Bioaccumulation and Risk Assessment of Potentially Toxic Elements in Soil-Rice System in Karst Area, Southwest China
- 1School of Environmental Studies, China University of Geosciences, Wuhan, China
- 2Key Laboratory of Karst Dynamics, Ministry of Natural Resources and Guangxi, Institute of Karst Geology, Chinese Academy of Geological Sciences, Guilin, China
- 3School of Medical Laboratory, Guilin Medical University, Guilin, China
The accumulation of potentially toxic elements (PTE) in a soil–rice system poses a significant issue of concern in agricultural soils, particularly in the polluted or high PTE geological background regions, such as karst areas. The source identification, bioaccumulation factors of PTE, and its health risk were investigated by correlation analysis, principal components analysis, and single/comprehensive assessments in a soil-rice system in Mashan County, Guangxi Province. The results showed that the mean contents of PTE in rice rhizosphere soil samples were higher than Guangxi surface soil, but lower than Mashan background. Of the samples, 84.21% have Cd content exceeding the soil environmental quality -- risk control standard for soil contamination of agricultural land in China (GB 15618-2018) risk screening value. The Nemerow comprehensive pollution index indicated that 21.05 and 26.32% of the soil samples were moderately and heavily polluted. The contents of pH-related exchangeable Ca, exchangeable Mg, and redox-related available Fe and available Mn in soil affected the bioaccumulation of PTE in rice. In all the rice samples, 55.26% of Cd and 31.58% of Pb exceed the maximum allowable value of contaminants in rice recommended by the national food safety standard for maximum levels of contaminants in foods in China (GB 2762-2017). The average targeted hazard quotient values (THQ) of PTE decreased in an order of As > Cd > Cr > Cu > Zn > Pb > Hg, and the degree of health risk it posed to the population was Children > Female > Male. The hazard index (HI) of all samples was greater than one due to all THQAs and the THQCd of more than half samples were above 1, which implied that the residents were exposed to non-carcinogenic risk by rice ingestion. Therefore, the PTE in the karst area with a high geological background can be absorbed and migrated by crops, leading to a greater health risk to humans, which should be paid attention to in future research and agricultural management.
Introduction
With population growth and economic development, the natural process of slow release of heavy metals is accelerated, resulting in heavy metal pollution of soil, water, and the atmosphere (Bing et al., 2021; Savignan et al., 2021; Egbueri et al., 2022a). Heavy metals can accumulate in soil and dissolve into groundwater during leaching, resulting in groundwater and surface contamination of water (Ayejoto et al., 2022; Egbueri et al., 2022b). Heavy metal pollution in cropland soils is a global environmental issue of concern owing to its toxicological effects on soil-plant systems and humans (Bashir et al., 2018; Hou D. et al., 2020; Kukusamude et al., 2021). Cobalt (Co), copper (Cu), nickel (Ni), iron (Fe), manganese (Mn), zinc (Zn), molybdenum (Mo), and selenium (Se) are essential elements of the human body; still, they would cause health problems after years of insufficient intake or excessive intake of food exceeding the safety limit (Giri et al., 2020), while arsenic (As), cadmium (Cd), chromium (Cr), mercury (Hg), and lead (Pb) are nonessential to the human body, and these can cause health problems even at low contents (Giri et al., 2019; Jalili et al., 2020). Human exposure to heavy metals is through inhalation, oral ingestion, and skin absorption (Sanaei et al., 2021; Ayejoto et al., 2022; Egbueri et al., 2022b). Rice is the most widely consumed grain on the planet, with global rice production exceeding 740 million tons in 2014, with Asian countries such as China, Thailand, Japan, and Indonesia leading the way in global rice production (Hu et al., 2016). Rice is one of the most essential staple foods in China, particularly in southern areas. The average consumption of rice is 219 g/person/day, which is nearly 50% higher than the global average (Hu et al., 2016). Dietary intake of rice grown in soils with a high content of potentially toxic elements (PTE) exceeding the maximum allowable contaminant concentration could be a serious threat to human health (Chen et al., 2018; Mu et al., 2020). Previous studies revealed that the content of PTE in rice grains is mainly determined by soil physicochemical properties, element availability, and rice varieties (Li et al., 2019).
The content of PTE in rice grains is correlated with its corresponding soil (Baruah et al., 2021). Approximately 19.4% of farmland in China has been reported contaminated by PTE in 2014 (Qu et al., 2016), with higher contents of Cd and Pb distributed in northwest, south, and southwest China than elsewhere (Bing et al., 2021). Weathering of parent materials is a natural source of heavy metals in soils. High geological background characteristics of soil heavy metals have been found in karst areas such as Southwest China (Wen et al., 2020b; Tang et al., 2021; Yang et al., 2021b), the Indo-China Peninsula (Mallongi et al., 2022), and Europe (Savignan et al., 2021). The soil in the karst valley densely populated and concentrated in rural towns is strongly affected by anthropic activities. Soil heavy metals can be affected by wet and dry deposition of fossil fuel combustion, fertilizer and pesticide application, sewage irrigation, and improper disposal of waste (Egbueri et al., 2020; Egbueri et al., 2021b; Savignan et al., 2021). In addition, the combination of a high geological background of heavy metals and human activities makes crops in karst areas vulnerable to heavy metal pollution, which will affect future environmental sustainability and human health (Zhang et al., 2019; Zhang et al., 2021; Mallongi et al., 2022).
Due to the enrichment of heavy metal elements in the carbonate weathering process and soil genesis, the resulting soil has significantly high contents of PTE (Wen et al., 2020b; Yang et al., 2021b). The first national pollutant survey reflected that the average contents of As, Cd, Cr, Cu, Hg, Ni, Pb, and Zn in the surface soils of Guangxi where karst is widely distributed were 2.0, 4.5, 1.6, 1.1, 2.6, 1.3, 1.4, and 1.4 times higher than that of China, respectively. Due to the limited arable land per capita, part of farmlands with a high heavy metal geological background in the karst area in Southwest China is still used to grow crops (Zhang et al., 2019).
Limestone soil developed from weathering of carbonate rocks may affect the accumulation of trace elements in the edible parts of crop plants (Wen et al., 2020a; Li et al., 2021; Tang et al., 2021; Zhang et al., 2021). Yang et al. (2021a) studied the excess rate and influencing factors of Cd in the soil-rice system in karst areas and proposed a new safety standard based on pH and soil Cd content. Soil carbonate in the karst area increased soil pH, and the adsorption of Cd by Fe/Mn oxide/hydroxide significantly reduced the bioavailability of soil Cd, and the excess rate of rice in the karst area was much lower than that in the non-karst area (Li et al., 2021). The limestone soil in the karst area is calcium-rich and alkaline. Unfortunately, to the best of our knowledge, the effect of exchangeable Ca and Mg on the immobilization of heavy metals in the soil-rice system has not been reported yet. Therefore, the purposes of this study were to (1) evaluate the contents of 7 PTE (As, Cd, Cr, Cu, Hg, Pb, and Zn) in rice grains and rhizosphere soils of the karst area, identifying the source of heavy metals and understanding the influence of soil physicochemical properties related to pH and redox on the accumulation of PTE in the soil-rice system; and (2) assess the potential health (non-carcinogenic) risk of these PTE to children and adult through rice consumption.
Materials and Methods
Study Area
The studied area is located west of Mashan County, Guangxi Province, China (Figure 1). This area is characterized by a mean annual rainfall of 1722.5 mm, with an annual average temperature of 21.8°C. The central part of the study area is characterized by thick limestone layers with dolomitic limestone intercalations. Soils are dominated by brown rendzina. The land use types are mainly shrubs in mountainous areas and abandoned farmland in depressions. Non-carbonate rocks, comprising sandstones and mudstones, are distributed to the west, south, and east of the study area. The land uses in this area mainly include secondary forests and reservoirs. The valley consists of Holocene clay and loamy sand sediments, distributed among the mountains, and the land uses include paddy fields, drylands, and villages. Agricultural activities are the source of income for residents in the study area. Cultivated land includes two-season rice, water and vegetable rotation, corn and orchards, etc. Excessive use of chemical fertilizers and pesticides to pursue higher yields may bring heavy metal pollution to the cultivated soil.
Samples
Thirty-eight groups of rice and rhizosphere soil samples were collected in early November 2017 (Figure 1). Field sampling was conducted according to a standardized sampling method (MLRPRC Ministry of Land and Resources of the People’s Republic of China, 2016). At each sampling point, rice and rhizosphere soil were collected from four sub-spots within 20 m of the surrounding area and combined into a compositional sample, sealed, and transported with a plastic bag. After removing the debris and roots, the fresh soil sample was passed through a 2-mm nylon sieve to improve uniformity. Air-dried soil samples and polished rice were ground to a particle size of not more than 74 um for trace element analysis.
Laboratory Analysis
Contents of Cd, Cr, Cu, Pb, and Zn measuring were performed on inductively coupled plasma (ICP)-mass spectrometry (PerkinElmer Inc., United States, NexION 300) after digesting the soil samples with <0.074-mm particle size by HCl—HF—HClO4—HNO3 mixture, and rice sample digested by HNO3–H2O2. After digesting by aqua regia, and reducting by potassium borohydride, As and Hg were determined by atomic fluorescence spectroscopy. Soil pH was determined at a 1:2.5 (w/v) soil/water ratio by a precision pH meter (PHS-3C) in the lab. The soil organic carbon (Corg) was determined through titration using the potassium dichromate oxidation–ferrous ammonium sulfate method. Exchangeable calcium (ECa) and magnesium (EMg) were leached with 1 mol/L ammonium acetate (pH = 7.0), and measured by ICP-emission spectrometry (PerkinElmer Inc., United States, OPTIMA 8300). The availably iron (AFe), copper (ACu), zinc (AZn), and manganese (AMn) were leached with leaching solution (0.1 mol/L hydrochloric acids for acid soil, and DTPA for calcareous soil). The leaching solution was determined by ICP-emission spectrometry. The available silicon (ASi) was leached with 0.025 mol/L citric acid at 30°C for 5 h equilibrating, and the filtrate was taken for colorimetric comparison with silicon molybdenum blue. Standard materials of soil (GBW07417 for effective state analysis of soil elements, GBW07427 for total concentration analysis of soil elements) and rice (GSB-1 and GSB-23, both for total concentration analysis of rice elements) covered all studied elements and were tested among every ninth sample, revealing that the average analytical errors were about 5%.
PTE Contamination Assessment
Pollution Load Index and Potential Ecological Risk Index
The contamination factor (CF) has been used to assess the pollution level of trace elements in the soil since the 1980s (Tomlinson et al., 1980; Kowalska et al., 2018). The CF values of PTE in soil were calculated as follows:
where Ci and CRefi are the contents of element “i” (mg/kg) in the soils and background value of the PTE in Mashan County, which was obtained from this study and a geochemical survey of land quality in 2016, respectively (Table 1).
Correspondingly, the pollution degree of a single element is grouped into four levels: low (CF < 1), moderate (1 ≤ CF < 3), equivalent (3 ≤ CF < 6), and extremely high (CF F 6) (Hakanson, 1980; Egbueri et al., 2021b; Egbueri and Agbasi, 2022).
To evaluate the overall degree of heavy metal contamination across all sampling sites, the pollution load index (PLI) was proposed to represent total pollution of all PTE in the soil, which is calculated as follows:
where n is the total number of the studied PTE consisting of As, Cd, Cr, Cu, Hg, Pb, and Zn. The contamination degrees were further classified as PLI <1, 1 ≤ PLI <2, 2 ≤ PLI <3, and PLI ≥3, which indicated low, moderate, high, and very high contamination, respectively (Egbueri et al., 2021b).
The potential ecological factor (PEF) has been widely applied to assess the ecological risk of toxic PTE in soils (Hakanson, 1980; Gu et al., 2021). The PEF values are calculated as follows:
where Tri is the biological toxicity coefficient for heavy metal i (10, 30, 2, 5, 40, 5, and one for As, Cd, Cr, Cu, Hg, Pb, and Zn, respectively) (Hakanson, 1980). The potential ecological risk index (RI) can be calculated by the following function:
The classification standards of ecological risks for PEF and RI are shown in Supplementary Table S1.
Nemeiro Comprehensive Pollution Index
Single pollution index (Pi) is calculated to assess heavy metal pollution in soils and rice as follows:
where Ci represents contents of the element i in the soil or rice sample, Si represents contents of component i in risk screening values for soil contamination of agriculture land GB 15618-2018 (MEEPRC Ministry of Ecology and Environment of the People’s Republic of China, 2018), or maximum levels of contaminants in foods GB 2762-2017 (NHFPCPRC and CFDA, National Health and Family Planning Commission and China Food and Drug Administration, 2017) in China, respectively. The risk screening values for soil and maximum levels of contaminants for rice were listed in Supplementary Table S2. As GB 2762-2017 only stipulates the content limits of As, Cd, Cr, Hg, and Pb in rice (Supplementary Table S2), these elements were assessed by Pi in this study. Soil or crop are polluted by metal i if Pi > 1 (Hu et al., 2017).
The Nemerow Comprehensive Pollution Index (NCPI) is simple in form and has good applicability. It is commonly used for heavy metal comprehensive pollution evaluation, and expressed as:
where Pimax and Piave represent the maximum and average Pi value of PTE, respectively. According to NCPI, five levels are used to classify the pollution level of PTE: clean (≤0.7), prevention (0.7 ∼1.0), light pollution (1.0∼2.0), moderate (2.0 ∼3.0), and heavy pollution (>3.0) (Hu et al., 2017; Kowalska et al., 2018).
Bioaccumulation factor
The transfer ratio of PTE from soil to the crop could be quantified by bioaccumulation factor (BAF) (Kumar et al., 2019) as follows:
where Ci rice and Ci soil are the contents of element i in the rice and the corresponding rhizosphere soils, respectively.
Human Exposure and Risk Assessment
Health risk for three groups of people—children, adult women, and adult men—through rice consumption was evaluated by estimated daily intake (EDI), targeted hazard quotient (THQ), and hazard index (HI), as proposed by (USEPAU.S. Environmental Protection Agency, 1989)
where EDIi is the total daily exposure of element i [mg/(kg bodyweightday)], Ci is the content of i in polished rice (mg/kg), and DI is the recommended dose of rice intake for residents during 2013–2018 [0.25 and 0.40 kg/day for children and adults, according to the minimum and maximum recommended amounts of cereals by Chinese Nutrition Society (CNS Chinese Nutrition Society, 2021)]; BW is the body weight of children (9 years old), adult men and women, which are 30, 66.2, and 57.3 kg on average, respectively (Zuo et al., 2019). THQi is the ratio of element i between EDI and reference dose (RfDis). The RfD is considered safe for lifetime exposure to PTE and was enumerated in Supplementary Table S3. It may cause side effects to health when THQ >1. HI assumes that eating certain types of food may result in simultaneous exposure to multiple PTE. If HI < 1, harmful health effects are assumed to be unlikely to happen, while there are non-carcinogenic risks that could arise when HI > 1 (Zeng et al., 2015).
Data Analysis
Chemometric analysis including correlation analysis, unrotated principal component analysis, and varimax-rotated factor analysis were performed for source apportionment of soil PTE and risk assessment of soil-rice system by SPSS Statistics 18 (IBM, United States). Boxplots were performed by Origin 8.5 (Origin Lab, United States).
Results and Discussion
Contents and Contamination Levels of PTE in the Soil
The statistical analysis results of PTE are exhibited in Table 1. On average, the contents of As, Cd, Cr, Cu, Hg, Pb, and Zn in soils were 7.45 ± 4.98, 1.53 ± 1.49, 83.13 ± 33.45, 24.3 ± 7.53, 0.18 ± 0.07, 32.67 ± 10.75, and 137.47 ± 70.75 mg/kg, respectively. Soil pH ranged from acidic (4.83) to alkaline (8.18). The results showed that the averaged contents of all PTE in soil samples were significantly lower than the corresponding local background values of Mashan County but higher than the background value of surface soil in Guangxi except for As (Hou Q. Y. et al., 2020) (Table 1). There were 6, 2, 2, 11, 6, and 7 in 38 samples of Cd, Cr, Cu, Hg, Pb, and Zn that exceeded the local background values.
The coefficient of variations (CV) of the PTE in the soils was, in a decreased order, Cd (97.35%), As (66.76%), Zn (51.47%), Cr (40.24%), Hg (37.55%), Pb (32.89%), and Cu (30.99%), respectively (Table 1), suggesting that Cd was obviously enriched in some soil samples, while Zn, Cr, Hg, Pb, and Cu were slightly enriched in some areas. In addition, results of the Kolmogorov-Smirnov test for normality also showed that all the studied soil element contents were skewed distributions.
PTE in almost all soil samples had a CF value below 3, except for one sample with CFCd of 3.24 (Figure 2A; Table 2), indicating that the contamination levels of PTE were low or slightly moderate (Kowalska et al., 2018). The average PLI value was 0.60 ± 0.27, and 5 out of 38 samples had a PLI value greater than 1, with the maximum value of 1.35. The relatively low PLI value indicated that the soil was mildly polluted due to the high geological background (Kowalska et al., 2018).
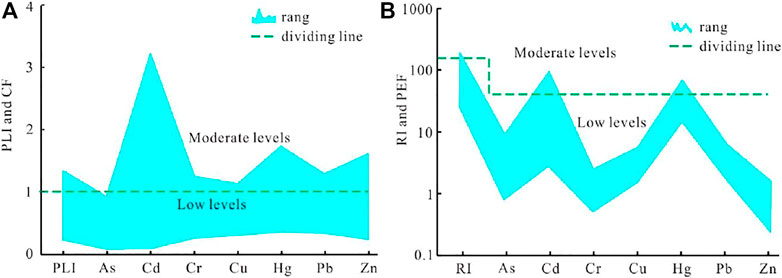
FIGURE 2. Contamination levels (A) and potential ecological risk (B) of each PTE in the soils of the karst. The blue bands indicate the range of contamination factor (CF) and pollution load index (PLI) (A), and potential ecological factor (PEF) and potential ecological risk index (RI) (B) of PTE in soils; and the green dotted line divides the low and moderate levels.

TABLE 2. Number of soil samples with PTE pollution assessed by contamination factor (CF) and pollution load index (PLI) in the study area.
The PEF of soil samples was 3.37 ± 2.25, 19.24 ± 18.73, 1.18 ± 0.48, 3.03 ± 0.94, 35.59 ± 13.37, 3.68 ± 1.21, and 0.69 ± 0.35 for As, Cd, Cr, Cu, Hg, Pb, and Zn, respectively (Figure 2B). Only 3 samples of Cd and 11 samples of Hg had a moderate potential risk in the study area (Figure 2B). The RI values ranged from 23.70 to 190.30, with an average value of 66.77 ± 35.00, demonstrating that all soil samples were at a pollution-free level.
However, according to the risk screening values of As, Cd, Cr, Cu, Hg, Pb, and Zn stipulated in GB 15618-2018, the contents of Cd in 84.21% of soil samples were above the risk screening values, followed by Zn, with 10.53% of samples exceeding the risk screening values, while As, Cr, Cu, Hg, and Pb in paddy soils were lower than the guideline (Figure 3). NCPI of 21.05 and 26.32% soil samples showed moderate and heavy pollution (Table 3), which is common in karst areas (Wen et al., 2020b). With the increase of weathering intensity, the content of Fe/Al/Mn oxides, organic carbon, and clay minerals increases, and leads to the enrichment of Cd in soil (Wen et al., 2020b; Yang et al., 2021b).
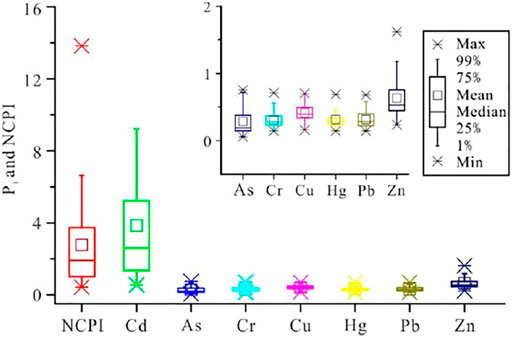
FIGURE 3. Boxplots of heavy metal single pollution index (Pi) and Nemerow Comprehensive Pollution Index (NCPI) of soil in the study area.

TABLE 3. Number of soil samples with PTE pollution assessed by Nemerow composite pollution index (NCPI) according to GB 15618-2018 in the study area.
Chemometric Analysis for Source Identification and Risk Assessment of PTE in Soil
Correlation Analysis
The correlation coefficients between soil pH, SOC, effective state, and heavy metals (As, Cd, Cr, Cu, Hg, Pb, and Zn) in the studied soils were calculated to study the influencing factors on the enrichment of heavy metals in soils (Supplementary Table S4). Contents of As, Cd, Cr, Cu, Hg, Pb, Zn, ASi, and AMn in soils were significantly positively correlated with each other (p < 0.01). High ECa and EMg content can increase the adsorption of heavy metal ions (Kokkinos et al., 2020), can also increase soil pH, and indirectly immobilize heavy metals (Hussain et al., 2021). The Corg was significantly positively correlated with Cd, Cu, Hg, and Pb, consistent with the results of previous studies (Mu et al., 2020; Zhang et al., 2021). Available Fe was significantly (p < 0.01) negatively correlated with pH, ECa, EMg, ASi, and all studied PTE, but significantly positively correlated with ACu and AZn, indicating higher Fe availability in lower pH soils, similar to those reported for paddy soil in Bangladesh (Rahman et al., 2018) and India (Baruah et al., 2021). All the studied PTE were almost significantly negatively correlated with AFe in soils, which indicated that the activity of Fe is not conducive to the accumulation of heavy metals (Yu et al., 2016). The ferromanganese nodules have a strong ability to enrich heavy metals. The change of redox conditions results in the layered distribution of iron, manganese, and heavy metals in the nodules. Different mineral species in the nodules have different release and adsorption capacities of elements in the face of environmental changes, which results in different correlations (Frierdich and Catalano, 2012; Liu et al., 2021).
Principal Component Analysis
PCA can be used to identify potential sources of the PTE (Egbueri and Agbasi, 2022; Mallongi et al., 2022). The correlation coefficients of most variables in this area were relatively high, and common factors can be extracted from them; the corresponding probability P is close to 0, and the Kaiser-Meyer-Olkin test value is 0.831, which is suitable for PCA to establish an adequate understanding of the sources of heavy metals and other parameters in the analyzed samples. Results of the PCA are presented in Table 4. It can be seen from Table 4 that the information of the sources of PTE can be represented by 3 PCs, and the 3 PCs can explain 86.6% of the total variables, indicating that the first three factors can reflect most of the information of all the data. It was clear that As, Cd, Cr, Cu, Hg, Pb, Zn, pH, ASi, and AFe have significant loadings in PC1. This suggests that there may be a closer correlation between these parameters. The average content of all PTE did not exceed the local background value, and the excess rate was very low, indicating a main natural source of these PTE (Kowalska et al., 2018). They could be attributed to the weathering of carbonate minerals (Egbueri et al., 2021a). Various degrees of soil pollution of PTE were reported in the karst regions (Zhang et al., 2013). During the weathering and pedogenesis of heavy metal-rich carbonate rocks, alkaline Earth elements were leached, and heavy metals in soil were enriched by residues such as Fe/Mn oxides and clay minerals (Wen et al., 2020b). The carbonate rocks weathered soil characterized by high pH, high calcium carbonate, and soil organic matter content, which play an essential role in immobilizing PTE in soil (Guo et al., 2006; Zhao et al., 2010; Wang et al., 2015).
In PC2, significant loadings were observed on the ACu, Corg, AZn, and Cu (Table 4). Rice roots and straws are the main sources of organic matter in paddy soils. The enrichment coefficients of Cd, Cu, and Zn in the soil-rice system are relatively high. Previous studies believed that heavy metals mainly accumulated in the roots, and there was a good correlation between Corg and PTE. For instance, human activities such as water irrigation and application of fertilizers and pesticides may have a significant impact on the enrichment of metals in farmland (Yang et al., 2013). Thus, PC2 can be attributed to agricultural activity.
Furthermore, PC3 was noticed to have obvious loadings on AMn and ECa (Table 4), having a negative and positive loading, respectively. The variation showed differences in their origin or impact. As discussed above, ECa affects the mobility of heavy metals by buffering pH. While AMn was significantly positively correlated with organic matter and clay content, extremely significantly negatively correlated with pH and calcium carbonate content, and extremely significantly positively correlated with cation exchange capacity (Pu et al., 2010).
Varimax-Rotated Factor Analysis
The Varimax twiddle factors extracted in this study for soil heavy metal association and source identification are shown in Table 4. Similar to PCA, 86.6% of the information about the heavy metal data was explained by three-factor categories. Factor 1 has high loadings for As, Cd, Cr, Cu, Hg, Pb, Zn, pH, ASi, AFe, PLI, and RI (Table 4). Combined with the fact that there are only individual quarries in the locality and no other industries, therefore, Factor 1 was judged to be the “weathering residual source”. Meanwhile, the correlation between As, Cd, Cr, Hg, Pb, Zn, SPI, and RI in Factor 2 became stronger than in PC3. This observation seems to confirm that they may have the same origin. The extremely positive correlation of ECa and EMg with Factor 2 suggested the source of carbonate rock, the nearby limestone quarries. Furthermore, leaded gasoline, diesel combustion, fossil fuel burning, braking, and vehicular emission were potentially elevated PTE levels in the soil. Therefore, Factor 2 may be attributed to the mixture of mineral powder and fuel exhaust particles in the farmland soil in the form of dry and wet deposition. Moreover, recent studies found that several elements such as Zn, Cd, Pb, and As in alluvial deposits are related to their easily transportable forms (soluble forms) (Fonseca et al., 2021). Toxic elements in soils developed from carbonate rocks can also be transported and deposited in downstream alluvial plains (Hou Q. et al., 2020). Factor 3 explained about 14.674% of the total variance and was considered loaded for Corg, ACu, Cu, and AZn (Table 4), which is the same as PC2 related to agricultural resources. They can come from wastewater irrigation, fertilizer application, pesticides, organic manures, compost, and sewage sludges.
Contents and Risk Assessment of PTE in Rice
The mean contents of As, Cd, Cr, Cu, Hg, Pb, and Zn in rough rice were 0.10 ± 0.03, 0.28 ± 0.26, 0.36 ± 0.29, 4.12 ± 3.12, 0.0045 ± 0.0014, 0.15 ± 0.17, and 14.64 ± 2.28 mg/kg, respectively (Table 1; Figure 4A). The Pi of the As, Cd, Cr, Hg, and Pb of rice samples varied from 0.29 to 1.05, 0.03 to 5.20, 0.03 to 1.54, 0.01 to 0.35, and 0.03 to 3.00, respectively. The average Pi decreased in the order of Cd (1.38) >Pb (0.77) > As (0.52) > Cr (0.36) > Hg (0.13) (Figure 4B). In the present study, 55.26% of Cd (21 samples) and 31.58% of Pb (12 samples) were higher than the maximum allowable value of 0.2 mg/kg for cereal grains recommended by NHFPCPRC and CFDA, National Health and Family Planning Commission and China Food and Drug Administration (2017), respectively, and 15.79% (6 samples) of the samples had both Cd and Pb exceeding the maximum allowable value. The exceedance rate of inorganic As and Cr was 2.6%. This is consistent with the low exceedance rates of As, Cr, and Hg in rice grain in the karst areas of Guangxi (Yang et al., 2021a). The excess rate of Pb in this study was relatively high but does not exceed the maximum allowable value in Yang et al. (2021a). The Cd in 26.67% (75 in total) of the rice samples exceeded the maximum allowable value in karst regions of Liujiang County, Guangxi, with the mean contents of 0.16 mg/kg (Tang et al., 2021). Even higher excessive rates were found in the karst area of Jinchengjiang, Guangxi, 95% (40 in total) of rice grains harvested from limestone soil had a Cd content that surpasses the maximum permissible concentration (Li et al., 2018). The CVs of PTE in rice decreased in an order of Pb (109.21%), Cd (92.88%), Cr (80.18%), Cu (75.87%), Hg (31.57%), As (30.26%), and Zn (15.56%) in this study. The high spatial heterogeneity of Cd may lead to different results of the exceeded rate in different areas.
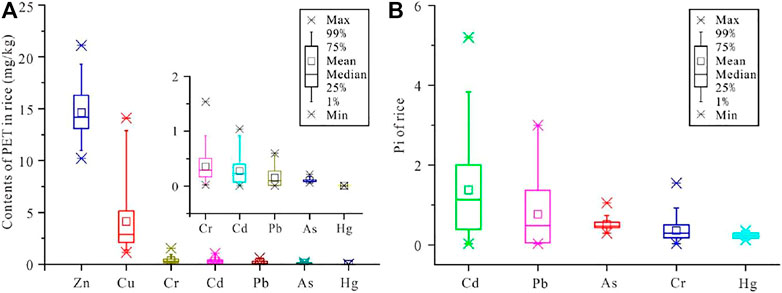
FIGURE 4. Boxplots of heavy metal contents (A) and single pollution index (Pi) (B) of rice in the study area (mg/kg).
Human Health Risk Assessments
The results of using EDI, THQ, and HI to assess the potential non-carcinogenic effect of long-term exposure to PTE of edible rice are shown in Table 5 and Figure 5. The average EDIs of As, Cd, Cr, Cu, Hg, Pb, and Zn for inhabitants by consuming rice were 0.00062, 0.00166, 0.00216, 0.02487, 0.00002, 0.00191, and 0.08849 mg/(kg BWday) for men, and 0.00072, 0.00192, 0.00250, 0.02874, 0.00002, 0.00107, and 0.10223 mg/(kg BWday) for women, and 0.00086, 0.00230, 0.00298, 0.03430, 0.00004, 0.00128, and 0.12204 mg/(kg BWday) for children. The average THQ values of PTE decreased in an order of As (2.08) >Cd (1.66) >Cr (0.72) >Cu (0.62) >Zn (0.29) >Pb (0.27) >Hg (0.09) for men, As (2.4) >Cd (1.92) >Cr (0.83) >Cu (0.72) >Zn (0.34) >Pb (0.31) >Hg (0.1) for women, and As (2.86)>Cd (2.3)>Cr (0.99) >Cu (0.86) >Zn (0.41) >Pb (0.37) >Hg (0.12) for children. The EDIs and THQs values of the PTE decreased in the order of children > women > men owing to the increasing body weight. The dietary risk of Cd and As in children is the highest, and decreases with the increasing body weight. The same results were reported by Chen et al. (2018), Baruah et al. (2021), and Mallongi et al. (2022). Children are also at higher health risks than adults by drinking substandard water. The HI, THQAs, and the average THQCd in all the samples were greater than 1 (Figure 5), indicating that residents suffer from non-carcinogenic risk by rice consumption. As and Cd were the biggest contributors to high HI. This is consistent with previous studies reporting that As and Cd intake of rice is the main contributor to health risks (Chen et al., 2018; Baruah et al., 2021; Tang et al., 2021). Given the high dietary Cd intake, effective agronomic measures should be considered to solve Cd pollution in the soil and reduce the transfer of Cd from the soil to edible crops (Hussain et al., 2021).

TABLE 5. The number of samples with non-carcinogenic risk [targeted hazard quotient (THQ)≥1] caused by rice intake of different groups in the study area.
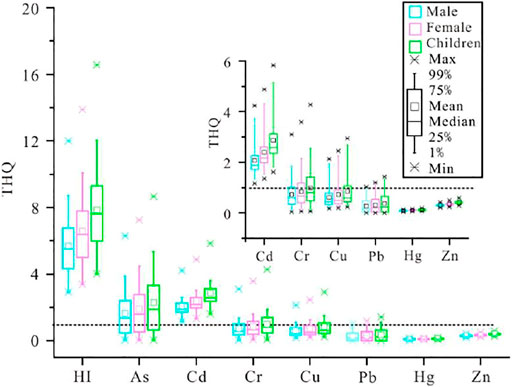
FIGURE 5. Box plots of targeted hazard quotient (THQ) for individual heavy metals and hazard index (HI) of men, women, and children (horizontal dotted line indicates the threshold value of the hazard quotient).
Influencing Factors of PTE Enrichment in Soil-Rice System
The BAF values of As, Cd, Cr, Cu, Hg, Pb, and Zn in rice were 0.02, 0.318, 0.005, 0.173, 0.030, 0.005, and 0.135, respectively, on average (Figure 6). The results are consistent with the fact that cadmium seems to prefer to be absorbed by the rice, although it is not an essential element. The average enrichment index for Cd, Zn, and Cu was 0.269, 0.160, and 0.083 in a soil–rice system in Wenling, Zhejiang Province, respectively, and varied significantly with heavy metals in paddy fields (Zhao et al., 2010). Cd can form a complex with plant peptide transporters; thus, it can be transported to the rice grains (Rizwan et al., 2012). Cd2+ could replace Ca2+ more easily than other PTE owing to their similar ion radius and same valence, and Cd would be transported actively into the grain through the Ca channel, while other PTEs could only be transported passively (Kim et al., 2002; Chang et al., 2014).
To determine the relationship between the PTE in rice and the corresponding rhizosphere soils, correlation analysis was performed. There was a certain correlation of PTE between rice and soil (Supplementary Table S5). The concentrations of Cd (p < 0.01), Hg (p < 0.01), Pb (p < 0.01), and Zn (p < 0.01) in soil correlated positively with As in rice, and the concentrations of As (p < 0.01), Cr (p < 0.05), Hg (p < 0.05), Pb (p < 0.05), and Zn (p < 0.05) in soil correlated positively with Hg in rice, indicating that these elements in soil promoted the accumulation of As and Hg in rice grains. However, the correlation of other heavy metals in rice and soil was not significant, even ACu was not significantly correlated with Cu in rice, indicating that the accumulation of heavy metals in the soil-rice system was also affected by other factors. Heavy metals are absorbed by soil organic matter and reduce the accumulation of heavy metals in crops. For example, the Hg content of rice, lgBAFHg, and lgBAFZn are negatively correlated with Corg (Supplementary Table S5). But the high Corg content will also increase the accumulation of As in rice (Norton et al., 2013). This study also found a significant positive correlation between As in rice and Corg in soil (Supplementary Table S5). Content of As, Cd, and Zn in rice and their lgBAF were significantly negatively correlated with pH, ECa, and EMg in soil, respectively, at different confidence levels (except that Cd was not significantly correlated with ECa) (Supplementary Table S5). Soil pH affects the dissolution of heavy metals, especially in acidic rice fields; low pH may result in increased solubility and high availability of heavy metals in rice. High exchangeable calcium and magnesium content can increase soil pH and indirectly immobilize heavy metals (Hussain et al., 2021), and also promote the adsorption capacity of heavy metals (Kokkinos et al., 2020), leading to negatively correlated results. However, the correlation between Hg in rice and elements in the soil is opposite to them. Hg in rice was significantly positively correlated with pH, ECa, and EMg in soil, but significantly negatively correlated with AFe, AZn, and ACu (Supplementary Table S5). Meanwhile, Cd and Zn in rice were significantly positively correlated with AFe, AZn, and ACu; lgBAF of As, Cd, and Zn were significantly positively correlated with AFe and AZn (Supplementary Table S5). This is consistent with previous reports that decreased rhizosphere Fe and Cd concentrations resulted in lower Cd concentrations in rice (Zhang et al., 2018). Iron is an essential and important element in plants, and the chemical properties and pathways into rice of Fe and Cd are similar, resulting in their high correlation in crops (Sharma et al., 2004; Chen et al., 2017; Liu et al., 2017). Changes in the redox environment affect the dissolution and bioavailability of heavy metals with different irrigation methods. Concentrations of iron oxides change due to redox changes in paddy fields, so it is reasonable that Fe oxide was significantly and positively correlated with BAF of As, Cd, and Zn since heavy metals may release from stable Fe oxide bound fraction which would increase the availability of heavy metals to rice. The correlation of AMn, AFe, and lgBAF of heavy metals can also be traced back to their correlation with heavy metal content in soil (Supplementary Tables S5 and S4), which may be related to changes in pH and redox conditions affecting the formation and evolution of iron-manganese nodules. Significantly negative correlations between ASi and lgBAFAs, lgBAFCd, lgBAFHg, and lgBAFZn indicate that Si can effectively alleviate the bioaccumulation of PTE (Supplementary Table S5), as suggested by a previous study (Li et al., 2018). In natural environments, soil properties such as pH, CEC, redox potential, minerals (phosphates, metal hydroxides, metal oxides, and clays), and SOM jointly affect the BCF of PTE (Ata-Ul-Karim et al., 2020). Future studies are recommended to investigate the interactions between soil properties to gain a comprehensive understanding of the bioavailability of PTE in soil-crop systems. For farmers, the use of unqualified mineral fertilizers or excessive use of pesticides should be avoided. Although the excess rate of As in soil and rice was very low, its non-carcinogenic risk to humans is high, so organic fertilizers should be used with caution to reduce the risk of As enrichment in rice. Meanwhile, attention should be paid to soil acidity and alkalinity, as well as the impact of irrigation methods on the accumulation of heavy metals in rice.
Conclusion
The average concentration of all studied PTE in rhizosphere soil of rice samples was below the local background value of Mashan County, but higher than the background value of surface soil in Guangxi except for As. Results of contaminant risk assessment using background values as reference values indicated a low-risk status. However, Cd in 84.21% of the samples exceeded the risk screening value of GB 15618-2018, and 21.05 and 26.32% of the samples were moderately and heavily polluted in the NCPI assessment. Weathering of parent rocks and alluvial deposits are the major source of heavy metals in soils, while fossil fuel combustion and agricultural activities also contribute to the accumulation of soil PTE. Cd in 55.26% and Pb in 31.58% of rice samples exceed the maximum allowable value of rice of China. The high excessive rate of Cd and Pb could be attributed to their high bioaccumulation factor and high content in the soil. Residents may be exposed to As and Cd through rice consumption, resulting in significant non-carcinogenic health risks, especially children. Health risks caused by excessive consumption of wild heavy metal-enriched rice should be avoided. Residents are expected to apply appropriate agricultural products and irrigation methods to mitigate the risk of PTE enrichment in rice, and future research should place a high value on rice cultivars and bioavailability of PTE in agricultural soils in the karst areas.
Data Availability Statement
The raw data supporting the conclusion of this article will be made available by the authors, without undue reservation.
Author Contributions
XZ had the original ideas, designed the research, and acquired financial support for the project leading to this publication. CZ collected the sample, carried out the data analysis, and prepared the original draft. HY, JL, and TZ analyzed data for figures and tables, and helped to prepare the original draft. All authors contributed to manuscript development and edited the final version.
Funding
This study was funded by the Natural Science Foundation of Guangxi (2017GXNSFBA198090), the Guangxi Science and Technology Base and Talent Project (AD20297090), Guangxi Key Science and Technology Innovation Base on Karst Dynamics (KDL and Guangxi202209), China Geological Survey (DD20160324-03), and Guilin Science and Technology Project (2020010403).
Conflict of Interest
The authors declare that the research was conducted in the absence of any commercial or financial relationships that could be construed as a potential conflict of interest.
Publisher’s Note
All claims expressed in this article are solely those of the authors and do not necessarily represent those of their affiliated organizations, or those of the publisher, the editors, and the reviewers. Any product that may be evaluated in this article, or claim that may be made by its manufacturer, is not guaranteed or endorsed by the publisher.
Supplementary Material
The Supplementary Material for this article can be found online at: https://www.frontiersin.org/articles/10.3389/fenvs.2022.866427/full#supplementary-material
References
Ata-Ul-Karim, S. T., Cang, L., Wang, Y., and Zhou, D. (2020). Effects of Soil Properties, Nitrogen Application, Plant Phenology, and Their Interactions on Plant Uptake of Cadmium in Wheat. J. Hazard. Mater. 384, 121452. doi:10.1016/j.jhazmat.2019.121452
Ayejoto, D. A., Agbasi, J. C., Egbueri, J. C., and Echefu, K. I. (2022). Assessment of Oral and Dermal Health Risk Exposures Associated with Contaminated Water Resources: an Update in Ojoto Area, Southeast Nigeria. Int. J. Environ. Anal. Chem., 1–21. doi:10.1080/03067319.2021.2023515
Baruah, S. G., Ahmed, I., Das, B., Ingtipi, B., Boruah, H., Gupta, S. K., et al. (2021). Heavy Metal(loid)s Contamination and Health Risk Assessment of Soil-rice System in Rural and Peri-Urban Areas of Lower Brahmaputra valley, Northeast India. Chemosphere 266, 129150. doi:10.1016/j.chemosphere.2020.129150
Bashir, S., Hussain, Q., Akmal, M., Riaz, M., Hu, H., Ijaz, S. S., et al. (2018). Sugarcane Bagasse-Derived Biochar Reduces the Cadmium and Chromium Bioavailability to Mash Bean and Enhances the Microbial Activity in Contaminated Soil. J. Soils Sediments 18, 874–886. doi:10.1007/s11368-017-1796-z
Bing, H., Qiu, S., Tian, X., Li, J., Zhu, H., Wu, Y., et al. (2021). Trace Metal Contamination in Soils from Mountain Regions across China: Spatial Distribution, Sources, and Potential Drivers. Soil Ecol. Lett. 3, 189–206. doi:10.1007/s42832-021-0080-8
Chang, C. Y., Yu, H. Y., Chen, J. J., Li, F. B., Zhang, H. H., and Liu, C. P. (2014). Accumulation of Heavy Metals in Leaf Vegetables from Agricultural Soils and Associated Potential Health Risks in the Pearl River Delta, South China. Environ. Monit. Assess. 186, 1547–1560. doi:10.1007/s10661-013-3472-0
Chen, H., Yang, X., Wang, P., Wang, Z., Li, M., and Zhao, F.-J. (2018). Dietary Cadmium Intake from rice and Vegetables and Potential Health Risk: A Case Study in Xiangtan, Southern China. Sci. Total Environ. 639, 271–277. doi:10.1016/j.scitotenv.2018.05.050
Chen, Z., Tang, Y.-T., Zhou, C., Xie, S.-T., Xiao, S., Baker, A. J. M., et al. (2017). Mechanisms of Fe Biofortification and Mitigation of Cd Accumulation in rice ( Oryza Sativa L.) Grown Hydroponically with Fe Chelate Fertilization. Chemosphere 175, 275–285. doi:10.1016/j.chemosphere.2017.02.053
CNS Chinese Nutrition Society (2021). Scientific Research Report on Dietary Guidelines for Chinese Residents. Beijing. (in Chinese).
Egbueri, J. C., and Agbasi, J. C. (2022). Data-driven Soft Computing Modeling of Groundwater Quality Parameters in Southeast Nigeria: Comparing the Performances of Different Algorithms. Environ. Sci. Pollut. Res. doi:10.1007/s11356-022-18520-8
Egbueri, J. C., Ayejoto, D. A., and Agbasi, J. C. (2022a). Pollution Assessment and Estimation of the Percentages of Toxic Elements to Be Removed to Make Polluted Drinking Water Safe: a Case from Nigeria. Toxin Rev., 1–15. doi:10.1080/15569543.2021.2025401
Egbueri, J. C., Enyigwe, M. T., and Ayejoto, D. A. (2022b). Modeling the Impact of Potentially Harmful Elements on the Groundwater Quality of a Mining Area (Nigeria) by Integrating NSFWQI, HERisk Code, and HCs. Environ. Monit. Assess. 194, 150. doi:10.1007/s10661-022-09789-w
Egbueri, J. C., Ezugwu, C. K., Unigwe, C. O., Onwuka, O. S., Onyemesili, O. C., and Mgbenu, C. N. (2021a). Multidimensional Analysis of the Contamination Status, Corrosivity and Hydrogeochemistry of Groundwater from Parts of the Anambra Basin, Nigeria. Anal. Lett. 54, 1–31. doi:10.1080/00032719.2020.1843049
Egbueri, J. C., Mgbenu, C. N., Digwo, D. C., and Nnyigide, C. S. (2021b). A Multi-Criteria Water Quality Evaluation for Human Consumption, Irrigation and Industrial Purposes in Umunya Area, southeastern Nigeria. Int. J. Environ. Anal. Chem., 1–25. doi:10.1080/03067319.2021.1907360
Egbueri, J. C., Ukah, B. U., Ubido, O. E., and Unigwe, C. O. (2020). A Chemometric Approach to Source Apportionment, Ecological and Health Risk Assessment of Heavy Metals in Industrial Soils from Southwestern Nigeria. Int. J. Environ. Anal. Chem., 1–19. doi:10.1080/03067319.2020.1769615
Fonseca, R., Pinho, C., Albuquerque, T., and Araújo, J. (2021). Environmental Factors and Metal Mobilisation in Alluvial Sediments-Minas Gerais, Brazil. Geosciences 11 (3), 110. doi:10.3390/geosciences11030110
Frierdich, A. J., and Catalano, J. G. (2012). Distribution and Speciation of Trace Elements in Iron and Manganese Oxide Cave Deposits. Geochimica et Cosmochimica Acta 91, 240–253. doi:10.1016/j.gca.2012.05.032
Giri, S., Mahato, M. K., Bhattacharjee, S., and Singh, A. K. (2020). Development of a New Noncarcinogenic Heavy Metal Pollution index for Quality Ranking of Vegetable, rice, and Milk. Ecol. Indicators 113, 106214. doi:10.1016/j.ecolind.2020.106214
Giri, S., Singh, A. K., and Mahato, M. K. (2019). Monte Carlo Simulation-Based Probabilistic Health Risk Assessment of Metals in Groundwater via Ingestion Pathway in the Mining Areas of Singhbhum Copper belt, India. Int. J. Environ. Health Res. 30, 447–460. doi:10.1080/09603123.2019.1599101
Gu, X., Xu, L., Wang, Z., Ming, X., Dang, P., Ouyang, W., et al. (2021). Assessment of Cadmium Pollution and Subsequent Ecological and Health Risks in Jiaozhou Bay of the Yellow Sea. Sci. Total Environ. 774, 145016. doi:10.1016/j.scitotenv.2021.145016
Guo, X., Zhang, S., Shan, X.-q., Luo, L., Pei, Z., Zhu, Y.-G., et al. (2006). Characterization of Pb, Cu, and Cd Adsorption on Particulate Organic Matter in Soil. Environ. Toxicol. Chem. 25, 2366–2373. doi:10.1897/05-636R.1
Hakanson, L. (1980). An Ecological Risk index for Aquatic Pollution control.a Sedimentological Approach. Water Res. 14, 975–1001. doi:10.1016/0043-1354(80)90143-8
Hou, D., O’Connor, D., Igalavithana, A. D., Alessi, D. S., Luo, J., Tsang, D. C. W., et al. (2020a). Metal Contamination and Bioremediation of Agricultural Soils for Food Safety and Sustainability. Nat. Rev. Earth Environ. 1, 366–381. doi:10.1038/s43017-020-0061-y
Hou, Q., Yang, Z., Yu, T., You, Y., Dou, L., and Li, K. (2020b). Impacts of Parent Material on Distributions of Potentially Toxic Elements in Soils from Pearl River Delta in South China. Sci. Rep. 10, 17394. doi:10.1038/s41598-020-74490-2
Hou, Q. Y., Yang, Z. F., Yu, T., Xia, X. Q., Cheng, H. X., and Zhou, G. H. (2020c). Soil Geochemical Parameters in China. Beijing: Geological Publishing House.
Hu, B., Jia, X., Hu, J., Xu, D., Xia, F., and Li, Y. (2017). Assessment of Heavy Metal Pollution and Health Risks in the Soil-Plant-Human System in the Yangtze River Delta, China. Ijerph 14, 1042. doi:10.3390/ijerph14091042
Hu, Y., Cheng, H., and Tao, S. (2016). The Challenges and Solutions for Cadmium-Contaminated Rice in China: A Critical Review. Environ. Int. 92-93, 515–532. doi:10.1016/j.envint.2016.04.042
Hussain, B., Ashraf, M. N., Shafeeq-ur-Rahman, R., Abbas, A., Li, J., and Farooq, M. (2021). Cadmium Stress in Paddy fields: Effects of Soil Conditions and Remediation Strategies. Sci. Total Environ. 754, 142188. doi:10.1016/j.scitotenv.2020.142188
Jalili, C., Kazemi, M., Taheri, E., Mohammadi, H., Boozari, B., Hadi, A., et al. (2020). Exposure to Heavy Metals and the Risk of Osteopenia or Osteoporosis: a Systematic Review and Meta-Analysis. Osteoporos. Int. 31, 1671–1682. doi:10.1007/s00198-020-05429-6
Kim, Y.-Y., Yang, Y.-Y., and Lee, Y. (2002). Pb and Cd Uptake in rice Roots. Physiol. Plantarum 116, 368–372. doi:10.1034/j.1399-3054.2002.1160312.x
Kokkinos, E., Chousein, C., Simeonidis, K., Coles, S., Zouboulis, A., and Mitrakas, M. (2020). Improvement of Manganese Feroxyhyte's Surface Charge with Exchangeable Ca Ions to Maximize Cd and Pb Uptake from Water. Materials 13, 1762. doi:10.3390/ma13071762
Kowalska, J. B., Mazurek, R., Gąsiorek, M., and Zaleski, T. (2018). Pollution Indices as Useful Tools for the Comprehensive Evaluation of the Degree of Soil Contamination-A Review. Environ. Geochem. Health 40, 2395–2420. doi:10.1007/s10653-018-0106-z
Kukusamude, C., Sricharoen, P., Limchoowong, N., and Kongsri, S. (2021). Heavy Metals and Probabilistic Risk Assessment via rice Consumption in Thailand. Food Chem. 334, 127402. doi:10.1016/j.foodchem.2020.127402
Kumar, S., Prasad, S., Yadav, K. K., Shrivastava, M., Gupta, N., Nagar, S., et al. (2019). Hazardous Heavy Metals Contamination of Vegetables and Food Chain: Role of Sustainable Remediation Approaches - A Review. Environ. Res. 179, 108792. doi:10.1016/j.envres.2019.108792
Li, C., Yang, Z., Yu, T., Hou, Q., Liu, X., Wang, J., et al. (2021). Study on Safe Usage of Agricultural Land in Karst and Non-karst Areas Based on Soil Cd and Prediction of Cd in rice: A Case Study of Heng County, Guangxi. Ecotoxicology Environ. Saf. 208, 111505. doi:10.1016/j.ecoenv.2020.111505
Li, D., Li, W., Lu, Q., Li, Y., Li, N., Xu, H., et al. (2018). Cadmium Bioavailability Well Assessed by DGT and Factors Influencing Cadmium Accumulation in rice Grains from Paddy Soils of Three Parent Materials. J. Soils Sediments 18, 2552–2561. doi:10.1007/s11368-018-1950-2
Li, D., Wang, L., Wang, Y., Li, H., and Chen, G. (2019). Soil Properties and Cultivars Determine Heavy Metal Accumulation in rice Grain and Cultivars Respond Differently to Cd Stress. Environ. Sci. Pollut. Res. 26, 14638–14648. doi:10.1007/s11356-019-04727-9
Liu, C., Massey, M. S., Latta, D. E., Xia, Y., Li, F., Gao, T., et al. (2021). Fe(II)-induced Transformation of Iron Minerals in Soil Ferromanganese Nodules. Chem. Geology. 559, 119901. doi:10.1016/j.chemgeo.2020.119901
Liu, H., Zhang, C., Wang, J., Zhou, C., Feng, H., Mahajan, M. D., et al. (2017). Influence and Interaction of Iron and Cadmium on Photosynthesis and Antioxidative Enzymes in Two rice Cultivars. Chemosphere 171, 240–247. doi:10.1016/j.chemosphere.2016.12.081
Mallongi, A., Astuti, R. D. P., Amiruddin, R., Hatta, M., and Rauf, A. U. (2022). Identification Source and Human Health Risk Assessment of Potentially Toxic Metal in Soil Samples Around Karst Watershed of Pangkajene, Indonesia. Environ. Nanotechnology, Monit. Manage. 17, 100634. doi:10.1016/j.enmm.2021.100634
MEEPRC Ministry of Ecology and Environment of the People's Republic of China (2018). Risk Control Standard for Soil Contamination of Agriculture Land. Beijing: MEEPRC
MLRPRC Ministry of Land and Resources of the People's Republic of China (2016). Specification of Land Quality Geochemical Assessment. Beijing: Geological Publishing House
Mu, T., Zhou, T., Li, Z., Hu, P., Luo, Y., Christie, P., et al. (2020). Prediction Models for rice Cadmium Accumulation in Chinese Paddy fields and the Implications in Deducing Soil Thresholds Based on Food Safety Standards. Environ. Pollut. 258, 113879. doi:10.1016/j.envpol.2019.113879
NHFPCPRC and CFDA, National Health and Family Planning Commission and China Food and Drug Administration (2017). National Standard for Food Safety: Limit of Contaminants in Food. Beijing: NHFPCPRC and CFDA
Norton, G. J., Adomako, E. E., Deacon, C. M., Carey, A.-M., Price, A. H., and Meharg, A. A. (2013). Effect of Organic Matter Amendment, Arsenic Amendment and Water Management Regime on rice Grain Arsenic Species. Environ. Pollut. 177, 38–47. doi:10.1016/j.envpol.2013.01.049
Pu, Y. L., Long, G. F., Gou, W. P., and Liu, S. Q. (2010). Status of Soil Available Manganese in Tibet and its Affecting Factors. J. SW China Nor.Univer.Natural Sci. Edition 35, 163. doi:10.13718/j.cnki.xsxb.2010.06.026
Qu, C., Shi, W., Guo, J., Fang, B., Wang, S., Giesy, J. P., et al. (2016). China's Soil Pollution Control: Choices and Challenges. Environ. Sci. Technol. 50, 13181–13183. doi:10.1021/acs.est.6b05068
Rahman, M. S., Biswas, P. K., Al Hasan, S. M., Rahman, M. M., Lee, S. H., Kim, K.-H., et al. (2018). The Occurrences of Heavy Metals in farmland Soils and Their Propagation into Paddy Plants. Environ. Monit. Assess. 190, 201. doi:10.1007/s10661-018-6577-7
Rizwan, M., Meunier, J.-D., Miche, H., and Keller, C. (2012). Effect of Silicon on Reducing Cadmium Toxicity in Durum Wheat (Triticum Turgidum L. Cv. Claudio W.) Grown in a Soil with Aged Contamination. J. Hazard. Mater. 209-210, 326–334. doi:10.1016/j.jhazmat.2012.01.033
Sanaei, F., Amin, M. M., Alavijeh, Z. P., Esfahani, R. A., Sadeghi, M., Bandarrig, N. S., et al. (2021). Health Risk Assessment of Potentially Toxic Elements Intake via Food Crops Consumption: Monte Carlo Simulation-Based Probabilistic and Heavy Metal Pollution index. Environ. Sci. Pollut. Res. 28, 1479–1490. doi:10.1007/s11356-020-10450-7
Savignan, L., Lee, A., Coynel, A., Jalabert, S., Faucher, S., Lespes, G., et al. (2021). Spatial Distribution of Trace Elements in the Soils of South-Western France and Identification of Natural and Anthropogenic Sources. Catena 205, 105446. doi:10.1016/j.catena.2021.105446
Sharma, S. S., Kaul, S., Metwally, A., Goyal, K. C., Finkemeier, I., and Dietz, K.-J. (2004). Cadmium Toxicity to Barley (Hordeum Vulgare) as Affected by Varying Fe Nutritional Status. Plant Sci. 166, 1287–1295. doi:10.1016/j.plantsci.2004.01.006
Tang, M., Lu, G., Fan, B., Xiang, W., and Bao, Z. (2021). Bioaccumulation and Risk Assessment of Heavy Metals in Soil-Crop Systems in Liujiang Karst Area, Southwestern China. Environ. Sci. Pollut. Res. 28, 9657–9669. doi:10.1007/s11356-020-11448-x
Tomlinson, D. L., Wilson, J. G., Harris, C. R., and Jeffrey, D. W. (1980). Problems in the Assessment of Heavy-Metal Levels in Estuaries and the Formation of a Pollution index. Helgolander Meeresunters 33, 566–575. doi:10.1007/BF02414780
USEPA U.S. Environmental Protection Agency (1989). “Risk-assessment Guidance for Superfund,” in Human Health Evaluation Manual. Part A. Interim Report (Final) (Washington: Office of Emergency and Remedial Response), Vol. 1.
Wang, C., Li, W., Yang, Z., Chen, Y., Shao, W., and Ji, J. (2015). An Invisible Soil Acidification: Critical Role of Soil Carbonate and its Impact on Heavy Metal Bioavailability. Sci. Rep. 5, 12735. doi:10.1038/srep12735
Wen, Y., Li, W., Yang, Z., Zhang, Q., and Ji, J. (2020b). Enrichment and Source Identification of Cd and Other Heavy Metals in Soils with High Geochemical Background in the Karst Region, Southwestern China. Chemosphere 245, 125620. doi:10.1016/j.chemosphere.2019.125620
Wen, Y., Li, W., Yang, Z., Zhuo, X., Guan, D.-X., Song, Y., et al. (2020a). Evaluation of Various Approaches to Predict Cadmium Bioavailability to rice Grown in Soils with High Geochemical Background in the Karst Region, Southwestern China. Environ. Pollut. 258, 113645. doi:10.1016/j.envpol.2019.113645
Yang, Q., Yang, Z., Filippelli, G. M., Ji, J., Ji, W., Liu, X., et al. (2021b). Distribution and Secondary Enrichment of Heavy Metal Elements in Karstic Soils with High Geochemical Background in Guangxi, China. Chem. Geology. 567, 120081. doi:10.1016/j.chemgeo.2021.120081
Yang, Q., Yang, Z., Zhang, Q., Liu, X., Zhuo, X., Wu, T., et al. (2021a). Ecological Risk Assessment of Cd and Other Heavy Metals in Soil-rice System in the Karst Areas with High Geochemical Background of Guangxi, China. Sci. China Earth Sci. 64, 1126–1139. doi:10.1007/s11430-020-9763-0
Yang, S., Zhou, D., Yu, H., Wei, R., and Pan, B. (2013). Distribution and Speciation of Metals (Cu, Zn, Cd, and Pb) in Agricultural and Non-agricultural Soils Near a Stream Upriver from the Pearl River, China. Environ. Pollut. 177, 64–70. doi:10.1016/j.envpol.2013.01.044
Yu, H.-Y., Li, F.-B., Liu, C.-S., Huang, W., Liu, T.-X., and Yu, W.-M. (2016). “Iron Redox Cycling Coupled to Transformation and Immobilization of Heavy Metals: Implications for Paddy Rice Safety in the Red Soil of South China,” in Advances in Agronomy. Editor D. L. Sparks (Academic Press), 279–317. doi:10.1016/bs.agron.2015.12.006
Zeng, F., Wei, W., Li, M., Huang, R., Yang, F., and Duan, Y. (2015). Heavy Metal Contamination in Rice-Producing Soils of Hunan Province, China and Potential Health Risks. Ijerph 12, 15584–15593. doi:10.3390/ijerph121215005
Zhang, B., Liu, L., Huang, Z., Hou, H., Zhao, L., and Sun, Z. (2021). Application of Stochastic Model to Assessment of Heavy Metal(loid)s Source Apportionment and Bio-Availability in rice fields of Karst Area. Sci. Total Environ. 793, 148614. doi:10.1016/j.scitotenv.2021.148614
Zhang, C., Li, Z., Yang, W., Pan, L., Gu, M., and Lee, D. (2013). Assessment of Metals Pollution on Agricultural Soil Surrounding a lead-zinc Mining Area in the Karst Region of Guangxi, China. Bull. Environ. Contam. Toxicol. 90, 736–741. doi:10.1007/s00128-013-0987-6
Zhang, Q., Zhang, L., Liu, T., Liu, B., Huang, D., Zhu, Q., et al. (2018). The Influence of Liming on Cadmium Accumulation in rice Grains via Iron-Reducing Bacteria. Sci. Total Environ. 645, 109–118. doi:10.1016/j.scitotenv.2018.06.316
Zhang, Z., Zhang, Q., Tu, C., Zhang, J., Lin, C., Fang, H., et al. (2019). Effects on Heavy Metals in Karst Region Soil and the Enrichment Characteristics of Rice-Rape Rotation. Pol. J. Environ. Stud. 28, 4485–4493. doi:10.15244/pjoes/99242
Zhao, K., Liu, X., Xu, J., and Selim, H. M. (2010). Heavy Metal Contaminations in a Soil-rice System: Identification of Spatial Dependence in Relation to Soil Properties of Paddy fields. J. Hazard. Mater. 181, 778–787. doi:10.1016/j.jhazmat.2010.05.081
Keywords: bioaccumulation, risk assessment, potentially toxic elements, soil-rice, karst
Citation: Zhang C, Zou X, Yang H, Liang J and Zhu T (2022) Bioaccumulation and Risk Assessment of Potentially Toxic Elements in Soil-Rice System in Karst Area, Southwest China. Front. Environ. Sci. 10:866427. doi: 10.3389/fenvs.2022.866427
Received: 31 January 2022; Accepted: 23 February 2022;
Published: 04 April 2022.
Edited by:
Johnbosco C. Egbueri, Chukwuemeka Odumegwu Ojukwu University, NigeriaReviewed by:
Johnson Agbasi, Chukwuemeka Odumegwu Ojukwu University, NigeriaAndrew Hursthouse, University of the West of Scotland, United Kingdom
Copyright © 2022 Zhang, Zou, Yang, Liang and Zhu. This is an open-access article distributed under the terms of the Creative Commons Attribution License (CC BY). The use, distribution or reproduction in other forums is permitted, provided the original author(s) and the copyright owner(s) are credited and that the original publication in this journal is cited, in accordance with accepted academic practice. No use, distribution or reproduction is permitted which does not comply with these terms.
*Correspondence: Xia Zou, zx0205@126.com