) 161(1):38–43. doi: 10.1016/j.jviromet.2009.05.011
4. Gong T, Liu L, Jiang W, Zhou R. Damp-sensing receptors in sterile inflammation and inflammatory diseases. Nat Rev Immunol (2020) 20(2):95–112. doi: 10.1038/s41577-019-0215-7
5. Sun L, Wu J, Du F, Chen X, Chen ZJ. Cyclic gmp-amp synthase is a cytosolic DNA sensor that activates the type I interferon pathway. Science (2013) 339(6121):786–91. doi: 10.1126/science.1232458
6. Wu J, Sun L, Chen X, Du F, Shi H, Chen C, et al. Cyclic gmp-amp is an endogenous second messenger in innate immune signaling by cytosolic DNA. Science (2013) 339(6121):826–30. doi: 10.1126/science.1229963
7. Hayden MS, Ghosh S. Nf-Kb in immunobiology. Cell Res (2011) 21(2):223–44. doi: 10.1038/cr.2011.13
8. Lawrence T. The nuclear factor nf-kappab pathway in inflammation. Cold Spring Harb Perspect Biol (2009) 1(6):a001651. doi: 10.1101/cshperspect.a001651
9. Asai R, Kato A, Kato K, Kanamori-Koyama M, Sugimoto K, Sairenji T, et al. Epstein-Barr Virus protein kinase Bglf4 is a virion tegument protein that dissociates from virions in a phosphorylation-dependent process and phosphorylates the viral immediate-early protein Bzlf1. J Virol (2006) 80(11):5125–34. doi: 10.1128/jvi.02674-05
10. Overton HA, McMillan DJ, Klavinskis LS, Hope L, Ritchie AJ, Wong-kai-in P. Herpes simplex virus type 1 gene Ul13 encodes a phosphoprotein that is a component of the virion. Virology (1992) 190(1):184–92. doi: 10.1016/0042-6822(92)91204-8
11. van Zeijl M, Fairhurst J, Baum EZ, Sun L, Jones TR. The human cytomegalovirus Ul97 protein is phosphorylated and a component of virions. Virology (1997) 231(1):72–80. doi: 10.1006/viro.1997.8523
12. Hanks SK, Quinn AM, Hunter T. The protein kinase family: Conserved features and deduced phylogeny of the catalytic domains. Science (1988) 241(4861):42–52. doi: 10.1126/science.3291115
13. Hanks SK, Quinn AM. Protein kinase catalytic domain sequence database: Identification of conserved features of primary structure and classification of family members. Methods Enzymol (1991) 200:38–62. doi: 10.1016/0076-6879(91)00126-h
14. Hanks SK, Hunter T. Protein kinases 6. the eukaryotic protein kinase superfamily: Kinase (Catalytic) domain structure and classification. FASEB J (1995) 9(8):576–96. doi: 10.1096/fasebj.9.8.7768349
15. Leader DP. Viral protein kinases and protein phosphatases. Pharmacol Ther (1993) 59(3):343–89. doi: 10.1016/0163-7258(93)90075-o
16. Cano-Monreal GL, Tavis JE, Morrison LA. Substrate specificity of the herpes simplex virus type 2 Ul13 protein kinase. Virology (2008) 374(1):1–10. doi: 10.1016/j.virol.2007.11.023
17. Baek MC, Krosky PM, Coen DM. Relationship between autophosphorylation and phosphorylation of exogenous substrates by the human cytomegalovirus Ul97 protein kinase. J Virol (2002) 76(23):11943–52. doi: 10.1128/jvi.76.23.11943-11952.2002
18. Kenyon TK, Grose C. Vzv Orf47 serine protein kinase and its viral substrates. Curr Top Microbiol Immunol (2010) 342:99–111. doi: 10.1007/82_2009_5
19. Soong W, Schultz JC, Patera AC, Sommer MH, Cohen JI. Infection of human T lymphocytes with varicella-zoster virus: An analysis with viral mutants and clinical isolates. J Virol (2000) 74(4):1864–70. doi: 10.1128/jvi.74.4.1864-1870.2000
20. Besser J, Sommer MH, Zerboni L, Bagowski CP, Ito H, Moffat J, et al. Differentiation of varicella-zoster virus Orf47 protein kinase and Ie62 protein binding domains and their contributions to replication in human skin xenografts in the scid-hu mouse. J Virol (2003) 77(10):5964–74. doi: 10.1128/jvi.77.10.5964-5974.2003
21. Anders PM, Montgomery ND, Montgomery SA, Bhatt AP, Dittmer DP, Damania B. Human herpesvirus-encoded kinase induces b cell lymphomas in vivo. J Clin Invest (2018) 128(6):2519–34. doi: 10.1172/jci97053
22. Chen X, Shan T, Sun D, Zhai H, Dong S, Kong N, et al. Host zinc-finger cchc-type containing protein 3 inhibits pseudorabies virus proliferation by regulating type I interferon signaling. Gene (2022) 827:146480. doi: 10.1016/j.gene.2022.146480
23. McKenzie J, Lopez-Giraldez F, Delecluse HJ, Walsh A, El-Guindy A. The Epstein-Barr virus immunoevasins Bcrf1 and Bplf1 are expressed by a mechanism independent of the canonical late pre-initiation complex. PloS Pathog (2016) 12(11):e1006008. doi: 10.1371/journal.ppat.1006008
24. Koyanagi N, Kato A, Takeshima K, Maruzuru Y, Kozuka-Hata H, Oyama M, et al. Regulation of herpes simplex virus 2 protein kinase Ul13 by phosphorylation and its role in viral pathogenesis. J Virol (2018) 92(17):e00807-18. doi: 10.1128/jvi.00807-18
25. Hu X, Wang M, Chen S, Jia R, Zhu D, Liu M, et al. The duck enteritis virus early protein, Ul13, found in both nucleus and cytoplasm, influences viral replication in cell culture. Poult Sci (2017) 96(8):2899–907. doi: 10.3382/ps/pex043
26. Kuny CV, Chinchilla K, Culbertson MR, Kalejta RF. Cyclin-dependent kinase-like function is shared by the beta- and gamma- subset of the conserved herpesvirus protein kinases. PloS Pathog (2010) 6(9):e1001092. doi: 10.1371/journal.ppat.1001092
27. Iwahori S, Hakki M, Chou S, Kalejta RF. Molecular determinants for the inactivation of the retinoblastoma tumor suppressor by the viral cyclin-dependent kinase Ul97. J Biol Chem (2015) 290(32):19666–80. doi: 10.1074/jbc.M115.660043
28. Wang JT, Doong SL, Teng SC, Lee CP, Tsai CH, Chen MR. Epstein-Barr Virus Bglf4 kinase suppresses the interferon regulatory factor 3 signaling pathway. J Virol (2009) 83(4):1856–69. doi: 10.1128/jvi.01099-08
29. Vandevenne P, Lebrun M, El Mjiyad N, Ote I, Di Valentin E, Habraken Y, et al. The varicella-zoster virus Orf47 kinase interferes with host innate immune response by inhibiting the activation of Irf3. PloS One (2011) 6(2):e16870. doi: 10.1371/journal.pone.0016870
30. Hwang S, Kim KS, Flano E, Wu TT, Tong LM, Park AN, et al. Conserved herpesviral kinase promotes viral persistence by inhibiting the irf-3-Mediated type I interferon response. Cell Host Microbe (2009) 5(2):166–78. doi: 10.1016/j.chom.2008.12.013
31. Bo Z, Miao Y, Xi R, Zhong Q, Bao C, Chen H, et al. Prv Ul13 inhibits cgas-Sting-Mediated ifn-B production by phosphorylating Irf3. Vet Res (2020) 51(1):118. doi: 10.1186/s13567-020-00843-4
32. Escalante CR, Nistal-Villán E, Shen L, García-Sastre A, Aggarwal AK. Structure of irf-3 bound to the prdiii-I regulatory element of the human interferon-beta enhancer. Mol Cell (2007) 26(5):703–16. doi: 10.1016/j.molcel.2007.04.022
33. Lv L, Cao M, Bai J, Jin L, Wang X, Gao Y, et al. Prv-encoded Ul13 protein kinase acts as an antagonist of innate immunity by targeting Irf3-signaling pathways. Vet Microbiol (2020) 250:108860. doi: 10.1016/j.vetmic.2020.108860
34. Kong Z, Yin H, Wang F, Liu Z, Luan X, Sun L, et al. Pseudorabies virus tegument protein Ul13 recruits Rnf5 to inhibit sting-mediated antiviral immunity. PloS Pathog (2022) 18(5):e1010544. doi: 10.1371/journal.ppat.1010544
35. Lv L, Bai J, Gao Y, Jin L, Wang X, Cao M, et al. Peroxiredoxin 1 interacts with Tbk1/Ikkϵ and negatively regulates pseudorabies virus propagation by promoting innate immunity. J Virol (2021) 95(19):e0092321. doi: 10.1128/jvi.00923-21
36. Fang M, Zhang A, Du Y, Lu W, Wang J, Minze LJ, et al. Trim18 is a critical regulator of viral myocarditis and organ inflammation. J BioMed Sci (2022) 29(1):55. doi: 10.1186/s12929-022-00840-z
37. Li Q, Lin L, Tong Y, Liu Y, Mou J, Wang X, et al. Trim29 negatively controls antiviral immune response through targeting sting for degradation. Cell Discovery (2018) 4:13. doi: 10.1038/s41421-018-0010-9
38. Alexopoulou L, Holt AC, Medzhitov R, Flavell RA. Recognition of double-stranded rna and activation of nf-kappab by toll-like receptor 3. Nature (2001) 413(6857):732–8. doi: 10.1038/35099560
39. Kawai T, Akira S. Signaling to nf-kappab by toll-like receptors. Trends Mol Med (2007) 13(11):460–9. doi: 10.1016/j.molmed.2007.09.002
40. Chang LS, Wang JT, Doong SL, Lee CP, Chang CW, Tsai CH, et al. Epstein-Barr Virus Bglf4 kinase downregulates nf-Kb transactivation through phosphorylation of coactivator uxt. J Virol (2012) 86(22):12176–86. doi: 10.1128/jvi.01918-12
41. Mounce BC, Mboko WP, Bigley TM, Terhune SS, Tarakanova VL. A conserved gammaherpesvirus protein kinase targets histone deacetylases 1 and 2 to facilitate viral replication in primary macrophages. J Virol (2013) 87(13):7314–25. doi: 10.1128/jvi.02713-12
42. Dong X, Feng P. Murine gamma herpesvirus 68 hijacks mavs and ikkβ to abrogate nfκb activation and antiviral cytokine production. PloS Pathog (2011) 7(11):e1002336. doi: 10.1371/journal.ppat.1002336
43. Schaller AM, Tucker J, Willis I, Glaunsinger BA. Conserved herpesvirus kinase Orf36 activates B2 retrotransposons during murine gammaherpesvirus infection. J Virol (2020) 94(14):e00262-20. doi: 10.1128/jvi.00262-20
44. Kassube SA, Fang J, Grob P, Yakovchuk P, Goodrich JA, Nogales E. Structural insights into transcriptional repression by noncoding rnas that bind to human pol ii. J Mol Biol (2013) 425(19):3639–48. doi: 10.1016/j.jmb.2012.08.024
45. Ponicsan SL, Houel S, Old WM, Ahn NG, Goodrich JA, Kugel JF. The non-coding B2 rna binds to the DNA cleft and active-site region of rna polymerase ii. J Mol Biol (2013) 425(19):3625–38. doi: 10.1016/j.jmb.2013.01.035
46. Darnell JE Jr., Kerr IM, Stark GR. Jak-stat pathways and transcriptional activation in response to ifns and other extracellular signaling proteins. Science (1994) 264(5164):1415–21. doi: 10.1126/science.8197455
47. Stark GR, Darnell JE Jr. The jak-stat pathway at twenty. Immunity (2012) 36(4):503–14. doi: 10.1016/j.immuni.2012.03.013
48. Sato Y, Koshizuka T, Ishibashi K, Hashimoto K, Ishioka K, Ikuta K, et al. Involvement of herpes simplex virus type 1 Ul13 protein kinase in induction of socs genes, the negative regulators of cytokine signaling. Microbiol Immunol (2017) 61(5):159–67. doi: 10.1111/1348-0421.12483
49. Vizcaíno C, Mansilla S, Portugal J. Sp1 transcription factor: A long-standing target in cancer chemotherapy. Pharmacol Ther (2015) 152:111–24. doi: 10.1016/j.pharmthera.2015.05.008
50. Barclay JL, Anderson ST, Waters MJ, Curlewis JD. Socs3 as a tumor suppressor in breast cancer cells, and its regulation by prl. Int J Cancer (2009) 124(8):1756–66. doi: 10.1002/ijc.24172
51. Yokota S, Yokosawa N, Okabayashi T, Suzutani T, Miura S, Jimbow K, et al. Induction of suppressor of cytokine signaling-3 by herpes simplex virus type 1 contributes to inhibition of the interferon signaling pathway. J Virol (2004) 78(12):6282–6. doi: 10.1128/jvi.78.12.6282-6286.2004
52. Yokota S, Yokosawa N, Okabayashi T, Suzutani T, Fujii N. Induction of suppressor of cytokine signaling-3 by herpes simplex virus type 1 confers efficient viral replication. Virology (2005) 338(1):173–81. doi: 10.1016/j.virol.2005.04.028
53. Shen X, Hong F, Nguyen VA, Gao B. Il-10 attenuates ifn-Alpha-Activated Stat1 in the liver: Involvement of Socs2 and Socs3. FEBS Lett (2000) 480(2-3):132–6. doi: 10.1016/s0014-5793(00)01905-0
54. Jones KA, Tjian R. Sp1 binds to promoter sequences and activates herpes simplex virus 'Immediate-early' gene transcription in vitro. Nature (1985) 317(6033):179–82. doi: 10.1038/317179a0
55. Ostler JB, Thunuguntla P, Hendrickson BY, Jones C. Transactivation of herpes simplex virus 1 (Hsv-1) infected cell protein 4 enhancer by glucocorticoid receptor and stress-induced transcription factors requires overlapping krüppel-like transcription factor 4/Sp1 binding sites. J Virol (2021) 95(4):e01776-20. doi: 10.1128/jvi.01776-20
56. Gu B, Rivera-Gonzalez R, Smith CA, DeLuca NA. Herpes simplex virus infected cell polypeptide 4 preferentially represses Sp1-activated over basal transcription from its own promoter. Proc Natl Acad Sci U.S.A. (1993) 90(20):9528–32. doi: 10.1073/pnas.90.20.9528
57. Kim DB, DeLuca NA. Phosphorylation of transcription factor Sp1 during herpes simplex virus type 1 infection. J Virol (2002) 76(13):6473–9. doi: 10.1128/jvi.76.13.6473-6479.2002
58. Akhtar LN, Benveniste EN. Viral exploitation of host socs protein functions. J Virol (2011) 85(5):1912–21. doi: 10.1128/jvi.01857-10
59. Choi EJ, Lee CH, Shin OS. Suppressor of cytokine signaling 3 expression induced by varicella-zoster virus infection results in the modulation of virus replication. Scand J Immunol (2015) 82(4):337–44. doi: 10.1111/sji.12323
60. Frey KG, Ahmed CM, Dabelic R, Jager LD, Noon-Song EN, Haider SM, et al. Hsv-1-Induced socs-1 expression in keratinocytes: Use of a socs-1 antagonist to block a novel mechanism of viral immune evasion. J Immunol (2009) 183(2):1253–62. doi: 10.4049/jimmunol.0900570
61. Michaud F, Coulombe F, Gaudreault E, Paquet-Bouchard C, Rola-Pleszczynski M, Gosselin J. Epstein-Barr Virus interferes with the amplification of ifnalpha secretion by activating suppressor of cytokine signaling 3 in primary human monocytes. PloS One (2010) 5(7):e11908. doi: 10.1371/journal.pone.0011908
62. Sasaki A, Yasukawa H, Suzuki A, Kamizono S, Syoda T, Kinjyo I, et al. Cytokine-inducible Sh2 protein-3 (Cis3/Socs3) inhibits janus tyrosine kinase by binding through the n-terminal kinase inhibitory region as well as Sh2 domain. Genes Cells (1999) 4(6):339–51. doi: 10.1046/j.1365-2443.1999.00263.x
63. Yasukawa H, Misawa H, Sakamoto H, Masuhara M, Sasaki A, Wakioka T, et al. The jak-binding protein jab inhibits janus tyrosine kinase activity through binding in the activation loop. EMBO J (1999) 18(5):1309–20. doi: 10.1093/emboj/18.5.1309
64. Wormald S, Zhang JG, Krebs DL, Mielke LA, Silver J, Alexander WS, et al. The comparative roles of suppressor of cytokine signaling-1 and -3 in the inhibition and desensitization of cytokine signaling. J Biol Chem (2006) 281(16):11135–43. doi: 10.1074/jbc.M509595200
65. Wang Y, van Boxel-Dezaire AH, Cheon H, Yang J, Stark GR. Stat3 activation in response to il-6 is prolonged by the binding of il-6 receptor to egf receptor. Proc Natl Acad Sci U.S.A. (2013) 110(42):16975–80. doi: 10.1073/pnas.1315862110
66. Carow B, Rottenberg ME. Socs3, a major regulator of infection and inflammation. Front Immunol (2014) 5:58. doi: 10.3389/fimmu.2014.00058
67. Karlsen AE, Heding PE, Frobøse H, Rønn SG, Kruhøffer M, Orntoft TF, et al. Suppressor of cytokine signalling (Socs)-3 protects beta cells against il-1beta-Mediated toxicity through inhibition of multiple nuclear factor-Kappab-Regulated proapoptotic pathways. Diabetologia (2004) 47(11):1998–2011. doi: 10.1007/s00125-004-1568-3
68. Zamanian-Daryoush M, Mogensen TH, DiDonato JA, Williams BR. Nf-kappab activation by double-Stranded-Rna-Activated protein kinase (Pkr) is mediated through nf-Kappab-Inducing kinase and ikappab kinase. Mol Cell Biol (2000) 20(4):1278–90. doi: 10.1128/mcb.20.4.1278-1290.2000
69. Gu L, Ge Z, Wang Y, Shen M, Zhao P, Chen W. Double-stranded rna-dependent kinase pkr activates nf-Kb pathway in acute pancreatitis. Biochem Biophys Res Commun (2018) 503(3):1563–9. doi: 10.1016/j.bbrc.2018.07.080
70. Paludan SR, Mogensen SC. Virus-cell interactions regulating induction of tumor necrosis factor alpha production in macrophages infected with herpes simplex virus. J Virol (2001) 75(21):10170–8. doi: 10.1128/jvi.75.21.10170-10178.2001
71. Hu Z, Du H, Lin G, Han K, Cheng X, Feng Z, et al. Grass carp (Ctenopharyngodon idella) pact induces cell apoptosis and activates nf-Кb Via pkr. Fish Shellfish Immunol (2020) 103:377–84. doi: 10.1016/j.fsi.2020.05.036
72. Li X, Wu Z, An X, Mei Q, Bai M, Hanski L, et al. Blockade of the Lrp16-Pkr-Nf-Kb signaling axis sensitizes colorectal carcinoma cells to DNA-damaging cytotoxic therapy. Elife (2017) 6:e27301. doi: 10.7554/eLife.27301
73. Gil J, Alcamí J, Esteban M. Activation of nf-kappa b by the dsrna-dependent protein kinase, pkr involves the I kappa b kinase complex. Oncogene (2000) 19(11):1369–78. doi: 10.1038/sj.onc.1203448
74. Schulz O, Pichlmair A, Rehwinkel J, Rogers NC, Scheuner D, Kato H, et al. Protein kinase r contributes to immunity against specific viruses by regulating interferon mrna integrity. Cell Host Microbe (2010) 7(5):354–61. doi: 10.1016/j.chom.2010.04.007
75. Yoon CH, Lee ES, Lim DS, Bae YS. Pkr, a P53 target gene, plays a crucial role in the tumor-suppressor function of P53. Proc Natl Acad Sci U.S.A. (2009) 106(19):7852–7. doi: 10.1073/pnas.0812148106
76. Cuddihy AR, Wong AH, Tam NW, Li S, Koromilas AE. The double-stranded rna activated protein kinase pkr physically associates with the tumor suppressor P53 protein and phosphorylates human P53 on serine 392 in vitro. Oncogene (1999) 18(17):2690–702. doi: 10.1038/sj.onc.1202620
77. Huang Q, Xie D, Mao H, Wang H, Wu Z, Huang K, et al. Ctenopharyngodon idella P53 mediates between nf-Kb and pkr at the transcriptional level. Fish Shellfish Immunol (2017) 69:258–64. doi: 10.1016/j.fsi.2017.08.012
78. Dey M, Cao C, Dar AC, Tamura T, Ozato K, Sicheri F, et al. Mechanistic link between pkr dimerization, autophosphorylation, and Eif2alpha substrate recognition. Cell (2005) 122(6):901–13. doi: 10.1016/j.cell.2005.06.041
79. Dar AC, Dever TE, Sicheri F. Higher-order substrate recognition of Eif2alpha by the rna-dependent protein kinase pkr. Cell (2005) 122(6):887–900. doi: 10.1016/j.cell.2005.06.044
80. Su Q, Wang S, Baltzis D, Qu LK, Wong AH, Koromilas AE. Tyrosine phosphorylation acts as a molecular switch to full-scale activation of the Eif2alpha rna-dependent protein kinase. Proc Natl Acad Sci U.S.A. (2006) 103(1):63–8. doi: 10.1073/pnas.0508207103
81. Rabouw HH, Langereis MA, Knaap RC, Dalebout TJ, Canton J, Sola I, et al. Middle East respiratory coronavirus accessory protein 4a inhibits pkr-mediated antiviral stress responses. PloS Pathog (2016) 12(10):e1005982. doi: 10.1371/journal.ppat.1005982
82. Sharma NR, Majerciak V, Kruhlak MJ, Zheng ZM. Kshv inhibits stress granule formation by viral Orf57 blocking pkr activation. PloS Pathog (2017) 13(10):e1006677. doi: 10.1371/journal.ppat.1006677
83. Dauber B, Pelletier J, Smiley JR. The herpes simplex virus 1 vhs protein enhances translation of viral true late mrnas and virus production in a cell type-dependent manner. J Virol (2011) 85(11):5363–73. doi: 10.1128/jvi.00115-11
84. Dauber B, Poon D, Dos Santos T, Duguay BA, Mehta N, Saffran HA, et al. The herpes simplex virus virion host shutoff protein enhances translation of viral true late mrnas independently of suppressing protein kinase r and stress granule formation. J Virol (2016) 90(13):6049–57. doi: 10.1128/jvi.03180-15
85. Li C, Zhu Z, Du X, Cao W, Yang F, Zhang X, et al. Foot-and-Mouth disease virus induces lysosomal degradation of host protein kinase pkr by 3c proteinase to facilitate virus replication. Virology (2017) 509:222–31. doi: 10.1016/j.virol.2017.06.023
86. Valchanova RS, Picard-Maureau M, Budt M, Brune W. Murine cytomegalovirus M142 and M143 are both required to block protein kinase r-mediated shutdown of protein synthesis. J Virol (2006) 80(20):10181–90. doi: 10.1128/jvi.00908-06
87. McKenna SA, Lindhout DA, Shimoike T, Aitken CE, Puglisi JD. Viral dsrna inhibitors prevent self-association and autophosphorylation of pkr. J Mol Biol (2007) 372(1):103–13. doi: 10.1016/j.jmb.2007.06.028
88. Poppers J, Mulvey M, Perez C, Khoo D, Mohr I. Identification of a lytic-cycle Epstein-Barr virus gene product that can regulate pkr activation. J Virol (2003) 77(1):228–36. doi: 10.1128/jvi.77.1.228-236.2003
89. Lussignol M, Queval C, Bernet-Camard MF, Cotte-Laffitte J, Beau I, Codogno P, et al. The herpes simplex virus 1 Us11 protein inhibits autophagy through its interaction with the protein kinase pkr. J Virol (2013) 87(2):859–71. doi: 10.1128/jvi.01158-12
90. He B, Gross M, Roizman B. The Gamma134.5 protein of herpes simplex virus 1 has the structural and functional attributes of a protein phosphatase 1 regulatory subunit and is present in a high molecular weight complex with the enzyme in infected cells. J Biol Chem (1998) 273(33):20737–43. doi: 10.1074/jbc.273.33.20737
91. Esteban M, García MA, Domingo-Gil E, Arroyo J, Nombela C, Rivas C. The latency protein Lana2 from kaposi's sarcoma-associated herpesvirus inhibits apoptosis induced by dsrna-activated protein kinase but not rnase l activation. J Gen Virol (2003) 84(Pt 6):1463–70. doi: 10.1099/vir.0.19014-0
92. He B, Gross M, Roizman B. The Gamma(1)34.5 protein of herpes simplex virus 1 complexes with protein phosphatase 1alpha to dephosphorylate the alpha subunit of the eukaryotic translation initiation factor 2 and preclude the shutoff of protein synthesis by double-stranded rna-activated protein kinase. Proc Natl Acad Sci U.S.A. (1997) 94(3):843–8. doi: 10.1073/pnas.94.3.843
93. Borghese F, Michiels T. The leader protein of cardioviruses inhibits stress granule assembly. J Virol (2011) 85(18):9614–22. doi: 10.1128/jvi.00480-11
94. Borghese F, Sorgeloos F, Cesaro T, Michiels T. The leader protein of theiler's virus prevents the activation of pkr. J Virol (2019) 93(19):e01010-19. doi: 10.1128/jvi.01010-19
95. Pennisi R, Musarra-Pizzo M, Lei Z, Zhou GG, Sciortino MT. Vhs, Us3 and Ul13 viral tegument proteins are required for herpes simplex virus-induced modification of protein kinase r. Sci Rep (2020) 10(1):5580. doi: 10.1038/s41598-020-62619-2
96. Costa-Mattioli M, Walter P. The integrated stress response: From mechanism to disease. Science (2020) 368(6489):eaat5314. doi: 10.1126/science.aat5314
97. Watanabe T, Imamura T, Hiasa Y. Roles of protein kinase r in cancer: Potential as a therapeutic target. Cancer Sci (2018) 109(4):919–25. doi: 10.1111/cas.13551
98. Raafat N, Sadowski-Cron C, Mengus C, Heberer M, Spagnoli GC, Zajac P. Preventing vaccinia virus class-I epitopes presentation by hsv-Icp47 enhances the immunogenicity of a tap-independent cancer vaccine epitope. Int J Cancer (2012) 131(5):E659–69. doi: 10.1002/ijc.27362
99. Aisenbrey C, Sizun C, Koch J, Herget M, Abele R, Bechinger B, et al. Structure and dynamics of membrane-associated Icp47, a viral inhibitor of the mhc I antigen-processing machinery. J Biol Chem (2006) 281(41):30365–72. doi: 10.1074/jbc.M603000200
100. Deruelle MJ, Van den Broeke C, Nauwynck HJ, Mettenleiter TC, Favoreel HW. Pseudorabies virus Us3- and Ul49.5-dependent and -independent downregulation of mhc I cell surface expression in different cell types. Virology (2009) 395(2):172–81. doi: 10.1016/j.virol.2009.09.019
101. Imai T, Koyanagi N, Ogawa R, Shindo K, Suenaga T, Sato A, et al. Us3 kinase encoded by herpes simplex virus 1 mediates downregulation of cell surface major histocompatibility complex class I and evasion of Cd8+ T cells. PloS One (2013) 8(8):e72050. doi: 10.1371/journal.pone.0072050
102. Koyanagi N, Imai T, Shindo K, Sato A, Fujii W, Ichinohe T, et al. Herpes simplex virus-1 evasion of Cd8+ T cell accumulation contributes to viral encephalitis. J Clin Invest (2017) 127(10):3784–95. doi: 10.1172/jci92931
103. Spranger S, Dai D, Horton B, Gajewski TF. Tumor-residing Batf3 dendritic cells are required for effector T cell trafficking and adoptive T cell therapy. Cancer Cell (2017) 31(5):711–23.e4. doi: 10.1016/j.ccell.2017.04.003
104. Canny SP, Goel G, Reese TA, Zhang X, Xavier R, Virgin HW. Latent gammaherpesvirus 68 infection induces distinct transcriptional changes in different organs. J Virol (2014) 88(1):730–8. doi: 10.1128/jvi.02708-13
105. Prabhakaran K, Sheridan BS, Kinchington PR, Khanna KM, Decman V, Lathrop K, et al. Sensory neurons regulate the effector functions of Cd8+ T cells in controlling hsv-1 latency ex vivo. Immunity (2005) 23(5):515–25. doi: 10.1016/j.immuni.2005.09.017
106. Knickelbein JE, Khanna KM, Yee MB, Baty CJ, Kinchington PR, Hendricks RL. Noncytotoxic lytic granule-mediated Cd8+ T cell inhibition of hsv-1 reactivation from neuronal latency. Science (2008) 322(5899):268–71. doi: 10.1126/science.1164164
107. Nair A, Hunzeker J, Bonneau RH. Modulation of microglia and Cd8(+) T cell activation during the development of stress-induced herpes simplex virus type-1 encephalitis. Brain Behav Immun (2007) 21(6):791–806. doi: 10.1016/j.bbi.2007.01.005
108. Menendez CM, Carr DJJ. Herpes simplex virus-1 infects the olfactory bulb shortly following ocular infection and exhibits a long-term inflammatory profile in the form of effector and hsv-1-Specific T cells. J Neuroinflamm (2017) 14(1):124. doi: 10.1186/s12974-017-0903-9
109. Lang A, Nikolich-Zugich J. Development and migration of protective Cd8+ T cells into the nervous system following ocular herpes simplex virus-1 infection. J Immunol (2005) 174(5):2919–25. doi: 10.4049/jimmunol.174.5.2919
110. Banerjee K, Biswas PS, Kumaraguru U, Schoenberger SP, Rouse BT. Protective and pathological roles of virus-specific and bystander Cd8+ T cells in herpetic stromal keratitis. J Immunol (2004) 173(12):7575–83. doi: 10.4049/jimmunol.173.12.7575
111. Coquel F, Silva MJ, Técher H, Zadorozhny K, Sharma S, Nieminuszczy J, et al. Samhd1 acts at stalled replication forks to prevent interferon induction. Nature (2018) 557(7703):57–61. doi: 10.1038/s41586-018-0050-1
112. Cabello-Lobato MJ, Wang S, Schmidt CK. Samhd1 sheds moonlight on DNA double-strand break repair. Trends Genet (2017) 33(12):895–7. doi: 10.1016/j.tig.2017.09.007
113. Hollenbaugh JA, Gee P, Baker J, Daly MB, Amie SM, Tate J, et al. Host factor Samhd1 restricts DNA viruses in non-dividing myeloid cells. PloS Pathog (2013) 9(6):e1003481. doi: 10.1371/journal.ppat.1003481
114. Kim ET, White TE, Brandariz-Núñez A, Diaz-Griffero F, Weitzman MD. Samhd1 restricts herpes simplex virus 1 in macrophages by limiting DNA replication. J Virol (2013) 87(23):12949–56. doi: 10.1128/jvi.02291-13
115. Sze A, Belgnaoui SM, Olagnier D, Lin R, Hiscott J, van Grevenynghe J. Host restriction factor Samhd1 limits human T cell leukemia virus type 1 infection of monocytes Via sting-mediated apoptosis. Cell Host Microbe (2013) 14(4):422–34. doi: 10.1016/j.chom.2013.09.009
116. Chen Z, Zhu M, Pan X, Zhu Y, Yan H, Jiang T, et al. Inhibition of hepatitis b virus replication by Samhd1. Biochem Biophys Res Commun (2014) 450(4):1462–8. doi: 10.1016/j.bbrc.2014.07.023
117. Laguette N, Sobhian B, Casartelli N, Ringeard M, Chable-Bessia C, Ségéral E, et al. Samhd1 is the dendritic- and myeloid-Cell-Specific hiv-1 restriction factor counteracted by vpx. Nature (2011) 474(7353):654–7. doi: 10.1038/nature10117
118. Chougui G, Munir-Matloob S, Matkovic R, Martin MM, Morel M, Lahouassa H, et al. Hiv-2/Siv viral protein X counteracts hush repressor complex. Nat Microbiol (2018) 3(8):891–7. doi: 10.1038/s41564-018-0179-6
119. Hrecka K, Hao C, Gierszewska M, Swanson SK, Kesik-Brodacka M, Srivastava S, et al. Vpx relieves inhibition of hiv-1 infection of macrophages mediated by the Samhd1 protein. Nature (2011) 474(7353):658–61. doi: 10.1038/nature10195
120. Goujon C, Rivière L, Jarrosson-Wuilleme L, Bernaud J, Rigal D, Darlix JL, et al. Sivsm/Hiv-2 vpx proteins promote retroviral escape from a proteasome-dependent restriction pathway present in human dendritic cells. Retrovirology (2007) 4:2. doi: 10.1186/1742-4690-4-2
121. Rudd SG, Tsesmetzis N, Sanjiv K, Paulin CB, Sandhow L, Kutzner J, et al. Ribonucleotide reductase inhibitors suppress Samhd1 ara-ctpase activity enhancing cytarabine efficacy. EMBO Mol Med (2020) 12(3):e10419. doi: 10.15252/emmm.201910419
122. Iwasawa C, Tamura R, Sugiura Y, Suzuki S, Kuzumaki N, Narita M, et al. Increased cytotoxicity of herpes simplex virus thymidine kinase expression in human induced pluripotent stem cells. Int J Mol Sci (2019) 20(4):810. doi: 10.3390/ijms20040810
123. Valle-Casuso JC, Allouch A, David A, Lenzi GM, Studdard L, Barré-Sinoussi F, et al. P21 restricts hiv-1 in monocyte-derived dendritic cells through the reduction of deoxynucleoside triphosphate biosynthesis and regulation of Samhd1 antiviral activity. J Virol (2017) 91(23):e01324-17. doi: 10.1128/jvi.01324-17
124. Yan J, Hao C, DeLucia M, Swanson S, Florens L, Washburn MP, et al. Cyclina2-Cyclin-Dependent kinase regulates Samhd1 protein phosphohydrolase domain. J Biol Chem (2015) 290(21):13279–92. doi: 10.1074/jbc.M115.646588
125. White TE, Brandariz-Nuñez A, Valle-Casuso JC, Amie S, Nguyen LA, Kim B, et al. The retroviral restriction ability of Samhd1, but not its deoxynucleotide triphosphohydrolase activity, is regulated by phosphorylation. Cell Host Microbe (2013) 13(4):441–51. doi: 10.1016/j.chom.2013.03.005
126. Szaniawski MA, Spivak AM, Cox JE, Catrow JL, Hanley T, Williams E, et al. Samhd1 phosphorylation coordinates the anti-Hiv-1 response by diverse interferons and tyrosine kinase inhibition. mBio (2018) 9(3):e00819-18. doi: 10.1128/mBio.00819-18
127. Businger R, Deutschmann J, Gruska I, Milbradt J, Wiebusch L, Gramberg T, et al. Human cytomegalovirus overcomes Samhd1 restriction in macrophages Via Pul97. Nat Microbiol (2019) 4(12):2260–72. doi: 10.1038/s41564-019-0557-8
128. Zhang K, Lv DW, Li R. Conserved herpesvirus protein kinases target Samhd1 to facilitate virus replication. Cell Rep (2019) 28(2):449–59.e5. doi: 10.1016/j.celrep.2019.04.020
129. Chen S, Bonifati S, Qin Z, St Gelais C, Kodigepalli KM, Barrett BS, et al. Samhd1 suppresses innate immune responses to viral infections and inflammatory stimuli by inhibiting the nf-Kb and interferon pathways. Proc Natl Acad Sci U.S.A. (2018) 115(16):E3798–e807. doi: 10.1073/pnas.1801213115
130. Reuvers TGA, Kanaar R, Nonnekens J. DNA Damage-inducing anticancer therapies: From global to precision damage. Cancers (Basel) (2020) 12(8):2098. doi: 10.3390/cancers12082098
131. Jackson SP, Bartek J. The DNA-damage response in human biology and disease. Nature (2009) 461(7267):1071–8. doi: 10.1038/nature08467
132. Luftig MA. Viruses and the DNA damage response: Activation and antagonism. Annu Rev Virol (2014) 1(1):605–25. doi: 10.1146/annurev-virology-031413-085548
133. Lilley CE, Carson CT, Muotri AR, Gage FH, Weitzman MD. DNA Repair proteins affect the lifecycle of herpes simplex virus 1. Proc Natl Acad Sci U.S.A. (2005) 102(16):5844–9. doi: 10.1073/pnas.0501916102
134. Wilkinson DE, Weller SK. Herpes simplex virus type I disrupts the atr-dependent DNA-damage response during lytic infection. J Cell Sci (2006) 119(Pt 13):2695–703. doi: 10.1242/jcs.02981
135. Li R, Zhu J, Xie Z, Liao G, Liu J, Chen MR, et al. Conserved herpesvirus kinases target the DNA damage response pathway and Tip60 histone acetyltransferase to promote virus replication. Cell Host Microbe (2011) 10(4):390–400. doi: 10.1016/j.chom.2011.08.013
136. Yedjou CG, Tchounwou HM, Tchounwou PB. DNA Damage, cell cycle arrest, and apoptosis induction caused by lead in human leukemia cells. Int J Environ Res Public Health (2015) 13(1):ijerph13010056. doi: 10.3390/ijerph13010056
137. van Attikum H, Gasser SM. Crosstalk between histone modifications during the DNA damage response. Trends Cell Biol (2009) 19(5):207–17. doi: 10.1016/j.tcb.2009.03.001
138. Bogliolo M, Lyakhovich A, Callén E, Castellà M, Cappelli E, Ramírez MJ, et al. Histone H2ax and fanconi anemia Fancd2 function in the same pathway to maintain chromosome stability. EMBO J (2007) 26(5):1340–51. doi: 10.1038/sj.emboj.7601574
139. Saldivar JC, Cortez D, Cimprich KA. The essential kinase atr: Ensuring faithful duplication of a challenging genome. Nat Rev Mol Cell Biol (2017) 18(10):622–36. doi: 10.1038/nrm.2017.67
140. Banerjee S, Smallwood A, Hultén M. Atp-dependent reorganization of human sperm nuclear chromatin. J Cell Sci (1995) 108(Pt 2):755–65. doi: 10.1242/jcs.108.2.755
141. Tarakanova VL, Leung-Pineda V, Hwang S, Yang CW, Matatall K, Basson M, et al. Gamma-herpesvirus kinase actively initiates a DNA damage response by inducing phosphorylation of H2ax to foster viral replication. Cell Host Microbe (2007) 1(4):275–86. doi: 10.1016/j.chom.2007.05.008
142. Chen MR, Chang SJ, Huang H, Chen JY. A protein kinase activity associated with Epstein-Barr virus Bglf4 phosphorylates the viral early antigen ea-d in vitro. J Virol (2000) 74(7):3093–104. doi: 10.1128/jvi.74.7.3093-3104.2000
143. Mounce BC, Tsan FC, Droit L, Kohler S, Reitsma JM, Cirillo LA, et al. Gammaherpesvirus gene expression and DNA synthesis are facilitated by viral protein kinase and histone variant H2ax. Virology (2011) 420(2):73–81. doi: 10.1016/j.virol.2011.08.019
144. Jha HC, Upadhyay SK, M AJP, Lu J, Cai Q, Saha A, et al. H2ax phosphorylation is important for Lana-mediated kaposi's sarcoma-associated herpesvirus episome persistence. J Virol (2013) 87(9):5255–69. doi: 10.1128/jvi.03575-12
145. Li R, Wang L, Liao G, Guzzo CM, Matunis MJ, Zhu H, et al. Sumo binding by the Epstein-Barr virus protein kinase Bglf4 is crucial for Bglf4 function. J Virol (2012) 86(10):5412–21. doi: 10.1128/jvi.00314-12
146. Ming X, Bo Z, Miao Y, Chen H, Bao C, Sun L, et al. Pseudorabies virus kinase Ul13 phosphorylates H2ax to foster viral replication. FASEB J (2022) 36(3):e22221. doi: 10.1096/fj.202101360RR
147. Yamamoto T, Ali MA, Liu X, Cohen JI. Activation of H2ax and atm in varicella-zoster virus (Vzv)-infected cells is associated with expression of specific vzv genes. Virology (2014) 452-453:52–8. doi: 10.1016/j.virol.2013.12.039
148. Daikoku T, Kudoh A, Sugaya Y, Iwahori S, Shirata N, Isomura H, et al. Postreplicative mismatch repair factors are recruited to Epstein-Barr virus replication compartments. J Biol Chem (2006) 281(16):11422–30. doi: 10.1074/jbc.M510314200
149. Wilkinson DE, Weller SK. Recruitment of cellular recombination and repair proteins to sites of herpes simplex virus type 1 DNA replication is dependent on the composition of viral proteins within prereplicative sites and correlates with the induction of the DNA damage response. J Virol (2004) 78(9):4783–96. doi: 10.1128/jvi.78.9.4783-4796.2004
150. Taylor TJ, Knipe DM. Proteomics of herpes simplex virus replication compartments: Association of cellular DNA replication, repair, recombination, and chromatin remodeling proteins with Icp8. J Virol (2004) 78(11):5856–66. doi: 10.1128/jvi.78.11.5856-5866.2004
151. Bhatt AP, Wong JP, Weinberg MS, Host KM, Giffin LC, Buijnink J, et al. A viral kinase mimics S6 kinase to enhance cell proliferation. Proc Natl Acad Sci U.S.A. (2016) 113(28):7876–81. doi: 10.1073/pnas.1600587113
152. Kawaguchi Y, Matsumura T, Roizman B, Hirai K. Cellular elongation factor 1delta is modified in cells infected with representative alpha-, beta-, or gammaherpesviruses. J Virol (1999) 73(5):4456–60. doi: 10.1128/jvi.73.5.4456-4460.1999
153. Kato K, Kawaguchi Y, Tanaka M, Igarashi M, Yokoyama A, Matsuda G, et al. Epstein-Barr Virus-encoded protein kinase Bglf4 mediates hyperphosphorylation of cellular elongation factor 1delta (Ef-1delta): Ef-1delta is universally modified by conserved protein kinases of herpesviruses in mammalian cells. J Gen Virol (2001) 82(Pt 6):1457–63. doi: 10.1099/0022-1317-82-6-1457
154. Kawaguchi Y, Van Sant C, Roizman B. Eukaryotic elongation factor 1delta is hyperphosphorylated by the protein kinase encoded by the U(L)13 gene of herpes simplex virus 1. J Virol (1998) 72(3):1731–6. doi: 10.1128/jvi.72.3.1731-1736.1998
155. Boutell C, Cuchet-Lourenço D, Vanni E, Orr A, Glass M, McFarlane S, et al. A viral ubiquitin ligase has substrate preferential sumo targeted ubiquitin ligase activity that counteracts intrinsic antiviral defence. PloS Pathog (2011) 7(9):e1002245. doi: 10.1371/journal.ppat.1002245
156. Tatham MH, Geoffroy MC, Shen L, Plechanovova A, Hattersley N, Jaffray EG, et al. Rnf4 is a poly-Sumo-Specific E3 ubiquitin ligase required for arsenic-induced pml degradation. Nat Cell Biol (2008) 10(5):538–46. doi: 10.1038/ncb1716
157. Liu J, Wu Z, Han D, Wei C, Liang Y, Jiang T, et al. Mesencephalic astrocyte-derived neurotrophic factor inhibits liver cancer through small ubiquitin-related modifier (Sumo)Ylation-related suppression of nf-Kb/Snail signaling pathway and epithelial-mesenchymal transition. Hepatology (2020) 71(4):1262–78. doi: 10.1002/hep.30917
158. Kubota T, Matsuoka M, Chang TH, Tailor P, Sasaki T, Tashiro M, et al. Virus infection triggers sumoylation of Irf3 and Irf7, leading to the negative regulation of type I interferon gene expression. J Biol Chem (2008) 283(37):25660–70. doi: 10.1074/jbc.M804479200
159. Bentz GL, Shackelford J, Pagano JS. Epstein-Barr Virus latent membrane protein 1 regulates the function of interferon regulatory factor 7 by inducing its sumoylation. J Virol (2012) 86(22):12251–61. doi: 10.1128/jvi.01407-12
160. Chang TH, Kubota T, Matsuoka M, Jones S, Bradfute SB, Bray M, et al. Ebola Zaire Virus blocks type I interferon production by exploiting the host sumo modification machinery. PloS Pathog (2009) 5(6):e1000493. doi: 10.1371/journal.ppat.1000493
161. Izumiya Y, Ellison TJ, Yeh ET, Jung JU, Luciw PA, Kung HJ. Kaposi's sarcoma-associated herpesvirus K-bzip represses gene transcription Via sumo modification. J Virol (2005) 79(15):9912–25. doi: 10.1128/jvi.79.15.9912-9925.2005
162. Izumiya Y, Lin SF, Ellison T, Chen LY, Izumiya C, Luciw P, et al. Kaposi's sarcoma-associated herpesvirus K-bzip is a coregulator of K-rta: Physical association and promoter-dependent transcriptional repression. J Virol (2003) 77(2):1441–51. doi: 10.1128/jvi.77.2.1441-1451.2003
163. Izumiya Y, Izumiya C, Van Geelen A, Wang DH, Lam KS, Luciw PA, et al. Kaposi's sarcoma-associated herpesvirus-encoded protein kinase and its interaction with K-bzip. J Virol (2007) 81(3):1072–82. doi: 10.1128/jvi.01473-06
164. Izumiya Y, Kobayashi K, Kim KY, Pochampalli M, Izumiya C, Shevchenko B, et al. Kaposi's sarcoma-associated herpesvirus K-rta exhibits sumo-targeting ubiquitin ligase (Stubl) like activity and is essential for viral reactivation. PloS Pathog (2013) 9(8):e1003506. doi: 10.1371/journal.ppat.1003506
165. Chang PC, Fitzgerald LD, Van Geelen A, Izumiya Y, Ellison TJ, Wang DH, et al. Kruppel-associated box domain-associated protein-1 as a latency regulator for kaposi's sarcoma-associated herpesvirus and its modulation by the viral protein kinase. Cancer Res (2009) 69(14):5681–9. doi: 10.1158/0008-5472.Can-08-4570
166. Hagemeier SR, Dickerson SJ, Meng Q, Yu X, Mertz JE, Kenney SC. Sumoylation of the Epstein-Barr virus Bzlf1 protein inhibits its transcriptional activity and is regulated by the virus-encoded protein kinase. J Virol (2010) 84(9):4383–94. doi: 10.1128/jvi.02369-09
167. Adamson AL, Kenney S. Epstein-Barr Virus immediate-early protein Bzlf1 is sumo-1 modified and disrupts promyelocytic leukemia bodies. J Virol (2001) 75(5):2388–99. doi: 10.1128/jvi.75.5.2388-2399.2001
168. Avey D, Tepper S, Pifer B, Bahga A, Williams H, Gillen J, et al. Discovery of a coregulatory interaction between kaposi's sarcoma-associated herpesvirus Orf45 and the viral protein kinase Orf36. J Virol (2016) 90(13):5953–64. doi: 10.1128/jvi.00516-16
169. Asai R, Ohno T, Kato A, Kawaguchi Y. Identification of proteins directly phosphorylated by Ul13 protein kinase from herpes simplex virus 1. Microbes Infect (2007) 9(12-13):1434–8. doi: 10.1016/j.micinf.2007.07.008
170. Purves FC, Ogle WO, Roizman B. Processing of the herpes simplex virus regulatory protein alpha 22 mediated by the Ul13 protein kinase determines the accumulation of a subset of alpha and gamma mrnas and proteins in infected cells. Proc Natl Acad Sci U.S.A. (1993) 90(14):6701–5. doi: 10.1073/pnas.90.14.6701
171. Baek MC, Krosky PM, Pearson A, Coen DM. Phosphorylation of the rna polymerase ii carboxyl-terminal domain in human cytomegalovirus-infected cells and in vitro by the viral Ul97 protein kinase. Virology (2004) 324(1):184–93. doi: 10.1016/j.virol.2004.03.015
172. Long MC, Leong V, Schaffer PA, Spencer CA, Rice SA. Icp22 and the Ul13 protein kinase are both required for herpes simplex virus-induced modification of the Large subunit of rna polymerase ii. J Virol (1999) 73(7):5593–604. doi: 10.1128/jvi.73.7.5593-5604.1999
173. Jenkins HL, Spencer CA. Rna polymerase ii holoenzyme modifications accompany transcription reprogramming in herpes simplex virus type 1-infected cells. J Virol (2001) 75(20):9872–84. doi: 10.1128/jvi.75.20.9872-9884.2001
174. Advani SJ, Brandimarti R, Weichselbaum RR, Roizman B. The disappearance of cyclins a and b and the increase in activity of the G(2)/M-phase cellular kinase Cdc2 in herpes simplex virus 1-infected cells require expression of the Alpha22/U(S)1.5 and U(L)13 viral genes. J Virol (2000) 74(1):8–15. doi: 10.1128/JVI.74.1.8-15.2000
175. Advani SJ, Weichselbaum RR, Roizman B. Cdc2 cyclin-dependent kinase binds and phosphorylates herpes simplex virus 1 U(L)42 DNA synthesis processivity factor. J Virol (2001) 75(21):10326–33. doi: 10.1128/jvi.75.21.10326-10333.2001
176. Advani SJ, Weichselbaum RR, Roizman B. Herpes simplex virus 1 activates Cdc2 to recruit topoisomerase ii alpha for post-DNA synthesis expression of late genes. Proc Natl Acad Sci U.S.A. (2003) 100(8):4825–30. doi: 10.1073/pnas.0730735100
177. Jacob RJ, Roizman B. Anatomy of herpes simplex virus DNA viii. properties of the replicating DNA. J Virol (1977) 23(2):394–411. doi: 10.1128/jvi.23.2.394-411.1977
178. Fraser KA, Rice SA. Herpes simplex virus immediate-early protein Icp22 triggers loss of serine 2-phosphorylated rna polymerase ii. J Virol (2007) 81(10):5091–101. doi: 10.1128/jvi.00184-07
179. Durand LO, Advani SJ, Poon AP, Roizman B. The carboxyl-terminal domain of rna polymerase ii is phosphorylated by a complex containing Cdk9 and infected-cell protein 22 of herpes simplex virus 1. J Virol (2005) 79(11):6757–62. doi: 10.1128/jvi.79.11.6757-6762.2005
180. Morrison EE, Wang YF, Meredith DM. Phosphorylation of structural components promotes dissociation of the herpes simplex virus type 1 tegument. J Virol (1998) 72(9):7108–14. doi: 10.1128/jvi.72.9.7108-7114.1998
181. Ren X, Harms JS, Splitter GA. Bovine herpesvirus 1 tegument protein Vp22 interacts with histones, and the carboxyl terminus of Vp22 is required for nuclear localization. J Virol (2001) 75(17):8251–8. doi: 10.1128/jvi.75.17.8251-8258.2001
182. van Leeuwen H, Okuwaki M, Hong R, Chakravarti D, Nagata K, O'Hare P. Herpes simplex virus type 1 tegument protein Vp22 interacts with taf-I proteins and inhibits nucleosome assembly but not regulation of histone acetylation by inhat. J Gen Virol (2003) 84(Pt 9):2501–10. doi: 10.1099/vir.0.19326-0
183. Huang J, You H, Su C, Li Y, Chen S, Zheng C. Herpes simplex virus 1 tegument protein Vp22 abrogates Cgas/Sting-mediated antiviral innate immunity. J Virol (2018) 92(15):e00841-18. doi: 10.1128/jvi.00841-18
184. Hamza MS, Reyes RA, Izumiya Y, Wisdom R, Kung HJ, Luciw PA. Orf36 protein kinase of kaposi's sarcoma herpesvirus activates the c-jun n-terminal kinase signaling pathway. J Biol Chem (2004) 279(37):38325–30. doi: 10.1074/jbc.M400964200
185. Lee CP, Huang YH, Lin SF, Chang Y, Chang YH, Takada K, et al. Epstein-Barr Virus Bglf4 kinase induces disassembly of the nuclear lamina to facilitate virion production. J Virol (2008) 82(23):11913–26. doi: 10.1128/jvi.01100-08
186. Cano-Monreal GL, Wylie KM, Cao F, Tavis JE, Morrison LA. Herpes simplex virus 2 Ul13 protein kinase disrupts nuclear lamins. Virology (2009) 392(1):137–47. doi: 10.1016/j.virol.2009.06.051
187. Kato A, Yamamoto M, Ohno T, Tanaka M, Sata T, Nishiyama Y, et al. Herpes simplex virus 1-encoded protein kinase Ul13 phosphorylates viral Us3 protein kinase and regulates nuclear localization of viral envelopment factors Ul34 and Ul31. J Virol (2006) 80(3):1476–86. doi: 10.1128/jvi.80.3.1476-1486.2006
188. Ng TI, Ogle WO, Roizman B. Ul13 protein kinase of herpes simplex virus 1 complexes with glycoprotein e and mediates the phosphorylation of the viral fc receptor: Glycoproteins e and I. Virology (1998) 241(1):37–48. doi: 10.1006/viro.1997.8963
189. Van Cleemput J, Koyuncu OO, Laval K, Engel EA, Enquist LW. Crispr/Cas9-constructed pseudorabies virus mutants reveal the importance of Ul13 in alphaherpesvirus escape from genome silencing. J Virol (2021) 95(6):e02286-20. doi: 10.1128/jvi.02286-20
190. Lukac DM, Renne R, Kirshner JR, Ganem D. Reactivation of kaposi's sarcoma-associated herpesvirus infection from latency by expression of the orf 50 transactivator, a homolog of the ebv r protein. Virology (1998) 252(2):304–12. doi: 10.1006/viro.1998.9486
191. Guito J, Lukac DM. Kshv rta promoter specification and viral reactivation. Front Microbiol (2012) 3:30. doi: 10.3389/fmicb.2012.00030
192. Damania B, Jeong JH, Bowser BS, DeWire SM, Staudt MR, Dittmer DP. Comparison of the Rta/Orf50 transactivator proteins of gamma-2-Herpesviruses. J Virol (2004) 78(10):5491–9. doi: 10.1128/jvi.78.10.5491-5499.2004
193. Simpson S, Fiches G, Jean MJ, Dieringer M, McGuinness J, John SP, et al. Inhibition of Tip60 reduces lytic and latent gene expression of kaposi's sarcoma-associated herpes virus (Kshv) and proliferation of kshv-infected tumor cells. Front Microbiol (2018) 9:788. doi: 10.3389/fmicb.2018.00788
194. Kedes DH, Lagunoff M, Renne R, Ganem D. Identification of the gene encoding the major latency-associated nuclear antigen of the kaposi's sarcoma-associated herpesvirus. J Clin Invest (1997) 100(10):2606–10. doi: 10.1172/jci119804
195. Shamay M, Liu J, Li R, Liao G, Shen L, Greenway M, et al. A protein array screen for kaposi's sarcoma-associated herpesvirus Lana interactors links Lana to Tip60, Pp2a activity, and telomere shortening. J Virol (2012) 86(9):5179–91. doi: 10.1128/jvi.00169-12
196. Sylvester PA, Jondle CN, Stoltz KP, Lanham J, Dittel BN, Tarakanova VL. Conserved gammaherpesvirus protein kinase counters the antiviral effects of myeloid cell-specific Stat1 expression to promote the establishment of splenic b cell latency. J Virol (2021) 95(17):e0085921. doi: 10.1128/jvi.00859-21
197. Liu T, Khanna KM, Chen X, Fink DJ, Hendricks RL. Cd8(+) T cells can block herpes simplex virus type 1 (Hsv-1) reactivation from latency in sensory neurons. J Exp Med (2000) 191(9):1459–66. doi: 10.1084/jem.191.9.1459
198. Doherty PC, Christensen JP, Belz GT, Stevenson PG, Sangster MY. Dissecting the host response to a gamma-herpesvirus. Philos Trans R Soc Lond B Biol Sci (2001) 356(1408):581–93. doi: 10.1098/rstb.2000.0786
199. Hollingworth R, Horniblow RD, Forrest C, Stewart GS, Grand RJ. Localization of double-strand break repair proteins to viral replication compartments following lytic reactivation of kaposi's sarcoma-associated herpesvirus. J Virol (2017) 91(22):e00930-17. doi: 10.1128/jvi.00930-17
200. Zimmermann A, Wilts H, Lenhardt M, Hahn M, Mertens T. Indolocarbazoles exhibit strong antiviral activity against human cytomegalovirus and are potent inhibitors of the Pul97 protein kinase. Antiviral Res (2000) 48(1):49–60. doi: 10.1016/s0166-3542(00)00118-2
201. Gershburg E, Hong K, Pagano JS. Effects of maribavir and selected indolocarbazoles on Epstein-Barr virus protein kinase Bglf4 and on viral lytic replication. Antimicrob Agents Chemother (2004) 48(5):1900–3. doi: 10.1128/aac.48.5.1900-1903.2004
202. Goswami R, Gershburg S, Satorius A, Gershburg E. Protein kinase inhibitors that inhibit induction of lytic program and replication of Epstein-Barr virus. Antiviral Res (2012) 96(3):296–304. doi: 10.1016/j.antiviral.2012.09.021
203. Hoke GD, Draper K, Freier SM, Gonzalez C, Driver VB, Zounes MC, et al. Effects of phosphorothioate capping on antisense oligonucleotide stability, hybridization and antiviral efficacy versus herpes simplex virus infection. Nucleic Acids Res (1991) 19(20):5743–8. doi: 10.1093/nar/19.20.5743
204. Crooke RM, Hoke GD, Shoemaker JE. In vitro toxicological evaluation of Isis 1082, a phosphorothioate oligonucleotide inhibitor of herpes simplex virus. Antimicrob Agents Chemother (1992) 36(3):527–32. doi: 10.1128/aac.36.3.527
205. Sun X, Bristol JA, Iwahori S, Hagemeier SR, Meng Q, Barlow EA, et al. Hsp90 inhibitor 17-dmag decreases expression of conserved herpesvirus protein kinases and reduces virus production in Epstein-Barr virus-infected cells. J Virol (2013) 87(18):10126–38. doi: 10.1128/jvi.01671-13
206. Lv L, Liu X, Jiang C, Wang X, Cao M, Bai J, et al. Pathogenicity and immunogenicity of a Gi/Ge/Tk/Ul13-Gene-Deleted variant pseudorabies virus strain in swine. Vet Microbiol (2021) 258:109104. doi: 10.1016/j.vetmic.2021.109104
207. Olotu FA, Soliman MES. Immunoinformatics prediction of potential b-cell and T-cell epitopes as effective vaccine candidates for eliciting immunogenic responses against Epstein-Barr virus. BioMed J (2021) 44(3):317–37. doi: 10.1016/j.bj.2020.01.002
208. Uldrick TS, Polizzotto MN, Aleman K, O'Mahony D, Wyvill KM, Wang V, et al. High-dose zidovudine plus valganciclovir for kaposi sarcoma herpesvirus-associated multicentric castleman disease: A pilot study of virus-activated cytotoxic therapy. Blood (2011) 117(26):6977–86. doi: 10.1182/blood-2010-11-317610
209. Wang QJ, Jenkins FJ, Jacobson LP, Kingsley LA, Day RD, Zhang ZW, et al. Primary human herpesvirus 8 infection generates a broadly specific Cd8(+) T-cell response to viral lytic cycle proteins. Blood (2001) 97(8):2366–73. doi: 10.1182/blood.v97.8.2366
Keywords: UL13, serine/threonine protein kinase, immune escape, viral replication, cGAS-STING, NF-κB
Citation: Zhou L, Cheng A, Wang M, Wu Y, Yang Q, Tian B, Ou X, Sun D, Zhang S, Mao S, Zhao X-X, Huang J, Gao Q, Zhu D, Jia R, Liu M and Chen S (2022) Mechanism of herpesvirus protein kinase UL13 in immune escape and viral replication. Front. Immunol. 13:1088690. doi: 10.3389/fimmu.2022.1088690
Received: 03 November 2022; Accepted: 15 November 2022;
Published: 30 November 2022.
Edited by:
Junji Xing, Houston Methodist Research Institute, United StatesReviewed by:
Yuexiu Zhang, The Ohio State University, United StatesXiaochuan Liu, University of California, Riverside, United States
Copyright © 2022 Zhou, Cheng, Wang, Wu, Yang, Tian, Ou, Sun, Zhang, Mao, Zhao, Huang, Gao, Zhu, Jia, Liu and Chen. This is an open-access article distributed under the terms of the Creative Commons Attribution License (CC BY). The use, distribution or reproduction in other forums is permitted, provided the original author(s) and the copyright owner(s) are credited and that the original publication in this journal is cited, in accordance with accepted academic practice. No use, distribution or reproduction is permitted which does not comply with these terms.
*Correspondence: Mingshu Wang, mshwang@163.com
†These authors have contributed equally to this work