Circulating ketone bodies and mortality in heart failure: a community cohort study
- 1Heart Disease Phenomics Laboratory, Epidemiology and Community Health Branch, National Heart, Lung, and Blood Institute, National Institutes of Health, Bethesda, MD, United States
- 2Office of Biostatistics Research, National Heart, Lung, and Blood Institute, National Institutes of Health, Bethesda, MD, United States
- 3Department of Laboratory Medicine, Clinical Center, National Institutes of Health, Bethesda, MD, United States
- 4Lipoprotein Metabolism Laboratory, Translational Vascular Medicine Branch, National Heart, Lung, and Blood Institute, National Institutes of Health, Bethesda, MD, United States
- 5Labcorp, Morrisville, NC, United States
- 6Division of Clinical Trials and Biostatistics, Department of Quantitative Health Sciences, Mayo Clinic College of Medicine and Science, Rochester, MN, United States
- 7Division of Epidemiology, Department of Quantitative Health Sciences, Mayo Clinic College of Medicine and Science, Rochester, MN, United States
Background: The relationship between ketone bodies (KB) and mortality in patients with heart failure (HF) syndrome has not been well established.
Objectives: The aim of this study is to assess the distribution of KB in HF, identify clinical correlates, and examine the associations between plasma KB and all-cause mortality in a population-based HF cohort.
Methods: The plasma KB levels were measured by nuclear magnetic resonance spectroscopy. Multivariable linear regression was used to examine associations between clinical correlates and KB levels. Proportional hazard regression was employed to examine associations between KB (represented as both continuous and categorical variables) and mortality, with adjustment for several clinical covariates.
Results: Among the 1,382 HF patients with KB measurements, the median (IQR) age was 78 (68, 84) and 52% were men. The median (IQR) KB was found to be 180 (134, 308) μM. Higher KB levels were associated with advanced HF (NYHA class III–IV) and higher NT-proBNP levels (both P < 0.001). The median follow-up was 13.9 years, and the 5-year mortality rate was 51.8% [95% confidence interval (CI): 49.1%–54.4%]. The risk of death increased when KB levels were higher (HRhigh vs. low group 1.23; 95% CI: 1.05–1.44), independently of a validated clinical risk score. The association between higher KB and mortality differed by ejection fraction (EF) and was noticeably stronger among patients with preserved EF.
Conclusions: Most patients with HF exhibited KB levels that were consistent with those found in healthy adults. Elevated levels of KB were observed in patients with advanced HF. Higher KB levels were found to be associated with an increased risk of death, particularly in patients with preserved EF.
1 Introduction
Heart failure (HF) is a complex clinical syndrome associated with metabolic alterations of several substrates, including glucose, amino acids, fatty acids, and ketone bodies (KB) (1). People with HF have higher circulating levels and myocardium utilization of KB (2–5). Increased KB oxidation may be a metabolic adaptation in HF (6), partly due to its higher phosphate-to-oxygen ratio compared with other substrates (7).
Animal and human studies have suggested KB supplementation as a potential therapeutic strategy in HF, postulating that KB could improve cardiac function by improving myocardial blood flow and cardiac remodeling, while reducing oxidative stress (8–11). However, due to the lack of standard clinical measurements of KB, there is a scarcity of human data in large cohorts of patients encompassing the entire HF syndrome, and their association with mortality in HF remains poorly understood. Addressing these gaps in knowledge in sufficiently large cohorts that allow for comprehensive correlative assessment and adjustment for key covariates is a prerequisite to promote supplementation interventions.
Nuclear magnetic resonance (NMR) spectroscopy now offers a high-throughput approach to measure circulating KB in epidemiologic studies, which will provide important insights into the associations between KB and HF prognosis. To do so, we measured the distribution of plasma KB in a large community cohort that includes individuals across the entire spectrum of the HF syndrome and examined the key clinical correlates and the association between KB and all-cause mortality.
2 Materials and methods
2.1 Patient population
This HF community cohort was assembled under the auspices of the Rochester Epidemiology Project (REP), a comprehensive record linkage system that captures clinical diagnoses, procedures, results, and outcomes in its catchment area (12, 13). Using natural language processing to analyze the content from electronic medical records, we assembled a community cohort consisting of all patients aged 20 years and older who were diagnosed with HF and lived in Olmsted, Dodge, or Fillmore Counties in Minnesota. The collection of data was previously published (14–16). This approach yielded 100% sensitivity compared with billing data, a reference method for case finding (17). Research nurses reviewed the records and validated the HF diagnosis using the Framingham criteria (18). Patients were approached in the hospital or after an outpatient encounter to provide written consent to participate in the study, including a blood draw, from 2 September 2003 to 16 June 2012. The Mayo Clinic and Olmsted Medical Center Institutional Review Boards approved this study.
2.2 Data collection
Nurse abstractors collected clinical information from both inpatient and outpatient records from all providers. Left ventricular ejection fraction (EF) was obtained from the closest available echocardiogram within 6 months prior to or 2 months following the date of enrollment. Body mass index (BMI) was calculated using weight (kilograms) from the last outpatient visit prior to enrollment divided by their earliest recorded adult height (meters) squared. The Meta-Analysis Global Group in Chronic HF (MAGGIC) score was calculated using sex, age, EF, systolic blood pressure, BMI, creatinine, New York Heart Association (NYHA) class, smoking status, diabetes, chronic obstructive pulmonary disease, HF diagnosis > 18 months ago, and the use of beta blocker, angiotensin-converting enzyme inhibitors, an/or angiotensin-receptor blockers (19). The MAGGIC score was originally derived to assess the mortality risk in patients with HF using the data collected from 39,000 HF patients, across the EF spectrum. Missing data were rare, and 10 multiple imputations by chain equations were performed to account for the missing components of the MAGGIC scores, including BMI (2.8%), NYHA class (0.4%), HF duration (0.1%), EF (1.8%), and creatinine (0.7%). N-terminal pro B-type natriuretic peptide (NT-proBNP) levels were measured at the NIDDK Clinical Laboratory Core using a Mesoscale multiplex assay, following the manufacturer’s instructions (https://www.mesoscale.com/en).
2.3 Biomarker measurements
NMR analyses of frozen EDTA plasma samples collected at enrollment were used to measure β-hydroxybutyrate (β-HB), acetoacetate (AcAc), and acetone performed on the high-throughput 400 MHz Vantera® Clinical Analyzer platform at the NHLBI Lipoprotein Metabolism Laboratory (Bethesda, MD). The three KB metabolites give rise to NMR signals that represent the basis of their quantification (20). For our analysis, the plasma levels of the three KB metabolites were summed to calculate the total KB as previously described (LabCorp, Morrisville, NC) (20).
2.4 Ascertainment of death
The patients were monitored until 31 March 2021, using data from REP. The REP obtains death date information from the healthcare providers that participate in the REP, from the State of Minnesota death certificates, and from the linkage of patient records to the National Death Index. The cause of death information is available from the Minnesota death certificates and from National Death Index. Patients who were still alive at the end of the follow-up period were censored on 31 March 2021, or the date of their last known healthcare contact, whichever was earlier.
2.5 Statistical analysis
Baseline characteristics were expressed as frequencies (percent) for categorical variables and as medians [interquartile range (IQR)] for continuous variables. Spearman correlation coefficients (ρ) were calculated across total KB and each KB metabolite (β-HB, AcAc, and acetone). KB levels were transformed into the natural logarithmic scale for all subsequent analyses and modeled both continuously (per 1 SD) and categorically. KB groups [low KB: ≤ 471.5 μM (N = 1,172); high KB: > 471.5 μM (N = 210)] were determined by a conditional inference tree method (ctree R Package), a recursive partitioning approach (21).
Multivariable linear regression was used to identify the clinical correlates independently associated with continuous KB levels. β-coefficients [95% confidence interval (CI)] were reported for each correlate.
The median follow-up time was calculated using the reverse Kaplan–Meier method (22). The survival by KB group was estimated using the Kaplan–Meier method and compared across groups by the log-rank test. Cox proportional hazards regression models were used to examine the association between KB and mortality. The models included (i) unadjusted, (ii) adjusted for age and sex, (iii) adjusted for MAGGIC score, and (iv) adjusted for MAGGIC and log2-transformed NT-proBNP (pg/ml). Stratified analyses were also performed by categorizing patients into reduced EF (< 50%) or preserved EF (≥ 50%).
Two-sided p-values of < 0.05 were considered statistically significant. The statistical analyses were performed using R version 3.6.2.
3 Results
3.1 Patient population
This HF cohort included 1,389 patients, with seven patients excluded due to the unavailability of their plasma samples, leaving 1,382 patients for analysis. The median (IQR) age for this analytic cohort was 78 (68, 84), and 52% were male (Table 1). Cardiometabolic risk factors were highly prevalent, including hypertension (91%), hyperlipidemia (85%), ischemic etiology (50%), and diabetes (36%). The median (IQR) MAGGIC score of the cohort was 24 (20, 29), and most patients were in NYHA class III–IV (69%). The distribution of patients with preserved EF (N = 785) and reduced EF (N = 597) was similar.
The median (IQR) and mean (SD) KB concentration for the cohort were 180 μM (134, 308) and 332 μM (549), respectively, with a right skewed distribution (Figure 1). The Spearman's rank correlation of β-HB, AcAc, and acetone were highly correlated with plasma total KB (ρ = 0.92, 0.85, and 0.73, respectively) (Supplementary Table 1).
3.2 Correlates of KB in HF
Following the multivariable adjustment, NYHA class III–IV (β = 0.22, 95% CI: 0.10–0.33; P < 0.001) and NT-proBNP levels (β = 0.11, 95% CI: 0.07–0.14; P < 0.001) were independently and positively associated with KB levels (Table 2). No independent association between KB levels and age, sex, BMI, hyperlipidemia, ischemic etiology, EF, and diabetes was observed (P > 0.05).
3.3 KB and mortality
The median (IQR) follow-up was 13.9 (11.5, 15.4) years. At the end of follow-up, 1,158 patients died with a 5-year mortality rate of 51.8% (95% CI: 49.1%–54.4%). A 1 SD increase in KB was associated with an increased risk of mortality (HR 1.09; 95% CI: 1.03–1.16). The increased risk remained even after further adjustment for the MAGGIC score (HR 1.06; 95% CI: 1.00–1.12), but it was attenuated after further adjustment for NT-proBNP (HR 1.01; 95% CI 0.95–1.08) (Figure 2).
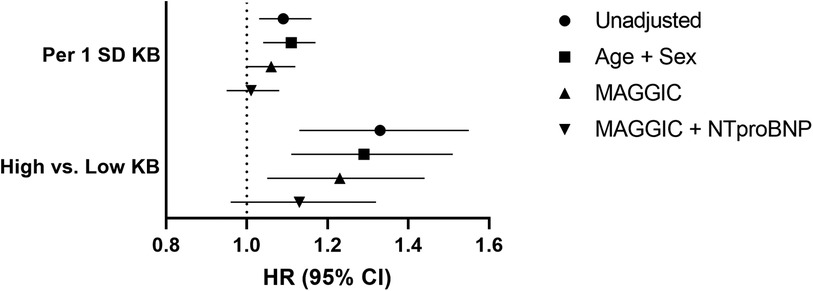
Figure 2. Ketone bodies and risk of mortality. Low KB: ≤ 471.5 μM (N = 1,172); High KB: > 471.5 μM (N = 210). Ketone bodies, KB; MAGGIC, Meta-analysis Global Group in Chronic Heart Failure; NT-proBNP, N-terminal pro B-type natriuretic peptide; standard deviation, SD.
When analyzing KB categorically, the 5-year mortality rate in the high KB group was 61.1% (95% CI: 53.9%–67.2%), compared with 50.2% (95% CI: 47.2%–53.0%) in the low KB group (Figure 3). Similar to the continuous results, the patients in the high KB group were at greater risk of mortality compared with the patients in the low KB group (HR 1.33; 95% CI: 1.13–1.55). An increased risk of mortality remained even after further adjustment for the MAGGIC score (HR 1.23; 95% CI: 1.05–1.44), but it was attenuated after further adjustment for NT-proBNP (HR 1.13; 95% CI 0.96–1.32) (Figure 2). The overall association between mortality and KB remained consistent regardless of the modelling strategy (KB modeled continuously or categorically). However, the association between KB and mortality differed by the EF group, with a higher risk of mortality observed in patients with preserved EF but not in patients with reduced EF (Figure 4).
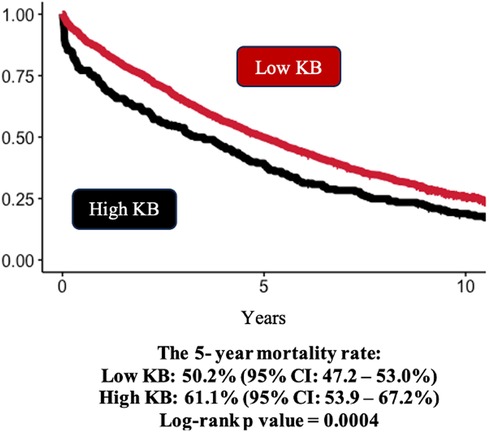
Figure 3. Kaplan–Meier survival curves by ketone bodies groups. Kaplan–Meier estimates and log-rank P-values of all-cause mortality. Low KB: ≤ 471.5 μM (N = 1,172); High KB: > 471.5 μM (N = 210). Patients in the high KB group had higher rates of mortality compared with those in the low KB group.
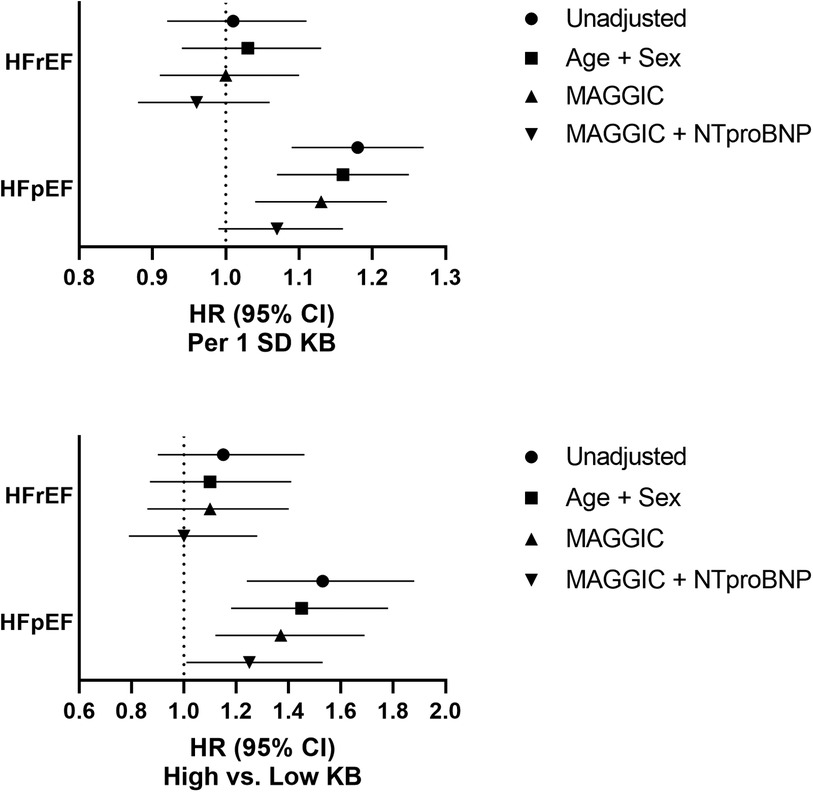
Figure 4. Ketone bodies and risk of mortality stratified by ejection fraction category. Low KB: ≤ 471.5 μM (N = 1,172); High KB: > 471.5 μM (N = 210). Ketone bodies, KB; MAGGIC, Meta-analysis Global Group in Chronic Heart Failure; NT-proBNP, N-terminal pro B-type natriuretic peptide; standard deviation, SD.
4 Discussion
Herein, we report on the distribution, clinical correlates, and association with mortality of plasma KB in a community cohort of HF patients. We revealed that, in this population, which represents the consecutive experience of a geographically defined community with HF, most KB levels were similar to values obtained in healthy adults (20). Higher KB levels were associated with higher NYHA class and higher NT-proBNP levels. Finally, while the elevated KB levels increased the risk of death modestly across the entire spectrum of the HF syndrome, the association differed by EF and was particularly strong among HF patients with preserved EF. The association was independent of the MAGGIC score and only partially attenuated after further adjustment for NT-proBNP, indicating that higher KB levels may not be protective in HF.
4.1 Distribution of KB in HF
To the best of our knowledge, no consensus reference values for KB have been established in HF. Prior reports indicated that patients with HF had elevated KB levels compared with patients without HF (2, 3, 5). One study of 45 patients with congestive HF reported KB levels ranging from 44 to 952 μM (2). In this HF community cohort, the median and mean KB concentrations were 180 μM and 332 μM, respectively. In non-fasting healthy adults, the KB levels, measured using the same method as our study, ranged between 100 and 600 μM and increased to 1,000 μM following prolonged fasting or exercise (20). As a point of reference, in diabetes or alcohol ketoacidosis, the KB levels can range between 6,000 and 20,000 μM (20, 23, 24). These findings suggest that most patients drawn from an HF community cohort have KB levels that fall in the expected range in healthy adults.
4.2 Correlates of KB in HF
Herein, we found that higher NYHA class and higher NT-proBNP were associated with higher KB levels, consistent with the postulate that KB levels increase with HF severity to maintain adequate cardiac function (2, 5, 25). Indeed, in a study of non-ischemic HF patients, exhaled acetone levels, which were correlated with blood KB levels, were associated with higher NYHA class and higher BNP (26). In a study of 45 patients with HF, higher KB levels were correlated with a lower EF (2), while another study of 46 patients with HF with reduced EF found higher levels of acetone among HF patients compared with controls (27). However, we observed no cross-sectional association between KB and EF categories after controlling for several potential confounding factors, indicating that our findings surrounding KB correlates apply to the entire spectrum of the HF syndrome.
The relationship between natriuretic peptides and KB in patients with HF is not entirely understood. Several studies have shown an association between natriuretic peptides and lipolysis (28), which can result in the production of KB. The stimulation of lipolysis by natriuretic peptides has been suggested as a potential mechanism of cachexia in patients with advanced HF (29). We found a strong positive association between KB levels and NT-proBNP. In a Dutch population cohort of 6,134 participants, β-HB concentrations were associated with higher NT-proBNP at baseline and an increased risk of HF with reduced EF (30). Several studies reported that elevated KB levels were positively associated with other natriuretic peptides, including BNP (31) and pro-atrial natriuretic peptide (2). These findings suggest that the interplay between KB and natriuretic peptides may be associated with HF severity. Further work is needed to understand the molecular underpinnings of this potential association.
Diabetes increases the risk of mortality in HF (32). Previous studies have shown higher KB levels may be associated with diabetes overall (20) and among patients with advanced HF and diabetes (33). In this cohort, we observed no relationship between diabetes and KB levels. Several reasons could explain the difference in findings, including large differences in the characteristics of the study population (age, race, ethnicity, disease status) and study design (clinical cohort vs. population-based study). However, in light of the recent evidence suggesting that sodium-glucose transporter 2 inhibitors, a drug class originally designed to treat diabetes, reduce the risk of HF mortality (34) and also increase KB levels (35), further efforts are needed to unravel the complex interplay between diabetes, KBs, and HF mortality.
4.3 KB and mortality
There is limited evidence on the association between circulating KB levels and HF mortality. A study of 615 HF patients found that patients with high AcAc concentrations (≥ 35 μM) were at a small but detectable increased risk of all-cause mortality (36). Several studies have found an association between higher breath acetone levels and HF (26, 27, 33, 37). Elevated levels of exhaled breath acetone have been reported to be an independent predictor of mortality in HF with reduced EF (25, 38). Using NMR spectroscopy, a population-based, multi-ethnic study including 6,796 subjects from the United States found that higher levels of β-HB and AcAc were associated with cardiovascular events and mortality (39). Another study of older adults found that higher KB levels were associated with both incident HF and all-cause mortality, independent of metabolic and cardiovascular risk factors (40). Although these results are aligned with our finding and support that KB may be associated with a poor prognosis in HF, it remains unclear whether increased KB metabolism is a cause or a consequence of HF (41). Hence, further studies are needed to evaluate a possible causal relationship between KB levels and mortality.
Although we observed no cross-sectional association between KB levels and EF categories, the stratified analyses showed that the association between KB levels and mortality differed by EF and was noticeably stronger if the EF was preserved. Conceptually, KB utilization occurs in HF regardless of EF (7); however, its utilization may differ by EF in patients with reduced EF using nearly three times as much KB compared with patients with preserved EF (42). The differences in KB utilization in the myocardium in the absence of any apparent association with circulating KB levels might provide a plausible biological mechanism for the observed differences in mortality by EF. Additional research is required in study populations that comprise the entire spectrum of HF with mortality data to further test this hypothesis.
While higher KB levels remained associated with increased mortality after adjustment for MAGGIC in our study, further adjustment for NT-proBNP attenuated the association between KB and mortality. This may reflect overadjustment of our model as the MAGGIC risk score uses 13 routinely collected long-established predictors of mortality in HF, specifically, NYHA classification and EF, which are associated with NT-proBNP (19, 43, 44).
KB supplementation has been suggested as a therapeutic intervention in human and animal studies (8, 45–47), arguing for protective effects of KB in patients with HF (7). Some studies reported that an increase in circulating KB levels is associated with a favorable increase in myocardial KB utilization in HF (4, 5, 48). In clinical studies, it has been posited that ketone supplementation may prevent pathological remodeling, improve cardiac work, and reduce cardiovascular risk factors in HF patients (10, 11, 49). A small, randomized crossover study of 16 patients with chronic HF and reduced EF reported a dose–response association with β-HB infusion and oxygen consumption without affecting myocardial external energy efficiency (11). However, there is clearly insufficient evidence regarding the therapeutic potential of KB in HF. Our findings of a modest but significant increase in the risk of death associated with higher levels of plasma KB do not support the notion that higher levels of KB would be protective in HF, but we cannot rule out the possibility that higher levels of KB may be an adaptive mechanism of advanced HF.
4.4 KB and metabolic alterations in HF
For over half a century, researchers have postulated that metabolic alterations may play a role in HF and cardiac cachexia, including KB (50). Evidence suggests that cardiac metabolism can impact both the structure and function of the heart (51). However, our understanding of the biological underpinnings of the association between KB and HF are complicated by the fact that several metabolic alterations are likely occurring simultaneously as is evident by multiple reports showing an association between KB and triglyceride levels (52, 53). Further work, probably involving more comprehensive metabolomics, may be needed to unravel the complex interplay between these metabolic alterations and the potential functional consequences of altered KB levels and mortality in HF.
4.5 Limitations and strengths
The majority of our cohort consisted of individuals who identified as non-Hispanic White, which potentially limits the generalizability of our findings to more ethnically and racially diverse populations. Fasting was not required to be enrolled in the study; however, most patients had NMR-based glucose levels consistent with fasting. Comparing results across published studies is challenging due to study heterogeneity, varying sample sizes, and differences in KB measurements. While we observed an association between KB and mortality, comorbidities and polypharmacy are highly prevalent in patients with HF. While we relied on the MAGGIC score as a key adjustment variable, this score does not fully account for all the possible prognostic indicators in HF such that we cannot rule out potential residual confounding.
Our study had several strengths. To the best of our knowledge, this study is among the first to characterize the distribution of KB in HF, establish clinical correlates of KB elevation, and assess the association between KB levels and mortality in a population-based cohort comprised of the entire spectrum of HF. By studying a community cohort, we optimize the applicability of our results to routine clinical practice. The long-term follow-up and rich clinical dataset available through the Rochester Epidemiology Project allowed us to control for several key covariates and potential confounders of the association between KB and mortality.
4.6 Conclusion
In a cohort of individuals with HF, the distribution of KB levels is primarily within the expected ranges for healthy individuals. These KB levels are correlated with indicators of HF severity and are independently associated with mortality in a population-based cohort comprised of the entire spectrum of HF, in particular among patients with preserved EF.
Data availability statement
The datasets generated for this study can be made available upon reasonable request to the corresponding author. Requests to access the datasets should be directed to Véronique L. Roger, veronique.roger@nih.gov.
Ethics statement
The studies involving humans were approved by The Mayo Clinic and Olmsted Medical Center Institutional Review Boards. The studies were conducted in accordance with the local legislation and institutional requirements. The participants provided their written informed consent to participate in this study.
Author contributions
RO: Conceptualization, Data curation, Formal Analysis, Writing – original draft, Writing – review & editing. KC: Investigation, Writing – review & editing. JJ: Methodology, Writing – review & editing. ST: Investigation, Writing – review & editing. MS: Investigation, Writing – review & editing. AW: Investigation, Writing – review & editing. AR: Resources, Writing – review & editing. JO: Methodology, Writing – review & editing. MC: Methodology, Writing – review & editing. NL: Methodology, Writing – review & editing. SB: Resources, Writing – review & editing. MH: Writing – review & editing. JS: Conceptualization, Formal Analysis, Supervision, Writing – original draft, Writing – review & editing. VR: Funding acquisition, Methodology, Supervision, Writing – original draft, Writing – review & editing.
Funding
The author(s) declare financial support was received for the research, authorship, and/or publication of this article.
The investigators were supported by the Intramural Research program of the National Heart Lung and Blood Institute of the National Institutes of Health (ZIAHL006278). This study also used in part the resources from the Rochester Epidemiology Project (REP) medical records-linkage system, which is supported by the National Institute on Aging (NIA; AG 058738), by the Mayo Clinic Research Committee, and by fees paid annually by REP users. The funding institution did not play a role design, conduct, analysis, or reporting nor in the decision to submit this manuscript for publication.
Acknowledgments
The authors thank Mary Walter and Yuhai Dai at the NIDDK Clinical Laboratory Core, NIH, USA, for their assistance. Research support was provided by the NIH Medical Research Scholars Program, a public–private partnership supported jointly by the NIH and contributions to the Foundation for the NIH from the American Association for Dental Research and the Colgate-Palmolive Company.
Conflict of interest
MC is an employee of and holds stocks in LabCorp. JO is a consultant, stockholder, and former employee of LabCorp.
The remaining authors declare that the research was conducted in the absence of any commercial or financial relationships that could be construed as a potential conflict of interest
Publisher's note
All claims expressed in this article are solely those of the authors and do not necessarily represent those of their affiliated organizations, or those of the publisher, the editors and the reviewers. Any product that may be evaluated in this article, or claim that may be made by its manufacturer, is not guaranteed or endorsed by the publisher.
Supplementary material
The Supplementary Material for this article can be found online at: https://www.frontiersin.org/articles/10.3389/fcvm.2024.1293901/full#supplementary-material
References
1. Lopaschuk GD, Karwi QG, Tian R, Wende AR, Abel ED. Cardiac energy metabolism in heart failure. Circ Res. (2021) 128(10):1487–513. doi: 10.1161/CIRCRESAHA.121.318241
2. Lommi J, Kupari M, Koskinen P, Näveri H, Leinonen H, Pulkki K, et al. Blood ketone bodies in congestive heart failure. J Am Coll Cardiol. (1996) 28(3):665–72. doi: 10.1016/0735-1097(96)00214-8
3. Lommi J, Koskinen P, Naveri H, Harkonen M, Kupari M. Heart failure ketosis. J Intern Med. (1997) 242(3):231–8. doi: 10.1046/j.1365-2796.1997.00187.x
4. Voros G, Ector J, Garweg C, Droogne W, Van Cleemput J, Peersman N, et al. Increased cardiac uptake of ketone bodies and free fatty acids in human heart failure and hypertrophic left ventricular remodeling. Circ Heart Fail. (2018) 11(12):e004953. doi: 10.1161/CIRCHEARTFAILURE.118.004953
5. Bedi KC, Snyder NW, Brandimarto J, Aziz M, Mesaros C, Worth AJ, et al. Evidence for intramyocardial disruption of lipid metabolism and increased myocardial ketone utilization in advanced human heart failure. Circulation. (2016) 133(8):706–16. doi: 10.1161/CIRCULATIONAHA.115.017545
6. Yurista SR, Nguyen CT, Rosenzweig A, de Boer RA, Westenbrink BD. Ketone bodies for the failing heart: fuels that can fix the engine? Trends Endocrinol Metab. (2021) 32(10):814–26. doi: 10.1016/j.tem.2021.07.006
7. Selvaraj S, Kelly DP, Margulies KB. Implications of altered ketone metabolism and therapeutic ketosis in heart failure. Circulation. (2020) 141(22):1800–12. doi: 10.1161/CIRCULATIONAHA.119.045033
8. Yurista SR, Chong CR, Badimon JJ, Kelly DP, de Boer RA, Westenbrink BD. Therapeutic potential of ketone bodies for patients with cardiovascular disease: JACC state-of-the-art review. J Am Coll Cardiol. (2021) 77(13):1660–9. doi: 10.1016/j.jacc.2020.12.065
9. Papazafiropoulou AK, Georgopoulos MM, Katsilambros NL. Ketone bodies and the heart. Arch Med Sci Atheroscler Dis. (2021) 6:e209–e14. doi: 10.5114/amsad.2021.112475
10. Gormsen LC, Svart M, Thomsen HH, Sondergaard E, Vendelbo MH, Christensen N, et al. Ketone body infusion with 3-hydroxybutyrate reduces myocardial glucose uptake and increases blood flow in humans: a positron emission tomography study. J Am Heart Assoc. (2017) 6(3):e005066. doi: 10.1161/JAHA.116.005066
11. Nielsen R, Moller N, Gormsen LC, Tolbod LP, Hansson NH, Sorensen J, et al. Cardiovascular effects of treatment with the ketone body 3-hydroxybutyrate in chronic heart failure patients. Circulation. (2019) 139(18):2129–41. doi: 10.1161/CIRCULATIONAHA.118.036459
12. Rocca WA, Yawn BP, St Sauver JL, Grossardt BR, Melton LJ 3rd. History of the Rochester epidemiology project: half a century of medical records linkage in a US population. Mayo Clin Proc. (2012) 87(12):1202–13. doi: 10.1016/j.mayocp.2012.08.012
13. Rocca WA, Grossardt BR, Brue SM, Bock-Goodner CM, Chamberlain AM, Wilson PM, et al. Data resource profile: expansion of the Rochester epidemiology project medical records-linkage system (E-REP). Int J Epidemiol. (2018) 47(2):368–368j. doi: 10.1093/ije/dyx268
14. Dunlay SM, Manemann SM, Chamberlain AM, Cheville AL, Jiang R, Weston SA, et al. Activities of daily living and outcomes in heart failure. Circ Heart Fail. (2015) 8(2):261–7. doi: 10.1161/CIRCHEARTFAILURE.114.001542
15. Dunlay SM, Gerber Y, Weston SA, Killian JM, Redfield MM, Roger VL. Prognostic value of biomarkers in heart failure: application of novel methods in the community. Circ Heart Fail. (2009) 2(5):393–400. doi: 10.1161/CIRCHEARTFAILURE.109.849299
16. Chamberlain AM, St Sauver JL, Gerber Y, Manemann SM, Boyd CM, Dunlay SM, et al. Multimorbidity in heart failure: a community perspective. Am J Med. (2015) 128(1):38–45. doi: 10.1016/j.amjmed.2014.08.024
17. Pakhomov SV, Buntrock J, Chute CG. Prospective recruitment of patients with congestive heart failure using an ad-hoc binary classifier. J Biomed Inform. (2005) 38(2):145–53. doi: 10.1016/j.jbi.2004.11.016
18. McKee PA, Castelli WP, McNamara PM, Kannel WB. The natural history of congestive heart failure: the Framingham study. N Engl J Med. (1971) 285(26):1441–6. doi: 10.1056/NEJM197112232852601
19. Pocock SJ, Ariti CA, McMurray JJ, Maggioni A, Køber L, Squire IB, et al. Predicting survival in heart failure: a risk score based on 39 372 patients from 30 studies. Eur Heart J. (2013) 34(19):1404–13. doi: 10.1093/eurheartj/ehs337
20. Garcia E, Shalaurova I, Matyus SP, Oskardmay DN, Otvos JD, Dullaart RPF, et al. Ketone bodies are mildly elevated in subjects with type 2 diabetes mellitus and are inversely associated with insulin resistance as measured by the lipoprotein insulin resistance index. J Clin Med. (2020) 9(2):321. doi: 10.3390/jcm9020321
21. Onodera W, Hara N, Aoki S, Asahi T, Sawamura N. Phylogenetic tree reconstruction via graph cut presented using a quantum-inspired computer. Mol Phylogenet Evol. (2023) 178:107636. doi: 10.1016/j.ympev.2022.107636
22. Altman DG, De Stavola BL, Love SB, Stepniewska KA. Review of survival analyses published in cancer journals. Br J Cancer. (1995) 72(2):511–8. doi: 10.1038/bjc.1995.364
23. Bienia R, Ripoll I. Diabetic ketoacidosis. JAMA. (1979) 241(5):510–1. doi: 10.1001/jama.1979.03290310050019
24. Schade DS, Eaton RP. Differential diagnosis and therapy of hyperketonemic state. JAMA. (1979) 241(19):2064–5. doi: 10.1001/jama.1979.03290450062031
25. Marcondes-Braga FG, Gioli-Pereira L, Bernardez-Pereira S, Batista GL, Mangini S, Issa VS, et al. Exhaled breath acetone for predicting cardiac and overall mortality in chronic heart failure patients. Esc Heart Failure. (2020) 7(4):1744–52. doi: 10.1002/ehf2.12736
26. Yokokawa T, Sugano Y, Shimouchi A, Shibata A, Jinno N, Nagai T, et al. Exhaled acetone concentration is related to hemodynamic severity in patients with non-ischemic chronic heart failure. Circ J. (2016) 80(5):1178–86. doi: 10.1253/circj.CJ-16-0011
27. Gladding PA, Cooper M, Young R, Loader S, Smith K, Zarate E, et al. Metabolomics and a breath sensor identify acetone as a biomarker for heart failure. Biomolecules. (2022) 13(1):13. doi: 10.3390/biom13010013
28. Madamanchi C, Alhosaini H, Sumida A, Runge MS. Obesity and natriuretic peptides, BNP and NT-proBNP: mechanisms and diagnostic implications for heart failure. Int J Cardiol. (2014) 176(3):611–7. doi: 10.1016/j.ijcard.2014.08.007
29. Kalra PR, Tigas S. Regulation of lipolysis: natriuretic peptides and the development of cachexia. Int J Cardiol. (2002) 85(1):125–32. doi: 10.1016/S0167-5273(02)00241-3
30. Flores-Guerrero JL, Westenbrink BD, Connelly MA, Otvos JD, Groothof D, Shalaurova I, et al. Association of beta-hydroxybutyrate with development of heart failure: sex differences in a Dutch population cohort. Eur J Clin Invest. (2021) 51(5):e13468. doi: 10.1111/eci.13468
31. Kashiwagi Y, Nagoshi T, Inoue Y, Tanaka Y, Takahashi H, Oi Y, et al. Close linkage between blood total ketone body levels and B-type natriuretic peptide levels in patients with cardiovascular disorders. Sci Rep. (2021) 11(1):6498. doi: 10.1038/s41598-021-86126-0
32. Dunlay SM, Givertz MM, Aguilar D, Allen LA, Chan M, Desai AS, et al. Type 2 diabetes mellitus and heart failure: a scientific statement from the American Heart Association and the Heart Failure Society of America: this statement does not represent an update of the 2017 ACC/AHA/HFSA heart failure guideline update. Circulation. (2019) 140(7):e294–324. doi: 10.1161/CIR.0000000000000691
33. Yokokawa T, Sato T, Suzuki S, Oikawa M, Yoshihisa A, Kobayashi A, et al. Elevated exhaled acetone concentration in stage C heart failure patients with diabetes mellitus. BMC Cardiovasc Disord. (2017) 17(1):280. doi: 10.1186/s12872-017-0713-0
34. Cardoso R, Graffunder FP, Ternes CMP, Fernandes A, Rocha AV, Fernandes G, et al. SGLT2 Inhibitors decrease cardiovascular death and heart failure hospitalizations in patients with heart failure: a systematic review and meta-analysis. EClinicalMedicine. (2021) 36:100933. doi: 10.1016/j.eclinm.2021.100933
35. Polidori D, Iijima H, Goda M, Maruyama N, Inagaki N, Crawford PA. Intra- and inter-subject variability for increases in serum ketone bodies in patients with type 2 diabetes treated with the sodium glucose co-transporter 2 inhibitor canagliflozin. Diabetes Obes Metab. (2018) 20(5):1321–6. doi: 10.1111/dom.13224
36. Yokokawa T, Yoshihisa A, Kanno Y, Abe S, Misaka T, Yamada S, et al. Circulating acetoacetate is associated with poor prognosis in heart failure patients. Ijc Heart Vasc. (2019) 25:100432. doi: 10.1016/j.ijcha.2019.100432
37. Kupari M, Lommi J, Ventila M, Karjalainen U. Breath acetone in congestive heart failure. Am J Cardiol. (1995) 76(14):1076–8. doi: 10.1016/S0002-9149(99)80304-X
38. Marcondes-Braga FG, Batista GL, Gutz IG, Saldiva PH, Mangini S, Issa VS, et al. Impact of exhaled breath acetone in the prognosis of patients with heart failure with reduced ejection fraction (HFrEF). one year of clinical follow-up. PLoS One. (2016) 11(12):e0168790. doi: 10.1371/journal.pone.0168790
39. Shemesh E, Chevli PA, Islam T, German CA, Otvos J, Yeboah J, et al. Circulating ketone bodies and cardiovascular outcomes: the MESA study. Eur Heart J. (2023) 44:1636–46. doi: 10.1093/eurheartj/ehad087
40. Niezen S, Connelly MA, Hirsch C, Kizer JR, Benitez ME, Minchenberg S, et al. Elevated plasma levels of ketone bodies are associated with all-cause mortality and incidence of heart failure in older adults: the CHS. J Am Heart Assoc. (2023) 12(17):e029960. doi: 10.1161/JAHA.123.029960
41. Taegtmeyer H. Failing heart and starving brain: ketone bodies to the rescue. Circulation. (2016) 134(4):265–6. doi: 10.1161/CIRCULATIONAHA.116.022141
42. Murashige D, Jang C, Neinast M, Edwards JJ, Cowan A, Hyman MC, et al. Comprehensive quantification of fuel use by the failing and nonfailing human heart. Science. (2020) 370(6514):364–8. doi: 10.1126/science.abc8861
43. Tschope C, Kasner M, Westermann D, Gaub R, Poller WC, Schultheiss HP. The role of NT-proBNP in the diagnostics of isolated diastolic dysfunction: correlation with echocardiographic and invasive measurements. Eur Heart J. (2005) 26(21):2277–84. doi: 10.1093/eurheartj/ehi406
44. Iwanaga Y, Nishi I, Furuichi S, Noguchi T, Sase K, Kihara Y, et al. B-type natriuretic peptide strongly reflects diastolic wall stress in patients with chronic heart failure: comparison between systolic and diastolic heart failure. J Am Coll Cardiol. (2006) 47(4):742–8. doi: 10.1016/j.jacc.2005.11.030
45. Cox PJ, Kirk T, Ashmore T, Willerton K, Evans R, Smith A, et al. Nutritional ketosis alters fuel preference and thereby endurance performance in athletes. Cell Metab. (2016) 24(2):256–68. doi: 10.1016/j.cmet.2016.07.010
46. Takahara S, Soni S, Phaterpekar K, Kim TT, Maayah ZH, Levasseur JL, et al. Chronic exogenous ketone supplementation blunts the decline of cardiac function in the failing heart. ESC Heart Fail. (2021) 8(6):5606–12. doi: 10.1002/ehf2.13634
47. Augustin K, Khabbush A, Williams S, Eaton S, Orford M, Cross JH, et al. Mechanisms of action for the medium-chain triglyceride ketogenic diet in neurological and metabolic disorders. Lancet Neurol. (2018) 17(1):84–93. doi: 10.1016/S1474-4422(17)30408-8
48. Aubert G, Martin OJ, Horton JL, Lai L, Vega RB, Leone TC, et al. The failing heart relies on ketone bodies as a fuel. Circulation. (2016) 133(8):698–705. doi: 10.1161/CIRCULATIONAHA.115.017355
49. Selvaraj S, Fu Z, Jones P, Kwee LC, Windsor SL, Ilkayeva O, et al. Metabolomic profiling of the effects of dapagliflozin in heart failure with reduced ejection fraction: dEFINE-HF. Circulation. (2022) 146(11):808–18. doi: 10.1161/CIRCULATIONAHA.122.060402
50. Pittman JG, Cohen P. The pathogenesis of cardiac cachexia. N Engl J Med. (1964) 271:403–9. doi: 10.1056/NEJM196408202710807
51. Rodolico D, Schiattarella GG, Taegtmeyer H. The lure of cardiac metabolism in the diagnosis, prevention, and treatment of heart failure. JACC Heart Fail. (2023) 11(6):637–45. doi: 10.1016/j.jchf.2023.02.007
52. Ferrannini E, Baldi S, Scozzaro T, Tsimihodimos V, Tesfaye F, Shaw W, et al. Fasting substrate concentrations predict cardiovascular outcomes in the CANagliflozin cardioVascular assessment study (CANVAS). Diabetes Care. (2022) 45(8):1893–9. doi: 10.2337/dc21-2398
Keywords: ketone bodies, biomarkers, heart failure, mortality, epidemiology
Citation: Oyetoro RO, Conners KM, Joo J, Turecamo S, Sampson M, Wolska A, Remaley AT, Otvos JD, Connelly MA, Larson NB, Bielinski SJ, Hashemian M, Shearer JJ and Roger VL (2024) Circulating ketone bodies and mortality in heart failure: a community cohort study. Front. Cardiovasc. Med. 11:1293901. doi: 10.3389/fcvm.2024.1293901
Received: 18 September 2023; Accepted: 11 January 2024;
Published: 24 January 2024.
Edited by:
Elena N. Dedkova, University of California, Davis, United StatesReviewed by:
Daniele Rodolico, University of Pisa, ItalyGiulia Ferrannini, Karolinska Institutet (KI), Sweden
Kyle Stephan McCommis, Saint Louis University, United States
© 2024 Oyetoro, Conners, Joo, Turecamo, Sampson, Wolska, Remaley, Otvos, Connelly, Larson, Bielinski, Hashemian, Shearer and Roger. This is an open-access article distributed under the terms of the Creative Commons Attribution License (CC BY). The use, distribution or reproduction in other forums is permitted, provided the original author(s) and the copyright owner(s) are credited and that the original publication in this journal is cited, in accordance with accepted academic practice. No use, distribution or reproduction is permitted which does not comply with these terms.
*Correspondence: Véronique L. Roger veronique.roger@nih.gov