- 1Department of Molecular Medicine, “Sapienza” University of Rome, Rome, Italy
- 2Istituto Pasteur Italia-Cenci Bolognetti Fondazione, Rome, Italy
- 3German Red Cross Blood Donor Services, Baden-Wuerttemberg-Hessia, Ulm, Germany
- 4Science for Life Laboratory, Department of Medicine Solna, Karolinska Institutet, Stockholm, Sweden
- 5Division of Infectious Diseases, Karolinska University Hospital, Stockholm, Sweden
- 6Department of Cellular Biotechnologies and Hematology, “Sapienza” University of Rome, Rome, Italy
- 7Department of Clinical and Molecular Medicine, “Sapienza” University of Rome, Rome, Italy
Natural killer (NK) cells are immune innate effectors playing a pivotal role in the immunosurveillance of multiple myeloma (MM) since they are able to directly recognize and kill MM cells. In this regard, among activating receptors expressed by NK cells, NKG2D represents an important receptor for the recognition of MM cells, being its ligands expressed by tumor cells, and being able to trigger NK cell cytotoxicity. The MHC class I-related molecule A (MICA) is one of the NKG2D ligands; it is encoded by highly polymorphic genes and exists as membrane-bound and soluble isoforms. Soluble MICA (sMICA) is overexpressed in the serum of MM patients, and its levels correlate with tumor progression. Interestingly, a methionine (Met) to valine (Val) substitution at position 129 of the α2 heavy chain domain classifies the MICA alleles into strong (MICA-129Met) and weak (MICA-129Val) binders to NKG2D receptor. We addressed whether the genetic polymorphisms in the MICA-129 alleles could affect MICA release during MM progression. The frequencies of Val/Val, Val/Met, and Met/Met MICA-129 genotypes in a cohort of 137 MM patients were 36, 43, and 22%, respectively. Interestingly, patients characterized by a Val/Val genotype exhibited the highest levels of sMICA in the sera. In addition, analysis of the frequencies of MICA-129 genotypes among different MM disease states revealed that Val/Val patients had a significant higher frequency of relapse. Interestingly, NKG2D was downmodulated in NK cells derived from MICA-129Met/Met MM patients. Results obtained by structural modeling analysis suggested that the Met to Val dimorphism could affect the capacity of MICA to form an optimal template for NKG2D recognition. In conclusion, our findings indicate that the MICA-129Val/Val variant is associated with significantly higher levels of sMICA and the progression of MM, strongly suggesting that the usage of soluble MICA as prognostic marker has to be definitely combined with the patient MICA genotype.
Introduction
Natural killer (NK) cells represent innate immune effectors playing a pivotal role in tumor surveillance. NK cell activation is regulated by a delicate balance between activating and inhibitory signals, with the latter being primarily transduced by receptors for MHC class I molecules (KIRs, CD94/NKG2A). Recognition of abnormal self on tumor cells triggers a number of non-MHC class I-restricted activating receptors, such as NK group 2D (NKG2D), DNAX accessory molecule-1 (CD226), and the natural cytotoxicity receptors (1).
NKG2D is an activating receptor expressed on the surface of NK cells, CD8+ T cells, and subsets of CD4+ T cells, invariant NKT cells (iNKT), and γδ T cells (1). NKG2D recognizes two families of ligands in humans: the MHC class I chain-related protein A/B (MICA/B) and the UL16-binding proteins (ULBP1-6) (1). In general, healthy adult tissues do not express NKG2D ligands on the cell surface, but the expression levels of these molecules can be significantly induced by various physiological and pathological “stress” circumstances, including infection by different pathogens (1), cell division (2), and neoplastic transformation (3). Among all known NKG2D ligands, MICA is the most polymorphic non classical class I gene, with 104 alleles identified to date (http://www.ebi.ac.uk/imgt/hla/, release 3.25.0). Some MICA polymorphisms have raised a great interest since they can affect MICA biology. For instance, the MICA*008 allele (rs67841474) contains a guanine (G) insertion that causes a premature stop codon that, in turn, crops 10 amino acids of the transmembrane domain as well as the cytoplasmic tail. In contrast to other MICA alleles that are shed as truncated soluble species after metalloproteinase-mediated cleavage, it is released from cells associated to exosomes (4). In addition, the single-nucleotide polymorphism causing a valine (Val) to methionine (Met) modification at position 129 of the α2 heavy chain domain classifies these MICA alleles into high-affinity (MICA-129Met) and low-affinity (MICA-129Val) binders to NKG2D receptor (5). It has also been recently reported that MICA-129Met, characterized by stronger and faster NKG2D signaling, is able to trigger relatively higher NK cell cytotoxicity and IFNγ release accompanied by rapid downregulation of NKG2D (6). Significant differences in binding affinities of MICA alleles for NKG2D could have different effects on NK cell activation, in particular under conditions of suboptimal MICA expression.
Multiple myeloma (MM) is a clonal B cell malignancy characterized by the expansion of plasma cells (PCs) in the bone marrow (BM) and is still an incurable disease with a median survival of few years. Its prognosis has been improved by the use of autologous hematopoietic stem cell transplantation (7) and new immunochemotherapeutic approaches (8–10). NK cells play a pivotal role in MM immunosurveillance by exerting direct cytotoxic effects through a number of activating receptors, including NKG2D (11, 12). However, several mechanisms have been identified that permit the escape of tumors bearing NKG2D ligands, including their release by tumor cells through proteolytic cleavage (13–17) or exosome secretion (4). Furthermore, it has been demonstrated that MICA is transferred to NK cells upon target conjugation and that this transfer is directly linked to molecular interactions between NKG2D and MICA, following accumulation of the ligand at the immunological synapse (18). Soluble MICA has been identified as an independent prognostic factor for the overall survival and progression-free survival of MM patients (19). In addition, endogenous anti-MICA antibodies and ligand shedding are critical determinants of host immunity during MM progression (20). It is, however, unknown whether functionally relevant polymorphisms of the MICA gene may also contribute to disease progression.
The aim of this study was to investigate the association of MICA genetic polymorphisms and MICA sera levels with progression of MM. Interestingly, our findings indicate that the MICA-129Met/Val dimorphism is associated with: (i) differential expression of both soluble and cell-surface MICA, (ii) expression levels of NKG2D on ex vivo NK cells isolated from the BM and peripheral blood (PBL) of MM patients, and (iii) the disease state.
Results
MICA-129Val Allele Is Associated With Higher Amount of Soluble MICA in the Sera of MM Patients
Soluble MICA has been proposed as a prognostic marker in MM since its levels correlate with tumor progression (19). However, the generation of soluble MICA can be affected by polymorphisms, regulating cell-surface expression, altering the efficacy of cleavage, and favoring MICA recruitment into exosome-like vesicles (4, 16, 21, 22). At first, we investigated whether soluble NKG2D ligands other than MICA in the sera derived from a cohort of MM patients at different disease states, namely MGUS (monoclonal gammopathy of undetermined significance), smoldering, onset, and relapse, were associated with MM progression. As shown in Figure 1A, we established that only soluble MICA but not other soluble NKG2D ligands including MICB and ULBP1-3 (data not shown) were associated to MM progression. Since we confirmed the importance of soluble MICA, we further explored whether MICA polymorphism could affect the amount of soluble MICA as well as MM progression. Therefore, MICA genotype was examined by isolating PBMCs DNA from a cohort of 137 MM patients at different disease states (Figure S1A in Supplementary Material). We also identified the sequence of a new MICA allele and the name MICA*085 has been officially assigned by the WHO Nomenclature Committee for factors of the HLA System. MICA alleles were further classified in three subgroups, MICA-129Val/Val, MICA-129Val/Met, and MICA-129Met/Met (Tables 1 and 2). Similarly to other studies (6, 23–25), the frequencies of MICA-129Val/Val, MICA-129Val/Met, and MICA-129Met/Met genotypes were 36, 42, and 22%, respectively (Figure S1B in Supplementary Material). Remarkably, the analysis of MICA-129 genotype frequencies among different MM states revealed that MICA-129Val/Val patients displayed a significantly higher percentage of relapse (Figure 1B; Figure S1C in Supplementary Material). In contrast, the frequencies of MICA-129Val/Met and MICA-129Met/Met genotypes were similar throughout all the different disease states (Figure 1B). Interestingly, MM patients characterized by the MICA-129Val/Val genotype also exhibited the highest levels of soluble MICA in the sera (Figure 1C). Consequently, correlation of soluble MICA with MM progression was observed only in the presence of the MICA-129Val allele (Figure 1D). We further explored the outcome of patients in response to the therapy among different MICA-129 genotypes (Figures 2A–C). As shown in Figure 2A, a similar response to chemotherapy was observed among the three genotypes. Interestingly, we observed that the highest frequency of relapse was developed by MICA-129Val/Val patients also after chemotherapeutic treatment (Figure 2B) suggesting that MICA polymorphism impacts on MM relapse.
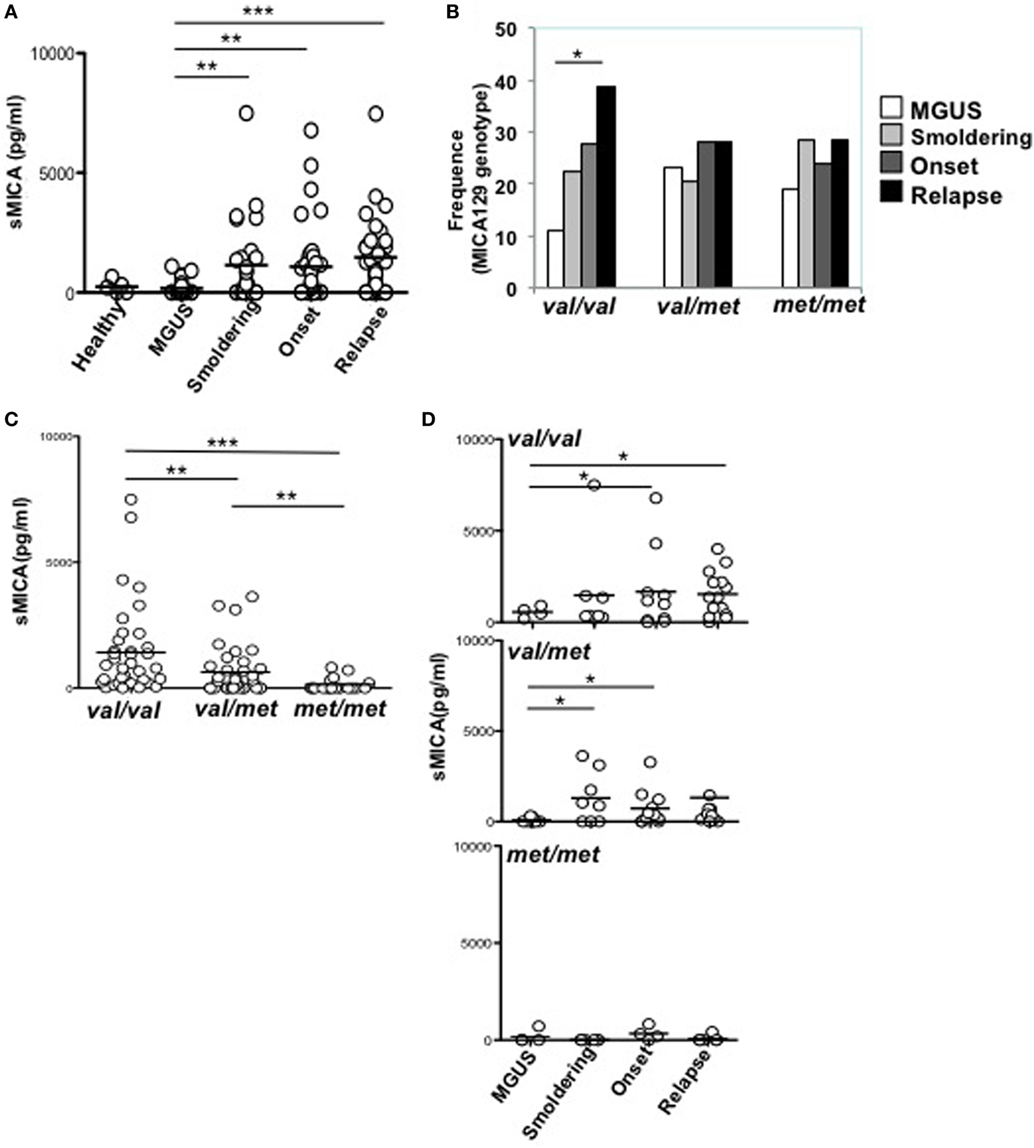
Figure 1. MICA-129Val allele is associated with higher amount of sMICA in the sera of multiple myeloma (MM) patients. (A) Sera derived from healthy donors, MGUS, and MM patients at different state disease were analyzed for the presence of soluble MICA through a specific enzyme-linked immunosorbent assay. Total number of patients, 97 (healthy, n = 5, MGUS, n = 16, smoldering, n = 22, onset, n = 26, relapse, n = 32). (B) Frequency distribution of MICA-129 genotypes among different MM disease states. χ2 test with n − 1 degrees of freedom was performed. (C,D) sMICA is associated with the presence of the MICA-129 valine (Val) allele in MM patients. Total number of patients analyzed, n = 91 (Val/Val, n = 37; Val/Met, n = 36; Met/Met, n = 18).
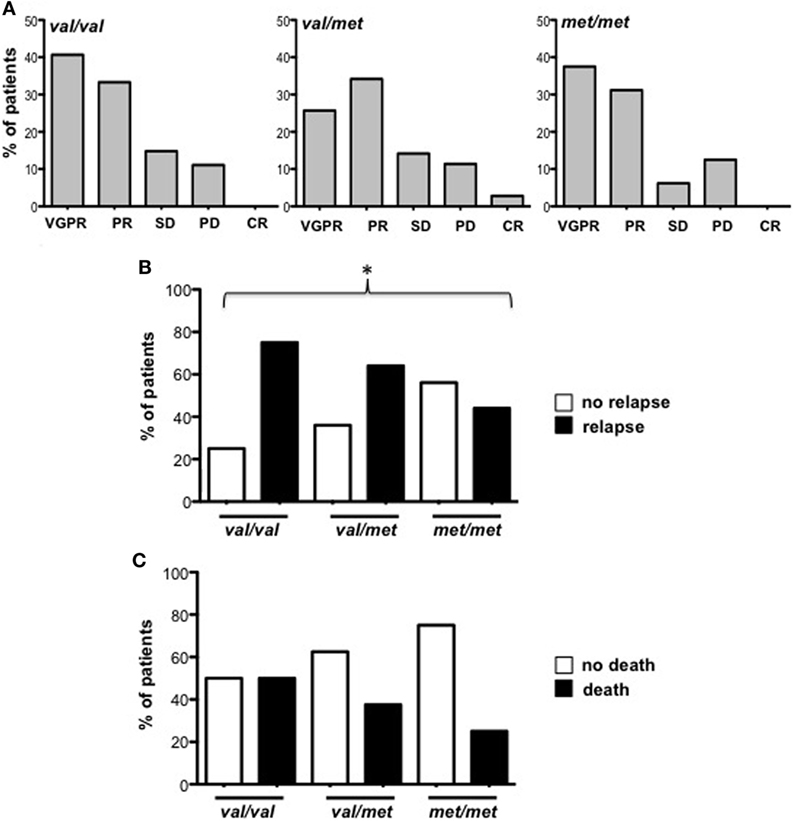
Figure 2. Patient outcome related to MICA-129 genotype. (A) Response to therapeutic treatment of multiple myeloma (MM) patients (Onset and Relapse). VGPR, very good partial response; PR, partial response; SD, stable disease; PD, partial disease, CR, complete remission. (B) Frequency of relapse development in MM patients after chemotherapy. χ2 test, p = 0.0413. (C) Frequency of deceased patients in MM patients after chemotherapy. Total patients, n = 74; Val/Val, n = 26; Val/Met, n = 32; Met/Met, n = 16. Abbreviations: Val, valine; Met, methionine.
We asked whether MICA cell-surface expression levels on primary malignant PCs isolated from patients could be related to the MICA genotype. As shown in Figures 3A–C, MICA expression on malignant PCs, was significantly higher in MICA-129Val/Val MM patients compared to MICA-129Met/Met MM patients, thus suggesting that the increased amount of soluble MICA in the sera of MICA-129Val/Val patients could be related to an higher expression of this allelic variant. Finally, we further classified MICA alleles into MICA short and long, based on the presence of the truncated MICA*008 allelic variant, but no differences regarding soluble MICA serum levels and the correlation with the disease state were found (data not shown).
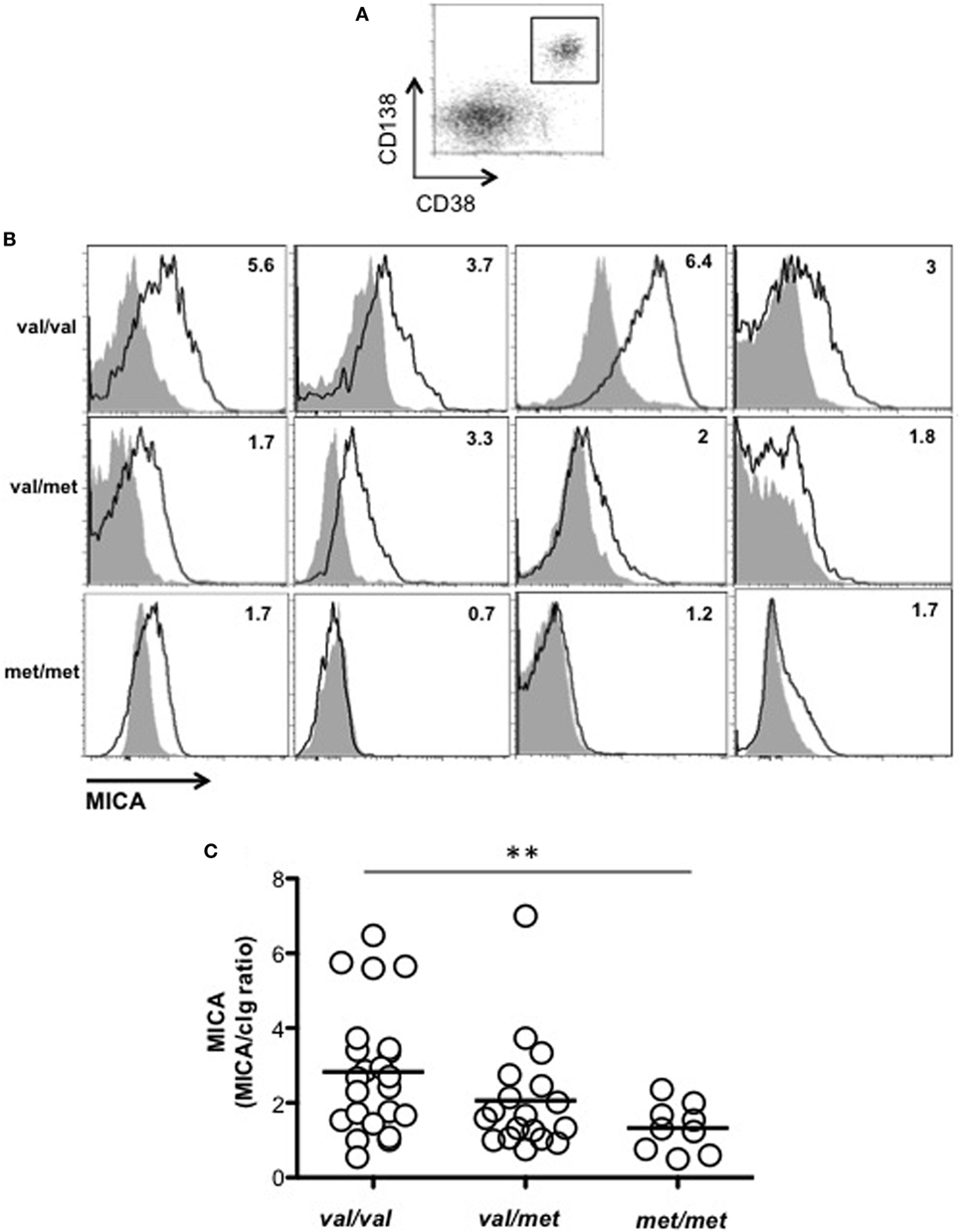
Figure 3. MICA cell-surface expression on malignant plasma cells (PCs) resulted higher in MICA-129Val/Val patients. Total cells derived from the bone marrow (BM) of patients at different disease states were stained with isotypic cIg or anti-MICA together with anti-CD38 and anti-CD138 mAbs. (A) MICA expression was analyzed by gating CD38+/CD138+ cells. (B) Representative histograms of different patients are reported. Values indicated in each histogram represent the ratio between the MFI value of MICA divided by the MFI value of the isotypic cIg. Solid gray histogram: cIg; black line: MICA. (C) Total number of patients analyzed, n = 50 (Val/Val = 23, Val/Met n = 18, Met/Met = 9) that were smoldering (n = 10), onset (n = 19), and relapse (n = 21). Abbreviations: Val, valine; Met, methionine.
MICA-129Met Allele Is Associated With an Increased NKG2D Downmodulation on NK Cells Isolated From Patients
We next investigated whether MICA-129 polymorphism was also associated with different levels of NKG2D expression in MM patients. To this aim, NKG2D expression levels on ex vivo NK cells isolated from either PBL or BM of MM patients were evaluated. Cells were stained with anti-human CD45, -CD56, -CD3, -CD138 mAbs, along with mAbs specific for NKG2D and DNAM-1 activating receptors. After CD138 (corresponding to PCs) gate exclusion, analysis was performed on CD45+CD56+CD3− total NK cells. Interestingly, our results demonstrate that NKG2D was significantly reduced on NK cells derived from MICA-129Met/Met patients compared to both MICA-129Val/Val and MICA-129Val/Met patients (Figures 4A,B). Indeed, the MICA-129Met allele that has a higher affinity to NKG2D, is able to induce significantly stronger downmodulation of NKG2D in both NK and CD8 T lymphocytes (6). Importantly, the expression levels of DNAM-1, used as control, were similar among all three different genotypes (Figures 4A,B), indicating that NKG2D downmodulation is an event likely associated to MICA-129 dimorphism. Decreased NKG2D expression on MICA-129Met/Met patients was also observed on NK cell subsets expressing low and high levels of CD16 as shown in Figures S2A,B in Supplementary Material. These results suggest that NKG2D downmodulation in MM patients depends essentially on MICA genotype and it is not associated with soluble MICA levels. To further support this observation, sera containing different amounts of soluble MICA (derived from patients carrying at least one Val allele) were incubated with PBL derived from healthy donors and NKG2D expression was evaluated after 16 h by immunofluorescence and FACS analysis by gating on CD56+CD3− NK cells. As shown in Figure 5, we did not observe a significant correlation between the levels of soluble MICA and the extent of NKG2D reduction.
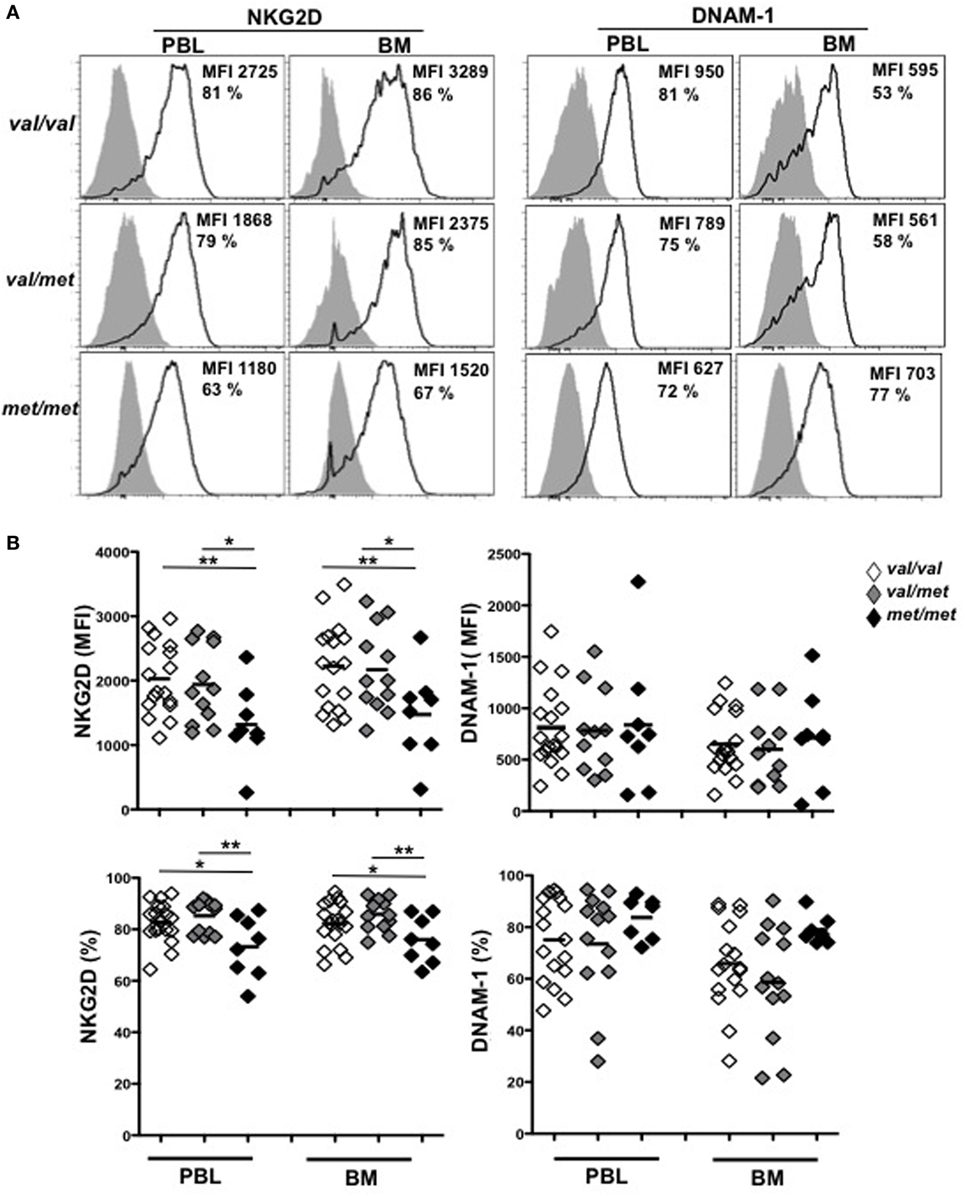
Figure 4. MICA-129Met allele is associated with an increased NKG2D downmodulation on natural killer (NK) cells gated from patients. Total cells derived from the bone marrow (BM) or the peripheral blood (PBL) derived from patients at different disease states (including smoldering, onset, and relapse) were stained with antibodies against CD45, CD138, CD56, and CD3. NKG2D or DNAM-1 expression was evaluated by gating on NK cells (CD45+CD138−CD3− CD56+). (A) Representative histograms are shown. (B) Values reported represent the MFI values of NKG2D or DNAM-1 subtracted from the MFI value of the isotypic cIg or the percentage of NKG2D and DNAM-1. Total number of patients analyzed, n = 40 (Val/Val = 19, Val/Met n = 13, Met/Met = 8). Abbreviations: Val, valine; Met, methionine.
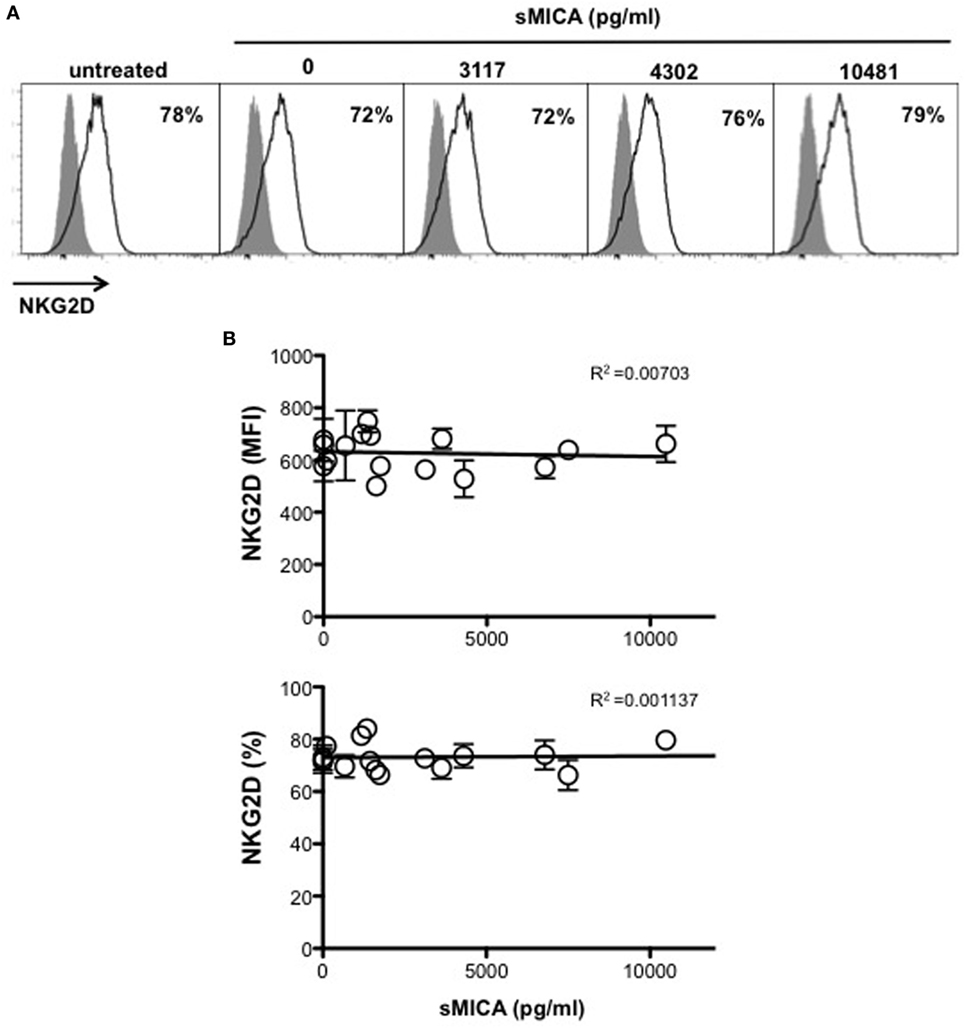
Figure 5. Soluble MICA levels in the serum of multiple myeloma (MM) patients carrying the Val allele do not correlate with change in NKG2D expression. Peripheral blood derived from healthy donors were incubated for 16 h with medium alone or serum derived from MGUS or MM patients at different disease states carrying at least one Val allele and containing variable levels of soluble MICA. Cells were harvested and NKG2D expression was evaluated by gating on CD3−CD56+ natural killer (NK) cells. (A) A representative experiment is shown. (B) Values reported on y axis represent the MFI value of NKG2D subtracted from the MFI value of the isotypic cIg (high panel) or the percentage of NKG2D positive cells (lower panel) and were correlated with soluble MICA levels of each patient as indicated on x axis. Total number of serum patients analyzed, n = 16 (3 MGUS, 6 smoldering, 6 onset, 1 relapse).
Residue Met129 Is Essential for Appropriate Positioning of the α2–1 Helix for NKG2D Recognition
Two crystal structures of MICA have been hitertho determined, one alone (26) and the other in complex with its receptor NKG2D (27). At first sight, MICA resembles classical MHC class I (MHC-I) molecules with three extracellular domains (α1, α2, and α3), a transmembrane segment that can vary significantly between different MICA alleles, and a carboxy-terminal cytoplasmic tail. However, in contrast to MHC-I, MICA does not bind to the β2microglobulin and does not present peptides in the cleft. Comparison of the two structures revealed that the receptor-free form of MICA is disordered within a section of the α2 region corresponding to residues 152 to 161, essential for NKG2D recognition (27) (Figure 6A). Interestingly, the crystal structure of MICA in complex with NKG2D revealed that these nine MICA residues, which link the helices α2–1 and α2–2, are ordered upon binding to NKG2D (Figure 6B). The crystal structures also revealed that residue Met129 is localized at the end of the small β8-strand, far away from the MICA/NKG2D interface. This residue forms, together with Trp127, Phe110, Phe117, and Leu118, a hydrophobic base on which the helix α2–1 docks (Figure 6C). The importance of such hydrophobic nucleus for the correct folding and orientation of a helix has been previously demonstrated (28). The hydrophobic residues Leu138, Ala139, Met140, and Val142 on the α2–1 helix face and interact with the β-sheet docking site described above (Figure 6C). The large and hydrophobic residue Met129 is at the heart of this putative nucleating site, forming van der Waals interactions with residues Gln136, Ala139, and Met140 all localized on the α2–1 helix (Figure 6C).
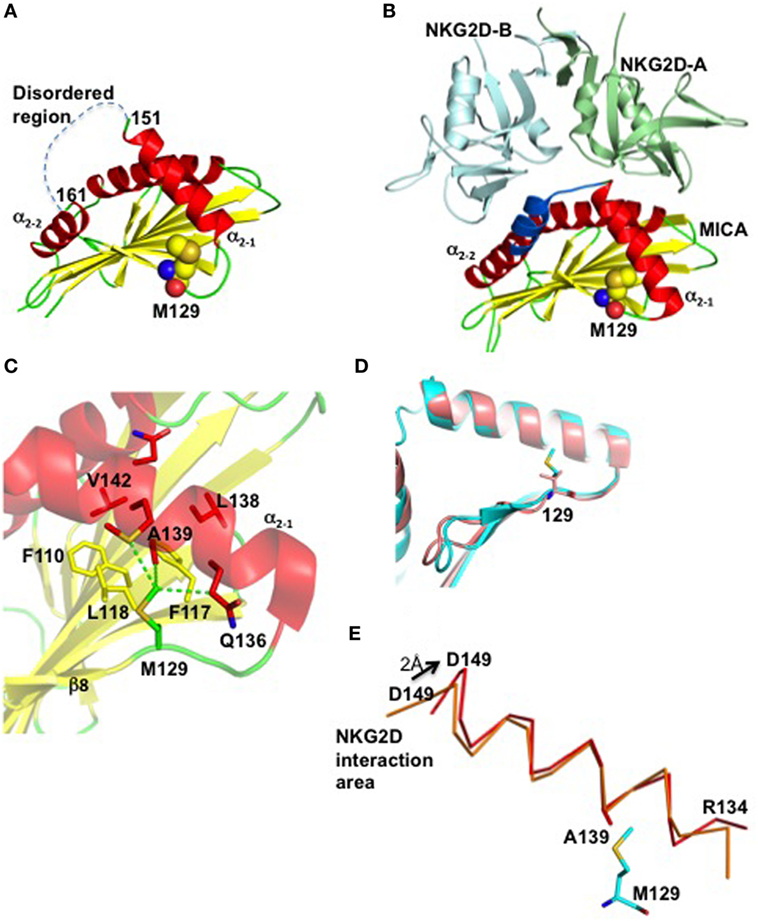
Figure 6. Residue Met129 is essential for appropriate positioning of the α2–1 helix for NKG2D recognition. (A) The crystal structure of the free form of MICA is colored according to its secondary structure, with helices, β-strands and loops in red, yellow, and green, respectively. Only the α1 and α2 regions of MICA are displayed. The region comprising residues 152–161 and which is not visible in the electron density due to disorder, is indicated by a dashed line. All atoms forming residue Met129 are indicated as balls with oxygen, nitrogen, sulfur, and carbon atoms colored in red, blue, orange, and yellow, respectively. (B) The crystal structure of the MICA/NKG2D complex reveals that the flexible 152–161 region (in blue) is stabilized through interactions with NKG2D. The two subunits of NKG2D receptor are displayed in green and cyan. Residue Met129 is localized far away from the MICA/NKG2D interface. (C) Residue Met129 plays a key role within a hydrophobic core formed between helix α2–1 and several β-sheet residues that surround and interact with Met129. The van der Waals interactions formed between Met129 and residues (in red) on the helix α2–1 are indicated by green dashed lines. (D) Superposition of the α1α2 domains of MICA and MICB, in pink and cyan, respectively, reveals that Met129 in MICA and Val129 in MICB take the same position and orientation, and demonstrates that MICA-129Met/Val dimorphism does not affect the conformation of the two MIC alleles (29). (E) The MICA α2–1 helix rotates following complex formation with NKG2D. Both ends of free MICA (red) are deviating from their position in the complex with NKG2D (orange), by 1.5 and 2.0 Å at the N- and C-termini, respectively.
The clear differences in binding affinity between the two MICA variants and NKG2D have been previously suggested to be due to conformational changes (5). However, this is in our opinion unlike since comparison of the crystal structure of MICB (30) which comprises a Val residue at position 129, with the crystal structure of MICA-129Met, reveals the similarity of their conformations (Figure 6D). Instead, molecular modeling analysis indicated that replacement of residue Met129 with the much smaller Val residue removes at least three van der Waals interactions between the α2–1 helix and the β-sheet on which it docks, and generates a solvent accessible cavity in this hydrophobic core. Furthermore, comparative analysis of the two crystal structures of MICA indicates that the α2–1 helix rotates slightly upon NKG2D binding around the contact between Met129 and Ala139, resulting in significant movements at both ends of this helix (Figure 6E). Mutation of residue Met129, which we believe acts a lever stop for the helix α2–1 to build the conformation optimal for the NKG2D binding, would clearly have a significant impact on the direction and tilting of the helix. Thus, the Met to Val dimorphism could affect the MICA transition from disorder to form an optimal template for NKG2D recognition, providing a reasonable, although still hypothetical, explanation for the difference in the binding capacity of the two MICA variants to NKG2D.
Discussion
In this study, we investigated the association of MICA genetic polymorphisms and sera levels with the progression of MM. Interestingly, our findings indicate that the MICA-129Met/Val dimorphism is associated with: (i) differential expression of both soluble and cell surface MICA, (ii) expression levels of NKG2D on ex vivo NK cells isolated from the BM and PBL of MM patients, and (iii) the disease state.
Polymorphisms of MICA have been largely investigated for their role in infections, autoimmune diseases, and cancer (31). Due to its functional consequences, a number of disease association studies with the MICA-129 dimorphism have been previously performed (32). Interestingly, we found an higher frequency of relapse in MM patients carrying the MICA-129Val/Val genotype that was also observed by analyzing the patients outcome in response to the therapy.
Other studies in different cancer models have reported that the MICA-129Val/Val genotype can be associated with higher risk for nasopharyngeal (NC) and breast cancer (33, 34). Increasing evidence has pointed out a key role for the NKG2D activating receptor and its ligands in the surveillance of MM. In particular Rebmann and coworkers have shown that soluble MICA levels correlate with tumor progression, and this molecule has been proposed as a prognostic marker in MM (19). Our findings demonstrate that increased levels of soluble MICA can be found in MM patients sera during the progression from MGUS to relapse and clearly show for the first time that the presence of soluble MICA is associated with the MICA-129Val allele. As matter of fact, in other pathological conditions, including Ulcerative Colitis and Hepatitis B infection (35, 36), the MICA-129Val/Val genotype has been associated with the highest soluble MICA serum levels.
In line with our in vivo results showing both a higher expression of cell surface and soluble MICA in MICA-129Val/Val patients, Isernhagen and colleagues reported that soluble MICA levels as well as its cell-surface expression were higher in a panel of tumor and melanoma cell lines carrying the MICA-129Val/Val genotype (21). It is possible that the MICA-129Val allele has a higher transcriptional activity which might explain its effect on soluble and cell-surface MICA levels (37). Another non-excluding possibility is that the higher amount of soluble MICA-129Val could be directly due to the 30-times lower affinity of this variant to NKG2D compared to MICA-129Met resulting in a reduced transfer to NK cells and its accumulation on the surface of MM target cells.
Previous studies suggested that elevated levels of soluble MICA in the sera of cancer patients correlate with an increased NKG2D downregulation on PBL NK cells and T lymphocytes (22). However, it is still unclear whether soluble MICA has the capability to directly induce NKG2D downregulation and/or if additional soluble factors in the sera of cancer patients contribute to this effect. Infact, Paschen and coworkers demonstrated that elevated levels of soluble NKG2D ligands (i.e., MICA and ULBP2) in the sera of melanoma patients were not associated with a significant downregulation of NKG2D expression on peripheral NK cells (38). Even NK cells from rheumatoid arthritis patients with relatively high soluble MICA levels, did not show diminished NKG2D expression (39). Furthermore in MM, soluble MICA was not significantly associated with NKG2D downregulation and in vitro experiments with MM patients’ serum and culture supernatants, did not result in changes in NKG2D expression (40). Similarly, by incubating NK cells with sera from patients containing different amounts of soluble MICA, we did not find a significant correlation between the levels of soluble MICA and the extent of NKG2D downmodulation. Interestingly, we observed that the lowest levels of NKG2D on NK cells from MM patients, were associated with the MICA-129Met/Met genotype. In line with these findings, it has been shown that the MICA-129Met allele, with proven higher affinity to NKG2D, is able to induce significantly stronger downmodulation of NKG2D in both NK and CD8 T lymphocytes, and to better stimulate IFNγ production as compared to the MICA-129Val allele (6). It should be considered that receptor endocytosis not only leads to reduced cell-surface receptor abundance but also controls signaling outcome in NK cells as shown by Molfetta and coworkers who reported that ubiquitin-dependent NKG2D/DAP10 endocytosis was required for the activation of extracellular signal-regulated kinase and NK cell functions (41, 42). It is possible that cancer cells carrying the MICA-129Met allele could better induce NK cell activation that corresponded to a substantial NKG2D reduction observed in patients. By performing in vitro degranulation assays on NKG2D-sensitive targets, “ex vivo” unstimulated NK cells derived from patients had very low levels (below 5%) of degranulation, independently of the MICA genotype (data not shown). This result is in accordance with previous evidences indicating that stimulation of NKG2D alone is not sufficient to trigger cytotoxicity and/or cytokine production in resting human NK cells (43). In fact, effector functions mediated by this receptor rely on different factors including the activation status of NK cells, the cooperation with other NK activating receptors or with distinct cytokines (44). Thus, in MM patients, the tumor microenvironment, the cytokine milieu and the expression of other NK cell activating ligands on cancer cells can dictate the final outcome of the NKG2D-mediated NK cell response.
Our results obtained by structural modeling analysis suggested that the Met to Val dimorphism could affect the capacity of MICA to form an optimal template for NKG2D recognition. It is possible that the lowest NKG2D levels in MM patients with a MICA-129Met/Met genotype reflects the capacity of the MICA-129Met allele to more efficiently engage NKG2D and trigger NK cell activity in a cell–cell contact manner and this event appears to be independent from soluble MICA sera levels that are instead predominant in individuals carrying the MICA-129Val allele.
In conclusion, our data indicate that the MICA-129Val allele is associated with significantly higher levels of soluble MICA and an higher frequency of relapse and strongly suggest that the MICA genotype could be used as prognostic marker in alternative to soluble MICA if further data with higher numeric dimension will confirm these findings. Altogether, these observations could help to develop more personalized predictive biomarkers in MM.
Materials and Methods
Clinical Samples
Sera, PBMCs, and BM samples were obtained from MM patients enrolled at the Division of Hematology (Sapienza University of Rome). Informed and written consent in accordance with the Declaration of Helsinki was obtained from all patients, and approval was obtained from the Ethics Committee of the Sapienza University of Rome (Rif. 3373). Patients were classified according to the disease state. Patients (Onset and Relapse) were treated according to standard therapeutic protocols including the usage of VMP (Bortezomib, Melphalan, Prednisone), VD (Bortezomib, Dexamethasone), and RD (Lenalidomide, Dexamethasone).
MICA Gene Typing
For the genotyping of MICA, genomic DNA derived from patients PBMCs was isolated from 1 × 106 cells using the Genomic DNA purification kit according to the manufacturer’s instructions (Bioline, London, UK). Sequence-based typing of MICA was performed as described before (45). The sequence of a new MICA allele was identified and the name MICA*085 has been officially assigned by the WHO Nomenclature Committee for factors of the HLA System in February 2015 (Genbank accession: KP262025).
Immunofluorescence and FACS Analysis
Analysis of MICA expression on patient-derived PCs was performed by gating the CD38+CD138+ PC population using the antibodies anti-MICA (clone 159227, R&D Systems, Minneapolis, MN, USA), anti-CD38/APC, and anti-CD138/FITC (both from BD Bioscience, San Jose, CA, USA) as previously reported (16); samples were acquired using a FACSCanto (BD Biosciences, San Jose, CA, USA) and a FACSCalibur (Becton Dickinson). Analysis of NKG2D and DNAM-1 on NK cells from PBMCs or BM aspirates was performed by gating on the CD45+CD138−CD3−CD56+ population using the antibodies anti-CD3/allophycocyanin-H7, anti-CD56/PE, anti-CD138/FITC, anti-CD45/PE-Cy7, anti-NKG2D/APC, or anti-DNAM-1/APC (BD Bioscience). In some experiments, anti-CD16/PerCP mAb was also used (BD Bioscience). In the experiments relative to Figure 4 and Figure S2 in Supplementary Material, all the patients-derived samples were acquired using a FACSCanto (BD Biosciences, San Jose, CA, USA). In the experiments relative to Figure 5, PBMCs derived from healthy donors were incubated with medium alone or serum derived from MGUS or MM patients for 16 h. After harvesting, cells were stained with antibodies from BD Bioscience: anti-CD3/PerCP, anti-CD56/APC, and anti-NKG2D/PE; samples were acquired using a FACSCalibur (Becton Dickinson).
Data analysis was performed using the FlowJo 9.3.2 program (TreeStar, Ashland, OR, USA).
Enzyme-Linked Immunosorbent Assay (ELISA)
Enzyme-linked immunosorbent assays for soluble MICA, MICB, and ULBP1 were from R&D Systems (Minneapolis, MN, USA), and performed as previously described (46), with modifications (AMO1 anti-MICA capture mAb, 2 µg/ml, BAMOMAB, Germany) (47). Soluble ULBP2 was detected as previously described (15). Absorbance values of triplicate samples were obtained by subtracting readings at 540 nm from readings at 450 nm. Net absorbance was obtained by subtracting the reagent blank absorbance. Before the assay, sera samples were diluted in PBS/0.1% Triton X-100 (vol/vol) and incubated for 30 min at 37 C.
Molecular Modeling of the MICA-Val129 Variant and Structural Analysis
In order to evaluate the structural consequences of the MICA polymorphism, we created a three-dimensional molecular model of the MICA-Val129 variant, using the crystal structures of the free MICA-Met129 molecule (PDB code 1B3J) and the MICA-Met129/NKG2D complex (PDB code 1HYR) (26, 27) as templates. The creation of the molecular model of the MICA-Val129 variant, as well as all comparative structural analyses, was performed using the program Coot (48). Figure 5 was created using the program PyMol (PyMol Molecular Graphics System, Version 1.5.0.4 Schrödinger, LLC).
Statistic
In all the experiments, statistic was performed using the unpaired Mann–Whitney test, except for Figure 1D in which the unpaired t-test with Welch’s correction was used. *<0.05; **<0.01; ***<0.001. χ2 test was used to analyze frequency data.
Ethics Statement
Informed and written consent in accordance with the Declaration of Helsinki was obtained from all patients, and approval was obtained from the Ethics Committee of the Sapienza University of Rome.
Author Contributions
FC, MG, and IN, extracted patients’ DNA, collected patients’ sera, and performed ELISA experiments. EV and AP performed experiments on bone marrow and peripheral blood of patients. DF, TSaribekyan, and JM performed MICA gene typing. AA and TSandalova performed structural modelling. EM, MP, and MR managed patients and evaluated clinical parameters. CF, ASoriani, MC, and CC, analyzed and discussed data. AZ and ASantoni designed the experiments and wrote the paper.
Conflict of Interest Statement
The authors declare that the research was conducted in the absence of any commercial or financial relationships that could be construed as a potential conflict of interest.
Funding
This work was supported by Associazione Italiana per la Ricerca sul Cancro (AIRC) [grant 16014 and multiunit ext. 9962]; the Italian Ministry of University and Research (MIUR) [PROT.20103FMJEN_001]; and PRIN 2015-W729WH to CC; the Swedish Research Council (Cancerfonden) and the Swedish Research Council (Vetenskapsrådet) to AA. EV is supported by a fellowship from AIRC (Associazione Italiana per la Ricerca sul Cancro).
Supplementary Material
The Supplementary Material for this article can be found online at https://www.frontiersin.org/articles/10.3389/fimmu.2018.00926/full#supplementary-material.
References
1. Lanier LL. NKG2D receptor and its ligands in host defense. Cancer Immunol Res (2015) 3:575–82. doi:10.1158/2326-6066.CIR-15-0098
2. Cerboni C, Zingoni A, Cippitelli M, Piccoli M, Frati L, Santoni A. Antigen-activated human T lymphocytes express cell-surface NKG2D ligands via an ATM/ATR-dependent mechanism and become susceptible to autologous NK-cell lysis. Blood (2007) 110:606–15. doi:10.1182/blood-2006-10-052720
3. Nausch N, Cerwenka A. NKG2D ligands in tumor immunity. Oncogene (2008) 27:5944–58. doi:10.1038/onc.2008.272
4. Ashiru O, Boutet P, Fernandez-Messina L, Aguera-Gonzalez S, Skepper JN, Vales-Gomez M, et al. Natural killer cell cytotoxicity is suppressed by exposure to the human NKG2D ligand MICA*008 that is shed by tumor cells in exosomes. Cancer Res (2010) 70:481–9. doi:10.1158/0008-5472.CAN-09-1688
5. Steinle A, Li P, Morris DL, Groh V, Lanier LL, Strong RK, et al. Interactions of human NKG2D with its ligands MICA, MICB, and homologs of the mouse RAE-1 protein family. Immunogenetics (2001) 53:279–87. doi:10.1007/s002510100325
6. Isernhagen A, Malzahn D, Viktorova E, Elsner L, Monecke S, von Bonin F, et al. The MICA-129 dimorphism affects NKG2D signaling and outcome of hematopoietic stem cell transplantation. EMBO Mol Med (2015) 7:1480–502. doi:10.15252/emmm.201505246
7. Vallet S, Pecherstorfer M, Podar K. Adoptive cell therapy in multiple myeloma. Expert Opin Biol Ther (2017) 17:1511–22. doi:10.1080/14712598.2017.1375095
8. Fionda C, Soriani A, Zingoni A, Santoni A, Cippitelli M. NKG2D and DNAM-1 ligands: molecular targets for NK cell-mediated immunotherapeutic intervention in multiple myeloma. Biomed Res Int (2015) 2015:178698. doi:10.1155/2015/178698
9. Boussi L, Niesvizky R. Advances in immunotherapy in multiple myeloma. Curr Opin Oncol (2017) 29:460–6. doi:10.1097/CCO.0000000000000407
10. Pittari G, Vago L, Festuccia M, Bonini C, Mudawi D, Giaccone L, et al. Restoring natural killer cell immunity against multiple myeloma in the era of new drugs. Front Immunol (2017) 8:1444. doi:10.3389/fimmu.2017.01444
11. Carbone E, Neri P, Mesuraca M, Fulciniti MT, Otsuki T, Pende D, et al. HLA class I, NKG2D, and natural cytotoxicity receptors regulate multiple myeloma cell recognition by natural killer cells. Blood (2005) 105:251–8. doi:10.1182/blood-2004-04-1422
12. Soriani A, Zingoni A, Cerboni C, Iannitto ML, Ricciardi MR, Di Gialleonardo V, et al. ATM-ATR-dependent up-regulation of DNAM-1 and NKG2D ligands on multiple myeloma cells by therapeutic agents results in enhanced NK-cell susceptibility and is associated with a senescent phenotype. Blood (2009) 113:3503–11. doi:10.1182/blood-2008-08-173914
13. Holdenrieder S, Stieber P, Peterfi A, Nagel D, Steinle A, Salih HR. Soluble MICB in malignant diseases: analysis of diagnostic significance and correlation with soluble MICA. Cancer Immunol Immunother (2006) 55:1584–9. doi:10.1007/s00262-006-0167-1
14. Holdenrieder S, Stieber P, Peterfi A, Nagel D, Steinle A, Salih HR. Soluble MICA in malignant diseases. Int J Cancer (2006) 118:684–7. doi:10.1002/ijc.21382
15. Waldhauer I, Steinle A. Proteolytic release of soluble UL16-binding protein 2 from tumor cells. Cancer Res (2006) 66:2520–6. doi:10.1158/0008-5472.CAN-05-2520
16. Zingoni A, Cecere F, Vulpis E, Fionda C, Molfetta R, Soriani A, et al. Genotoxic stress induces senescence-associated ADAM10-dependent release of NKG2D MIC ligands in multiple myeloma cells. J Immunol (2015) 195(2):736–48. doi:10.4049/jimmunol.1402643
17. Zingoni A, Vulpis E, Nardone I, Soriani A, Fionda C, Cippitelli M, et al. Targeting NKG2D and NKp30 ligands shedding to improve NK cell-based immunotherapy. Crit Rev Immunol (2016) 36:445–60. doi:10.1615/CritRevImmunol.2017020166
18. McCann FE, Eissmann P, Onfelt B, Leung R, Davis DM. The activating NKG2D ligand MHC class I-related chain A transfers from target cells to NK cells in a manner that allows functional consequences. J Immunol (2007) 178:3418–26. doi:10.4049/jimmunol.178.6.3418
19. Rebmann V, Schutt P, Brandhorst D, Opalka B, Moritz T, Nowrousian MR, et al. Soluble MICA as an independent prognostic factor for the overall survival and progression-free survival of multiple myeloma patients. Clin Immunol (2007) 123:114–20. doi:10.1016/j.clim.2006.11.007
20. Jinushi M, Vanneman M, Munshi NC, Tai YT, Prabhala RH, Ritz J, et al. MHC class I chain-related protein A antibodies and shedding are associated with the progression of multiple myeloma. Proc Natl Acad Sci U S A (2008) 105:1285–90. doi:10.1073/pnas.0711293105
21. Isernhagen A, Schilling D, Monecke S, Shah P, Elsner L, Walter L, et al. The MICA-129Met/Val dimorphism affects plasma membrane expression and shedding of the NKG2D ligand MICA. Immunogenetics (2015) 68:109–23. doi:10.1007/s00251-015-0884-8
22. Chitadze G, Bhat J, Lettau M, Janssen O, Kabelitz D. Generation of soluble NKG2D ligands: proteolytic cleavage, exosome secretion and functional implications. Scand J Immunol (2013) 78:120–9. doi:10.1111/sji.12072
23. Fuerst D, Neuchel C, Niederwieser D, Bunjes D, Gramatzki M, Wagner E, et al. Matching for the MICA-129 polymorphism is beneficial in unrelated hematopoietic stem cell transplantation. Blood (2016) 128:3169–76. doi:10.1182/blood-2016-05-716357
24. Boukouaci W, Busson M, Peffault de Latour R, Rocha V, Suberbielle C, Bengoufa D, et al. MICA-129 genotype, soluble MICA, and anti-MICA antibodies as biomarkers of chronic graft-versus-host disease. Blood (2009) 114:5216–24. doi:10.1182/blood-2009-04-217430
25. Lopez-Hernandez R, Valdes M, Lucas D, Campillo JA, Martinez-Garcia P, Salama H, et al. Association analysis of MICA gene polymorphism and MICA-129 dimorphism with inflammatory bowel disease susceptibility in a Spanish population. Hum Immunol (2010) 71:512–4. doi:10.1016/j.humimm.2010.02.003
26. Li P, Willie ST, Bauer S, Morris DL, Spies T, Strong RK. Crystal structure of the MHC class I homolog MIC-A, a gammadelta T cell ligand. Immunity (1999) 10:577–84. doi:10.1016/S1074-7613(00)80057-6
27. Li P, Morris DL, Willcox BE, Steinle A, Spies T, Strong RK. Complex structure of the activating immunoreceptor NKG2D and its MHC class I-like ligand MICA. Nat Immunol (2001) 2:443–51. doi:10.1038/87757
28. Wensley BG, Kwa LG, Shammas SL, Rogers JM, Clarke J. Protein folding: adding a nucleus to guide helix docking reduces landscape roughness. J Mol Biol (2012) 423:273–83. doi:10.1016/j.jmb.2012.08.003
29. Muller S, Zocher G, Steinle A, Stehle T. Structure of the HCMV UL16-MICB complex elucidates select binding of a viral immunoevasin to diverse NKG2D ligands. PLoS Pathog (2010) 6:e1000723. doi:10.1371/journal.ppat.1000723
30. Holmes MA, Li P, Petersdorf EW, Strong RK. Structural studies of allelic diversity of the MHC class I homolog MIC-B, a stress-inducible ligand for the activating immunoreceptor NKG2D. J Immunol (2002) 169:1395–400. doi:10.4049/jimmunol.169.3.1395
31. Chen D, Gyllensten U. MICA polymorphism: biology and importance in cancer. Carcinogenesis (2014) 35:2633–42. doi:10.1093/carcin/bgu215
32. Isernhagen A, Malzahn D, Bickeboller H, Dressel R. Impact of the MICA-129Met/Val dimorphism on NKG2D-mediated biological functions and disease risks. Front Immunol (2016) 7:588. doi:10.3389/fimmu.2016.00588
33. Douik H, Ben Chaaben A, Attia Romdhane N, Romdhane HB, Mamoghli T, Fortier C, et al. Association of MICA-129 polymorphism with nasopharyngeal cancer risk in a Tunisian population. Hum Immunol (2009) 70:45–8. doi:10.1016/j.humimm.2008.10.008
34. Ouni N, Ben Chaaben A, Kablouti G, Lajnef M, Ayari F, Abaza H, et al. MICA-129Met/Val polymorphism is associated with early-onset breast cancer risk. Immunol Invest (2017) 46(6):603–14. doi:10.1080/08820139.2017.1336175
35. Tong HV, Toan NL, Song LH, Bock CT, Kremsner PG, Velavan TP. Hepatitis B virus-induced hepatocellular carcinoma: functional roles of MICA variants. J Viral Hepat (2013) 20:687–98. doi:10.1111/jvh.12089
36. Zhao J, Jiang Y, Lei Y, Zou K, Wang C, Huang S, et al. Functional MICA-129 polymorphism and serum levels of soluble MICA are correlated with ulcerative colitis in Chinese patients. J Gastroenterol Hepatol (2011) 26:593–8. doi:10.1111/j.1440-1746.2010.06524.x
37. Lo PH, Urabe Y, Kumar V, Tanikawa C, Koike K, Kato N, et al. Identification of a functional variant in the MICA promoter which regulates MICA expression and increases HCV-related hepatocellular carcinoma risk. PLoS One (2013) 8:e61279. doi:10.1371/journal.pone.0061279
38. Paschen A, Sucker A, Hill B, Moll I, Zapatka M, Nguyen XD, et al. Differential clinical significance of individual NKG2D ligands in melanoma: soluble ULBP2 as an indicator of poor prognosis superior to S100B. Clin Cancer Res (2009) 15:5208–15. doi:10.1158/1078-0432.CCR-09-0886
39. Groh V, Bruhl A, El-Gabalawy H, Nelson JL, Spies T. Stimulation of T cell autoreactivity by anomalous expression of NKG2D and its MIC ligands in rheumatoid arthritis. Proc Natl Acad Sci U S A (2003) 100:9452–7. doi:10.1073/pnas.1632807100
40. von Lilienfeld-Toal M, Frank S, Leyendecker C, Feyler S, Jarmin S, Morgan R, et al. Reduced immune effector cell NKG2D expression and increased levels of soluble NKG2D ligands in multiple myeloma may not be causally linked. Cancer Immunol Immunother (2010) 59:829–39. doi:10.1007/s00262-009-0807-3
41. Quatrini L, Molfetta R, Zitti B, Peruzzi G, Fionda C, Capuano C, et al. Ubiquitin-dependent endocytosis of NKG2D-DAP10 receptor complexes activates signaling and functions in human NK cells. Sci Signal (2015) 8:ra108. doi:10.1126/scisignal.aab2724
42. Molfetta R, Quatrini L, Zitti B, Capuano C, Galandrini R, Santoni A, et al. Regulation of NKG2D expression and signaling by endocytosis. Trends Immunol (2016) 37:790–802. doi:10.1016/j.it.2016.08.015
43. Bryceson YT, March ME, Ljunggren HG, Long EO. Synergy among receptors on resting NK cells for the activation of natural cytotoxicity and cytokine secretion. Blood (2006) 107:159–66. doi:10.1182/blood-2005-04-1351
44. Bryceson YT, March ME, Ljunggren HG, Long EO. Activation, coactivation, and costimulation of resting human natural killer cells. Immunol Rev (2006) 214:73–91. doi:10.1111/j.1600-065X.2006.00457.x
45. Furst D, Solgi G, Recker K, Mytilineos D, Schrezenmeier H, Mytilineos J. Sequence-based typing of major histocompatibility complex class I chain-related gene A alleles by use of exons 2-5 information. Tissue Antigens (2011) 77:201–5. doi:10.1111/j.1399-0039.2010.01601.x
46. Cerboni C, Ardolino M, Santoni A, Zingoni A. Detuning CD8+ T lymphocytes by down-regulation of the activating receptor NKG2D: role of NKG2D ligands released by activated T cells. Blood (2009) 113:2955–64. doi:10.1182/blood-2008-06-165944
47. Cox MC, Battella S, La Scaleia R, Pelliccia S, Di Napoli A, Porzia A, et al. Tumor-associated and immunochemotherapy-dependent long-term alterations of the peripheral blood NK cell compartment in DLBCL patients. Oncoimmunology (2015) 4:e990773. doi:10.4161/2162402X.2014.990773
Keywords: multiple myeloma, natural killer cells, NKG2D receptor, MICA polymorphism, predictive biomarker
Citation: Zingoni A, Vulpis E, Cecere F, Amendola MG, Fuerst D, Saribekyan T, Achour A, Sandalova T, Nardone I, Peri A, Soriani A, Fionda C, Mariggiò E, Petrucci MT, Ricciardi MR, Mytilineos J, Cippitelli M, Cerboni C and Santoni A (2018) MICA-129 Dimorphism and Soluble MICA Are Associated With the Progression of Multiple Myeloma. Front. Immunol. 9:926. doi: 10.3389/fimmu.2018.00926
Received: 12 December 2017; Accepted: 13 April 2018;
Published: 01 May 2018
Edited by:
Nicola Giuliani, Università degli Studi di Parma, ItalyReviewed by:
Antonio Pierini, University of Perugia, ItalyAlessandro Poggi, Ospedale Policlinico San Martino, Italy
Antonio Curti, Università degli Studi di Bologna, Italy
Copyright: © 2018 Zingoni, Vulpis, Cecere, Amendola, Fuerst, Saribekyan, Achour, Sandalova, Nardone, Peri, Soriani, Fionda, Mariggiò, Petrucci, Ricciardi, Mytilineos, Cippitelli, Cerboni and Santoni. This is an open-access article distributed under the terms of the Creative Commons Attribution License (CC BY). The use, distribution or reproduction in other forums is permitted, provided the original author(s) and the copyright owner are credited and that the original publication in this journal is cited, in accordance with accepted academic practice. No use, distribution or reproduction is permitted which does not comply with these terms.
*Correspondence: Alessandra Zingoni, alessandra.zingoni@uniroma1.it