Effects of drought and salt stress on seed germination of ephemeral plants in desert of northwest China
- 1State Key Laboratory of Desert and Oasis Ecology, Xinjiang Institute of Ecology and Geography, Chinese Academy of Sciences, Ürümqi, China
- 2University of Chinese Academy of Sciences, Beijing, China
- 3Yili Botanical Garden, Xinjiang Institute of Ecology and Geography, Xinjiang, China
- 4School of Geography and Tourism, Qufu Normal University, Rizhao, China
- 5College of Life Science, Shihezi University, Shihezi, China
Seed germination is the most sensitive stage of plant life history. Studying its response to drought and salinity can analysis the response and adaptation characteristics of desert plants to the environment. In this experiment, the seeds of four common desert ephemeral plants in Xinjiang (Ixiolirion tataricum, Nepeta micrantha, Lepidium apetalum, and Plantago minuta) were used as materials. To study the germination characteristics of seeds under drought, we used salt stress, and coupled salt-drought stress under treatments and explored the germination recovery ability of rehydrated seeds after salt and drought stress treatments. The results showed that: (1) Under salt stress, the germination ability of four plant seeds was inhibited in different degrees. Overall, the degree of inhibition was proportional to the concentration of NaCl solution. (2) Drought stress slowed the seed germination process, and the greater the degree of stress, the more pronounced the slowdown; PEG treatment showed no significant effect on the germination of the four seeds at low concentrations and significant inhibition at medium and high concentrations. (3) The coupled salt-drought treatment significantly alleviated the stress effect of one factor and improved the germination characteristics of seeds. (4) Seeds that did not germinate under different concentrations of salt stress, drought stress, and coupled stresses rapidly recover germination when the stress was relieved or lifted. Ephemeral plants are sensitive to environmental changes, and this study aims to provide a reference basis for vegetation restoration and ecological rehabilitation in arid and semi-arid areas.
Introduction
Plants are affected by various factors during growth and development, such as temperature, light, water, nutrients, etc. (Zhao et al., 2007; Jisha et al., 2013; Bulut and Gürkan, 2017; Gheidary et al., 2017). Among them, moisture and salinity are the main environmental factors affecting plant survival and growth, especially in arid and semi-arid areas (Dodd and Donovan, 1999; Ashrafa and Harris, 2004; Wang et al., 2009; Wu et al., 2011; Shen et al., 2015; Liu et al., 2021). Drought stress and salt stress usually inhibit plants’ normal life activities, leading them unable to grow, develop and reproduce normally. Under drought stress, a series of corresponding physiological and biochemical responses will occur in plants due to water deficit, which in turn cause dehydration of plant protoplasm and metabolic disorders, affecting normal plant growth (Sarker et al., 2005; Sircelj et al., 2005; Silva et al., 2009; Silva et al., 2013). However, under salt stress, in addition to osmotic stress and oxidative stress, plants are also subjected to ion toxicity, which disturbs the balance of nutrients in plants and causes poisoning or even death (Athar et al., 2008; Li et al., 2010; Betoni et al., 2011; Wu et al., 2013; Martins et al., 2019).
All stages of plant growth period are affected by drought stress and salt stress, among which seed germination is the most sensitive period to environmental stress during the whole growth and development of plants (Gutterman, 1993; Omami et al., 1999; Debez et al., 2004; Endo et al., 2014; Luan et al., 2014). Seed germination is related to vegetative growth and reproductive growth of plants in the later stage (Luan et al., 2014), while seed germination and seedling growth will directly affect the distribution and diversity of plants (Wulff, 1986; Souza and Fagundes, 2014). And in recent years, with global warming, the degree of harm to drought and salinity has gradually increased (Trenberth et al., 2014; Gad et al., 2021; Zhou et al., 2022). Therefore, it is of great theoretical and practical significance to study the germination characteristics of plant seeds under salt and drought stress to comprehensively consider the salt and drought resistance of plants and the distribution and diversity of plant populations, especially in arid and semi-arid ecosystems.
Ephemeral plants are an essential part of the desert ecosystem and play an important ecological function. They are a kind of plants that use early spring rainwater and melting snow water to rapidly complete the life cycle of growth, flowering, and fruiting in a short period in spring or early summer (Ueckermann and Van Rooyen, 2000; Huang et al., 2015; Chen et al., 2019b). They are mainly distributed in arid desert areas in northern Xinjiang, where the climate is dry, rainfall is scarce, evaporation is strong, and the soil has a high salt content (Qi et al., 2021). Living in harsh desert habitats for a long time, these plants have exercised and formed the unique skill to complete the entire life course in a short time quickly and the adaptability to drought, salinity, and other adversities. Whether plants can germinate and grow into seedlings in a salt and drought environment is the premise of their survival, growth, and reproduction (Taleisnik et al., 1998; Khan and Gulzar, 2003; Al-Taisan, 2010). The average growth and development of ephemeral desert plants and the successful completion of their life cycle are related to their unique seed germination mechanism (Gutterman, 1993; Wei et al., 2007; Baskin and Baskin, 2014; Lai et al., 2016). Therefore, it is essential to study the drought resistance and salt tolerance of ephemeral plants in this area during germination. The research will also provide vital primary data for revealing the response of these plants to climate change.
Ephemeral plants are an important plant group distributed in northern Xinjiang. This kind of plants not only have important ecological value in increasing the roughness of desert surface, preventing and fixing sand, but also can be used as important industrial raw materials, forage and medicinal plant resources (Chen et al., 2019b). Among them, Ixiolirion tataricum is an important group species and a relatively large early spring ephemeral plant in the desert vegetation of the same habitat. Lepidium apetalum is a common herb on saline-alkali land in arid desert area of northwest China, and it is also a valuable group species and medicinal plant in arid and semi-arid areas of China. Nepeta micrantha has the unique aromatic odor of the Labiatae plants, and is a unique aromatic plant resource with development and utilization potential in Xinjiang. Plantago minuta are auxiliary species of ephemeral plants in the area. Therefore, this paper uses different concentrations of NaCl and PEG solutions to simulate different degrees of salt stress, drought stress and coupled stress. The seed germination characteristics of four widely distributed and representative ephemeral plants in Gurbantunggut Desert were studied and analyzed, and the salt tolerance and drought resistance characteristics of these four ephemeral plant seeds were deeply understood. The purpose is to promote the research on the stress resistance of desert plant seeds in China, and to provide reference for the development and utilization of desert plant germplasm resources and desert vegetation restoration and ecological environment construction.
Materials and methods
Study site and study materials
Gurbantunggut Desert (44°11′−46°20′N, 84°31′−90°00′E), is located in the center of the Junggar Basin in Xinjiang and covers an area of about 48,800 square km2. It is the second-largest desert in China, and the largest fixed and semi-fixed desert in terms of area (Chen et al., 2019a). The area has a temperate arid desert climate, with average annual evaporation of more than 2,000 mm, while the average annual precipitation is generally less than 150 mm, and the desert hinterland is only 70–100 mm, mainly concentrated in spring and summer (Liu et al., 2020). The overall topography of the study area is high in the southeast and low in the northwest. The dunes in the east have a high elevation and are typical dunes. Small dunes and cracked lands alternate in the west, and there are scattered salinized soils. The northern part of the region is low and has many saline soils, which is the leading distribution area of saline plants. The southern and oasis areas are staggered. In recent years, the region has been affected by temperature rise and precipitation increase; the climate characteristics of “hotter and wetter” have increased soil salinity in some low-lying areas, forming a large area of saline soil in the basin (Du, 2021). The unique topography and environmental-climate variations have led to this area’s rapid development of ephemeral plant taxa.
The seeds of four desert plants, I. tataricum (Amaryllidaceae; perennial herb), N. micrantha (Lamiaceae; annual), L. apetalum (Brassicaceae; annual or biennial herb), and P. minuta (Plantaginaceae; annual or perennial herb), were collected from the Gurbantunggut Desert. The collected seeds were dried naturally at room temperature after removing impurities and empty deflated grains and then packed in envelopes (paper bag) and stored in a cool and dry place indoors for later use.
Experimental design
Concentration setting
Polyethylene glycol (PEG) is a kind of high molecular polymer, which has good hydrophilicity. Its addition to water can reduce the water potential of the solution, and cause drought stress to plants. PEG-6000 is a large molecule, which cannot pass through the plant cell wall into the cell, and non-toxic to cells (van der Weele et al., 2000). NaCl is the most abundant salt in salinized soil in arid and semi-arid area (Tobe et al., 2001). Therefore, NaCl and PEG-6000 were used to simulate salt and drought stress in this study.
Salt regime: four concentrations of NaCl solution such as 50, 100, 200, and 400 mmol/L were set to simulate the salt stress environment.
Drought regime: four concentrations of PEG solution such as 50, 100, 200, and 400 g/L were set to simulate the drought stress environment.
Coupled stress: Eight combinations of 50 mmol/L NaCl + 50 g/L PEG, 50 mmol/L NaCl + 100 g/LPEG, 50 mmol/L NaCl + 200 g/L PEG, 50 mmol/L NaCl + 400 g/LPEG, 100 mmol/L NaCl + 50 g/LPEG, 100 mmol/L NaCl + 100 g/L PEG, 100 mmol/L NaCl + 200 g/L PEG, 100 mmol/L NaCl + 400 g/L PEG were set up to simulate the coupled salt-drought stress environment.
Germination test
The Laboratory of Ecological Processes and Biological Adaptation in Arid Areas, Xinjiang Institute of Ecology and Geography, Chinese Academy of Sciences, tested the germination of seeds. This experiment made use of the Petri dish germination method. Choose seeds that are consistent in size and have a whole grain, then soak them in a solution of 0.1% potassium permanganate for 5 min and rinse them with distilled water for later use.
Add the prepared treatment solutions of each concentration into the Petri dish with two layers of filter paper (the amount added was such that the seeds did not flow with the solution when the Petri dishes were tilted). With 25 seeds in each dish, four replicates, with the addition of the appropriate amount of distilled water as a control. We placed the treated Petri dish in the incubator for germination. We used the results of the previous studies of our group to set the optimum temperature and light conditions for seed germination of each plant. Germination conditions are as follows: I.tataricum (Total Darkness, 15/5°C), N. micrantha (Day and night alternation, 30/15°C), L. apetalum (Day and night alternation, 30/15°C), P. minuta (Day and night alternation, 20/5°C) (Chen et al., 2019b). The experiment lasted for 6 weeks, with re-sprouting at week 6. Change the filter paper and solution every 3 days.
Index measurement
During the germination period, the seed germination state was observed and regularly recorded daily, and the germination standard was set when the radicle length reached 1/2 of the seed length. After the experiment, we calculated the germination percentage, the germination potential, the germination index, the cumulative germination percentage, and the re-germination percentage. The specific calculation method is as follows (Yang and Li, 2014):
(Gt is the number of germination in t time, Dt is the corresponding number of germination days).
Statistical analysis
Seed germination was measured using final germination percentage (FGP). The data were subjected to analysis of variance (ANOVA). Before conducting ANOVA, the data were tested for homogeneity of variance and then transformed with arcsine square root if necessary. FGP was analyzed as dependent variables, and salt and drought were fixed effects with a one-way ANOVA. The effects of different concentrations of NaCl and PEG on seed germination percentage, germination potential, and germination index of four ephemeral desert plants and their interaction effects were analyzed using one-way ANOVA. If the ANOVA result showed a significant difference, Duncan’s test was used to determine the differences among treatments. All statistical analyses were performed using SPSS 19.0 software, and figures were plotted using Origin 2019 software.
Results
Effects of salt stress on seed germination
Seed germination characteristics
Germination percentage and potential are essential indicators for assessing the seed germination ability and quality grade. The seeds treated with different concentrations of NaCl solution were compared with the control group. The germination percentage of I. tataricum and N. micrantha seeds decreased with the increase in NaCl concentration (Table 1 and Figures 1A,B). It shows that salt stress has a strong inhibitory effect on the germination of these two seeds. The germination of L. apetalum seeds was unaffected under the treatment of 50–200 mmol/L NaCl solution, and its germination percentage was not significantly different compared with the control (0 mmol/L) (P > 0.05; Table 1 and Figure 1C), which showed that it has good tolerance to salt stress. However, the germination percentage of P. minuta seeds first increased and then decreased with the increase of NaCl concentration (Table 1 and Figure 1D), indicating that slight salt stress promoted rather than inhibited the germination of P. minuta seeds. Under the highest salt stress treatment of 400 mmol/L NaCl solution, the seed germination of the four tested species was severely inhibited (Table 1 and Figures 1A–D).
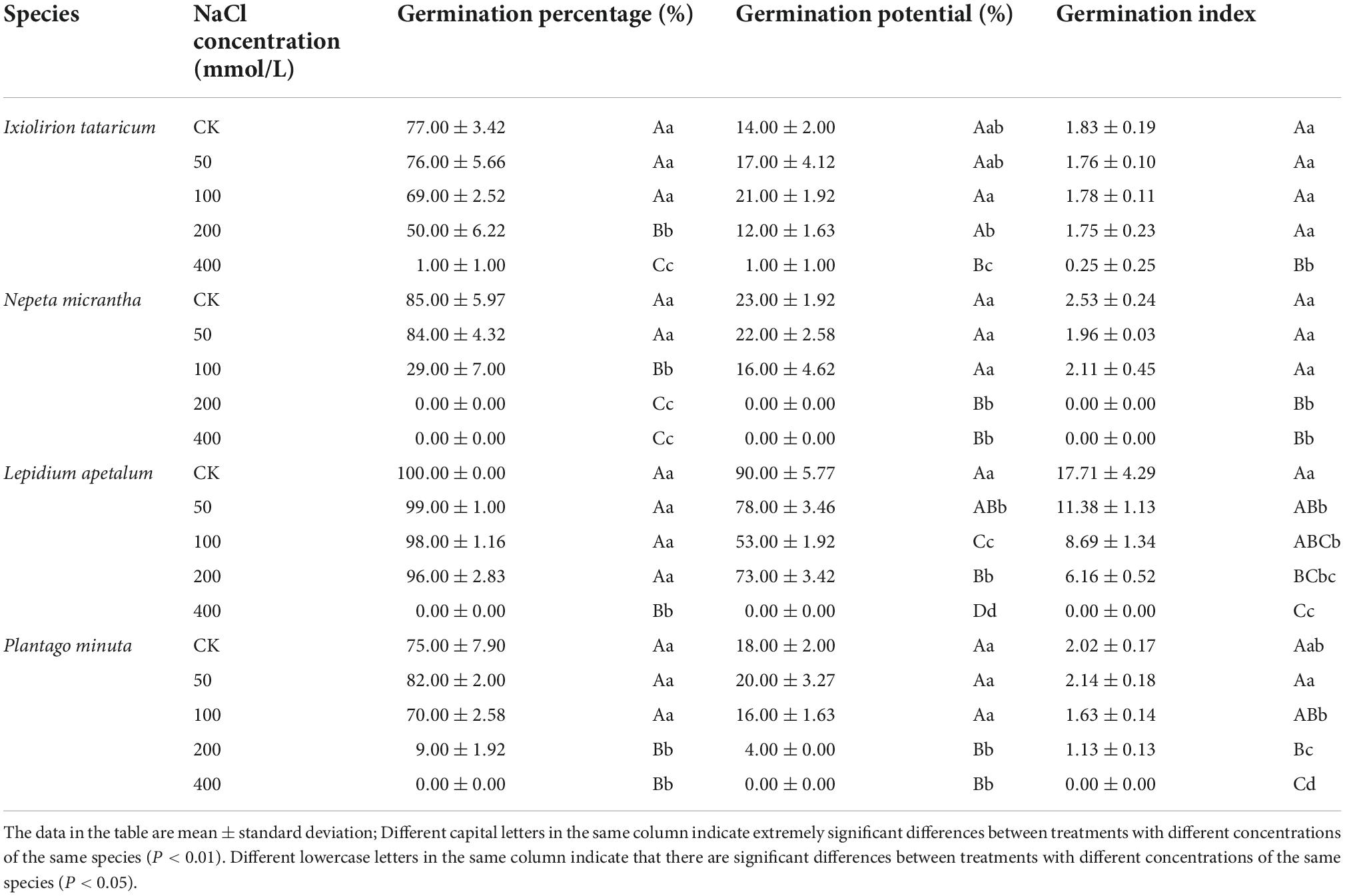
Table 1. Effects of different concentrations of NaCl on germination percentage, germination potential, and germination index.
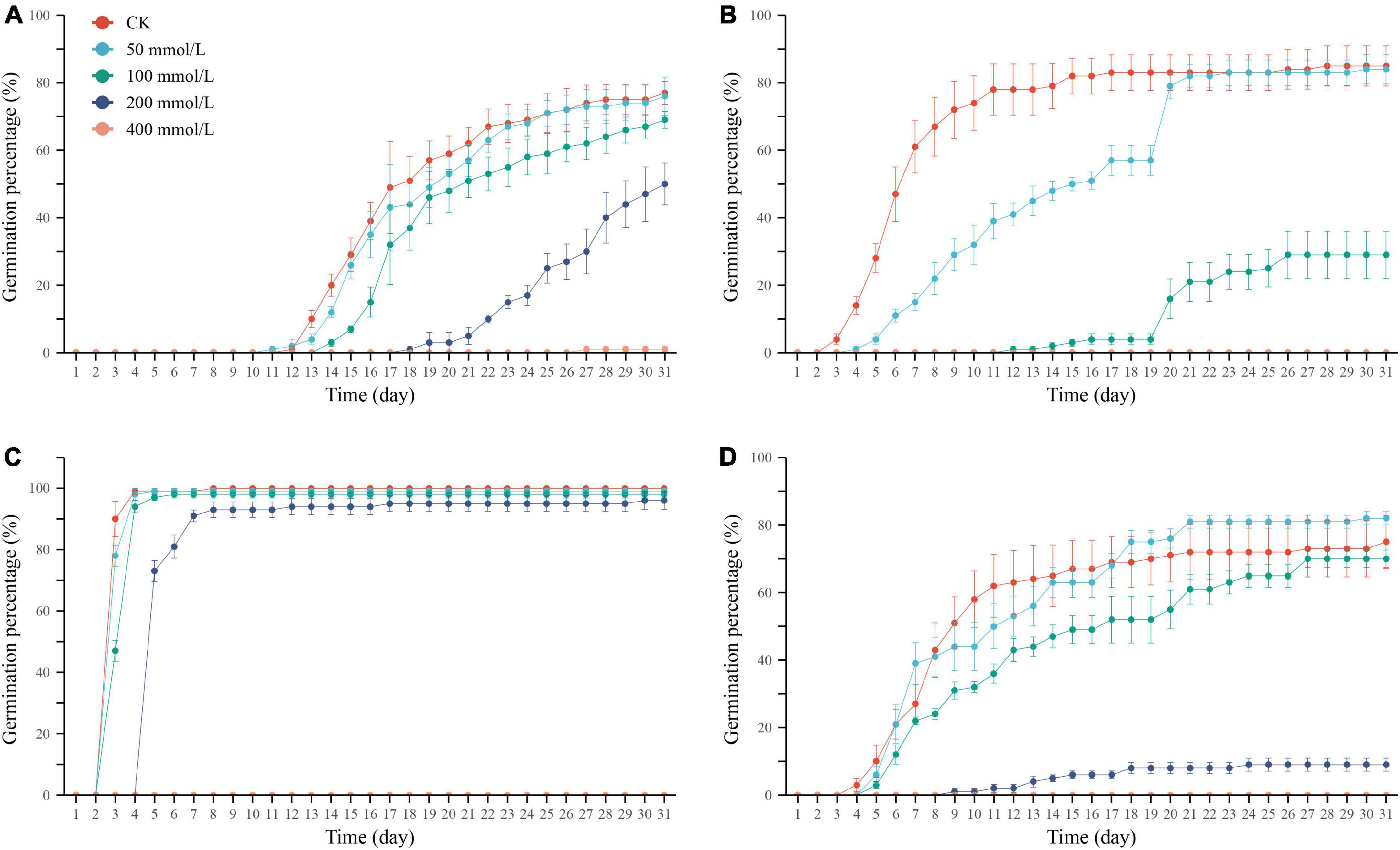
Figure 1. Cumulative germination percentage of Ixiolirion tataricum (A), Nepeta micrantha (B), Lepidium apetalum (C), and Plantago minuta (D) seeds at different concentrations of NaCl. Bars represent standard deviation.
The cumulative germination percentage of the four species in control and treated groups gradually increased with time and stabilized after reaching their respective peaks (Figures 1A–D). The days of initiation of germination of I. tataricum seeds under each concentration of NaCl treatment were days 12, 11, 14, 18, and 27, respectively (Figure 1A). The starting germination days of N. micrantha seeds were days 3, 4, and 12, respectively, and no seeds germinated under the treatment of 200 mmol/L and 400 mmol/L NaCl solutions (Figure 1B). The days of initial germination of L. apetalum seeds were days 3, 3, 3, and 5, respectively (Figure 1C). The days of germination of P. minuta seeds were days 4, 5, 5, and 9, respectively (Figure 1D). Both L. apetalum and P. minuta seeds did not germinate under 400 mmol/L NaCl solution treatment. It can be seen that the germination nodes of the seeds of the four desert plants were delayed with increasing NaCl concentration, and their germination process was delayed.
The germination potential of N. micrantha seeds showed a decreasing trend in line with its germination percentage, and the germination potential of seeds treated with low concentrations (50–100 mmol/L NaCl) was not significantly different from that of CK (P > 0.01; Table 1). The germination potential of I. tataricum and P. minuta seeds was not consistent with their germination percentage; that is, it increased first with the increase of NaCl solution concentration, and after reaching a peak value, it turned to decrease with the increase of solution concentration. However, the trend of L. apetalum was the opposite, and the difference between the treatments of concentration was highly significant (P < 0.01; Table 1). The germination potential of P. minuta seeds reached a maximum under the treatment of 50 mmol/L NaCl solution, and that of I. tataricum seeds reached a maximum under the treatment of 100 mmol/L NaCl solution (Table 1). This indicates that a relatively neat germination process could be maintained when subjected to slight salt stress.
The germination index is an important indicator to measure seed vigor. The effects of different concentrations of NaCl solution on the seed germination index of L. apetalum and P. minuta were highly significant (P < 0.01; Table 1). However, the effects of a low concentration of NaCl solution on the seed germination index of I. tataricum and N. micrantha was not significant (P > 0.01; Table 1). The germination index of I. tataricum and L. apetalum seeds showed a decreasing trend with the concentration of NaCl solution. The germination index of N. micrantha seeds first decreased, then increased, and finally reduced to zero (Table 1). The germination index of seeds of all three plants reached the maximum at a NaCl concentration of zero (CK). In contrast, the germination index of seeds of P. minuta increased, then decreased, and reached the maximum NaCl solution concentration of 50 mmol/L (Table 1).
In conclusion, NaCl salt stress has an inhibitory effect on the germination of four desert plant seeds, and the degree of inhibition was proportional to the concentration of NaCl solution. But different plants have different responses to salinity in the seed germination stage, among which the salt tolerance of L. apetalum was the strongest, I. tataricum and P. minuta, and N. micrantha was the worst.
Seed recovery germination percentage
After rehydrating the ungerminated seeds with different concentrations of NaCl solution for 6 days, the germination percentage of I. tataricum was 31.04, 45, 39.58, 51.07, and 81.79%, respectively (Figure 2). The germination percentage of N. micrantha was 100, 96.43, 97.55, 97, and 82%, respectively (Figure 2). The germination percentage of P. minuta was 93.75, 68.75, 81.40, 83.58, and 85%, respectively (Figure 2). And the ungerminated seeds of L. apetalum in 100–400 mmol/L NaCl solution, which all germinated after rehydration (Figure 2). The seeds treated with 400 mmol/L NaCl were almost unable to germinate, and the germination process was completely inhibited. However, when the salt stress was relieved, all four seeds could germinate usually. The germination percentage was above 80% (Figures 1, 2). The results showed that although the high concentrations of NaCl had a strong inhibitory effect on the germination of plant seeds, it did not cause the seeds to lose their germination ability permanently. The seeds treated with each concentration of NaCl still had a high recovery germination percentage after rehydration.
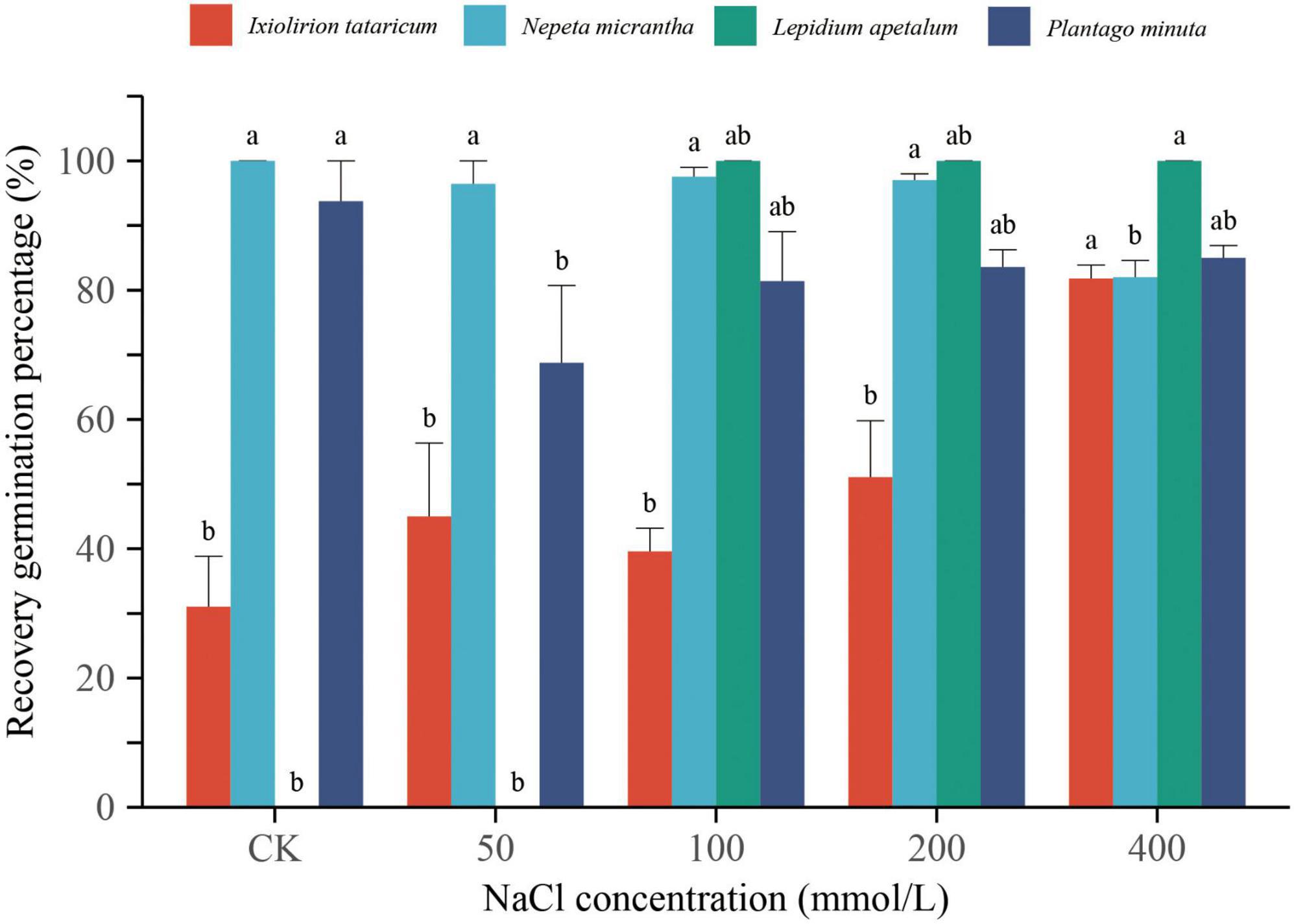
Figure 2. Effects of different concentrations of NaCl on the recovery germination percentage of Ixiolirion tataricum, Nepeta micrantha, Lepidium apetalum, and Plantago minuta seeds. Different lowercase letters in the figure indicated significant differences among different concentrations of the same species (P < 0.05).
Effects of drought stress on seed germination
Seed germination characteristics
Drought treatment with different concentrations of PEG had other effects on the germination percentage, germination potential, and germination index of the four desert plant seeds. The result of a low concentration (50–100 g/L) of PEG on seed germination was not significant, and its germination percentage was not significantly different from that of the control (0 g/L) (P > 0.05; Table 2 and Figure 3). However when the concentration of PEG-6000 solution was 200 g/L, the germination percentage of the four seeds decreased rapidly and was significantly different from the other concentrations treatments (P < 0.05), indicating that the seeds at this time were under severe drought stress but did not reach the threshold of ultimately limiting their germination. In contrast, when the concentration of PEG-6000 solution was 400 g/L, the seeds of N. micrantha, L. apetalum, and P. minuta didn’t germinate. Compared with salt stress, PEG solution also inhibited seed germination, but its germination percentage was still high, which indicated that the seeds of four desert plants had certain drought resistance.
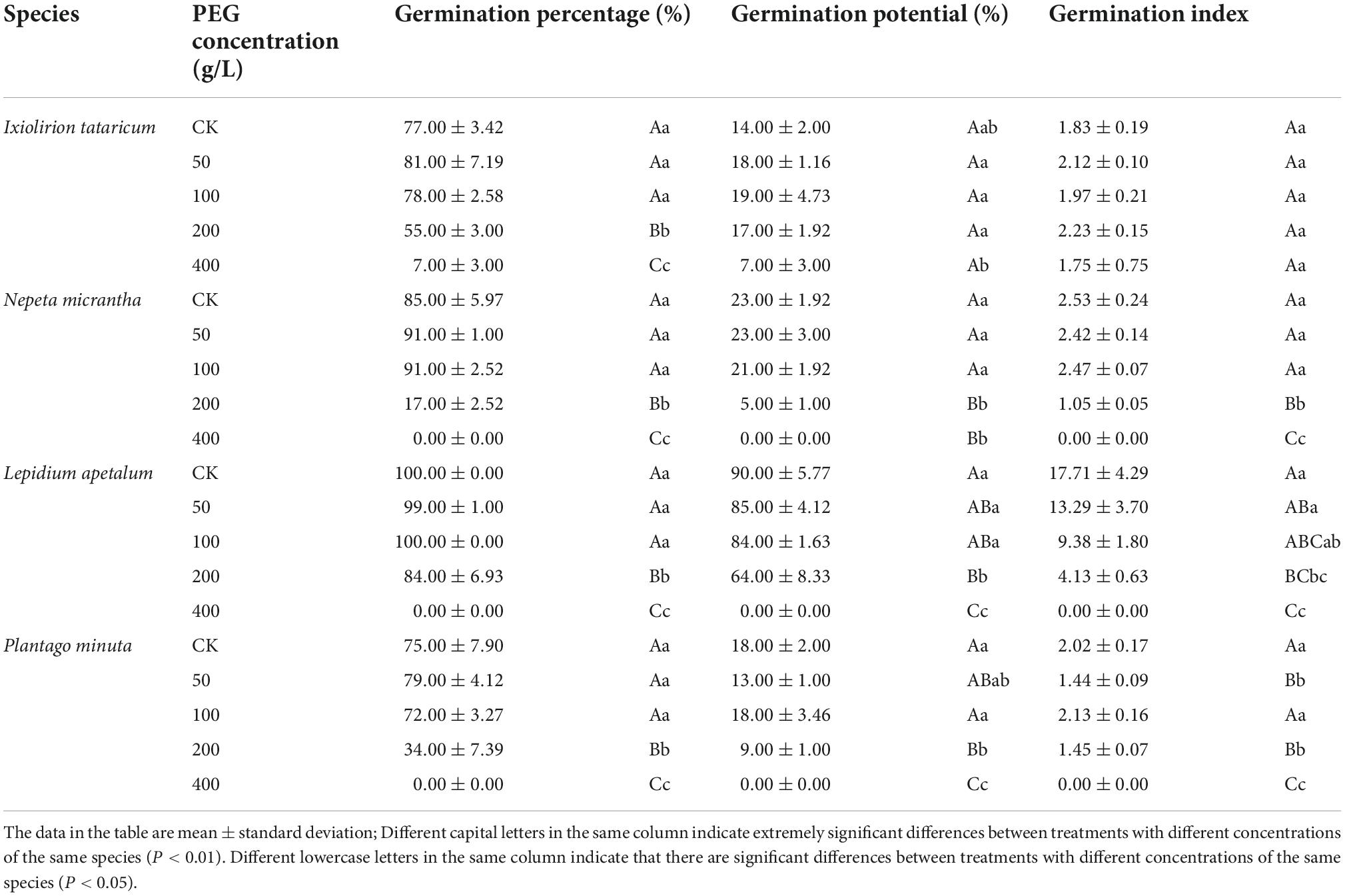
Table 2. Effects of different concentrations of PEG on germination percentage, germination potential, and germination index.
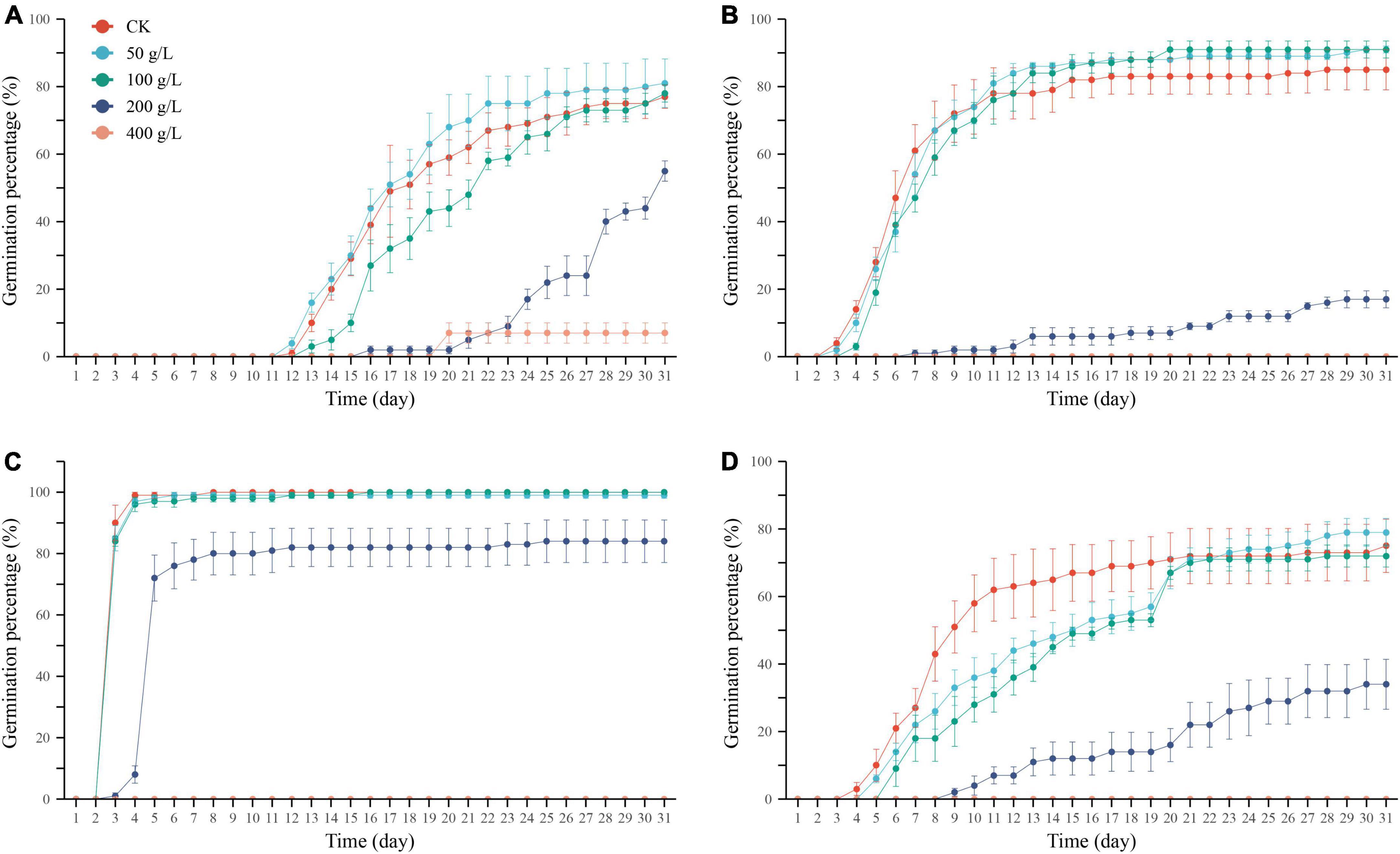
Figure 3. Cumulative germination percentage of Ixiolirion tataricum (A), Nepeta micrantha (B), Lepidium apetalum (C), and Plantago minuta (D) seeds at different concentrations of PEG. Bars represent standard deviation.
In the range of PEG simulated drought stress set in this experiment, the time to start germination of the four seeds was the same as the control or delayed by 1–2 days under the low concentration (50–100 g/L) PEG treatment, which was delayed under the medium concentration (200 g/L) PEG treatment, and was inhibited entirely under the high concentration (400 g/L) PEG treatment for all three seeds except for a few germinations of I. tataricum seeds, which could not germinate usually (Figures 3A–D). These results indicated that the seed germination process slowed down with the increase of drought stress, and the greater the degree of stress, the more pronounced the degree of slowing down. With the rise of germination days, the cumulative germination percentage of the four seeds increased gradually in both the control and treatment groups, and I. tataricum, N. micrantha, L. apetalum, and P. minuta germinated rapidly on the 12th, 3rd, 3rd, and 5th day, respectively (Figures 3A–D). With the increase in PEG concentration, the degree of simulated drought stress intensified, and the cumulative germination percentage of seeds showed a downward trend. The low concentration of PEG treatment had no significant effect on the germination process of N. micrantha and L. apetalum seeds. Still, the high PEG treatment concentration severely inhibited the four seeds’ germination process.
With the increase of PEG solution concentration, the germination potential of I. tataricum seeds showed a trend of increasing and then decreasing. Its germination potential was the highest under the treatment of 100 g/L PEG solution, which was 5% higher than that of the CK group (Table 2). The germination potential of the seeds of N. micrantha and L. apetalum decreased gradually with the concentration of PEG solution. There was no significant difference between the germination potential of the 50∼100 g/L PEG solution treatment and the control group (P > 0.05; Table 2). The germination potential of P. minuta seeds was not significantly different from the control group under the treatment of 50–100 g/L PEG solution (P > 0.05). Still, it was significantly lower than the control group under the therapy of 200–400 g/L PEG solution (P < 0.05). When the concentration of PEG was 400 g/L, the germination potential of the seeds of the other three plants was zero, except I. tataricum.
The seed germination index of I. tataricum treated with 50∼200 g/L PEG concentration was higher than that of the control, but there was no significant difference among each treatment (P > 0.05; Table 2). The germination index of seeds of P. minuta was higher than the control under 100 g/L PEG concentration treatment and significantly lower than the control under the remaining concentration treatments. The germination index of seeds of N. micrantha and L. apetalum was lower than the control at all concentrations of PEG solution. Among them, N. micrantha was not significantly different from the control at low concentrations (P > 0.05) but was significantly lower than the control at high concentrations (P < 0.05; Table 2). The germination index of L. apetalum seeds was significantly lower than that of the control at the other concentrations, except that the 50 g/L PEG treatment was higher than the control. As with germination percentage and potential, the germination index was zero for all three plant seeds except I. tataricum.
Different concentrations of drought treatments had specific effects on the seed germination of the four plants. The high concentrations of PEG (400 g/L) treatment have severely inhibited seed germination and affected seed germination ability, germination speed, and vigor level.
Seed recovery germination percentage
The seeds that did not germinate in the above PEG solution were washed and transferred to distilled water to continue germination. The results showed that the seeds of the four desert plants could resume germination quickly, and the recovery germination percentage was high (Figure 4). Among them, I. tataricum and N. micrantha had the highest recovery germination percentage under the treatment of 50 g/L PEG, both of which were 100%. L. apetalum had the highest recovery germination percentage under the treatment of 200 g/L PEG, which was 100%. The recovery germination percentage of P. minuta under each concentration treatment was above 80%, which was not significantly different from the control (P > 0.05; Figure 4). No seeds germinated under 400 g/L PEG treatment in N. micrantha, L. apetalum, and P. minuta, but the germination percentage was higher after rehydration. The results showed that the PEG solution did not make the plant seeds lose their vitality but only temporarily inhibited the seed germination. The seeds maintained a high germination potential, and when the drought stress was relieved, the seeds could quickly resume germination.
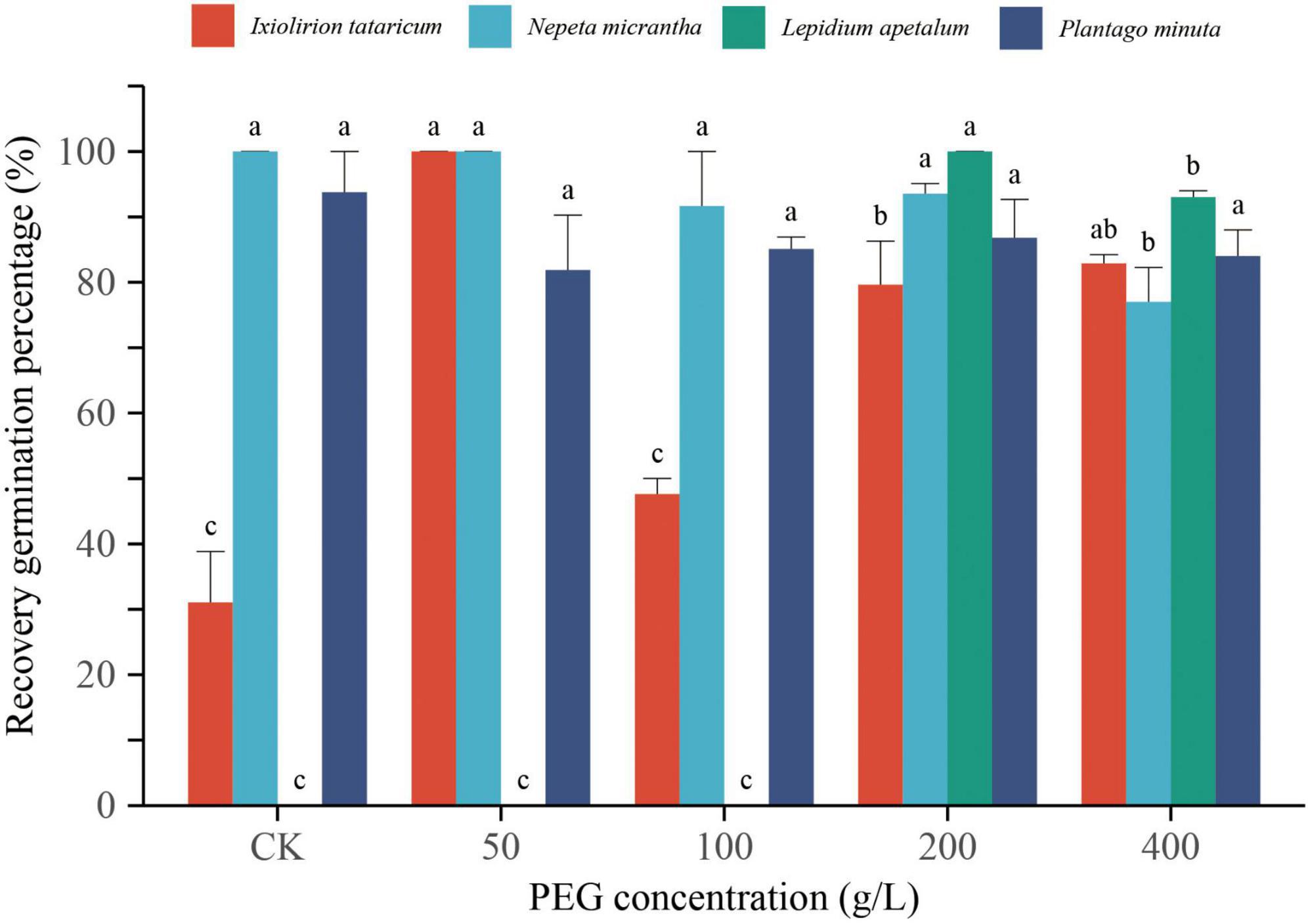
Figure 4. Effects of different concentrations of PEG on the recovery germination percentage of Ixiolirion tataricum, Nepeta micrantha, Lepidium apetalum, and Plantago minuta seeds. Different lowercase letters in the figure indicated significant differences among different concentrations of the same species (P < 0.05).
Effects of coupled salt-drought on seed germination
Seed germination characteristics
A certain concentration of coupled salt-drought can promote the germination percentage, germination potential, and germination index of four desert plant seeds (Table 3). When the concentration of NaCl was 50 mmol/L, there was no significant difference between the germination percentage of I. tataricum and L. apetalum seeds at a PEG concentration of 0∼200 g/L and the control (P > 0.05; Table 3). Among them, the germination percentage of I. tataricum seeds treated with 100 g/L PEG was slightly higher than that of the control, which was 6% higher; and the germination percentage of L. apetalum seeds treated with 50 g/L and 200 g/L PEG were consistent with CK, both 100%. The germination percentage of N. micrantha seeds at PEG concentrations of 100 g/L and 200 g/L was higher than that of the control, but the difference was insignificant (P > 0.05; Table 3); among them, the germination percentage of 100 g/L PEG was the highest. The germination percentage of P. minuta seeds at the PEG concentration of 0 g/L and 50 g/L was higher than that of the control, but the difference was insignificant (P > 0.05; Table 3). Among them, the germination percentage of seeds was the highest when the PEG concentration was 0 g/L; it was 7% higher than that of CK. When the NaCl concentration was 100 mmol/L, the germination percentage of four kinds of seeds at PEG concentrations of 0∼400 g/L was lower than that of the control, among which there was no significant difference between the germination percentage of I. tataricum and L. apetalum seeds at PEG concentration of 0∼200 g/L and the control (P > 0.05; Table 3). The germination percentage of N. micrantha and P. minuta seeds at PEG concentrations of 100∼400 g/L significantly differed from the control’s (P > 0.05; Table 3). In general, when the concentration of NaCl was 50 mmol/L, the germination percentage of the seeds of I. tataricum and N. micrantha increased first. They then decreased with the increase of soil water content. At the same time, the germination percentage of the seeds of L. apetalum and P. minuta increased gradually with the addition of soil water content. When the concentration of NaCl was 100 mmol/L, the germination percentage of the four kinds of seeds increased gradually with the addition of soil water.
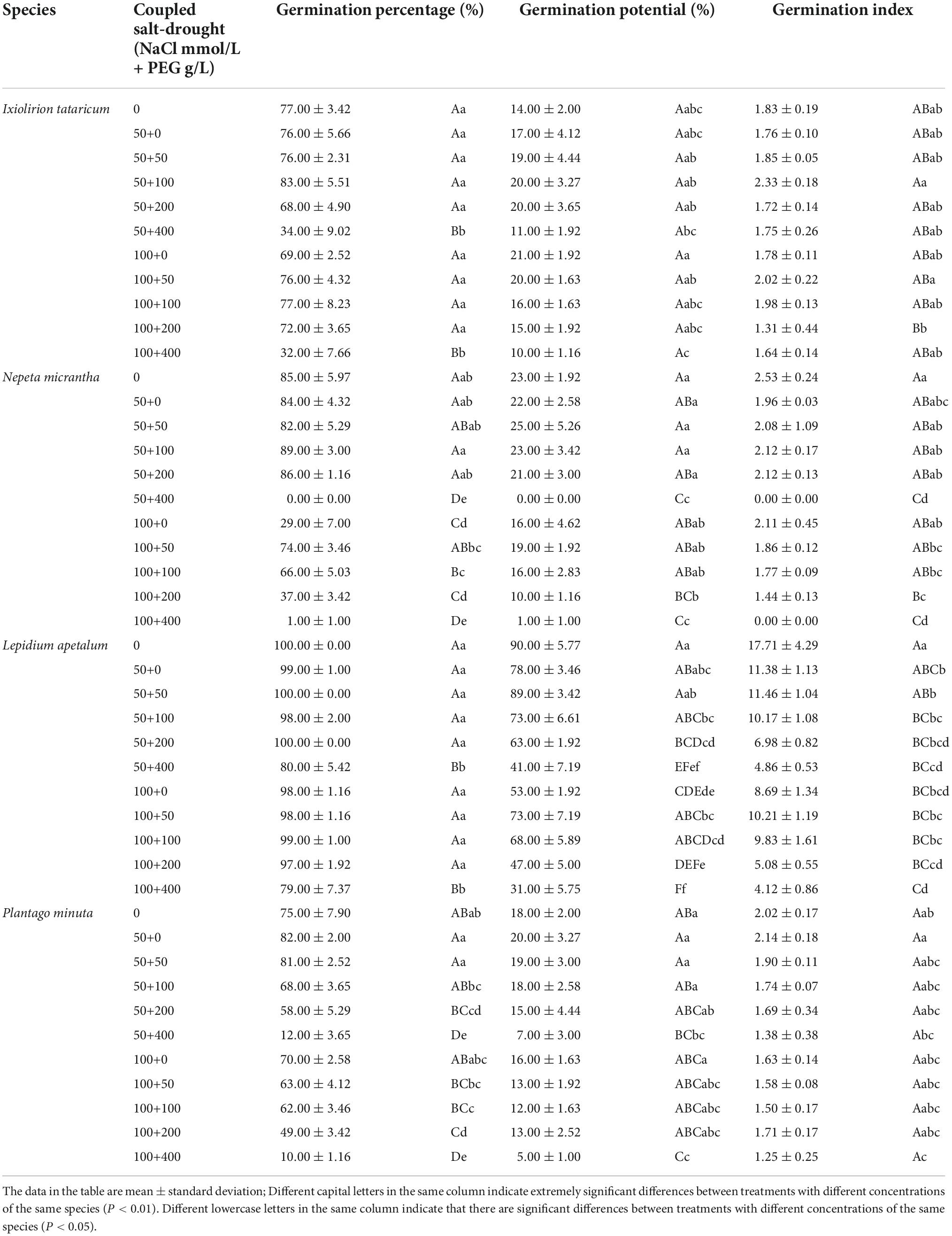
Table 3. Effects of coupled salt-drought on germination percentage, germination potential, and germination index.
The effects of different concentrations of coupled salt-drought stress on the seed germination process (Figure 5). The germination initiation time of L. apetalum seeds under each concentration treatment were similar. They all started to germinate in large quantities at the 3 days, indicating that L. apetalum seeds were not sensitive to NaCl and PEG, and germination was almost unaffected. When the NaCl concentration was certain, the germination initiation time of the seeds of the remaining three plants was delayed with increasing PEG concentration. The cumulative germination percentage of the four seeds increased gradually with time. The effects of different concentrations of coupled salt-drought stress on the seed germination were different. Among which, the cumulative germination percentage of I. tataricum and N. micrantha seeds were highest under the treatment of 50 mmol/L+100 g/L (Figures 5A,B); the cumulative germination percentage of P. minuta seeds was highest under the treatment of 50 mmol/L+0 g/L (Figure 5D), and the cumulative germination percentage of L. apetalum seeds was not significantly different under the remaining coupled except for 50 mmol/L + 400 g/L and 100 mmol/L + 400 g/L (Figure 5C). It can be seen that low concentration of coupled salt-drought can improve the germination percentage of plant seeds, and then improve the tolerance of plants to adversity.
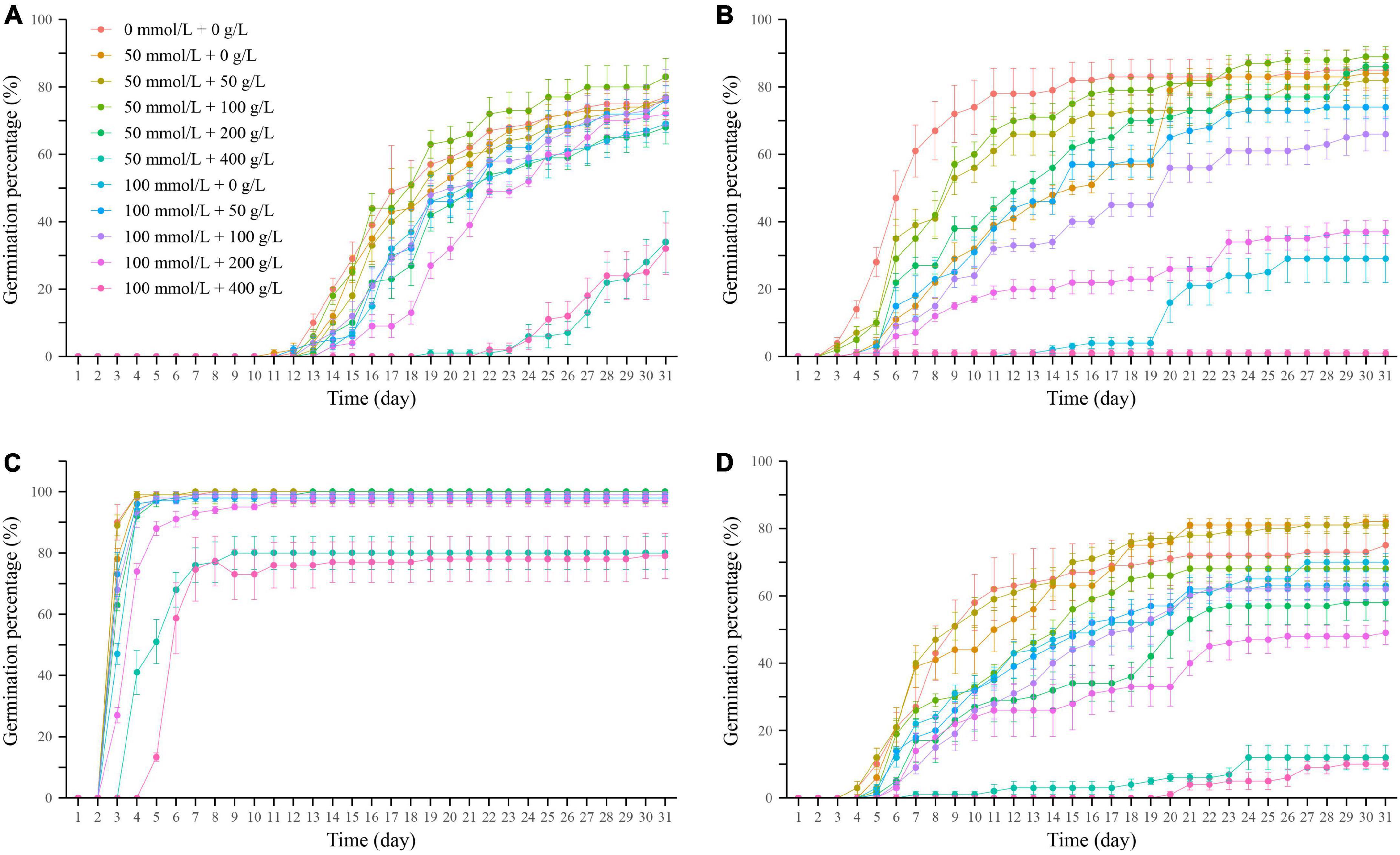
Figure 5. Cumulative germination percentage of Ixiolirion tataricum (A), Nepeta micrantha (B), Lepidium apetalum (C), and Plantago minuta (D) seeds under coupled salt-drought. Bars represent standard deviation.
Under the coupled salt-drought stress, the low concentration of salt-drought interaction could improve the germination characteristics of plant seeds. Under the specific NaCl treatment, the germination potential of N. micrantha and L. apetalum seeds increased first and then decreased with the increase of PEG concentration (Table 3). The germination potential of the P. minuta seeds gradually reduced with increasing PEG concentration (Table 3). The germination potential of I. tataricum seeds reached the maximum under the coupled stress of 100 mmol/L+0 g/L, which was 7% higher than that of the control. The germination potential of N. micrantha seeds was slightly higher than that of the control under the stress of 50 mmol/L+50 g/L, and the germination potential of other coupled conditions was lower than that of the control. The germination potential of L. apetalum seeds under each coupled treatment was significantly lower than that of the control. The germination potential of P. minuta seeds was higher than that of the control under the coupled of 50 mmol/L+0 g/L and 50 mmol/L+50 g/L, but the difference was not significant (P > 0.05; Table 3).
Different concentration coupled treatments have different effects on the germination index of plant seeds (Table 3). Low concentration of coupled salt-drought promoted the germination index of I. tataricum seeds; for example, the germination index of I. tataricum seeds under four coupled stresses of 50 mmol/L+50 g/L, 50 mmol/L+100 g/L, 100 mmol/L+50 g/L, and 100 mmol/L+100 g/L was higher than CK. The germination index of N. micrantha seeds under the coupled stress of 50 mmol/L+50 g/L, 50 mmol/L+100 g/L, 50 mmol/L+200 g/L and 100 mmol/L+0 g/L was no significant difference with CK (P > 0.05; Table 3). The germination index of L. apetalum seeds under all coupled stress treatments was significantly lower than the control (P < 0.05; Table 3). Under the coupled stress of 50 mmol/L+0 g/L, the germination index of P. minuta seeds was higher, and there was no significant difference with the control (P > 0.05; Table 3).
The above results indicate that a low concentration of PEG treatment can improve the tolerance of plant seeds to a salt environment under specific NaCl treatment. In contrast, a high PEG concentration produces the opposite effect and thus inhibits seed germination.
Seed recovery germination
Seeds that did not germinate under each coupled stress treatment could recover germination after the re-germination test in distilled water (Figure 6). I. tataricum had the highest recovery germination percentage of 72.25% under the coupled stress of 50 mmol/L + 400 g/L. Seeds of N. micrantha that did not germinate under coupled stress of 50 mmol/L + 200 g/L and 50 mmol/L + 400 g/L, as well as seeds of L. apetalum that did not germinate under coupled stress of 100 mmol/L + 0 g/L, 100 mmol/L + 50 g/L, 100 mmol/L + 200 g/L, and 100 mmol/L + 400 g/L all germinated after rehydration. The recovery germination percentage of P. minuta under the coupled stress of 100 mmol/L+100 g/L was as high as 97.73%. As with the results of single stress, the high concentration of salt and drought stress inhibited seed germination, but the seeds did not lose viability. They could continue to germinate until the germination percentage reached the level of the control group after the stress was relieved.
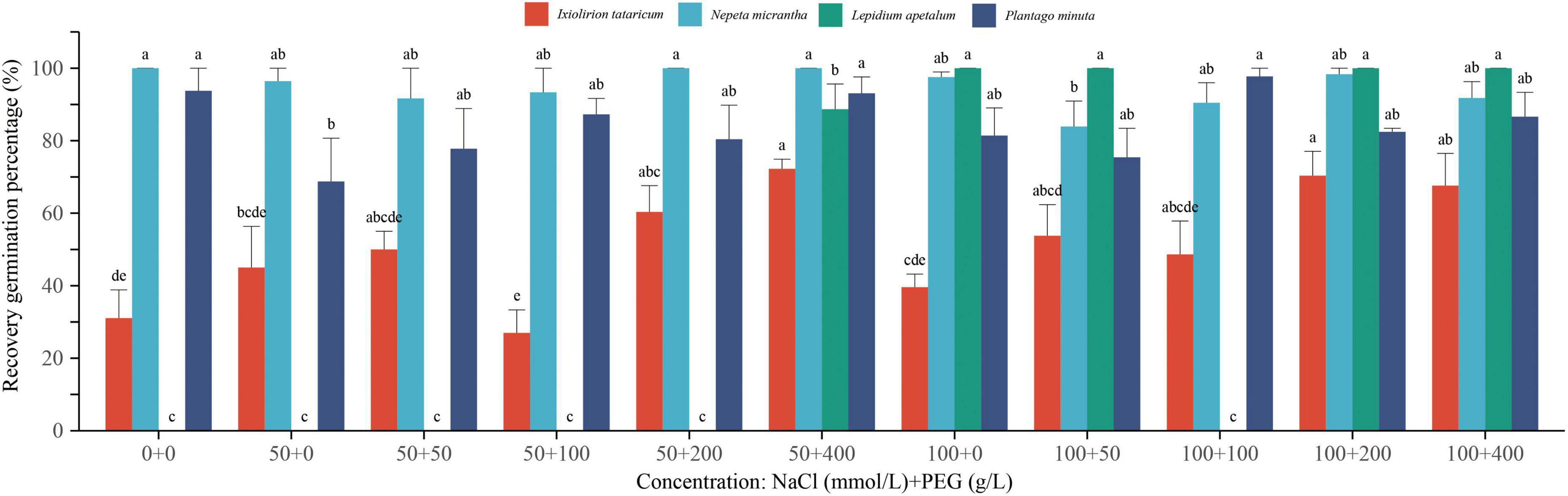
Figure 6. Effects of coupled salt-drought on the recovery germination percentage of Ixiolirion tataricum, Nepeta micrantha, Lepidium apetalum, and Plantago minuta seeds. Different lowercase letters in the figure indicated significant differences among different concentrations of the same species (P < 0.05).
Discussion
Effect of salt stress on seed germination
In the desert areas of northwest China, special climatic conditions such as dry climate, scarce rainfall, and intense evaporation have formed large areas of saline soils. Salt stress has also become an important limiting environmental factor affecting seed germination in this area. Salt stress affects plant seed germination, vegetation distribution range, and population pattern. Among them, salt stress has a certain degree of inhibition on the germination of plant seeds, such as reducing the germination percentage of seeds, prolonging the germination time of seeds, and causing the seeds to lose germination vigor (Khan and Gul, 2006; Baskin and Baskin, 2014; Santo et al., 2017). In this study, with increasing NaCl concentration, the germination percentage of seeds of I. tataricum and N. micrantha decreased until they did not germinate under a high concentration of the salt solution (400 mmol/L NaCl) (Table 1 and Figures 1A,B). The germination percentage of L. apetalum seeds was elementary unaffected under 50–200 mmol/L NaCl treatment (Table 1 and Figure 1C), indicating its strong salt tolerance under low and medium concentrations of salt stress conditions. The germination percentage of P. minuta under 50 mmol/L NaCl treatment was higher than that of the control. It showed a trend of first increasing and then decreasing with the increase of NaCl concentration, which indicated that the low concentration of salt stimulated the germination of P. minuta seeds. But the germination percentage would gradually decrease with the increase of salt concentration and inhibit seeds germination when the salt concentration was too high (Table 1 and Figure 1D). The results showed that slight salt stress had no significant effect on seed germination and even promoted seed germination. Severe salt stress inhibited seed germination, and the greater the stress, the more significant the inhibition. The reason may be that a low salt stress concentration improves cell membranes’ osmoregulation and traces Na+ stimulation, thereby promoting seed germination (Zhao et al., 2014; Qi et al., 2021). However, a high concentration of salt will produce osmotic stress on seeds, resulting in reduced extracellular water potential, affecting the water absorption and swelling of seeds, and preventing them from breaking through the seed coat, thus inhibiting the germination of seeds (Maas and Nieman, 1978; Jamil et al., 2006). At the end of the germination cycle, the seeds that did not germinate under each concentration of salt treatment were subjected to rehydration experiments. It was found that some of the seeds could resume germination. Among them, the recovery germination percentage of seeds under 400 mmol/L NaCl treatment was higher relative to that of control, both above 80% (Figure 2). The reason for this phenomenon may be that the salt concentration did not exceed the tolerance limit of plant seeds and did not cause permanent damage to the seeds, but only affected the water absorption capacity of the seeds making them unable to germinate generally due to insufficient water. Therefore, when the salt stress was reduced or disappeared, the seeds still had a solid recovery germination capacity (Ungar, 1995; Mamut et al., 2019).
The desert environment is extremely harsh to plant survival. For desert plants, salt is one of the leading environmental factors that affect their seed germination (Almansouri et al., 2001; Luan et al., 2014). In recent years, soil salinization has become increasingly severe due to global climate change (Dagar et al., 2016; Yan et al., 2020). Studies have shown that salt stress injures plants in two main aspects: osmotic pressure caused by excessive soil salt and ion poisoning caused by salt ion accumulation (Munns, 2002; Hakim et al., 2010; Luan et al., 2014). These injuries affect almost the whole life course of plants. For example, they are lowering the soil water potential, making it difficult for seeds to absorb water causing physiological drought, which eventually leads to seeds failing to sprout due to lack of water. As well as impairing cell membrane function, causing the imbalance of intracellular ion balance, affecting normal physiological metabolism, and eventually causing plant growth inhibition or even death, etc. (Li et al., 2006; Parihar et al., 2014; Wang et al., 2020). To avoid or alleviate the harm of salt stress, desert ephemeral plants have produced a series of growth mechanisms and germination strategies in response to adaptation to salt stress through long-term exercise and evolution in adversity. The concentrated distribution area of ephemeral plants in the Gurbantunggut Desert belongs to a temperate continental climate characterized by low precipitation and strong evaporation, high temperature and long sunshine, and frequent adversity stress. The seeds of many ephemeral plants in this area germinate in late spring and early summer when precipitation is concentrated. Sufficient water and low evaporation reduce the salt concentration of the soil solution, thus reducing or avoiding salt damage to the plants and enabling some seeds to germinate, usually under slight salt stress (Qu et al., 2008; Wang et al., 2013). However, after mid-May, the desert temperature rises sharply, the light is intense, and the evaporation increases. The hot summer months cause the water within the soil to move upward while driving deep salt enrichment in the surface layer of the ground. And the brief small amount of summer rainfall will cause the surface soil water to evaporate rapidly, thus causing a continuous increase in the surface soil salinity (Gutterman, 1993; Khan and Ungar, 1996; Almansouri et al., 2001; Xu et al., 2011). Higher soil surface salinity will induce seed dormancy (Khan and Ungar, 1997), allowing them to remain in the soil to survive adverse environmental conditions and wait for a suitable time to germinate. When sufficient rainfall occurs later, the soil surface salinity will be diluted and leached by rainwater, seed dormancy will be broken, and some seeds can germinate again (Khan and Sheikh, 1996). The seeds of the four desert ephemeral plants showed a high recovery germination percentage when salt stress was relieved or lifted, which reflected their adaptation to the harsh living environments to some extent. This germination strategy produced in response to salt adversity ensures this population’s existence and development to a certain extent.
Effects of drought stress on seed germination
Water is another key environmental factor affecting seed germination and seedling establishment (Lauenroth et al., 1994; Tobe et al., 2005), and plays an important role in determining vegetation distribution. However, the response of seed germination of desert ephemeral plants to water varies with drought degree and species. The results of this study showed that low concentration PEG-6000 solution (50–100 g/L) had no significant effect on seed germination of the four ephemeral plants, while medium and high concentration PEG-6000 solution (200–400 g/L) significantly inhibited the seed germination (Table 2 and Figure 3). When the concentration of PEG solution was 200 g/L, the germination percentage of seeds of all four plants was above 20%, indicating that this concentration had not yet reached the threshold of ultimately limiting their germination and that the seeds of the four plants were tolerant to drought stress. However, when the concentration of the PEG solution was as high as 400 g/L, the germination of the other three seeds except I. tataricum was completely inhibited. Still, with the lifting of the drought stress, the seeds would quickly recover germination, and the germination percentage was high. The seeds under all concentrations of stress had an excellent germination percentage. The results of rehydration experiments showed that PEG simulated drought stress only inhibited seed germination by inhibiting the water absorption process of seeds. Drought stress did not destroy the internal physiological structure and characteristics of seeds to inactivate them. So when the seeds are rehydrated, they can continue to germinate (Heshmat et al., 2011). This phenomenon may occur because the growth of ephemeral plants is susceptible to water changes. Under extreme conditions of high temperature and low rainfall, drought will affect the water absorption of seeds, so they cannot germinate and grow normally. To avoid species extinction, the seeds of ephemeral plants will be dormant. The seeds will show a high germination potential after the conditions improve and drought stress is relieved later (Si et al., 2020). This non-disposable germination mechanism is a response of ephemeral desert plants to their ability to adapt to environmental change.
Ephemeral plants are not a single functional adaptation to the natural environment of the dry and hot desert. Ephemeral plants make full use of favorable conditions such as desert winter and spring snowmelt and rain to quickly germinate and complete the whole life cycle in a short time to avoid harsh environmental conditions such as summer heat and drought. Therefore, most scholars think that they have no or little physiological structures of typical xerophytes and belong to the type of plants that escape drought and harsh habitats (Lan and Zhang, 2008). In an unpredictable desert environment, ephemeral plant seeds have formed many unique germination mechanisms to escape drought and other adversity stress, such as rapid germination, delayed germination (or dormancy), and asynchronous germination. In general, under the influence of a typical desert climate, ephemeral plants show the characteristics of rapid germination in early spring. Still, under conditions such as snow cover in winter and abundant precipitation in summer and autumn, some ephemeral plants will rapidly adapt to this environmental change and show the phenomenon of germination in spring, summer, and autumn (Yao and Tan, 2005). Germination of seeds scattered in different seasons can make full use of environmental resources, reduce the competition among sibling seeds and facilitate the expansion and continuation of the population (Yao and Tan, 2005; Leverett, 2017). For the harsh and austere desert environment, this unique germination strategy is the basis to ensure the sustainable survival and reproduction of the population in the specific environment.
Effects of coupled salt-drought on seed germination
Salinity and drought stresses occur simultaneously in deserts of arid and semi-arid areas (Shen et al., 2014). In this study, by observing the seed germination experiments of I. tataricum, N. micrantha, L. apetalum, and P. minuta. under coupled salt-drought stress; we found that the low concentration of coupled salt-drought treatment could improve the germination characteristics of plant seeds and promote the germination percentage, germination potential, and germination index of the seeds of four desert plants (Table 3). When salt is the main factor, PEG simulated drought stress to a certain extent can alleviate the inhibition of salt ions on seed germination. It may be due to the adsorption of salt ions by the PEG macromolecules, thus alleviating the ion toxicity of medium and high salt concentrations in seeds (Bajji et al., 1998). When water is the main factor, a low concentration of NaCl can alleviate the stress effect of medium and high concentration of PEG on seed germination. The stress caused by PEG can be helped by NaCl, implying that drought stress only reduces the enzyme activity in seeds but does not destroy the seed interior (Heshmat et al., 2011). It shows that the coupled salt-drought treatment can significantly alleviate the stress effect of a particular factor. The results imply that I. tataricum, N. micrantha, L. apetalum, and P. minuta seeds may respond differently to osmotic stress caused by NaCl and PEG. They cannot only mitigate the damage caused by single stress through coupled salt-drought but also improve their adaptation to the external environment through interaction, showing cross-adaptation of plants, thus improving their tolerance. In this study, the seeds of the four plants have a solid ability to recover germination after the stress was relieved or lifted, which helped the seeds to adapt to the complex and variable desert environment during the germination period.
In summary, the seeds of these four ephemeral plants have a good ecological adaptation mechanism to the arid saline-alkali environment, and the germination of seeds can tolerate certain drought stress, but salt stress and drought stress generally still inhibit seed germination. Under mild adversity stress, the seeds adopt the strategy of rapid germination, and the seeds use sufficient water to germinate rapidly in a short time. When the adversity stress is strengthened, the seeds will enter forced dormancy and keep a temporary or lasting seed bank in the soil. When the late conditions are suitable, the bet-hedging strategy will be adopted, and the seeds will germinate in batches, so as to avoid the risk of population extinction due to great changes in the external environment, thus making the seeds have greater adaptability to harsh desert environment. The germination strategies of the seeds of four ephemeral plants at different salinity and drought levels reflect an adaptive characteristic of ephemeral plants to their harsh habitats during seed germination. These germination strategies are adapted to the environment that make the populations of these four ephemeral plants widely distributed in the saline desert environment of Xinjiang. Desert plants play an important role in maintaining vulnerable ecosystems in arid and semi-arid desert areas. In recent years, with climate change and human activities, drought has intensified and vegetation has degraded in desert areas. Ephemeral plants have inestimable ecological value for the establishment of plant communities in deserts, the protection of biodiversity, and windbreak and sand fixation. Therefore, the adaptability to the environment and germination strategies of these four widely distributed and representative ephemeral plants in the Gurbantunggut Desert in this study will provide a theoretical basis for the development and utilization of ephemeral plant germplasm resources in the vast arid and saline areas of Xinjiang and the increase of vegetation coverage in the desert system, and play a guiding role in the restoration and reconstruction of damaged ecosystems.
Conclusion
In this paper, the germination characteristics of four ephemeral plants in the Gurbantunggut Desert of Xinjiang under drought, salt stress, and coupled salt-drought stress were investigated, and the following conclusions were obtained:
(1) Salt stress and drought stress inhibited the germination process of the four seeds, and the inhibitory effect became more obvious as the degree of stress increased. However, the stress did not cause permanent damage to the seeds. Still, it only temporarily inhibited the water uptake process of the seeds so that they could not usually germinate due to insufficient water. The seeds could continue to sprout when the stress was reduced or disappeared.
(2) The coupled salt-drought treatment can significantly alleviate the stress effect of a single factor and improve the germination characteristics of the seeds. Among them, a low concentration of PEG treatment can enhance the tolerance of plant seeds to the salt environment. At the same time, a low concentration of NaCl can also alleviate the stress effect of medium and high concentrations of PEG on seed germination.
(3) The germination time of ephemeral desert plant seeds has strong plasticity. Unique seed germination mechanisms such as rapid and asynchrony germination have evolved in long-term adaptation to the harsh desert environment.
Data availability statement
The raw data supporting the conclusions of this article will be made available by the authors, without undue reservation.
Author contributions
YL, HL, YC, and LZ conceived and designed the study and wrote the manuscript. YL, LZ, KK, and JS formed most of the experiments. YL, LZ, and KK analyzed the data. All authors contributed to the article and approved the submitted version.
Funding
This research was supported by the National Natural Science Foundation of China (32171513, 32160526, 31971428, and U2003214), the Youth Innovation Promotion Association of the Chinese Academy of Sciences (2018477), and the High-level Talents Training Program of Xinjiang Institute of Ecology and Geography.
Acknowledgments
We are grateful to the research group members for their support and contribution to seed collection. We thank Huang Yunjie for his guidance and suggestions on chart making.
Conflict of interest
The authors declare that the research was conducted in the absence of any commercial or financial relationships that could be construed as a potential conflict of interest.
Publisher’s note
All claims expressed in this article are solely those of the authors and do not necessarily represent those of their affiliated organizations, or those of the publisher, the editors and the reviewers. Any product that may be evaluated in this article, or claim that may be made by its manufacturer, is not guaranteed or endorsed by the publisher.
References
Almansouri, M., Kinet, J. M., and Lutts, S. (2001). Effect of salt and osmotic stresses on germination in durum wheat (Triticum durum Desf.). Plant Soil 231, 243–254. doi: 10.1023/a:1010378409663
Al-Taisan, W. A. (2010). Comparative effects of drought and salt stress on germination and seedling growth of Pennisetum divisum (Gmel.) Henr. Am. J. Appl. Sci. 7, 640–646. doi: 10.3844/ajassp.2010.640.646
Ashrafa, M., and Harris, P. J. C. (2004). Potential biochemical indicators of salinity tolerance in plants. Plant Sci. 166, 3–16.
Athar, H. U. R., Khan, A., and Ashraf, M. (2008). Exogenously applied ascorbic acid alleviates salt-induced oxidative stress in wheat. Environ. Exp. Bot. 63, 224–231.
Bajji, M., Kinet, J. M., and Lutts, S. (1998). Salt stress effects on roots and leaves of Atriplex halimus L. and their corresponding callus cultures. Plant Sci. 137, 131–142. doi: 10.1016/S0168-9452(98)00116-2
Baskin, C. C., and Baskin, J. M. (2014). Seeds: Ecology, Biogeography, and Evolution of Dormancy and Germination, 2nd Edn. San Diego: Academic Press.
Betoni, R., Scalon, S. D. Q., and Mussury, R. M. (2011). Salinity and temperature in the germination and vigor of Guazuma ulmifolia LAM. (Sterculiaceae) seeds. Rev. Arvore. 35, 605–616. doi: 10.1590/s0100-67622011000400004
Bulut, H., and Gürkan, H. (2017). “Drought stress duo to climate change and effects on plants,” in Proceedings of the 8th Atmospheric Sciences Symposium, (Istanbul-Turkey), 123–130.
Chen, Y. F., Cao, Q. M., Li, D. X., Liu, H. L., and Zhang, D. Y. (2019b). Effects of temperature and light on seed germination of ephemeral plants in the Gurbantunggut Desert, China: implications for vegetation restoration. J. Arid Land 11, 916–927. doi: 10.1007/s40333-019-0027-4
Chen, Y. F., Shi, X., Zhang, L. W., Baskin, J. M., Baskin, C. C., Liu, H. L., et al. (2019a). Effects of increased precipitation on the life history of spring- and autumn-germinated plants of the cold desert annual Erodium oxyrhynchum (Geraniaceae). Aob Plants 11:lz004. doi: 10.1093/aobpla/plz004
Dagar, J. C., Sharma, P. C., Chaudhari, S. K., Jat, H. S., and Ahamad, S. (2016). “Climate change vis-a-vis saline agriculture: impact and adaptation strategies,” in Innovative Saline Agriculture, eds J. Dagar, P. Sharma, D. Sharma, and A. Singh (New Delhi: Springer), 5–53.
Debez, A., Ben Hamed, K., Grignon, C., and Abdelly, C. (2004). Salinity effects on germination, growth, and seed production of the halophyte Cakile maritima. Plant Soil 262, 179–189.
Dodd, G. L., and Donovan, L. A. (1999). Water potential and ionic effects on germination and seedling growth of two cold desert shrubs. Am. J. Bot. 86, 1146–1153. doi: 10.2307/2656978
Du, B. C. X. (2021). Vegetation Change Trend and its Response to Key Ecological Factors in Junggar Basin. Dissertation/master’s thesis. Shihezi: Shihezi University.
Endo, A., Nelson, K. M., Thoms, K., Abrams, S. R., Nambara, E., and Sato, Y. (2014). Functional characterization of xanthoxin dehydrogenase in rice. J. Plant Physiol. 171, 1231–1240.
Gad, M., Chao, H. B., Li, H. X., Zhao, W. G., Lu, G. Y., and Li, M. T. (2021). QTL mapping for seed germination response to drought stress in Brassica napus. Front. Plant Sci. 11:629970. doi: 10.3389/fpls.2020.629970
Gheidary, S., Akhzari, D., and Pessarakli, M. (2017). Effects of salinity, drought, and priming treatments on seed germination and growth parameters of Lathyrus sativa L. J. Plant Nutr. 40, 1507–1514. doi: 10.1080/01904167.2016.1269349
Hakim, M. A., Juraimi, A. S., Begum, M., Hanafi, M. M., Ismail, M. R., and Selamat, A. (2010). Effect of salt stress on germination and early seedling growth of rice (Oryza sativa L.). Afr. J. Biotechnol. 9, 1911–1918. doi: 10.5897/ajb09.1526
Heshmat, O., Saeed, H. A., and Fardin, K. (2011). The improvememt of seed germination traits in canola (Bassica napus L.) as affected by saline and drought stress. Int. J. Agric. Technol. 7, 611–622.
Huang, G., Li, Y., and Padilla, F. M. (2015). Ephemeral plants mediate responses of ecosystem carbon exchange to increased precipitation in a temperate desert. Agric. Forest. Meteorol. 201, 141–152. doi: 10.1016/j.agrformet.2014.11.011
Jamil, M., Deog Bae, L., Kwang Yong, J., Ashraf, M., Sheong Chun, L., and Shik Rha, E. (2006). Effect of salt (NaCl) stress on germination and early seedling growth of four vegetables species. J. Cent. Eur. Agric. 7, 273–282. doi: 10.5513/jcea.v7i2.370
Jisha, K. C., Vijayakumari, K., and Puthur, J. T. (2013). Seed priming for abiotic stress tolerance: an overview. Acta Physiol. Plant 35, 1381–1396. doi: 10.1007/s11738-012-1186-5
Khan, M. A., and Gul, B. (2006). “Halophyte seed germination,” in Ecophysiology of High Salinity Tolerant Plants, eds M. A. Khan and D. J. Weber (Dordrecht: Springer), 11–30.
Khan, M. A., and Gulzar, S. (2003). Germination responses of Sporobolus ioclados: a saline desert grass. J. Arid. Environ. 53, 387–394. doi: 10.1006/jare.2002.1045
Khan, M. A., and Ungar, I. A. (1996). Influence of salinity and temperature on the germination of Haloxylon recurvum bunge ex. Boiss. Ann. Bot. 78, 547–551. doi: 10.1006/anbo.1996.0159
Khan, M. A., and Ungar, I. A. (1997). Effects of thermoperiod on recovery of seed germination of halophytes from saline conditions. Am. J. Bot. 84, 279–293. doi: 10.2307/2446089
Khan, S. S., and Sheikh, K. H. (1996). Effects of different levels of salinity on seed germination and growth of Capsicum annuum L. Biologia 22, 15–16.
Lai, L. M., Chen, L. J., Jiang, L. H., Zhou, J. H., Zheng, Y. R., and Shimizu, H. (2016). Seed germination of seven desert plants and implications for vegetation restoration. Aob Plants 8:lw031. doi: 10.1093/aobpla/plw031
Lan, H. Y., and Zhang, F. C. (2008). Reviews on special mechanisms of adaptability of early-spring ephemeral plants to desert habitats in Xinjiang. Acta Bot. Boreal. Occident. Sin. 28, 1478–1485.
Lauenroth, W. K., Sala, O. E., Coffin, D. P., and Kirchner, T. B. (1994). The importance of soil water in the recruitment of bouteloua gracilis in the shortgrass steppe. Ecol. Appl. 4, 741–749. doi: 10.2307/1942004
Leverett, L. D. (2017). Germination phenology determines the propensity for facilitation and competition. Ecology 98, 2437–2446. doi: 10.1002/ecy.1933
Li, D. L., Wang, J. H., Li, A. D., Liu, H. J., and An, F. G. (2006). Salt tolerance of 3 Ceratoides pps varieties in germination period. J. Desert Res. 26, 1009–1014.
Li, R. L., Shi, F. C., Fukuda, K., and Yang, Y. L. (2010). Effects of salt and alkali stresses on germination, growth, photosynthesis and ion accumulation in alfalfa (Medicago sativa L.). Soil Sci. Plant Nutr. 56, 725–733. doi: 10.1111/j.1747-0765.2010.00506.x
Liu, B. H., Liu, X. H., Liu, F. C., Ma, H. L., Ma, B. Y., and Peng, L. (2021). Stress tolerance of Xerocomus badius and its promotion effect on seed germination and seedling growth of annual ryegrass under salt and drought stresses. AMB Expr. 11, 15–23. doi: 10.1186/s13568-020-01172-7
Liu, J. G., Liu, W. G., Long, X. E., Chen, Y. G., Huang, T. W., Huo, J. S., et al. (2020). Effects of nitrogen addition on C: N: P stoichiometry in moss crust-soil continuum in the N-limited Gurbantünggüt Desert, Northwest China. Eur. J. Soil Biol. 98:103174. doi: 10.1016/j.ejsobi.2020.103174
Luan, Z. H., Xiao, M. X., Zhou, D. W., Zhang, H. X., Tian, Y., Wu, Y., et al. (2014). Effects of salinity, temperature, and polyethylene glycol on the seed germination of sunflower (Helianthus annuus L.). Sci. World J. 2014:170418. doi: 10.1155/2014/170418
Maas, E. V., and Nieman, R. H. (1978). “Physiology of plant tolerance to salinity,” in Crop Tolerance to Suboptimal Land Conditions, ed. G. A. Jung (Madison Wisconsin: American Society of Agronomy), 277–300.
Mamut, J., Tan, D. Y., Baskin, C. C., and Baskin, J. M. (2019). Effects of water stress and NaCl stress on different life cycle stages of the cold desert annual Lachnoloma lehmannii in China. J. Arid Land 11, 774–784. doi: 10.1007/s40333-019-0015-8
Martins, R., José, A., Faria, J. M., and Melo, L. A. (2019). Effect of water and salt stress on seeds germination and vigor of different Eucalyptus species. J. Trop. For. Sci. 31, 12–18. doi: 10.26525/jtfs2019.31.1.012018
Munns, R. (2002). Comparative physiology of salt and water stress. Plant Cell Environ. 25, 239–250. doi: 10.1046/j.0016-8025.2001.00808.x
Omami, E. N., Haigh, A. M., Medd, R. W., and Nicol, H. I. (1999). Changes in germinability, dormancy and viability of Amaranthus retroflexus as affected by depth and duration of burial. Weed Res. 39, 345–354. doi: 10.1046/j.1365-3180.1999.00149.x
Parihar, P., Singh, S., Singh, R., Singh, V. P., and Prasad, S. M. (2014). Effect of salinity stress on plants and its tolerance strategies: a review. Environ. Sci. Pollut. R. 22, 4056–4075. doi: 10.1007/s11356-014-3739-1
Qi, R. L., Srty, Li, G. T., Wang, M. Z., He, L., and Yang, W. B. (2021). Effects of drought and salt stress on germination characteristics of Halimodendron halodendron (Pall.) Voss. Northern Hortic. 15, 81–88.
Qu, X. X., Huang, Z. Y., Baskin, J. M., and Baskin, C. C. (2008). Effect of temperature, light and salinity on seed germination and radicle growth of the geographically widespread halophyte shrub Halocnemum strobilaceum. Ann. Bot. 101, 293–299. doi: 10.1093/aob/mcm047
Santo, A., Mattana, E., Frigau, L., Pastor, A. M., Morello, M. C. P., and Bacchetta, G. (2017). Effects of NaCl stress on seed germination and seedling development of Brassica insularis Moris (Brassicaceae). Plant Biol. 19, 368–376. doi: 10.1111/plb.12539
Sarker, B. C., Hara, M., and Uemura, M. (2005). Proline synthesis, physiological responses and biomass yield of eggplants during and after repetitive soil moisture stress. Sci. Hortic. 103, 387–402. doi: 10.1016/j.scienta.2004.07.010
Shen, X. X., Chai, M. W., Li, R. L., and Qiu, G. Y. (2014). Interactive effects of salt and drought stresses on seed germination of Ammopiptanthus mongolicus in northwest China. Appl. Mech. Mater. 614, 653–657.
Shen, X. X., Chai, M. W., Xiang, J., Li, R. L., and Qiu, G. Y. (2015). Survival strategies of Ammopiptanthus mongolicus and Zygophyllum xanthoxylon in saline and drought environments, northwest China. Acta Physiol. Plant 37, 213–220. doi: 10.1007/s11738-015-1962-0
Si, W., Zhuang, D. B., Zhang, Y. W., and Zhao, J. M. (2020). Ecological adaptation of ephemeral plants and its evolutionary significance. Guangdong Canye 54, 13–17. doi: 10.1002/ece3.1590
Silva, E. C., Albuquerque, M. B., Azevedo, A. D., and Silva, C. D. (2013). “Drought and Its Consequences to Plants – From Individual to Ecosystem,” in Responses of Organisms to Water Stress, ed. S. Akinci (Turkey: Intechopen), 17–47.
Silva, E. C., Nogueira, R. J. M. C., Vale, F. H. A., Melo, N. F., and Araújo, F. P. (2009). Water relations and organic solutes production in four umbu tree (Spondias tuberosa) genotypes under intermittent drought. Braz. J. Plant Physiol. 21, 43–53. doi: 10.1590/s1677-04202009000100006
Sircelj, H., Tausz, M., Grill, D., and Batic, F. (2005). Biochemical responses in leaves of two apple tree cultivars subjected to progressing drought. J. Plant Physiol. 162, 1308–1318. doi: 10.1016/j.jplph.2005.01.018
Souza, M. L., and Fagundes, M. (2014). Seed size as key factor in germination and seedling development of Copaifera langsdorffii (Fabaceae). Am. J. Plant Sci. 5, 2566–2573. doi: 10.4236/ajps.2014.517270
Taleisnik, E., Pérez, H., Córdoba, A., Moreno, H., Garcia Seffino, L., Arias, C., et al. (1998). Salinity effects on the early development stages of panicum coloratum: cultivar differences. Grass Forage Sci. 53, 270–278. doi: 10.1046/j.1365-2494.1998.00139.x
Tobe, K., Li, X., and Omasa, K. (2005). Effects of irrigation on seedling emergence and seedling survival of a desert shrub Haloxylon ammodendron (Chenopodiaceae). Aust. J. Bot. 53, 529–534. doi: 10.1071/BT04210
Tobe, K., Zhang, L., Qiu, G. Y., Shimizu, H., and Omasa, K. (2001). Characteristics of seed germination in five non-halophytic Chinese desert shrub species. J. Arid Environ. 47, 191–201. doi: 10.1006/jare.2000.0689
Trenberth, K. E., Dai, A. G., Van Der Schrier, G., Jones, P. D., Barichivich, J., Briffa, K. R., et al. (2014). Global warming and changes in drought. Nat. Clim. Change 4, 17–22. doi: 10.1038/nclimate2067
Ueckermann, C., and Van Rooyen, M. W. (2000). Insect pollination and seed set in four ephemeral plant species from Namaqualand. S. Afr. J. Bot. 66, 28–30. doi: 10.1016/s0254-6299(15)31048-6
Ungar, I. A. (1995). “Seed development and germination,” in Seed Germination and Seed-Bank Ecology in Halophytes, eds J. Kigel and G. Galili (New York, NY: Marcel Dekke), 599–628.
van der Weele, C. M., Spollen, W. G., Sharp, R. E., and Baskin, T. I. (2000). Growth of Arabidopsis thaliana seedlings under water deficit studied by control of water potential in nutrient-agar media. J. Exp. Bot. 51, 1555–1562. doi: 10.1093/jexbot/51.350.1555
Wang, J. R., Wang, L., Xu, X. Y., Yan, H. Y., Hu, S. X., Gao, C. B., et al. (2020). Experimental study on salt tolerance of seeds of four annual plants during germination. Pratacult. Sci. 37, 237–244.
Wang, W. B., Kim, Y. H., Lee, H. S., Kim, K. Y., Deng, X. P., and Kwak, S. S. (2009). Analysis of antioxidant enzyme activity during germination of alfalfa under salt and drought stresses. Plant Physiol. Biochem. 47, 570–577.
Wang, Y., Jiang, G. Q., Han, Y. N., and Liu, M. M. (2013). Effects of salt, alkali and salt–alkali mixed stresses on seed germination of the halophyte Salsola ferganica (Chenopodiaceae). Acta Ecol. Sin. 33, 354–360. doi: 10.1016/j.chnaes.2013.09.010
Wei, Y., Dong, M., and Huang, Z. Y. (2007). Seed polymorphism, dormancy and germination of Salsola affinis (Chenopodiaceae), a dominant desert annual inhabiting the Junggar Basin of Xinjiang. China. Aust. J. Bot. 55, 464–470. doi: 10.1071/bt06016
Wu, C. H., Wang, Q. Z., Xie, B., Wang, Z. W., Cui, J., and Hu, T. M. (2011). Effects of drought and salt stress on seed germination of three leguminous species. Afr. J. Biotechnol. 10, 17954–17961. doi: 10.5897/ajb11.2018
Wu, D. Z., Shen, Q. F., Cai, S. G., Chen, Z. H., Dai, F., and Zhang, G. P. (2013). Ionomic responses and correlations between elements and metabolites under salt stress in wild and cultivated barley. Plant Cell Physiol. 54, 1976–1988. doi: 10.1093/pcp/pct134
Wulff, R. D. (1986). Seed size variation in desmodium paniculatum ll. effects on seedling growth and physiological performance. J. Ecol. 74, 115–121. doi: 10.2307/2260352
Xu, X. Y., Fan, R., Zheng, R., Li, C. M., and Yu, D. Y. (2011). Proteomic analysis of seed germination under salt stress in soybeans. J. Zhejiang Univ. Sci. B 12, 507–517. doi: 10.1631/jzus.B1100061
Yan, F., Zhu, Y. C., Zhao, Y. A., Wang, Y., Li, J. W., Wang, Q. Y., et al. (2020). De novo transcriptome sequencing and analysis of salt-, alkali-, and drought-responsive genes in Sophora alopecuroides. BMC Genomics 21:423. doi: 10.1186/s12864-020-06823-4
Yang, C. J., and Li, G. Y. (2014). Effect of Nacl stress on germination of birch seeds. J. Chem. Pharm. Res. 6, 1980–1986.
Yao, H., and Tan, D. Y. (2005). Size-dependent reproductive output and life-history startegies in four ephemeral species of Trigonella. Acta Phytoecol. Sin. 29, 954–960.
Zhao, T. J., Liu, Y., Yan, Y. B., Feng, F., Liu, W. Q., and Zhou, H. M. (2007). Identification of the amino acids crucial for the activities of drought responsive element binding factors (DREBs) of Brassica napus. FEBS Lett. 581, 3044–3050. doi: 10.1016/j.febslet.2007.05.067
Zhao, Y. Y., Lu, Z. H., and He, L. (2014). Effects of saline-alkaline stress on seed germination and seedling growth of Sorghum bicolor (L.) Moench. Appl. Biochem. Biotech. 173, 1680–1691. doi: 10.1007/s12010-014-0956-5
Keywords: arid desert areas, ephemeral plant, salt and drought stress, seed germination, recovery germination
Citation: Lu Y, Liu H, Chen Y, Zhang L, Kudusi K and Song J (2022) Effects of drought and salt stress on seed germination of ephemeral plants in desert of northwest China. Front. Ecol. Evol. 10:1026095. doi: 10.3389/fevo.2022.1026095
Received: 23 August 2022; Accepted: 06 October 2022;
Published: 25 October 2022.
Edited by:
Dechang Cao, Kunming Institute of Botany (CAS), ChinaReviewed by:
Xie Jiangbo, Zhejiang Agriculture and Forestry University, ChinaCristian Atala, Pontificia Universidad Católica de Valparaíso, Chile
Copyright © 2022 Lu, Liu, Chen, Zhang, Kudusi and Song. This is an open-access article distributed under the terms of the Creative Commons Attribution License (CC BY). The use, distribution or reproduction in other forums is permitted, provided the original author(s) and the copyright owner(s) are credited and that the original publication in this journal is cited, in accordance with accepted academic practice. No use, distribution or reproduction is permitted which does not comply with these terms.
*Correspondence: Huiliang Liu, liuhuiliang@ms.xjb.ac.cn