- 1Department of Surgery, The Johns Hopkins University School of Medicine, Baltimore, MD, United States
- 2Department of Surgery, University of Virginia, Charlottesville, VA, United States
The need for distinguishing benign from malignant thyroid nodules has led to the pursuit of differentiating molecular markers. The most common molecular tests in clinical use are Afirma® Gene Expression Classifier (GEC) and Thyroseq® V2. Despite the rapidly developing field of molecular markers, several limitations exist. These challenges include the recent introduction of the histopathological diagnosis “Non-Invasive Follicular Thyroid neoplasm with Papillary-like nuclear features”, the correlation of genetic mutations within both benign and malignant pathologic diagnoses, the lack of follow-up of molecular marker negative nodules, and the cost-effectiveness of molecular markers. In this manuscript, we review the current published literature surrounding the diagnostic value of Afirma® GEC and Thyroseq® V2. Among Afirma® GEC studies, sensitivity (Se), specificity (Sp), positive predictive value (PPV), and negative predictive value (NPV) ranged from 75 to 100%, 5 to 53%, 13 to 100%, and 20 to 100%, respectively. Among Thyroseq® V2 studies, Se, Sp, PPV, and NPV ranged from 40 to 100%, 56 to 93%, 13 to 90%, and 48 to 97%, respectively. We also discuss current challenges to Afirma® GEC and Thyroseq® V2 utility and clinical application, and preview the future directions of these rapidly developing technologies.
Introduction
Thyroid nodules are common among adults over the age of 60 years, with a prevalence of 50–70% (1, 2). Moreover, the incidence of thyroid cancer in the United States has increased by 211% between 1975 and 2013 (3), due to both an improved detection of small (<2 cm) thyroid nodules by thyroid ultrasonography and a true increase in thyroid cancer incidence (4). Nevertheless, the vast majority (85–95%) of thyroid nodules are benign (5). For this reason, the ability to distinguish between benign and malignant nodules is important in order to spare patients unnecessary diagnostic surgery.
Fine needle aspiration (FNA) to facilitate this distinction first became widely practiced in the early 1980s (6), and is widely recognized as the gold standard initial diagnostic procedure in the differential diagnosis of thyroid nodules, being accurate, safe, and cost effective (7, 8). The sensitivity (Se), specificity (Sp), positive predictive value (PPV), negative predictive value (NPV), false-negative (FN) rate, and false-positive (FP) ranges of an FNA are 88.2–97.0%, 47.0–98.2%, 52.0–98.0%, 89.0–96.3%, 0.5–10.0%, and 1.0–7.0%, respectively (9).
In 2009, the Bethesda classification system for FNA reporting was introduced by the National Cancer Institute, and recently, revised, included six categories based upon cytopathological features, with an associated malignancy rate and standardized management recommendation for each category (Table 1) (10). FNA reliably establishes the diagnosis of a benign or malignant nodule in 70–80% of all cases (11) and has decreased the proportion of benign nodules unnecessarily resected from 86 to 50% (12). However, 20–30% of FNA cases have indeterminate or suspicious cytological results that include Bethesda III, IV, and V categories (12) and, of these, 6–75% are malignant on final surgical pathology (13, 14). Due to the uncertainty of malignancy in these patients, their management has been challenging, usually including a repeat FNA or a diagnostic lobectomy. For this reason, the need for distinguishing benign from malignant lesions in this subset of thyroid nodules has led to the pursuit of differentiating molecular markers.
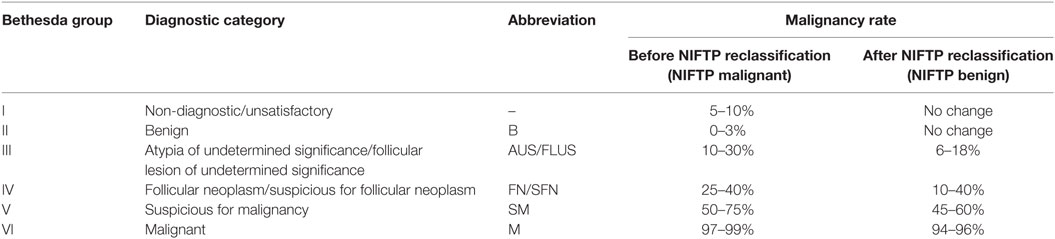
Table 1. The Bethesda system for reporting thyroid cytopathology (10).
Interest in achieving this distinction increased in 2002 with the recognition of the oncogenic role of the BRAF V600E mutation in approximately 58–69% of papillary thyroid cancers (PTC) (15, 16). However, genetic testing for BRAF V600E alone for the detection of PTCs is inadequate for clinical decision making due to its low sensitivity of 60% for PTC (17). Indeed, our group first published its use in indeterminate and suspicious thyroid lesions and found it to add minimal clinical value (18). In addition to studying the diagnostic utility of BRAF V600E, numerous studies have investigated the association of BRAF V600E and patient prognosis. However, the correlation between BRAF V600E and clinical features of PTCs has yielded inconsistent results. Some studies report that BRAF V600E is associated with a more advanced phenotype including an increased risk of lymph node metastasis, cancer recurrence, and patient mortality (19–21), while others report no such associations (22). Moreover, thyroid cancer with BRAF V600E and TERT promoter mutations has been associated with worse clinico-pathological outcomes (23, 24). BRAF testing can also be useful in deciding treatment in the setting of known metastatic thyroid cancer. Direct tyrosine kinase inhibitors, such as vemurafenib (25), dabrafenib (26), and sorafenib (27), have been shown to be effective in BRAF V600E metastatic thyroid cancers.
Since mutational analysis of single genes has not proven adequate in guiding management decisions in indeterminate or suspicious thyroid nodules, attention turned to using panels of molecular markers. Currently, the most common molecular tests in clinical use are Afirma® Gene Expression Classifier (GEC) and Thyroseq® V2 (28). Introduced in 2011 by Veracyte, the Afirma® GEC has been considered a “rule-out” malignancy test. It includes a 142-gene expression molecular assay and uses microarray technology to measure the mRNA expression profiles to determine whether a thyroid nodule is “suspicious” or “benign.” The test’s primary aim is to spare patients with cytologically indeterminate FNA samples unnecessary diagnostic surgery (29). Among indeterminate/suspicious nodules (Bethesda Type III–V), the test has both a high Se (92%) and high NPV (93%) (30) (Table 2). In contrast, it has a low Sp (52%) and PPV (47%), and cannot accurately identify malignant lesions alone.
ThyroSeq® v2, introduced in 2014 by CBL Path, is designed to identify malignant thyroid nodules by next generation sequencing (NGS), detecting 14 thyroid cancer-related genetic mutations, including RAS and BRAF mutations, 42 types of gene fusions associated with thyroid cancer, including PAX8/PPARγ and RET/PTC rearrangements, and mRNA expression levels for 16 genes; it is therefore considered a “rule-in” malignancy test (29). Among Bethesda Type III – IV nodules, the test is marketed as having a high Se, Sp, PPV, and NPV of 90–91%, 92–93%, 77–83%, and 96–97%, respectively, as well as having the ability to stratify risk based on the mutation detected (52, 53) (Table 3).
In this manuscript, we review the current published literature surrounding Afirma® GEC and Thyroseq® V2, discuss current challenges to their utility; and clinical application, and preview the future directions of these rapidly developing technologies.
Clinical Management of Indeterminate Thyroid Nodules
The 2015 American Thyroid Association (ATA) (60) and the 2016 American Association of Clinical Endocrinologists (AACE) (9) clinical guidelines recommend “considering,” molecular testing for indeterminate nodules. If molecular testing is being considered, ATA recommends that patients “should be counseled regarding the potential benefits and limitations of testing and about the possible uncertainties in the therapeutic and long-term clinical implications of results” (strong recommendation, low-quality evidence) (60). However, long-term outcome data on the use of molecular markers for therapeutic decision-making is currently unavailable. A recent report estimated that standard application of the GEC for all indeterminate thyroid nodules would result in only a 7.2% decrease in thyroidectomy volume (61). Similarly, two studies by our group showed that molecular markers did not significantly affect the surgical decision-making process, where only 7.9–8.4% of patients had altered clinical management as a result of molecular testing (39, 62). For these reasons, patient benefit from molecular marker use in routine clinical practice is likely marginal. Moreover, the AACE 2016 guidelines recommend molecular testing to complement cytologic evaluation in indeterminate nodules (Grade A recommendation), but only when the “results are expected to influence clinical management” (Grade A recommendation) (9). Testing for detection of BRAF, RET/PTC, PAX8/PPRG, and RAS mutations can be considered (Grade B recommendation). Furthermore, with the exception of BRAF V600E, there is insufficient evidence “to recommend in favor of or against the use of mutation testing as a guide to determine the extent of surgery” (Grade A recommendation) (9).
Importantly, molecular testing is not recommended in patients with an indeterminate thyroid nodule if other indications for surgery are present such as a nodule greater than 4 cm, compressive symptoms, or personal preference (63). The utility of molecular testing in Bethesda Type V nodules at institutions with a high prevalence of malignancy is low, and provides little additional benefit from a “positive” test result due to the similar PPV as that of a Bethesda Type V FNA result. Moreover, a diagnostic lobectomy would still be recommended in the case of a “negative” result. Finally, a limitation of the current molecular markers is their insufficient data to recommend use among pediatric patients (≤18 years) (64). Until these tests can be validated using this patient population, they cannot be routinely used to complement the indeterminate FNA cytology results.
Current Published Literature
Current published literature regarding Afirma® and Thyroseq® V2 validation studies are summarized in Tables 2 and 3. The data were summarized from the results of a PubMed search for English language studies that reported diagnostic accuracy in observational clinical settings published for GEC and Thyroseq® V2 up to November 30, 2017. References from the retrieved articles were also searched for additional studies. Inclusion criteria included reporting molecular marker diagnostic accuracy or enough information to calculate sensitivity, specificity, NPV, and PPV among Bethesda Type III or IV lesions. All calculations were made using the available published information. To adhere to current clinical guidelines, non-invasive follicular thyroid neoplasm with papillary-like nuclear features (NIFTP) and malignant pathologies were categorized as malignant or “requiring resection.” Of the published literature, only two studies included pediatric patients in their cohort (31, 32).
Among Afirma® GEC studies, Se, Sp, PPV, and NPV ranged from 75 to 100%, 5 to 53%, 13 to 100%, and 20 to 100%, respectively. Among Thyroseq® V2 studies, Se, Sp, PPV, and NPV ranged from 40 to 100%, 56 to 93%, 13 to 90%, and 48 to 97%, respectively. Valderrabano et al. report that the wide variation among reported diagnostic values can be explained by different defining characteristics of the study populations such as institutional prevalence of malignancy sample size, Bethesda Type included or combination thereof used, the proportions of each Bethesda Type, the definition of “benign” used in the study, and Hürthle cell (HC) predominance (65). Furthermore, among post-validation studies, the molecular test outcome itself influenced the clinical management.
Supporting this, numerous studies have reported a lower specificity or higher false positive rate in GEC tests among indeterminate nodules with HC predominance. Brauner et al. reported 86% (37/43) patients with a GEC suspicious result had unnecessary surgery (46). The authors include a grouped cohort analysis of 122 HC predominant nodules between 2012 and 2014, showing 85 of 95 (89.5%) benign pathologies identified as GEC suspicious (46). Another study by Parajuli et al. reported on GEC’s increase in false positive rate among HC predominant nodules, but did not observe the same increase in Thyroseq® V2 (66). Additional studies assessing Thyroseq®’s performance in HC predominant nodules are lacking.
One component of GEC, the Medullary Thyroid Cancer (MTC) Classifier, has been far less studied (Table 4). Among the few existing studies, Se, Sp, PPV, and NPV ranged from 91 to 100%, 100%, 98 to 100%, and 99 to 100%, respectively. Further evaluation of the MTC Classifier is needed to establish its clinical efficacy.
Current Challenges of Molecular Marker Assays
Current challenges to the application of molecular markers are fourfold: (A) the recent introduction of the histopathological diagnosis NIFTP, (B) the correlation of genetic mutations within both benign and malignant pathologic diagnoses, (C) the lack of follow-up of molecular marker negative nodules, and (D) the cost-effectiveness of molecular markers.
Non-Invasive Follicular Thyroid Neoplasm With Papillary-Like Nuclear Features
In March 2015, Nikiforov et al. introduced the new histopathological term NIFTP, previously known as encapsulated follicular variant of papillary thyroid cancer, representing an indolent entity with very low risk of recurrence (70). Major diagnostic characteristics of NIFTP include features of FVPTC, such as a follicular growth pattern and nuclear features of PTC (enlargement, crowding, elongation, irregular contours, grooves, pseudoinclusions, and chromatin clearing), but a lack of vascular or capsular invasion, key features differentiating NIFTP from FVPTC.
This new diagnosis represents a dramatic shift in thyroid pathology where an estimated 61% of lesions previously classified as FVPTCs will now be classified as NIFTP, thus decreasing the percentage of “malignancies” on final pathology compared with FNA. On pre-operative cytology, NIFTP is associated with FNA Bethesda Category III, IV, V, or VI in 15, 56, 27, and 2% of tumor samples, respectively (71). As a consequence, this has created a shift in the malignancy rate associated with each Bethesda category. Strickland et al. evaluated a cohort of 655 FNAs with subsequent resection specimens (72). When taking into account the new NIFTP diagnosis, indeterminate, and suspicious FNA samples of Bethesda III, IV, and V had an absolute decrease in rate of malignancy by 17.6, 8.0, and 41.5%, respectively (72). Similarly, Faquin et al. reported an absolute decrease in rate of malignancy in Bethesda III, IV, and V by 13.6, 15.1, and 23.4%, respectively (73).
Despite NIFTP’s extremely low-recurrence rate of 0.6% (two cases), there remains disagreement regarding NIFTP’s true malignant potential (74–80). Despite its likely benign and, at worst, indolent nature, current ATA guidelines recommend lobectomy as definitive therapy for NIFTP. More importantly, however, and, apropos of this review, Afirma® GEC and Thyroseq® V2 validation studies occurred before the establishment of NIFTP as a distinct entity. Because of this one needs to be circumspect about the real utility of these marker panels. And, as a consequence, these molecular diagnostic panels require recalibration to appropriately account for the newly introduced entity, NIFTP; a lesion that should likely not be considered malignant (70, 81, 82).
Correlating Mutations to Pathology
The correlation between presence of mutations and malignancy is imprecise. Among 967 Bethesda Type III, IV, and V nodules, the detection of any mutation conferred the risk of histologic malignancy of 88, 87, and 95%, respectively (83). However, even in nodules with no detected mutations, the malignancy rates were 6, 14, and 28%, respectively. A systematic review by our group included 8,162 patients, of whom 42.5% had benign lesions (84). Among the benign lesions, RAS mutations, RET/PTC rearrangements, and PAX8/PPAR-gamma rearrangements were present up to 48, 68, 55% of the time, respectively. Thus, benign nodules frequently harbor mutations, while some malignant lesions harbor no detected mutations. The combination of the variable and potentially high level of mutations among benign nodules may explain the low specificity and PPV seen in Afirma. Furthermore, their prominence in benign lesions may also challenge the reported PPV of Thyroseq V2.
This issue is further complicated when an indolent tumor, such as NIFTP, should be resected according to current ATA guidelines. NIFTP is commonly associated with RAS mutations (8/27, 29.6%) and its diagnosis is incompatible with the presence of BRAF V600E mutations (70, 79). Moreover, Nikiforov et al. describe that 22% (6/27) of NIFTP samples harbor no detectable mutations. To conform to the recommendation that this indolent lesion be resected, new validation studies must show the reliable identification of NIFTP by molecular markers, an unlikely occurrence given the fact that benign lesions also harbor them.
Molecular Marker Negative Nodule Follow-Up
Despite a number of studies exploring the diagnostic value of GEC and Thyroseq® V2, the current published literature includes discrepancies in the follow-up of molecular marker negative nodules and their consideration as a benign pathology (85, 86). Consequently, this may lead to inaccuracies in diagnostic value calculation. A systematic review by Duh et al. (85) highlighted these issues. They included 12 studies and discussed the exclusion of cytologically indeterminate, GEC benign nodules from diagnostic performance calculations (malignant versus benign), leading to an erroneous decrease in Sp and NPV. This is due to the lack of surgical pathology specimens to establish a definitive diagnosis, as well as a lack of follow-up of GEC benign nodules to establish a reference diagnosis. To establish a diagnostic “reference standard” in these nodules that have not undergone surgery and include them in calculations, the authors argue that they should be considered as “true negative” only if no suspicious changes are noted on scheduled interval ultrasound examinations. However, even the natural history of benign thyroid nodules has been described to involve size changes. Indeed, a 5-year prospective study involving 1,567 sonographically or cytologically benign thyroid nodules showed nodule growth in 11.1% (87). However, thyroid cancer was diagnosed in five original nodules (0.3%), of which, only two had an increase in size. Furthermore, a retrospective study ranging from 1 month to 5 years, reported that 39% of the 268 benign thyroid nodules showed at least a 15% change in nodule volume (88). Only one of the 74 repeat-FNAs was malignant. The authors conclude that an increase in nodule volume alone is not a reliable predictor of malignancy.
Two studies have described their experience with follow-up of GEC benign nodules on ultrasound. A study by Angel et al. including 56 patients with cytologically indeterminate, GEC benign nodules followed for a median of 13 months exhibited similar growth (≥20% in two dimensions or ≥50% in volume) to cytologically benign nodules (86). Furthermore, in a grouped cohort analysis by Kloos et al. of 443 GEC benign patients in six studies with a reported follow-up time of 7–26 months, 380 patients (85.8%) were spared unnecessary surgery (89). Clearly, the currently available follow-up periods are inadequate for a definitive assessment, and larger, prospective studies are needed to further evaluate the behavior of cytologically indeterminate, molecular marker “negative” thyroid nodules to help guide recommendations for management.
Cost-Effectiveness
The cost-effectiveness of GEC and Thyroseq® has also been an intense area of research. The cost for GEC and MTC is $4,875 while the cost for Afirma® MTC alone is $975 and that of Afirma® BRAF is $475 (Table 5). The cost of Thyroseq® V2 is $3200 (29). Despite these high costs, insured patient costs are capped at $300 for either GEC or ThyroSeq® V2. Numerous studies have reported on the cost-effectiveness of both GEC (90, 91) and Thyroseq® V2 (92).
A 5-year cost effectiveness study of routine use of GEC reported 74% fewer operations for benign nodules with no increase in untreated cancers. Compared with standard clinical management based only on indeterminate FNA results, GEC may lower overall costs (standard cost $12,172 versus GEC cost $10,719) and improve quality of life for patients (91). Another study reported that to be cost effective, GEC’s specificity would have to be greater than 68% and decrease the number of unnecessary surgeries performed on benign nodules by more 50% (90). However, a study by Yip et al. compared the average cost per patient with Bethesda IV nodules larger than 1 cm extending 10 years from follicular neoplasm diagnosis in three groups: standard of care, GEC, and Thyroseq®. The authors reported a 13% increase in average cost per patient when using GEC at $13,027 (range $12,373–$13,666) when compared with the standard of care $11,505 (range $10,676–$12,347), but a 30% reduction in those using Thyroseq® cost $7,683 (range $7,174–$8,333) (92).
Despite the conflicting results of GEC cost-effectiveness studies and the paucity of analyses on Thyroseq®, these studies highlight the need to closely examine cost-effectiveness with the use of genetic studies. However, the true impact of thyroid molecular tests on a population’s health care costs can only be determined taking cancer prevalence into account. Furthermore, cost-effectiveness also depends on proper education to ensure that these tests are only used in indicated clinical settings.
Conclusion and Future Direction
Molecular markers are a rapidly developing field despite the current limitations. Veracyte and CBL Path have begun to address the challenges discussed here. In 2017, Veracyte launched the Afirma® Genomic Sequencing Classifier (GSC), its newest product which tests a total of 10,196 genes. The Afirma® GSC test system is composed of a series of classifiers including parathyroid (mRNA expression), MTC (mRNA expression), BRAF (mRNA expression + variants), and RET/PTC fusion (fusion transcripts). GSC also includes a follicular content index (mRNA expression), HC index (mRNA expression and mitochondrial transcripts), and Hürthle neoplasm index (mRNA expression and chromosomal level loss of heterozygosity). The reliance on mRNA could prevent detection of mutations undetectable in transcriptome-based assays, such as telomerase reverse transcriptase (hTERT) promoter mutations. Veracyte states that GSC addresses the weaknesses of GEC by significantly increasing molecular test specificity, using a validation cohort with 15 NIFTP samples, and improving the diagnostic performance among HC lesions. Among Bethesda Type III and IV nodules, Veracyte quotes a Se, Sp, NPV, and PPV of 91, 68, 96, and 50%, respectively, when compared with the GEC parameters of 89 50, 93, and 46%, respectively. Among HC lesions, Sp has increased from 11.8 to 58.8%.
Similarly, in 2017 CBL Path sought to address similar issues with the release of Thyroseq® V3 (93). It has an expanded its assay from 56 to 112 genes, detecting mutations, gene fusions, gene expression alterations, and copy number variations. In a prospective double-blind multicenter study using 257 nodules with Bethesda Types III–V (including 11 NIFTP samples, 10 HC carcinomas, 34 HC adenomas, and 5 hyperplastic nodules with HC predominance), Thyroseq® V3 is reported to have an increased diagnostic value, including a Se of 98% (from 96.9%) and specificity of 81.8% (from 74.0%). Moreover, HC samples had an Se and Sp of 100.0 and 66.7%, respectively.
As experience accumulates with these next generation tests, we will gain a better understanding of how well they mitigate the limitations and challenges addressed herein. As our understanding of genetic drivers of malignant cancers and our understanding of NIFTP’s malignant potential becomes clearer, molecular markers will continue to more accurately identify malignant nodules as well as to spare patients from unnecessary surgery.
Author Contributions
ZS conducted the literature review. ZS, PS, and CU drafted and revised the manuscript. MZ drafted and revised the manuscript and, as the senior and corresponding author, takes full responsibility.
Conflict of Interest Statement
The authors declare that the research was conducted in the absence of any commercial or financial relationships that could be construed as a potential conflict of interest.
References
1. Gharib H. Changing trends in thyroid practice: understanding nodular thyroid disease. Endocr Pract (2004) 10(1):31–9. doi:10.4158/EP.10.1.31
2. Guth S, Theune U, Aberle J, Galach A, Bamberger C. Very high prevalence of thyroid nodules detected by high frequency (13 MHz) ultrasound examination. Eur J Clin Invest (2009) 39(8):699–706. doi:10.1111/j.1365-2362.2009.02162.x
3. Howlader N, Noone A, Krapcho M, Miller D, Bishop K, Altekruse S, et al. SEER Cancer Statistics Review, 1975–2013. Bethesda, MD: National Cancer Institute (2016).
4. Hahn SY, Shin JH, Lim HK, Jung SL, Oh YL, Choi IH, et al. Preoperative differentiation between noninvasive follicular thyroid neoplasm with papillary-like nuclear features (NIFTP) and non-NIFTP. Clin Endocrinol (2017) 86(3):444–50. doi:10.1111/cen.13263
5. Brito JP, Yarur AJ, Prokop LJ, McIver B, Murad MH, Montori VM. Prevalence of thyroid cancer in multinodular goiter versus single nodule: a systematic review and meta-analysis. Thyroid (2013) 23(4):449–55. doi:10.1089/thy.2012.0156
6. Nguyen G-K, Lee MW, Ginsberg J, Wragg T, Bilodeau D. Fine-needle aspiration of the thyroid: an overview. Cytojournal (2005) 2(1):12. doi:10.1186/1742-6413-2-12
7. Werga P, Wallin G, Skoog L, Hamberger B. Expanding role of fine-needle aspiration cytology in thyroid diagnosis and management. World J Surg (2000) 24(8):907–12. doi:10.1007/s002680010163
8. Gharib H, Goellner J, Johnson D. Fine-needle aspiration cytology of the thyroid. A 12-year experience with 11,000 biopsies. Clin Lab Med (1993) 13(3):699–709.
9. Gharib H, Papini E, Garber J, Duick D, Harrell R, Hegedüs L, et al. American Association of Clinical Endocrinologists, American College of Endocrinology, and Associazione Medici Endocrinologi Medical guidelines for clinical practice for the diagnosis and management of thyroid nodules—2016 update. Endocr Pract (2016) 22(5):622–39. doi:10.4158/EP161208.GL
10. Cibas ES, Ali SZ. The 2017 Bethesda system for reporting thyroid cytopathology. J Am Soc Cytopathol (2017) 6(6):217–22. doi:10.1016/j.jasc.2017.09.002
11. Bongiovanni M, Spitale A, Faquin WC, Mazzucchelli L, Baloch ZW. The Bethesda system for reporting thyroid cytopathology: a meta-analysis. Acta Cytol (2012) 56(4):333–9. doi:10.1159/000339959
12. Cibas ES, Ali SZ. The Bethesda system for reporting thyroid cytopathology. Am J Clin Pathol (2009) 132(5):658–65. doi:10.1309/AJCPPHLWMI3JV4LA
13. Baloch ZW, LiVolsi VA, Asa SL, Rosai J, Merino MJ, Randolph G, et al. Diagnostic terminology and morphologic criteria for cytologic diagnosis of thyroid lesions: a synopsis of the National Cancer Institute thyroid fine-needle aspiration state of the science conference. Diagn Cytopathol (2008) 36(6):425–37. doi:10.1002/dc.20830
14. Mazzaferri EL. Management of a solitary thyroid nodule. N Engl J Med (1993) 328(8):553–9. doi:10.1056/NEJM199302253280807
15. Cancer Genome Atlas Research Network. Integrated genomic characterization of papillary thyroid carcinoma. Cell (2014) 159(3):676–90. doi:10.1016/j.cell.2014.09.050
16. Cohen Y, Xing M, Mambo E, Guo Z, Wu G, Trink B, et al. BRAF mutation in papillary thyroid carcinoma. J Natl Cancer Inst (2003) 95(8):625–7. doi:10.1093/jnci/95.8.625
17. Pusztaszeri MP, Krane JF, Faquin WC. BRAF testing and thyroid FNA. Cancer Cytopathol (2015) 123(12):689–95. doi:10.1002/cncy.21614
18. Cohen Y, Rosenbaum E, Clark DP, Zeiger MA, Umbricht CB, Tufano RP, et al. Mutational analysis of BRAF in fine needle aspiration biopsies of the thyroid: a potential application for the preoperative assessment of thyroid nodules. Clin Cancer Res (2004) 10(8):2761–5. doi:10.1158/1078-0432.CCR-03-0273
19. Xing M, Alzahrani AS, Carson KA, Viola D, Elisei R, Bendlova B, et al. Association between BRAF V600E mutation and mortality in patients with papillary thyroid cancer. JAMA (2013) 309(14):1493–501. doi:10.1001/jama.2013.3190
20. Kebebew E, Weng J, Bauer J, Ranvier G, Clark OH, Duh Q-Y, et al. The prevalence and prognostic value of BRAF mutation in thyroid cancer. Ann Surg (2007) 246(3):466. doi:10.1097/SLA.0b013e318148563d
21. Xing M, Westra WH, Tufano RP, Cohen Y, Rosenbaum E, Rhoden KJ, et al. BRAF mutation predicts a poorer clinical prognosis for papillary thyroid cancer. J Clin Endocrinol Metab (2005) 90(12):6373–9. doi:10.1210/jc.2005-0987
22. Fugazzola L, Puxeddu E, Avenia N, Romei C, Cirello V, Cavaliere A, et al. Correlation between B-RAFV600E mutation and clinico-pathologic parameters in papillary thyroid carcinoma: data from a multicentric Italian study and review of the literature. Endocr Relat Cancer (2006) 13(2):455–64. doi:10.1677/erc.1.01086
23. Liu R, Bishop J, Zhu G, Zhang T, Ladenson PW, Xing M. Mortality risk stratification by combining BRAF V600E and TERT promoter mutations in papillary thyroid cancer: genetic duet of BRAF and TERT promoter mutations in thyroid cancer mortality. JAMA Oncol (2017) 3(2):202–8. doi:10.1001/jamaoncol.2016.3288
24. Liu X, Qu S, Liu R, Sheng C, Shi X, Zhu G, et al. TERT promoter mutations and their association with BRAF V600E mutation and aggressive clinicopathological characteristics of thyroid cancer. J Clin Endocrinol Metab (2014) 99(6):E1130–6. doi:10.1210/jc.2013-4048
25. Kim KB, Cabanillas ME, Lazar AJ, Williams MD, Sanders DL, Ilagan JL, et al. Clinical responses to vemurafenib in patients with metastatic papillary thyroid cancer harboring BRAFV600E mutation. Thyroid (2013) 23(10):1277–83. doi:10.1089/thy.2013.0057
26. Falchook GS, Millward M, Hong D, Naing A, Piha-Paul S, Waguespack SG, et al. BRAF inhibitor dabrafenib in patients with metastatic BRAF-mutant thyroid cancer. Thyroid (2015) 25(1):71–7. doi:10.1089/thy.2014.0123
27. Brose MS, Nutting CM, Jarzab B, Elisei R, Siena S, Bastholt L, et al. Sorafenib in radioactive iodine-refractory, locally advanced or metastatic differentiated thyroid cancer: a randomised, double-blind, phase 3 trial. Lancet (2014) 384(9940):319–28. doi:10.1016/S0140-6736(14)60421-9
28. Zhang M, Lin O. Molecular testing of thyroid nodules: a review of current available tests for fine-needle aspiration specimens. Arch Pathol Lab Med (2016) 140(12):1338–44. doi:10.5858/arpa.2016-0100-RA
29. Nishino M. Molecular cytopathology for thyroid nodules: a review of methodology and test performance. Cancer Cytopathol (2016) 124(1):14–27. doi:10.1002/cncy.21612
30. Alexander EK, Kennedy GC, Baloch ZW, Cibas ES, Chudova D, Diggans J, et al. Preoperative diagnosis of benign thyroid nodules with indeterminate cytology. N Engl J Med (2012) 367(8):705–15. doi:10.1056/NEJMoa1203208
31. Hang J-F, Westra WH, Cooper DS, Ali SZ. The impact of noninvasive follicular 1 thyroid neoplasm with papillary-like nuclear features on the performance of Afirma gene expression classifier. Cancer Cytopathol (2017) 125(9):683–91. doi:10.1002/cncy.21879
32. Harrison G, Sosa JA, Jiang X. Evaluation of the Afirma gene expression classifier in repeat indeterminate thyroid nodules. Arch Pathol Lab Med (2017) 141(7):985–9. doi:10.5858/arpa.2016-0328-OA
33. Kay-Rivest E, Tibbo J, Bouhabel S, Tamilia M, Leboeuf R, Forest V-I, et al. The first Canadian experience with the Afirma® gene expression classifier test. J Otolaryngol Head Neck Surg (2017) 46(1):25. doi:10.1186/s40463-017-0201-7
34. Samulski TD, LiVolsi VA, Wong LQ, Baloch Z. Usage trends and performance characteristics of a “gene expression classifier” in the management of thyroid nodules: an institutional experience. Diagn Cytopathol (2016) 44(11):867–73. doi:10.1002/dc.23559
35. Wu JX, Young S, Hung ML, Li N, Yang SE, Cheung DS, et al. Clinical factors influencing the performance of gene expression classifier testing in indeterminate thyroid nodules. Thyroid (2016) 26(7):916–22. doi:10.1089/thy.2015.0505
36. Yang SE, Sullivan PS, Zhang J, Govind R, Levin MR, Rao JY, et al. Has Afirma gene expression classifier testing refined the indeterminate thyroid category in cytology? Cancer Cytopathol (2016) 124(2):100–9. doi:10.1002/cncy.21624
37. Chaudhary S, Hou Y, Shen R, Hooda S, Li Z. Impact of the Afirma gene expression classifier result on the surgical management of thyroid nodules with category III/IV cytology and its correlation with surgical outcome. Acta Cytol (2016) 60(3):205–10. doi:10.1159/000446797
38. Abeykoon JP, Mueller L, Dong F, Chintakuntlawar AV, Paludo J, Mortada R. The effect of implementing gene expression classifier on outcomes of thyroid nodules with indeterminate cytology. Horm Cancer (2016) 7(4):272–8. doi:10.1007/s12672-016-0263-4
39. Noureldine SI, Najafian A, Han PA, Olson MT, Genther DJ, Schneider EB, et al. Evaluation of the effect of diagnostic molecular testing on the surgical decision-making process for patients with thyroid nodules. JAMA Otolaryngol Head Neck Surg (2016) 142(7):676–82. doi:10.1001/jamaoto.2016.0850
40. Al-Qurayshi Z, Deniwar A, Thethi T, Mallik T, Srivastav S, Murad F, et al. Association of malignancy prevalence with test properties and performance of the gene expression classifier in indeterminate thyroid nodules. JAMA Otolaryngol Head Neck Surg (2016) 143(4):403–8. doi:10.1001/jamaoto.2016.3526
41. Witt RL. Outcome of thyroid gene expression classifier testing in clinical practice. Laryngoscope (2016) 126(2):524–7. doi:10.1002/lary.25607
42. Wong KS, Angell TE, Strickland KC, Alexander EK, Cibas ES, Krane JF, et al. Noninvasive follicular variant of papillary thyroid carcinoma and the Afirma gene-expression classifier. Thyroid (2016) 26(7):911–5. doi:10.1089/thy.2015.0644
43. Zhu Q-L, Faquin WC, Samir AE. Relationship between sonographic characteristics and Afirma gene expression classifier results in thyroid nodules with indeterminate fine-needle aspiration cytopathology. AJR Am J Roentgenol (2015) 205(4):861–5. doi:10.2214/AJR.14.13984
44. Celik B, Whetsell CR, Nassar A. Afirma GEC and thyroid lesions: an institutional experience. Diagn Cytopathol (2015) 43(12):966–70. doi:10.1002/dc.23378
45. Marti JL, Avadhani V, Donatelli LA, Niyogi S, Wang B, Wong RJ, et al. Wide inter-institutional variation in performance of a molecular classifier for indeterminate thyroid nodules. Ann Surg Oncol (2015) 22(12):3996–4001. doi:10.1245/s10434-015-4486-3
46. Brauner E, Holmes BJ, Krane JF, Nishino M, Zurakowski D, Hennessey JV, et al. Performance of the Afirma gene expression classifier in Hürthle cell thyroid nodules differs from other indeterminate thyroid nodules. Thyroid (2015) 25(7):789–96. doi:10.1089/thy.2015.0049
47. McIver B, Castro MR, Morris JC, Bernet V, Smallridge R, Henry M, et al. An independent study of a gene expression classifier (Afirma) in the evaluation of cytologically indeterminate thyroid nodules. J Clin Endocrinol Metab (2014) 99(11):4069–77. doi:10.1210/jc.2013-3584
48. Lastra RR, Pramick MR, Crammer CJ, LiVolsi VA, Baloch ZW. Implications of a suspicious Afirma test result in thyroid fine-needle aspiration cytology: an institutional experience. Cancer Cytopathol (2014) 122(10):737–44. doi:10.1002/cncy.21455
49. Han PA, Olson MT, Fazeli R, Prescott JD, Pai SI, Schneider EB, et al. The impact of molecular testing on the surgical management of patients with thyroid nodules. Ann Surg Oncol (2014) 21(6):1862–9. doi:10.1245/s10434-014-3508-x
50. Alexander EK, Schorr M, Klopper J, Kim C, Sipos J, Nabhan F, et al. Multicenter clinical experience with the Afirma gene expression classifier. J Clin Endocrinol Metab (2013) 99(1):119–25. doi:10.1210/jc.2013-2482
51. Harrell R, Bimston D. Surgical utility of Afirma: effects of high cancer prevalence and oncocytic cell types in patients with indeterminate thyroid cytology. Endocr Pract (2013) 20(4):364–9. doi:10.4158/EP13330.OR
52. Nikiforov YE, Carty SE, Chiosea SI, Coyne C, Duvvuri U, Ferris RL, et al. Highly accurate diagnosis of cancer in thyroid nodules with follicular neoplasm/suspicious for a follicular neoplasm cytology by ThyroSeq v2 next-generation sequencing assay. Cancer (2014) 120(23):3627–34. doi:10.1002/cncr.29038
53. Nikiforov YE, Carty SE, Chiosea SI, Coyne C, Duvvuri U, Ferris RL, et al. Impact of the multi-gene ThyroSeq next-generation sequencing assay on cancer diagnosis in thyroid nodules with atypia of undetermined significance/follicular lesion of undetermined significance cytology. Thyroid (2015) 25(11):1217–23. doi:10.1089/thy.2015.0305
54. Taye A, Gurciullo D, Miles BA, Gupta A, Owen RP, Inabnet WB, et al. Clinical performance of a next-generation sequencing assay (ThyroSeq v2) in the evaluation of indeterminate thyroid nodules. Surgery (2018) 163(1):97–103. doi:10.1016/j.surg.2017.07.032
55. Valderrabano P, Khazai L, Leon ME, Thompson ZJ, Ma Z, Chung CH, et al. Evaluation of ThyroSeq v2 performance in thyroid nodules with indeterminate cytology. Endocr Relat Cancer (2017) 24(3):127–36. doi:10.1530/ERC-16-0512
56. Shrestha RT, Evasovich MR, Amin K, Radulescu A, Sanghvi TS, Nelson AC, et al. Correlation between histological diagnosis and mutational panel testing of thyroid nodules: a two-year institutional experience. Thyroid (2016) 26(8):1068–76. doi:10.1089/thy.2016.0048
57. Khatami R, Heimann A, Hwang S, Shroyer K, Wu M. ThyroSeq V2 application study for indeterminate thyroid FNAs with surgical follow-up; experience at a university hospital. Am J Clin Pathol (2016) 5(5):S80–1. doi:10.1093/ajcp/aqw165.018
58. Tolerado G, Godley FA, Cerda SR, Mcaneny D, Doherty GM, Braverman L, et al. Large Independent Prospective Study to Evaluate the Performance of ThyroSeq2 Multigene Next Generation Sequencing Panel Analysis on Cancer Diagnosis in Thyroid Nodules with Indeterminate Cytopathology. ThyroSeq Press Release: ThyroSeq (2016). Report No.: 1050–7256.
59. Shrestha RT, Evasovich MR, Shahi M, Radulescu AI, Amin K, Burmeister L. Surgical outcomes of Thyroseq® markers at the university of Minnesota. Endocrine Society. Boston (2016).
60. Haugen BR, Alexander EK, Bible KC, Doherty GM, Mandel SJ, Nikiforov YE, et al. 2015 American Thyroid Association management guidelines for adult patients with thyroid nodules and differentiated thyroid cancer: the American Thyroid Association guidelines task force on thyroid nodules and differentiated thyroid cancer. Thyroid (2016) 26(1):1–133. doi:10.1089/thy.2015.0020
61. Dedhia PH, Rubio GA, Cohen MS, Miller BS, Gauger PG, Hughes DT. Potential effects of molecular testing of indeterminate thyroid nodule fine needle aspiration biopsy on thyroidectomy volume. World J Surg (2014) 38(3):634–8. doi:10.1007/s00268-013-2430-x
62. Noureldine SI, Olson MT, Agrawal N, Prescott JD, Zeiger MA, Tufano RP. Effect of gene expression classifier molecular testing on the surgical decision-making process for patients with thyroid nodules. JAMA Otolaryngol Head Neck Surg (2015) 141(12):1082–8. doi:10.1001/jamaoto.2015.2708
63. Ferris RL, Baloch Z, Bernet V, Chen A, Fahey TJ III, Ganly I, et al. American thyroid association statement on surgical application of molecular profiling for thyroid nodules: current impact on perioperative decision making. Thyroid (2015) 25(7):760–8. doi:10.1089/thy.2014.0502
64. Francis GL, Waguespack SG, Bauer AJ, Angelos P, Benvenga S, Cerutti JM, et al. Management guidelines for children with thyroid nodules and differentiated thyroid cancer: the American Thyroid Association guidelines task force on pediatric thyroid cancer. Thyroid (2015) 25(7):716–59. doi:10.1089/thy.2014.0460
65. Valderrabano P, Leon ME, Centeno BA, Otto KJ, Khazai L, McCaffrey JC, et al. Institutional prevalence of malignancy of indeterminate thyroid cytology is necessary but insufficient to accurately interpret molecular marker tests. Eur J Endocrinol (2016) 174(5):621–9. doi:10.1530/EJE-15-1163
66. Parajuli S, Jug R, Ahmadi S, Jiang X. Hurthle cell predominance impacts results of GEC and molecular panel performance in indeterminate thyroid nodules. J Am Soc Cytopathol (2017) 6(5):S70–1. doi:10.1016/j.jasc.2017.06.175
67. Pankratz DG, Hu Z, Kim SY, Monroe RJ, Wong MG, Traweek ST, et al. Analytical performance of a gene expression classifier for medullary thyroid carcinoma. Thyroid (2016) 26(11):1573–80. doi:10.1089/thy.2016.0262
68. Kloos RT, Monroe RJ, Traweek ST, Lanman RB, Kennedy GC. A genomic alternative to identify medullary thyroid cancer preoperatively in thyroid nodules with indeterminate cytology. Thyroid (2016) 26(6):785–93. doi:10.1089/thy.2016.0001
69. Chudova D, Wilde JI, Wang ET, Wang H, Rabbee N, Egidio CM, et al. Molecular classification of thyroid nodules using high-dimensionality genomic data. J Clin Endocrinol Metab (2010) 95(12):5296–304. doi:10.1210/jc.2010-1087
70. Nikiforov YE, Seethala RR, Tallini G, Baloch ZW, Basolo F, Thompson LD, et al. Nomenclature revision for encapsulated follicular variant of papillary thyroid carcinoma: a paradigm shift to reduce overtreatment of indolent tumors. JAMA Oncol (2016) 2(8):1023–9. doi:10.1001/jamaoncol.2016.0386
71. Maletta F, Massa F, Torregrossa L, Duregon E, Casadei GP, Basolo F, et al. Cytological features of “noninvasive follicular thyroid neoplasm with papillary-like nuclear features” and their correlation with tumor histology. Hum Pathol (2016) 54:134–42. doi:10.1016/j.humpath.2016.03.014
72. Strickland KC, Howitt BE, Marqusee E, Alexander EK, Cibas ES, Krane JF, et al. The impact of noninvasive follicular variant of papillary thyroid carcinoma on rates of malignancy for fine-needle aspiration diagnostic categories. Thyroid (2015) 25(9):987–92. doi:10.1089/thy.2014.0612
73. Faquin WC, Wong LQ, Afrogheh AH, Ali SZ, Bishop JA, Bongiovanni M, et al. Impact of reclassifying noninvasive follicular variant of papillary thyroid carcinoma on the risk of malignancy in the Bethesda System for Reporting Thyroid Cytopathology. Cancer Cytopathol (2015) 124(3):181–7. doi:10.1002/cncy.21631
74. Piana S, Frasoldati A, Di Felice E, Gardini G, Tallini G, Rosai J. Encapsulated well-differentiated follicular-patterned thyroid carcinomas do not play a significant role in the fatality rates from thyroid carcinoma. Am J Surg Pathol (2010) 34(6):868–72. doi:10.1097/PAS.0b013e3181dbee07
75. Vivero M, Kraft S, Barletta JA. Risk stratification of follicular variant of papillary thyroid carcinoma. Thyroid (2013) 23(3):273–9. doi:10.1089/thy.2012.0369
76. Ganly I, Wang L, Tuttle RM, Katabi N, Ceballos GA, Harach HR, et al. Invasion rather than nuclear features correlates with outcome in encapsulated follicular tumors: further evidence for the reclassification of the encapsulated papillary thyroid carcinoma follicular variant. Hum Pathol (2015) 46(5):657–64. doi:10.1016/j.humpath.2015.01.010
77. Rosario PW, Penna GC, Calsolari MR. Noninvasive encapsulated follicular variant of papillary thyroid carcinoma: is lobectomy sufficient for tumours ≥1 cm? Clin Endocrinol (2014) 81(4):630–2. doi:10.1111/cen.12387
78. Baloch ZW, LiVolsi VA. Encapsulated follicular variant of papillary thyroid carcinoma with bone metastases. Mod Pathol (2000) 13(8):861–5. doi:10.1038/modpathol.3880153
79. Howitt BE, Paulson VA, Barletta JA. Absence of BRAF V600E in non-infiltrative, non-invasive follicular variant of papillary thyroid carcinoma. Histopathology (2015) 67(4):579–82. doi:10.1111/his.12680
80. Liu Z, Zhou G, Nakamura M, Koike E, Li Y, Ozaki T, et al. Encapsulated follicular thyroid tumor with equivocal nuclear changes, so-called well-differentiated tumor of uncertain malignant potential: a morphological, immunohistochemical, and molecular appraisal. Cancer Sci (2011) 102(1):288–94. doi:10.1111/j.1349-7006.2010.01769.x
81. Sahli ZT, Umbricht CB, Schneider EB, Zeiger MA. Thyroid nodule diagnostic markers in the face of the new diagnosis, NIFT-P: time for a reset? Thyroid (2017) 27(11):1393–9. doi:10.1089/thy.2017.0238
82. Jiang X, Harrison GP, Datto MB. Young investigator challenge: molecular testing in noninvasive follicular thyroid neoplasm with papillary-like nuclear features. Cancer Cytopathol (2016) 124(12):893–900. doi:10.1002/cncy.21802
83. Nikiforov YE, Ohori NP, Hodak SP, Carty SE, LeBeau SO, Ferris RL, et al. Impact of mutational testing on the diagnosis and management of patients with cytologically indeterminate thyroid nodules: a prospective analysis of 1056 FNA samples. J Clin Endocrinol Metab (2011) 96(11):3390–7. doi:10.1210/jc.2011-1469
84. Najafian A, Noureldine S, Azar F, Atallah C, Trinh G, Schneider EB, et al. RAS mutations, and RET/PTC and PAX8/PPAR-gamma chromosomal rearrangements are also prevalent in benign thyroid lesions: implications thereof and a systematic review. Thyroid (2017) 27(1):39–48. doi:10.1089/thy.2016.0348
85. Duh Q-Y, Busaidy NL, Rahilly-Tierney C, Gharib H, Randolph G. A systematic review of the methods of diagnostic accuracy studies of the Afirma gene expression classifier. Thyroid (2017) 27(10):1215–22. doi:10.1089/thy.2016.0656
86. Angell TE, Frates MC, Medici M, Liu X, Kwong N, Cibas ES, et al. Afirma benign thyroid nodules show similar growth to cytologically benign nodules during follow-up. J Clin Endocrinol Metab (2015) 100(11):E1477–83. doi:10.1210/jc.2015-2658
87. Durante C, Costante G, Lucisano G, Bruno R, Meringolo D, Paciaroni A, et al. The natural history of benign thyroid nodules. JAMA (2015) 313(9):926–35. doi:10.1001/jama.2015.0956
88. Alexander EK, Hurwitz S, Heering JP, Benson CB, Frates MC, Doubilet PM, et al. Natural history of benign solid and cystic thyroid nodules. Ann Intern Med (2003) 138(4):315–8. doi:10.7326/0003-4819-138-4-200302180-00010
89. Kloos RT, Hanna JW, Haroldson J, Barth N. Systematic review of clinical utility and longer term follow up of Afirma GEC testing. Endocr Soc (2016). doi:10.1210/endo-meetings.2016.THPTA.14.SAT-284
90. Labourier E. Utility and cost-effectiveness of molecular testing in thyroid nodules with indeterminate cytology. Clin Endocrinol (2016) 85(4):624–31. doi:10.1111/cen.13096
91. Li H, Robinson KA, Anton B, Saldanha IJ, Ladenson PW. Cost-effectiveness of a novel molecular test for cytologically indeterminate thyroid nodules. J Clin Endocrinol Metab (2011) 96(11):E1719–26. doi:10.1210/jc.2011-0459
92. Yip L, Apfel A, Singh A, Yoo J, McCoy K, Stang MT, et al. Comprehensive cost analysis of available molecular tests for thyroid nodules with follicular neoplasm cytology. International Thyroid Congress and American Thyroid Association. Lake Buena Vista, FL (2015).
Keywords: thyroid cancer, non-invasive follicular thyroid neoplasm with papillary-like nuclear features, molecular test, Afirma, Thyroseq
Citation: Sahli ZT, Smith PW, Umbricht CB and Zeiger MA (2018) Preoperative Molecular Markers in Thyroid Nodules. Front. Endocrinol. 9:179. doi: 10.3389/fendo.2018.00179
Received: 14 February 2018; Accepted: 03 April 2018;
Published: 18 April 2018
Edited by:
Derek LeRoith, Icahn School of Medicine at Mount Sinai, United StatesReviewed by:
Salvatore Benvenga, Università degli Studi di Messina, ItalyCaterina Mian, Università degli Studi di Padova, Italy
Vasyl Vasko, Uniformed Services University of the Health Sciences, United States
Copyright: © 2018 Sahli, Smith, Umbricht and Zeiger. This is an open-access article distributed under the terms of the Creative Commons Attribution License (CC BY). The use, distribution or reproduction in other forums is permitted, provided the original author(s) and the copyright owner are credited and that the original publication in this journal is cited, in accordance with accepted academic practice. No use, distribution or reproduction is permitted which does not comply with these terms.
*Correspondence: Martha A. Zeiger, maz4c@virginia.edu