The nature and controls on downstream change of channel sediment along the Shiyang River, Northwest China
- 1College of Resources and Environment, Tibet Agricultural and Animal Husbandry University, Linzhi, China
- 2Key Laboratory of Western China’s Environmental Systems (Ministry of Education), College of Earth and Environmental Sciences, Lanzhou University, Lanzhou, China
- 3School of Geographic Sciences, Xinyang Normal University, Xinyang, China
- 4Wuwei No. 1 Middle School, Wuwei, China
- 5School of Municipal and Surveying Engineering, Hunan City University, Yiyang, China
The grain size composition and distribution of river sediments are important for understanding regional geomorphological evolution, source-sink processes and drainage ecology. The Shiyang River basin, an inland river system in northwestern China, provides an environmental context within which to investigate the relationship between sediment particle size and environmental factors. Based on the analysis of the grain size characteristics of modern riverbed sediments, basin geomorphological parameters and lithology, it is found that the median grain size (Md) of river sediments shows a decrease trend from upstream to downstream in the basin. One of the tributaries named the Jinta River shows an obvious downstream fining trend (exponentially decreasing) of Md, which is related to the old geomorphological development stages and relatively homogeneous lithology in the basin. The downstream fining trend of Md along the Xiying River, another tributary, shows complex fluctuations, which might be affected by the tectonically active, young geomorphic development stage, complex lithology of the basin, and the sediment confluence of tributaries. The gravel-sand transition zone occurs in the plain section of the river about 28 km out of the mountain, which is related to the combination of regional geomorphological features, river morphology and hydraulic sorting.
Introduction
The grain size composition of sediments and its distribution characteristics along the river are important indicators for regional geomorphic evolution, deconstruction of basin analysis, and evaluation of watershed ecological health (Allen, 2008; Duller et al., 2010). River sediment grain size is one of the characterizing indicators of river hydrodynamic conditions and transport environment (Knighton, 1999), and its size and distribution characteristics are related to many factors such as regional geology, geomorphology, climate and hydrology (Allen, 2008; Duller et al., 2010; Blom et al., 2017). Fluvial abrasion and sorting of the reconstituted population, in the absence of further inputs, often produces relatively systematic downstream fining trends (Sternberg, 1875; Yatsu, 1955; Gale et al., 2019; Ivan and Tomáš, 2021a). Sternberg (1875) suggested that abrasion is the main cause of sediment decomposition and grain size refinement. Moreover, the hydraulic sorting effect, i.e., the preferential downstream transport of fine-grained material, is also an important factor in the refinement of river sediments (Krumbein, 1941; Leopold and Maddock, 1953). The difference in slope lithology determines the initial grain size of river sediments and is an influential factor controlling the variation of grain size along the river (Yatsu, 1955; Kodama, 1994a; Rice, 1999). In addition, factors such as channel slope, width, depth, and flow velocity can also affect the transport capacity and sediment distribution characteristics of a river. Disturbed by tributaries and other lateral source factors and influenced by human activities will break the natural river sediment grain size along the refinement trend, showing complex segmental changes (Williams and Wolman, 1985; Rice, 1999; Surian, 2002).
Shulist (1941) proposed that the channel slope is the main factor affecting the distribution of particle size along the river, and it determines the transport capacity, when the gradient decreases, the transport capacity of the river is limited, and only finer particles can be transported. Leopold and Maddock (1953) argued that the gradient is not the only factor affecting the sediment size, and that the influence of channel morphology on the grain size variation characteristics is equally important. Alternatively, lateral material may also be important factors in causing irregular variations in sediment size along the channel, such as tributaries, slope-forming material, and land use (Knighton, 1980; Rice and Church, 1996; Surian, 2002; Dawson, 2010; Church and Kellerhals, 2011). In recent years, human activities have greatly altered the natural properties of the river channel and become an important factor affecting the distribution of grain size along the channel (Williams and Wolman, 1985; Surian, 2002). In addition, many studies have found that gravel-sand transitional reaches often occur during the process of grain size changes, with the change of river channel slope and channel morphology (Ferguson, 2003; Jerolmack and Brzinski, 2010; Frings, 2011; Miller et al., 2015).
However, the impact of these environmental factors on the grain size change along the river is still controversial (Blom et al., 2017). For example, affected by the characteristics of the tributary sediments, the inflow of the tributary can cause the coarsening or refinement of the main stream channel sediment (Blom et al., 2017; Ivan and Tomáš, 2021b). Abrasion and sorting also have different effects on the refinement of river sediments in different river sections (Gale et al., 2019). The Shiyang River Basin (SYRB), which is an inland river system in Northwestern China, provides a good case for comprehensive comparative analysis of the impact of the above factors on the channel sediments. In this paper, we aim to analyze the influencing factors in combination with the geomorphic parameters, lithology and other environmental factors in the basin, so as to improve our understanding of river sediment grain size variation. This study can provide specific cases to explore the variability of sediment grain size characteristics among different rivers, and provide some ideas to reveal the general process of river sediment transport and deposition.
Study area
The Shiyang River originates from the northern slope in the eastern Qilian Mountains with an altitude of above 4,500 m above sea level (asl), flows through the Hexi Corridor at an altitude of 1,500 m, and finally disappears in the Tengger Desert. The topography is high in the south and low in the north, with a relief of 3,000 m (Figure 1A). The geomorphic units consist of the Qilian Mountains in the south, the Hexi Corridor in the center, and the low hills and desert areas in the north. The geological structure in the Basin is complex, mainly includes active Minle-Damaying Fault (M-D), Huangcheng-Shuangta Fault (H-S), Wuwei Basin South Fault (WWBS) and the Lenglongling Fault (LLL) (Figure 1B). The upper reaches of the basin are mainly Ordovician metamorphic rocks, while the middle and lower parts are dominated by loose deposits of the Quaternary (Figure 1B). In the Qilian Mountains, the upper Shiyang River is composed of eight major tributaries from west to east, including the Xida, Dongda, Xiying, Jinta, Zamu, Huangyang, Gulang, and Dajing Rivers. The tributaries come out of the mountains and converge near Wuwei to form the main stream of the Shiyang River, which together constitute an important ecological safeguard for the Hexi Corridor and prevent the convergence of the Badain Jaran and Tengger Deserts (Figure 1A).
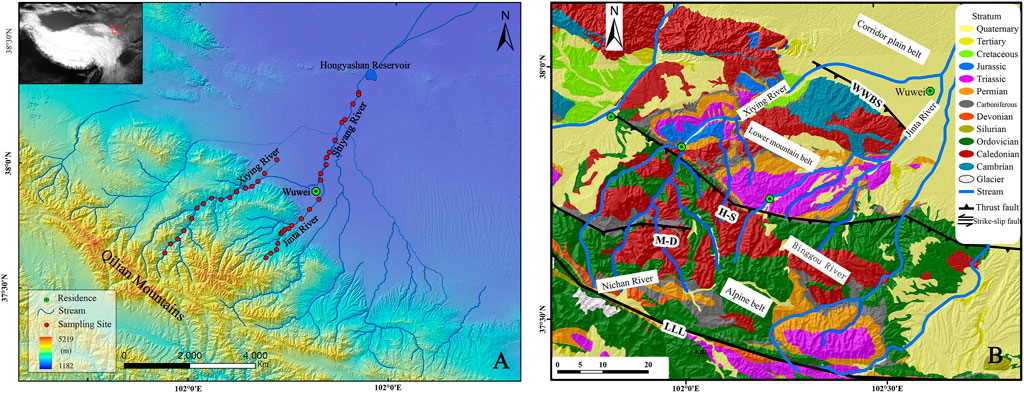
FIGURE 1. Location (A) and geological map (B) of the study area. Red dots of (A) indicate sample sites.
The SYRB, located at the intersection of the East Asian monsoon and westerly wind belts (Chen et al., 2008), possesses a typical continental arid and semi-arid climate. The spatial distribution of annual precipitation is characterized by less in the north and more in the south, more in the mountains, and less in the plains (Kang et al., 2009). Due to the large vertical height difference, the vegetation cover types and soil types in the study area are diverse. The area above 4,300 m asl is an alpine ice and snow zone, with sparse vegetation and a maximum precipitation of 600 mm. The altitude between 4,300 and 4,000 m asl is dominated by alpine sub-ice and snow sparse vegetation. It is mainly alpine dormant vegetation and alpine meadow vegetation between 4,000 and 3,500 m asl, and a mountainous forest zone dominated by cold temperate coniferous forest at 3,500–2,700 m asl. There are mainly mountain grassland, mountain desert steppe at 2,700–2,400 m and 2,300–2,000 m asl, respectively. The Hexi Corridor plain area in the middle reaches of the Basin is 1,500–2,000 m asl, with an annual precipitation of 150–300 mm. The elevation of the Minqin Basin in the lower reaches of the Basin is 1,300–1,500 m asl, and the annual precipitation is less than 150 mm. The main vegetation type is semi-shrub salt desert.
Methods
The median grain size (Md) of river sediments is the most basic characteristic parameter to measure river transport capacity, indicating the central tendency of sediment grain size frequency distribution and the average kinetic energy condition of flowing water. In this paper, the upstream mountain tributaries Jinta River, Xiying River and the main stream of Shiyang River (Shiyangdahe) in the plain area are selected as the research areas. The material composition of the river was measured using the “Wolman gravel counting method” (Wolman, 1954). The Jinta River-Shiyang River section was selected from the upper tributary Binggou River to Hongyashan Reservoir, and a total of 24 sample points were selected. For the Xiying River, the study section is near the Ningchan River to the mountain pass in the upper reaches of the basin (Figure 1A), a total of 16 samples were selected.
Bed materials were sampled at approximately equal interval along each river channel. With the sediments consisting primarily of particles larger than 2 mm in size, sampling was conducted according to the procedures outlined by Wolman (1954). In river sections, take one bank at the full bank level as the starting point, walk along the jagged path in the river (Figure 2A) a photograph of the gravel in the field is shown in (Figure 2B), select the gravel in which the toe of the index finger steps in each step, and measure the middle axis of the gravel, i.e., the b axis, with a tape measure (Figure 2C). The distance between measurements along the river section is about 3–4 km, and at least 200 gravels were measured for each cross section. Then the measured data were processed in Excel for statistical analysis and grain size classification, and the Md of the section was calculated and obtained (Figures 2D, E). For the sandy river in the lower reaches of the Basin, after collecting samples in the field, the particle size was measured and analyzed using a Malvern Mastersizer 2000 laser particle sizer in the laboratory.
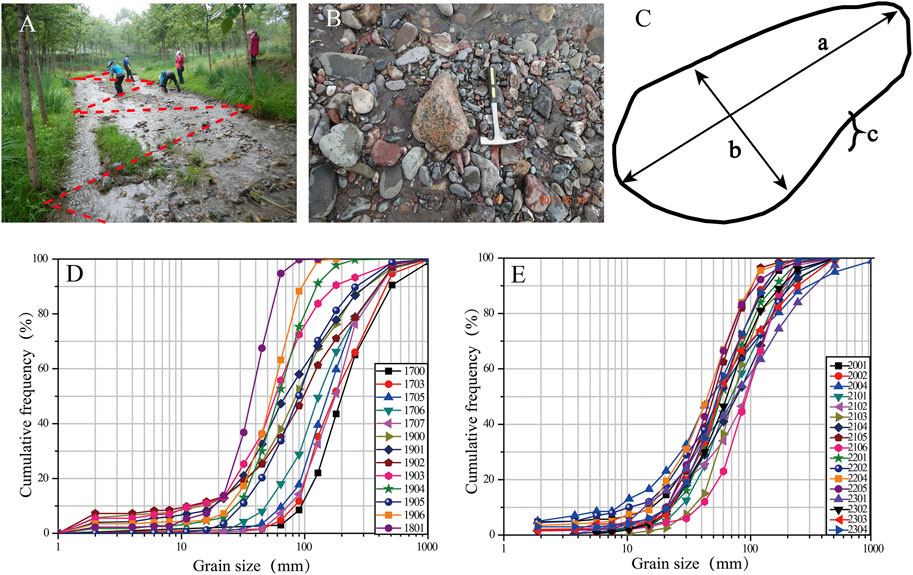
FIGURE 2. Diameter measurement method and field photographs of channel sediments. (A,B) show field survey methods and photos; (C): Measuring shaft “B” axis; (D,E) indicate frequency accumulation curves of the samples.
Results
Grain size distribution
The statistical results show (Figure 3A) that two channel types, i.e., gravelly and sandy channels, can be identified along the river section from the Jinta River to Hongyashan Reservoir. The section of the Jinta River from its source to Shaojiazhuang is a gravelly channel with a Md of 38–190 mm and an average of ∼106 mm (Figure 3B); the section from Shaojiazhuang to Hongyashan Reservoir is a sandy channel with a Md of 0.13–0.62 mm (Figure 3B). The modern riverbed sediments in the Xiying River are mainly gravels, with a Md of 50–103 mm and an average of 70.6 mm (Figure 3C).
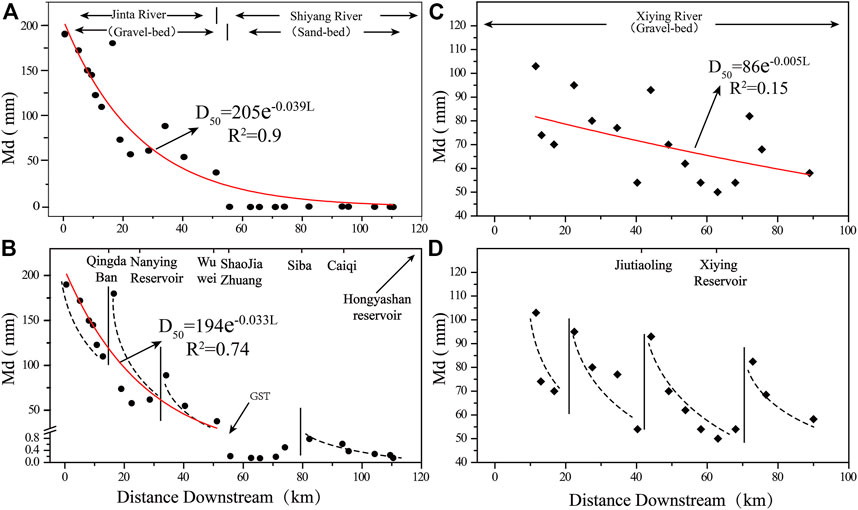
FIGURE 3. The variation characteristics of Md in channel sediments along the channel; (A,B): The variation of Md along Jinta - Shiyang River. (C,D): The variation of Md along Xiying River. The black solid line is the demarcation of the phase change, and the black dashed line is the general trend. Red solid line is the fitting result.
Downstream changes in riverbed sediment
As the distance from the river source increases, the particle size of the river sediment generally decreases exponentially, as shown in Formula Eq. 1:
where Di is a characteristic linear dimension at some distance L, D0 is the characteristic dimension at L = 0, L is the distance from the river source, and ∂ is a size diminution coefficient that expresses the rate of grain-size decreases toward downstream.
In general, there is a strong exponential relationship (R2 = 0.9) between the sediment Md and the distance L in the reach from Jinta to Shiyang Rivers, and the ∂ is about 0.039 (Figure 3A). In the gravelly section of Jinta River, there is a significant exponential decreasing relationship between Md and the distance L, and the ∂ is about 0.033 (Figure 3B). In the sandy section of the Shiyang River, the refinement trend of Md is not obvious with the increase of distance L, which shows a fluctuating change of increasing first and then decreasing. The exponential decreasing relationship between Md and river length of the Xiying River is relatively weak, with a low coefficient R2 of 0.15, and a ∂ of 0.005 (Figure 3C).
The Md of riverbed sediment in the Shiyang River section showed a general trend of gradual decrease from upstream to downstream, which is consistent with the trend of refinement along the grain size in most studies (Table 1). A comparison of the global stream sediment refinement model (Figure 4A) shows that most of the gravelly channels are less than 102 km long, while the sandy channels can stretch for thousands of kilometers, indicating that the transport distance of gravel in the channel is limited, generally not more than 100 km. The fining coefficient of gravelly river is generally greater than 0.01, while that of sandy river is generally lower than 0.01, that is, the refinement trend of gravelly channels is more obvious than that of sandy channels (Figures 4B, C). In addition, the fining coefficient α of river sediments was inversely correlated with river length (Figure 4A), which probably attributed to the fact that the longer the river, the more complex the response of river sediment grain size to factors such as landforms, climate, and tributary confluence, thus showing a more complex change trend.
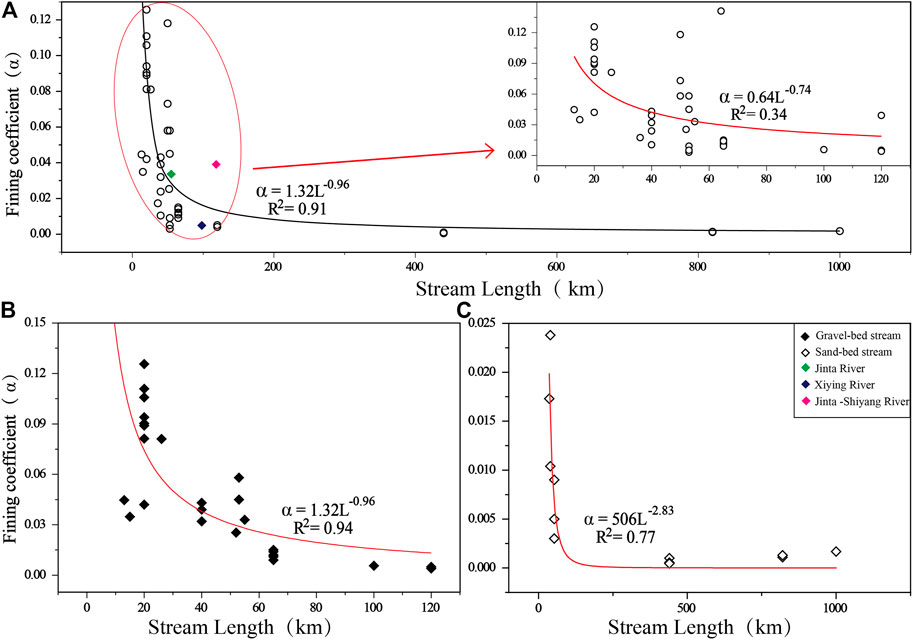
FIGURE 4. Relationship between river length and refinement index of some rivers in the world (A). Red solid line is the fitting result. The sub-graph shows the relationship between river length and sediment refinement index within 120 km of stream length. (B,C) indicate relationships between river length and sediment particle size refinement index of some gravel-bed, and sand-bed streams in the world, respectively.
Abundant studies suggest that the downstream fining of river bed materials can be attributed to sorting and abrasion. However, lateral inputs of coarse sediment, basin geomorphic development stage, lithology, human activities and tributary confluence may break the downstream fining trend (Figure 3). For example, in the Jinta River, there is a sudden increase in the Md of river sediment near Qingdaban and below the Nanying Reservoir. The sudden change in Md of river sediment in the Xiying River mainly occurs at about 20 km from the source, near Jiutiaoling, and at the head of the Xiying River canal (Figures 3B, D), and the occurrence of these sudden change in grain size makes the distribution of river sediment along the river show an obvious segmental decreasing trend.
The gravel-sand transition
The results show that the Gravel-Sand Transition (GST) is evident in the Hexi Corridor area at about 28 km downstream from the outlet of the Jinta River (Figure 3B). Previous studies have shown that the GST of river sediments is usually related to slope, local base level, excessive sand supply or gravel wear, selective sorting, etc., (Yatsu, 1955; Gregory et al., 1995; Knighton, 1999; Dubille and Lavé, 2015; Ferguson and Ashworth, 2010; Ehda et al., 2021). Especially after the rivers flow out of the mountain, the channel is not limited laterally by topography, and the rapid decrease in sand transport capacity of the river is the main reason for the formation of the GST zone. In addition, the GST is accompanied by a shift in channel planform from multi-channel to single-channel (Dubille and Lavé, 2015), e.g., gravelly reaches in the upper part of the gravel-sand transition zone are usually braided streams with a large channel slope (Gregory et al., 1995; Venditti and Church, 2014), while downstream channels typically evolve into single-channel sandy streambeds (Frings, 2011; Dubille and Lavé, 2015; Dingle et al., 2020). From the mountain pass of Jinta River to Wuwei City, the landforms are dominated by alluvial fans, wide and shallow river channels. The river channel presents a braided river style, with the channel slope of 0.02. In the Hexi Corridor plain area, it is dominated by narrow and deep meandering rivers, with a slope of less than 0.005 (Figure 5). The GST occurs at the distance of about 10–40 km downstream from most river outlets (Dingle et al., 2017; Ehda et al., 2021), suggesting that the gravel transport distance is related to the geomorphic characteristics of the watershed, sediment supply, and the transport capacity of flowing water.
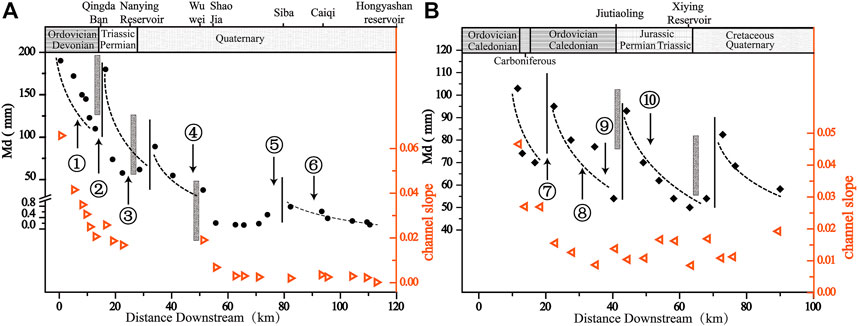
FIGURE 5. The relationship between slope, tributary inflow and Md of sediments in Jinta—Shiyanghe River (A,B) Xiying River. ①-⑩ indicate Xigou, Binggou, Dashui, Yangjiaba, Baita, Hongshui, Qingyang, Tuoluo, Shuiguan, and Xiangshui Rivers, respectively. The gray strips indicate human settlements and reservoirs.
It was suggested that the GST occur at the distance of about 10–40 km downstream from the mountain pass (Dingle et al., 2017; Ehda et al., 2021), indicating that the gravel transport distance is limited downstream the outlet. After the river exits the mountain pass, the stream gradient decreases rapidly in the lower reaches, and the channel is no longer restricted, accelerating lateral swings, resulting in large deposits of coarse gravel and continued transport of fine-grained material (Paola et al., 1992). And in the GST region, the presence of some gravel further promotes the fragmentation of matter and the production of fine particles (Bradley, 1970; Kodama, 1994a). Therefore, under the condition of river hydraulic separation, fine particles are preferentially transported faster and farther downstream than coarse particles. In addition, some rivers passing through Wuwei City have been artificially channelized, which will also affect the original transport process of river sediments.
Discussions
Influence of tributary inflow on sediment downstream fining
River sediments are mainly originated from the slope process, tributary inflow and river bank erosion along the river. Tributary confluence generally leads to a sudden increase in sediment grain size (Ichim and Radoane, 2010; Brewer and Lewin, 1993; Rice, 1998). If the transport capacity of the confluent section is weak, the coarse-grained materials will be deposited on the riverbed (Lane, 1995), and this deposition will in turn lead to a reduction in the channel slope in the confluence section, resulting in a reduction in runoff shear stress and deposition of fine-grained material (Dawson, 2010). Our results show that the confluence of Binggou River, Dashui River and Baita River, tributaries of the Jinta River, showed coarsening of the grain size of the riverbed material in the confluence section (Figure 5A). In addition, the confluence of Qingyang River and Shuiguan River, tributaries of the Xiying River, similarly caused an increase in the sediment particle size of the main channel (Figure 5B).
Especially the larger the volume of an input and the greater the grain size disparity between it and the main stem material, the greater is the expectation that the main-stem texture is changed notably. At confluences the situation is complicated by the concomitant influx of water which, if sufficiently large, modifies ambient stresses and, in turn, the bed material. A tributary which introduces a significant quantity of water but little sediment could, by increasing the capacity of the main stem, produce a significant change in texture. Therefore, the relative volume of a sediment input, its size characteristics relative to the recipient channel and, at tributaries, the relative contribution of water, may be controls on the occurrence of particle size discontinuities. The volume of material carried by tributaries depends on the volume of sediment supplied to the river and the ability of the stream transport, while the volume of water flowing out of the basin depends mainly on the climate, vegetation and watershed area (Knighton, 1980; Rice, 1998). In the Qilian Mountains, the Xiying and Jinta Rivers are located in the same climatic unit, the vegetation difference is small, and the watershed area can be used to indirectly reflect the tributary flow (Table 2). For example, probably influenced by flow and sediment size, some of the tributary confluences, such as the Xigou, Tuoluo, and Xiangshui Rivers, cannot break the along-channel refinement of the river sediments (Table 2).
After the confluence of the tributaries, the sorting action and abrasion process of the water flow will continue to act on the river sediments. Except for the area near the confluence, the overall pattern of longitudinal changes in river sediments is basically not affected, and the overall trend is decreasing.
Influence of lithologic composition on sediment downstream fining
Watershed lithology is an important factor influencing the sediment yield, which determines the initial grain size of the river sediment and the anti-erosion ability (Rice, 1999). The lithology of the Jinta River basin is distributed uniform, with Ordovician metamorphic rocks and Caledonian granites in the source area, Carboniferous-Permian and Triassic sedimentary rocks in the upstream area, and Quaternary loose sediments in the middle and downstream areas. The lithology of the Xiying River basin is complex, with Ordovician metamorphic rocks and granites dominating, Silurian granites and Triassic granites and sedimentary rocks distributed locally, with a few Carboniferous and Permian strata exposed near the watershed; some Cretaceous and Cambrian strata are distributed near the outlet (Figure 1B). From the viewpoint of the stratigraphic lithology along the river channel, the distribution of lithological resistance strength and weakness along the Jinta River channel is uniform, which indicates that the lithology of the source of sedimentary material in the river channel is relatively uniform. However, the distribution of lithological resistance in the Xiying River channel is more complex, which indicates that the sources of sedimentary material are diverse, thus the grain size changes are more complex along the channel.
Influence of the geomorphic evolution stage on sediment downstream fining
The geomorphic parameters that reflect the development stage of the basin geomorphology (Bull and Mcfadden, 1977; Keller and Pinter, 2002; Peters and Balen, 2007), such as drainage density (Dd), hypsometric integral (HI), basin shape index (Bs), and valley width-to-height ratio (Vf) of the basin, are shown in Table 3. The Vf values in the Jinta River basin are larger than those in the Xiying River, while the HI and Bs values in the Jinta River basin are smaller than those in the Xiying River, indicating that the regional tectonic activity in the Xiying River is more active than that in the Jinta River basin. Moreover, the geomorphic development stage in the Jinta River basin is tend to a mature stage, and the river longitudinal profiles tend to be graded (Yatsu, 1955). Therefore, in the Jinta River basin, which is relatively tectonically stable and has a relatively mature development stage, the refinement of river sediment shows an obvious exponential decreasing, while in the Xiying River basin, which is relatively tectonically active and has a relatively young development stage, the trend of refinement of river sediment grain size is often broken.
Influence of channel slope on sediment downstream fining
The decrease of channel slope will weaken the transport capacity of the river and lead to the accumulation of coarser fractions in the river sediment. By comparison, it was found that the change of channel slope in the Jinta-Shiyang Rivers generally showed a decreasing downstream trend, which basically followed the same trend as the change of river sediment grain size. For example, the gravel-sand transition zone near Wuwei corresponds to a decrease in channel slope (Figure 5A), which may be due to the fact that this area is a transition area from mountainous to plain area, and the lower channel slope reduces the transport capacity of the river, which can only transport finer fractions of material downstream, so that there is an obvious gravel-sand transition zone downstream. Similarly, the Xiying River channel shows a general trend of fluctuating lowering of channel slope downstream, and the fluctuating change corresponds to the area of sudden increase of grain size (Figure 5B), indicating that, except for strong tectonic activities, the along-channel change of channel slope has a significant influence on the change of grain size of river sediments.
Influence of human activities on sediment downstream fining
Increasing human activities, such as village settlements and the construction of dams and reservoirs, can regulate river runoff, breaking the original water-sand balance of the river, for example, leading to a reduction in the transport capacity of the river, and inability to transport coarse-grained material downstream (Surian, 2002). For example, in the upstream of the Xiying River near Jiutiaoling (Figure 5A), the construction of houses and bank stabilization have led to an obvious coarsening of river sediment grain size along the river. In addition, the construction of reservoirs, has changed the river environment and weakened the river transport capacity, and the downstream river sediment grain size of reservoirs has also coarsened (Figure 5B).
In addition, the construction of Tianma Lake near Wuwei reduced the riverbed sediment transported downstream, breaking the original refinement pattern of river sediment grain size, and an obvious abrupt change area of gravelly sand transformation appeared in the area below Wuwei. Below Tianma Lake, the impact of human activities decreased, and the grain size of river sediments gradually increased and continued to show a refinement trend below Siba.
Conclusion
Based on the analysis of the variation of river sediment grain size along the Shiyang River basin in northwestern China, it was found that the river particle size generally shows a trend of refinement along the river channel. The refinement index of gravelly channels is generally larger than that of sandy channels. And the GST zone occurs at about 28 km downstream from the outlet of the Jinta River in the Hexi Corridor area. The tributary confluence is the main reason why the sediment refinement trend is broken, and the difference of geomorphic development stage and lithology in the basin is also the main reason why the sediment grain size distribution along the two tributary channels of Jinta and Xiying Rivers is different. Human activities, such as sand and gravel extraction, bank stabilization and damming, reservoir storage, change the natural properties of local rivers and affect the distribution of sediment grain size along the rivers.
Data availability statement
The raw data supporting the conclusion of this article will be made available by the authors, without undue reservation.
Author contributions
Project administration and experimental design: GH Writing, original draft: PL, and HG Writing, review and editing: all authors. Methodology and investigation: HG, PL, ZL, YW, and FL.
Funding
This study was supported by the Second Tibetan Plateau Scientific Expedition and Research Program (STEP) (Grant No. 2019QZKK0205) and the National Natural Science Foundation of China (Grant Nos. 42171002).
Conflict of interest
The authors declare that the research was conducted in the absence of any commercial or financial relationships that could be construed as a potential conflict of interest.
Publisher’s note
All claims expressed in this article are solely those of the authors and do not necessarily represent those of their affiliated organizations, or those of the publisher, the editors and the reviewers. Any product that may be evaluated in this article, or claim that may be made by its manufacturer, is not guaranteed or endorsed by the publisher.
References
Allen, P. (2008). From landscapes into geological history. Nature 451, 274–276. doi:10.1038/nature06586
Blom, A., Viparelli, E., and Chavarrías., V. (2017). The graded alluvial river: Profile concavity and downstream fining. Geophys. Res. Lett. 43 (12), 6285–6293. doi:10.1002/2016GL068898
Bradley, W. C. (1970). Effect of weathering on abrasion of granitic gravel, Colorado river (Texas). Geol. Soc. Am. Bull. 81 (1), 61–80. doi:10.1130/0016-7606(1970)81[61:EOWOAO]2.0.CO;2
Bradley, W. C., Fahnestock, R. K., and Rowekamp, E. T. (1972). Coarse sediment transport by flood flows on knik river, Alaska. Geol. Soc. Am. Bull. 83 (5), 1261–1284. doi:10.1130/0016-7606(1972)83[1261:CSTBFF]2.0.CO;2
Brewer, P., and Lewin, J. (1993). In-transport modification of alluvial sediment: Field evidence and laboratory experiments. Sediment 17, 23–35.
Brierley, G. J., and Hickin, E. J. (2010). The downstream gradation of particle sizes in the squamish river, British columbia. Earth Surf. Process. Landforms 10 (6), 597–606. doi:10.1002/esp.3290100607
Bull, W. B., and McFadden, L. D. (1977). “Tectonic geomorphology north and south of the garlock fault, California,” in Geomorphology of arid regions. Proceedings of the eighth annual geomorphology symposium. Editor Doehring (Binghamton: State University of New York at Binghamton), 115–138. doi:10.1016/S0379-6779(00)01411-9
Chen, D., Chen, J., Hu, X., Su, H., Chen, Y., Zhang, J., et al. (2018). Characteristics and analysis on the sediment grain size along the Liyuan River on the north piedmont of the Qilian Shan. Quat. Sci. 38 (6), 1336–1347. doi:10.11928/j.issn.1001-7410.2018.06.02
Chen, F., Yu, Z., Yang, M., Ito, E., Wang, S., Madsen, D. B., et al. (2008). Holocene moisture evolution in arid central Asia and its out-of-phase relationship with Asian monsoon history. Quat. Sci. Rev. 27 (3-4), 351–364. doi:10.1016/j.quascirev.2007.10.017
Church, M., and Kellerhals, R. (2011). On the statistics of grain size variation along a gravel river. Can. J. Earth Sci. 15 (7), 1151–1160. doi:10.1139/e78-121
Dawson, M. (2010). Sediment size variation in a braided reach of the sunwapta river, alberta, Canada. Earth Surf. Process. Landforms 13 (7), 599–618. doi:10.1002/esp.3290130705
Deigaard, R., and Fredsee, J. (1978). Longitudinal grain sorting by current in alluvial streams. Nord. Hydrol. 9 (1), 7–16. doi:10.2166/nh.1978.0002
Dingle, E., Attal, M., and Sinclair, H. (2017). Abrasion-set limits on himalayan gravel flux. Nature 544, 471–474. doi:10.1038/NATURE22039
Dingle, E. H., Sinclair, H. D., Venditti, J. G., Attal, M., Kinnaird, T. C., Creed, M., et al. (2020). Sediment dynamics across gravel-sand transitions: Implications for river stability and floodplain recycling. Geology 48 (5), 468–472. doi:10.1130/g46909.1
Dubille, M., and Lavé., J. (2015). Rapid grain size coarsening at sandstone/conglomerate transition: Similar expression in himalayan modern rivers and pliocene molasse deposits. Basin Res. 27 (1), 26–42. doi:10.1111/bre.12071
Duller, R. A., Whittaker, A. C., Fedele, J. J., Whitchurch, A. L., Springett, J., Smithells, R., et al. (2010). From grain size to tectonics. J. Geophys. Res. 115, F03022. doi:10.1029/2009jf001495
Ehda, B., Kmk, A., and Jgva, C. (2021). The gravel-sand transition and grain size gap in river bed sediments. Earth-Science Reviews. Int. Geol. J. Bridg. Gap between Res. Articles Textb. 222. doi:10.1016/j.earscirev.2021.103838
Ferguson, R., and Ashworth, P. (2010). Slope-induced changes in channel character along a gravel-bed stream: The allt dubhaig, scotland. Earth Surf. Process. Landforms 16 (1), 65–82. doi:10.1002/esp.3290160108
Ferguson, R. (2003). Emergence of abrupt gravel to sand transitions along rivers through sorting processes. Geology 31 (2), 159–162. doi:10.1130/0091-7613(2003)031<0159:eoagts>2.0.co;2
Frings, R., M. (2011). Sedimentary characteristics of the gravel-sand transition in the river rhine. J. Sediment. Res. 81 (1), 52–63. doi:10.2110/jsr.2011.2
Gale, S. J., Ibrahim, Z. Z., Lal, J., and Sicinilawa, U. (2019). Downstream fining in a megaclast-dominated fluvial system: The sabeto river of Western viti levu, Fiji. Geomorphology 330 (1), 151–162. doi:10.1016/j.geomorph.2019.01.009
Gregory, H., Sambrook, S., Robert, I., and Ferguson, (1995). The gravel-sand transition along river channels. J. Sediment. Res. 65 (2a), 423–430. doi:10.1306/D42680E0-2B26-11D7-8648000102C1865D
Hoey, T. B., and Bluck, B. J. (1999). Identifying the controls over downstream fining of river gravels. J. Sediment. Res. 69 (1), 40–50. doi:10.2110/jsr.69.40
Ivan, S., and Tomás, G. (2021a). Influence of tributaries on downstream bed sediment grain sizes under flysch conditions. J. Mt. Sci. 18 (4), 847–862. doi:10.1007/s11629-020-6182-4
Ichim, I., and Radoane, M. (2010). Channel sediment variability along a river: a case study of the siret river (romania). Earth Surf. Process. Landf. 15 (3), 211–225. doi:10.1002/esp.3290150304
Ivan, S., and Tomás., G. (2021b). channel sediment variability along a river: A case study of the siret river (Romania). Earth Surf. Process. Landforms 15 (3), 211–225. doi:10.1002/esp.3290150304
Jerolmack, D. J., and Brzinski, T. A. (2010). Equivalence of abrupt grain-size transitions in alluvial rivers and eolian sand seas: A hypothesis. Geology 38 (8), 719–722. doi:10.1130/g30922.1
Kang, S., Su, X., and Tong, L. (2009). Water resources transformation law and water-saving regulation model at scale in arid area of northwest China: A case study of the Shiyang River basin in gansu. China: China Water Power Press Publishers.
Keller, E. A., and Pinter, N. (2002). Active tectonics: Earthquakes, uplift, and landscape. 2nd Ed. New Jersey: Prentice-Hall, 304–326.
Knighton, A. D. (1980). Longitudinal changes in size and sorting of stream-bed material in four English rivers. Geol. Soc. Am. Bull. 91 (1), 55–62. doi:10.1130/0016-7606(1980)91<55:lcisas>2.0.co;2
Knighton, A. D. (1999). The gravel–sand transition in a disturbed catchment. Geomorphology 27 (27), 325–341. doi:10.1016/s0169-555x(98)00078-6
Kodama, Y. (1994a). Downstream changes in the lithology and grain size of fluvial gravels, the watarase river, Japan: Evidence of the role of abrasion in downstream fining. J. Sediment. Res. 64 (1), 68–75.
Kodama, Y. (1994b). Experimental study of abrasion and its role in producing downstream fining in gravel-bed rivers. J. Sediementary Res. 64, 76–85. doi:10.2110/jsr.64.76
Krumbein, W. C. (1941). The effects of abrasion on the size, shape and roundness of rock fragments. J. Geol. 49 (5), 482–520. doi:10.1086/624985
Lane, E. W. (1995). The importance of fluvial morphology in Hydraulic Engineering, 81. US: American Society of Civil Engineers, 1–17.
Leopold, L. B., and Maddock, T. (1953). The hydraulic geometry of stream channels and some physiographic implications. Usgs Professional Paper. 252.
Miller, K. L., Reitz, M. D., and Jerolmack, D. J. (2015). Generalized sorting profile of alluvial fans. Geophys. Res. Lett. 41 (20), 7191–7199. doi:10.1002/2014gl060991
Moussavi-Harami, R., Mahboubi, A., and Khanehbad, M. (2004). Analysis of controls on downstream fining along three gravel-bed rivers in the band-e-golestan drainage basin ne Iran. Geomorphology 61 (1/2), 143–153. doi:10.1016/j.geomorph.2003.12.005
Nordin, C. F., Meade, R. H., Curtis, W. F., Bósio, N. J., and Landim, P. M. B. (1980). Size distribution of amazon river bed sediment. Nature 286 (5768), 52–53. doi:10.1038/286052a0
Paola, C., Parker, G., Seal, R., Sinha, S. K., Southard, J. B., and Wilcock, P. R. (1992). Downstream fining by selective deposition in a laboratory flume. Science 258 (5089), 1757–1760. doi:10.1126/science.258.5089.1757
Peters, G., and Balen, R. (2007). Tectonic geomorphology of the northern upper rhine graben, Germany. Glob. Planet. Change 58 (1-4), 310–334. doi:10.1016/j.gloplacha.2006.11.041
Rana, S. A., Simons, D. B., and Mahmood, K. (1973). Analysis of sediment sorting in alluvial channels. Am. Soc. Civ. Eng. 99 (11), 1967–1980. doi:10.1061/jyceaj.0003786
Rice, S., and Church, M. (1996). Sampling surficial fluvial gravels; the precision of size distribution percentile sediments. J. Sediment. Res. 66 (3), 654–665. doi:10.2110/jsr.66.654
Rice, S. (1999). The nature and controls on downstream fining within sedimentary links. J. Sediment. Res. 69 (1), 32–39. doi:10.2110/jsr.69.32
Rice, S. (1998). Which tributaries disrupt downstream fining along gravel-bed rivers? Geomorphology 22 (1), 39–56. doi:10.1016/s0169-555x(97)00052-4
Shulist, S. (1941). Rational equation of river-bed profile. Eos Trans. Am. Geophys. Union 22 (3), 622–631. doi:10.1029/tr022i003p00622
Sternberg, H. (1875). Untersuchungen über Längen-und Querprofil geschiebeführender Flüsse. Z. für Bauwes. 25, 484–506.
Surian, N. (2002). Downstream variation in grain size along an alpine river: Analysis of controls and processes. Geomorphology 43 (1-2), 137–149. doi:10.1016/s0169-555x(01)00127-1
Ta, W. Q., Wang, H. B., and Jia, X. P. (2011). Downstream fining in contrasting reaches of the sand-bedded Yellow River. Hydrol. Process 25, 3693–3700. doi:10.1002/hyp.8065
Venditti, J. G., and Church, M. (2014). Morphology and controls on the position of a gravel-sand transition: Fraser River, British Columbia. Earth Surf. 119 (9), 1959–1976. doi:10.1002/2014jf003147
Williams, G. P., and Wolman, M. G. (1985). The downstream effects of dams on alluvial rivers, 1286. USGS professional paper.
Wolman, M. G. (1954). A method of sampling coarse river-bed material. Eos Trans. Am. Geophys. Union 35 (6), 951–956. doi:10.1029/TR035i006p00951
Keywords: river sediment, median grain size, downstream fining, gravel-sand transition, Shiyang River
Citation: Li P, Gao H, Yan T, Li Z, Wu Y and Liu F (2023) The nature and controls on downstream change of channel sediment along the Shiyang River, Northwest China. Front. Earth Sci. 11:1112772. doi: 10.3389/feart.2023.1112772
Received: 30 November 2022; Accepted: 13 January 2023;
Published: 24 January 2023.
Edited by:
Weiming Liu, Institute of Mountain Hazards and Environment (CAS), ChinaReviewed by:
Yuanxu Ma, Aerospace Information Research Institute (CAS), ChinaChenge An, Tsinghua University, China
Copyright © 2023 Li, Gao, Yan, Li, Wu and Liu. This is an open-access article distributed under the terms of the Creative Commons Attribution License (CC BY). The use, distribution or reproduction in other forums is permitted, provided the original author(s) and the copyright owner(s) are credited and that the original publication in this journal is cited, in accordance with accepted academic practice. No use, distribution or reproduction is permitted which does not comply with these terms.
*Correspondence: Hongshan Gao, gaohsh@lzu.edu.cn