Comparing the Gut Microbiome in Autism and Preclinical Models: A Systematic Review
- 1School of Health and Biomedical Sciences, Royal Melbourne Institute of Technology (RMIT), Bundoora, VIC, Australia
- 2Medical Laboratory Technology Department, Faculty of Applied Medical Sciences, Jazan University, Jazan, Saudi Arabia
- 3Department of Microbiology, Anatomy, Physiology and Pharmacology, School of Life Sciences, La Trobe University, Bundoora, VIC, Australia
Many individuals diagnosed with autism spectrum disorder (ASD) experience gastrointestinal (GI) dysfunction and show microbial dysbiosis. Variation in gut microbial populations is associated with increased risk for GI symptoms such as chronic constipation and diarrhoea, which decrease quality of life. Several preclinical models of autism also demonstrate microbial dysbiosis. Given that much pre-clinical research is conducted in mouse models, it is important to understand the similarities and differences between the gut microbiome in humans and these models in the context of autism. We conducted a systematic review of the literature using PubMed, ProQuest and Scopus databases to compare microbiome profiles of patients with autism and transgenic (NL3R451C, Shank3 KO, 15q dup), phenotype-first (BTBR) and environmental (Poly I:C, Maternal Inflammation Activation (MIA), valproate) mouse models of autism. Overall, we report changes in fecal microbial communities relevant to ASD based on both clinical and preclinical studies. Here, we identify an overlapping cluster of genera that are modified in both fecal samples from individuals with ASD and mouse models of autism. Specifically, we describe an increased abundance of Bilophila, Clostridium, Dorea and Lactobacillus and a decrease in Blautia genera in both humans and rodents relevant to this disorder. Studies in both humans and mice highlighted multidirectional changes in abundance (i.e. in some cases increased abundance whereas other reports showed decreases) for several genera including Akkermansia, Bacteroides, Bifidobacterium, Parabacteroides and Prevotella, suggesting that these genera may be susceptible to modification in autism. Identification of these microbial profiles may assist in characterising underlying biological mechanisms involving host-microbe interactions and provide future therapeutic targets for improving gut health in autism.
Introduction
Autism spectrum disorder (ASD) is a heterogeneous neurodevelopmental condition diagnosed based on stereotyped or repetitive patterns of behavior, impaired communication interactions as well as language delay (American Psychiatric Association, 2013). Several studies also indicate that changes in the gut microbiome are associated with ASD (Strati et al., 2017). Individuals with ASD commonly experience various comorbidities including intellectual disability, sleeplessness, immune disorders, metabolic impairments, anxiety, epilepsy and gastrointestinal (GI) disorders (Matson et al., 2011; Hsiao, 2014; Lai et al., 2019). An assessment of the prevalence of GI symptoms in autism revealed as many as 70-90% of individuals diagnosed with ASD reported gut dysfunction (Adams et al., 2011; Gorrindo et al., 2012). People with ASD frequently report moderate to severe diarrhea, constipation as well as abdominal pain (Holingue et al., 2018) and increased gut permeability (Esnafoglu et al., 2017). In addition, GI problems experienced by children with ASD tend to be more frequent and severe than those reported in neuro-typical children (Vuong and Hsiao, 2017). In the autistic population, GI symptoms also correlate with the severity of core autism symptom such as sensory issues, sleep problems, comorbid psychopathology, challenging behavior, communication and language difficulties, increased social withdrawal and anxiety levels as well as irritability (Gorrindo et al., 2012; Attlee et al., 2015).
GI dysfunction experienced by individuals with ASD is likely driven by disruption of the microbiota-gut brain axis. This crucial interplay between the gut microbiome, the nervous system and the immune system maintains homeostatic microbial levels and maintains health. Changes in each of these domains are well established in both clinical and preclinical studies of autism (Bourgeron, 2009; Lee et al., 2020), Margolis et al., 2021). Nervous system changes occurring in autism not only affect the brain but may also alter the intrinsic nervous system of the gut (the enteric nervous system; ENS) which is in constant crosstalk with the GI microbiome and the immune system (i.e., reviewed in Margolis et al. (2021)). Dysbiosis; a reduction in microbial diversity leading to an increase in pathogenic bacteria is common in ASD patients (Valicenti-McDermott et al., 2006; Sekirov et al., 2010; Clemente et al., 2012; Martín et al., 2014; Luna et al., 2017; Thursby and Juge, 2017; Almeida et al., 2019; Rinninella et al., 2019; Xu et al., 2019b), but factors such as genetics, diet and living environment also influence the gut microbiome. An ideal approach for assessing changes in the microbiome in the absence of confounding factors is by studying preclinical models of ASD. In line with clinical findings, studies in a range of mouse models of autism also show altered microbial profiles (Hsiao, 2014; Hosie et al., 2019; Sgritta et al., 2019; Sharon et al., 2019).
Despite evidence for dysbiosis in both clinical and mouse model studies further characterisation of microbiome profiles across both clinical and animal model datasets is needed to pinpoint how microbial changes impact gut physiology in people with ASD. Identifying overlapping patterns of microbial variation in clinical populations and mouse models of autism will provide the basis for modulating the microbiome to improve gut health in individuals with ASD. Therefore, the characterization of gut microbial dysbiosis in people with ASD may provide insights into the underlying mechanisms of GI dysfunction symptoms in autism.
Factors Influencing Microbial Dysbiosis in Autism
The gut microbiome is influenced by multiple interlinked factors including genetics, the environment (stress, diet, lifestyle) and/or immune status. The microbiome interacts directly with both the immune and nervous system to modulate not only gut function but also influence mood and behaviour (Lozupone et al., 2012). Children with ASD frequently show dysbiosis (Finegold et al., 2010; De Angelis et al., 2013; Luna et al., 2017; Strati et al., 2017) and appear to be more susceptible to immune dysfunction and increased intestinal permeability compared to the general population (Strati et al., 2017; Rose et al., 2018; Matta et al., 2019). Characterization of microbial profiles will not only enable the identification of which microbes are altered in autism but may also help to understand the influence of the microbiome in ASD.
Genetic changes are prominent in ASD with more than 1000 single nucleotide (point) mutations associated with this disorder (Levy et al., 2011; Sanders et al., 2011; Guang et al., 2018). As yet, the precise relationship between host genetics and microbial dysbiosis is for the most part unknown. However, a study by Liu et al., for example, provides mechanistic evidence where host genetics shape the fecal microbial community (Liu et al., 2016). Many ASD-associated mutations occur in genes that encode scaffolding proteins, synaptic cytoplasmic and cell-adhesion molecules as well as various molecules involved in neurotransmission or regulation of synaptic protein synthesis (Guang et al., 2018). For example, a missense mutation in the neuroligin 3 (Nlgn3) protein was identified in autism patients (Jamain et al., 2003). Nlgn3 regulates neuronal morphology and dendritic outgrowth (Xu et al., 2019a) and impairs synaptic function in the central nervous system (Tabuchi et al., 2007; Etherton et al., 2011; Hosie et al., 2018). Multiple mutations associated with ASD also occur in the gene encoding the synaptic scaffolding protein ‘SH3 and multiple ankyrin repeat domains 3’ (SHANK3) (Durand et al., 2007). Alterations in SHANK3 modify cortical neuronal connectivity and increase the risk of ASD diagnosis (Pagani et al., 2019). These same mutations modify the enteric nervous system leading to abnormal gastrointestinal motility and structural changes in the GI tract (Hosie et al., 2019; Sauer et al., 2019; Herath et al., 2020; Lee et al., 2020; Leembruggen et al., 2020).
In addition to genetic influences, environmental factors can modify microbial profiles and could contribute to the etiology of complex disorders. Several medications, including the anti-epileptic drug, valproate, modify gut microbial function including fatty acid production (Poolchanuan et al., 2020). It has also been shown that the risk of ASD diagnosis is increased in infants born to mothers prescribed valproate (Christensen et al., 2013). In keeping with clinical findings, maternal immune dysregulation is associated with microbial dysbiosis in rodent offspring (Hsiao et al., 2013) demonstrating the importance of immune-microbial crosstalk during development. It is therefore clinically relevant to assess changes in the microbiome due to both genetic and environmental influences.
Mouse Models Relevant to ASD
Gut microbial changes and the development of ASD-relevant behaviors have been assessed in multiple preclinical models to assist in unravelling the impacts of autism-associated gene mutations on gut microbiota (Newell et al., 2016; Coretti et al., 2017; Golubeva et al., 2017; Lim et al., 2017; Cristiano et al., 2018; Hosie et al., 2019; Sauer et al., 2019; Sgritta et al., 2019; Septyaningtrias et al., 2020). To understand the mechanisms underlying microbial changes in autism, mouse models are commonly used to highlight shifts in microbial profiles in a controlled environment.
It is important to define microbial profiles in mouse models of ASD to understand how host-microbe interactions might influence GI dysfunction. The NL3R451C, SHANK3 KO and 15q dup mice express autism-associated gene mutations/duplications and show autism-relevant behaviors such as repetitive grooming and reduced interest in novel mice (Tabuchi et al., 2007; Nakatani et al., 2009; Peça et al., 2011). Both NL3R451C and SHANK3 KO mice demonstrate GI dysfunction (Hosie et al., 2019; Sauer et al., 2019). NL3R451C mice show altered faecal microbial populations (Hosie et al., 2019) and SHANK3 KO mice also exhibit shifts in the microbiome (Sauer et al., 2019); Sgritta et al., 2019). In addition to transgenic models, phenotype-first models such as Black and Tan BRachyury (BTBR) mice show behavioral endophenotypes relevant to ASD symptoms, including increased repetitive self-grooming behaviors, abnormal ultrasonic vocalization patterns and well as social approach and reciprocal social interaction deficits (McFarlane et al., 2008; Scattoni et al., 2008; Pearson et al., 2011; Pobbe et al., 2011).
Here we analyzed microbial data from six well-established mouse models of ASD that resulted from our systematic search terms. In addition to highlighting similarities across these datasets, we compare mouse model and clinical ASD microbial studies. Given that shifts in microbial profiles frequently correlate with GI symptoms, a better understanding of how microbes impact the host will enhance treatments for GI dysfunction in patients with ASD. Here we have undertaken a systematic review of the literature to compare preclinical and clinical studies of the microbiome in the context of autism spectrum disorder.
Materials and Methods
An electronic literature search was conducted in PubMed, ProQuest and Scopus database engines to identify appropriate studies using the following medical subject heading terms and text words: “Autism” AND “Gastrointestinal” AND “Microbe OR Microbiome OR Microbiota”. Selected studies were limited to English language studies and there was no restriction regarding age groups, gender/sex, time frame or location. The Preferred Reporting Items for Systematic Reviews and Meta-Analyses (PRISMA) guidelines were applied for the included studies (Page et al., 2021).
Selection Criteria for Study Inclusion/Exclusion
The criteria set for this systematic review included inclusion/exclusion criteria and study eligibility. The inclusion criteria were as follows: primary studies focused only on data relating to changes in the GI microbiome sampled from people with autism and from mouse models of autism. There were no limitations regarding age groups, gender/sex, time frame or location. Exclusion criteria applied included: discarding any study focused on species other than human or mice, studies that did not assess microbial diversity (such as studies focused on specific microbes), studies that did not use molecular methods for microbial analysis, or that included subjects receiving specific treatments or factors which influence the GI microbial community. Furthermore, studies that did not provide relevant results but instead focused on the GI microbiome products such as chemical metabolites or toxins, studies that were not primary articles such as reviews, case reports or meta-analysis studies were omitted.
The PubMed, ProQuest, and Scopus electronic database searches identified 1457 articles (n = 281, 499, and 677, respectively) based on screening the titles and abstracts for the search terms used (Figure 1). From these articles, 21 were selected (13 clinical studies and 9 articles detailing ASD mouse models). During the screening process, a total of 1412 articles out of 1457 were removed due to duplication (n= 1017), other species studies such as rodent or hamster studies (n= 5), containing meta-analysis or being a systematic review (n= 22), or were narrative reviews or non-English studies (n= 373). Afterwards, the remaining 45 articles were screened for eligibility (full-text assessment), 22 articles were fully assessed for eligibility and 23 articles were excluded for the following reasons; the study method did not use molecular analysis for microbial diversity (n= 3), the study focused on specific microbes or species only (n= 9), the study entailed the use of factors that influence the microbiome such as drug treatment or probiotic supplementation (n= 6), or the study did not provide relevant results or specify ASD (n= 5). Finally, 22 studies met the inclusion criteria and were extracted for analysis. Among the 22 studies, 13 reported clinical cases of ASD patients (Table 1) and 9 reported microbial diversity within mouse models of ASD (3 studies for mouse models using environmental factors and 6 studies in genetic mouse models) (Table 2).
Data Extraction
A data extraction form was designed for the eligible studies based on the selection criteria applied [61]. The information extracted for analysis included: the first author’s name, year of publication, species (human or mice), sample size, gender for human and sex for mice, background strain (mice only), type of mutation or environmental agent (mice only), type of specimen collected for analysis, diagnostic methods used for microbial diversity, the unit used to describe microbial community results, microbial taxonomic rank (i.e., phylum, order, class or family).
Results
Predominant Properties of the GI Microbiome in ASD
Thirteen clinical studies met the selection criteria and were assessed in the current study. Most cases reported were male children, where the percentage of males exceeded 80% of the total cases in each study. DNA extraction from faecal specimens and next generation sequencing 16S rRNA gene sequencing were the main methods used for all studies. Since bacteria within gut microbial populations are grouped/classified taxonomically into species, genera, families, orders and classes as well as phyla, analytical methods of interest for this review appropriately classified gut bacterial groupings. Specifically, all studies included in the current review classified microbial taxa and diversity using operational taxonomic unit (OTU) calling using 97% similarity thresholds.
Gut microbial differences occurring in individuals with ASD were compared (Table 3). Overall, in both ASD individuals and control individuals Firmicutes and Bacteroidetes were the most predominant microbial taxa while Proteobacteria and Actinobacteria were the least predominant phyla (Son et al., 2015; Strati et al., 2017; Carissimi et al., 2019; Kong et al., 2019; Liu et al., 2019; Niu et al., 2019; Dan et al., 2020; Zou et al., 2020). In individuals with ASD, four studies reported an increase in the Firmicutes/Bacteroidetes ratio driven by either a decrease in Bacteroidetes (Strati et al., 2017; Kong et al., 2019; Niu et al., 2019) or an increase in Firmicutes (Dan et al., 2020). Conversely, two studies reported a decrease in the Firmicutes/Bacteroidetes ratio in ASD individuals driven by a reduction in Firmicutes (Liu et al., 2019; Zou et al., 2020).
Most studies focused on the phylum and genus level, however, some family level analyses showed increased abundance of Veillonellaceae, Pseudomonadaceae, Enterobacteriaceae, Bacteroidaceae, Ruminucoccaceae, Lachnospiraceae, Prevotellaceae in individuals with ASD (Rose et al., 2018; Liu et al., 2019; Zou et al., 2020). In addition, almost 27 genera had altered abundance in ASD patients (Gondalia et al., 2012; Strati et al., 2017; Kong et al., 2019; Liu et al., 2019; Dan et al., 2020; Wang et al., 2020; Zou et al., 2020; Zurita et al., 2020) compared to neurotypical controls. Of these, some genera were highlighted in multiple clinical studies including Bacteroides (increased in two studies (Zou et al., 2020; Zurita et al., 2020), decreased in two studies (Niu et al., 2019; Dan et al., 2020), Ruminococcus increased in two studies (Wang et al., 2020; Zurita et al., 2020), decreased in a single study (Niu et al., 2019), Bifidobacterium increased in a sole study (Dan et al., 2020), decreased in two of the included studies (Niu et al., 2019; Wang et al., 2020), Akkermansia increased in one study (Zurita et al., 2020), decreased in another (Zou et al., 2020) and Clostridium increased in one study (Wang et al., 2020) whereas it was decreased in another report (Zou et al., 2020).
When considering morphology (Figure 2), the largest group of microbes that showed altered abundance were spherical (Coprococcus, Dialister, Ruminococcus, Veillonella and Victivallales) or rod shaped (Alistipes, Butyricimonas, Catenibacterium, Cetobacterium, Collinsella, Corynebacterium, Escherichia-Shigella, Lachnospiracie incertae sedis, Roseburia, Oscillospira).
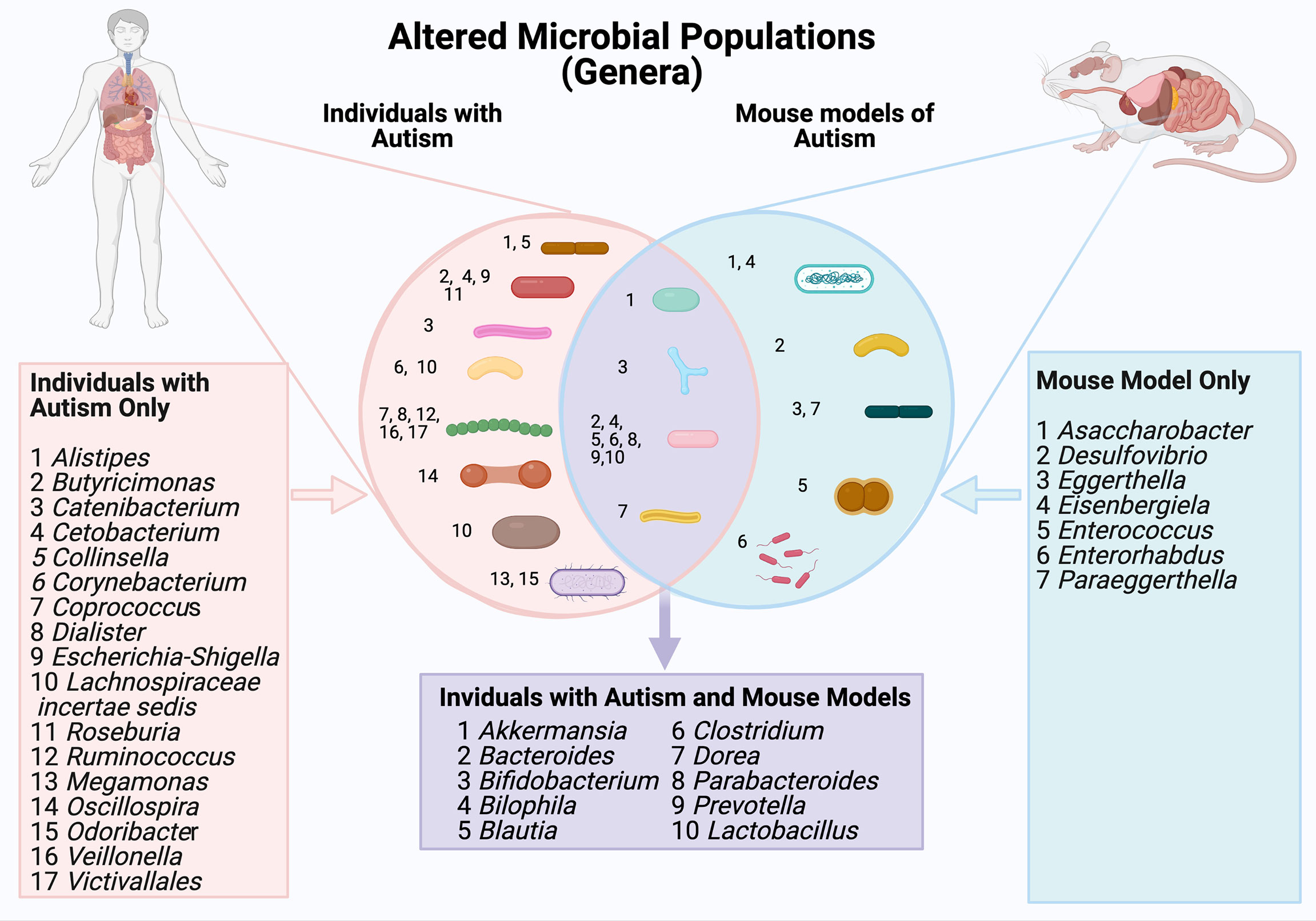
Figure 2 A comparison of gut microbial populations in autism: clinical and mouse models. Microbial genera are grouped by cellular morphology (i.e., spherical, rod shaped and bifurcated).
Predominant Properties of the GI Microbiome in Mice
Nine studies investigating the microbiome in mouse models of ASD met the selection criteria for this study. The mouse models studied in these publications were: NL3R451C (Nlgn3tm1Sud/J), SHANK3 KO and 15q dup mice, and BTBR (BTBR T tf/J). Three of the included studies were conducted on models expressing ASD-associated genetic mutations (NL3R451C, SHANK3 KO and 15q dup mice) (Hosie et al., 2019; Sauer et al., 2019; Septyaningtrias et al., 2020), in addition to three studies of a ‘phenotype first’ model of ASD (BTBR mice) (Newell et al., 2016; Golubeva et al., 2017; Cristiano et al., 2018) as well as three studies conducted on environmental ASD mouse models that show behavioral and neuropathological symptoms relevant to autism through maternal administration of polyinosinic: polycytidylic acid (poly I:C) or valproic acid (VPA) (Lim et al., 2017), maternal immune activation (MIA) (Hsiao et al., 2013), or transplantation of microbial samples from individuals with ASD to germ-free mice (Sharon et al., 2019).
Gut microbial differences in mouse models of ASD were compared (Table 4). Overall, Firmicutes and Bacteroidetes were the predominant taxa at the phylum level for all ASD mouse models studied. Multiple studies report changes in the ratios of Firmicutes and Bacteroidetes in these models. A study by Lim et al. (2017) reported a small increase in Firmicutes phylum alongside a slight decrease in Bacteroidetes phylum relative to controls (Lim et al., 2017), while Hsiao et al. (2013) showed an increase in Bacteroidetes and a decrease in Firmicutes phyla (Hsiao et al., 2013). In addition, Sauer et al. (2019) reported a higher abundance of the phylum Actinobacteria and found that the phyla Deferribacteres, Chlamydiae and Tenericutes were only present in Shank3αβ KO mice when compared to controls (Sauer et al., 2019). Furthermore, offspring of germ-free mice engrafted with ASD patient microbial samples have altered expression of amplicon sequence variants within the Bacteroidetes phyla, Verrucomicrobia, α- and β-Proteobacteria phyla (Sharon et al., 2019). At the class level, Hsiao et al. (2013) reported that Clostridia and Bacteroidia are predominant in maternal immune activation (MIA) offspring (Hsiao et al., 2013) compared to untreated control mice. Furthermore, four studies on genetically modified mice (Newell et al., 2016; Golubeva et al., 2017; Hosie et al., 2019; Sauer et al., 2019) focused on mouse faecal microbial abundance at the family level found that i) Lachnospiraceae is more abundant in NL3R451C mice, ii) a decrease in S24–7 family Enterobacteriacea and Lachnospiraceae in BTBR mice and iii) the Bifidobacteriaceae and Eggerthellaceae families are predominant in Shank3 KO mice compared to controls.
Studies of environmental factors influencing autism-like phenotypes identified increased abundance of Lachnospiraceae, Prevotellaceae and Porphyromonadaceae at the family level in MIA offspring mice (Hsiao et al., 2013). Similarly, Lachnospiraceae also showed increased abundance in both the Poly I:C and valproic acid models together with an increased abundance of Rikenellaceae, Peptostreptococcaceae, Enterococcaceae (Lim et al., 2017). At the family level, an increased abundance of Lachnospiraceae and a decreased abundance of Bacteriodetes was also identified in offspring mice grafted with autistic humanized microbiome (Sharon et al., 2019).
At the genus level, a range of bacteria including Asaccharobacter, Eggerthella, Enterorhabdus and Paraeggerthella had elevated abundance in Shank3 knockout mice (Sauer et al., 2019). Similarly, in the environmental VPA model, the abundance of both Prevotella and Desulfovibrio was decreased (Lim et al., 2017). In contrast, an increased abundance of Lactobacillus was also reported in the BTBR mouse model of ASD compared to a reference mouse strain (Newell et al., 2016). One report also showed an increased abundance of Akkermansia and Sutterella in feces from mice that received fecal samples from individuals with ASD (Sharon et al., 2019). Incidentally, microbes modified in ASD mouse models were predominantly rod shaped (i.e., Asaccharobacter, Desulfovibrio, Eggerthella, Eisenbergiela, Enterorhabdus and Paraeggerthella).
Comparison of Microbial Profiles in Autism Patients and Mouse Preclinical Models
Detailed comparisons made at multiple classification levels between ASD clinical samples and autism mouse models revealed subtle changes in the composition of the faecal microbiome. Firmicutes and Bacteroidetes remained the predominant phyla in both control and autism-relevant groups in both human and mouse studies. Differences in the Firmicutes : Bacteroidetes ratio were observed in 6 clinical studies (Strati et al., 2017; Kong et al., 2019; Liu et al., 2019; Niu et al., 2019; Dan et al., 2020; Zou et al., 2020) and in four ASD mouse studies (Newell et al., 2016; Golubeva et al., 2017; Hosie et al., 2019; Sauer et al., 2019). Interestingly, an altered abundance of Actinobacteria and Proteobacteria phylum was identified in both ASD patient studies and mouse models of ASD (Kong et al., 2019; Niu et al., 2019; Sauer et al., 2019; Dan et al., 2020; Wang et al., 2020). In both clinical studies and autism mouse models, the abundance of Actinobacteria and Proteobacteria was influenced by the presence of ASD or an ASD-associated mutation (Kong et al., 2019; Sauer et al., 2019; Dan et al., 2020; Zou et al., 2020). In addition, Clostridia and Bacteroidia classes were present at higher abundance in both ASD patients and mouse models of ASD compared to non-ASD subjects/controls (Hsiao et al., 2013; Berding and Donovan, 2020).
Gut microbial differences occurring in both ASD patients and mouse models of ASD were identified (Table 5 and Figure 2). At the family level, Peptostreptococcaceae, Lachnospiraceae, Prevotellaceae showed altered abundance in both patient and mouse models (Hsiao et al., 2013; Golubeva et al., 2017; Lim et al., 2017; Rose et al., 2018; Hosie et al., 2019; Liu et al., 2019; Zou et al., 2020). At the genus level, the abundance of Bilophilia, Clostridium, Dorea and Lactobacillus was increased in both clinical studies and mouse models (Golubeva et al., 2017; Lim et al., 2017; Strati et al., 2017; Wang et al., 2020; Zou et al., 2020). Also at the genus level, there was altered abundance of Akkermansia, Bacteroides, Bifidobacterium, Prevotella and Parabacteroides in both clinical autistic participants and in mouse models (Golubeva et al., 2017; Cristiano et al., 2018; Niu et al., 2019; Sharon et al., 2019; Zou et al., 2020; Zurita et al., 2020). In contrast, the abundance of the genera Blautia was decreased in both mouse models and clinical case studies (Golubeva et al., 2017; Niu et al., 2019). Interestingly, the morphology of bacteria with altered abundance in both human and mouse model samples was overwhelmingly rod shaped (i.e.Bacteroides, Biolphila, Blautia, Clostridium, Dorea, Parabacteroides, Prevotella, Lactobacillus).
Changes in specific components of gut microbiota profiles are observed in samples from children with ASD and across multiple mouse models of autism. Changes in the abundance of Bacteroides, Akkermansia, Bifidobacterium, Bilophila, Blautia, Clostridium and Dorea, Parabacteroides, Prevotella and Lactobacillus are seen in both children with ASD and mouse models (highlighted in mauve). The direction of change in abundance levels in each of these clinical samples/models are specified in Table 5.
Discussion
We conducted a systematic review of microbial diversity in ASD patients and in mouse models of ASD. Twenty-two studies were included (13 articles describing dysbiosis in clinical cases of ASD and 9 articles focused on microbial shifts in ASD mouse models) which further validate mouse models with respect to observations from clinical cohorts.
Clinical Microbial Dysbiosis in ASD
Microbial dysbiosis profiles identified from clinical ASD cases have been associated with intestinal inflammation and GI symptoms such as constipation and diarrhea, which are commonly observed in individuals with ASD (Adams et al., 2011; Gorrindo et al., 2012; Rose et al., 2018; Pagani et al., 2019). Clinical reports highlighted an increased abundance of numerous bacterial genera in stool samples from individuals with ASD, including pathogenic microbes linked to GI inflammation and severe symptoms for ASD patients such as Clostridium, Blautia, Dialister, Escherichia-Shigella, Prevotella and Streptococcus. Others, such as microbes belonging to the Akkermansia genus of mucin-degrading bacterium, were increased in prevalence in ASD individuals, and are known to affect the structure of the gastrointestinal tract through metabolic activities and correlate with changes in gut permeability (Wang et al., 2011; Dao et al., 2016). In contrast, the abundance of members of the Bacteroides genus (microbes providing beneficial carbohydrate fermentation associated with the production of the neurotransmitter gamma aminobutyric acid (GABA) in the gut (Strandwitz et al., 2019) was increased in ASD clinical samples.
The current study concurs with previous assessments of the literature demonstrating a variance in microbial diversity of individuals with ASD. Similar to the systematic review by Iglesias-Vázquez et al., (2020) that focused on the phylum and genera level, we identified changes in the predominance of Firmicutes and Bacteroidetes, as well as altered abundance of Proteobacteria, Actinobacteria and Verrucomicrobia in stool samples of ASD patients. As per our findings, these authors also showed an increase in Bacteroides, Parabacteroides and Clostridium. In contrast, Iglesias-Vázquez et al., 2020 reported a decreased abundance of Coprococcus and Bifidobacterium in stool samples from ASD patients, whereas we identified studies with increases in their abundance (Dan et al., 2020; Zurita et al., 2020). Additionally, a review by Srikantha & Mohajeri (2019) reported altered microbial diversity in ASD patients with increases in the Firmicutes to Bacteroidetes ratio, and an increase in abundance of Clostridium genera, similar to observations in the current review.
Of particular interest, we noted an increased abundance of Clostridium in several clinical studies. In individuals with autism, an increased abundance of Clostridium, a gram-positive anaerobic bacterium, is associated with increased GI inflammation in the colon (Song et al., 2004; Finegold et al., 2010; Luna et al., 2017). Clostridium spp. have multiple virulence factors, which include extracellular toxins (alpha, beta, epsilon and iota toxin) and hydrolytic enzymes (Popoff and Poulain, 2010). A higher abundance of Clostridium isolated from the faecal specimens of individuals with ASD compared to that of control subjects has been reported in multiple studies (Hsiao, 2014; Argou-Cardozo and Zeidán-Chuliá, 2018). Relevant to ASD populations, Emanuele et al. (2010) identified higher levels of enterotoxin in serum samples from ASD patients than controls and therefore proposed that these enterotoxins significantly contribute to severe GI symptoms in autistic individuals (Emanuele et al., 2010).
In addition, we report altered abundance (increased in one study and decreased in two) of the probiotic Bifidobacterium. Bifidobacteria regulate immune activity via fermentation of complex polysaccharides in the gastrointestinal tract and as such are believed to exert positive health benefits on the host (Menard et al., 2005; Popoff and Poulain, 2010; Dinan et al., 2013; Delcarua et al., 2016). Similarly, both increases and decreases in abundance were reported in Ruminococcus, an important bacterium for the digestion of starch. Relevant to this review, abundance of Ruminococcus has been proposed as an indicator for irritable bowel syndrome (Malinen et al., 2010).
Overall, analyses of stool samples from participants diagnosed with ASD revealed an imbalance of gut microbial communities including bacteria previously reported to alter gut function. Although precise biological mechanisms are unknown, microbial alterations may contribute to GI symptoms and behavioral changes in ASD via gut-brain axis dysfunction.
Preclinical Microbial Dysbiosis in Mouse Models of ASD
Firmicutes and Bacteroidetes phyla comprise the predominant murine microbial community, making up more than 70% of the microbial profile. Importantly, this finding was consistent across model construct (i.e., mice expressing autism-associated genetic mutations or subjected to environmental factors associated with ASD-relevant phenotypes in mice such as maternal immune disorders or valproic acid exposure). Nevertheless, we observed subtle differences in predominant bacterial phyla in each of the ASD mice models, which likely reflects differences in genetic mutations and environmental factors. In a study by Lim and colleagues, mice exposed to polyinosinic: polycytidylic acid (poly I:C) or valproic acid (VPA) showed a subtle increase in the abundance of Firmicutes and a decrease in abundance of Bacteroidetes (Lim et al., 2017). In contrast, BTBR mice showed an increased abundance of bacteria within the Bacteroidetes phylum and a decrease in bacteria belonging to the Firmicutes phylum (Cristiano et al., 2018). In BTBR mice, a decrease in Bifidobacterium and Blautia abundance has been shown to reduce bile-metabolism due to impaired tryptophan metabolism in the gut (Golubeva et al., 2017). This observation further indicates that microbial dysbiosis may lead to dysregulation of host-microbial interactions and contribute to pathophysiological outcomes. In addition, a higher abundance of the phylum Actinobacteria alongside a significantly lower abundance of the phyla Proteobacteria and Verrucomicrobia were reported in faecal samples from Shank3αβ KO mice (Sauer et al., 2019). In the same preclinical model, microbes within the phyla Deferribacteres, Chlamydiae and Tenericutes were present exclusively in Shank3αβ KO mice and not controls (Sauer et al., 2019). Collectively, these findings show that the microbial community is altered in a variety of mouse models of ASD.
In the majority of ASD mouse models assessed, an increased abundance of potentially pathogenic microbes (i.e., correlating with severe gastrointestinal symptoms in patients such as Clostridium) and a decreased abundance of probiotic microbes (which play an important role in immunity as well as in maintaining physiological homeostasis of the gut ecosystem) such as Bifidobacterium and Blautia was observed.
A Comparison of Clinical and Preclinical Microbial Profiles in ASD
When comparing across species, most human and mouse microbiome studies reported an altered Bacteroidetes : Firmicutes ratio at the phylum level. At the family level, Lachnospiraceae and Prevotellaceae showed increased abundance in clinical studies but showed variation in the mice studies with both increased and decreased changes in abundance. Conversely, Peptostreptococcaceae had decreased abundance in clinical studies and increased abundance in mice studies. At the genus level, an increased abundance of microbes such as Clostridium, Bacteroides, Bilophila, Dorea and Parabacteroides were observed in both mouse models of autism and autistic individuals. However, the abundance of Bifidobacterium and Blautia genera fluctuated across ASD patient studies but were decreased in several mouse models of autism. This variation in findings could be due to species, genetic and/or environmental factors that directly affect the microbial ecosystem within the gastrointestinal tract. It is therefore important to study microbial diversity in mouse models where external/influencing factors can be more readily controlled and to characterize responses to specific gene mutations or environmental insults.
Conclusions
Here we highlight common cross-species alterations of gut microbial profiles in clinical cases of ASD and mouse models of autism. We demonstrate an increase in abundance of genus level microbes such as Clostridium, Lactobacillus, Dorea and Bilophilia and a decrease of Blautia in both the clinical setting and in mouse models of autism. Given the presence of these changes across both clinical and preclinical studies, these microbial profiles could potentially contribute to GI symptoms observed in individuals with ASD. This systematic review provides further support for preclinical models as tools to identify potential therapeutic targets for treating gastrointestinal disorders in ASD.
Author Contributions
SH, AF, EH-Y conceived the study. MA conducted the literature searches with support from SH and EH-Y. AS, JW and AF contributed to the interpretation of microbial results. All authors drafted the manuscript. All authors contributed to the article and approved the submitted version.
Funding
This research was funded by an Australian Research Council Future Fellowship to EH-Y (FT160100126) and a National Health and Medical Research Council Ideas grant to EH-Y and AF (APP2003848).
Conflict of Interest
The authors declare that the research was conducted in the absence of any commercial or financial relationships that could be construed as a potential conflict of interest.
Publisher’s Note
All claims expressed in this article are solely those of the authors and do not necessarily represent those of their affiliated organizations, or those of the publisher, the editors and the reviewers. Any product that may be evaluated in this article, or claim that may be made by its manufacturer, is not guaranteed or endorsed by the publisher.
References
Adams, J. B., Johansen, L. J., Powell, L. D., Quig, D., Rubin, R. A. (2011). Gastrointestinal Flora and Gastrointestinal Status in Children With Autism – Comparisons to Typical Children and Correlation With Autism Severity. BMC Gastroenterol. 11 (1), 22. doi: 10.1186/1471-230X-11-22
Almeida, A., Mitchell, A. L., Boland, M., Forster, S. C., Gloor, G. B., Tarkowska, A., et al. (2019). A New Genomic Blueprint of the Human Gut Microbiota. Nature 568 (7753), 499–504. doi: 10.1038/s41586-019-0965-1
American Psychiatric Association (2013). Diagnostic and Statistical Manual of Mental Disorders: DSM-5. 5th Edn (Washington, D.C: American Psychiatric Publishing).
Argou-Cardozo, I., Zeidán-Chuliá, F. (2018). Clostridium Bacteria and Autism Spectrum Conditions: A Systematic Review and Hypothetical Contribution of Environmental Glyphosate Levels. Med. Sci. 6 (2), 29. doi: 10.3390/medsci6020029
Attlee, A., Kassem, H., Hashim, M., Obaid, R. S. (2015). Physical Status and Feeding Behavior of Children With Autism. Indian J. Pediatr. 82 (8), 682–687. doi: 10.1007/s12098-015-1696-4
Berding, K., Donovan, S. M. (2020). Dietary Patterns Impact Temporal Dynamics of Fecal Microbiota Composition in Children With Autism Spectrum Disorder. Front. Nutr. 6. doi: 10.3389/fnut.2019.00193
Bourgeron, T. (2009). A Synaptic Trek to Autism. Curr. Opin. Neurobiol. 19 (2), 231–234. doi: 10.1016/j.conb.2009.06.003
Carissimi, C., Laudadio, I., Palone, F., Fulci, V., Cesi, V., Cardona, F., et al. (2019). Functional Analysis of Gut Microbiota and Immunoinflammation in Children With Autism Spectrum Disorders. Dig Liver Dis. 51 (10), 1366–1374. doi: 10.1016/j.dld.2019.06.006
Christensen, J., Grønborg, T. K., Sørensen, M. J., Schendel, D., Parner, E. T., Pedersen, L. H., et al. (2013). Prenatal Valproate Exposure and Risk of Autism Spectrum Disorders and Childhood Autism. JAMA 309 (16), 1696–1703. doi: 10.1001/JAMA.2013.2270
Clemente, J. C., Ursell, L. K., Parfrey, L. W., Knight, R. (2012). The Impact of the Gut Microbiota on Human Health: An Integrative View. Cell 148 (6), 1258–1270. doi: 10.1016/j.cell.2012.01.035
Coretti, L., Cristiano, C., Florio, E., Scala, G., Lama, A., Keller, S., et al. (2017). Sex-Related Alterations of Gut Microbiota Composition in the BTBR Mouse Model of Autism Spectrum Disorder. Sci. Rep. 7 (1), 45356. doi: 10.1038/srep45356
Cristiano, C., Pirozzi, C., Coretti, L., Cavaliere, G., Lama, A., Russo, R., et al. (2018). Palmitoylethanolamide Counteracts Autistic-Like Behaviours in BTBR T+tf/J Mice: Contribution of Central and Peripheral Mechanisms. Brain Behavior Immun. 74, 166–175. doi: 10.1016/j.bbi.2018.09.003
Dan, Z., Mao, X., Liu, Q., Guo, M., Zhuang, Y., Liu, Z., et al. (2020). Altered Gut Microbial Profile is Associated With Abnormal Metabolism Activity of Autism Spectrum Disorder. Gut Microbes 11 (5), 1246–1267. doi: 10.1080/19490976.2020.1747329
Dao, M. C., Everard, A., Aron-Wisnewsky, J., Sokolovska, N., Prifti, E., Verger, E. O., et al. (2016). Akkermansia Muciniphila and Improved Metabolic Health During a Dietary Intervention in Obesity: Relationship With Gut Microbiome Richness and Ecology. Gut 65 (3), 426. doi: 10.1136/gutjnl-2014-308778
De Angelis, M., Piccolo, M., Vannini, L., Siragusa, S., De Giacomo, A., Serrazzanetti, D. I., et al. (2013). Fecal Microbiota and Metabolome of Children With Autism and Pervasive Developmental Disorder Not Otherwise Specified. PLoS One 8 (10), e76993. doi: 10.1371/journal.pone.0076993
Delcarua, C., Alexandrua, I., Podgoreanua, P., Cristeab, V. C., Bleotuc, C., Chifiriucc, M. C., et al. (2016). Antagonistic Activities of Some Bifidobacterium Sp. Strains Isolated From Resident Infant Gastrointestinal Microbiota on Gram-Negative Enteric Pathogens. Anaerobe 39, 39–44. doi: 10.1016/j.anaerobe.2016.02.010
Dinan, T. G., Stanton, C., Cryan, J. F. (2013). Psychobiotics: A Novel Class of Psychotropic. Biol. Psychiatry 74 (10), 720–726. doi: 10.1016/j.biopsych.2013.05.001
Durand, C. M., Betancur, C., Boeckers, T. M., Bockmann, J., Chaste, P., Fauchereau, F., et al. (2007). Mutations in the Gene Encoding the Synaptic Scaffolding Protein SHANK3 are Associated With Autism Spectrum Disorders. Nat. Genet. 39 (1), 25–27. doi: 10.1038/ng1933
Emanuele, E., Orsi, P., Boso, M., Broglia, D., Brondino, N., Barale, F., et al. (2010). Low-Grade Endotoxemia in Patients With Severe Autism. Neurosci. Lett. 471 (3), 162–165. doi: 10.1016/j.neulet.2010.01.033
Esnafoglu, E., Cırrık, S., Ayyıldız, S. N., Erdil, A., Ertürk, E. Y., Daglı, A., et al. (2017). Increased Serum Zonulin Levels as an Intestinal Permeability Marker in Autistic Subjects. J. Pediatr. 188, 240–244. doi: 10.1016/j.jpeds.2017.04.004
Etherton, M., Földy, C., Sharma, M., Tabuchi, K., Liu, X., Shamloo, M., et al. (2011). Autism-Linked Neuroligin-3 R451C Mutation Differentially Alters Hippocampal and Cortical Synaptic Function. Proc. Natl. Acad. Sci. 108 (33), 13764–13769. doi: 10.1073/pnas.1111093108
Finegold, S. M., Dowd, S. E., Gontcharova, V., Liu, C., Henley, K. E., Wolcott, R. D., et al. (2010). Pyrosequencing Study of Fecal Microflora of Autistic and Control Children. Anaerobe 16 (4), 444–453. doi: 10.1016/j.anaerobe.2010.06.008
Golubeva, A. V., Joyce, S. A., Moloney, G., Burokas, A., Sherwin, E., Arboleya, S., et al. (2017). Microbiota-Related Changes in Bile Acid & Tryptophan Metabolism are Associated With Gastrointestinal Dysfunction in a Mouse Model of Autism. EBioMedicine 24, 166–178. doi: 10.1016/j.ebiom.2017.09.020
Gondalia, S. V., Palombo, E. A., Knowles, S. R., Cox, S. B., Meyer, D., Austin, D. W. (2012). Molecular Characterisation of Gastrointestinal Microbiota of Children With Autism (With and Without Gastrointestinal Dysfunction) and Their Neurotypical Siblings. Autism Res. 5 (6), 419–427. doi: 10.1002/aur.1253
Gorrindo, P., Williams, K. C., Lee, E. B., Walker, L. S., McGrew, S. G., Levitt, P. (2012). Gastrointestinal Dysfunction in Autism: Parental Report, Clinical Evaluation, and Associated Factors. Autism Res. Off. J. Int. Soc. Autism Res. 5 (2), 101–108. doi: 10.1002/aur.237
Guang, S., Pang, N., Deng, X., Yang, L., He, F., Wu, L., et al. (2018). Synaptopathology Involved in Autism Spectrum Disorder. Front. Cell. Neurosci. 12. doi: 10.3389/fncel.2018.00470
Herath, M., Hosie, S., Bornstein, J. C., Franks, A. E., Hill-Yardin, E. L. (2020). The Role of the Gastrointestinal Mucus System in Intestinal Homeostasis: Implications for Neurological Disorders. Front. Cell Infect. Microbiol. 10. doi: 10.3389/fcimb.2020.00248
Holingue, C., Newill, C., Lee, L.-C., Pasricha, P. J., Daniele Fallin, M. (2018). Gastrointestinal Symptoms in Autism Spectrum Disorder: A Review of the Literature on Ascertainment and Prevalence. Autism Res. 11 (1), 24–36. doi: 10.1002/aur.1854
Hosie, S., Ellis, M., Swaminathan, M., Ramalhosa, F., Seger, G. O., Balasuriya, G. K., et al. (2019). Gastrointestinal Dysfunction in Patients and Mice Expressing the Autism-Associated R451C Mutation in Neuroligin-3. Autism Res. Off. J. Int. Soc. Autism Res. 12 (7), 1043–1056. doi: 10.1002/aur.2127
Hosie, S., Malone, D. T., Liu, S., Glass, M., Adlard, P. A., Hannan, A. J., et al. (2018). Altered Amygdala Excitation and CB1 Receptor Modulation of Aggressive Behavior in the Neuroligin-3R451C Mouse Model of Autism. Front. Cell. Neurosci. 12 (234). doi: 10.3389/fncel.2018.00234
Hsiao, E. Y. (2014). Gastrointestinal Issues in Autism Spectrum Disorder. Harvard Rev. Psychiatry 22 (2), 104–111. doi: 10.1097/HRP.0000000000000029
Hsiao, E. Y., McBride, S. W., Hsien, S., Sharon, G., Hyde, E. R., McCue, T., et al. (2013). Microbiota Modulate Behavioral and Physiological Abnormalities Associated With Neurodevelopmental Disorders. Cell 155 (7), 1451–1463. doi: 10.1016/j.cell.2013.11.024
Iglesias-Vázquez, L., Van Ginkel Riba, G., Arija, V., Canals, J. (2020). Composition of Gut Microbiota in Children With Autism Spectrum Disorder: A Systematic Review and Meta-Analysis. Nutrients 12 (3), 792. doi: 10.3390/nu12030792
Jamain, S., Quach, H., Betancur, C., Råstam, M., Colineaux, C., Gillberg, I. C., et al. (2003). Mutations of the X-Linked Genes Encoding Neuroligins NLGN3 and NLGN4 are Associated With Autism. Nat. Genet. 34 (1), 27–29. doi: 10.1038/ng1136
Kong, X., Liu, J., Cetinbas, M., Sadreyev, R., Koh, M., Huang, H., et al. (2019). New and Preliminary Evidence on Altered Oral and Gut Microbiota in Individuals With Autism Spectrum Disorder (ASD): Implications for ASD Diagnosis and Subtyping Based on Microbial Biomarkers. Nutrients 11 (9), 2128. doi: 10.3390/nu11092128
Lai, M. C., Kassee, C., Besney, R., Bonato, S., Hull, L., Mandy, W., et al. (2019). Prevalence of Co-Occurring Mental Health Diagnoses in the Autism Population: A Systematic Review and Meta-Analysis. Lancet Psychiatry 6 (10), 819–829. doi: 10.1016/S2215-0366(19)30289-5
Lee, C. Y. Q., Franks, A. E., Hill-Yardin, E. L. (2020). Autism-Associated Synaptic Mutations Impact the Gut-Brain Axis in Mice. Brain behavior Immun. 88, 275–282. doi: 10.1016/j.bbi.2020.05.072
Leembruggen, A. J. L., Balasuriya, G. K., Zhang, J., Schokman, S., Swiderski, K., Bornstein, J. C., et al. (2020). Colonic Dilation and Altered Ex Vivo Gastrointestinal Motility in the Neuroligin-3 Knockout Mouse. Autism Res. 13 (5), 691–701. doi: 10.1002/aur.2109
Levy, D., Ronemus, M., Yamrom, B., Lee, Y. H., Leotta, A., Kendall, J., et al. (2011). Rare De Novo and Transmitted Copy-Number Variation in Autistic Spectrum Disorders. Neuron 70 (5), 886–897. doi: 10.1016/j.neuron.2011.05.015
Lim, J. S., Lim, M. Y., Choi, Y., Ko, G. (2017). Modeling Environmental Risk Factors of Autism in Mice Induces IBD-Related Gut Microbial Dysbiosis and Hyperserotonemia. Mol. Brain 10 (1), 14. doi: 10.1186/s13041-017-0292-0
Liu, S., da Cunha, A. P., Rezende, R. M., Cialic, R., Wei, Z., Bry, L., et al. (2016). The Host Shapes the Gut Microbiota via Fecal microRNA. Cell Host Microbe 19 (1), 32–43. doi: 10.1016/j.chom.2015.12.005
Liu, S., Li, E., Sun, Z., Fu, D., Duan, G., Jiang, M., et al. (2019). Altered Gut Microbiota and Short Chain Fatty Acids in Chinese Children With Autism Spectrum Disorder. Sci. Rep. 9 (1), 287. doi: 10.1038/s41598-018-36430-z
Lozupone, C. A., Stombaugh, J. I., Gordon, J. I., Jansson, J. K., Knight, R. (2012). Diversity, Stability and Resilience of the Human Gut Microbiota. Nature 489 (7415), 220–230. doi: 10.1038/nature11550
Luna, R. A., Oezguen, N., Balderas, M., Venkatachalam, A., Runge, J. K., Versalovic, J., et al. (2017). Distinct Microbiome-Neuroimmune Signatures Correlate With Functional Abdominal Pain in Children With Autism Spectrum Disorder. Cell. Mol. Gastroenterol. Hepatol. 3 (2), 218–230. doi: 10.1016/j.jcmgh.2016.11.008
Malinen, E., Krogius-Kurikka, L., Lyra, A., Nikkilä, J., Jääskeläinen, A., Rinttilä, T., et al. (2010). Association of Symptoms With Gastrointestinal Microbiota in Irritable Bowel Syndrome. World J. Gastroenterol. 16 (36), 4532–4540. doi: 10.3748/wjg.v16.i36.4532
Margolis, K. G., Cryan, J. F., Mayer, E. A. (2021). The Microbiota-Gut-Brain Axis: From Motility to Mood. Gastroenterology 160 (5), 1486–1501. doi: 10.1053/j.gastro.2020.10.066
Martín, R., Miquel, S., Ulmer, J., Langella, P., Bermúdez-Humarán, L. G. (2014). Gut Ecosystem: How Microbes Help Us. Beneficial Microbes 5 (3), 219–233. doi: 10.3920/BM2013.0057
Matson, M. L., Matson, J. L., Beighley, J. S. (2011). Comorbidity of Physical and Motor Problems in Children With Autism. Res. Dev. Disabil. 32 (6), 2304–2308. doi: 10.1016/j.ridd.2011.07.036
Matta, S. M., Hill-Yardin, E. L., Crack, P. J. (2019). The Influence of Neuroinflammation in Autism Spectrum Disorder. Brain Behav. Immun. 79, 75–90. doi: 10.1016/j.bbi.2019.04.037
McFarlane, H. G., Kusek, G. K., Yang, M., Phoenix, J. L., Bolivar, V. J., Crawley, J. N. (2008). Autism-Like Behavioral Phenotypes in BTBR T+tf/J Mice. Genes Brain Behav. 7 (2), 152–163. doi: 10.1111/j.1601-183X.2007.00330.x
Menard, S., Laharie, D., Asensio, C., Vidal-Martinez, T., Candalh, C., Rullier, A., et al. (2005). Bifidobacterium Breve and Streptococcus Thermophilus Secretion Products Enhance T Helper 1 Immune Response and Intestinal Barrier in Mice. Exp. Biol. Med. 230 (10), 749–756. doi: 10.1177/153537020523001008
Nakatani, J., Tamada, K., Hatanaka, F., Ise, S., Ohta, H., Inoue, K., et al. (2009). Abnormal Behavior in a Chromosome- Engineered Mouse Model for Human 15q11-13 Duplication Seen in Autism. Cell 137 (7), 1235–1246. doi: 10.1016/j.cell.2009.04.024
Newell, C., Bomhof, M. R., Reimer, R. A., Hittel, D. S., Rho, J. M., Shearer, J. (2016). Ketogenic Diet Modifies the Gut Microbiota in a Murine Model of Autism Spectrum Disorder. Mol. Autism 7 (1), 37. doi: 10.1186/s13229-016-0099-3
Niu, M., Li, Q., Zhang, J., Wen, F., Dang, W., Duan, G., et al. (2019). Characterization of Intestinal Microbiota and Probiotics Treatment in Children With Autism Spectrum Disorders in China. Front. Neurol. 10. doi: 10.3389/fneur.2019.01084
Pagani, M., Bertero, A., Liska, A., Galbusera, A., Sabbioni, M., Barsotti, N., et al. (2019). Deletion of Autism Risk Gene Shank3 Disrupts Prefrontal Connectivity. J. Neurosci. Off. J. Soc. Neurosci. 39 (27), 5299–5310. doi: 10.1523/JNEUROSCI.2529-18.2019
Page, M. J., Moher, D., Bossuyt, P. M., Boutron, I., Hoffmann, T. C., Mulrow, C. D., et al. (2021). PRISMA 2020 Explanation and Elaboration: Updated Guidance and Exemplars for Reporting Systematic Reviews. Bmj 372, n160. doi: 10.1136/bmj.n160
Pearson, B. L., Pobbe, R. L. H., Defensor, E. B., Oasay, L., Bolivar, V. J., Blanchard, D. C., et al. (2011). Motor and Cognitive Stereotypies in the BTBR T+tf/J Mouse Model of Autism. Genes Brain Behav. 10 (2), 228–235. doi: 10.1111/j.1601-183X.2010.00659.x
Peça, J., Feliciano, C., Ting, J. T., Wang, W., Wells, M. F., Venkatraman, T. N., et al. (2011). Shank3 Mutant Mice Display Autistic-Like Behaviours and Striatal Dysfunction. Nature 472 (7344), 437–442. doi: 10.1038/nature09965
Pobbe, R. L. H., Defensor, E. B., Pearson, B. L., Bolivar, V. J., Blanchard, D. C., Blanchard, R. J. (2011). General and Social Anxiety in the BTBR T+ Tf/J Mouse Strain. Behav. Brain Res. 216 (1), 446–451. doi: 10.1016/j.bbr.2010.08.039
Poolchanuan, P., Unagul, P., Thongnest, S., Wiyakrutta, S., Ngamrojanavanich, N., Mahidol, C., et al. (2020). An Anticonvulsive Drug, Valproic Acid (Valproate), has Effects on the Biosynthesis of Fatty Acids and Polyketides in Microorganisms. Sci. Rep. 10 (1), 9300. doi: 10.1038/s41598-020-66251-y
Popoff, M. R., Poulain, B. (2010). Bacterial Toxins and the Nervous System: Neurotoxins and Multipotential Toxins Interacting With Neuronal Cells. Toxins 2 (4), 683–737. doi: 10.3390/toxins2040683
Rinninella, E., Raoul, P., Cintoni, M., Franceschi, F., Miggiano, G. A. D., Gasbarrini, A., et al. (2019). What is the Healthy Gut Microbiota Composition? A Changing Ecosystem Across Age, Environment, Diet, and Diseases. Microorganisms 7 (1), 14. doi: 10.3390/microorganisms7010014
Rose, D. R., Yang, H., Serena, G., Sturgeon, C., Ma, B., Careaga, M., et al. (2018). Differential Immune Responses and Microbiota Profiles in Children With Autism Spectrum Disorders and Co-Morbid Gastrointestinal Symptoms. Brain behavior Immun. 70, 354–368. doi: 10.1016/j.bbi.2018.03.025
Sanders, S. J., Ercan-Sencicek, A. G., Hus, v.e., Luo, R., Murtha, M. T., et al. (2011). Multiple Recurrent De Novo CNVs, Including Duplications of the 7q11.23 Williams Syndrome Region, are Strongly Associated With Autism. Neuron 70 (5), 863–885. doi: 10.1016/j.neuron.2011.05.002
Sauer, A. K., Bockmann, J., Steinestel, K., Boeckers, T. M., Grabrucker, A. M. (2019). Altered Intestinal Morphology and Microbiota Composition in the Autism Spectrum Disorders Associated SHANK3 Mouse Model. Int. J. Mol. Sci. 20 (9), 2134. doi: 10.3390/ijms20092134
Scattoni, M. L., Gandhy, S. U., Ricceri, L., Crawley, J. N. (2008). Unusual Repertoire of Vocalizations in the BTBR T+tf/J Mouse Model of Autism. PLoS One 3 (8), e3067. doi: 10.1371/journal.pone.0003067
Sekirov, I., Russell, S. L., Antunes, L. C., Finlay, B. B. (2010). Gut Microbiota in Health and Disease. Physiol. Rev. 90 (3), 859–904. doi: 10.1152/physrev.00045.2009
Septyaningtrias, D. E., Lin, C.-W., Ouchida, R., Nakai, N., Suda, W., Hattori, M., et al. (2020). Altered Microbiota Composition Reflects Enhanced Communication in 15q11-13 CNV Mice. Neurosci. Res. 161, 59–67. doi: 10.1016/j.neures.2019.12.010
Sgritta, M., Dooling, S. W., Buffington, S. A., Momin, E. N., Francis, M. B., Britton, R. A., et al. (2019). Mechanisms Underlying Microbial-Mediated Changes in Social Behavior in Mouse Models of Autism Spectrum Disorder. Neuron 101 (2), 246–259.e246. doi: 10.1016/j.neuron.2018.11.018
Sharon, G., Cruz, N. J., Kang, D.-W., Gandal, M. J., Wang, B., Kim, Y.-M., et al. (2019). Human Gut Microbiota From Autism Spectrum Disorder Promote Behavioral Symptoms in Mice. Cell 177 (6), 1600–1618.e1617. doi: 10.1016/j.cell.2019.05.004
Song, Y., Liu, C., Finegold, S. M. (2004). Real-Time PCR Quantitation of Clostridia in Feces of Autistic Children. Appl. Environ. Microbiol. 70 (11), 6459–6465. doi: 10.1128/AEM.70.11.6459-6465.2004
Son, J. S., Zheng, L. J., Rowehl, L. M., Tian, X., Zhang, Y., Zhu, W., et al. (2015). Comparison of Fecal Microbiota in Children With Autism Spectrum Disorders and Neurotypical Siblings in the Simons Simplex Collection. PLoS One 10 (10), e0137725. doi: 10.1371/journal.pone.0137725
Strandwitz, P., Kim, K. H., Terekhova, D., Liu, J. K., Sharma, A., Levering, J., et al. (2019). GABA-Modulating Bacteria of the Human Gut Microbiota. Nat. Microbiol. 4 (3), 396–403. doi: 10.1038/s41564-018-0307-3
Strati, F., Cavalieri, D., Albanese, D., De Felice, C., Donati, C., Hayek, J., et al. (2017). New Evidences on the Altered Gut Microbiota in Autism Spectrum Disorders. Microbiome 5 (1), 24. doi: 10.1186/s40168-017-0242-1
Tabuchi, K., Blundell, J., Etherton, M. R., Hammer, R. E., Liu, X., Powell, C. M., et al. (2007). A Neuroligin-3 Mutation Implicated in Autism Increases Inhibitory Synaptic Transmission in Mice. science 318 (5847), 71–76. doi: 10.1126/science.1146221
Thursby, E., Juge, N. (2017). Introduction to the Human Gut Microbiota. Biochem. J. 474 (11), 1823–1836. doi: 10.1042/bcj20160510
Valicenti-McDermott, M., McVicar, K., Rapin, I., Wershil, B. K., Cohen, H., Shinnar, S. (2006). Frequency of Gastrointestinal Symptoms in Children With Autistic Spectrum Disorders and Association With Family History of Autoimmune Disease. J. Dev. Behav. Pediatr. 27 (2 Suppl), S128–S136. doi: 10.1097/00004703-200604002-00011
Vuong, H. E., Hsiao, E. Y. (2017). Emerging Roles for the Gut Microbiome in Autism Spectrum Disorder. Biol. Psychiatry 81 (5), 411–423. doi: 10.1016/j.biopsych.2016.08.024
Wang, L., Christophersen, C. T., Sorich, M. J., Gerber, J. P., Angley, M. T., Conlon, M. A. (2011). Low Relative Abundances of the Mucolytic Bacterium Akkermansia Muciniphila and Bifidobacterium Spp. In Feces of Children With Autism. Appl. Environ. Microbiol. 77 (18), 6718–6721. doi: 10.1128/AEM.05212-11
Wang, Y., Li, N., Yang, J.-J., Zhao, D.-M., Chen, B., Zhang, G.-Q., et al. (2020). Probiotics and Fructo-Oligosaccharide Intervention Modulate the Microbiota-Gut Brain Axis to Improve Autism Spectrum Reducing Also the Hyper-Serotonergic State and the Dopamine Metabolism Disorder. Pharmacol. Res. 157, 104784. doi: 10.1016/j.phrs.2020.104784
Xu, J., Du, Y.-L., Xu, J.-W., Hu, X.-G., Gu, L.-F., Li, X.-M., et al. (2019a). Neuroligin 3 Regulates Dendritic Outgrowth by Modulating Akt/mTOR Signaling. Front. Cell. Neurosci. 518. doi: 10.3389/fncel.2019.00518
Xu, M., Xu, X., Li, J., Li, F. (2019b). Association Between Gut Microbiota and Autism Spectrum Disorder: A Systematic Review and Meta-Analysis. Front. Psychiatry 10 (473). doi: 10.3389/fpsyt.2019.00473
Zou, R., Xu, F., Wang, Y., Duan, M., Guo, M., Zhang, Q., et al. (2020). Changes in the Gut Microbiota of Children With Autism Spectrum Disorder. Autism Res. Off. J. Int. Soc. Autism Res. 13 (9), 1614–1625. doi: 10.1002/aur.2358
Keywords: autism, mouse model, gastrointestinal tract, microbiota, genetics, environmental
Citation: Alamoudi MU, Hosie S, Shindler AE, Wood JL, Franks AE and Hill-Yardin EL (2022) Comparing the Gut Microbiome in Autism and Preclinical Models: A Systematic Review. Front. Cell. Infect. Microbiol. 12:905841. doi: 10.3389/fcimb.2022.905841
Received: 28 March 2022; Accepted: 02 June 2022;
Published: 01 July 2022.
Edited by:
Jaime Garcia-Mena, Instituto Politécnico Nacional de México (CINVESTAV), MexicoReviewed by:
Xiaotang Fan, Third Military Medical Univeristy, ChinaFederica Chiappori, Consiglio Nazionale delle Ricerche, Italy
Copyright © 2022 Alamoudi, Hosie, Shindler, Wood, Franks and Hill-Yardin. This is an open-access article distributed under the terms of the Creative Commons Attribution License (CC BY). The use, distribution or reproduction in other forums is permitted, provided the original author(s) and the copyright owner(s) are credited and that the original publication in this journal is cited, in accordance with accepted academic practice. No use, distribution or reproduction is permitted which does not comply with these terms.
*Correspondence: Elisa L. Hill-Yardin, elisa.hill@rmit.edu.au
†These authors have contributed equally to this work