- 1Department of Infectious Diseases, St. Jude Children’s Research Hospital, Memphis, TN, United States
- 2Department of Pathology, St. Jude Children’s Research Hospital, Memphis, TN, United States
- 3Department of Microbiology, Immunology, and Biochemistry, University of Tennessee Health Science Center (UTHSC), Memphis, TN, United States
Cystic fibrosis (CF) is an autosomal recessive gene disorder that affects tens of thousands of patients worldwide. Individuals with CF often succumb to progressive lung disease and respiratory failure following recurrent infections with bacteria. Viral infections can also damage the lungs and heighten the CF patient’s susceptibility to bacterial infections and long-term sequelae. Vitamin A is a key nutrient important for immune health and epithelial cell integrity, but there is currently no consensus as to whether vitamin A should be monitored in CF patients. Here we evaluate previous literature and present results from a CF mouse model, showing that oral vitamin A supplements significantly reduce lung lesions that would otherwise persist for 5-6 weeks post-virus exposure. Based on these results, we encourage continued research and suggest that programs for the routine monitoring and regulation of vitamin A levels may help reduce virus-induced lung pathology in CF patients.
Cystic Fibrosis (CF), the Disease
CF is a recessive genetic disorder that affects tens of thousands of patients globally. The disease is due to mutations in the cystic fibrosis transmembrane conductance regulator gene (CFTR) that encodes an ion channel protein. Mutations result in abnormal epithelial fluid transport in respiratory and digestive tracts (1). Individuals with CF often succumb to progressive lung disease and respiratory failure following recurrent infections with bacteria including Staphylococcus aureus, Haemophilus influenza, and Pseudomonas aeruginosa (2, 3). Although some reports suggest that CF patients clear viruses as well as their healthy counterparts (4), virus infections can damage the lung, increase susceptibility to bacterial infections, and worsen outcomes. CF patients with respiratory viral infections often have culture-positive bacterial pathogens (5, 6). In one study, viral respiratory infections were evident among 65% of exacerbations in patients with CF (7, 8). These data highlight the importance of virus control as an integral component of CF patient care.
Vitamin A
Vitamin A is an essential nutrient, necessary for healthy immune responses against respiratory pathogens, control of infectious diseases, and the integrity of mucosal epithelial cells. Vitamin A effector functions occur at the cell surface, within the cell cytoplasm, and within the nucleus of target cells (9–11). Experiments in animal models have shown that when vitamin A is low, host susceptibility to pathogens is increased (12–15). In vitamin A deficient animals, vaccine responses are weak, and serious outcomes follow viral infections and bacterial co-infections (15–17). After clearance of replicating pathogens, residual foreign antigens and consequent inflammatory responses may persist (18).
Vitamin A Deficiencies/Insufficiencies Are a Global Concern
It is noteworthy that vitamin A deficiency/insufficiency, a condition once presumed to affect developing countries only, is now frequent in the developed world including the United States (19, 20). A unique concern is raised for the CF patient due to pancreatic insufficiency and malabsorption (21–23). Some centers report that 10-40% of their CF patients are vitamin A deficient (VAD) (24). Vitamin A monitoring is sometimes performed as a routine and supplementation is sometimes used as a treatment option in deficient patients (25, 26). Sapiejka et al. observed higher blood vitamin A levels in patients who received vitamin A supplements. They also found that low FEV1 levels (<80%) were significantly more frequent in patients with vitamin A levels <300 ng/ml.
Unfortunately, health guidelines vary (24–31) and many clinical centers overlook vitamin A. Even when serum vitamin A levels appear normal in an individual (32), there may be inadequate vitamin A levels in parenchymal tissues (33). Tissue deficits can be missed in humans when standard blood tests are employed and may be revealed by vitamin A dose response tests (34). When vitamin A levels are insufficient/deficient in an animal, whether due to a nutritional deficiency or an underlying health condition, vitamin supplements can improve pathogen-specific immune responses and pathogen control (13, 17, 35, 36).
Experiments in a CF Mouse Model Demonstrate That Persistent Lung Pathology Following a Respiratory Virus Infection Is Reduced by Oral Vitamin A Supplements
We used a CF mouse model to ask if vitamin A supplements could reduce virus-induced lung pathology that persists after a parainfluenza virus respiratory infection. Experiments were with Cftrtm1Unc Tg(FABPCFTR)1Jaw mice that were purchased from Jackson Laboratories (Bar Harbor, Maine) or bred from Jackson mice at St Jude Children’s Research Hospital. Cftrtm1Unc Tg(FABPCFTR)1Jaw mice are homozygous for a mutation in the mouse Cftr gene. The targeted knock-out allele (Cftrtm1Unc) was originally created by insertion of a neomycin selection cassette into the gene at sequences corresponding to codon 489. Mice were additionally homozygous for a functional human CFTR gene expressed under the control of the rat intestinal fatty acid-binding protein 2 (Fabp2) gene promoter (FABP-hCFTR) (37, 38). The latter gene was necessary to rescue protein function in the mouse intestinal epithelium, to correct malabsorption. Otherwise, mice did not survive to adulthood. We note that in humans with CF, malabsorption is often corrected using pancreatic enzyme replacement therapy (39). Mice were termed ‘CF’ for simplicity. A preliminary test of serum retinol binding protein (RBP), a surrogate for serum vitamin A (retinol), showed no significant differences between mice carrying the CFTR mutation and controls.
Experiments were designed and performed in triplicate to test the benefits of vitamin A supplements for reduction of residual lung pathology that persisted several weeks after a viral infection in CF animals. In each of three experiments, there were two groups of mice with 7-8 mice per group. Both groups were infected with parainfluenza virus (Sendai virus, SeV). Test CF mice, but not controls, were additionally treated with oral doses of vitamin A on days -7, -3, 0, and +3 relative to the intranasal infection. The extent and severity of residual pulmonary lesions were then assessed at 5-6 weeks post-infection, a time after virus had been cleared (no residual viral antigen was detected by immunohistochemical staining at that time), to identify persistent consequences of a virus infection in the CF model.
After SeV infections of CF mice, vitamin A-supplemented mice exhibited fewer lung lesions at 5-6 weeks compared to unsupplemented mice. In Figure 1, data are shown from one experiment. The three types of lesions represented in this Figure (interstitial inflammation, alveolar inflammation, and septal thickening) were: (a) reduced in supplemented mice compared to controls in each of the three experiments, and (b) significantly reduced in supplemented mice compared to controls in at least one of the three experiments.
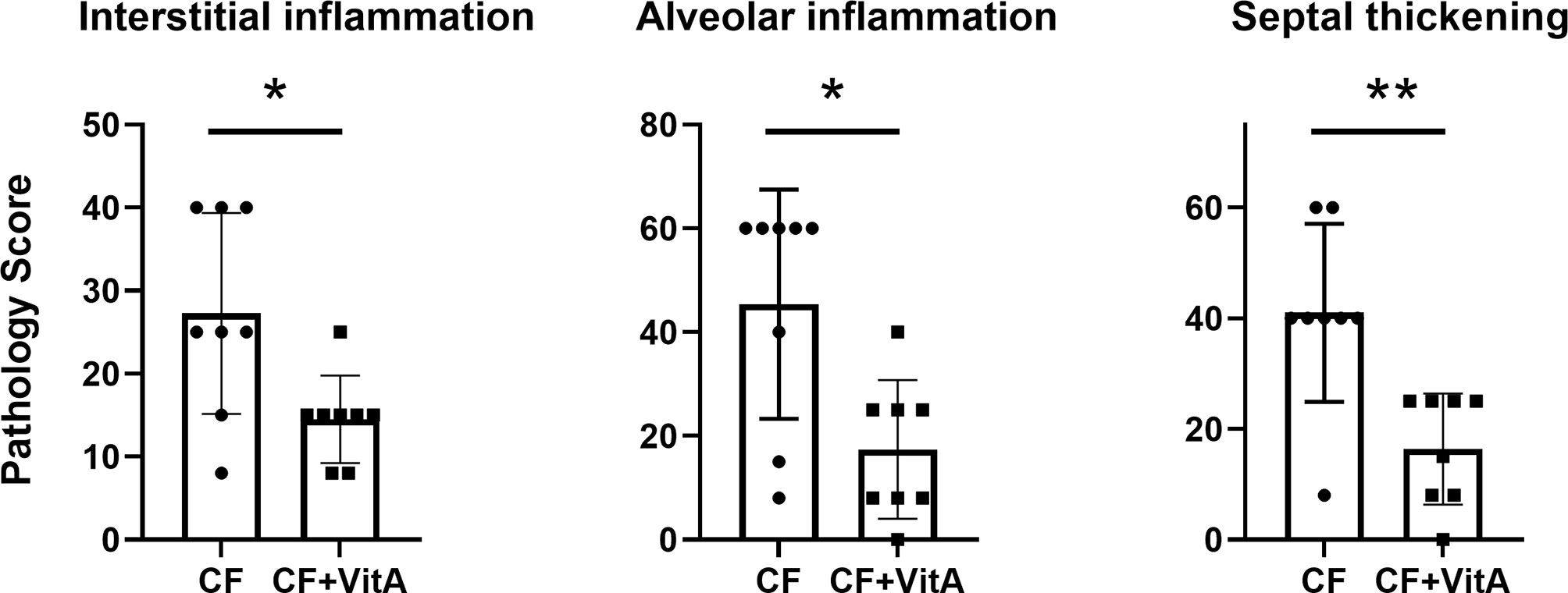
Figure 1 Vitamin A supplements at the time of parainfluenza virus infections reduce lung lesions scored 5-6 weeks post-infection. Data are from one of three experiments demonstrating the reduction of lung lesions in CF mice supplemented with vitamin A at the time of a virus infection. Lesions were given relative scores. Each symbol represents a different test mouse (no lesions were observed in control, uninfected mice). In each of the three experiments, the vitamin A-supplemented mice (CF+VitA) exhibited significantly or marginally reduced scores for interstitial inflammation, alveolar inflammation, and septal thickening at weeks 5-6 post-virus infection compared to mice that received no supplement (CF). Means with standard deviations are shown. Mann Whitney tests were performed to compare groups (*p < .05, **p < .01).
Figure 2 shows images of lung tissues observed 5-6 weeks after SeV infections in CF mice that did not receive (left column) or did receive (right column) vitamin A supplements. In Figure 2A are shown lesions from an unsupplemented mouse that extended from terminal airways to the lung margins, sometimes coalescing with lesions surrounding other bronchioles to involve entire lung lobes. Higher magnification images revealed lesions with indistinct borders and alveoli filled with debris and cellular infiltrates (Figure 2C). These cell infiltrates consisted mostly of large (activated) alveolar macrophages, with scattered clusters of neutrophils, both intact and degenerating (see arrow, Figure 2E). In contrast, the lesions in a CF mouse that received vitamin A supplements at the time of SeV infection were sharply demarcated and relatively small, rarely reaching the lung margin and generally only partially surrounding terminal airways (Figure 2B). Higher magnification images revealed thickened alveolar septa and predominantly clear alveolar spaces with relatively little cellular debris and few alveolar macrophages (Figures 2D, F). Peribronchiolar lymphoid aggregates were observed (see arrow, Figure 2D). Septal thickening involved interstitial inflammatory cell infiltrates (lymphocytic) and diffuse hypertrophy of alveolar epithelium (Figure 2F). Images from normal areas of tissue that were unaffected by virus are shown in Figures 2G, H from an unsupplemented and supplemented mouse, respectively. Here, there were no notable differences between alveoli. Figures 2I, J provide the highest (60X) magnifications of inflamed virus-damaged areas from an unsupplemented and supplemented mouse, respectively. In the unsupplemented mouse (Figure 2I), as described above, alveoli were filled with clusters of neutrophils (arrows) and large foamy alveolar macrophages (alveolar inflammation). In contrast, in the vitamin A-supplemented mouse (Figure 2J), smaller pulmonary lesions at 5-6 weeks after SeV infection were characterized by air-filled alveoli with septa thickened by hypertrophic alveolar epithelium and interstitial lymphocytic infiltrates (interstitial inflammation). Lymphocytic infiltrates were especially prominent surrounding blood vessels (near asterisk). The open alveolar spaces contained normal-sized alveolar macrophages with relatively small amounts of cytoplasm (arrow).
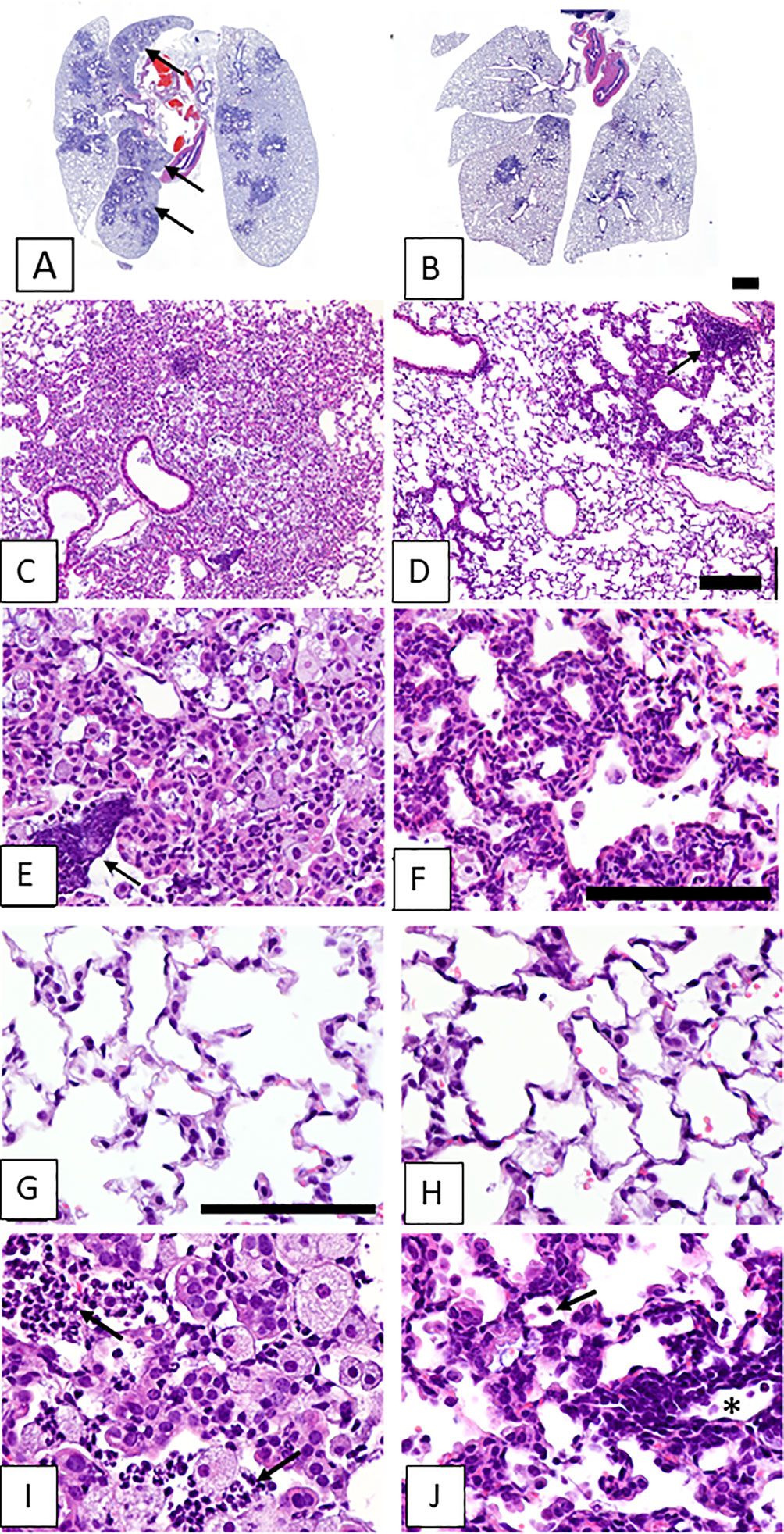
Figure 2 Lung lesions from vitamin-unsupplemented and supplemented in CF mice 5-6 weeks post-parainfluenza virus infections. Images represent lung tissues sampled 5-6 weeks after an SeV infection. Images in the left column were taken when mice received no vitamin A supplements. Images in the left column represent a mouse that received no vitamin A supplements. Images in the right column represent a mouse that received vitamin A supplements at the time of SeV infections. Panel (A) shows lesions from an unsupplemented mouse. These frequently extended from terminal airways to the lung margins and coalesced with lesions surrounding other bronchioles to involve entire lung lobes (arrows). (C) Lesions were characterized by indistinct borders and alveoli filled with debris and cellular infiltrates. (E) Cell infiltrates consisted mostly of large (activated) alveolar macrophages, with scattered clusters of neutrophils, both intact and degenerating (arrow). Panel (B) shows Pulmonary lesions from a mouse taken 5-6 weeks after an SeV infection with vitamin A supplementation. Lesions were small and sharply demarcated, rarely reaching the lung margin and not affecting entire lung lobes. (D) At higher magnification, lesions were observed with generally clear alveolar spaces, sharply demarcated from surrounding normal parenchyma. Peribronchiolar lymphoid aggregates were also evident (arrow). (F) Septal thickening in the lesioned area was due to interstitial inflammatory cell infiltrates (lymphocytic) and diffuse hypertrophy of alveolar epithelium. (G, H) Images include areas of normal tissue for comparison to areas of diseased tissue. Images were from unsupplemented and supplemented mice, respectively. (I, J) Images show lesioned areas at 60X magnification in unsupplemented and supplemented mice, respectively. (I) a virus-damaged area in which alveoli were filled with clusters of neutrophils (arrows) and large foamy alveolar macrophages (alveolar inflammation). (J) Pulmonary lesions were characterized by air-filled alveoli with septa thickened by hypertrophic alveolar epithelium and interstitial lymphocytic infiltrates (interstitial inflammation). Lymphocytic infiltrates were prominent surrounding blood vessels (near asterisk). Open alveolar spaces contained normal-sized alveolar macrophages with relatively small amounts of cytoplasm (arrow). Scale bars: B = 1 mm; D = 200 μm; F, G = 100 μm.
This study had limitations in that it used a single CF mouse model and focused on a single parainfluenza virus infection and a single vitamin A dosing regimen. The mechanism by which vitamin A protected animals from virus-induced pathology was not defined in this study. Studies were performed after virus had been cleared and when viral antigens were no longer detected by immunohistochemical studies. Possibly, the employment of a more sensitive assay such as the polymerase chain reaction (40) or a T-cell based assay (18) would have detected residual nucleic acids or peptide fragments pertinent to long-term health outcomes. Follow-up studies are warranted to define mechanisms by which vitamin A protected the animals. Experiments may include different CF models (41–43), different pathogens, and different vitamin A dosing regimens with comprehensive measurements of virus titers, inflammatory cell subsets in the lung (aided by flow cytometry), vitamin levels (in blood, liver, and lung), animal weights, and immune functions throughout the time course.
Why Might Vitamin A Supplements reduce Lung Lesions Post-Virus Infection?
Vitamin A is pleiotropic in function, affecting numerous cell types including B cells, T cells, dendritic cells (DCs) and respiratory tract epithelial cells. As an example, vitamin A influences the expression of α4β7 and CD103, a component of the αEβ7 homing receptor, on T cells and DCs. These homing receptors regulate adherence and motility of immune cells, important for rapid virus-specific immune responses (44–47). As a second example, vitamin A assists B cell production of IgA, a key effector for the local prevention of respiratory tract infections (35, 48–50). In some systems, vitamin A has been associated with the induction of interferon (IFN) α/β and the support of IFN functions (51–53). By enhancing adaptive and innate immune functions, vitamin A may improve viral clearance and thereby prevent lung damage.
There are a multitude of additional effects (both immunostimulatory and immunoregulatory) of vitamin A on pathogen control including influences on myeloid cell expansion (54) and Treg or Th17 maturation [with variable effects in vitro and in vivo (12)]. Mechanisms and cause-effect relationships are complex in that an influence of vitamin A on one cell type (e.g., upregulation of B cell activity) may have numerous downstream influences (e.g., clearance of antigen and reduction of cytokines) (12, 17, 18, 55). Disease consequences can, in turn, influence levels of RBP (an acute-phase protein and an important blood escort for retinol) and vitamin A.
Apart from affecting immune responses, vitamin A also supports the growth and integrity of epithelial cells (14), features associated with wound prevention and repair. When introduced into in vitro systems, vitamin A assists the establishment of epithelial monolayers and organ culture systems (56). Epithelial cells of the respiratory tract express retinaldehyde dehydrogenase 2 (RALDH2 or ALDH1A2), an enzyme necessary for conversion of retinaldehyde to its end-stage metabolite, retinoic acid (48).
Our previous studies have shown that VAD associates with delayed clearance of respiratory viruses and viral antigens (15, 18). Vitamin A supplements can improve vitamin A levels in peripheral tissues, including the lung, and improve immune-mediated clearance of respiratory viral infections (12, 13, 35, 36). Consequently, epithelial barriers are maintained and antigen-driven inflammatory responses are resolved (18). Altogether, the benefits of vitamin A are clearly multi-factorial and include support of (i) rapid immune function, (ii) rapid clearance of virus and viral antigens, and (iii) epithelial growth, to prevent lung pathology and improve clinical health.
Should Vitamin A Supplements Be Used Indiscriminately in CF Patients?
There is unfortunately no consensus guideline for vitamin A monitoring and control in CF patients. Replete vitamin A levels are best attained by maintenance of healthy diets, but supplementation programs provide a back-up solution when patients repeatedly suffer malnutrition. Some centers encourage vitamin A supplementation, while others do not (24, 27–30). Indiscriminate use of vitamin A supplements is sometimes advocated, but the fine-tuning of vitamin supplementation programs may yield a better result. This is because vitamin A supplements, particularly if administered at high doses, can cause harm (57). As an example, Bresee et al. showed that when vitamin A supplements were administered to children who were hospitalized with respiratory syncytial virus (RSV) infections, the supplemented children experienced longer hospital stays than placebo controls (58). In our study of influenza vaccine immunogenicity in children, a supplement with vitamin A+D improved responses in children who were vitamin A+D insufficient or deficient at baseline, but reduced responses in children who were vitamin replete (20). Baseline vitamin levels [and levels of other nuclear receptor ligands that may cross-regulate vitamin functions (10, 59)] will clearly influence outcome.
Few controlled clinical studies of vitamin A supplementation in CF patients have been performed (25, 60). In one small study of CF patients, high-dose beta-carotene supplementation was compared to placebo and was found to reduce requirements for antibiotics during a three-month period (25), demonstrating a prophylactic benefit. Additional, controlled clinical trials are needed to determine precisely when vitamin A supplements provide benefit and what characteristics (e.g., age, obesity, sex, and baseline vitamin levels) associate with the best outcome among CF patients.
Conclusion
In a mouse model for CF, vitamin A supplements were shown to improve lung integrity 5-6 weeks after a respiratory viral infection. Results encourage additional basic research and the performance of new clinical trials to determine when diet modifications and/or vitamin A supplements are beneficial to this patient population. Our perspective is that vitamin A should be routinely monitored and regulated in CF patients to improve lung health and protect from persistent tissue damage caused by infectious disease.
Methods
Experiments were reviewed and approved by the IACUC of St. Jude Children’s Research Hospital (St. Jude). All experiments were with Cftrtm1Unc Tg(FABPCFTR)1Jaw mice that were purchased from Jackson Laboratories (Bar Harbor, Maine) or bred from Jackson mice at St Jude. To breed mice at St. Jude, females that were heterozygous for the mouse Cftrtm1Unc Tg and homozygous for FABP-hCFTR were crossed to males that were homozygous for Cftrtm1Unc Tg and homozygous for FABP-hCFTR. Progeny were genotyped at Transnetyx (Cordova, Tennessee) and mice that were homozygous for both Cftrtm1Unc Tg and FABP-hCFTR were selected for study. Mice were anesthetized with isoflurane and infected intranasally with 250 plaque forming units (pfu) of Sendai virus (SeV, a mouse parainfluenza virus-type 1) in 30 μl phosphate buffered saline. Test mice were supplemented with vitamin A (retinyl palmitate, 600 IU/mouse, Nutrisorb A, Interplexus Inc. [Kent, WA] in 100 μl PBS) by oral gavage on days -7, -3, 0, and +3 relative to infection. The route of administration and dose were selected to test a simple, clinically translatable, prophylactic methodology. Mice were rested for 5-6 weeks post-infection and then euthanized with CO2. The lungs were infused with formalin, fixed by immersion in 10% neutral buffered formalin for several weeks, embedded in paraffin, sectioned, and stained with hematoxylin and eosin stains. Slides were stained for residual viral antigens (18), but scored negative. Slides were analyzed and scored by a veterinary pathologist who was blinded to mouse groups at the time of analyses in the Veterinary Pathology Core Department at St. Jude. Scores were given for interstitial inflammation, alveolar inflammation, alveolar protein/fibrin, septal thickening, lymphoid nodules, epithelial hyperplasia, bronchiolization, and fibrosis. Each of these types of pulmonary lesions was assigned a severity grade on a 1-5 ranked scale as follows: 0 = no lesions (no changes outside of normal limits; 0% tissue affected); 1 = minimal, focal to multifocal, inconspicuous (rare lesions, barely above normal limits; <5% tissue affected); 2 = mild, multifocal, prominent (small, widely separated or focal lesions, limited severity; <10% tissue affected); 3 = moderate, multifocal, prominent; 4 = marked, multifocal or coalescing, lobar (>60% tissue affected); 5 = severe, extensive or diffuse, multilobar, with consolidation (>80% tissue affected). Intermediate severity grades were also assigned as needed. Grades were then converted to weighted semi-quantitative scores to better reflect the extent of damage and lung involvement as follows: 0 = 0; 1 = 1; 1.5 = 8; 2 = 15; 2.5 = 25; 3 = 40; 3.5 = 60; 4 = 80; 4.5 = 90; 5 = 100. Semiquantitative scores are shown for each animal on Y axes. Experiments were performed in triplicate with 7-8 mice per group in each experiment.
Data Availability Statement
The raw data supporting the conclusions of this article will be made available by the authors, without undue reservation.
Ethics Statement
The animal study was reviewed and approved by the IACUC at St. Jude.
Author Contributions
All authors contributed to the design, methodology, and interpretation of experiments. JLH authored the first draft of the paper and all authors reviewed and edited the paper. All authors contributed to the article and approved the submitted version.
Funding
Research was supported, in part, by NIH NCI P30CA21765 and ALSAC.
Conflict of Interest
The authors declare that the research was conducted in the absence of any commercial or financial relationships that could be construed as a potential conflict of interest.
Publisher’s Note
All claims expressed in this article are solely those of the authors and do not necessarily represent those of their affiliated organizations, or those of the publisher, the editors and the reviewers. Any product that may be evaluated in this article, or claim that may be made by its manufacturer, is not guaranteed or endorsed by the publisher.
Acknowledgments
We thank Bart G. Jones for technical assistance.
References
1. Riordan JR. The Cystic Fibrosis Transmembrane Conductance Regulator. Annu Rev Physiol (1993) 55:609–30. doi: 10.1146/annurev.ph.55.030193.003141
2. Huang P, Lazarowski ER, Tarran R, Milgram SL, Boucher RC, Stutts MJ. Compartmentalized Autocrine Signaling to Cystic Fibrosis Transmembrane Conductance Regulator at the Apical Membrane of Airway Epithelial Cells. Proc Natl Acad Sci USA (2001) 98:14120–5. doi: 10.1073/pnas.241318498
3. Kiedrowski MR, Bomberger JM. Viral-Bacterial Co-Infections in the Cystic Fibrosis Respiratory Tract. Front Immunol (2018) 9:3067. doi: 10.3389/fimmu.2018.03067
4. Ramsey BW, Gore EJ, Smith AL, Cooney MK, Redding GJ, Foy H. The Effect of Respiratory Viral Infections on Patients With Cystic Fibrosis. Am J Dis Child (1989) 143:662–8. doi: 10.1001/archpedi.1989.02150180040017
5. Wat D, Gelder C, Hibbitts S, Cafferty F, Bowler I, Pierrepoint M, et al. The Role of Respiratory Viruses in Cystic Fibrosis. J Cyst Fibros (2008) 7:320–8. doi: 10.1016/j.jcf.2007.12.002
6. Esther CR Jr., Lin FC, Kerr A, Miller MB, Gilligan PH. Respiratory Viruses Are Associated With Common Respiratory Pathogens in Cystic Fibrosis. Pediatr Pulmonol (2014) 49:926–31. doi: 10.1002/ppul.22917
7. Wark PA, Tooze M, Cheese L, Whitehead B, Gibson PG, Wark KF, et al. Viral Infections Trigger Exacerbations of Cystic Fibrosis in Adults and Children. Eur Respir J (2012) 40:510–2. doi: 10.1183/09031936.00202311
8. Petersen NT, Hoiby N, Mordhorst CH, Lind K, Flensborg EW, Bruun B. Respiratory Infections in Cystic Fibrosis Patients Caused by Virus, Chlamydia and Mycoplasma–Possible Synergism With Pseudomonas Aeruginosa. Acta Paediatr Scand (1981) 70:623–8. doi: 10.1111/j.1651-2227.1981.tb05757.x
9. Napoli JL. Functions of Intracellular Retinoid Binding-Proteins. Subcell Biochem (2016) 81:21–76. doi: 10.1007/978-94-024-0945-1_2
10. Evans RM, Mangelsdorf DJ. Nuclear Receptors, RXR, and the Big Bang. Cell (2014) 157:255–66. doi: 10.1016/j.cell.2014.03.012
11. Hurwitz JL, Penkert RR, Xu B, Fan Y, Partridge JF, Maul RW, et al. Hotspots for Vitamin-Steroid-Thyroid Hormone Response Elements Within Switch Regions of Immunoglobulin Heavy Chain Loci Predict a Direct Influence of Vitamins and Hormones on B Cell Class Switch Recombination. Viral Immunol (2016) 29:132–6. doi: 10.1089/vim.2015.0104
12. Surman SL, Penkert RR, Jones BG, Sealy RE, Hurwitz JL. Vitamin Supplementation at the Time of Immunization With a Cold-Adapted Influenza Virus Vaccine Corrects Poor Mucosal Antibody Responses in Mice Deficient for Vitamins A and D. Clin Vaccine Immunol (2016) 23:219–27. doi: 10.1128/CVI.00739-15
13. Penkert RR, Cortez V, Karlsson EA, Livingston B, Surman SL, Li Y, et al. Vitamin A Corrects Tissue Deficits in Diet-Induced Obese Mice and Reduces Influenza Infection After Vaccination and Challenge. Obes (Silver Spring) (2020) 28:1631–6. doi: 10.1002/oby.22929
14. Surman SL, Penkert RR, Sealy RE, Jones BG, Marion TN, Vogel P, et al. Consequences of Vitamin A Deficiency: Immunoglobulin Dysregulation, Squamous Cell Metaplasia, Infectious Disease, and Death. Int J Mol Sci (2020) 21:5570. doi: 10.3390/ijms21155570
15. Penkert RR, Smith AP, Hrincius ER, McCullers JA, Vogel P, Smith AM, et al. Vitamin A Deficiency Dysregulates Immune Responses Toward Influenza Virus and Increases Mortality After Bacterial Coinfections. J Infect Dis (2020) 223:1806–16. doi: 10.1093/infdis/jiaa597
16. Penkert RR, Iverson A, Rosch JW, Hurwitz JL. Prevnar-13 Vaccine Failure in a Mouse Model for Vitamin A Deficiency. Vaccine (2017) 35:6264–8. doi: 10.1016/j.vaccine.2017.09.069
17. Penkert RR, Rowe HM, Surman SL, Sealy RE, Rosch J, Hurwitz JL. Influences of Vitamin A on Vaccine Immunogenicity and Efficacy. Front Immunol (2019) 10:1576. doi: 10.3389/fimmu.2019.01576
18. Penkert RR, Surman SL, Jones BG, Sealy RE, Vogel P, Neale G, et al. Vitamin A Deficient Mice Exhibit Increased Viral Antigens and Enhanced Cytokine/Chemokine Production in Nasal Tissues Following Respiratory Virus Infection Despite the Presence of FoxP3+ T Cells. Int Immunol (2016) 28:139–52. doi: 10.1093/intimm/dxv064
19. Jones BG, Penkert RR, Xu B, Fan Y, Neale G, Gearhart PJ, et al. Binding of Estrogen Receptors to Switch Sites and Regulatory Elements in the Immunoglobulin Heavy Chain Locus of Activated B Cells Suggests a Direct Influence of Estrogen on Antibody Expression. Mol Immunol (2016) 77:97–102. doi: 10.1016/j.molimm.2016.07.015
20. Patel N, Penkert RR, Jones BG, Sealy RE, Surman SL, Sun Y, et al. Baseline Serum Vitamin A and D Levels Determine Benefit of Oral Vitamin a&D Supplements to Humoral Immune Responses Following Pediatric Influenza Vaccination. Viruses (2019) 11:907. doi: 10.3390/v11100907
21. Norsa L, Zazzeron L, Cuomo M, Claut L, Bulfamante AMC, Biffi A, et al. Night Blindness in Cystic Fibrosis: The Key Role of Vitamin A in the Digestive System. Nutrients (2019) 11:1876. doi: 10.3390/nu11081876
22. Lindblad A, Diczfalusy U, Hultcrantz R, Thorell A, Strandvik B. Vitamin A Concentration in the Liver Decreases With Age in Patients With Cystic Fibrosis. J Pediatr Gastroenterol Nutr (1997) 24:264–70. doi: 10.1097/00005176-199703000-00006
23. Greer RM, Buntain HM, Lewindon PJ, Wainwright CE, Potter JM, Wong JC, et al. Vitamin A Levels in Patients With CF Are Influenced by the Inflammatory Response. J Cyst Fibros (2004) 3:143–9. doi: 10.1016/j.jcf.2004.04.003
24. Turck D, Braegger CP, Colombo C, Declercq D, Morton A, Pancheva R, et al. ESPEN-ESPGHAN-ECFS Guidelines on Nutrition Care for Infants, Children, and Adults With Cystic Fibrosis. Clin Nutr (2016) 35:557–77. doi: 10.1016/j.clnu.2016.03.004
25. de Vries JJ, Chang AB, Bonifant CM, Shevill E, Marchant JM. Vitamin A and Beta (Beta)-Carotene Supplementation for Cystic Fibrosis. Cochrane Database Syst Rev (2018) 8:CD006751. doi: 10.1002/14651858.CD006751.pub5
26. Woestenenk JW, Broos N, Stellato RK, Arets HG, van der Ent CK, Houwen RH. Vitamin A Intake and Serum Retinol Levels in Children and Adolescents With Cystic Fibrosis. Clin Nutr (2016) 35:654–9. doi: 10.1016/j.clnu.2015.04.010
27. Elborn JS, Davies J, Mall MA, Flume PA, Plant B. Current Strategies for the Long-Term Assessment, Monitoring, and Management of Cystic Fibrosis Patients Treated With CFTR Modulator Therapy. J Cyst Fibros (2017) 16:163–4. doi: 10.1016/j.jcf.2016.11.009
28. Ruseckaite R, Pekin N, King S, Carr E, Ahern S, Oldroyd J, et al. Evaluating the Impact of 2006 Australasian Clinical Practice Guidelines for Nutrition in Children With Cystic Fibrosis in Australia. Respir Med (2018) 142:7–14. doi: 10.1016/j.rmed.2018.07.007
29. van der Haak N, King SJ, Crowder T, Kench A, Painter C, Saxby N, et al. Highlights From the Nutrition Guidelines for Cystic Fibrosis in Australia and New Zealand. J Cyst Fibros (2020) 19:16–25. doi: 10.1016/j.jcf.2019.05.007
30. Dodge JA, Turck D. Cystic Fibrosis: Nutritional Consequences and Management. Best Pract Res Clin Gastroenterol (2006) 20:531–46. doi: 10.1016/j.bpg.2005.11.006
31. Sommerburg O, Hammerling S, Schneider SP, Okun J, Langhans CD, Leutz-Schmidt P, et al. CFTR Modulator Therapy With Lumacaftor/Ivacaftor Alters Plasma Concentrations of Lipid-Soluble Vitamins A and E in Patients With Cystic Fibrosis. Antioxid (Basel) (2021) 10:483. doi: 10.3390/antiox10030483
32. Li L, Somerset S. Dietary Intake and Nutritional Status of Micronutrients in Adults With Cystic Fibrosis in Relation to Current Recommendations. Clin Nutr (2016) 35:775–82. doi: 10.1016/j.clnu.2015.06.004
33. Trasino SE, Tang XH, Jessurun J, Gudas LJ. Obesity Leads to Tissue, But Not Serum Vitamin A Deficiency. Sci Rep (2015) 5:15893. doi: 10.1038/srep15893
34. Ferraz IS, Daneluzzi JC, Vannucchi H, Jordao AA Jr, Ricco RG, Del Ciampo LA, et al. Detection of Vitamin A Deficiency in Brazilian Preschool Children Using the Serum 30-Day Dose-Response Test. Eur J Clin Nutr (2004) 58:1372–7. doi: 10.1038/sj.ejcn.1601978
35. Surman SL, Jones BG, Sealy RE, Rudraraju R, Hurwitz JL. Oral Retinyl Palmitate or Retinoic Acid Corrects Mucosal IgA Responses Toward an Intranasal Influenza Virus Vaccine in Vitamin A Deficient Mice. Vaccine (2014) 32:2521–4. doi: 10.1016/j.vaccine.2014.03.025
36. Surman SL, Jones BG, Rudraraju R, Sealy RE, Hurwitz JL. Intranasal Administration of Retinyl Palmitate With a Respiratory Virus Vaccine Corrects Impaired Mucosal IgA Response in the Vitamin A-Deficient Host. Clin Vaccine Immunol (2014) 21:598–601. doi: 10.1128/CVI.00757-13
37. Zhou L, Dey CR, Wert SE, DuVall MD, Frizzell RA, Whitsett JA. Correction of Lethal Intestinal Defect in a Mouse Model of Cystic Fibrosis by Human CFTR. Science (1994) 266:1705–8. doi: 10.1126/science.7527588
38. Steagall WK, Elmer HL, Brady KG, Kelley TJ. Cystic Fibrosis Transmembrane Conductance Regulator-Dependent Regulation of Epithelial Inducible Nitric Oxide Synthase Expression. Am J Respir Cell Mol Biol (2000) 22:45–50. doi: 10.1165/ajrcmb.22.1.3789
39. Munce D, Lim M, Akong K. Persistent Recovery of Pancreatic Function in Patients With Cystic Fibrosis After Ivacaftor. Pediatr Pulmonol (2020) 55:3381–3. doi: 10.1002/ppul.25065
40. Hurwitz JL, Jones BG, Charpentier E, Woodland DL. Hypothesis: RNA and DNA Viral Sequence Integration Into the Mammalian Host Genome Supports Long-Term B Cell and T Cell Adaptive Immunity. Viral Immunol (2017) 30:628–32. doi: 10.1089/vim.2017.0099
41. Mall M, Grubb BR, Harkema JR, O’Neal WK, Boucher RC. Increased Airway Epithelial Na+ Absorption Produces Cystic Fibrosis-Like Lung Disease in Mice. Nat Med (2004) 10:487–93. doi: 10.1038/nm1028
42. van Doorninck JH, French PJ, Verbeek E, Peters RH, Morreau H, Bijman J, et al. A Mouse Model for the Cystic Fibrosis Delta F508 Mutation. EMBO J (1995) 14:4403–11. doi: 10.1002/j.1460-2075.1995.tb00119.x
43. Keiser NW, Engelhardt JF. New Animal Models of Cystic Fibrosis: What Are They Teaching Us? Curr Opin Pulm Med (2011) 17:478–83. doi: 10.1097/MCP.0b013e32834b14c9
44. Reilly EC, Lambert Emo K, Buckley PM, Reilly NS, Smith I, Chaves FA, et al. TRM Integrins CD103 and CD49a Differentially Support Adherence and Motility After Resolution of Influenza Virus Infection. Proc Natl Acad Sci USA (2020) 117:12306–14. doi: 10.1073/pnas.1915681117
45. Mora JR, Iwata M, Von Andrian UH. Vitamin Effects on the Immune System: Vitamins A and D Take Centre Stage. Nat Rev Immunol (2008) 8:685–98. doi: 10.1038/nri2378
46. Rudraraju R, Surman SL, Jones BG, Sealy R, Woodland DL, Hurwitz JL. Reduced Frequencies and Heightened CD103 Expression Among Virus-Induced CD8(+) T Cells in the Respiratory Tract Airways of Vitamin A-Deficient Mice. Clin Vaccine Immunol (2012) 19:757–65. doi: 10.1128/CVI.05576-11
47. Vlasova AN, Chattha KS, Kandasamy S, Siegismund CS, Saif LJ. Prenatally Acquired Vitamin A Deficiency Alters Innate Immune Responses to Human Rotavirus in a Gnotobiotic Pig Model. J Immunol (2013) 190:4742–53. doi: 10.4049/jimmunol.1203575
48. Rudraraju R, Jones BG, Surman SL, Sealy RE, Thomas PG, Hurwitz JL. Respiratory Tract Epithelial Cells Express Retinaldehyde Dehydrogenase ALDH1A and Enhance IgA Production by Stimulated B Cells in the Presence of Vitamin a. PloS One (2014) 9:e86554. doi: 10.1371/journal.pone.0086554
49. Seo GY, Jang YS, Kim J, Choe J, Han HJ, Lee JM, et al. Retinoic Acid Acts as a Selective Human IgA Switch Factor. Hum Immunol (2014) 75:923–9. doi: 10.1016/j.humimm.2014.06.021
50. Jones BG, Oshansky CM, Bajracharya R, Tang L, Sun Y, Wong SS, et al. Retinol Binding Protein and Vitamin D Associations With Serum Antibody Isotypes, Serum Influenza Virus-Specific Neutralizing Activities and Airway Cytokine Profiles. Clin Exp Immunol (2016) 183:239–47. doi: 10.1111/cei.12718
51. Chelbi-Alix MK, Pelicano L. Retinoic Acid and Interferon Signaling Cross Talk in Normal and RA-Resistant APL Cells. Leukemia (1999) 13:1167–74. doi: 10.1038/sj.leu.2401469
52. Giandomenico V, Lancillotti F, Fiorucci G, Percario ZA, Rivabene R, Malorni W, et al. Retinoic Acid and IFN Inhibition of Cell Proliferation Is Associated With Apoptosis in Squamous Carcinoma Cell Lines: Role of IRF-1 and TGase II-Dependent Pathways. Cell Growth Differ (1997) 8:91–100.
53. Kohge N, Sato S, Hamamoto S, Akagi S, Uchida Y, Miyake T, et al. Retinol Supplements Antiviral Action of Interferon in Patients With Chronic Hepatitis C: A Prospective Pilot Study. J Clin Biochem Nutr (2010) 46:36–42. doi: 10.3164/jcbn.09-48
54. Kuwata T, Wang IM, Tamura T, Ponnamperuma RM, Levine R, Holmes KL, et al. Vitamin A Deficiency in Mice Causes a Systemic Expansion of Myeloid Cells. Blood (2000) 95:3349–56. doi: 10.1182/blood.V95.11.3349
55. Hurwitz JL, Jones BG, Penkert RR, Gansebom S, Sun Y, Tang L, et al. Low Retinol-Binding Protein and Vitamin D Levels Are Associated With Severe Outcomes in Children Hospitalized With Lower Respiratory Tract Infection and Respiratory Syncytial Virus or Human Metapneumovirus Detection. J Pediatr (2017) 187:323–7. doi: 10.1016/j.jpeds.2017.04.061
56. Luengen AE, Kniebs C, Buhl EM, Cornelissen CG, Schmitz-Rode T, Jockenhoevel S, et al. Choosing the Right Differentiation Medium to Develop Mucociliary Phenotype of Primary Nasal Epithelial Cells In Vitro. Sci Rep (2020) 10:6963. doi: 10.1038/s41598-020-63922-8
57. Genaro Pde S, Martini LA. Vitamin A Supplementation and Risk of Skeletal Fracture. Nutr Rev (2004) 62:65–7. doi: 10.1111/j.1753-4887.2004.tb00026.x
58. Bresee JS, Fischer M, Dowell SF, Johnston BD, Biggs VM, Levine RS, et al. Vitamin A Therapy for Children With Respiratory Syncytial Virus Infection: A Multicenter Trial in the United States. Pediatr Infect Dis J (1996) 15:777–82. doi: 10.1097/00006454-199609000-00008
59. Jones BG, Sealy RE, Penkert RR, Surman SL, Maul RW, Neale G, et al. Complex Sex-Biased Antibody Responses: Estrogen Receptors Bind Estrogen Response Elements Centered Within Immunoglobulin Heavy Chain Gene Enhancers. Int Immunol (2019) 31:141–56. doi: 10.1093/intimm/dxy074
Keywords: cystic fibrosis, vitamin A, respiratory virus infection, mouse model, prevention
Citation: Sealy RE, Surman SL, Vogel P and Hurwitz JL (2021) Might Routine Vitamin A Monitoring in Cystic Fibrosis Patients Reduce Virus-Mediated Lung Pathology? Front. Immunol. 12:704391. doi: 10.3389/fimmu.2021.704391
Received: 02 May 2021; Accepted: 19 October 2021;
Published: 09 November 2021.
Edited by:
Susana Alvarez, Centro de Referencia para Lactobacilos (CONICET), ArgentinaReviewed by:
Susana Salva, Centro de Referencia para Lactobacilos (CONICET), ArgentinaRoberto Rosales-Reyes, National Autonomous University of Mexico, Mexico
Ángel Gabriel Valdivieso, Institute for Biomedical Research (CONICET), Argentina
Copyright © 2021 Sealy, Surman, Vogel and Hurwitz. This is an open-access article distributed under the terms of the Creative Commons Attribution License (CC BY). The use, distribution or reproduction in other forums is permitted, provided the original author(s) and the copyright owner(s) are credited and that the original publication in this journal is cited, in accordance with accepted academic practice. No use, distribution or reproduction is permitted which does not comply with these terms.
*Correspondence: Julia L. Hurwitz, Julia.hurwitz@stjude.org