Pretreatment and Multi-Feed Anaerobic Co-digestion of Agro-Industrial Residual Biomass for Improved Biomethanation and Kinetic Analysis
- 1Centre for Energy and Environment, Malaviya National Institute of Technology, Jaipur, India
- 2Department of Microbiology, School of Life Sciences, Central University of Rajasthan Bandarsindri, Kishangarh, India
Batch biochemical methane potential (BMP) test of agro-industrial, agricultural, and municipal solid waste (MSW) individuals like groundnut straw, rice bran, and guar husk, mung bean husk (MBH), wheat straw (WS), and organic fraction of municipal solid waste (OFMSW), under mesophilic conditions, were performed to evaluate the biogas potential. BMP test for multi-feed anaerobic co-digestion (AcoD) of WS, OFMSW, and MBH at five mixing ratio was performed to evaluate the synergistic effect of multi feed-stocks of blending the feedstocks. Mixture ratio having OFMSW:WS:MBH of 25:5:70% composition resulted into 37, 20, and 4% higher methane yield up to 280 ml/g Volatile solid (VS) in comparison with mono-digestion of OFMSW, WS and MBH. Ultrasonic pretreatment was also performed and the experimental results showed that varying the sonication time have a significant improvement on substrate's biodegradation and solubilization augmenting the methane yield from OFMSW, WS, and MBH by 71, 75, and 46%, respectively at sonication period of 60 min. Effect of pretreatment on the substrate studied through Scanning electron microscopy (SEM), XRD and FT-IR analysis. Cone and Exponential models showed an average coefficient of determination R2 = 0.9855 and R2 = 0.9893, respectively, and RMSE values for cone model was lower than that of Exponential model showing Cone model was more précised.
Introduction
Developing country India facing energy security problem and striving to fulfill its energy demand by natural resources like coal, crude oil, and natural gas. Renewable energy sources like wind, solar, hydro, biomass, and geothermal have huge potential to wipe out this problem of energy demand with sustainability and an excellent alternative to declining fossil fuel reserves. Biomass is considered as carbon neutral for energy production through biogas, ethanol, and pellets production, still, its direct burning practiced over the country, its conversion to renewable bio-energy production is a sustainable way. As 686 MT (million tons) biomass is generated in the country annually (Hiloidhari et al., 2014; Wang et al., 2017; Kumar et al., 2018). Agricultural crops residues, organic fraction of municipal solid waste (OFMSW), food wastes, agro-industrial wastes, aquatic plants, and algae, animal dung etc. all are considered as biomass.
Agriculture, MSW, and agro-industrial waste materials embody potential sources of renewable energy production and making a contribution toward energy production independent from fossil fuels.
Organic Waste Generation
Agriculture biomass or waste are alternative feedstock to energy and chemical production. Leftover straw, stalk and husk of a crop plant are the crop residues after its harvesting (Devi et al., 2017). Agricultural wastes are agriculture crop residues i.e. residues from group of cereals, oilseeds, pulses, horticulture, and other crops. Most of the agricultural residues used for animal feeding, fuel for domestic application or gasification (Hiloidhari et al., 2014; Report RRECL, 2017).
Around 25% of biomass from crop residues is burnt on a global basis (Devi et al., 2017). Its on-farm burning, categorized as underutilization of resources and leading to environmental impacts (Hiloidhari et al., 2014; Kumar et al., 2018). Agricultural crop residues can be utilized into biogas production, textile making process, fuel and manure, etc. to mitigate the problem of on farm in-situ burning of surplus crop residues which is a source of GHG, smoke, aerosols, and particulate matters etc. (Nguyen et al., 2016; Devi et al., 2017). Animal dung from cattle, goats, ships, and poultry are highly loaded from organic matter content and having huge pollution potential of methane gas. It is utilized as potential substrate or inoculum in co-digestion in the biogas plants (Bundhoo et al., 2016). Also, Shah et al. (2015) has reported the anaerobic co-digestion of blends of maize residues, water hyacinth, giant reed, and poultry litter for biogas production at community scale.
India generates crop residues 686 MT annually, out of which 34% of crop residue is estimated as surplus (Hiloidhari et al., 2014). For Rajasthan state, agricultural activity generates 52.6 MT/year residues out of which 9.25% remain surplus (Report RRECL, 2017). The surplus fraction of residue availability from cereals is 29%, from oilseeds is 18%, and 23% from pulses crop in Rajasthan (Hiloidhari et al., 2014). Wheat (Triticum) crops cultivation is 2nd highest among cereals in the country, and 5th highest in the Rajasthan state. Wheat straw [Crop Residue Ratio (CRR) = 1.5] production is 15.8 MT/year having 30% share to total biomass generation in the state (Annual Report MA FW, 2017; Report RRECL, 2017). Wheat straw has composition of 30.1–39.2% cellulose, 22.2–34.0% hemicellulose, and 6.5–22.1% lignin (Chandra et al., 2012a,b; Bolado-Rodríguez et al., 2016; Yadav et al., 2018).
MSW generation is increasing in almost all cities with urbanization and population growth, accompanied by its economy. MSW handling is one of the chief issue facing Indian cities. Its poor and incautious management entails enormous impacts on public health, environment, and climate change (Shekdar, 2009; Yadav and Samadder, 2017; Gollapalli and Kota, 2018). Currently, India generates 62 MT MSW per year where collection and treatment efficiency is around 90 and 27%, respectively, which means 79% MSW directly goes to unsanitary landfilling (Planning Commission Report, 2014; MSW Report, 2017; Yadav and Samadder, 2017). Jaipur, one of the metro cities of India, generates 1,000 tons per day (TPD) MSW, 31% share alone in total state MSW generation with ~3.04 million population (Census, 2011; Municipal solid waste, 2017). Waste to energy potential in India with organic and inorganic portions are open for conversion to biofuel and for power generation. Presently, India has 2,554 MW potential as renewable energy potential from waste generated i.e., MSW and waste water (Kalyani and Pandey, 2014; MNRE Annual Report, 2017).
Improved income and population in a city led to a changed lifestyle of urban dwellers, and also causing an escalation in waste quantity and MSW composition altered. Knowledge of the composition of MSW is important for selecting the suitable waste processing and disposal practices since MSW volume and its composition differs considerably with the places having changes in food habits, cultural traditions, lifestyles, socio-economic conditions, and climate (Yay, 2015; Mboowa et al., 2017). OFMSW has composition around 17.5% cellulose, 10.7% hemicellulose, 9.6% lignin, 37.8% raw fiber, 7.7% protein, and 9.6% fat or oil (Rao and Singh, 2004). However, its composition varies with their nutrient content due to its heterogeneous nature with respect to place to place even in the same city. Through AD process, OFMSW is capable of generating 300–400 m3 biogas/ ton of VS of OFMSW (Dhar et al., 2017).
The agro-industrial sector represents one of the important sectors of the economy, responsible for processing or production of different foodstuffs and meanwhile also generating large quantity of waste. Major agro-industrial waste comes from food processing, juice and wine industry, edible oil refineries, slaughterhouse, and dairy processing. Many food processing wastes are sent to animal feeding, and chemical production and rest goes to landfilling (Bundhoo et al., 2016; Pellera and Gidarakos, 2017). Although glycerine as ideal co-substrate being pure and 100% degradability, other products like cheese whey, olive mill waste, sugar by-products, have been used for co-digestion with positive results (Mata-Alvarez et al., 2014). Here, Mung bean husk, groundnut shell, guar husk and rice bran have been studied as agro-industrial waste for biogas potential.
Mung bean (Vigna radiata) production is 4,77,245 tons sharing 20% of pulse production as 2nd highest production after gram pulse which has 44% share in pulses production in the state (Rajasthan Agri-Statistics Report, 2017). Groundnut (Arachis hypogea) crop production is 8,08,143 tons production in Rajasthan with 15.56% share to country (Rajasthan Agri-Statistics Report, 2017). Its shells (CRR = 0.3) are discarded waste, which is a carbohydrate rich biomass having composition 25.57% carbohydrate, crude protein 4.43% and lipid 0.50%, crude fiber 59%, ash content 2.5%, and moisture 8% (Abdulrazak et al., 2014; Hiloidhari et al., 2014). Guar or cluster bean, with the botanical name Cyamopsis tetragonoloba drought tolerant legume grown in the Rajasthan state of India. Guar meal is a protein and fiber rich nutritional food for livestock containing germ and hull part of it (Janampet et al., 2016). Rajasthan produces Guar crop 2,204,931 tons annually in 2014–15. Its residues are stalks and husk. Rice (Oryza sativa) crop is most widely consumed food grain in the world. This cereal has 103.73 MT cultivation in India being 2nd highest in India after sugarcane crop. Its production is 2,84,131 tons annually in Rajasthan state. Residues from rice milling industry are rice husk and bran. (Rajasthan Agri-Statistics Report, 2017). Rice bran has composition around 4.6% cellulose, 8.4% hemicellulose, 2.8% lignin, 28.5% starch, and 13.9% protein (Favaro et al., 2017).
Anaerobic Digestion
The AD is biological process categorized in the effective waste management as well as waste to energy technologies. As it avoids the natural emission of greenhouse gases like CH4 and CO2 from self-degradation of organic waste. Main products of AD process are CH4 for renewable energy production and a digestate as bio-fertilizer for soil amendment (Vivekanand et al., 2013; Bundhoo et al., 2016). As AD owing several benefits concerning environment, economy and maintenance, also have been used for processing agricultural waste, MSW, sewage sludge, livestock effluents, agro-industrial waste, therefore AD process being dealt in this study.
The AD consists of four consecutive processes i.e., Hydrolysis, acidogenesis, acetogenesis, and methanation as shown in Figure 1. In first phase, large polymers breakdown into monomers, carbohydrates into simple sugar, fat into fatty acid, and glycerol, protein into amino acid and peptide. In second phase, these monomers break down into carbonic acid, alcohols, H2, CO2, NH3. In this phase, O2 and CO2 consumed by facultative bacteria to create the anaerobic condition. In third phase i.e., acetogenesis, previous phase products are converted into acetic acid, H2 and CO2. This acetic acid and hydrogen are converted into methane by different methanogens i.e. archea in the last phase of AD i.e., methanogenesis (Kumar et al., 2018; Negi et al., 2018).
Nutritional imbalance and operating factors like biodegradability and chemical composition of single substrate make its direct utilization tough. Therefore, different nutrition composition substrates are digested together, also biogas yield gets improved. Anaerobic co-digestion provides benefits like process stabilization, pH and buffer capacity, avoid inhibitory or toxic compounds (formation of Volatile fatty acids (VFA)/ammonia), microorganism's good synergistic effect (Mata-Alvarez et al., 2014; Hagos et al., 2017; Pellera and Gidarakos, 2017). AcoD is a technique for simultaneous treatment of different organic residues proving better synergism through diverse co-substrates and microbial activity by improving methane yield in process. Suitable co-substrate is that which compensate with excess metabolites produced by another substrate which inhibit the methanogens in AD (Zhang et al., 2016). In simple words, AcoD is performed to balance the C/N ratio of the feedstock.
BMP test is a batch experiment of AD determining the biomethane potential of a substrate. This test is performed in laboratory for examining the biodegradability and methane conversion efficiency of organic substrates, assessing different combination of co-substrates, feasibility of different biomasses for AD, also helps in achieving optimal condition for AD process (Cho et al., 2013; Vivekanand et al., 2013; Hagos et al., 2017). A feasibility study of organic waste energy potential in college campus shows mixed food and green waste has biogas potential of 400–660 ml/g VS of the mixed substrate (Paritosh et al., 2018).
Kacprzak et al. (2010), experimented combination of agriculture waste (corn silage, carrot residues), agro-industrial waste (beet pulp silage and cheese whey) with industrial waste: glycerine (waste from biodiesel production), resulting highest methane yield with mixture of corn silage, cheese whey and glycerine (Kacprzak et al., 2010; Mata-Alvarez et al., 2014). Blending of few agro-industrial waste i.e. brewery spent grain, carbonated soft drink sludge, with either powdered rice husk or soya bean cake resulted into enhanced biogas production than individuals (Uzodinma et al., 2007). Vivekanand et al. (2018) co-digested manure, fish ensilage and whey as multi feed-stocks and produced 84% higher methane production than individual substrate digestion. Food waste co-digestion with straw (containing straw of wheat, maize, and sorgos) showed 39.5 and 149.7% augmented methane yield at 5:1 mixing ratio as compared to mono-digestion of food waste and straw, respectively (Yong et al., 2015). Co-digestion of OFMSW and rice straw in the ratio of 2:1 yielded 57% higher methane (403 ml/g VS) than the other combinations (Negi et al., 2018). Batch test of co-digestion of OFMSW with pretreated sludge and rice straw experimented at different blending ratio, and ratio 3:0.5:0.5 yields maximum biogas 558 ml/g VS (Abudi et al., 2016). Shekdar (2009) has reported the different treatment methods for improving the biogas production from OFMSW. MSW, bovine slaughterhouse, manure and crop residues were mixed and evaluated for methane production in mesophilic and thermophilic condition and mesophilic condition results showed 57% lower methane in comparison with the thermophilic condition (Pagés-Díaz et al., 2013). Methane yield from corn straw and manure get increased up to 22.4% by means of mixing another substrate i.e., fruit and vegetable waste (Wang et al., 2018).
The organic fraction of municipal waste and agro-industrial waste are mostly investigated and used for co-substrates with manure (Mata-Alvarez et al., 2014; Abudi et al., 2016). Selection of suitable co-substrates and the ratio of mixing are key factors aiming synergism to enhanced methane production. Municipal, agro-industrial and agricultural waste have been individually utilized as a source of energy through this technique. So far little work performed regarding synergistic effect from these three different sector waste or biomass (Sharholy et al., 2008; Vivekanand et al., 2018).
Pretreatment Process
Utilizing bioenergy from biomass with higher efficiency is also a major concern, for this different pretreatment techniques are applied. Lignocellulosic biomass composed of cellulose, hemicellulose, and lignin, where lignin has the amorphous 3D structure of phenylpropanoid units covering cellulose fibers hindering access from enzymes. For deconstructing lignin structure, biological, physical or chemical, or physiochemical pretreatment techniques are available (Bussemaker and Zhang, 2013; Kumar et al., 2018; Yadav et al., 2018).
Ultrasonic Pretreatment
Sound waves or energy having frequency beyond 20 kHz, inaudible to human beings, called as ultrasonic waves. These waves are responsible for producing vibration in the suspension leading the formation of cavitation bubbles of vapor. The formation of microbubbles and its growth and collapse called cavitation effect, it occurs only due to more ultrasonic energy (e.g., 1 W/cm3 for water) than molecular attractive forces. Microbubbles collapse during wave compression generating high temperature and pressure spot on the biomass disrupting the outer structure. This cavitation delivers physical effects on the substrate due to shear forces to the surface of the substrate leading its structure lysis (Nakashima et al., 2016; Rodriguez et al., 2017; Wang et al., 2017).
Depending on the ultrasonication condition, effective physical disintegration can be achieved augmenting AD yield. It was also proven to be effective and versatile in comparison with other pretreatment such as acid, base thermal and bacterial for fat-rich solid substrates especially waste coming from meat processing (Cesaro and Belgiorno, 2014). Pretreatment parameters should require to be near at room temperature and pressure, mild severity with significant delignification. Ultrasonication exclusiveness is due to its parameters ranges near to atmospheric conditions i.e., room temperature, atmospheric pressure, no chemicals required (Nakashima et al., 2016; Xiong et al., 2017). Factors influencing cavitation effect are vibration frequency, ultrasound power (or amplitude), temperature and viscosity of liquid (solvent), surface tension, and time duration (Clark and Nujjoo, 2000; Bussemaker and Zhang, 2013; Rasapoor et al., 2016).
Deepanraj et al. (2017) found that the ultrasonication (20 kHz, 130 W, 30 min) as more effective pretreatment than autoclave and microwave for biogas production from food waste. Castrillón et al. (2011) analyzed ultrasound (20 kHz, 100 W, 4 min) pretreatment and co-digestion of cattle manure with 4% glycerin both showing 121 and 400% increment, respectively on biogas production in mesophilic and thermophilic condition. Cesaro et al. (2012) sonicated mixture of organic solid waste and sewage sludge for 30 and 60 min duration at different energy densities (0.1, 0.2 and 0.4 W/ml). Twenty-four percent enhanced biogas from the sonicated mixture due to enhanced solubilization and enhanced biodegradability of organic matter enhanced during the AD. Cho et al. (2013) conducted sonication for microalgae at different power amplitude for 15 and 30 min duration without temperature control on microalgae Scenedesmus, and methane increment was around 14–75%. Effect of ultrasonic power density and sonication time was analyzed by performing ultrasonic pretreatment of OFMSW on biogas production and proving low power density and higher sonication time give better results than high power density and low sonication time due to simultaneously temperature increment with respect to time making more effective. Effect of sonication on municipal sludge has widely investigated, however, there is an inadequate study on different organic residues (Cesaro et al., 2014; Rasapoor et al., 2016; Zeynali et al., 2017).
As such this study investigates the feasibility and potential of energy production from AcoD of multi feed-stocks from different waste sectors i.e., agricultural, agro-industrial, and municipal solid waste, through BMP tests to alleviate the energy as well as waste management problem. Also exploring the ultrasonic pretreatment for organic residues from above-mentioned sectors at controlled temperature condition and to validate experimental pretreatment results with two classical kinetic models which can be used to describe and evaluate the batch BMP test for AD process.
Materials and Methods
Feed Stocks Collection and Preparation
Inoculum
Microbial inoculum used in this study was collected from the continuous Durgapura biogas plant feeding cow dung as substrate. The inoculum was incubated anaerobically at 37°C for a week to reduce endogenous biogas production. Inoculum has 7.5% TS and VS content as 59.3% of TS. The inoculum was diluted to a TS content of 1.2% with water. Furthermore, divided into 400 mL aliquots in 610 mL batch serum bottles. This diluted inoculum has pH 7.4 and conductivity 2.3 mS.
Feed-Stocks or Substrates
The organic fraction of MSW was collected from MNIT campus and two residence of Malviya Nagar, which is a source segregated waste i.e. without any contamination of non-biodegradable waste. This waste is mainly composed of leftover cooked food, vegetable peelings, dry green waste of fallen leaves, grass trimmings. With the help of household blender organic wastes were comminuted and mixed homogenously to have better solubility shown in Figure 2A, then stored at 4°C till use (Izumi et al., 2010; Negi et al., 2018).
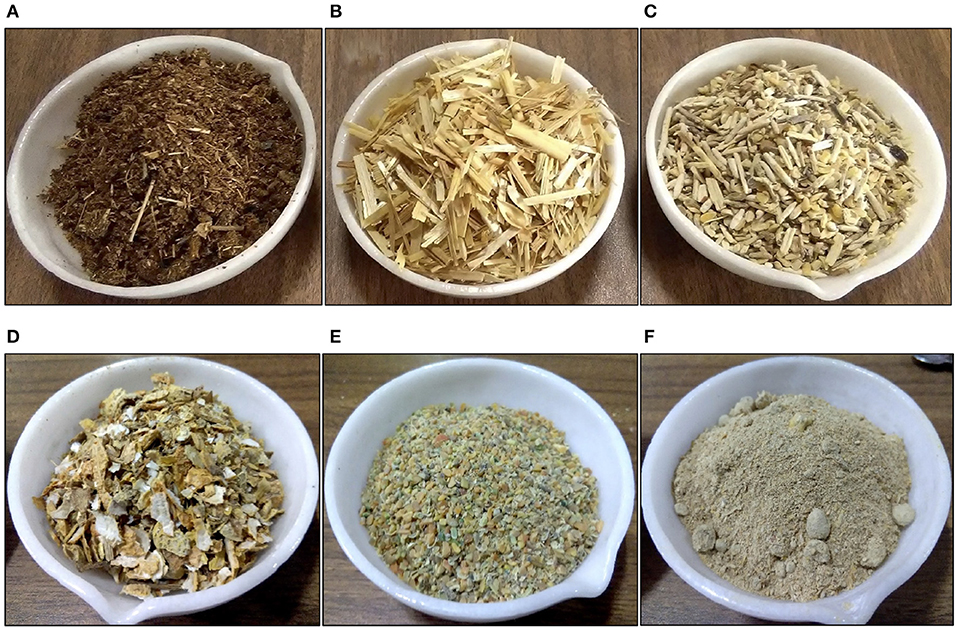
Figure 2. Substrates. (A) Organic fraction of MSW, (B) Wheat straw, (C) Mung bean husk, (D) Groundnut shell, (E) Guar husk and (F) Rice bran.
Wheat straw as an agricultural waste collected from Jaisinghpura village, Jaipur, the collected WS sample shown in Figure 2B. Agro-industrial waste: (i) Mung bean husk sample shown in Figure 2C is procured from Sunny Daal Mill industry, Sitapura, Jaipur, MBH residue from mill contains almost equal amount of broken small beans and husk, (ii) Groundnut shell waste sample (Figure 2D) collected from groundnut processing industry (Rajasthan Agro Product, Sikar Road, Jaipur), which is latter being burnt or disposed, (iii) Guar husk and (iv) Rice bran samples shown in Figures 2E, F, respectively which were collected from Unique Organic Ltd, Sitapura Industrial Area, Jaipur.
Biochemical Methane Potential Test
A BMP test for biogas production was performed in triplicates of each 6 different substrates, with control bottles i.e., negative control having inoculum alone, and positive control having cellulose as substrates. Subsequently, total 24 bottles flushed with nitrogen and closed with a rubber cap, and transferred to the shaker (REMI CIS 24, India) for incubation (37 °C, 90 rpm, 30 days) to figure out best agro-industrial substrate for co-digestion. 1.55 g substrate VS was added to diluted inoculum bottles (610 ml) having a working volume of 400 ml (Vivekanand et al., 2013; Paritosh et al., 2018).
Biogas Volume and Methane Composition
Pressure measurement in head space of each batch reactors was made periodically through Digital pressure meter (TESTO model 512, Germany, shown in Figure 3A) for biogas volume measurement. After this, biogas was released, reducing head space pressure to atmospheric pressure. The ideal gas law was used for calculating the biogas volume in the headspace volume of the batch reactors. Biogas production from inoculum control bottles is subtracted from biogas production by each substrate (Donoso-Bravo et al., 2010; Vivekanand et al., 2018).
Ten millimeters gas-tight glass syringe used for sampling of biogas for methane composition analysis. Methane composition was analyzed by Gas chromatography (Thermo SCIENTIFIC Trace 1110) equipped with Porapak column and Thermal Conductivity Detector (TCD) where helium gas was used as carrier gas (Zhen et al., 2016).
BMP Test of Mixtures
Five ratios were selected based on nutritional content (C/N ratio) of individual substrates and mixed on VS basis (Mata-Alvarez et al., 2014; Pellera and Gidarakos, 2017; Vivekanand et al., 2018). BMP test of these mixtures was performed for an incubation period of 60 days at 37°C with shaking of 90 rpm.
Ultrasonic Pretreatment
Each selected substrate suspension (10 g in 200 mL of water) in a beaker (500 mL) was sonicated (Wang et al., 2017; Xiong et al., 2017). Ultrasonic pretreatment was carried out in a probe-type sonicator (Ultrasonic Processor-sonicator EI-250UP, Electrosonic Industries, Mumbai, India) equipped with a probe of 15 mm diameter (shown in Figure 3B) operating at frequency 22 kHz and power of 250 W.
Ultrasonication pretreatment was performed by immersing probe to a half depth of suspension. Here, only pretreatment time is accounted as pretreatment variable i.e., for 30, 60, and 90 min (Nakashima et al., 2016). The temperature of suspension was maintained constant at 32 ± 1°C by recirculating cold water in the provided water bath and manual stirring is performed to have uniform pretreatment effect on substrates. Here, the project aims to investigate the influence of ultrasonic pretreatment duration without temperature effect on methane production.
Afterward, BMP test was performed to evaluate the effect of ultrasonic pretreatment on methane production from different substrates.
Analytical Studies
Proximate analysis i.e. Moisture content, Total solids (TS) and VS of the inoculum and substrates were determined by standard methods from American Public Health Association (APHA, 1999). Ultimate analysis i.e. carbon, hydrogen and nitrogen content were determined by CHNS analyser (Thermo Finnigan, FLASH EA 1112 series, Italy). pH of batch bottles was measured by a pH meter (LMPH 10, Labman Scientific Instruments Pvt. Ltd. India) in the beginning and end of the BMP test. The proximate and ultimate results of selected substrates are shown in Table 1.
Surface Morphology
Surface morphology of different pretreatment level of the respective substrate was observed by morphological change through scanning electron microscope SEM (Nova NanoSEM 450, Netherland) observation.
Crystallinity
Crystallinity index of untreated and pretreated substrates was examined through X-ray diffraction test using a XPERT-PRO X-ray diffractometer (XRD), and the samples were scanned in 2θ ranged from 5–50° with a step size of 0.02° (Liu et al., 2015; Wang et al., 2017). The decrease in CrI ascribed to disruption of crystalline structure, whereas an increase in CrI ascribed to removal of lignin and remained crystalline cellulose (Nakashima et al., 2016).
The crystallinity index (CrI) is calculated by following equation (1):
Icr = Diffraction intensity from 002 plane of crystalline part (cellulose) at 2θ = 22.6°, and Iamor = Diffraction intensity of amorphous part (cellulose, hemicellulose, and lignin) 2θ = 18° (Kumar et al., 2009; Liu et al., 2015; Wang et al., 2017).
Chemical Structure
Effect of pretreatment on different substrates can be observed by a change in chemical compositions through Spectrum 10.4.00 FTIR spectrophotometer (PerkinElmer, USA). FT-IR can be used to characterize the intrinsic biodegradability of a biomass. FT-IR spectroscopy analysis of selected samples of untreated and pretreated FW was performed in the range of 4000–400 cm−1 to characterize the effect of treatment on the functional groups and the chemical structure.
Kinetics Study
The different kinetics models have been used in previous studies to determine the methane yield by fitting the measured and predicted methane yield. Cone model and Exponential kinetic (or First order kinetic) model were chosen to fit the methane yield. Principal kinetic patterns (digestion mechanism of the organic substrate) of methane production from BMP test can be described through these models. Both models have an assumption of methane production in the batch test is proportional to methanogenic bacteria growth rate. These two models were used to compare and understand the kinetics of methane production from AD (El-Mashad, 2013; Zhen et al., 2016; Syaichurrozi, 2018).
Hydrolysis process as rate limiting step is the basis for using Exponential kinetic model describing the AD assuming no accumulation of intermediary elements (Veeken and Hamelers, 1999; Li K. et al., 2015). And, cone model gives better fit with methane production than other models reported by El-Mashad (2013), Li K. et al. (2015) and Paritosh et al. (2017). The equations of Cone model (Equation 2), and Exponential model (Equation 3) were shown below:
B = Accumulative methane yield (ml/g VS), P = Final methane yield (ml/g VS), k = hydrolysis rate constant (1/day), n = shape factor, t = digestion time (day) for t ≥0. With the help of non-linear least square fitting of accumulative methane yield, parameter “k” and “n” were estimated (Syaichurrozi, 2018).
The coefficient of determination (R2) and Root mean square error (RMSE) were used as indicators for model fitness, and to compare the accuracy of both models. R2 is an index for the goodness of fit, and RMSE gives standard deviation between measured and predicted values, the better fit model shows low RMSE (Li K. et al., 2015). RMSE can be calculated through following formula:
Where, Yp and Ym are predicted and measured methane yield (ml/g VS), respectively, and n is a number of measurements performed (El-Mashad, 2013).
Results and Discussion
BMP Test of Different Agro-Industrial Waste
The results of I-BMP test of individual substrates showing biogas production are shown in Figure 4 of different agro-industrial waste for digestion time of 30 days. Accumulated biogas production in 30 days of digestion period from raw Groundnut shell, Mung bean husk, Guar husk, and Rice bran are also defined in Table 2. Mung bean husk showed maximum potential of biogas among other agro-industrial waste (i.e., groundnut shell, guar husk, and rice bran) therefore it was selected as co-substrate with wheat straw and OFMSW for the multi feed-stock AD. Biogas production from rice bran was low due to its high protein content making unstable AD process (Favaro et al., 2017).
BMP Test of Selected Substrates and Their Mixtures
The II-BMP test of selected substrate i.e., OFMSW, WS, and MBH, and anaerobic co-digestion of five different mixing ratio shown in Table 2 of these substrates were performed for a retention time of 60 days. Accumulative biogas production in 60 days from above-mentioned substrates and mixtures are shown in Figure 5. Biogas production of OFMSW, WS, and MBH in mono-digestion mode were 397, 431, and 468 ml/g VS, respectively. AcoD having mixture M3 having a composition of OFMSW, WS, and MBH at 42, 7.5, and 50.5%, respectively ratios produced maximum biogas production in comparison with other mixing ratios.
Comparison of biogas and methane production of substrates in mono-digestion and co-digestion mode with total methane fraction are shown in Table 2. Methane produced and its total fraction in biogas from WS, OFMSW, and MBH are 225 (52%), 203 (51%), and 267 (57%) ml/g VS. WS cellulosic enzymatic hydrolysis is the slow due presence of hemicellulose and lignin can be seen from accumulative biogas production curve in Figure 5 (Zheng et al., 2018). Methane production through AcoD of mixtures were 256 (M1), 226 (M2), 275 (M3), 280 (M4), and 84 (M5) ml/g VS. Mixture M4 showed maximum methane production with 280 ml/g VS with methane fraction of 59%, whereas mixture M5 has minimum methane production 84 ml/g VS, due to the nutritional imbalance i.e., high C/N ratio of 52 which led to the accumulation of VFA inhibiting the methanogens.
The enhanced methane yield from mixtures M1-M4 showed the good synergistic effect of co-digestion. For better methane yield mixture of such substrates should have a composition of OFMSW and MBH in the range of 25–42%, and 50–70%, respectively. The synergistic effect of co-digestion can be strengthened by optimizing the above-mentioned range of specific substrate fractions. The mixture M4 methane yield showed 37, 20, and 4% higher methane yield in comparison with mono-digestion of OFMSW, WS, and MBH.
Analytical Study of Ultrasonic Pretreatment on Different Substrates
Untreated and pretreated substrates were digested anaerobically at mesophilic conditions, for which III-BMP test was performed whose results were shown in Table 2. The effect of pretreatment was evaluated by analyzing following sections of respective substrates.
Wheat Straw (WS)
Methane yield
Ultrasonication enhance biogas and methane yield due to improved solubility of organic matters. This can be validated with enhancement of accumulative biogas yield from WS are shown in Figure 6. Biogas yield due to pretreatment are similar with results from previous pretreatment studies on WS (Li Y. et al., 2015). Methane yield and methane fraction in biogas from pretreated WS samples 314 (67%), 357 (65%), and 314 (61%) ml/g VS at sonication time of 30, 60, and 90 min, respectively. Highest methane yield (357 ml/g VS) was obtained at 60 min of sonication pretreatment. The increment of 75% was observed in methane yield of ultrasonic pretreated WS at sonication period of 60 min than untreated WS. After 60 min sonication, methane yield decreases in WS, it was due to an increased particle size of samples because of reflocculation phenomena so lowered the hydrolysis rate. Re-flocculation occurred due to excess radical formation in the suspension (Rasapoor et al., 2016; Zeynali et al., 2017).
Surface morphology
The morphological changes induced by ultrasonic pretreatment on Wheat straw samples with increment in sonication time were observed by SEM images which are shown in Figures 7A–D. The untreated sample has smooth surface whereas pretreated samples showing erosion on the surfaces which can be recognized as the action of ultrasound pretreatment caused by the cavitation effect of sonication. As sonication time increases more surface erosion occurs on the outer surface of the WS samples which can be observed from Figures 7A–D. Also, images Figures 7B–D of pretreated WS samples reflecting some of the lignin cover on the surface are removed and broken resulting into the more accessible area of microfibrils for faster enzymatic hydrolysis, leading methane augmentation (Wang et al., 2016; Zheng et al., 2018).
Crystallinity
Crystallinity is a factor affecting hydrolysis significantly, its higher value represents better pretreatment effect if the purpose is to destroy amorphous fraction i.e. hemicellulose and lignin structure (Wang et al., 2017). Untreated and pretreated WS samples have similar XRD patterns shown in Figure 8, revealing significant disruption in the structure of WS samples. The crystallinity index (CrI) of pretreated WS samples calculated according to equation (1) whose values for untreated WS, WS-30, WS-60, and WS-90 are 39.9, 40.8, 38.7, and 37.4, respectively. Here, crystallinity increases from 39.9 to 40.8 after 30 min sonication showing deconstruction the lignin cover of WS samples due to cavitation effect induced by ultrasound frequency in water. Afterward, as sonication time is increases resulted in decreased crystallinity. The decrease in CrI may be ascribed as partial disruption of crystalline cellulose structure (Zheng et al., 2018). This shows pretreatment destroys concurrently lignin, hemicellulose, and cellulose structure i.e., in a non-selective manner (Xiong et al., 2017).
Chemical structure
The FT-IR spectra of untreated and pretreated WS samples are shown in Figure 9. The absorbance band of 3350–3450 cm−1 assigned to the –OH stretching vibrations, peak intensity is weakened as pretreatment increases showed cellulose content in pretreated samples is higher than untreated one (Zheng et al., 2018). In FT-IR spectroscopy, 1400–1700 cm−1 band shows the presence of C=C of aromatic rings which are found in lignin. In this spectra, the peak near 1430 and 1640 cm−1 are diminishing in pretreated samples that indicates the degradation of lignin. The peak at 1735 cm−1 which represents ester bond C = O also diminishing with pretreatment indicating degradation of ester linkages between lignin and carbohydrates corresponding to the hemicellulose (Liu et al., 2009). Apart from lignin and hemicellulose, the crystalline cellulose defined by peak 1049 cm−1 also degrades indicating reduction in crystalline cellulose part. The intensity of peak 2922 and 1375cm−1 which is related to asymmetric methylene stretching and CH2 wagging, respectively of cellulose, becomes weaker with increasing pretreatment severity (Singh et al., 2011; Raj et al., 2015). The pretreatment effect is consistent with results of XRD analysis.
Mung Bean Husk (MBH)
The effect of pretreatment was evaluated by analyzing methane yield, changes in surface morphology and chemical structure of pretreated MBH samples.
Methane yield
Enhancement in accumulative biogas yield from pretreated MBH substrate are shown in Figure 10. Methane yield and methane fraction (% of biogas) of pretreated MBH samples were 350 (68%), 382 (69%), and 379 (65%) ml/g VS at sonication time of 30, 60, and 90 min, respectively. Highest methane yield (382 ml/g VS) was obtained at 60 min of sonication. Ultrasonication pretreatment enhanced methane fraction from 57 to 69% of biogas volume. In case of MBH pretreatment, biogas volume was consistently increasing with an increment of sonication time in performed pretreatment conditions. Ultrasonic pretreated MBH has a maximum increment of methane yield up to 46% at sonication 60 min.
Surface morphology
SEM images of different pretreated MBH samples were obtained shown in Figure 11 to prove the substrate structural change caused by ultrasonication pretreatment. Before and after pretreatment SEM images show surfaces of MBH samples have significant changes can be observed. Untreated samples exhibit compact and regular surface structure, where microfibers can't be observed. After sonication pretreatment, MBH samples becomes coarse and scattered making microfibrils more porous compared to untreated MBH. Thus, pretreatment enhances surface defibrillation and coarseness as sonication time increases, confirming the positive effect in disrupting the structure of MBH samples.
Chemical structure
The FT-IR analysis demonstrates that untreated and pretreated samples of MBH have similar spectra pattern shown in Figure 12, showing the surface functional groups were significantly affected by pretreatment. The –OH bond at 3430 cm−1 represents the OH groups in cellulose whose degradation can be observed with pretreatment. As can be seen from FT-IR spectra, peak 1659 and 1530 cm−1 corresponds to C=C stretching of aromatic ring originated from lignin, were diminishing with pretreatment severity to MBH samples. Also, peak at 1247 cm−1 of ether bonds in pretreated MBH samples were almost disappearing in comparison to untreated MBH sample. This indicates ultrasonic pretreatment could disrupt or remove ether linkages between carbohydrates and lignin (Liu et al., 2009). Reduction in the peak of 1018 cm−1 of C-O stretching corresponding to hemicellulose showing degradation of hemicellulose. Also, the intensity at 2928 cm−1 of –CH aliphatic stretching represents CH2 and CH3 groups corresponding to cellulose and hemicellulose which were diminishing as sonication time increases for MBH samples. Also, peak of –CH2 wagging at wavenumber 1403 cm−1 is also weakening than the untreated samples (Smidt et al., 2002; Chandra, 2015; Li et al., 2018). FT-IR spectra results showed pretreatment affect lignin, hemicellulose, and cellulose simultaneously.
Organic Fraction of Municipal Solid Waste (OFMSW)
The effect of pretreatment was evaluated by analyzing methane yield, and changes in surface morphology of pretreated OFMSW samples.
Methane yield
Accumulated biogas yield were measured for defined sonication time to OFMSW is shown in the Figure 13. Table 2 also showing methane yield and methane fraction of pretreated OFMSW samples 291 (60%), 331 (66%), and 287 (58%) ml/g VS at sonication time of 30, 60, and 90 min, respectively with respect to 193 (50%) ml/g VS from untreated OFMSW. Highest methane yield (331 ml/g VS) was obtained at 60 min of sonication pretreatment. The increment of 71% in methane yield and methane fraction enhanced from 50 to 66% of biogas, were observed from ultrasonic pretreated OFMSW at sonication period of 60 min than untreated OFMSW. Methane yield resulted from ultrasound pretreatment of OFMSW were comparable with results of Cesaro and Belgiorno (2013). Methane yield from pretreated OFMSW samples increases with ultrasonication time due to the organic matter solubilization making the higher availability of substrate for digestion. After 60 min of ultrasonication, methane yield decreases in OFMSW, it was due to increased particle size of samples because of radical formation initiating the polymerization reaction during ultrasonic pretreatment (González-Fernández et al., 2012).
Surface morphology
SEM analysis of untreated and pretreated OFMSW samples helped to comprehend the ultrasonication effect. SEM images of OFMSW samples are shown in Figures 14A–D. Untreated OFMSW has more tight particles having composites of organic compounds having clusters of particles, whereas ultrasonicated OFMSW samples showed bigger particle cluster were rugged, and broken into smaller particles sizes, thereby augmenting surface area of pretreated samples and improving the organic matters solubility. Thus ultrasonication effect led changes in physical structure to OFMSW samples achieving better disintegration, so pretreated OFMSW samples have the more microbial accessibility of carbon for enzymatic hydrolysis than untreated one (Deepanraj et al., 2017).
Kinetic Study Results
The degradation kinetics of selected substrates were described by using Cone and Exponential kinetic models for III-BMP test. Table 3 shows the experimental methane yield (ml/g VS) from BMP-III test and predicted methane yield (ml/g VS) for respective kinetic models with relevant kinetic parameters. For both models, hydrolysis rate constant “k” increasing with pretreatment duration of respective substrate samples with few exceptions. Hydrolysis rate constant “k” reflects biodegradability of substrates. High “k” refers to the faster rate of degradation of organic substrate, enhancing methane production (Veeken and Hamelers, 1999; Zhen et al., 2015; Mao et al., 2017).
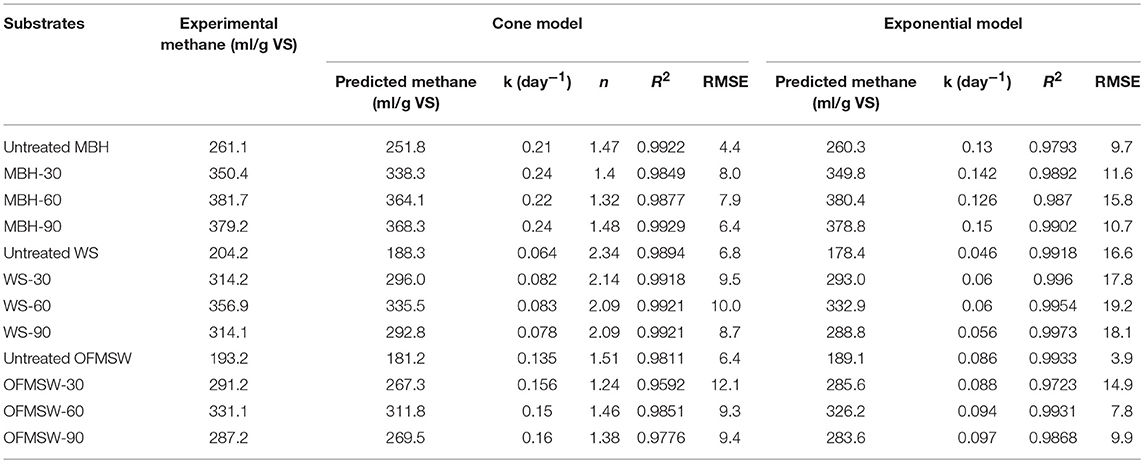
Table 3. Parameters of Cone and Exponential kinetic model obtained from untreated and pretreated samples.
The studied model results of OFMSW shows improvement in measured methane and maximum methane potential was independent of “k,” that rise in “k” doesn't convey high methane production. This inference was similar to Zhen et al. (2016) and Keymer et al. (2013). In case of cone model, “k” ranges between 0.21 and 0.24 day−1 for MBH, 0.064–0.083 day−1 for WS, and 0.135–0.16 day−1 for OFMSW, in case of exponential kinetic model “k” ranges 0.13–0.15 day−1 for MBH, 0.046–0.06 day−1 for WS, and 0.086–0.097 day−1 for OFMSW. The “k” values are comparatively lower for lignocellulosic biomass, therefore lignin content is higher in WS, than substrate MBH, and OFMSW, as it contains green waste.
The calculated RMSE and R2 values for both models are shown in Table 3, their lower values confirming the model's good agreement with experimental results. RMSE values vary in between 4.4–12.1 for Cone model and 3.9–19.2 for the Exponential model. This reflects Cone model with a lower range of RMSE, is more accurate than the Exponential kinetic model. The cone model (R2: 0.9592–0.9929) and Exponential kinetic model (R2: 0.9723–0.9973) showed a good fit to predicted and measured data of different untreated and pretreated substrates in mono-digestion. Figure 15A,B shows relation between predicted methane yield against measured values for both Cone and Exponential kinetic model through R2.
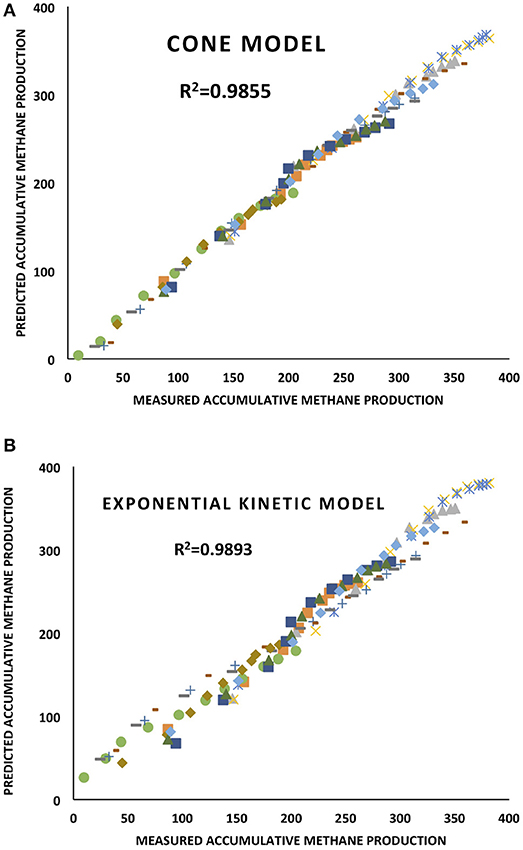
Figure 15. Predicted methane yield against measured values for (A) cone model, (B) exponential kinetic model.
Conclusion
This study shows multi-feed of wheat straw, mung bean husk, and OFMSW anaerobic co-digestion shows good synergy for enhanced methane production. Mixing of such co-substrates results into 37, 20, and 4% higher methane yield in comparison with mono-digestion of OFMSW, wheat straw and mung bean husk. The experimental results showed ultrasonic pretreatment with varying sonication time have a significant effect on methane yield from OFMSW, wheat straw, and Mung bean husk. Pretreatment significant effects on different substrates were validated through BMP test, SEM, XRD, and FTIR analysis. Ultrasonication time can be increased up to 60 min with stable temperature pretreatment constraint. Methane yield of ultrasonic pretreated wheat straw, mung bean husk and OFMSW have a maximum increment of 75, 46, and 71%, respectively at sonication period of 60 min. Two classical kinetic models i.e., Cone and Exponential were used for validating the experimental results of III-BMP test. Cone and Exponential model have an average coefficient of determination R2 = 0.9855 and R2 = 0.9893, respectively, but the range of RMSE for cone model results is lower than that of Exponential model showing Cone model is more précised.
Author Contributions
KP performed the experiments and VV conceived and designed the experiments. KP carried out the experiments and wrote the manuscript. VV and NP worked on and corrected the manuscript critically.
Conflict of Interest Statement
The authors declare that the research was conducted in the absence of any commercial or financial relationships that could be construed as a potential conflict of interest.
Acknowledgments
KP thanks MNIT for assistantship and SAIF, IIT Bombay for CHN characterization. Authors are thankful to Department of Biotechnology-GoI (Grant No. BT/RLF/Re-Entry/04/2013) and Department of Science and Technology-GoI (Grant No. ECR/2016/000989) for financial support.
References
Abdulrazak, S., Otie, D., and Oniwapele, Y. A. (2014). Proximate analysis and anti-nutritional factors of groundnut and melon husk. Online J. of Anim. Feed Res. 4, 25–28.
Abudi, Z. N., Hu, Z., Sun, N., Xiao, B., Rajaa, N., Liu, C., et al. (2016). Batch anaerobic co-digestion of OFMSW (organic fraction of municipal solid waste), TWAS (thickened waste activated sludge) and RS (rice straw): influence of TWAS and RS pretreatment and mixing ratio. Energy 107, 131–140. doi: 10.1016/j.energy.2016.03.141
Annual Report MA and FW (2017). Agricultural Statistics at a Glance 2016. Ministry of Agriculture & Farmers Welfare (Govermentment. of India).
APHA, (1999). Standard Methods for Examination of Water and Waste Water, 17th edn. American Public Health Association, Washington, DC.
Bolado-Rodríguez, S., Toquero, C., Martín-Juárez, J., Travaini, R., and García-Encina, P. A. (2016). Effect of thermal, acid, alkaline and alkaline-peroxide pretreatments on the biochemical methane potential and kinetics of the anaerobic digestion of wheat straw and sugarcane bagasse. Bioresour. Technol. 201, 182–190. doi: 10.1016/j.biortech.2015.11.047
Bundhoo, Z. M., Mauthoor, S., and Mohee, R. (2016). Potential of biogas production from biomass and waste materials in the Small Island Developing State of Mauritius. Renew. Sustainable Energy Rev. 56, 1087–1100. doi: 10.1016/j.rser.2015.12.026
Bussemaker, M. J., and Zhang, D. (2013). Effect of ultrasound on lignocellulosic biomass as a pretreatment for biorefinery and biofuel applications. Indust. Eng. Chem. Res. 52, 3563–3580. doi: 10.1021/ie3022785
Castrillón, L., Fernández-Nava, Y., Ormaechea, P., and Marañón, E. (2011). Optimization of biogas production from cattle manure by pre-treatment with ultrasound and co-digestion with crude glycerin. Bioresour. Technol. 102, 7845–7849. doi: 10.1016/j.biortech.2011.05.047
Census, (2011). Census in India. Available online at: http://www.census2011.co.in/ (Accessed November 17, 2017).
Cesaro, A., and Belgiorno, V. (2013). Sonolysis and ozonation as pretreatment for anaerobic digestion of solid organic waste. Ultrasonics Sonochem. 20, 931–936. doi: 10.1016/j.ultsonch.2012.10.017
Cesaro, A., and Belgiorno, V. (2014). Pretreatment methods to improve anaerobic biodegradability of organic municipal solid waste fractions. Chemical Eng. J. 240, 24–37. doi: 10.1016/j.cej.2013.11.055
Cesaro, A., Naddeo, V., Amodio, V., and Belgiorno, V. (2012). Enhanced biogas production from anaerobic codigestion of solid waste by sonolysis. Ultrasonics Sonochem. 19, 596–600. doi: 10.1016/j.ultsonch.2011.09.002
Cesaro, A., Velten, S., Belgiorno, V., and Kuchta, K. (2014). Enhanced anaerobic digestion by ultrasonic pretreatment of organic residues for energy production. J. Clean. Prod. 74, 119–124. doi: 10.1016/j.jclepro.2014.03.030
Chandra, R. (2015). Advances in Biodegradation and Bioremediation of Industrial Waste. Boca Raton, FL: CRC Press.
Chandra, R., Takeuchi, H., and Hasegawa, T. (2012b). Methane production from lignocellulosic agricultural crop wastes: a review in context to second generation of biofuel production. Renew. Sustainable Energy Rev. 16, 1462–1476. doi: 10.1016/j.rser.2011.11.035
Chandra, R., Takeuchi, H., Hasegawa, T., and Kumar, R. (2012a). Improving biodegradability and biogas production of wheat straw substrates using sodium hydroxide and hydrothermal pretreatments. Energy 43, 273–282. doi: 10.1016/j.energy.2012.04.029
Cho, S., Park, S., Seon, J., Yu, J., and Lee, T. (2013). Evaluation of thermal, ultrasonic and alkali pretreatments on mixed-microalgal biomass to enhance anaerobic methane production. Bioresour. Technol. 143, 330–336. doi: 10.1016/j.biortech.2013.06.017
Clark, P. B., and Nujjoo, I. (2000). Ultrasonic sludge pretreatment for enhanced sludge digestion. Water Environ. J. 14, 66–71. doi: 10.1111/j.1747-6593.2000.tb00228.x
Deepanraj, B., Sivasubramanian, V., and Jayaraj, S. (2017). Effect of substrate pretreatment on biogas production through anaerobic digestion of food waste. Int. J. of Hydrogen Energy 42, 26522–26528. doi: 10.1016/j.ijhydene.2017.06.178
Devi, S., Gupta, C., Jat, S. L., and Parmar, M. S. (2017). Crop residue recycling for economic and environmental sustainability: the case of India. Open Agricult. 2, 486–494. doi: 10.1515/opag-2017-0053
Dhar, H., Kumar, S., and Kumar, R. (2017). A review on organic waste to energy systems in India. Bioresour. Technol. 245, 1229–1237. doi: 10.1016/j.biortech.2017.08.159
Donoso-Bravo, A., Pérez-Elvira, S. I., and Fdz-Polanco, F. (2010). Application of simplified models for anaerobic biodegradability tests. Evaluation of pre-treatment processes. Chemical Eng. J. 160, 607–614. doi: 10.1016/j.cej.2010.03.082
El-Mashad, H. M. (2013). Kinetics of methane production from the codigestion of switchgrass and Spirulina platensis algae. Bioresour. Technol. 132, 305–312. doi: 10.1016/j.biortech.2012.12.183
Favaro, L., Cagnin, L., Basaglia, M., Pizzocchero, V., van Zyl, W. H., and Casella, S. (2017). Production of bioethanol from multiple waste streams of rice milling. Bioresour. Technol. 244, 151–159. doi: 10.1016/j.biortech.2017.07.108
Gollapalli, M., and Kota, S. H. (2018). Methane emissions from a landfill in north-east India: performance of various landfill gas emission models. Environ. Pollut. 234, 174–180. doi: 10.1016/j.envpol.2017.11.064
González-Fernández, C., Sialve, B., Bernet, N., and Steyer, J. P. (2012). Comparison of ultrasound and thermal pretreatment of Scenedesmus biomass on methane production. Bioresour. Technol. 110, 610–616. doi: 10.1016/j.biortech.2012.01.043
Hagos, K., Zong, J., Li, D., Liu, C., and Lu, X. (2017). Anaerobic co-digestion process for biogas production: progress, challenges and perspectives. Renew. Sustainable Energy Rev. 76, 1485–1496. doi: 10.1016/j.rser.2016.11.184
Hiloidhari, M., Das, D., and Baruah, D. C. (2014). Bioenergy potential from crop residue biomass in India. Renew. Sustainable Energy Rev. 32, 504–512. doi: 10.1016/j.rser.2014.01.025
Izumi, K., Okishio, Y. K., Nagao, N., Niwa, C., Yamamoto, S., and Toda, T. (2010). Effects of particle size on anaerobic digestion of food waste. Int. Biodet. Biodegrad. 64, 601–608. doi: 10.1016/j.ibiod.2010.06.013
Janampet, R. S., Malavath, K. K., Neeradi, R., Chedurupalli, S., and Thirunahari, R. (2016). Effect of feeding guar meal on nutrient utilization and growth performance in Mahbubnagar local kids. Vet. World 9:1043. doi: 10.14202/vetworld.2016.1043-1046
Kacprzak, A., Krzystek, L., and Ledakowicz, S. (2010). Co-digestion of agricultural and industrial wastes. Chemical Papers 64, 127–131. doi: 10.2478/s11696-009-0108-5
Kalyani, K. A., and Pandey, K. K. (2014). Waste to energy status in India: a short review. Renew. Sustainable Energy Rev. 31, 113–120. doi: 10.1016/j.rser.2013.11.020
Keymer, P., Ruffell, I., Pratt, S., and Lant, P. (2013). High pressure thermal hydrolysis as pre-treatment to increase the methane yield during anaerobic digestion of microalgae. Bioresour. Technol. 131, 128–133. doi: 10.1016/j.biortech.2012.12.125
Kumar, R., Mago, G., Balan, V., and Wyman, C. E. (2009). Physical and chemical characterizations of corn stover and poplar solids resulting from leading pretreatment technologies. Bioresour. Technol. 100, 3948–3962. doi: 10.1016/j.biortech.2009.01.075
Kumar, S., Paritosh, K., Pareek, N., Chawade, A., and Vivekanand, V. (2018). De-construction of major Indian cereal crop residues through chemical pretreatment for improved biogas production: an overview. Renew. Sustainable Energy Rev. 90, 160–170. doi: 10.1016/j.rser.2018.03.049
Li, G., Sun, Y., Guo, W., and Yuan, L. (2018). Comparison of various pretreatment strategies and their effect on chemistry and structure of sugar beet pulp. J. Clean. Prod. 181, 217–223. doi: 10.1016/j.jclepro.2018.01.259
Li, K., Liu, R., and Sun, C. (2015). Comparison of anaerobic digestion characteristics and kinetics of four livestock manures with different substrate concentrations. Bioresour. Technol. 198, 133–140. doi: 10.1016/j.biortech.2015.08.151
Li, Y., Merrettig-Bruns, U., Strauch, S., Kabasci, S., and Chen, H. (2015). Optimization of ammonia pretreatment of wheat straw for biogas production. J. Chem. Technol. Biotechnol. 90, 130–138. doi: 10.1002/jctb.4297
Liu, L., Sun, J., Li, M., Wang, S., Pei, H., and Zhang, J. (2009). Enhanced enzymatic hydrolysis and structural features of corn stover by FeCl3 pretreatment. Bioresour. Technol. 100, 5853–5858. doi: 10.1016/j.biortech.2009.06.040
Liu, X., Zicari, S. M., Liu, G., Li, Y., and Zhang, R. (2015). Pretreatment of wheat straw with potassium hydroxide for increasing enzymatic and microbial degradability. Bioresour. Technol. 185, 150–157. doi: 10.1016/j.biortech.2015.02.047
Mao, C., Zhang, T., Wang, X., Feng, Y., Ren, G., and Yang, G. (2017). Process performance and methane production optimizing of anaerobic co-digestion of swine manure and corn straw. Sci. Rep. 7, 9379. doi: 10.1038/s41598-017-09977-6
Mata-Alvarez, J., Dosta, J., Romero-Güiza, M. S., Fonoll, X., Peces, M., and Astals, S. (2014). A critical review on anaerobic co-digestion achievements between 2010 and 2013. Renew. Sust. Energy Rev. 36, 412–427. doi: 10.1016/j.rser.2014.04.039
Mboowa, D., Quereshi, S., Bhattacharjee, C., Tonny, K., and Dutta, S. (2017). Qualitative determination of energy potential and methane generation from municipal solid waste (MSW) in Dhanbad (India). Energy 123, 386–391. doi: 10.1016/j.energy,.2017.02.009
MNRE Annual Report (2017). Waste to Energy Potential in India 2017. Ministry of New and Renewable Energy. Available online at: http://mnre.gov.in/file-manager/annual-report/2016-2017/EN/pdf/1.pdf (Accessed December 15, 2017).
MSW Report (2017). Annual Review Report 2014-15. Implementation of Municipal solid wastes (Management and Handling) Rules, 2000. Central Pollution Control Board, Ministry of Environment, Forest and Climate Change, Govnerment of India.
Municipal solid waste, Central Pollution Control Board (2017). (CPCB), Ministry of Environment, Forest and Climate Change, Govnerment of India. Available online at: http://cpcb.nic.in/wast/municipalwast/trend_46_cities_list.pdf (Accessed November 17, 2017).
Nakashima, K., Ebi, Y., Kubo, M., Shibasaki-Kitakawa, N., and Yonemoto, T. (2016). Pretreatment combining ultrasound and sodium percarbonate under mild conditions for efficient degradation of corn stover. Ultrasonics Sonochem. 29, 455–460. doi: 10.1016/j.ultsonch.2015.10.017
Negi, S., Dhar, H., Hussain, A., and Kumar, S. (2018). Biomethanation potential for co-digestion of municipal solid waste and rice straw: a batch study. Bioresour. Technol. 254, 139–144. doi: 10.1016/j.biortech.2018.01.070
Nguyen, V. H., Topno, S., Balingbing, C., Nguyen, V. C. N., Röder, M., Quilty, J., et al. (2016). Generating a positive energy balance from using rice straw for anaerobic digestion. Energy Reports 2, 117–122. doi: 10.1016/j.egyr.2016.05.005
Pagés-Díaz, J., Sárvári-Horváth, I., Pérez-Olmo, J., and Pereda-Reyes, I. (2013). Co-digestion of bovine slaughterhouse wastes, cow manure, various crops and municipal solid waste at thermophilic conditions: a comparison with specific case running at mesophilic conditions. Water Sci. Technol. 67, 989–995. doi: 10.2166/wst.2013.647
Paritosh, K., Mathur, S., Pareek, N., and Vivekanand, V. (2017). Feasibility study of waste (d) potential: co-digestion of organic wastes, synergistic effect and kinetics of biogas production. Int. J. Environmental Sci. Technol. 15, 1009–1018. doi: 10.1007/s13762-017-1453-5
Paritosh, K., Yadav, M., Mathur, S., Balan, V., Liao, W., Pareek, N., et al. (2018). Organic fraction of municipal solid waste: overview of treatment methodologies to enhance anaerobic biodegradability. Front. Energy Res. 6, 75. doi: 10.3389/fenrg.2018.00075
Pellera, F. M., and Gidarakos, E. (2017). Anaerobic digestion of solid agroindustrial waste in semi-continuous mode: evaluation of mono-digestion and co-digestion systems. Waste Management 68, 103–119. doi: 10.1016/j.wasman.2017.06.026
Planning Commission Report (2014). Reports of the Task Force on Waste to Energy (Vol-I) (in the context of Integrated MSW management). Available online at: http://planningcommission.nic.in/reports/genrep/rep_wte1205.pdf (Accessed September 15, 2017).
Raj, T., Kapoor, M., Gaur, R., Christopher, J., Lamba, B., Tuli, D. K., et al. (2015). Physical and chemical characterization of various Indian agriculture residues for biofuels production. Energy Fuels 29, 3111–3118. doi: 10.1021/ef5027373
Rajasthan Agri-Statistics Report. (2017). Rajasthan Agricultural Statistics At A Glance 2015-16. Jaipur: Commissionerate of Agriculture. Available online at: http://www.agriculture.rajasthan.gov.in/content/dam/agriculture/Agriculture%20Department/ecitizen/agriculture-statistics/agriculture_statistics_2015-16.pdf
Rao, M. S., and Singh, S. P. (2004). Bioenergy conversion studies of organic fraction of MSW: kinetic studies and gas yield–organic loading relationships for process optimisation. Bioresour. Technol. 95, 173–185. doi: 10.1016/j.biortech.2004.02.013
Rasapoor, M., Ajabshirchi, Y., Adl, M., Abdi, R., and Gharibi, A. (2016). The effect of ultrasonic pretreatment on biogas generation yield from organic fraction of municipal solid waste under medium solids concentration circumstance. Energy Convers. Management 119, 444–452. doi: 10.1016/j.enconman.2016.04.066
Report RRECL (2017). Biomass Fuel Supply Study (Rajasthan) for Rajasthan Renewable Energy Corporation Limited (RRECL). ABI ENERGY Consultancy Services Pvt Ltd, Chennai.
Rodriguez, C., Alaswad, A., Benyounis, K. Y., and Olabi, A. G. (2017). Pretreatment techniques used in biogas production from grass. Renew. Sustainable Energy Rev. 68, 1193–1204. doi: 10.1016/j.rser.2016.02.022
Shah, F. A., Mahmood, Q., Rashid, N., Pervez, A., Iqbal, A., and Shah, M. M. (2015). Anaerobic digestion of water hyacinth, giant reed, maize and poultry waste for biogas generation. EC Agriculture 22, 277–284.
Sharholy, M., Ahmad, K., Mahmood, G., and Trivedi, R. C. (2008). Municipal solid waste management in Indian cities–A review. Waste Manage 28, 459–467. doi: 10.1016/j.wasman.2007.02.008
Shekdar, A. V. (2009). Sustainable solid waste management: an integrated approach for Asian countries. Waste Manage 29, 1438–1448. doi: 10.1016/j.wasman.2008.08.025
Singh, D., Zeng, J., Laskar, D. D., Deobald, L., Hiscox, W. C., and Chen, S. (2011). Investigation of wheat straw biodegradation by Phanerochaete chrysosporium. Biomass Bioenergy 35, 1030–1040. doi: 10.1016/j.biombioe.2010.11.021
Smidt, E., Lechner, P., Schwanninger, M., Haberhauer, G., and Gerzabek, M. H. (2002). Characterization of waste organic matter by FT-IR spectroscopy: application in waste science. Appl. Spectroscopy 56, 1170–1175. doi: 10.1366/000370202760295412
Syaichurrozi, I. (2018). Biogas production from co-digestion Salvinia molesta and rice straw and kinetics. Renew. Energy 115, 76–86. doi: 10.1016/j.renene.2017.08.023
Uzodinma, E. O. U., Ofoefule, A. U., Eze, J. I., and Onwuka, N. D. (2007). Biogas production from blends of agro-industrial wastes. Trends in Appl. Sci. Res. 2, 554–558. doi: 10.3923/tasr.2007.554.558
Veeken, A., and Hamelers, B. (1999). Effect of temperature on hydrolysis rates of selected biowaste components. Bioresour. Technol. 69, 249–254. doi: 10.1016/S0960-8524(98)00188-6
Vivekanand, V., Mulat, D. G., Eijsink, V. G. H., and Horn, S. J. (2018). Synergistic effects of anaerobic co-digestion of whey, manure and fish ensilage. Bioresour. Technol. 249, 35–41. doi: 10.1016/j.biortech.2017.09.169
Vivekanand, V., Olsen, E. F., Eijsink, V. G., and Horn, S. J. (2013). Effect of different steam explosion conditions on methane potential and enzymatic saccharification of birch. Bioresour. Technol. 127, 343–349. doi: 10.1016/j.biortech.2012.09.118
Wang, Q., Hu, J., Shen, F., Mei, Z., Yang, G., Zhang, Y., et al. (2016). Pretreating wheat straw by the concentrated phosphoric acid plus hydrogen peroxide (PHP): investigations on pretreatment conditions and structure changes. Bioresour. Technol. 199, 245–257. doi: 10.1016/j.biortech.2015.07.112
Wang, S., Li, F., Zhang, P., Jin, S., Tao, X., Tang, X., et al. (2017). Ultrasound assisted alkaline pretreatment to enhance enzymatic saccharification of grass clipping. Energy Convers. Manage. 149, 409–415. doi: 10.1016/j.enconman.2017.07.042
Wang, X., Li, Z., Bai, X., Zhou, X., Cheng, S., Gao, R., et al. (2018). Study on improving anaerobic co-digestion of cow manure and corn straw by fruit and vegetable waste: methane production and microbial community in CSTR process. Bioresour. Technol. 249, 290–297. doi: 10.1016/j.biortech.2017.10.038
Xiong, Z. Y., Qin, Y. H., Ma, J. Y., Yang, L., Wu, Z. K., Wang, T. L., et al. (2017). Pretreatment of rice straw by ultrasound-assisted Fenton process. Bioresour. Technol. 227, 408–411. doi: 10.1016/j.biortech.2016.12.105
Yadav, M., Paritosh, K., Chawade, A., Pareek, N., and Vivekanand, V. (2018). Genetic engineering of energy crops to reduce recalcitrance and enhance biomass digestibility. Agriculture 8, 1–15. doi: 10.3390/agriculture8060076
Yadav, P., and Samadder, S. R. (2017). A global prospective of income distribution and its effect on life cycle assessment of municipal solid waste management: a review. Environ. Sci. Pollut. Res. 24, 9123–9141. doi: 10.1007/s11356-017-8441-7
Yay, A. S. E. (2015). Application of life cycle assessment (LCA) for municipal solid waste management: a case study of Sakarya. J. Clean. Prod. 94, 284–293. doi: 10.1016/j.jclepro.2015.01.089
Yong, Z., Dong, Y., Zhang, X., and Tan, T. (2015). Anaerobic co-digestion of food waste and straw for biogas production. Renew. Energy 78, 527–530. doi: 10.1016/j.renene.2015.01.033
Zeynali, R., Khojastehpour, M., and Ebrahimi-Nik, M. (2017). Effect of ultrasonic pre-treatment on biogas yield and specific energy in anaerobic digestion of fruit and vegetable wholesale market wastes. Sustain. Environ. Res. 27, 259–264. doi: 10.1016/j.serj.2017.07.001
Zhang, Q., Hu, J., and Lee, D. J. (2016). Biogas from anaerobic digestion processes: research updates. Renew. Energy 98, 108–119. doi: 10.1016/j.renene.2016.02.029
Zhen, G., Lu, X., Kobayashi, T., Kumar, G., and Xu, K. (2016). Anaerobic co-digestion on improving methane production from mixed microalgae (Scenedesmus sp., Chlorella sp.) and food waste: kinetic modeling and synergistic impact evaluation. Chemical Eng. J. 299, 332–341. doi: 10.1016/j.cej.2016.04.118
Zhen, G., Lu, X., Kobayashi, T., Li, Y. Y., Xu, K., and Zhao, Y. (2015). Mesophilic anaerobic co-digestion of waste activated sludge and Egeria densa: performance assessment and kinetic analysis. Applied Energy 148, 78–86. doi: 10.1016/j.apenergy.2015.03.038
Keywords: agricultural waste, agro-industrial waste, organic fraction of municipal solid waste, anaerobic digestion, ultrasonic pretreatment, kinetics
Citation: Prajapati KK, Pareek N and Vivekanand V (2018) Pretreatment and Multi-Feed Anaerobic Co-digestion of Agro-Industrial Residual Biomass for Improved Biomethanation and Kinetic Analysis. Front. Energy Res. 6:111. doi: 10.3389/fenrg.2018.00111
Received: 30 June 2018; Accepted: 02 October 2018;
Published: 23 October 2018.
Edited by:
Mohammad Rehan, King Abdulaziz University, Saudi ArabiaReviewed by:
Qaisar Mahmood, COMSATS University Islamabad, PakistanSamir Jaiwantrao Deshmukh, Prof.Ram Meghe Institute of Technology & Research, India
Copyright © 2018 Prajapati, Pareek and Vivekanand. This is an open-access article distributed under the terms of the Creative Commons Attribution License (CC BY). The use, distribution or reproduction in other forums is permitted, provided the original author(s) and the copyright owner(s) are credited and that the original publication in this journal is cited, in accordance with accepted academic practice. No use, distribution or reproduction is permitted which does not comply with these terms.
*Correspondence: Vivekanand Vivekanand, vivekanand.cee@mnit.ac.in