- 1Joint International Research Laboratory of Agriculture and Agri-Product Safety, Ministry of Education of China, Yangzhou University, Yangzhou, China
- 2College of Animal Science and Technology, Yangzhou University, Yangzhou, China
- 3College of Veterinary Medicine, Nanjing Agricultural University, Nanjing, China
- 4College of Veterinary Medicine, Yangzhou University, Yangzhou, China
As a first-line barrier against bacterial infection of mammary tissues, bovine mammary epithelial cells (bMECs) are generally believed to be involved in the immune response due to exogenous stress. Due to the escalating crisis of antibiotic resistance, there is an urgent need for new strategies to combat pathogenic bacteria-infected bovine mastitis. In this study, isolated bMECs and Institute of Cancer Research (ICR) mice were used for Escherichia coli infection and caffeic acid (CA) pretreatment experiments in vitro and in vivo. The inhibitory effect of CA on bacterial growth and biofilm formation was also demonstrated with bacteria strains isolated from mastitis-infected milk. It was demonstrated that CA supplementation prohibits the growth of the predominant strains of bacteria isolated from clinical bovine mastitis milk samples. CA was found to disrupt the biofilm formation of E. coli B1 in a sub-minimum inhibitory concentration (sub-MIC) and inhibited the adherence property of E. coli on bMECs by decreasing the staining of bacteria on cell surfaces in vitro. In addition, CA was found to attenuate proinflammatory and oxidative responses in cells infected with E. coli. The pretreatment of bMECs with CA also restored altered lipid homeostasis caused by E. coli stimulation. The protective role of CA was further confirmed via the administration of CA in mice followed by representative Gram-negative bacterial infection. Collectively, these findings highlight the potential of CA to mediate Gram-negative infections and indicate that it has the potential to be developed as a novel antibacterial drug.
Introduction
Bovine mastitis is characterized by the invasion and colonization of bacteria in mammary tissue, threatening dairy production and milk quality (1–3). Clinical mastitis infection by Escherichia coli or Klebsiella pneumoniae is environmentally linked, as compared to contagious pathogens such as Staphylococcus aureus and Streptococcus agalactiae (4, 5). Excessive inflammation induced by toxins secreted from these pathogens leads to pathological courses such as lesions on the mammary tissue, which impair the cow’s performance as a milk producer (6). It has been reported that endotoxins including lipopolysaccharide (LPS), the component that envelops the wall of Gram-negative bacteria, can induce a storm of inflammatory responses in bovine mammary epithelial cells (bMECs) (7, 8). This kind of induction of inflammation appears to elicit several sets of innate immune response, oxidative stress, and metabolic reprogramming-related genes (9, 10). Epidemiological investigations reveal that Gram-negative bacteria, such as E. coli and K. pneumoniae, in bovine mastitis are becoming more common on dairy farms worldwide and that pathogens are becoming multidrug-resistant (MDR) (11, 12).
Along with therapeutic strategies, such as antibiotic therapy, epithelial cells act as a first barrier against invading bacteria and as a bridge for the host’s defense response (13, 14). bMECs are involved not only in the production of milk but also in immune regulation stimulated by exogenous pathogens (15–17). During E. coli mastitis, often-generalized inflammatory responses are regulated by the number of immune molecules, such as toll-like receptor 4 and the NF-κB complex transcription factor (18, 19). These, in turn, act as a master switch to activate a wealth of proinflammatory genes (20). Moreover, the defensive process of bMECs has also been suggested to elicit oxidative damage and metabolic changes in response to E. coli infections (21). Eradicating bacteria at an earlier time is of great importance to control the inflammation of epithelial cells and restore tissue homeostasis (22). Epithelial cells usually regulate multiple defensive mechanisms against bacterial invasion via alteration of cell integrity, apoptosis, and autophagy (22, 23). For MDR bacteria, a prolonged attachment to cells provides opportunities for the invasion to remain viable and remain in the cytosol of epithelial cells (24). With respect to the persistent drug resistance of pathogens related to bovine mastitis, antibiotics are still effective in clearing bacteria, but overuse or misuse leads to the problems such as MDR, antibiotic tolerance, and lack of food safety (25–27). Therefore, it is urgent to develop alternative strategies to antibiotics in the treatment of MDR bacteria-infected bovine mastitis.
Caffeic acid (3,4-dihydroxycinnamic acid; CA) is a phenolic compound that is present in several plants (28). It has been employed as a strategy for combating pathogenic bacteria or chronic infection due to its antimicrobial and anti-inflammatory activities, as well as properties related to boosting immunity (29). To uncover the protective role of CA in bMECs, a study revealed that CA suppresses LPS-induced inflammatory responses via the suppression of NF-κB/MAPK cascades (30). However, being predominantly biologically active, caffeic acid might also participate in antioxidation and metabolic homeostasis in bMECs, as well as interactions between pathogens and host-cell immune responses, but these qualities remain largely unknown. The present study investigated the role of CA in alleviating E. coli-induced detrimental effects in bMECs with regard to the inhibition of inflammation responses, oxidative stress, and alteration of fatty acid metabolism. Moreover, reductions in Gram-negative bacteria growth and the internalization properties of bMECs were also characterized, as well as the amelioration of damage in mouse mammary glands. The results may provide insights into the exploitation of strategies against Gram-negative pathogen-induced bovine mastitis, as well as the treatment for homologous infectious diseases.
Materials and methods
Ethics
The experimental protocols were verified by the Animal Experiment Committee of Yangzhou University according to the Regulations for the Administration of Affairs Concerning Experimental Animals in China. The experiments were strictly implemented in accordance with the approved guidelines and regulations (YZU-2022002-096).
Cell culture conditions
Primary bMEC cultures were performed as described previously (21, 31). In brief, three Holstein cows in mid-lactation (30.26 ± 3.1 kg/day of milk production, 2.7 parity, and 175 ± 6 days in milk) with absence of clinical incidence of diseases were employed for isolation of cells. The bMECs were purified by differential centrifugation, and the digested solution containing fibroblasts was discarded after trypsin digestion for 3 min, and then the culture medium was added to continue the cell culture. Cell passage from the fourth to sixth generation was used in the current study; 2 × 105 of cells were seeded in six-well plates with medium containing Roswell Park Memorial Institute (RPMI) with 10% heat-inactivated fetal bovine serum (Gibco, San Diego, CA, USA) and antibiotics (penicillin 100 IU/ml; streptomycin 100 μg/ml) (Thermo Fisher Scientific, Pleasanton, CA, USA). The incubation of cells was maintained at 37°C with a 5% CO2 condition.
Experimental design
Cell experiment
The E. coli-induced inflammatory model was established and described previously (32). The optimized CA doses in the current study were selected according to a previous study, with few modifications (30). Bacteria used in the infection experiment were quantified by surviving bacteria counting method (colony-forming unit (CFU) counting). No toxicity of CA treatment to cells was found at concentrations from 10 to 500 μg/ml via the cell viability test. Cells stimulated with E. coli (1 × 107 CFU/ml) for 6 h were selected as the positive control (E. coli); cells pretreated with CA at concentrations of either 125 or 250 μg/ml for 12 h, followed by the E. coli (1 × 107 CFU/ml) challenge for 6 h (C125+E; C250+E). Cells treated with phosphate-buffered saline (PBS) for 18 h were selected as negative control (NC).
Bacteria and reagents
All strains were isolated from the milk of dairy cattle with clinical signs of mastitis, which were validated by the California Mastitis Test (CMT) according to the recommendation of the U.S. National Mastitis Council (33, 34). The isolation and identification of all strains used in the current study are described in the Supplementary Material. The pathogenicities of isolated E. coli, S. aureus, Pseudomonas aeruginosa, and K. pneumoniae strains in mice have been determined, and results are indicative of the serious infection with histological changes in tissues like the liver and spleen. Unless otherwise noted, bacteria were grown in Mueller–Hinton broth (MHB; HB6231, Qingdao Hope Bio-technology) or on MH agar (MHA, HB6232) plates at 37°C. Caffeic acid with a purity of more than 98% was purchased from Sigma-Aldrich (Sigma, St. Louis, MO, USA; C0625).
Animal experiment
Six to 8-week-old male Institute of Cancer Research (ICR) mice and 1-week lactating female ICR mice were obtained from the Center of Comparative Medicine at Yangzhou University. One week prior, infection was set for adaption of mice to the standard environment conditions (23°C ± 2°C; 55% ± 10% of humidity). The experimental design was described as follows: male ICR mice (70 in total) were separated into seven groups, with each group comprising 10 mice:
1. Control group: mice normally treated were taken as control.
2. Bacteria challenge group: mice received an intraperitoneal injection with E. coli YZ6 or K. pneumoniae SH1 (1 × 108 CFU/ml) on day 11 for 6 h.
3. CA + bacteria group: mice were orally administrated with CA at a dose of 25 or 50 mg/kg/day for 10 days, following E. coli or K. pneumoniae challenge at day 11 for 6 h.
Lactating ICR female mice (40 in total) were separated into four groups with each group comprising 10 mice:
1. Control group: mice normally treated were taken as control.
2. E. coli challenge group: mice received intramammary injection with E. coli B1 (1 × 106 CFU/ml) on day 11 for 6 h.
3. CA + E. coli group: mice were orally administrated with CA at a dose of 25 or 50 mg/kg/day for 10 days, followed by E. coli intramammary injection on day 11 for 6 h.
Animals were placed under anesthesia at 6 h after pathogenic injection and sacrificed by cervical dislocation. The doses of CA at 25 or 50 mg/kg were used in this study based on the acute toxicity test with pathological observation of animals treated with CA at a range of 10 to 100 mg/kg. Anticoagulation tubes (EDTA) were used for collecting the peripheral blood from the removed eyeballs. Mammary tissues were excised and rinsed in cold PBS, and all the samples were stored at −80°C immediately after collection.
Lethality assessment
The mortality rates were determined for a duration of 24 h in groups as selected in the present study. The time points for monitoring the death of mice were 8 h, 12 h, 18 h, and 24 h post-injection of bacteria.
Histological determination
Pieces of mammary tissues sampled from all experimental groups were fixed in buffered formalin phosphate (10%). Then, fixed samples were paraffin-embedded, sectioned, and stained with hematoxylin and eosin, and a phase-contrast microscope was used for imaging (Nikon, Tokyo, Japan).
Peripheral malondialdehyde content
The concentration of malondialdehyde (MDA) in peripheral plasma from all groups was determined by ELISA commercial kit and performed in accordance with the manufacturer’s instruction (Jiancheng Bioengineering Institute, Nanjing, China).
Minimum inhibitory concentration and minimum bactericidal concentration determination
The determination of minimum inhibitory concentration (MIC) for CA on each bacterial strain was performed using the standard microdilution approach recommended by the Clinical and Laboratory Standards Institute (CLSI) (2017). Briefly, a twofold dilution of CA with an equal volume of bacterial cultures containing 1.5 × 106 CFU/ml in a 96-well microliter plate is available. The MIC values were recognized as the lowest concentrations of the CA with invisible bacteria growth. For minimum bactericidal concentration (MBC) of CA, bacteria cultures containing CA equal to or higher than MIC were streaked on blood agar plates and incubated at 37°C for 24 h. The concentration of CA at which no visible colony was observed on the plate after 24-h incubation was defined as MBC.
Bacteria growth curve determination
The overnight bacterial culture at 37°C from a single colony was prepared and diluted at 1:10,000 into an MHB medium. CA at concentration of 0, 0.5625, 1.25, 2.5, and 5 μg/ml was added to the cultures. The optical density at OD600nm was measured by a spectrophotometer (Tecan, Spark, Switzerland) at 0, 4, 6, 8, 12, and 24 h of incubation. Experiments were performed in triplicate.
Biofilm morphology assay
CA was diluted to 0.5× MIC, 1× MIC, 2× MIC, and 4× MIC in MHB medium. The overnight culture was added to a six-well plate placed with crawling slides for 24-h incubation at 37°C without shaking. For live or dead cell determination in matured biofilm, the 24-h incubated biofilm was added with or without CA for an additional 24 h. The slides were washed with PBS to remove planktonic cells for staining with SYTO9 (Green) and PI (Red) pre-mixture using LIVE/DEAD BacLight Bacterial Viability Kit (L7007, Thermo Fisher Scientific, Waltham, MA, USA). The images were captured using DMi8 Microsystems BmbH (Leica, Wetzlar, Germany). For the biofilm formation inhibition experiment, 40 μl of overnight culture was seeded to a six-well plate containing 1,960 μl of diluted CA. Cells were stained with 1.5% Cresyl Violet (CV) and visualized using DMi8 Microsystems BmbH (Leica, Wetzlar, Germany).
Scanning of bacteria adhesion
For scanning the level of E. coli adhesion, cell sample nuclei were counterstained with Hoechst 33342 (Beyotime, Beijing, China). The bacterial was pHrodo green probes labeled prior to the infection of bMECs (Thermo Scientific, MA); 1 × 106 CFU bacteria and increasing doses of CA at 75, 125, and 250 μg/ml were cocultured with bMECs at 37°C for 3 h. The images were captured using DMi8 Microsystems BmbH (Leica, Wetzlar, Germany).
Counting for bacteria adhesion to bovine mammary epithelial cell
Cells were seeded on the 12-well plates until the growth of 90% prior to the bacteria challenge 1 × 106 CFU bacteria and increasing doses of CA at 75, 125, and 250 μg/ml were cocultured with bMECs at 37°C for 3 h. The cells were washed three times with PBS to remove the planktonic cells and re-suspended with trypsin. Cocultured suspension was spread on an MH agar plate with 10 times’ dilution. The final CFUs were determined by counting the colonies after incubation at 37°C for 18 h.
5-Ethynyl-2′-deoxyuridine determination
The BeyoClick 5-ethynyl-2′-deoxyuridine (EdU) cell proliferation kit with Alexa Fluor 555 (Beyotime, Shanghai, China) was applied for the determination of cell proliferation under different treatments and performed as described previously (35). EdU solution with a concentration of 10 μM was used to incubate cells for 2 h; 4% paraformaldehyde was used for fixation, and 0.3% Triton X-100 was used to improve the permeabilization of the cells. The nuclei of cells were stained using Hoechst 33342 for 10-min incubation at room temperature (RT) free of light. The proliferation rate was assessed by imaging with a fluorescence microscope DMi8 Microsystems GmbH (Leica, Wetzlar, Germany).
Cell migration assay
The scratch-wound assay was used for evaluating the migration of bMEC. Confluent cells grown in a six-well plate were linearly scratched using a micropipette tip as selected treatments. The interval gap of the cells was monitored at 0, 12, and 24 h after scratching. A phase-contrast microscope was used for capturing images (Nikon, Tokyo, Japan). ImageJ software was used for evaluating the wound closure rate (LOCI). Experiments were performed in triplicate.
ELISA for cytokine analysis
For the experiment in vitro, the level of TNF-α and IL-6 in the cultured medium was measured by ELISA, which was conducted using the DuoSet ELISA bovine IL-6 (R&D Systems, Minneapolis, MN, USA) and DuoSet ELISA bovine TNF-α (R&D Systems, Minneapolis, MN, USA) kits in accordance with the manufacturer’s instructions.
For the experiment in vivo, contents of TNF-α, IL-1β, and IL-6 in murine mammary gland were quantified by using a commercial Quantikine ELISA kit for mice (MLB00C, MTA00B, and M6000B, respectively, R&D Systems, Minneapolis, MN, USA) according to the manufacturer’s instructions. The evaluation reports for the sensitivity and specificity of these kits could be found at https://www.rndsystems.com/.
Reactive oxygen species, malondialdehyde, glutathione peroxidase, and total antioxidative capacity determination
The level of reactive oxygen species (ROS) production in all groups of cells was determined using an intracellular staining kit that contains labeled 2′,7′-dichlorofluorescein diacetate (DCFH-DA) (Beyotime, Shanghai, China) and was captured by DMi8 Microsystems BmbH (Leica, Wetzlar, Germany). MDA, glutathione peroxidase (GSH-Px), and total antioxidative capacity (T-AOC) were determined at 532, 420, and 520 nm of absorbance, respectively. The results were recorded by using a spectrophotometric diagnostic kit according to the manufacturer’s instructions (Jiancheng Technology, Nanjing, China).
Oil red O staining
The formation of lipid droplets was stained by oil red O using the commercial kit (C0157S, Beyotime, Beijing, China). Briefly, cells grown in a 12-well plate with crawling slides were treated according to the experiment design. The crawling slides were dried and stained with oil red O dye at RT for 30 min. The cell nucleus was dyed dark blue, and the other section of the cell was dyed light blue using Hematoxylin Staining Solution (C0107B, Beyotime, Beijing, China). A phase-contrast microscope was used for capturing images (Nikon, Tokyo, Japan).
RNA extraction and qRT-PCR
Total RNA was extracted from cells using an RNA-easy Isolation Reagent (Vazyme, Nanjing, China) according to the manufacturer’s instruction. The obtained RNA quality was assessed using a 2100 bioanalyzer (Agilent Technologies, Santa Clara, CA, USA), and the RNA quality number (RQN) was >7.0 for all samples. cDNA was synthesized by HiScript III RT SuperMix (R323-01, Vazyme, China), and AceQuniversal SYBR Master Mix for qPCR was used in the qRT-PCR experiment (Vazyme, China) on an ABI QuantStudio PCR system (Applied Biosystems, Foster City, CA, USA). Primers involved in the present study were described previously (36, 37). Internal control genes including GAPDH, UXT, and RPS9 were selected for normalization of each target gene’s expression by using geometric mean. The selection of the internal genes was illustrated in bMECs by previous publications (38). The 2−ΔΔCt approach was utilized for relative quantification (39).
Western blotting
Western blotting was conducted as previously described (10). Briefly, extracted total protein from bMECs using radioimmunoprecipitation assay (RIPA) lysis buffer (Beyotime, Shanghai, China) was quantified with bicinchoninic acid (BCA) measurement (Pierce, Rockford, IL, USA). The protein was adjusted to an equal amount for the separation on 4%–20% sodium dodecyl sulfate (SDS)–polyacrylamide gels. The separated proteins were then transferred onto nitrocellulose-made membranes (Millipore, Billerica, MA, USA) and incubated with target primary antibodies (Cell Signaling Technology (CST), Danvers, MA, USA) overnight at 4°C on the rotator. The primary antibodies for phospho-p65, p65, TLR4, MYD88, Nrf2, SOD1, SOD2, Gpx1, PPARγ, FASN, SCD, CPT1α, and internal reference (GAPDH) were commercially obtained from Cell Signaling Technology (#3033, #8242, #14358, #4283, #12721, #37385, #13141, #3286, #2435, #3180, #2794, #97361, and #5174) and diluted as 1:1,000. Primary antibodies for SREBP1 and PPARα were obtained commercially from Abcam (Cambridge, UK; ab28481, ab126285) and diluted as 1:2,000. The blots were incubated with horseradish peroxidase-coupled (HRP) secondary antibodies with a dilution of 5,000 (#7074, CST) after moderate washing with TBST. The intensity of GAPDH was taken as normalization of each blot for quantification. The blots were analyzed and quantified by using ImageJ software (LOCI).
Immunofluorescence observation
Cells were seeded onto a crawling slide (φ20mm) installed with a 12-well plate; 4% paraformaldehyde was used to fix cells for 15 min after the selected treatments, and the wells were washed three times with PBS and incubated with Triton-X (0.5%) for 15 min at RT. Slides were then blocked by incubating with bovine serum albumin (BSA) (5%) at 37°C for 1 h. A primary antibody (targeting the p65) was used to incubate cells at 4°C overnight. Fluorescein isothiocyanate (FITC)-labeled secondary antibody was used for staining the cells. After three times washing with PBS, DAPI (1 μg/ml, D8417, Sigma-Aldrich) was used for staining of nuclei for 5 min at RT. DMi8 Microsystems BmbH was used for capturing images (Leica, Wetzlar, Germany).
Statistical analysis
mRNA and protein expression data were log-2 back-transformed for ease of interpretation and reported in the figures. Data were analyzed using one-way ANOVA with Dunnett’s post-hoc test by SAS Statistics (v. 9.2, SAS Institute Inc., Cary, NC, USA). Data were expressed as means ± SEM. p-Values <0.05 were considered statistically significant differences. Experiments were performed in triplicate.
Results
Bactericidal effect of caffeic acid on Gram-negative bacteria
To evaluate the inhibitory effect of CA on pathogens derived from bovine mastitis, we selected isolates from the clinical and standard strains for MIC and MBC determination, the results of which are listed in Table 1. The MIC of CA against E. coli strains ranged from 250 to 500 μg/ml, and the MBC was 500 μg/ml. For K. pneumoniae strains, the MIC of CA was 500 μg/ml, whereas the MBC was from 500 to 1000 μg/ml. P. aeruginosa strains showed less sensitivity to CA, as compared to other Gram-negative bacteria, as a result of MIC being at 1,000 mg/ml and MBC being at 1,000 to 2,000 μg/ml. As bacteria that induce bovine mastitis, S. aureus strains presented a similar sensitivity to CA, with MIC and MBC ranging from 250 to 500 μg/ml.
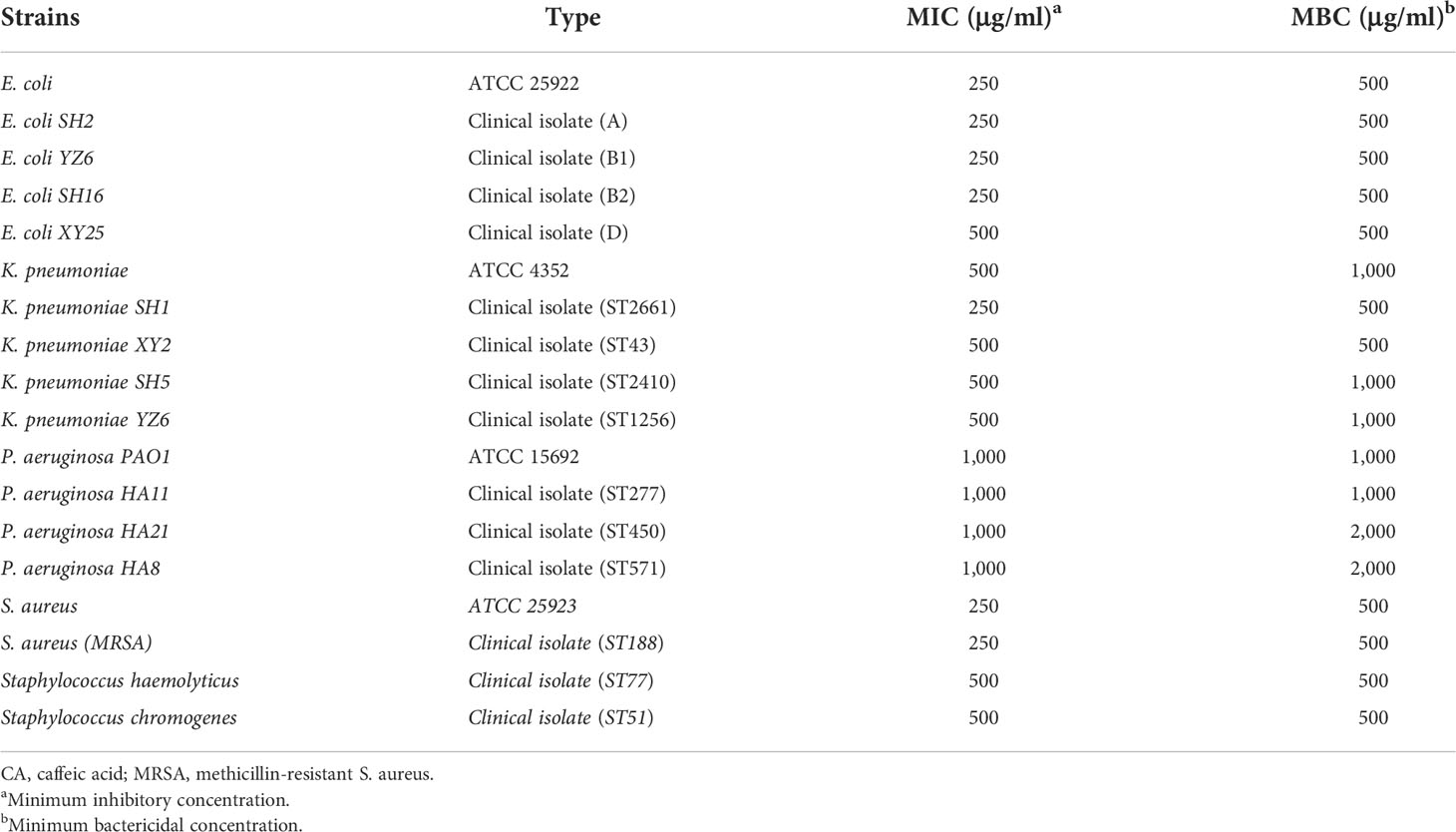
Table 1 MIC and MBC of CA for clinical isolated Gram-positive S. aureus and gram-negative Escherichia coli, Klebsiella pneumoniae, and Pseudomonas aeruginosa.
To further validate the antibacterial activity of CA, we tested the most sensitive types (lower MIC and MBC) of E. coli, K. pneumoniae, P. aeruginosa, and S. aureus strains isolated from milk (Figure 1A). The growth curve showed that E. coli B1 did not grow at concentrations of CA higher than 250 μg/ml, while 0.5× MIC (125 μg/ml) did not eliminate the activity of E. coli B1 as compared to the higher doses. Concentrations of CA less than 500 μg/ml exhibited no effect on bacterial activity in the coculture of P. aeruginosa PAO1. Consistently, no values were observed at 1,000 μg/ml of CA, which is consistent with the results of MIC on P. aeruginosa PAO1. The concentration of CA at 500 μg/ml significantly blocked the growth of K. pneumoniae SH1 (ST2661). Notably, the inhibitory effect of CA on Gram-positive methicillin-resistant Staphylococcus aureus (MRSA) appeared to be dose-dependent during a 24-h coculture. In summary, these results demonstrate the potential antibacterial efficacy of CA against pathogenic bacteria, particularly E. coli B1.
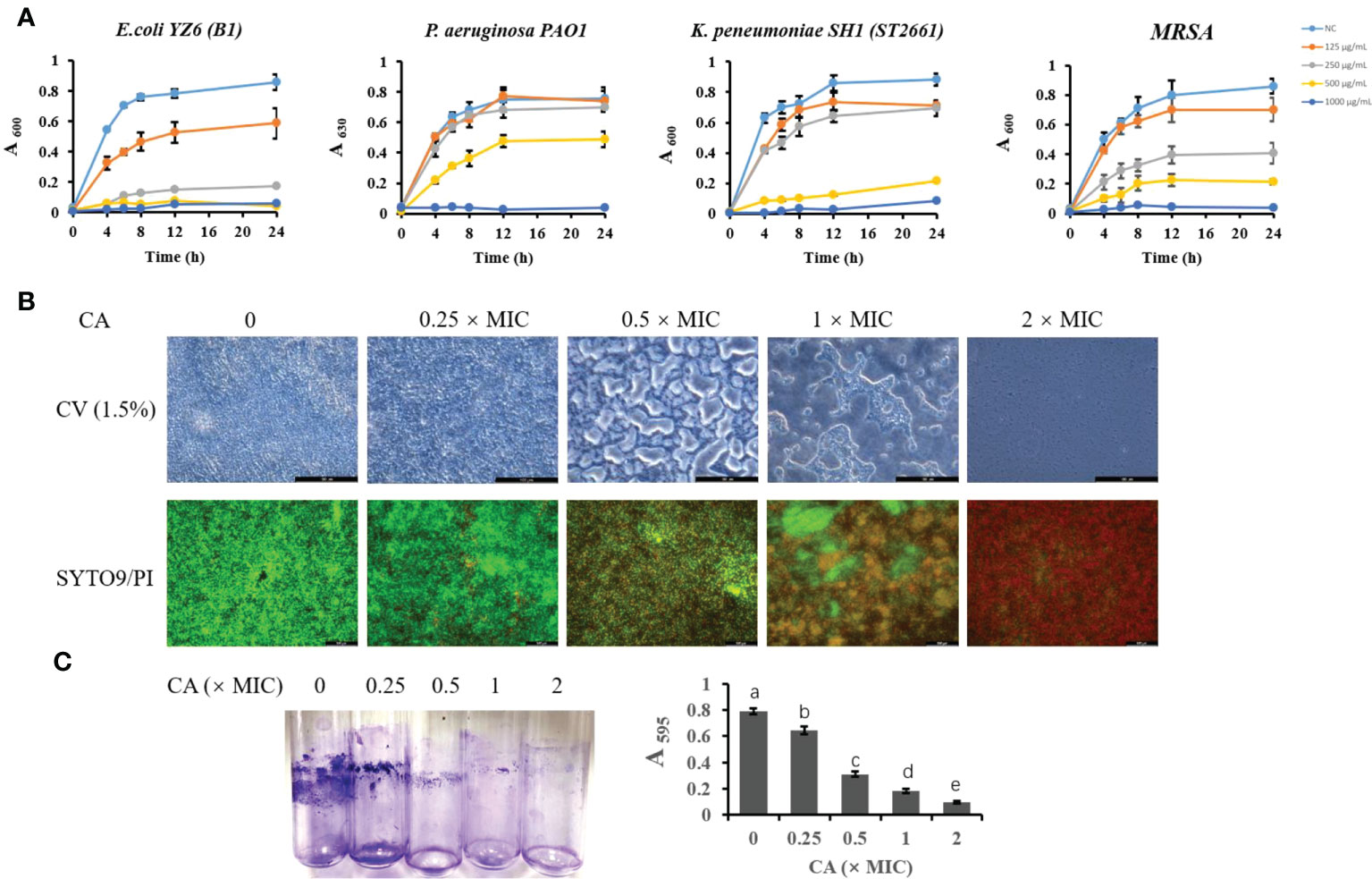
Figure 1 The inhibitory effect of CA on bacterial growth and Escherichia coli biofilm formation. (A) Effect of CA supplementation on growth of E. coli B1, PAO1, Klebsiella pneumoniae, and MRSA in LB medium for 24h. (B) The effect of CA on E. coli B1 biofilm formation. The inhibitory effect of CA on biofilm growth was determined using CV (1.5%) staining, while eradication of matured biofilm by CA was determined using SYTO9 (green) and PI (red) staining. (C) Biomass of biofilm formation was determined in tubes and quantified using the absorbance measured at 595 nm. The letters in superscript indicate that the difference between groups was significant (p < 0.05). Data are presented as mean ± SEM. The results are representative of three independent experiments. CA, caffeic acid; MRSA, methicillin-resistant Staphylococcus aureus; LB, Luria broth; CV, Cresyl Violet; PI, propidium iodide.
Caffeic acid disrupted the biofilm formation of Escherichia coli B1
The biofilm formation of bacteria is significantly relevant to increases in drug resistance. CV staining is applied to not only bacteria but also the extracellular matrices of biofilms. In the present study, an increase in CA concentration gradually decreased the biomass of biofilm in the E. coli B1 strain (Figures 1B, C). Notably, when the concentration of CA was at 2× MIC (500 μg/ml), biofilm formation was completely inhibited. A LIVE/DEAD staining assay showed that an increased CA concentration eradicated mature biofilm as a result of the decreased viability of cells. The concentration of CA at 2× MIC (500 μg/ml) completely eliminated cells in the biofilm matrix, which is consistent with the use of MBC on E. coli B1.
Caffeic acid reduced Escherichia coli adhesion of host epithelial cells
To determine the adhesion activity of E. coli B1 and the inhibitory effect of CA on the adhesion of E. coli in bMECs, in the present study, we determined the adhered bacterial counts. As shown in Figure 2A, pHrodo green-probed E. coli adhered to bMECs with a strong stain, while CA inhibited the adhesion of E. coli after the amount added to the cocultured medium was increased. Counting bacteria for adhesion activity consistently showed that an increased amount of CA significantly reduced the adhesion on bMECs with a decreased CFU plated on an MH agar plate (Figure 2B).
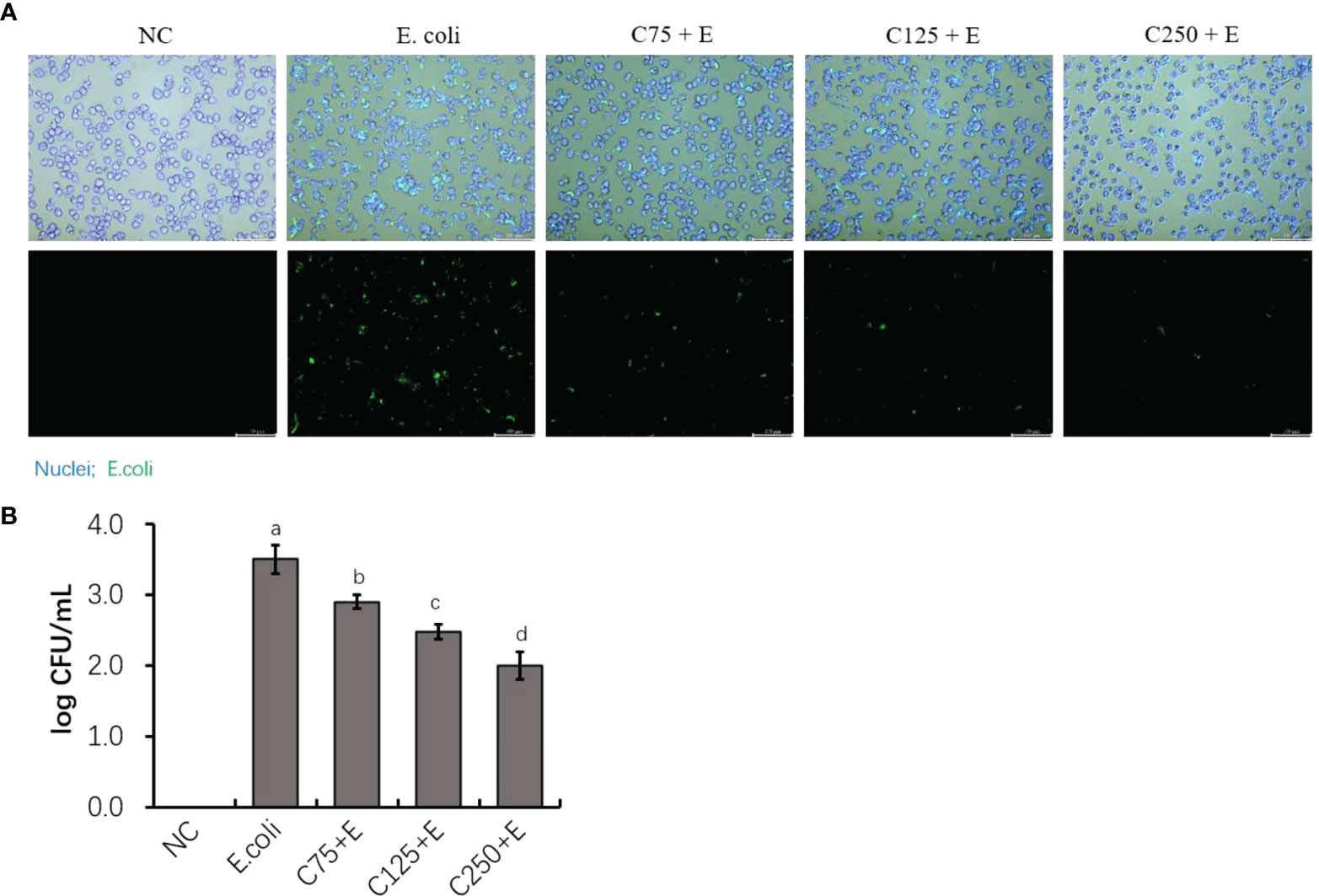
Figure 2 The inhibition of adherence property of Escherichia coli B1 in bMECs by CA supplementation. (A) Image of bacterial adhesion in bMEC. Cells were infected with bacteria for 3 h, along with supplementation of CA at doses of 75, 125, and 250 μg/ml. Nuclei were counterstained with Hoechst 33342 (blue), while E. coli were labeled with pHrodo (green). Scale bar = 100 μm. (B) Counting for adhered bacteria in bMEC. Cells were seeded on the 12-well plates, and 1 × 106 colony-forming unit (CFU) bacteria and increasing doses of CA at 75, 125, and 250 μg/ml were cocultured with bMECs at 37°C for 3h. The final CFUs were determined by counting the colonies after incubation at 37°C for 18.h. Data are presented as mean ± SEM. The results are representative of three independent experiments. The letters in superscript indicate that the difference between groups was significant (p < 0.05). Data are representative of three independent replicates. bMECs, bovine mammary epithelial cells; CA, caffeic acid.
Impeded cell proliferation was recovered by supplementation with caffeic acid
To determine the proliferation activity of bMECs exposed to different treatments in the present study, we used scratch wound assays and EdU staining (Figure 3). E. coli infection inhibited cell growth by reducing cell migration at both 12 and 24 h. Pretreatment with CA at 125 μg/ml restored less cell activity than at 250 μg/ml, which indicated that the effect of CA on bMEC proliferation is dose dependent. The results of the EdU assay consistently showed that pretreatment with CA at 125 or 250 μg/ml displayed strengthened EdU staining as compared to the E. coli group (p < 0.05), and the effect was dose-dependent.
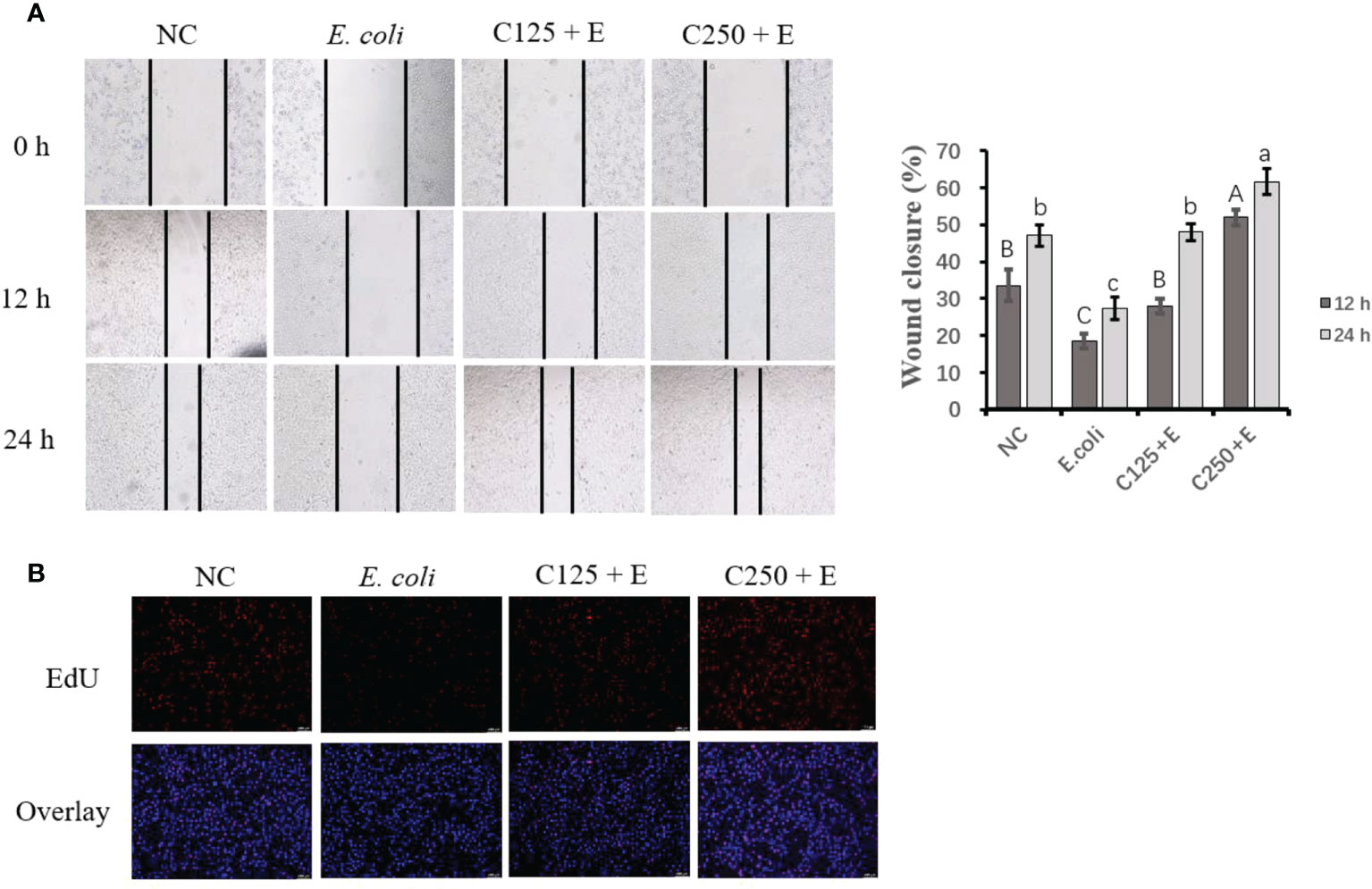
Figure 3 The effect of CA pretreatment on cell proliferation followed by Escherichia coli B1 stimulation. (A) Wound healing assay was performed for the migration ability of bMEC; the closure rate was imaged at 0, 12, and 24 h after scraping. (B) EdU determination for cell proliferation. Cells were observed in ×200 magnification (scale bar = 100 μm). Cells were pretreated with CA at doses of 125 or 250 μg/ml for 24 h, followed by the induction of E. coli B1 for 6h. Results are expressed as means ± SEM. Letters in lowercase indicate difference between groups at 12 h, while uppercase letters indicate difference between groups at 24 h (p < 0.05). CA, caffeic acid; bMEC, bovine mammary epithelial cell; EdU, 5-ethynyl-2′-deoxyuridine.
Pretreatment with caffeic acid inhibited proinflammatory responses in bovine mammary epithelial cells induced by Escherichia coli
To uncover the protective effect of CA on responses to E. coli stimulation in bMECs, we determined the expression of mRNA and proteins related to proinflammation. Cells infected with E. coli upregulated the mRNA expression of TLR4, MYD88, IL6, and IL1B. However, pretreatment with CA at 125 or 250 μg/ml significantly attenuated the expression of these genes as compared to those in the E. coli group (p < 0.05) (Figure 4C). Moreover, the expression of proteins related to TLR4 signaling was in agreement with the results of our gene expression determination. Meanwhile, an ELISA verified the downstream factors that are regulated by TLR4/NF-κB signaling, and the increased secretion of TNF-α and IL-6 in the cultured medium was inhibited by pretreatment with CA at 125 or 250 μg/ml (p < 0.05) (Figure 4B). Notably, the elevated ratio of phosphorylated p65 to total p65 in the E. coli group was lowered by the CA pretreatment at 125 or 250 μg/ml in a dose-dependent manner (Figure 4D). The results consistently showed that cells pretreated with CA at 125 or 250 μg/ml, followed by E. coli infection, weakened the staining of p65 as compared to that of the E. coli group (Figure 4A).
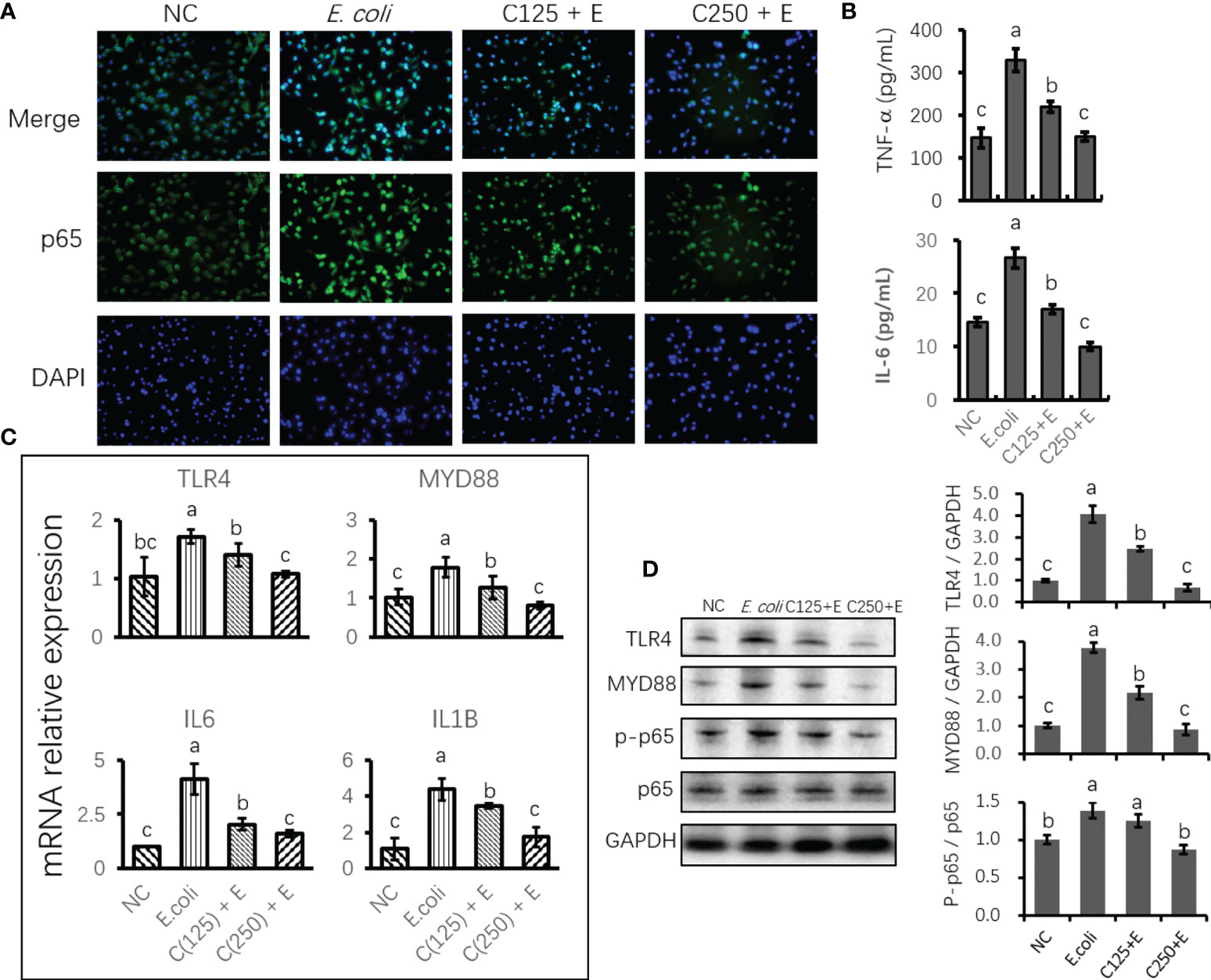
Figure 4 The effect of CA pretreatment on Escherichia coli-induced proinflammatory signaling components. (A) Immunofluorescence of p-p65 proteins expressed in bMECs and imaged using DMi8 Microsystems BmbH. Cells were observed at ×200 magnification. (B) Determination of the concentration of TNF-α and IL-6 in cultured medium using ELISA test. (C) The mRNA expression of TLR4 and NF-κB signaling genes. Abundance of each gene was normalized by the geometric mean of the internal control genes (GAPDH, UXT, and RPS9). Abundance of genes in the NC group was set as 1.0. (D) Immunoblots were determined for the expression of proteins related to TLR4 and NF-κB signaling. Experiments were performed as three independent replicates. Results are expressed as means ± SEM. Letters in superscript indicate that the difference between groups was significant (p < 0.05). CA, caffeic acid; bMECs, bovine mammary epithelial cells; NC, negative control.
Escherichia coli-induced oxidative stress in bovine mammary epithelial cells was attenuated by caffeic acid pretreatment
The E. coli-induced production of ROS in bMECs was demonstrated by the increased staining of intracellular ROS in the E. coli group (Figure 5A). However, ROS production was inhibited by CA supplementation as a result of reduced staining in cells pretreated with CA at 125 or 250 μg/ml. Similarly, E. coli stimulation enhanced the concentration of MDA, and pretreatment with 125 and 250 μg/ml of CA significantly suppressed the accumulation of MDA as compared to the E. coli group. Regarding the antioxidative capacity (Figure 5B), GSH-Px and T-AOC activity were induced by E. coli infection, whereas CA pretreatment improved the antioxidant enzyme activity by increasing GSH-Px and T-AOC activity compared to the E. coli group.
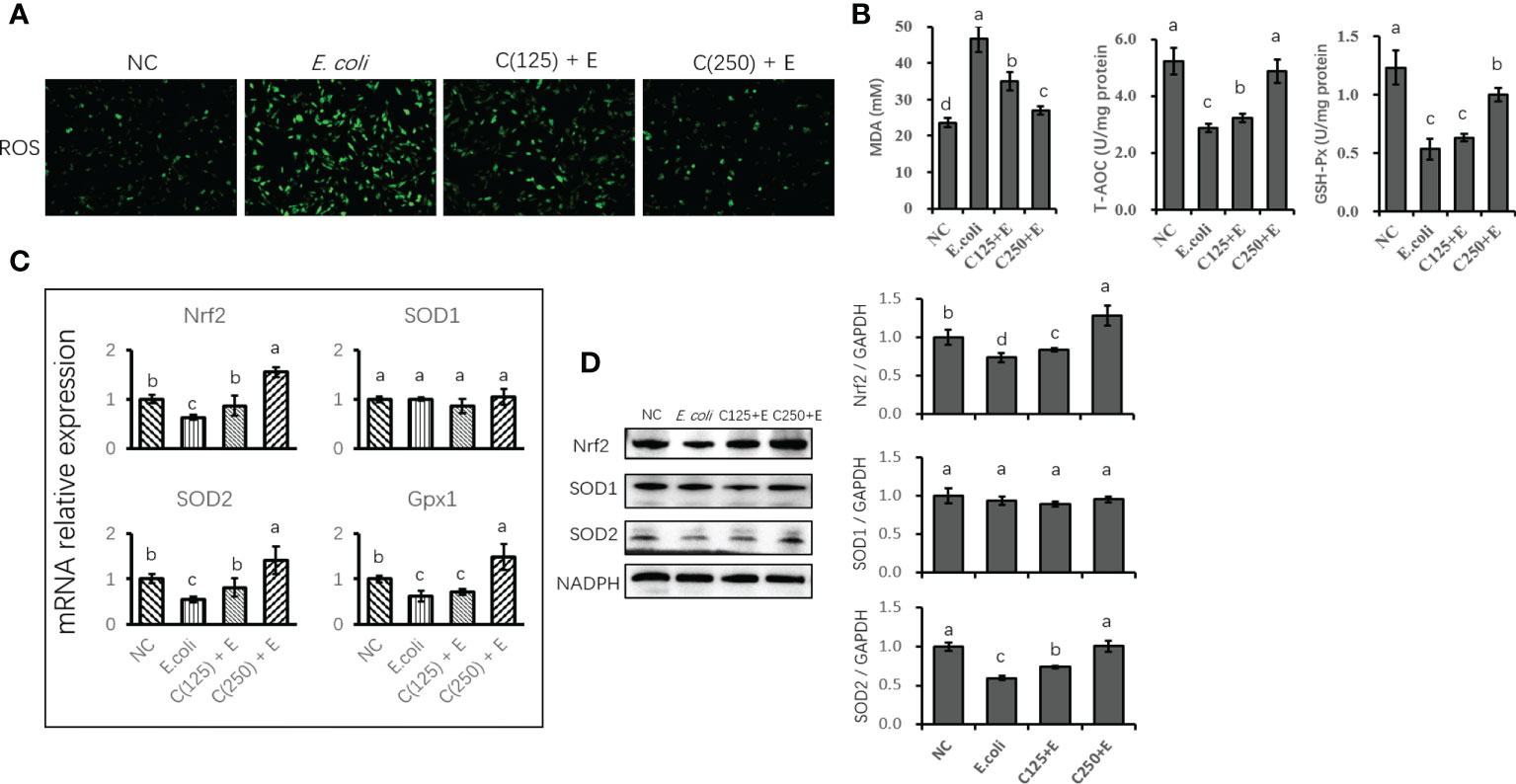
Figure 5 The antioxidative effect of CA pretreatment on cells stimulated with Escherichia coli. (A) DCFH-DA probe for intracellular ROS content was captured and determined. Cells were observed in a ×200 magnification. (B) The absorbance of intensity for MDA, GSH-Px, and T-AOC was obtained at 532, 420, and 520 nm, respectively. (C) The expression of genes related to antioxidants and Nrf2 signaling. Abundance of each gene was normalized by the geometric mean of the internal control genes (GAPDH, UXT, and RPS9). Abundance of genes in NC group was set as 1.0. (D) Immunoblots were determined for the expression of proteins related to antioxidants and Nrf2 signaling. Experiments were performed independently in triplicate. Results are expressed as means ± SEM. Letters in superscript indicate that the difference between groups was significant (p < 0.05). CA, caffeic acid; DCFH-DA, 2′,7′-dichlorofluorescein diacetate; ROS, reactive oxygen species; MDA, malondialdehyde; GSH-Px, glutathione peroxidase; T-AOC, total antioxidative capacity; NC, negative control.
The expression of genes related to antioxidants was determined using qPCR (Figure 5C). E. coli infection destroyed the antioxidant capacity of bMECs by reducing the expression of antioxidant enzyme-encoding genes (Nrf2 and SOD2). Pretreatment with CA at 125 or 250 μg/ml reversed the suppression of Nrf2 and SOD2 gene expression. However, SOD1 gene expression was not affected by either E. coli stimulation or CA supplementation as compared to the control group (p < 0.05). Additionally, the protein expression of Nrf2 and SOD2 was consistent with the gene expression compared to the results of the reversed downregulation of Nrf2 and SOD2 induced by E. coli infection (Figure 5D).
Caffeic acid protected bovine mammary epithelial cells from the detrimental effects of Escherichia coli infection on lipid homeostasis
We further studied the effect of CA on the E. coli-induced alteration of lipid metabolism (Figure 6). The expression of genes and proteins related to fatty acid anabolism was downregulated by E. coli infection, including SREBP1, SCD, FAS, and PPARγ. However, pretreatment with CA at 125 or 250 μg/ml reversed the suppression of lipogenic genes and proteins induced by E. coli in a dose-dependent manner. In contrast, genes and proteins associated with lipid catabolism were upregulated by the E. coli infection, whereas pretreatment with CA inhibited the expression of PPARα and CPT1, as compared to the E. coli group in Figures 6A, C (p < 0.05). Regarding the secretion of cellular lipids in Figure 6B, E. coli infection in bMECs abolished lipid production in relation to oil red O staining, as compared to the control group (p < 0.05). In contrast, bMECs pretreated with CA at 125 and 250 μg/ml recovered their lipid synthesis following E. coli stimulation.
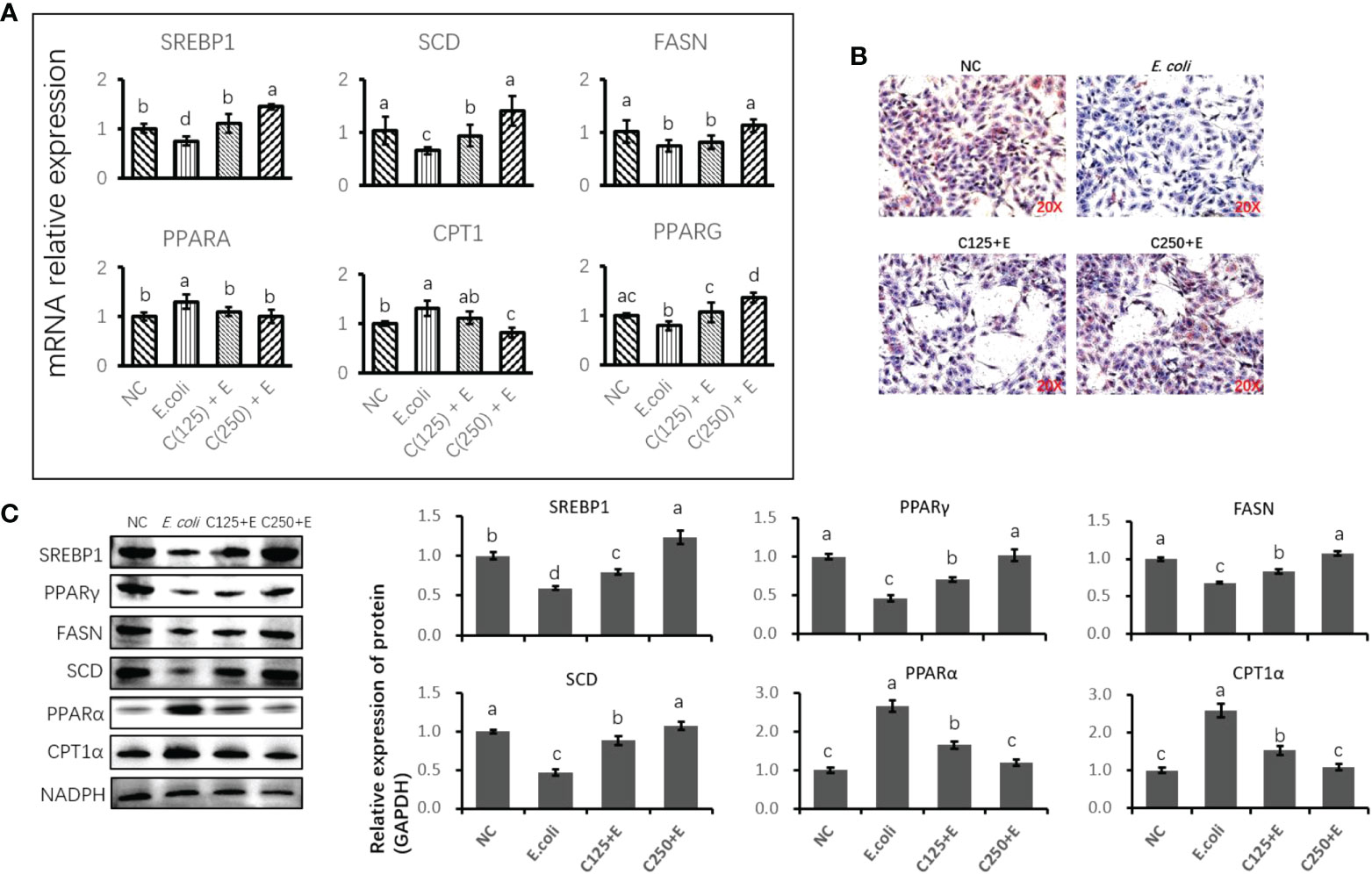
Figure 6 The effect of CA pretreatment on lipid metabolism in bMECs challenged by Escherichia coli. (A) The expression of genes related to lipid metabolism. Abundance of each gene was normalized by the geometric mean of the internal control genes (GAPDH, UXT, and RPS9). Abundance of genes in NC group was set as 1.0. (B) Lipid accumulation in bMECs by using oil red O staining assay. Cells were observed in ×200 magnification. (C) Immunoblots were determined for the expression of proteins related to lipid metabolism. Experiments were performed independently in triplicate. Results are expressed as means ± SEM. Letters in superscript indicate that the difference between groups was significant (p < 0.05). CA, caffeic acid; bMECs, bovine mammary epithelial cells; NC, negative control.
Mouse lethality and histological alterations in mammary gland
As shown in Figure 7A, the injection of E. coli or K. pneumoniae induced an 80% murine mortality rate within 18 h. However, pretreatment with CA at 50 mg/kg before an E. coli or K. pneumoniae injection significantly enhanced the viability of mice, with an increased survival rate of 30% or 40% as compared to the infected groups (Figure 7A). Regarding histological changes in mammary tissue (Figure 7B), the E. coli-infected group displayed evident histopathologic abnormalities as compared to the control group, including acinar congestion, inflammatory cell infiltration, and flocculus injury. In contrast, pretreatment with CA at 25 or 50 mg/kg significantly alleviated the histological changes induced by E. coli. Histopathological changes in the mammary glands were evaluated according to their injury degree score, including the integrity of mammary tissues and the infiltrated inflammatory cells. The pathological score was evaluated on a scale of 0–5, as described previously (40).
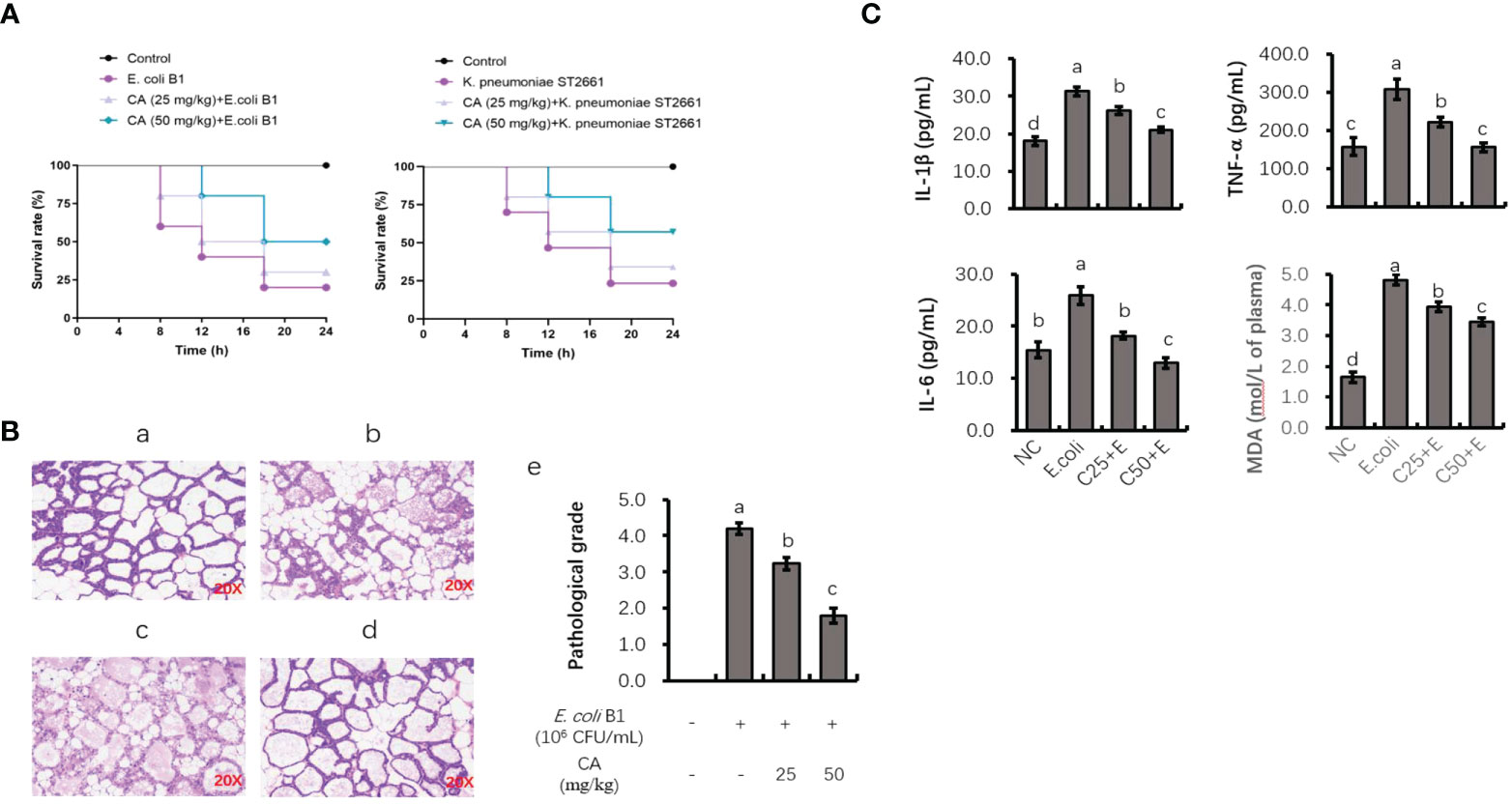
Figure 7 The protective role of CA on pathogenic bacteria-induced lethality and mammary tissue damage. (A) Mortality of mice administrated with CA followed by Escherichia coli or Klebsiella pneumoniae challenge. Mice were intraperitoneally injected with E. coli or K. pneumoniae, with oral administration of CA at 25 and 50 mg/kg for 10 days prior to E. coli or K. pneumoniae injection. (B) H&E staining of the mammary tissue after E. coli injection. Images from each experimental group were original magnification by ×200. (a) Control group; (b) mice treated with intramammary E. coli; (c, d) CA treated (25 and 50 m/kg) followed by E. coli injection; (e) evaluation of pathological grade. (C) Concentrations of IL-1β, TNF-α, and IL-6 in mice mammary tissue and peripheral content of MDA. Letters in superscript indicate that the difference between groups was significant (p < 0.05). CA, caffeic acid; MDA, malondialdehyde.
Analysis of TNF-α, IL-1β, and IL-6 levels in mouse mammary tissue and malondialdehyde content in peripheral plasma
To investigate the anti-inflammatory role of CA in vivo, the effects of CA on the E. coli B1-induced production of proinflammatory cytokines (TNF-α, IL-1β, and IL-6) in mammary tissue were studied (Figure 7C). The production of TNF-α, IL-1β, and IL-6 was significantly elevated in the E. coli-infected group, whereas mice administrated with CA at both 25 and 50 mg/kg reversed the production of these cytokines in a dose-dependent manner (p < 0.05). Meanwhile, CA supplementation suppressed the content of MDA in peripheral plasma, which was increased in the E. coli-infected mice.
Discussion
The sharp increase in bovine mastitis associated with MDR bacterial pathogens has become a serious issue for the dairy industry and a threat to public health, especially with respect to Gram-negative pathogens. Therefore, developing alternative strategies other than antibiotics to interfere with interactions between pathogenic bacteria and host-cell immune responses is a promising approach to circumvent this problem. The present study investigated the protective effects of CA in terms of antibacterial activities and immune-boosting bMECs. CA eradicates bacterial activity by disrupting both biofilm formation and the adhesion/internalization of the host’s epithelial cells. Meanwhile, CA supplementation enhances the host cell’s defenses by deactivating inflammatory and oxidative responses, as well as reprogramming lipid homeostasis in bMECs infected with E. coli B1. In addition, we demonstrated that the administration of CA in mice is effective in combating mammary tissue damage and systemic inflammation induced by clinically isolated Gram-negative bacterial infections.
Caffeic acid and its derivatives have been found to exhibit antimicrobial activity toward a diverse range of pathogenic microorganisms, including Gram-negative and Gram-positive bacteria. For instance, CA potentiates an antibacterial effect on S. aureus strains isolated from infected wounds alone and in combination with antibiotics–phytochemicals with a MIC range of 256–1,024 μg/ml (41). In addition, phenolic compounds, including CA, have been suggested to exert antimicrobial activity by inhibiting Enterobacteriaceae growth in the digestive tract (41). However, the preventive and therapeutic potential of CA in bacterial infections, such as bovine mastitis, is still elusive. In the present study, we selected prevalent strains isolated from milk produced by cows with clinical bovine mastitis to determine the MIC and MBC of CA. As stated in a previous study, E. coli and K. pneumoniae are the most isolated bacteria in Chinese farms (12), and the MIC and MBC values for MDR E. coli and K. pneumoniae in this study indicate that CA is effective in inhibiting Gram-negative MDR bacteria. Moreover, the growth curves validate the inhibitory effects of CA on the activities of E. coli and K. pneumoniae. However, a better understanding of the pharmacological mechanisms is necessary to determine which CA acts as an antibacterial agent.
It is notable that antimicrobial actions mainly occur when changing the permeability of cell membranes, enzyme activity, biofilm formation, or the structure of proteins and DNA, as well as blocking the adhesion or internalization of host cells. In the case of bovine mastitis infections caused by pathogenic bacteria, biofilm penetrating the teat and sticking to the internal surface of mammary tissue is suggested to help develop MDR in E. coli strains (42, 43). Therefore, taking into consideration the blocking of biofilm formation to potentiate the elimination of troublesome pathogens is one way of finding alternatives to antibacterial agents. In this study, a 2× MIC of CA was found to curb the formation of biofilm and eradicate already formed, mature biofilm by killing bacteria. Therefore, when considering the increasing emergence of MDR bacteria, CA is an agent that can reduce the difficulty of using antibiotic treatments. However, unlike other studies, we found that combination therapies using CA and antimicrobial agents such as cephalothin, imipenem, lincomycin, and penicillin showed no synergetic effect on E. coli inhibition (data not shown).
Except for biofilm protection, the adhesion/internalization of extracellular bacteria such as E. coli is believed to enhance bacterial survival by increasing antibiotic tolerance in the host cell’s cytosol (26, 44). As such, the inhibited adhesion or internalization of E. coli caused by increasing the amount of CA indicates that CA is capable of reducing interactions between E. coli and bMECs and, therefore, the invasion of cells. However, the mechanism by which CA blocks the adherence properties of pathogenic bacteria in epithelial cells needs to be further investigated.
Generally, the host triggers inflammatory responses to reduce the detrimental effects induced by pathogen-associated molecular patterns (PAMPs) such as LPS, the major constituent of Gram-negative bacteria walls. E. coli strains usually cause acute coliform mastitis, and they play an important role in mammary epithelial cell impairment when the bacteria are lysed by immune cells such as phagocytes. To detect signs of proinflammatory molecule changes, the components of the TLR4/NF-κB signaling pathway can be monitored by using an E. coli B1-infected cell model. In the present study, the supplementation of CA downregulated TLR4/NF-κB signaling components induced by E. coli B1 infection, which is consistent with downstream-regulated cytokine cascades such as IL-6 and TNF-α. Moreover, it has been reported that CA can prevent LPS-induced bMEC inflammatory injuries via NF-κB and MAPK activation (30). Along with its role in prohibiting the translocation of p65 into the nucleus, the current study additionally validated the role of CA in protecting bMECs from pathogen-directed infections by inhibiting TLR4/NF-κB signaling.
Oxidative stress-induced tissue injuries manifest as Nrf2 signaling activation due to interactions with NF-κB-mediated inflammation. Accumulated levels of oxygen-free radicals, such as ROS, and assessments of the antioxidant capacity of cells are used as indicators of oxidative damage. During LPS-induced acute phase responses, macrophages sensitive to the LPS-induced generation of ROS seem to be regulated by the MAPK and NF-κB transduction pathways. Similarly, bMECs and macrophages infected with extended-spectrum beta-lactamase (ESBL) E. coli have been reported to show signs of oxidative stress via their interactions with TLR4/NF-κB pathway (45). In the present study, the decreased capacity of antioxidants caused by stimulating bMECs with E. coli B1 was reversed using a CA pretreatment, as indicated by changes in antioxidative enzyme activities. Hence, the result is in agreement with studies finding that CA is a viable alternative for ameliorating a wide spectrum of disorders with its anti-inflammatory and antioxidation properties (46).
As one of the main functions of bMECs is the production of milk fat, we evaluated the regulatory effect of CA on the E. coli B1-induced imbalance of fatty acid metabolism. The intracellular content of lipids refers to the accumulation of packaged lipid droplets, which are the precursors of the fat that is secreted into milk. In the present study, expressions of genes and proteins related to fatty acid synthesis were downregulated, whereas the expression of fatty acid oxidation-associated genes and proteins was upregulated by E. coli infection. Lipid metabolism in bMECs infected with E. coli appears to switch from fatty acid anabolism to catabolism since activating the inflammatory and acute phase responses requires significant amounts of energy. Moreover, in conditions of pathogenic bacteria infection, lipogenesis and lipolysis indicate an inverse relationship in bMECs between the immune response and milk fat synthesis (47). The present study reveals that CA pretreatment is capable of controlling the lipid homeostasis of bMECs, as well as restoring lipogenesis, which is impeded by the stimulation of pathogens. Collectively, this provides evidence that CA promotes lipid synthesis along with a decrease in lipid oxidation, which may contribute to the detoxification of oxidative stress and a reduction in the inflammatory responses induced by E. coli B1 infection.
Since the efficacy of CA’s antimicrobial and protective effects in host bMECs was validated in vitro, we conducted an in vivo experiment by administrating CA and E. coli or K. pneumoniae to mice in order to determine survival rates. Infecting mice with E. coli or K. pneumoniae strains led to a 20% mortality rate; however, the survival rate was elevated by the administration of CA. Clinically isolated E. coli strains induce murine mastitis, which is believed to elicit histological changes along with the elevated expression of proinflammatory genes (48). After injecting E. coli or K. pneumoniae strains into the lactating mammary tissue of mice, serious damage to histological integrity induced by this pathogenic infection was consistently treated by CA administration. Moreover, the acute phase responses concurred with the elevated cytokines in the mammary tissue, and MDA content in peripheral plasma was attenuated by administrating CA. These results indicate that CA can effectively reduce inflammation in mouse mammary glands induced by Gram-negative bacteria.
In summary, versatile CA possesses antimicrobial and immune-boosting properties, coupled with an ability to interfere with interactions between pathogenesis and host-cell responses, suggesting that it represents a promising alternative to tackle pathogenic bacteria-infected bovine mastitis. Moreover, the verification of CA in the development of potential therapeutic agents for bovine mastitis will encourage work into uncovering more candidates for non-antibiotic treatment. Nevertheless, more clinical trials are necessary to verify the potential activity of CA in the treatment of bovine mastitis.
Data availability statement
The datasets presented in this study can be found in online repositories. The names of the repository/repositories and accession number(s) can be found in the article/Supplementary Material.
Ethics statement
This study was reviewed and approved by the Animal Experiment Committee of Yangzhou University.
Author contributions
TX and ZY provided the study concept and design. ZY and TX developed the methodology and wrote, reviewed, and revised the paper. TX, GC and RL acquired, analyzed, and interpreted the data and performed statistical analysis. HZ and YY provided technical and material support. All authors contributed to the article and approved the submitted version.
Funding
This study was supported by the National Natural Science Foundation of China (32102731, 31872324) and the Open Project Program of Joint International Research Laboratory of Agriculture and Agri-Product Safety, the Ministry of Education of China, Yangzhou University (JILAR-KF202204).
Conflict of interest
The authors declare that the research was conducted in the absence of any commercial or financial relationships that could be construed as a potential conflict of interest.
Publisher’s note
All claims expressed in this article are solely those of the authors and do not necessarily represent those of their affiliated organizations, or those of the publisher, the editors and the reviewers. Any product that may be evaluated in this article, or claim that may be made by its manufacturer, is not guaranteed or endorsed by the publisher.
Supplementary material
The Supplementary Material for this article can be found online at: https://www.frontiersin.org/articles/10.3389/fimmu.2022.1005430/full#supplementary-material
References
1. Seegers H, Fourichon C, Beaudeau F. Production effects related to mastitis and mastitis economics in dairy cattle herds. Vet Res (2003) 34(5):475–91. doi: 10.1051/vetres:2003027
2. Vliegher S, Fox LK, Piepers S, McDougall S, Barkema HW. Invited review: Mastitis in dairy heifers: nature of the disease, potential impact, prevention, and control. J Dairy Sci (2012) 95(3):1025–40. doi: 10.3168/jds.2010-4074
3. Giovannini AEJ, van den Borne BHP, Wall SK, Wellnitz O, Bruckmaier RM, Spadavecchia C. Experimentally induced subclinical mastitis: are lipopolysaccharide and lipoteichoic acid eliciting similar pain responses? Acta Vet Scand (2017) 59(1):40. doi: 10.1186/s13028-017-0306-z
4. Esener N, Green MJ, Emes RD, Jowett B, Davies PL, Bradley AJ, et al. Discrimination of contagious and environmental strains of streptococcus uberis in dairy herds by means of mass spectrometry and machine-learning. Sci Rep (2018) 8(1):17517. doi: 10.1038/s41598-018-35867-6
5. Fitzpatrick, Garvey, Gleeson. Comparing the efficacy of teat disinfectant products against bovine mastitis-causing contagiousand environmental bacteria. Adv Anim Biosci (2018) 9(1):196. doi: 10.1017/S2040470018000031
6. Carl-Fredrik J, Josef D, Ann-Marie G, Ida W, Moazzami AA, Karin S, et al. The effect of lipopolysaccharide-induced experimental bovine mastitis on clinical parameters, inflammatory markers, and the metabolome: A kinetic approach. Front Immunol (2018) 9:1487. doi: 10.3389/fimmu.2018.01487
7. Xu T, Liu R, Lu X, Wu X, Heneberg P, Mao Y, et al. Lycium barbarum polysaccharides alleviate LPS-induced inflammatory responses through PPARγ/MAPK/NF-κB pathway in bovine mammary epithelial cells. J Anim Sci (2022) 100(1):skab345. doi: 10.1093/jas/skab345
8. Xu T, Wu X, Lu X, Liang Y, Yang Z. Metformin activated AMPK signaling contributes to the alleviation of LPS-induced inflammatory responses in bovine mammary epithelial cells. BMC Vet Res (2021) 17(1):97. doi: 10.1186/s12917-021-02797-x
9. Xu T, Liu R, Zhu H, Zhou Y, Pei T, Yang Z. The inhibition of LPS-induced oxidative stress and inflammatory responses is associated with the protective effect of (-)-Epigallocatechin-3-Gallate on bovine hepatocytes and murine liver. Antioxidants (2022) 11(5):914. doi: 10.3390/antiox11050914
10. Xu T, Ma N, Yan W, Shi X, Chang G, Loor JJ, et al. Sodium butyrate supplementation alleviates the adaptive response to inflammation and modulates fatty acid metabolism in lipopolysaccharide-stimulated bovine hepatocytes. J Agric Food Chem (2018) 66(25):6281–90. doi: 10.1021/acs.jafc.8b01439
11. Yu ZN, Wang J, Ho H, Wang YT, Huang SN, Han RW. Prevalence and antimicrobial-resistance phenotypes and genotypes of escherichia coli isolated from raw milk samples from mastitis cases in four regions of China. J Global Antimicrobial Resist (2020) 22:94–101. doi: 10.1016/j.jgar.2019.12.016
12. Yang Y, Peng Y, Jiang J, Gong Z, Shang S. Isolation and characterization of multidrug-resistant klebsiella pneumoniae from raw cow milk in jiangsu and Shandong provinces, China. Transbound Emerg Dis (2020) 68(3):1033–39. doi: 10.1111/tbed.13787
13. Ramanan, Deepshika, Cadwell, Ken. Intrinsic defense mechanisms of the intestinal epithelium. Cell Host Microbe (2016) 19(4):434–41. doi: 10.1016/j.chom.2016.03.003
14. Brubaker SW, Monack DM. Cell-intrinsic defense at the epithelial border wall: Salmonella pays the price. Immunity (2017) 46(4):522–4. doi: 10.1016/j.immuni.2017.03.021
15. Döpfer D, Nederbragt H, Almeida RA, Gaastra W. Studies about the mechanism of internalization by mammary epithelial cells of escherichia coli isolated from persistent bovine mastitis. Vet Microbiol (2001) 80(3):285–96. doi: 10.1016/S0378-1135(01)00307-8
16. Günther J, Koczan D, Wei Y, Nürnberg G, Seyfert HM. Assessment of the immune capacity of mammary epithelial cells: comparison with mammary tissue after challenge with escherichia coli. Vet Res (2009) 40(4):448–64. doi: 10.1051/vetres/2009014
17. Gilbert FB, Cunha P, Jensen K, Glass EJ. Differential response of bovine mammary epithelial cells to staphylococcus aureus or escherichia coli agonists of the innate immune system. Vet Res (2013) 44(1):1–23. doi: 10.1186/1297-9716-44-40
18. Blum SE, Heller ED, Jacoby S, Krifucks O, Leitner G. Comparison of the immune responses associated with experimental bovine mastitis caused by different strains of escherichia coli. J Dairy Res (2017) 84(2):190–7. doi: 10.1017/s0022029917000206
19. Chang G, Petzl W, Vanselow J, Günther J, Shen X, Seyfert HM. Epigenetic mechanisms contribute to enhanced expression of immune response genes in the liver of cows after experimentally induced escherichia coli mastitis. Vet J (2015) 203(3):339–41. doi: 10.1016/j.tvjl.2014.12.023
20. Hayden MS, Ghosh S. NF-κB in immunobiology. Cell Res (2011) 21(2):223–44. doi: 10.1038/cr.2011.13
21. Ma N, Wei G, Zhang H, Dai H, Roy AC, Shi X, et al. Cis-9, trans-11 CLA alleviates lipopolysaccharide-induced depression of fatty acid synthesis by inhibiting oxidative stress and autophagy in bovine mammary epithelial cells. Antioxidants (2022) 11(1):55. doi: 10.3390/antiox11010055
22. Quesnell RR, Klaessig S, Watts JL, Schukken YH. Bovine intramammary escherichia coli challenge infections in late gestation demonstrate a dominant antiinflammatory immunological response. J Dairy Sci (2012) 95(1):117–26. doi: 10.3168/jds.2011-4289
23. Dai H, Wei G, Wang Y, Ma N, Chang G, Shen X. Sodium butyrate promotes lipopolysaccharide-induced innate immune responses by enhancing mitogen-activated protein kinase activation and histone acetylation in bovine mammary epithelial cells. J Dairy Sci (2020) 103(12):11636–52. doi: 10.3168/jds.2020-18198
24. Wei Z, Fu Y, Zhou E, Tian Y, Yao M, Li Y, et al. Effects of niacin on staphylococcus aureus internalization into bovine mammary epithelial cells by modulating NF-κB activation. Microbial Pathog (2014) 71–72:62–7. doi: 10.1016/j.micpath.2014.03.005
25. Dego OK, Oliver SP, Almeida RA. Host-pathogen gene expression profiles during infection of primary bovine mammary epithelial cells with escherichia coli strains associated with acute or persistent bovine mastitis. Vet Microbiol (2012) 155(2-4):291–7. doi: 10.1016/j.vetmic.2011.08.016
26. de Souza Santos M, Orth K. Intracellular vibrio parahaemolyticus escapes the vacuole and establishes a replicative niche in the cytosol of epithelial cells. mBio (2014) 5(5):e01506–14. doi: 10.1128/mBio.01506-14
27. Liu X, Liu F, Ding S, Shen J, Zhu K. Sublethal levels of antibiotics promote bacterial persistence in epithelial cells. Adv Sci (2020) 7(18):1900840. doi: 10.1002/advs.201900840
28. Stojković D, Petrović J, Soković M, Glamočlija J, Kukić-Marković J, Petrović S. In situ antioxidant and antimicrobial activities of naturally occurring caffeic acid, p-coumaric acid and rutin, using food systems. J Sci Food Agricult (2013) 93(13):3205–8. doi: 10.1002/jsfa.6156
29. Mulat M, Khan F, Muluneh G, Pandita A. Phytochemical profile and antimicrobial effects of different medicinal plant: Current knowledge and future perspectives. Curr Tradition Med (2020) 6(1):24–42. doi: 10.2174/22150838MTAw5MDQE1
30. Liu M, Fang G, Yin S, Zhao X, Liu Z. Caffeic acid prevented LPS-induced injury of primary bovine mammary epithelial cells through inhibiting NF- κ b and MAPK activation. Mediators Inflamm (2019) 2019:1–12. doi: 10.1155/2019/1897820
31. Chen Z, Chu S, Wang X, Sun Y, Xu T, Mao Y, et al. MiR-16a regulates milk fat metabolism by targeting Large tumor suppressor kinase 1 (LATS1) in bovine mammary epithelial cells. J Agric Food Chem (2019) 67(40):11167–78. doi: 10.1021/acs.jafc.9b04883
32. Liu R, Zhu H, Zhao J, Wu X, Lu X, Xu T, et al. Lycium barbarum polysaccharide inhibits e. coli -induced inflammation and oxidative stress in mammary epithelial cells of dairy cows Via SOCS3 activation and MAPK suppression. Agriculture (2022) 12:598. doi: 10.3390/agriculture12050598
33. Hogan JS, Gonzalez RN, Harmon RJ, Nickerson SC, Smith KL. Laboratory handbook on bovine mastitis. Madison, WI: National Mastitis Council (1999).
34. Blum S, Heller ED, Krifucks O, Sela S, Hammer-Muntz O, Leitner G. Identification of a bovine mastitis escherichia coli subset. Vet Microbiol (2008) 132(1-2):135–48. doi: 10.1016/j.vetmic.2008.05.012
35. Xu T, Lu X, Arbab AAI, Wu X, Mao Y, Loor JJ, et al. Metformin acts to suppress β-hydroxybutyric acid-mediated inflammatory responses through activation of AMPK signaling in bovine hepatocytes. J Anim Sci (2021) 99(7):skab153. doi: 10.1093/jas/skab153
36. Abaker JA, Xu TL, Jin D, Chang GJ, Zhang K, Shen XZ. Lipopolysaccharide derived from the digestive tract provokes oxidative stress in the liver of dairy cows fed a high-grain diet. J Dairy Sci (2017) 100(1):666–78. doi: 10.3168/jds.2016-10871
37. Chang G, Zhang K, Xu T, Jin D, Seyfert HM, Shen X, et al. Feeding a high-grain diet reduces the percentage of LPS clearance and enhances immune gene expression in goat liver. BMC Vet Res (2015) 11:67. doi: 10.1186/s12917-015-0376-y
38. Zhou, Peng JJ, Loor. Methionine and valine activate the mammalian target of rapamycin complex 1 pathway through heterodimeric amino acid taste receptor (TAS1R1/TAS1R3) and intracellular Ca2+ in bovine mammary epithelial cells. J Dairy Sci (2018) 101(12):11354–63. doi: 10.3168/jds.2018-14461
39. Pfaffl MW. A new mathematical model for relative quantification in real-time RT-PCR. Nucleic Acids Res (2001) 29(9):e45. doi: 10.1093/nar/29.9.e45
40. Shao G, Tian Y, Wang H, Liu F, Xie G. Protective effects of melatonin on lipopolysaccharide-induced mastitis in mice. Int Immunopharmacol (2015) 29(2):263–8. doi: 10.1016/j.intimp.2015.11.011
41. Kępa M, Miklasińska-Majdanik M, Wojtyczka RD, Idzik D, Korzeniowski K, Smoleń-Dzirba J, et al. Antimicrobial potential of caffeic acid against staphylococcus aureus clinical strains. BioMed Res Int (2018) 2018:7413504. doi: 10.1155/2018/7413504
42. Gędas A, Olszewska MA. Chapter 1 - biofilm formation and resistance. In: Simoes M, Borges A, Chaves Simoes L, editors. Recent trends in biofilm science and technology, Pittsburgh: Academic Press (2020). p. 1–21.
43. Tyerman JG, Ponciano JM, Joyce P, Forney LJ, Harmon LJ. The evolution of antibiotic susceptibility and resistance during the formation of escherichia colibiofilms in the absence of antibiotics. BMC Evolution Biol (2013) 13(1):22. doi: 10.1186/1471-2148-13-22
44. Owrangi B, Masters N, Vollmerhausen TL, O’Dea C, Kuballa A, Katouli M. Comparison between virulence characteristics of dominant and non-dominant escherichia coli strains of the gut and their interaction with caco-2 cells. Microb Pathog (2017) 105:171–6. doi: 10.1016/j.micpath.2017.02.032
45. Zhuang C, Liu G, Barkema HW, Zhou M, Xu S, Rahman S, et al. Selenomethionine suppressed TLR4/NF-κB pathway by activating selenoproteins to alleviate ESBL escherichia coli-induced inflammation in bovine mammary epithelial cells and macrophages. Front Microbiol (2020) 11:1461. doi: 10.3389/fmicb.2020.01461
46. Toma L, Sanda GM, Niculescu LS, Deleanu M, Stancu CS, Sima AV. Caffeic acid attenuates the inflammatory stress induced by glycated LDL in human endothelial cells by mechanisms involving inhibition of AGE-receptor, oxidative, and endoplasmic reticulum stress. BioFactors (2017) 43(5):685–97. doi: 10.1002/biof.1373
47. Moyes KM, Drackley JK, Morin DE, Bionaz M, Rodriguez-Zas SL, Everts RE, et al. Gene network and pathway analysis of bovine mammary tissue challenged with streptococcus uberis reveals induction of cell proliferation and inhibition of PPARgamma signaling as potential mechanism for the negative relationships between immune response and lipid metabolism. BMC Genomics (2009) 10:542. doi: 10.1186/1471-2164-10-542
Keywords: bovine mastitis, gram-negative bacteria, antimicrobial, caffeic acid, anti-inflammatory activity
Citation: Xu T, Zhu H, Liu R, Wu X, Chang G, Yang Y and Yang Z (2022) The protective role of caffeic acid on bovine mammary epithelial cells and the inhibition of growth and biofilm formation of Gram-negative bacteria isolated from clinical mastitis milk. Front. Immunol. 13:1005430. doi: 10.3389/fimmu.2022.1005430
Received: 28 July 2022; Accepted: 23 September 2022;
Published: 20 October 2022.
Edited by:
Yong Huang, Northwest A&F University, ChinaReviewed by:
Hongyan Zhang, South China Agricultural University, ChinaYuefeng Chu, Lanzhou Veterinary Research Institute (CAAS), China
Lili Zhang, Jiangsu Academy of Agricultural Sciences (JAAS), China
Yawang Sun, Southwest University, China
Yang Liu, China Agricultural University, China
Maria Soledad Orellano, Polymat, Spain
Copyright © 2022 Xu, Zhu, Liu, Wu, Chang, Yang and Yang. This is an open-access article distributed under the terms of the Creative Commons Attribution License (CC BY). The use, distribution or reproduction in other forums is permitted, provided the original author(s) and the copyright owner(s) are credited and that the original publication in this journal is cited, in accordance with accepted academic practice. No use, distribution or reproduction is permitted which does not comply with these terms.
*Correspondence: Zhangping Yang, yzp@yzu.edu.cn