Assessing and Managing Risk in Historic Urban Areas: Current Trends and Future Research Directions
- 1Department of Geography and Environmental Management, University of the West of England, Bristol, United Kingdom
- 2Department of Civil Engineering, ISISE, University of Minho, Guimarães, Portugal
Historic urban centres are, almost by definition, risk-prone areas. The buildings in the historical sites are often highly vulnerable to natural and human-made hazards, not only due to their construction and material characteristics but also because they are usually very degraded due to ineffective maintenance and conservation policies. Moreover, the recent world tourism boom has led to a significant increase in the number of people who live, work and visit these areas, which, together with land use and climate change-related impacts, make historic centres particularly exposed areas. This paper addresses the issue of assessing and managing risk in historic urban centres departing from the complexity of defining the historic city and the concept of risk, providing a comprehensive discussion on current trends and future research directions in this field. After analysing the most suitable methodologies to assess the vulnerability of these areas to different hazards, the focus is on data collection and organisation-related issues and how the different vulnerability assessment outputs can be used to manage and mitigate risk. Vulnerability and loss scenarios, evacuation and emergency planning, and retrofit and cost-benefit analyses are some of the aspects addressed herein. This discussion includes some considerations on the accuracy of these approaches and aspects related to their calibration and validation, covering from empirical calibration models to advanced artificial Intelligence-based techniques.
1 Introduction
Urban areas are, by definition, highly vulnerable to natural hazards (Ferreira et al., 2013). The cities are physical places where several actors, stakeholders, assets and activities converge. In particular, the long-term processes and transformations of the historic centres result in a wide variety of uses and unique configurations. These singularities, which are often a manifestation of cultural values, also demand a very specific and straightforward analysis of the historic city and the challenges it must face, such as risks associated with natural hazards. The impact of certain disastrous events—including those commonly named as natural disasters (Chmutina and von Meding, 2019)—brings a series of irreversible consequences, such as human and economic losses and the destruction of irreplaceable architectural and cultural heritage. Then, it becomes relevant to promote sustainable development and resilient communities towards risks.
A recent United Nations report (United Nations Office for Disaster Risk Reduction, 2018) focused on the 1998–2017 period, concludes that floods (43.4%), storms (28.2%) and earthquakes (7.8%) are three most significant hazards in terms of recurrence. However, when unfolding these figures, it is possible to observe that the proportion of people affected by each of these hazards is significantly different—56% of human casualties are related to earthquakes, 11% to floods, and 17% to storms. A similar dissonant trend can be observed when analysing economic losses. For the 1998–2017 period, storms were responsible for about US$ 1,330 billion in losses (46% of the total amount of economic losses recorded), whereas earthquakes and floods were responsible for about US$ 655 billion each. The impact of natural hazards on heritage, particularly in World Heritage sites, has also been analysed by (UNESCO, 2007) in several case studies, in an attempt to identify possible combinations of factors (location, uses, external events, etc.) that can lead to the development of damage and decay processes in historic cities.
Besides natural hazards, man-made phenomena can also negatively affect historic cities. Wars are an archetypical example of this, damaging or completely destroying several cultural heritage sites every year. Although much discussion has been done on this topic after World War II (Moustafa, 2016), the difficulties in protecting cultural heritage in war contexts is still a real issue today, as is, for different reasons, the increasing pressures resulting from mass tourism, real estate speculations and gentrification (García-Hernández et al., 2017). These phenomena are worldwide spread and have contributed to a significant loss of heritage value and identity.
The risk analysis for historic urban areas must encompasses a series of conceptual frameworks, mostly related to the urban components and their functions. There are some interesting efforts towards framing historic cities in generalised and comprehensive structures, such as the so-called Historic Urban Landscape (HUL). This concept is the core of a systematic framework for studying urban phenomena. The HUL Guidebook developed by UNESCO (2016) proposes a series of strategies for assessing and planning more sustainable historic cities. The implementation of the HUL approach is still a relatively novel field, namely due to the complexity of conciliating the interests of numerous agents. Some experiences, however, are aimed to propose holistic and comprehensive strategies in which cities can be understood by means of models, diverse characterisations and semantic descriptions (Angrisano et al., 2016; Rey-Pérez and Pereira Roders, 2020; Ramírez Eudave and Ferreira, 2021a).
One of the most important steps of the HUL approach consists of assessing the vulnerability of the city towards natural events, socio-economic stresses and climatic change. In this context, it becomes determinant to anticipate the challenges that a city will reasonably face, compromising its functions, material assets, inhabitants and immaterial values. Some estimative on this field express the proportion of the long-term impact that these events would have. For example, the Financial Risk and Opportunities to Build Resilience in Europe inform (World Bank, 2021) states that major disasters create liabilities that can exceed 17% of Gross Domestic Product in European countries. Furthermore, it presents a scenario in which there is a 10% chance of having an earthquake or flood that will exhaust the existing finance. Preparedness towards these scenarios is a key for guaranteeing the sustainable future of historic cities.
In this framework, the present manuscript provides a compilation and discussion of research focused on the assessment of the vulnerability and risk in urban areas to natural and human-made hazards, including earthquake, fire and flood. By covering a wide range of approaches and research experiences, such a review is mainly targeted to researchers, practitioners and decision-makers interested in a broad but integrative approach to the topic.
2 Proactivity Towards Urban Risks: Understanding for Anticipating
Risk awareness in historic cities should start with establishing the perturbations imposed by the events and the sensibility that the systems and components of the city have towards them. It is relevant to understand the performance conditions of the city (i.e., its functions and the elements that sustain them) and their association with mechanisms of perturbation, being, in this sense, convenient to start this discussion by exploring the concept of risk and its components.
2.1 A Brief Literature Review
Literature devoted to characterisation, assessment, and mitigation of urban risks is vast due to the wide variety of environments, hazard sources, and approaches. For this reason, any attempt to put together and discuss the literature on this topic is doomed to failure. Even so, it is essential to mention in the context of this paper a few works that, thanks to their groundbreaking nature, have shaped the current knowledge in this field. The description of the city is still an open discussion in which the vision of diverse stakeholders can determine how the city is understood. For example (de Carvalho et al., 2019), discusses how the vial networks of city centres are seen and perceived from different agents, such as residents, retailers and carriers. This work schematises the relations existing among decision-makers, logistic operators and users by putting together a series of mixed indicators and interests in which there is a shared responsibility and/or impact: Air quality, induced damage on historical constructions, economic prosperity, visual impact, productivity, safety, etc. This work emphasises the need to involve agents that are often considered out-of-scope to assess the vulnerability of cultural assets and the historic urban landscape. Furthermore, these authors explore a multi-criteria structure based on economic, environmental, social, operational and cultural factors for deciding the most suitable location of distribution facilities based on its impact on diverse aspects of the historical city, from architectural impact and visual pollution to local gains.
Another example of a comprehensive approach for assessing the impact of implementing energy-conservation measures in historic urban areas was recently proposed by (Egusquiza et al., 2018). In this work, the authors resort to the concept of Heritage Impact Assessment (HIA) to assess the invasiveness and impact that specific actions have at the level of a component, building or district. Most of the impacts considered are related to changes in the visual environment, which reflects a relevant issue when working on protected areas of cultural interest. Among other relevant aspects, this analysis emphasises the need for having multi-criteria and comprehensive frameworks for assessing enhancement actions, such as those related to energetic efficiency. Furthermore, this multi-scale analysis can be scaled and transposed to other phenomena, including the seismic vulnerability assessment of historic cities.
As discussed by (Julià and Ferreira, 2021), the use of multi-criteria and multi-risk assessment approaches is fundamental to get a proper contextualisation of the city towards complex and mixed events. The effects of climate change are an illustrative example of this. The work of Quesada-Ganuza et al. (2021), for example, provides a literature-based identification of the link between risk, vulnerability and systems-related aspects that interact in the context of complex climate-change-related events. Besides the multi-criteria comprehensive assessment, Coletti et al. (2020) explores another utmost important aspect in this context, time-dependency, discussing the plausibility of risks according to the evolution of the levels of risk over time. This approach is based on both quantitative and qualitative inputs, which are then used to feed a geographical information database. The outcomes of this analysis present time-dependent scenarios associated with urban functions along the day, assembling a synergic and more detailed risk analysis framework.
2.2 Risk: A Challenging Concept
A complete description of risk must include the likelihood, nature and behaviour of the hazard, the sensitivity that the systems or components of the city have towards the hazard, and the elements that are or can be exposed to damage or loss. There is a minimum set of concepts for approaching any kind of risk. A general and comprehensive conceptual framework is given in the international standard ISO 31000, which offer a series of principles and guidelines for risk management (International Organization for Standardization, 2006). This standard covers all the conceptual basis, from the identification of the risk processes to risk management, mitigation and acceptability. Moreover, several international documents comprehensively address the concept of risk in the context of natural events; the most significant of those are probably the Sendai Framework for Disaster Risk Reduction (UNISDR, 2015). Still in this context, a robust state-of-art review on scientific risk management has been consolidated by the Disaster Risk Management Knowledge Centre of the European Union (Poljanšek et al., 2017).
Generally speaking, risk can be understood as the “uncertainty of achieving objectives due to external factors and influences”. Although this definition is particularly tailored to organisations, it is suitable for analysing almost any organised system, such as urban entities. Considering that the basic premise of a city is to ensure a series of financial, health, safety, environmental and even cultural functions. In that case, the first approach of risk in the context of a historic city is the uncertainty of achieving these functions as a result of external factors and influences, including disruptions and performance diminution. Those functions can include issues as diverse as housing, transit, commerce, leisure, culture, education, etc. From this risk definition, ISO 31000 standard suggest a series of concepts such as:
• Risk evaluation: Process of comparing the results of the risk analysis with risk criteria to determine whether the risk and/or its magnitude is acceptable or tolerable according to the predictable consequences and the admissible perturbations on the urban functions.
• Risk criteria: Terms of reference against which the significance of a risk is evaluated.
• Risk source: The element that, alone or in combination, has the intrinsic potential to give rise to the risk.
• Event: The occurrence or change of a particular set of circumstances.
• Consequence: The outcome of an event affecting objectives.
• Likelihood: The chance of something happening.
Mathematically, risk (R) can be understood as the product (or confluence) of a hazard, i.e., an event that changes a set of circumstances (H), the vulnerability of the city towards the changes imposed by the hazardous event (V), and the elements that are exposed to damage or loss as a consequence of the event (E): R = H ⊗ V ⊗ E (Maio et al., 2018). This qualitative expression can and has been used to frame diverse types of hazards, vulnerability and exposure. The historic city can be read from many points of view, but a very common strategy is to conceptualise it as a configuration of the built space (i.e., taking buildings, infrastructure and anthropic assets as the configuration elements of the city). This codification of the city results useful for determining schematic models that are helpful for understanding the city as a functional organism with interdependent uses, activities and components.
A feasible approach for the functional characterisation of the historic city is the Failure Mode and Event Analysis (FMEA) approach (Shamseddin Alizadeh et al., 2015), which starts from the identification of the subsystems and components of a general system (i.e., the historic city). These subsystems and components are described, including their functions and interactions among subsystems (e.g., the dependencies). Each subsystem is associated with a set of performance requirements (i.e., the agents that sustain the functions of the subsystem) and a set of potential failure modes, usually known as disruptive events or situations. Each failure mode is analysed on its root causes and effects (in the short, medium and long term). Furthermore, the means and methods for detecting the root causes are established, supporting a set of mitigation measures. This approach can be extended and complemented with other analysis tools, such as Failure Tree and Event Tree Analysis, which are specifically aimed to analyse a chained series of events that trigger or are triggered a determinate failure Ramírez Eudave and Ferreira (2021c).
2.3 Main Risks in Urban Areas
It is possible to list a wide variety of relevant hazards associated with the historic city. From those, earthquakes, urban fires and floods have proved to be particularly meaningful due to their impact on buildings, infrastructures and people. In the context of risk and its components, engineering can promote actions for mitigating and reducing the vulnerability of constructions and infrastructure towards these hazardous events. Nevertheless, any intervention must frame hazards in descriptive metrics for measuring their magnitude and impact, identifying the parameters that influence the most the vulnerability of buildings and infrastructure, and, finally, paving the road for mitigating the adverse effects of those event. Figure 1 summarises the three most frequently used descriptors for analysing earthquake, fire and flood risk in urban areas: the metric for measuring the intensity of the event, which can be qualitative, quantitative or semi-quantitative; the vulnerability of the built environment; and the ultimate objective of the analysis in terms of prevention measures, damage limitation, and post-event recover. It is worth noting that, although these descriptions are associated in Figure 1 with earthquake, fire and flood hazards, they are representative and be used to guide risk analyses for other hazard sources.
3 Data-Acquisition Strategies and Assessment Methodologies
According to the HUL approach, consequent risk assessment depends on a representative characterisation of the historic city and, therefore, an adequate data-acquisition approach. A phenomenon can often be assessed and characterised with different approaches and resolutions. As an example, the seismic vulnerability of a building can be measured by resorting to simplified parametric-based procedures, for which a relatively small amount of input data is required, or using highly complex computational models able to simulate the dynamic behaviour of the structure. As comprehensively discussed in Ferreira et al. (2019), the selection of the approaches depends on the availability of resources. The type of approach to use and the way how it will be implemented are two aspects that must be considered carefully before carrying out any assessment.
3.1 Data-Acquisition Strategies: Scales and Tools
One of the most critical challenges in this context is the need of performing observations in different scales for assessing a phenomenon. For example, a comprehensive assessment of the fire vulnerability of a building should include not only specific details of the structural features of the building but also territorial-scale characteristics, such as water availability and distance to firefight infrastructures. This need to acquire multi-scale data from the city makes it essential to use complementary tools and approaches for covering specific scale-related requirements.
Acquiring data in existing buildings usually involves carrying out fieldwork and visual inspection. One of the most common strategies for supporting on-site inspections is to organise the descriptors of the surveyed entity in a datasheet. Among other practical advantages, these datasheets facilitate gathering all the data required for the assessment in an organised and methodological manner. It is important to remark that the design of the survey sheet may compromise the success of the work, and thus datasheets should be clear, straightforward, and tailored to the specificities of the buildings to be assessed. In fact, the selection of descriptors is another crucial aspect to get representative semantic models (Ramírez Eudave and Ferreira, 2021a). Thought to evaluate each construction characteristic relevant for the analysis, several datasheets (such as the one of Blyth et al. (2020) for the city of Leiria, Portugal) were constructed in the form of checklists organised into different categories: building identity and use of the building, general information, openings, layout, elements connected to the façades and also connections and damages Anglade et al. (2020).
On-site inspections have a series of intrinsic advantages, such as data acquisition from a primary source and better conditions for supporting expert judgements. However, these field campaigns are often limited because they require a relatively high investment in terms of time and human resources. Hence, it is convenient to explore strategies for optimising them without losing the quality of on-site observations.
Given the proliferation and accessibility of mobile communication devices (such as tablets and smartphones), it makes sense to explore the potential of their use in site inspection campaigns. These possibilities depart from a relatively simple digitalisation of the datasheets for being fulfilled on-site (using text editors, for example) to the implementation of databases for being feed in real-time. The suitability and complexity of these strategies depend, of course, on a series of determinant conditions, such as the survey’s purpose, the sample’s dimension and the amount of data. A promising aspect of integrating field data acquisition and databases lies in the possibility of involving the so-called Geographic Information Systems (GIS). GIS platforms facilitate the use of several layers of information and relate them to a given spatial context. Since mapping plays a crucial role in presenting vulnerability and risk results, reducing the gap between data acquisition and geographic databases is a very attractive strategy.
Nowadays, there is a wide set of open-source and freeware tools that permit to support entire workflows integrating GIS environments, online data storage (cloud storage) and file distribution in multiple devices. An example of integration was performed for assessing the seismic vulnerability of a series of constructions in Atlixco (Mexico) (Eudave and Miguel Ferreira, 2021). This experience departs from the setup of a GIS database by using an open-source platform, that of QGIS. This software facilitates the addition of geo-referenced layers of information containing several types of data. Then, the database file is stored in a cloud service, Mergin, which offers integrated free storage for QGIS through a dedicated plug-in. Finally, the files stored in Mergin are accessible from mobile devices (based on iOS and Android OS) through a free smartphone app, Input. This app permits to consult and edit the layers of the primary QGIS file, adding data to the attribute tables. These changes in the attribute tables can be synchronised with the file stored in the Mergin service, and, consequently, these changes are immediately visible in the original database. This integration facilitates data treatment and offers an intuitive approach for data-acquisition campaigns (Figure 2), providing thus a more user-friendly environment for users that may be not used to specialised acquisition tools.
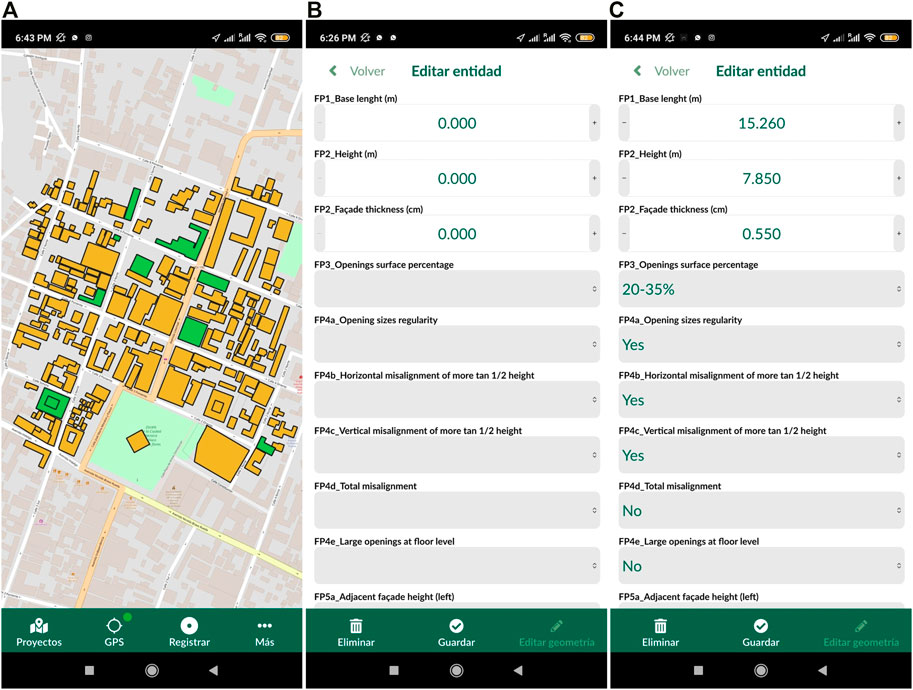
FIGURE 2. Screenshots of the user interface of the Input app. The user interface facilitates using the device’s GPS localisation (A) to associate geographical data to an attribute table where quantitative and qualitative vulnerability-related parameters are programmed (B,C). Adapted from Eudave and Miguel Ferreira (2021).
However, on-site data acquisition can often be replaced and/or complemented with remote-sensing and digital-based data collection methods, mainly when the external observation of the building provides enough information to perform the assessment. As a matter of fact, remote-sensing tools associated with GIS platforms have been increasingly used to get accurate three-dimensional models of the city, opening a broad set of opportunities for integrated and comprehensive urban models utilisable for multiple ends. This situation has aimed the design of common frameworks for sharing information between several digital models for the city and its components, establishing bridges among several platforms and environments.
In this regard, it is worth mentioning the CityGML standard and the Level-of-Detail framework. CityGML is a model of information intended to have a common set of classes and relationships for coding the components of the city (Kutzner et al., 2020). The result is a framework where the city is described as a network of elements defined by geometrical, topological, semantic and visual parameters, and where spatial information is managed and organised more efficiently, facilitating interaction with other standards and urban analysis. The CityGML standard also considers the capacity for representing different levels of granulometry of information according to the Level-of-Detail (LoD), which, through a series of well-defined criteria, establishes thresholds for the generalisations accepted for a model. A more detailed model intrinsically demands more resources during its creation and management. LoD permits a broad spectrum of scales, from low-detailed regional models (LoD 0) to highly detailed building models where building and fittings are modelled with a high level of accuracy (LoD 5). This versatility enables the potential interaction with other types of models at the scale of the building, such as the Building Information Models (BIM). Applicable both to new and existing buildings, BIM can be understood as a set of processes and methodologies that permit managing all the aspects of a building over its entire lifespan. BIM is based on the organisation of components and their relations, allowing to work at different levels, according to the level of detail and the number of processes included in the model. Thanks to the Industry Foundation Classes (IFC) standard, it is feasible to have a shared repository of elements and systems (Donkers et al., 2016). This integration has reached capabilities such as the automatic and semi-automatic conversion of elements among standards, as shown by Donkers et al. (2016).
In Ramírez Eudave and Ferreira (2020), the authors suggest a possible integration of data acquisition strategies, three-dimensional urban models, and BIM files, organised in a general GIS framework. This workflow would facilitate the integration of urban-scale risk assessment strategies that can be further developed and detailed if needed, generating risk-oriented databases inside accumulative and comprehensive GIS models. Figure 3 schematises how the first step for describing the urban environment is the selection of descriptors (i.e., the attributes that are considered as representative). These descriptors must be coded as qualitative, quantitative or semi-quantitative data in correspondence to the physical reality. For example, the height of a building can be a descriptor for a group of constructions. When the height of a specific construction is measured, coded and registered, that descriptor becomes a part of the description of the building. This association can be performed by on-site observations, remote surveys or even documental sources. The set of descriptions is, therefore, a model of the physical entity and can be stored in multiple formats and supports. Among other ends, the model can be used to perform vulnerability assessments if there is coherence and correspondence between the vulnerability assessment approach and the information contained in the model.
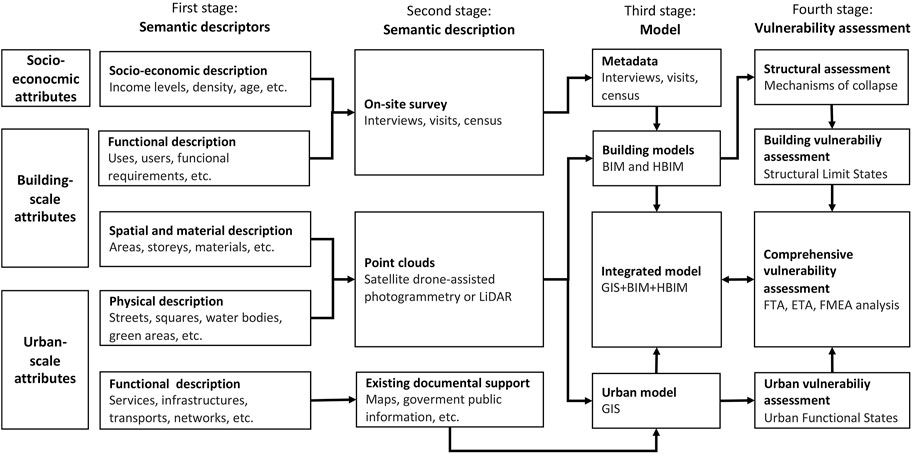
FIGURE 3. Potential multi-scale integration for data-acquisition. Four main stages are considered in this framework for characterising the urban environment: Selection of descriptors, surveying processes, modelling and assessment. The model also contemplates a multi-scale classification for each step. Adapted from Ramírez Eudave and Ferreira (2020).
3.2 Assessing Earthquake, Fire and Flood Risk in Historic Urban Areas
Because of the scale and the amount of data involved, simplified methodologies, often based on sets of empirically-defined evaluation parameters, are usually more adequate to perform large-scale assessments. However, the suitability of using simplified models of the city and its elements depends on multiple factors, such as the limits imposed for processing the information, the heterogeneity among the constructions (e.g., uses, constructive systems, the urban layout, etc.) and the data acquisition capacities. It is convenient to observe that these and some other conditions may constrain the advantages offered by simplified approaches; a successful assessment should be aware of the method’s reliability in the context of its limitations and assumptions.
3.2.1 Earthquake
According to United Nations, earthquakes are the natural hazard with the highest ratio between the amount of material and human losses and the number of events disasters (United Nations Office for Disaster Risk Reduction, 2018). Hence, a good understanding of the seismic vulnerability level of the building stock is critical, particularly in active seismic regions. Assessing the seismic vulnerability of large building stocks is still a demanding task, not only due to the complexity inherent to the assessment itself but also to the difficulty in managing all the data required to characterise the structures accurately. Historic Urban Areas are particularly challenging contexts with different building typologies (often divergent) coexisting in the same space, which significantly limits the resource to any general assumption or generalisation. Nevertheless, the definition of building typologies is not only possible but highly useful in the context of risk assessment, as discussed by Santos et al. (2013) and Salazar and Ferreira (2020). In what regards to masonry buildings, which is the most recurring structural system in Historic Urban areas (Roca et al., 2010), the adoption of masonry-oriented simplified strategies for approaching the historic city has become common.
Gavarini (2001) offers a definition of vulnerability devoted to the structural performance of constructions in historical centres: “the seismic vulnerability of a building is a quantity associated with its ‘weakness’ in front of earthquakes of a given intensity so that the value of this quantity and the knowledge of seismic hazard allow to evaluate the expected damages from future earthquakes”. Vicente et al. (2005) highlight this causal relation when he defines seismic vulnerability as “an intrinsic property of the building structure, a characteristic of its own behaviour to seismic action described by a cause-effect relationship, in which the earthquake is the cause, and the effect is the damage suffered”.
The Gruppo Nazionale per la Difesa dai Terremoti (GNDT-SSN, 1994) is the author of one of the most recognised proposals for assessing the seismic vulnerability of large samples of buildings. From the detailed analysis of large sets of post-earthquake data gathered in the sequence of several seismic events in Italy, GNDT’s proposal grounds on implicit and explicit correlations found between the damages suffered by the buildings and their material, construction and geometrical properties, operationalised through a series of evaluation parameters which allow estimating the seismic vulnerability of the building, see Table 1. According to this formulation, each parameter is evaluated based on four increasing vulnerability classes (A, B, C and D) and weighted through a series of weights, pi whose value illustrates the relative importance of that parameter (building feature) to the overall seismic vulnerability of the building. Although the application of this method is limited to the building typologies for which those correlations were obtained, it has been successfully adapted and applied in countless vulnerability assessment works in many countries and contexts, including Coimbra (Vicente et al., 2011), Seixal (Ferreira et al., 2013), Horta (Ferreira et al., 2017b) and Leiria (Blyth et al., 2020), in Portugal; Annaba, in Algeria Athmani et al. (2015); Timisoara, in Romania (Mosoarca et al., 2019); Osijeck, in Croatia (Hadzima-Nyarko et al., 2016), and Atlixco, in Mexico (Ramírez Eudave and Ferreira, 2021b); which proves the versatility the approach.
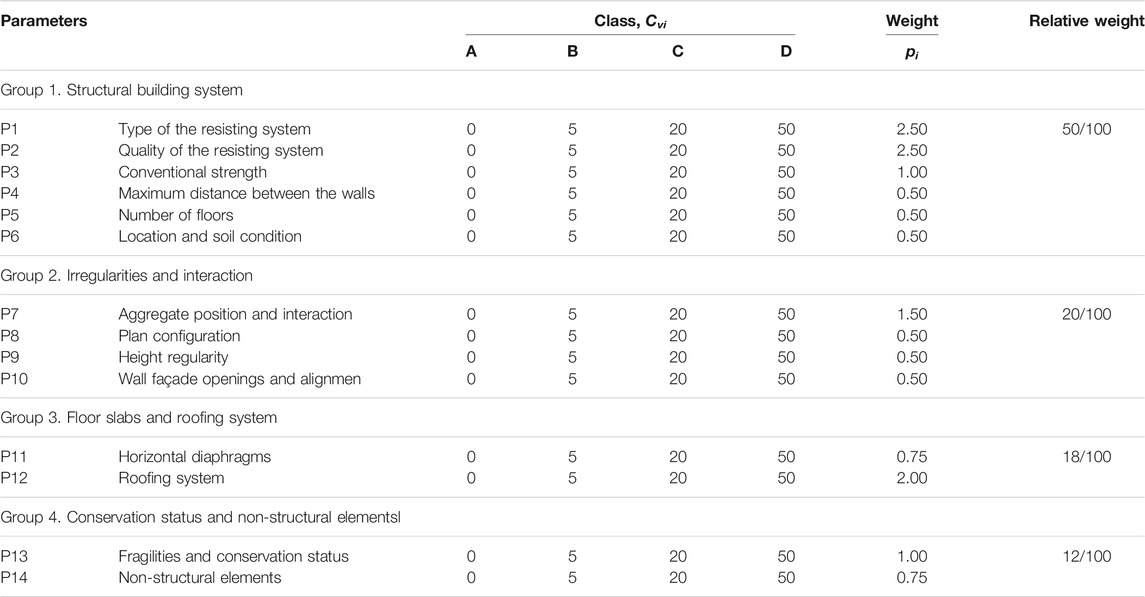
TABLE 1. Vulnerability Index parameters, vulnerability classes, Cvi, and weights, pi, calibrated by Ferreira et al., 2017b.
As presented in Figure 4, the vulnerability, expressed here in the form of a vulnerability curve, makes the bridge between the hazard and the probability of reaching discrete levels of damage for different seismic intensity levels. According to the schematisation illustrated in the figure, for such, a probability density function associated with a discrete seismic hazard value should be first defined. Then, for a given hazard value, and knowing the vulnerability of a building typology, V, a mean damage grade and a dispersion value can be obtained from the vulnerability function, E(D|h)v, and the fragility curves, respectively. Finally, to assess the absolute risk, i.e., to estimate the value of losses, it is necessary to convert damages into losses using damage factors. As an example, to estimate economic losses, a repair cost is associated with each damage state, using a damage factor (dimensionless quantity) defined as the quotient between the repair cost and the replacement cost. Thus, the damage indicators are converted into losses using a loss function, E(L|d)v, and finally, the probability distribution of losses is obtained and described by its probability density function, PL(L>l)v (Vicente, 2008). This vulnerability-based approach makes it relatively easy to estimate the seismic vulnerability of large building stocks, see Figure 5. Given that the expected level of damage is based on the vulnerability of the building and on a deterministic hazard indicator, it is then possible to create scenarios associated with different return periods.
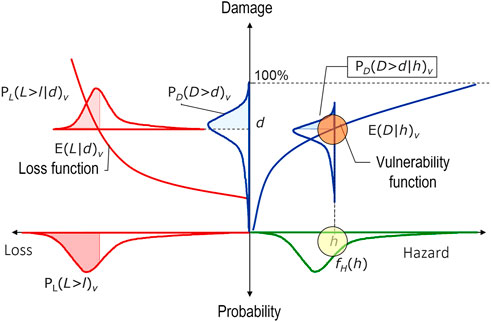
FIGURE 4. Conceptual framework of the vulnerability-based approaches. Adapted from Vicente (2008).
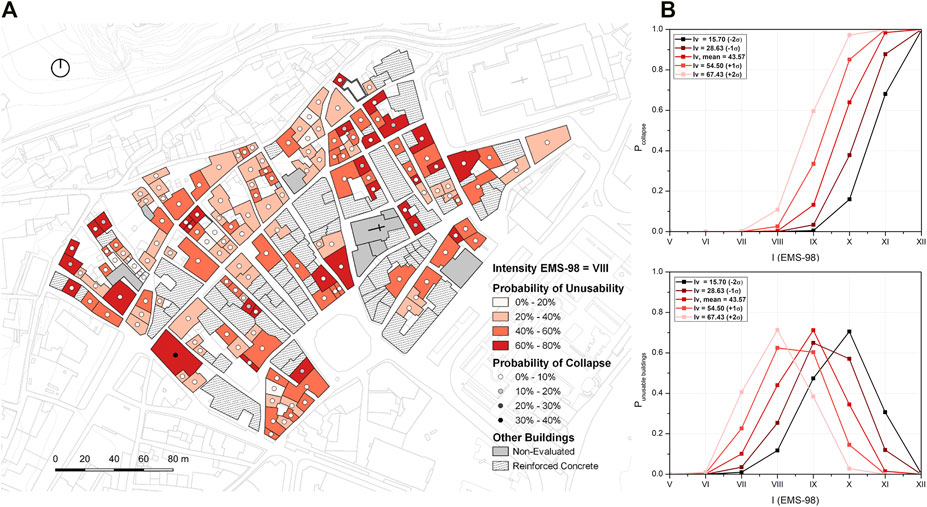
FIGURE 5. Mapping of the probability of collapses for an intensity VIII in the 1998 European Macroseismic Scale (EMS-98) (A), and collapse and building unusable probability curves obtained from the mean value of the vulnerability distribution (B). For details on how these outputs were obtained please refer to Blyth et al. (2020).
The possibility of using this approach to identify characteristic vulnerabilities and to get a feeling of the possible impact resulting from the application of specific seismic retrofitting techniques is another remarkable feature of these index-based approaches, and which makes them very interesting decision-making supporting tools. An example of this can be found in (Ferreira et al., 2017a). In that work, the authors use a simplified index-based approach to evaluate the impact of adopting different large-scale retrofitting strategies, measured in terms of material, human and economic losses. A GIS tool wherein georeferenced graphical information was combined and connected to a relational database containing the main structural characteristics of the buildings was used by the authors to improve results visualisation and interpretation. According to the authors, the work aimed not only to demonstrate how simplified seismic vulnerability assessment approaches can be used to analyse the impacts resulting from the implementation of large-scale retrofitting programs but also to prove that investing in prevention strategies designed to mitigate urban vulnerability is one of the most effective strategies, both from the human and economic standpoint.
The advantage of having a probabilistic-based approach for earthquake-related losses and collapses is that it also facilitates a framework for establishing risk acceptability thresholds based on a series of metrics. It is possible, for example, to estimate the number of buildings that are expected to be unusable after a determined event making it possible, as a result, to estimate the number of homeless people. Or, in the same line, to estimative the number of building collapses and, based on that, the potential number of human casualties. Last but not least, damage indices can be used to estimate replacement costs and, based on that, to assess the economic viability of different seismic rehabilitation interventions and identify the buildings that, due to the extension of the damages, are economically viable to be repaired. If associated with the probability of exceeding certain intensity levels, this procedure allows establishing thresholds for acceptable risk.
Another relevant application of index-based approaches is to analyse the potential accumulation of debris resulting from the partial or global collapse of buildings, which can compromise pedestrian safety in post-earthquake scenarios, see Figure 6. Based on such results, decision-makers can base their decisions on the knowledge of the urban areas that can be potentially inaccessible, which routes can constitute alternatives for evacuation purposes, and where debris removal actions should be concentrated (Bernardini and Ferreira, 2022). Furthermore, urban evacuation scenarios are privileged tools to promote awareness-raising actions among the general population.
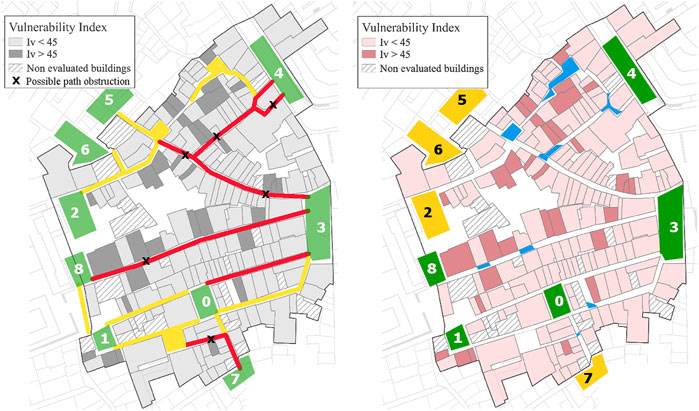
FIGURE 6. Identification of gathering areas (in green and yellow, including identification code 0–8) and of the streets with >50% (red) and <50% (yellow) of the area occupied by debris. Streets blockage positions induced by debris are also identified with an “X”, and “spontaneous” gathering areas are identified in blue (Bernardini and Ferreira, 2021). Maps like these facilitate the identification of critical failures on urban functions in post-event scenarios, providing valuable information for emergency planning and response decisions.
3.2.2 Fire
The characterisation of fire risk in urban areas is a very challenging task due to the many technical attributes involved, including numerous non-linear and multidimensional interactions. This is even more true in the case of existing buildings, not only because the number and the magnitude of the uncertainties involved in the process are more significant but also because most of the existing fire-oriented risk assessment methodologies are devoted to new structures. There are, however, a number of methods that, originally developed to existing structures or not, can be applied for that end (Julià and Ferreira, 2021). A very suitable method in the context of historic city centres is the ARICA method—an acronym for “Fire-risk Assessment in Ancient City Centres” (“Avaliação do Risco de Incêndio em Centros Urbanos Antigos”, in Portuguese). Based on the Portuguese fire safety code (Granda and Ferreira, 2019), this method is based on the evaluation of four global fire risk factors: Three global risk factors and an efficiency factor (FGE), covering all the fire cycle from ignition and development to evacuation and combat, see Figure 7.
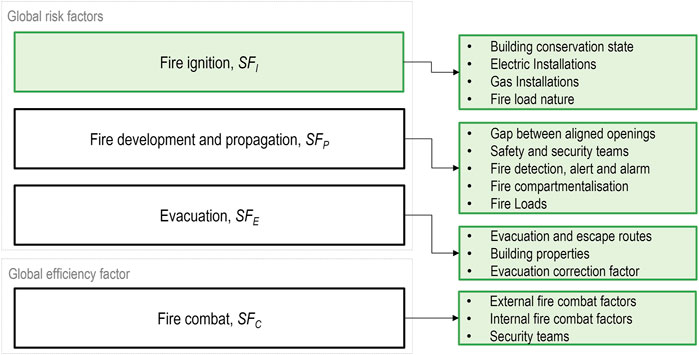
FIGURE 7. Global Risk Factors and Global Efficiency Factors and their corresponding partial factors. Adapted from (Granda and Ferreira, 2019).
In this semi-quantitative method, each of the parameters listed in Figure 7—all associated to a quantitative value that reflects the degree of adequacy of the building in relation to a series of features prescribed in the Portuguese Fire Safety code—is evaluated individually. For the sake of example, the partial factor “Fire detection, alert and alarm” can take a value between 0.50 and 2.00, where 0.50 represents the best-case scenario of a building with an operational automatic fire detection system, and 2.00 corresponds to the worst-case scenario of a building with no fire detection, alert and alarm system in place. Then, by dividing the weighted average of the four sub-factors mentioned above by a reference risk factor related to the building’s use, it is possible to get a fire risk indicator, FRI, which is directly relatable to the level of fire safety equivalent to that corresponding to full compliance with the Portuguese Fire Safety code. Despite the simplifications involved, the results obtained with this approach are valuable for providing fire risk maps similar to the ones shown in Figure 8 (left), which present the results of the application of the ARICA method to the 436 buildings that compose the Historic City Centre of Guimarães (Granda and Ferreira, 2019).
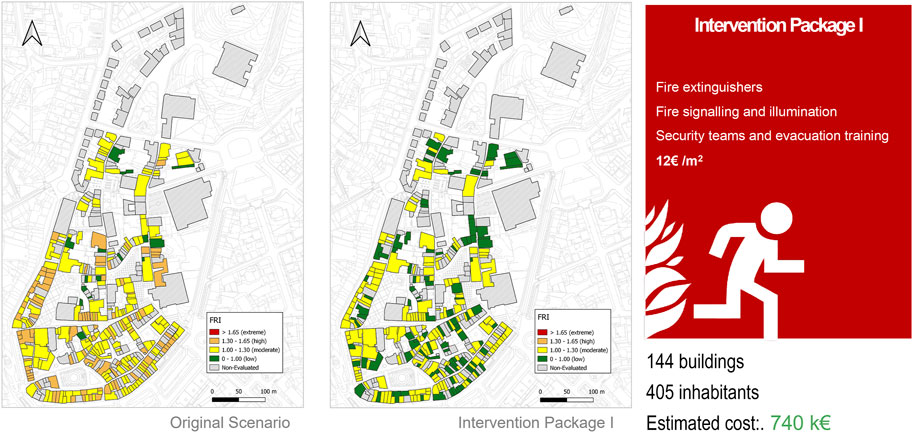
FIGURE 8. Cost-benefit analysis of implementing an intervention package for the city of Guimarães. Adapted from Tozo Neto and Ferreira (2020).
Similarly to what was discussed earlier for the index-based seismic vulnerability assessment approaches, the ARICA method can also be used to identify the areas of the city where there is a higher concentration of vulnerable buildings, as well as to quantify the potential human and economic benefit resulting from the application of different retrofitting strategies (Tozo Neto and Ferreira, 2020), see Figure 8. Also in this case, the cost-benefit analysis is conducted by emulating a series of actions specifically tailored to address the vulnerabilities identified in the partial factor assessment. In this specific case of the example illustrated in Figure 8, Intervention Package I (IP-I) included fire risk mitigation interventions possible to be applied in a straightforward and not expensive way, without the need for any modification in the existing structure. It is composed of essential fire safety items, such as fire extinguishers and signalling, including also measures related to risk perception and training. From this analysis, the authors concluded that the adoption of the set of measures included in this IP-I would lead to a significant reduction of the level of fire risk identified in the Original Scenario (Figure 8, left). According to the authors, and as can be observed in Figure 8, the number of buildings identified with low-risk increased from 16 (about 6% of the total) to 108 (about 40% of the total). Moreover, the buildings that in the Original Scenario were categorised with high risk saw their level of risk reduced to moderate with the application of these measures.
As can be easily understood from this example, this process allows simulating a set of generic actions that would be suitable for mitigating a number of vulnerability-related aspects under the basis of parametric costs (e.g., based on units of area) that can be easily calculated. And this can be repeated and reimplemented as many times as necessary until an acceptable level of risk is reached, constituting thus a flexible and relatively simple approach for defining risk management policies.
3.2.3 Flood
Though flooding can be associated with several sources, it is often related to heavy rainfalls and the lack of capacity of natural watercourses for conveying the water flow (Julià and Ferreira, 2021). There are two fundamental types of approaches to assess flood risk: those focused on analysing the hazard and those focused on the vulnerability assessment of the element at risk. The hazard-oriented approaches are primarily based on parameters as the extent, velocity and depth of the flows, whereas the vulnerability-oriented approaches are based on the characterisation of the elements exposed to the flood (e.g., buildings and/or infrastructures) for obtaining a flood vulnerability indicator that mirrors the propensity of these elements to withstand with the flood with more or less damage. Although these two approaches are very different in scope and purpose, they can both be used to get valuable risk-related outputs, either when used independently or together, as per the example shown in Figure 9 where a flood hazard and a flood vulnerability indicator are put together to get a flood risk matrix. The components of the hazard module are the maximum extent of the flood (for a 100-year peak flow scenario), the average surface velocity of the flow (in m/s) and the maximum depth (the distance between the floor and the surface of water) associated with the maximum extent. The vulnerability module assesses the sensibility of the construction (based on its heritage status, age, number of storeys, condition and material of the building) and the level of exposure (partial or total; with or without openings) of a wall towards the water flow. The detailed criteria for grading each parameter can be found in (Ferreira and Santos, 2020).
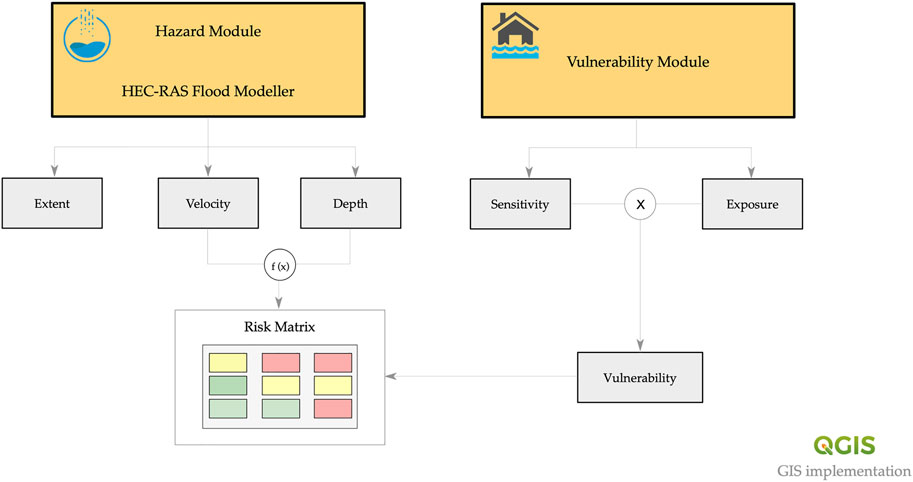
FIGURE 9. Conceptual framework of the flood risk assessment approach proposed by Ferreira and Santos (2020). The final risk matrix is obtained from the combination of hazard and vulnerability results.
In the approach schematised in Figure 9, the flood hazard was assessed using the hydraulic method. The assessment process involved acquiring and preparing geometric data, estimating the peak flow, hydraulic modelling, and GIS post-processing and mapping. Regarding flood vulnerability, it was assessed using the simplified flood vulnerability assessment methodology proposed by (Miranda and Ferreira, 2019). This methodology is based on the evaluation of two vulnerability components, an exposure and a sensitivity component, which, through an index, quantify the vulnerability of the building to flood inundation. Whereas the exposure component is given by one single parameter/feature, the orientation of the front wall of the building, the sensitivity component brings together a series of parameters related to the physical characteristics of the buildings, namely its type of structural system, conservation state, number of storeys, age, and heritage status.
Moreover, one of the significant advantages of this type of index-based approaches in general, and this one in particular, is the possibility of crossing and analysing together not only the final hazard and vulnerability indicators but the intermediate hazard outputs and building features that led to those indicators, as explored and discussed by (Ferreira and Santos, 2020). An illustrative example of this is the joint analysis of the water depth estimation for a specific peak flow scenario and the exposure and conservation state of the buildings, shown in Figure 10. Such an output allows getting a feeling of the buildings that are likely to be affected for that specific scenario and, most importantly, those likely to be more affected due to their higher level of exposure and poorer conservation state. The assessment of these features is, of course, intimately related to the three-dimensional configuration of the city, thus favouring the use of GIS environments.
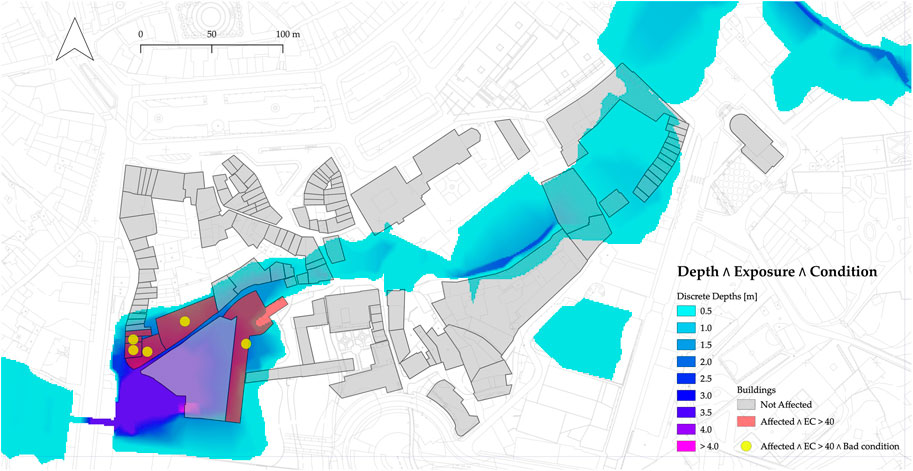
FIGURE 10. Example of a joint analysis of water depth, exposure, and condition (i.e., buildings’ conservation state) indicators (Ferreira and Santos, 2020). This kind of output makes it easy to analyse flood risk scenarios from the combination of hazard and physical vulnerability-related results.
Despite the empirical nature of the methodology, it can be extremely valuable to support decision-making processes by making it easy to compare and identify the assets at higher risk, as explained before. Final risk matrices play an essential part in this decision-making process by providing an additional layer of information related to the level of risk decision-makers and stakeholders are willing to accept, see Figure 11. The number and thresholds for the levels of risk can be decided according to a broad series of criteria, facilitating the use of this tool for establishing bridges among stakeholders. Last but not least, this type of approach can also be used in a simple but effective way to analyse how different flood risk mitigation interventions, at the building or the urban scale, can impact the level of risk associated with each construction. Furthermore, this approach can support emergency planning and response actions, namely those related to evacuations and immediate actions; for example, (Quagliarini et al., 2022).
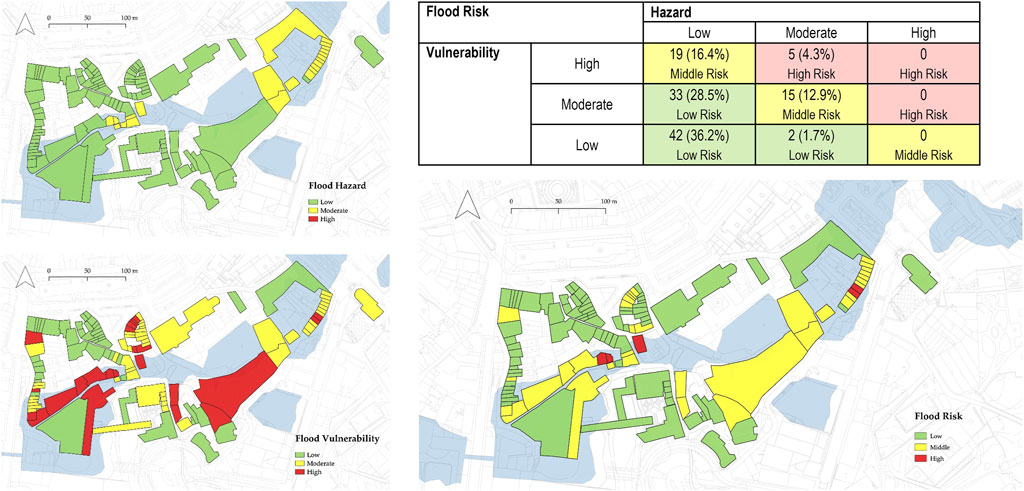
FIGURE 11. Example of a flood risk matrix based on the combination of the level of Hazard and Vulnerability obtained for each building. The numbers and the percentages included in the tables represent the number and percentage of buildings categorised with that specific level of risk.
4 Building Knowledge as a Part of Resilience Enhancing Processes: The Use of Artificial Intelligence Approaches
The methodologies reviewed succinctly in the above sections have resulted from a thorough analysis of several seismic, fire and flood events and various applications to case study areas in various contexts and geographies. Although this is done today using stabilised scientific processes and taking advantage of state-of-the-art numerical tools, the idea of learning from past destructive events and applying that knowledge in construction practice is far from new. As a matter of fact, this was common practice before the advent of modern science and, therefore, it is mirrored in a very clear way in intervention, uses, configurations and technologies used for centuries in our city. Just as an example, the destruction of the city of Lisbon after the 1755 Earthquake led to the development of a new type of seismic-resistant structural system known as “gaiola Pombalina” or Pombaline cage, in a direct translation to the English (Ferreira et al., 2017a). The diversity of structural systems and adaptative strategies is the living example of resilience towards adverse situations and conditions (Baquedano et al., 2021). Hence, building significant learning from the basis of past events is not only a proactive task but a reactive part of long-term resilience and adaptation processes.
As seen in the previous sections, large-scale vulnerability assessment methods are often based on a few empirical-based parameters defined through the statistical analysis of large sets of post-event damage data, usually resorting to very simple mathematic expressions. However, the inability of these traditional methods to handle missing or noisy data or to identify certain behaviour patterns opens up space to the use of innovative computer-based solutions. In the last couple of years, artificial intelligence-based approaches are becoming increasingly popular in many civil engineering applications (Ferreira et al., 2020). Such approaches, which include Machine Learning, Heuristics, Metaheuristics or Bayesian networks, just to mention some, are extremely valuable for understanding complex events, classifying data and optimising resources. These tools are especially useful for supporting processes in which it is difficult to guess the origin of a determinate outcome that may result from different processes. To give an example outside the scope of this paper’s topic, this is the case of the identification between viral and bacterial meningitis, in which artificial intelligence may play a relevant role when advanced clinical tools are not available (D’Angelo et al., 2019).
The assessment of the effects of different types of hazards is one of the many fields in which these approaches may be potentially valuable. In fact, it is possible to identify many works in the literature that can be easily relatable to common issues identified in urban risk analyses. Pasha et al. (2020), for example, have studied how to optimise a route based on several parameters. Although this study is applied to supply chain-related problems, the rationale behind the strategy outlined by the authors also applies to disaster scenarios where supply networks and vehicle logistics are conditioned for numerous aspects determined by the loss or significant reduction of urban functions. In the same line, efficiency-related decisions during emergency evacuations have been explored by Dulebenets et al. (2019). This model proposes the characterisation of vulnerable population groups, emergency shelters, evacuation routes, traffic conditions, etc., in order to minimise individuals’ travel time and to prioritise groups for which specific vulnerability indicators have been found.
Following this very same rationale, the use of robust statistic approaches and the selective systematisation of data has permitted a better understanding of the nature of risk, particularly nowadays with the exponential growth of machine learning, data mining and artificial intelligence applications. In fact, even if the significance of empirical knowledge is undeniable, the wide range of increasingly powerful and cheaper computational resources available today open possibilities that were not only unreachable but unimaginable just a few decades ago.
In the specific case of vulnerability and risk assessment methodologies, when compared with traditional statistical methods, innovative computed-based approaches present the great advantage of being able to handle missing or noisy data and managing nonlinearities and identifying specific behaviour patterns hardly identifiable through traditional approaches (Ferreira et al., 2020). This is not only valid for developing new methodologies or assessment approaches but also for calibrating existent ones, as discussed by (Ferreira et al., 2020) where the authors compared the performance of a traditional vulnerability index methodology and an innovative approach based on Artificial Neural Networks (ANNs) though comparing their results with real post-earthquake damage observation. As schematised in Figure 12, the characterisation of past hazardous events and the finding of the attributes that may have conditioned the ability of the buildings to cope with them can be a valuable asset towards finding reliable and accurate vulnerability indicators. Each one of the letters in Figure 12 represents a building representative of a specific typology, such as, for example, a traditional masonry building with granite stone walls and wooden floors. The buildings labelled with the letters A to K has been subjected to a seismic event. From those, A to G revealed an adequate seismic performance (with different levels of damage), whereas H to K collapsed. The characteristics of all these buildings were then assessed to find statistically significant features shared between the buildings included in the first and in the second group. Through this process, it is possible to isolate and understand the factors that influence the most the seismic performance of that specific building typology. Finally, the assessment of those characteristics on buildings with an unknown level of seismic vulnerability (L to V) would allow estimating the level of damage that those buildings would likely suffer if subjected to a seismic event similar to the one(s) used in the analysis.
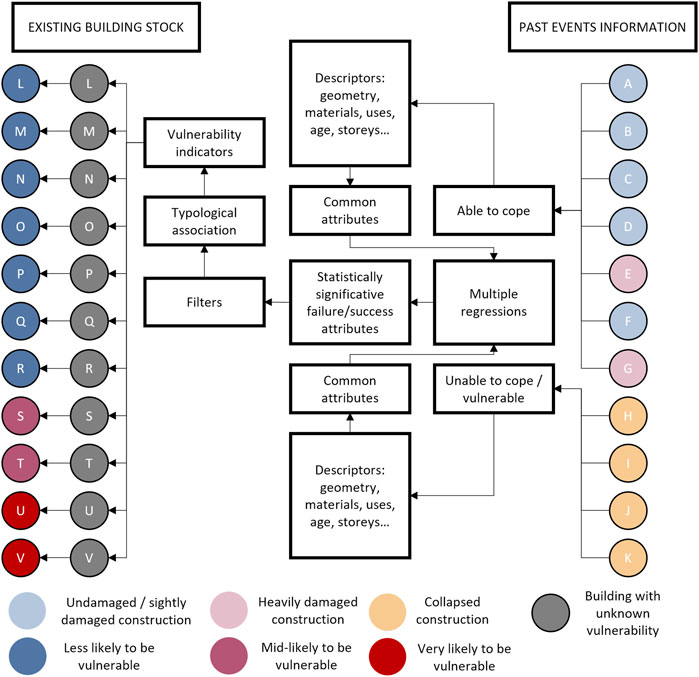
FIGURE 12. Example of workflow for analysing past-events data for assessing existing buildings. Buildings on the right side (letters A to K) were assessed after a determined seismic event, feeding a series of processes (in the middle) to find the typology’s vulnerability sources. Buildings with an unknown level of vulnerability (letters L to V) are compared with these vulnerability sources to identify the structures that are potentially more prone to damage in the sequence of a future seismic event.
Furthermore, exciting advances in the use of Multiobjective Evolutionary Algorithms (MOEAs), a framework for conducting comparatives in which the sensitivity of inputs and robustness of models can be tested, has been recently observed. The work of Zhi-Zhong et al. (Liu et al., 2020) illustrates how such a framework can be used to overcome some of the limitations of Many-Objective Problems (MaOP). The quality of the solutions depends on the obtention of the problem features—a task that can, itself, be done using learning automatons (Zhao and Zhang, 2020).
5 Final Remarks
Built cultural heritage is at increasing risk due to climate change and anthropic actions. The frequency and severity of extreme events are rising, imposing new challenges on traditional risk management practices in urban areas. Since disasters will continue to occur, it is necessary to anticipate those challenges by forecasting potential scenarios in the most accurate and reliable way possible—and risk assessment is the key for that. Nowadays, digital tools facilitate many tasks related to risk identification, assessment and management, such as geometric survey, damage mapping, monitoring, vulnerability assessment, management and more. Despite the level of uncertainty associated with vulnerability assessment methodologies based on statistical approaches and damage observation, they offer a very interesting compromise between the amount of input data and computational effort required to perform the analysis and the reliability of the results produced, which make them very suitable tools for assessing large urban areas. Furthermore, the use of geographical databases and the assistance of remote-sensing tools are opening a vast horizon on interoperability and comprehensiveness for urban-scale models. As discussed in the present article:
• Simplified vulnerability and risk assessment approaches can play a significant role in providing preliminary scenarios and pointing the way to the definition of more efficient and customised strategies for managing and mitigating risk. The knowledge built on the consequences of past events permits refining and calibrating our present-day vulnerability and risk models, allowing us to understand better the phenomena involved in these processes. This is relevant not only for the decision-making process, allowing for more accurate and effective decisions, but also because it promotes better engagement of stakeholders and professionals with different expertise and backgrounds, which is critical towards promoting safer and more sustainable urban areas. Despite so, it is important to acknowledge that these approaches are based on several assumptions an generalisations, which must be understood and recognised. Although they are very handy and suitable to assess large datasets, they are likely to overcome important local phenomena or conditions, which may completely change the outcome of the analysis. In this sense, the implementation of these approaches must always be preceded by a discussion about its suitability and pertinence according to the context.
• As addressed in Section 4, artificial intelligence-based approaches are extremely promising towards getting more accurate and reliable risk assessment models. However, it is crucial to recognise and acknowledge the need for more and better training data, under the risk of the results obtained from these models can be dangerously misleading.
The present study addresses strategies for characterising, assessing and treating risk in historic urban areas. Most of the experiences discussed herein are still to be fully established or validated, which means that their potential is not entirely exploited yet, or even understood in some cases. The potential for improvement and development in this field is, therefore, readiness, and new, more accurate, and more excited risk assessment approaches able to overcome the limitations of the current ones can be just around the corner.
Data Availability Statement
The original contributions presented in the study are included in the article/Supplementary Material, further inquiries can be directed to the corresponding author.
Author Contributions
Conceptualisation, TMF; investigation, TMF and RRE; writing—original draft preparation, RRE and TMF; writing—review and editing, TMF and RRE; supervision, TMF; funding, TMF. All authors have read and agreed to the published version of the manuscript.
Funding
The project “MIT-RSC-Multi-risk Interactions Towards Resilient and Sustainable Cities” (MIT-EXPL/CS/0018/2019) leading to this work is co-financed by the ERDF-European Regional Development Fund through the Operational Program for Competitiveness and Internationalisation-COMPETE 2020, the North Portugal Regional Operational Program-NORTE 2020 and by the Portuguese Foundation for Science and Technology-GCT under the MIT Portugal Program at the 2019 PT call for Exploratory Proposals in “Sustainable Cities”. Rafael Ramírez Eudave is funded by the Portuguese Foundation for Science and Technology (FCT) through the grant number PD/ BD/150385/2019.
Conflict of Interest
The authors declare that the research was conducted in the absence of any commercial or financial relationships that could be construed as a potential conflict of interest.
Publisher’s Note
All claims expressed in this article are solely those of the authors and do not necessarily represent those of their affiliated organizations, or those of the publisher, the editors and the reviewers. Any product that may be evaluated in this article, or claim that may be made by its manufacturer, is not guaranteed or endorsed by the publisher.
References
Anglade, E., Giatreli, A.-M., Blyth, A., Di Napoli, B., Parisse, F., Namourah, Z., et al. (2020). Seismic Damage Scenarios for the Historic City Center of Leiria, Portugal: Analysis of the Impact of Different Seismic Retrofitting Strategies on Emergency Planning. Int. J. Disaster Risk Reduction 44, 101432. doi:10.1016/j.ijdrr.2019.101432
Angrisano, M., Biancamano, P. F., Bosone, M., Carone, P., Daldanise, G., De Rosa, F., et al. (2016). Towards Operationalizing UNESCO Recommendations on”Historic Urban Landscape”: A Position Paper. Aestimum 69, 165–210. doi:10.13128/Aestimum-20454
Athmani, A. E., Gouasmia, A., Ferreira, T. M., Vicente, R., and Khemis, A. (2015). Seismic Vulnerability Assessment of Historical Masonry Buildings Located in Annaba City (Algeria) Using Non Ad-Hoc Data Survey. Bull. Earthquake Eng. 13, 2283–2307. doi:10.1007/s10518-014-9717-7
Baquedano, P., Eudave, R. R., Miranda, F. N., Graus, S., and Ferreira, T. M. (2021). “Traditional Earth Construction in Latin America: A Review on the Construction Systems and Reinforcement Strategies,” in Masonry Construction in Active Seismic Regions (Cambridge, United Kingdom: Elsevier, Woodhead Publishing), 99–121. doi:10.1016/B978-0-12-821087-1.00011-9
Bernardini, G., and Ferreira, T. M. (2021). Combining Structural and Non-structural Risk-Reduction Measures to Improve Evacuation Safety in Historical Built Environments. Int. J. Architectural Heritage 1–19. doi:10.1080/15583058.2021.2001117
Bernardini, G., and Ferreira, T. M. (2022). “Emergency and Evacuation Management Strategies in Earthquakes: towards Holistic and User-Centered Methodologies for Their Design and Evaluation,” in Seismic Vulnerability Assessment of Civil Engineering Structures at Multiple Scales (Cambridge, United Kingdom: Elsevier, Woodhead Publishing), 275–321. doi:10.1016/B978-0-12-824071-7.00002-0
Blyth, A., Di Napoli, B., Parisse, F., Namourah, Z., Anglade, E., Giatreli, A. M., et al. (2020). Assessment and Mitigation of Seismic Risk at the Urban Scale: an Application to the Historic City center of Leiria, Portugal. Bull. Earthquake Eng. 18, 2607–2634. doi:10.1007/s10518-020-00795-2
Chmutina, K., and von Meding, J. (2019). A Dilemma of Language: “Natural Disasters” in Academic Literature. Int. J. Disaster Risk Sci. 10, 283–292. doi:10.1007/s13753-019-00232-2
Coletti, A., De Nicola, A., Di Pietro, A., La Porta, L., Pollino, M., Rosato, V., et al. (2020). A Comprehensive System for Semantic Spatiotemporal Assessment of Risk in Urban Areas. J. Contingencies Crisis Management 28, 178–193. doi:10.1111/1468-5973.12309
D’Angelo, G., Pilla, R., Tascini, C., and Rampone, S. (2019). A Proposal for Distinguishing between Bacterial and Viral Meningitis Using Genetic Programming and Decision Trees. Soft Comput. 23, 11775–11791. doi:10.1007/s00500-018-03729-y
de Carvalho, N. L. A., Ribeiro, P. C. C., García-Martos, C., Fernández, C. G., and Vieira, J. G. V. (2019). Urban Distribution Centres in Historical Cities from the Perspective of Residents, Retailers and Carriers. Res. Transportation Econ. 77, 100744. doi:10.1016/j.retrec.2019.100744
Donkers, S., Ledoux, H., Zhao, J., and Stoter, J. (2016). Automatic Conversion of IFC Datasets to Geometrically and Semantically Correct CityGML LOD3 Buildings. Trans. GIS 20, 547–569. doi:10.1111/tgis.12162
Dulebenets, M. A., Pasha, J., Abioye, O. F., Kavoosi, M., Ozguven, E. E., Moses, R., et al. (2019). Exact and Heuristic Solution Algorithms for Efficient Emergency Evacuation in Areas with Vulnerable Populations. Int. J. Disaster Risk Reduction 39. doi:10.1016/j.ijdrr.2019.101114
Egusquiza, A., Prieto, I., Izkara, J. L., and Béjar, R. (2018). Multi-scale Urban Data Models for Early-Stage Suitability Assessment of Energy Conservation Measures in Historic Urban Areas. Energy and Buildings 164, 87–98. doi:10.1016/j.enbuild.2017.12.061
Eudave, R., and Ferreira, T. M. (2021). “Proposal for a Suitable Workflow for Assessing the Seismic Vulnerability of Historical Buildings. Atlixco (Puebla, México) as a Case Study,” in 1st Croatian Conference on Earthquake Engineering (Zagreb, Croatia: University of Zagreb Faculty of Civil Engineering), 23–34. doi:10.5592/CO/1CroCEE.2021.22
Ferreira, T. M., Estêvão, J., Maio, R., and Vicente, R. (2020). The Use of Artificial Neural Networks to Estimate Seismic Damage and Derive Vulnerability Functions for Traditional Masonry. Front. Struct. Civil Eng. 14, 609–622. doi:10.1007/s11709-020-0623-6
Ferreira, T. M., Maio, R., and Vicente, R. (2017a). Analysis of the Impact of Large Scale Seismic Retrofitting Strategies through the Application of a Vulnerability-Based Approach on Traditional Masonry Buildings. Earthquake Eng. Eng. Vibration 16, 329–348. doi:10.1007/s11803-017-0385-x
Ferreira, T. M., Maio, R., and Vicente, R. (2017b). Seismic Vulnerability Assessment of the Old City centre of Horta, Azores: Calibration and Application of a Seismic Vulnerability index Method. Bull. Earthquake Eng. 15, 2879–2899. doi:10.1007/s10518-016-0071-9
Ferreira, T. M., Mendes, N., and Silva, R. (2019). Multiscale Seismic Vulnerability Assessment and Retrofit of Existing Masonry Buildings. Buildings 9. doi:10.3390/buildings9040091
Ferreira, T. M., and Santos, P. P. (2020). An Integrated Approach for Assessing Flood Risk in Historic City Centres. Water (Switzerland) 12, 1648. doi:10.3390/w12061648
Ferreira, T. M., Vicente, R., Mendes da Silva, J. A., Varum, H., and Costa, A. (2013). Seismic Vulnerability Assessment of Historical Urban Centres: Case Study of the Old City centre in Seixal, Portugal. Bull. Earthquake Eng. 11, 1753–1773. doi:10.1007/s10518-013-9447-2
García-Hernández, M., de la Calle-Vaquero, M., and Yubero, C. (2017). Cultural Heritage and Urban Tourism: Historic City Centres under Pressure. Sustainability (Switzerland) 9. doi:10.3390/su9081346
Gavarini, C. (2001). Seismic Risk in Historical Centers. Soil Dyn. Earthquake Eng. 21, 459–466. doi:10.1016/S0267-7261(01)00027-6
GNDT-SSN (1994). Scheda di Esposizione e Vulnerabilità e di Rilevamento Danni di Primo e Secondo Livello (Murata e Cemento Armato). Tech. rep.Rome, Italy: GNDT-SSN.
Granda, S., and Ferreira, T. M. (2019). Assessing Vulnerability and Fire Risk in Old Urban Areas: Application to the Historical Centre of Guimarães. Fire Technology 55, 105–127. doi:10.1007/s10694-018-0778-z
Hadzima-Nyarko, M., Pavić, G., and Lešić, M. (2016). Seismic Vulnerability of Old Confined Masonry Buildings in Osijek, Croatia. Earthquake and Structures 11, 629–648. doi:10.12989/eas.2016.11.4.629
International Organization for Standardization (2006). ISO 31000 Risk Management — Principles and Guidelines. 61010-1 © Iec:2001 2006, 13.
Julià, P. B., and Ferreira, T. M. (2021). From Single- to Multi-hazard Vulnerability and Risk in Historic Urban Areas: A Literature Review. Springer Netherlands, 0123456789. doi:10.1007/s11069-021-04734-5
Kutzner, T., Chaturvedi, K., and Kolbe, T. H. (2020). CityGML 3.0: New Functions Open up New Applications. PFG - J. Photogrammetry, Remote Sensing Geoinformation Sci. 88, 43–61. doi:10.1007/s41064-020-00095-z
Liu, Z. Z., Wang, Y., and Huang, P. Q. (2020). AnD: A many-objective Evolutionary Algorithm with Angle-Based Selection and Shift-Based Density Estimation. Inf. Sci. 509, 400–419. doi:10.1016/j.ins.2018.06.063
Maio, R., Ferreira, T. M., and Vicente, R. (2018). A Critical Discussion on the Earthquake Risk Mitigation of Urban Cultural Heritage Assets. Int. J. Disaster Risk Reduction 27, 239–247. doi:10.1016/j.ijdrr.2017.10.010
Miranda, F. N., and Ferreira, T. M. (2019). A Simplified Approach for Flood Vulnerability Assessment of Historic Sites. Nat. Hazards 96, 713–730. doi:10.1007/s11069-018-03565-1
Mosoarca, M., Onescu, I., Onescu, E., Azap, B., Chieffo, N., and Szitar-Sirbu, M. (2019). Seismic Vulnerability Assessment for the Historical Areas of the Timisoara City, Romania. Eng. Fail. Anal. 101, 86–112. doi:10.1016/j.engfailanal.2019.03.013
Moustafa, L. H. (2016). Cultural Heritage and Preservation: Lessons from World War II and the Contemporary Conflict in the Middle East. The Am. Archivist 79, 320–338. doi:10.17723/0360-9081-79.2.320
M. Poljanšek, M. Marin Ferrer, T. De Groeve, and I. Clark (Editors) (2017). Science for Disaster Risk Management 2017. Knowing Beter and Losing Less (Luxembourg: Publications Office of the European Union). EUR 28034 EN. doi:10.2788/842809
Pasha, J., Dulebenets, M. A., Kavoosi, M., Abioye, O. F., Wang, H., and Guo, W. (2020). An Optimization Model and Solution Algorithms for the Vehicle Routing Problem with a ’Factory-In-A-Box. IEEE Access 8, 134743–134763. doi:10.1109/ACCESS.2020.3010176
Quagliarini, E., Romano, G., Bernardini, G., and D’Orazio, M. (2022). Leaving or Sheltering? a Simulation-Based Comparison of Flood Evacuation Strategies in Urban Built Environments. 113–123. doi:10.1007/978-981-16-6269-0_10
Quesada-Ganuza, L., Garmendia, L., Roji, E., and Gandini, A. (2021). Do we Know How Urban Heritage Is Being Endangered by Climate Change? A Systematic and Critical Review. Int. J. Disaster Risk Reduction 65, 102551. doi:10.1016/j.ijdrr.2021.102551
Ramírez Eudave, R., and Ferreira, T. M. (2021b). On the Potential of Using the Mexican National Catalogue of Historical Monuments for Assessing the Seismic Vulnerability of Existing Buildings: a Proof-Of-Concept Study. Bull. Earthquake Eng. 19, doi:10.1007/s10518-021-01154-5
Ramírez Eudave, R., and Ferreira, T. M. (2021a). Characterisation of the Historic Urban Landscape through the Aristotelian Four Causes: Towards Comprehensive GIS Databases. Remote Sensing 13, 1879. doi:10.3390/rs13101879
Ramírez Eudave, R., and Ferreira, T. M. (2020). On the Suitability of a Unified GIS-BIM-HBIM Framework for Cataloguing and Assessing Vulnerability in Historic Urban Landscapes: a Critical Review. Int. J. Geographical Inf. Sci. 00, 1–31. doi:10.1080/13658816.2020.1844208
Ramírez Eudave, R., and Ferreira, T. M. (2021c). Towards a Semi-quantitative Approach for Assessing Evacuation Scenarios in the Context of Popocatépetl Volcano, México—The Case of San Pedro Tlalmimilulpan. GeoHazards 2, 1–16. doi:10.3390/geohazards2010001
Rey-Pérez, J., and Pereira Roders, A. (2020). Historic Urban Landscape: A Systematic Review, Eight Years after the Adoption of the HUL Approach. J. Cult. Heritage Management Sustainable Development 10, 233–258. doi:10.1108/JCHMSD-05-2018-0036
Roca, P., Cervera, M., Gariup, G., and Pela’, L. (2010). Structural Analysis of Masonry Historical Constructions. Classical and Advanced Approaches. Arch. Comput. Methods Eng. 17, 299–325. doi:10.1007/s11831-010-9046-1
Salazar, L. G. F., and Ferreira, T. M. (2020). Seismic Vulnerability Assessment of Historic Constructions in the Downtown of Mexico City. Sustainability (Switzerland) 12, 1–21. doi:10.3390/su12031276
Santos, C., Ferreira, T. M., Vicente, R., and Mendes da Silva, J. R. (2013). Building Typologies Identification to Support Risk Mitigation at the Urban Scale – Case Study of the Old City centre of Seixal, Portugal. J. Cult. Heritage 14, 449–463. doi:10.1016/j.culher.2012.11.001
Shamseddin Alizadeh, S., Rasoulzadeh, Y., and P Moshashaie, S. V. (2015). Failure Modes and Effects Analysis (FMEA) Technique: A Literature Review. Scientific J. Rev.doi:10.14196/sjr.v4i1.1805
Tozo Neto, J., and Ferreira, T. M. (2020). Assessing and Mitigating Vulnerability and Fire Risk in Historic Centres: A Cost-Benefit Analysis. J. Cult. Heritage 45, 279–290. doi:10.1016/j.culher.2020.04.003
UNESCO (2016). The HUL Guidebook: Managing Heritage in Dynamic and Constantly Changing Urban Environments: A Practical Guide to UNESCO’s Recommendation on the Historic Urban Landscape. Paris, France. 59.
UNISDR (2015). The Sendai Framework for Disaster Risk Reduction 2015-2030: The challenge for Science. Sendai, Japan: Royal Society Meeting Note. 9. March 18, 2015.
United Nations Office for Disaster Risk Reduction (2018). Economic Losses, Poverty and DISASTERS 1998-2017. Geneva, Switzerland.
Vicente, R. (2008). Estratégias e metodologias para intervenções de reabilitação urbana. Avaliação da vulnerabilidade e do risco sísmico do edificado da Baixa de Coimbra. Aveiro, Portugal: Phd thesis, University of Aveiro.
Vicente, R., Mendes da Silva, J., and Varum, H. (2005). “Seismic Vulnerability Assessment of Buildings in the Old City centre of Coimbra,” in Proceedings of the International Conference 250th Anniversary of the 1755 Lisbon Earthquake (Lisbon: The publish is Springer Science+Business Media B.V.) November 1–4, 2005, 1–4.
Vicente, R., Parodi, S., Lagomarsino, S., Varum, H., and Silva, J. A. (2011). Seismic Vulnerability and Risk Assessment: Case Study of the Historic City centre of Coimbra, Portugal. Bull. Earthquake Eng. 9, 1067–1096. doi:10.1007/s10518-010-9233-3
World Bank (2021). Financial Risk and Opportunities to Build Resilience in Europe. Washington, D.C.: World Bank. doi:10.1596/35685
Keywords: risk assessment, seismic vulnerability, fire risk, flood risk, historic urban areas
Citation: Ferreira TM and Ramírez Eudave R (2022) Assessing and Managing Risk in Historic Urban Areas: Current Trends and Future Research Directions. Front. Earth Sci. 10:847959. doi: 10.3389/feart.2022.847959
Received: 03 January 2022; Accepted: 23 February 2022;
Published: 15 March 2022.
Edited by:
Irasema Alcántara-Ayala, National Autonomous University of Mexico, MexicoReviewed by:
Maxim A. Dulebenets, Florida Agricultural and Mechanical University, United StatesFabio Matano, National Research Council (CNR), Italy
Copyright © 2022 Ferreira and Ramírez Eudave. This is an open-access article distributed under the terms of the Creative Commons Attribution License (CC BY). The use, distribution or reproduction in other forums is permitted, provided the original author(s) and the copyright owner(s) are credited and that the original publication in this journal is cited, in accordance with accepted academic practice. No use, distribution or reproduction is permitted which does not comply with these terms.
*Correspondence: Tiago Miguel Ferreira, Tiago.Ferreira@uwe.ac.uk