Toad venom-derived bufadienolides and their therapeutic application in prostate cancers: Current status and future directions
- 1Key Laboratory of Tropical Medicinal Resource Chemistry of Ministry of Education, Key Laboratory of Tropical Medicinal Plant Chemistry of Hainan Province, College of Chemistry and Chemical Engineering, Hainan Normal University, Haikou, Hainan, China
- 2Hainan General Hospital & Hainan Affiliated Hospital of Hainan Medical University, Haikou, Hainan, China
- 3The Fifth People’s Hospital of Hainan Province & Affiliated Dermatology Hospital of Hainan Medical University, Haikou, Hainan, China
Cancer is the second leading cause of death worldwide. Specially, the high incidence rate and prevalence of drug resistance have rendered prostate cancer (PCa) a great threat to men’s health. Novel modalities with different structures or mechanisms are in urgent need to overcome these two challenges. Traditional Chinese medicine toad venom-derived agents (TVAs) have shown to possess versatile bioactivities in treating certain diseases including PCa. In this work, we attempted to have an overview of bufadienolides, the major bioactive components in TVAs, in the treatment of PCa in the past decade, including their derivatives developed by medicinal chemists to antagonize certain drawbacks of bufadienolides such as innate toxic effect to normal cells. Generally, bufadienolides can effectively induce apoptosis and suppress PCa cells in-vitro and in-vivo, majorly mediated by regulating certain microRNAs/long non-coding RNAs, or by modulating key pro-survival and pro-metastasis players in PCa. Importantly, critical obstacles and challenges using TVAs will be discussed and possible solutions and future perspectives will also be presented in this review. Further in-depth studies are clearly needed to decipher the mechanisms, e.g., targets and pathways, toxic effects and fully reveal their application. The information collected in this work may help evoke more effects in developing bufadienolides as therapeutic agents in PCa.
1 Introduction
The quality of life of cancer patients have been improved significantly due to the progress of application of new technologies, including drug development, especially precision medicine. Targeted therapies and the cutting-edge immunotherapies have reached a new paradigm for cancer treatment, which work together with renovated surgery and radiotherapy, etc., to markedly improve treatment outcomes. However, there are still many challenges in treating certain types of cancer including prostate cancer (PCa) which has two unique characteristics. The first one is the high prevalence since it’s one of the leading cancers in men and one of the leading causes of deaths among men worldwide (Gomella, 2017; Schatten, 2018; Zhang et al., 2022a). The second characteristic is the high incidence of drug resistance, since more than 90% of PCa will eventually develop resistance to androgen-depredation therapy (ADT), termed as castration-resistant PCa (CRPC), and later second resistance to subsequent chemotherapies (Armstrong and Gao, 2015; Cohen et al., 2021; Liotti et al., 2021; Morel et al., 2021; Ji et al., 2022; Peery et al., 2022). It’s known that various factors contribute to the development of drug resistance in PCa, such as the alteration/mutation of androgen receptor (AR) or oncogenes, metabolism adaptation, overexpression of ATP-binding cassette (ABC) transporters, apoptosis resistance, enhanced DNA repair and cellular defensive systems against toxic inducers, etc. (Peery et al., 2017; Wang et al., 2020a; de Leeuw et al., 2020; Messina et al., 2020; Peery et al., 2020; Yang et al., 2020; Do and Webster, 2021; Filon et al., 2022). Thus, structurally and mechanistically renovated agents that can effectively suppress PCa and/or less likely develop resistance are in urgent need.
Toad venom, also named as Chan-Su, is a traditional Chinese medicine that has shown therapeutic efficacies in clinic (mainly in China) and has been widely used for the treatment of cancer, cardiovascular diseases, pain, and inflammation/inflammatory diseases as shown in Figure 1 (Gao et al., 2017; Li et al., 2021a; Xu et al., 2021; Zheng et al., 2022). Originally derived from the skin and auricular glands of Chinese toad, toad venom is used to repel toad’s natural enemies primarily, working as a protective agent. Known for the toxic effects to cause cardiac arrhythmia, toad venom-derived agents (TVAs) usually work as an inhibitor of Na+/K+-ATPase and a regulator of calcium homeostasis, which leads to seizure and coma, etc., thereby causing toxic effects (Chen and Kovarikova, 1967; Bick et al., 2002; Lopez-Lopez et al., 2008). In addition to toxic effects, however, toad venom has therapeutic effects that can be applied to treat certain diseases. Till now, due to its strict export ban to other countries by state law, drugs that contain toad venom are only approved for clinical use in China, such as Chansu injection, Liu Shen Wan, Xin Bao Wan, Chan-Su Wan, Hua-Chan Wan (made of isolated cinobufagin in toad venom), Kyushin, Zuo Xiang Bao Xin Wan, etc. (Morishita et al., 1992). In addition to Chinese Chan-Su in the application of cancer treatments, toad venom from other species has also been reported, including Indian toad venom (Gomes et al., 2011), although they have not been fully studied for its application.
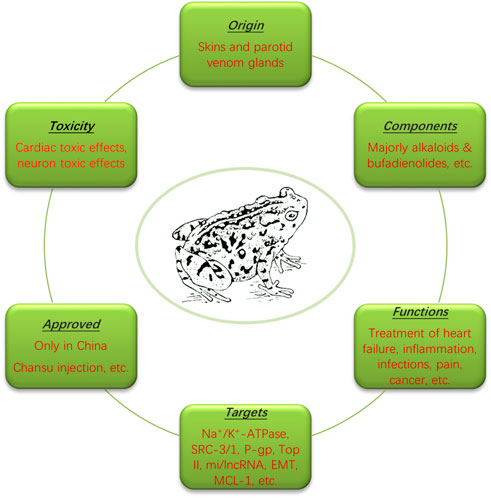
FIGURE 1. The origin, components, functions, toxicity and mechanisms of toad venom. Originated from the skin and auricular glands of Chinese toad, toad venom contains mostly alkaloids and bufadienolides, functioning to treat heart disease, inflammation, infection, pain and cancer through regulating Na+/K+-ATPase, SRC-3 and -1, etc. Drugs containing toad venom are only approved in China.
In this review, we focused on the applications of bufadienolides, especially those isolated pure compounds in TVAs, in treating PCa in the past 10 years. While it is true that not too many studies have been published as of December 2022, and that the research and application of TVAs in cancer treatment are still at its early stage, the information collected could certainly serve as a base for their further exploration in PCa treatment.
2 Bufadienolides in toad venom and their therapeutic implication in cancers
In total, several dozens of different components were identified and characterized in toad venom (Zhang et al., 2005; Wang et al., 2018a; Cao et al., 2019). Their pharmacological effects can be majorly attributed to alkaloids (Dai et al., 2018a; Dong et al., 2022) and bufadienolides which share steroids scaffold in common (Qu et al., 2012). Both alkaloids and bufadienolides are among the most prominent and most-studied compounds in toad venom. Growing studies have confirmed that both alkaloids and bufadienolides can work in treating cardiovascular diseases and cancers (Chen et al., 2020). Our special and major interest in this review falls in these bufadienolides (Figure 2).
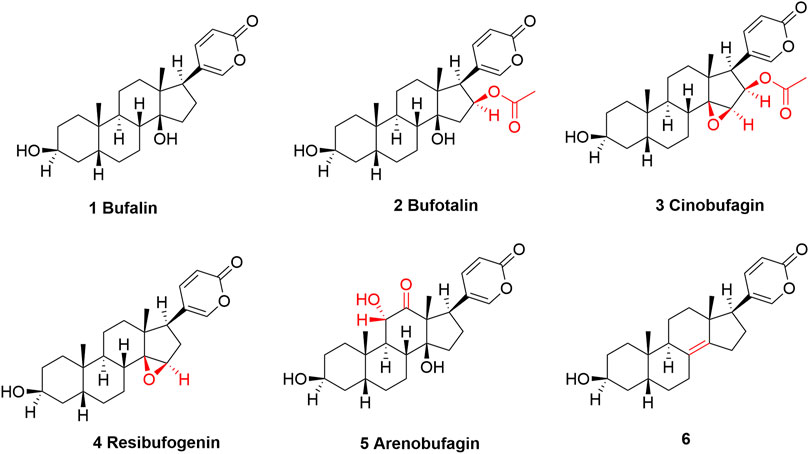
FIGURE 2. The structures of bufalin and its derivatives found in toad venom which show cancer-suppressing effects. Structurally, bufalin can be regarded as a parent compound, and all other TVAs are modified on bufalin at different positions. The structural differences are highlighted in red.
There are several prominent members that are categorized as bufadienolides (Figure 2) (Cunha-Filho et al., 2010; Zhang et al., 2014), including Bufalin, (3β,14-dihydroxy-5β-bufa-20,22-dienolide, shown as compound 1) (Zhang et al., 2020a), bufatalin [(3β,14,16β-Trihydroxy-5β-bufa-20,22-dienolide) 16-acetate, shown as compound 2] (Zhang et al., 2022b), cinobufagin, (3β-Hydroxy-14,15β-epoxy-5β-bufa-20,22-dienolid-16β-yl acetate, shown as 3) (Toma et al., 1987), resibufogenin [(3β,5β,15β)-14,15-epoxy-3-hydroxy-bufa-20,22-dienolide, shown as 4] (Yang et al., 2021a), and arenobufagin [(3β,5β,11α)-3,11,14-Trihydroxy-12-oxobufa-20,22-dienolide, shown as 5] (Zhang et al., 2013). These compounds are structurally related, and specially, all of them can be regarded as bufalin’s derivatives, with minor differences at certain position, which are shown and highlighted in Figure 2. It appears that TVAs have broad-spectrum anticancer potential (Liu et al., 2019; Niu et al., 2021; Jia et al., 2022). Research has indicated that they, either by single use or as a mixture when combined with other agents, are effective in treating acute myeloid leukemia (Hirasaki et al., 2022), lung cancer (Xie et al., 2018; Li et al., 2021b; Meng et al., 2021), colorectal cancer (Li et al., 2019; Bai et al., 2021; Meng et al., 2021), liver cancer (Zhang et al., 2012; Zhao et al., 2019; Zhang et al., 2020b; Yang et al., 2021b), breast cancer (Zhu et al., 2018), oral cancer (Jo et al., 2021), gastric cancer (Xiong et al., 2019), Ehrlich ascites carcinoma (Giri et al., 2018), melanoma (Pan et al., 2019; Zhang et al., 2020c; Kim et al., 2020), nasopharyngeal carcinoma (Pan et al., 2020; Hou et al., 2022), osteosarcoma (Cao et al., 2017; Dai et al., 2018b; Zhang et al., 2019a), cholangiocarcinoma (Ren et al., 2019), myeloma (Baek et al., 2015), etc.
3 Therapeutic application of bufadienolides in PCa
3.1 Mono-therapy of TVAs in PCa
Bufalin is one of the most intensively studied compounds among all TVAs (Wang et al., 2018b; Lan et al., 2019; Soumoy et al., 2022). A study by Zhang et al. (2018) showed that bufalin worked as an anticancer agent via a p53-mediated mechanism in PCa cells both in-vitro and in-vivo. In p53-mutant DU145 cells and p53-wild type LNCaP cells, bufalin (5–100 nM, 48 h) treatment could upregulate the expression of cleaved poly (ADP-ribose) polymerase (PARP), and downregulate steroid receptor co-activator 1/3 (SRC1/3), AR and prostate specific antigen (PSA). This study showed that bufalin increased p53 expression in LNCaP cells, but decreased p53 in DU145 cells, however, cleaved PARP or p53 was not observed in p53-null PC-3 cells although inhibited proliferation was identified, suggesting a p53-mediated efficacy (Zhang et al., 2018). The microarray detection of certain mRNA levels indicated that in LNCaP cells, bufalin treatment increased p53 and its transcriptional target P21CIP1, as well as mRNAs related to cellular stress and DNA damage response, and certain senescence-associated genes, such as CYR61/CCNI, CTGF/CCN2 and CDKN1A, which were then been validated by the subsequent assays of cell cycle distribution (sub G0/1) and the presence of senescence-like phenotype (Zhang et al., 2018). The knockdown of p53 could attenuate bufalin-induced apoptosis as indicated by the decreased level of cleaved PARP. Finally, in the in-vivo model of LNCaP xenograft, bufalin (1.5 mg/kg body weight, IP, daily) for 9 weeks inhibited tumor growth, resulting in a 67% decrease as compared to untreated group, without affecting body weight significantly which might suggest a safe profile. More importantly, in bufalin-treated tumors, phospho-p53 was increased, confirming the on-target effect and a network of bufalin with p53 (Zhang et al., 2018).
A recent study by Zhang et al. (2019) found that bufalin can alter the expression of both microRNAs (miRNAs) and long non-coding RNAs (lncRNAs) that are critical for PCa (Zhang et al., 2019b). In CRPC DU145 and PC-3 cells, bufalin suppressed the cell viability in a dose-dependent manner, with an IC50 value of 0.89 and 1.28 μM, respectively. At lower than the corresponding IC50 (to be more specific, at half of the corresponding IC50), bufalin could significantly reduce the migration and invasion of DU145 and PC-3 cells as confirmed by the wound healing assay and transwell assay. The authors screened lncRNA alteration after bufalin treatment (0.1–5 µM) using a lncRNA microarray, and they identified that HOX transcript antisense RNA (HOTAIR) was one of the mostly reduced (Zhang et al., 2019b). HOTAIR targets and inhibits miR-520b as confirmed by RNA immunoprecipitation assay; meanwhile, miR-520b can negatively regulate the expression of fibroblast growth factor receptor 1 protein (FGFR1) which plays a pivotal role in PCa progression and metastasis (Yang et al., 2013; Wang et al., 2019). The authors also investigated and confirmed the positive correlation of HOTAIR and FGFR1 with PCa bone metastasis, and that the overexpression of HOTAIR could reverse bufalin-induced cancer-suppressing effects. Thus, this study indicated that bufalin can inhibit PCa proliferation, migration and invasion via regulating the HOTAIR-miR520b-FGFR1 loop (Zhang et al., 2019b).
MiRNA-181, composed with subunits miRNA-181a and b, targets apoptosis-associated proteins such as Bcl-2 family members, functioning as a tumor suppressor (Liu et al., 2017; Pei et al., 2020). Zhai et al. (2013) found that in bufalin (10 μM, 24 h)-treated PC-3 cells, miRNA-181a, but not the others such as miRNA-10b, −17, 18a, 20a, 21, −106, −155, −221 and −372, was markedly upregulated (5-fold), which was later confirmed to be a dose-dependent manner (1, 10 and 15 µM) (Zhai et al., 2013). In PC-3 cells, bufalin (15 µM) significantly decreased the expression of Bcl-2, an anti-apoptotic protein, accompanied with caspase-3 protein activation (via testing the level of cleaved caspase-3), which is essential in promoting apoptosis (Kesavardhana et al., 2020). At the same time, the rescue experiments showed that bufalin-induced apoptosis and caspase-3 proteins activation can be partially reversed by miR-181a inhibitor co-treatment (100 nM), validating the targeted effects of bufalin toward miR-181a (Zhai et al., 2013).
Structurally, bufalin is a hydrophobic compound that may encounter poor absorption and bioavailability (Shao et al., 2021). Thus, Liu and Huang (2016) constructed an amphiphilic targeting brush-type copolymers that can deliver bufalin to CRPC cells, which exhibited controlled drug release and higher anticancer capability than free bufalin both in-vitro and in-vivo (Liu and Huang, 2016). This constructed BUF-loaded micellar nanoparticle BUF-NP-(G3-C12) was found to have an IC50 value of 8.0 ng/mL, which was lower than that of free bufalin (which was 13.3 ng/mL) in CRPC DU145 cells; and consistent results were also observed in inducing apoptosis. In DU145 xenograft model, when used by intravenous injection iv) at an equivalent 1.0 mg bufalin/kg, BUF-NP-(G3-C12) showed significantly higher tumor-inhibiting effects than that of free bufalin. Importantly, it didn’t change body weight as compared to vehicle control, suggesting its safety (Liu and Huang, 2016). Further evaluation is clearly needed to develop it as a drug candidate for PCa.
Chen et al. (2017) reported that arenobufagin, among five bufadienolides including cinobufotalin, bufarenogin, 19-oxocinobufotalin and 19-hydroxybufalin, showed the highest potency in suppressing the progress of epithelial-mesenchymal transition (EMT) in PC-3 cells, leading to decreased ability of migration and invasion (Chen et al., 2017). Arenobufagin (8 nM) time-dependently (24, 36 and 48 h) downregulated EMT markers in PC-3 cells, including slug, zinc finger E-box binding homeobox 1 (ZEB1), snail, N-cadherin, vimentin and Twist1 as confirmed by the Western bolt experiment. In addition, β-catenin was reduced at both mRNA and expression levels by arenobufagin, which then lead to the downregulation of its downstream genes including Met, LEF, TCF, c-Myc and cyclin D1. These effects can be reversed by β-catenin overexpression, suggesting the network of arenobufagin with β-catenin. Arenobufagin (1 mg/kg) reduced tumor growth without altering the body weight or causing harms to major organs including heart, liver, spleen, lung and kidney. In the in-vivo PC-3 cells pulmonary metastases model, arenobufagin markedly reduced the number and size of tumor metastatic foci in lung tissues, suggesting its dual role in preventing tumor growth and metastasis, warranting further study (Chen et al., 2017).
Niu et al. (2018) reported the anticancer effects and the mode of action of another TVA, cinobufagin, in CRPC PC-3 cells. Cinobufagin could significantly suppress PC-3 cells proliferation, with an approximately IC50 of 100 nM (24 h) or 50 nM (48 h), suggesting a dose- and time-dependent manner. When tested in colony formation, cinobufagin possessed a much lower IC50 (slightly lower than 5 nM). Mechanistically, cinobufagin induced apoptosis of PC-3 cells via down-regulating anti-apoptotic MCL-1 protein (Niu and Qin, 2018). Cinobufagin appears to be much more potent than bufalin, which has an IC50 of 1.28 μM in PC-3 cells.
3.2 Combinational therapy of TVAs in PCa
In addition to its role in working alone to suppress PCa, TVA bufalin has also been found to work as a chemo-sensitizer when combined with other conventional therapeutics.
Bufalin was identified as a possible DNA topoisomerase II (Top II) inhibitor (Hashimoto et al., 1997; Pastor and Cortes, 2003). Previous in-vitro studies showed that sequential administration of different Top isomer inhibitors exhibited improved outcomes as compared to simultaneous administration, suggesting a feasible combinational strategy (Cho and Cho-Chung, 2003; Griffith and Kemp, 2003). Recently, Gu and Zhang (2021) investigated the combination of low-dose (0.4–0.8 mg/kg) bufalin with hydroxycamptothecin, a Top I inhibitor (Gu and Zhang, 2021). In this study, CRPC DU145 cells xenograft model in nude mice were constructed and treated by hydroxycamptothecin (2 mg/kg) combined with 0.4 mg/kg, 0.6 mg/kg or 0.8 mg/kg bufalin, respectively. The results showed that among all treatments, the combination of hydroxycamptothecin with 0.6 mg/kg bufalin showed the strongest tumor-reducing effect (93% inhibition) than the other two combinations or monotherapy, bufalin at 1 mg/kg (∼30% inhibition) or hydroxycamptothecin at 2 mg/kg (58% inhibition), without altering body weight significantly (Gu and Zhang, 2021). This combination, named as H6B, induced significantly higher apoptosis but reduced proliferating cell nuclear antigen (PCNA) proteins in the tumors than the other treatments as confirmed by the TUNEL assay and immunohistochemistry, respectively. Western blot assay showed that H6B increased pro-apoptotic proteins such as Bax, p53 and programmed cell death protein 4 (PDCD4); whereas it decreased anti-apoptotic proteins such as Bcl-XL and p-AKT (Gu and Zhang, 2021). While this study presented a possible combinational treatment that was safe and can be further validated in other models and even in humans, it remains unclear if H6B inhibit Top I/II in the treated tumor tissue.
4 Other therapeutic implication of TVAs in PCa
Growing evidence has suggested that TVAs may have other therapeutic application in treating PCa.
Firstly, both cinobufagin and bufalin could inhibit P-glycoprotein (P-gp, also named as ABCB1 or multidrug resistance mutation 1, MDR1) (Yuan et al., 2017; Madugula and Neerati, 2020; Zhan et al., 2020; Neerati and Munigadapa, 2022). Since P-gp plays an essential role, and sometime the leading role in inducing anticancer drug of both conventional and targeted therapies resistance via transporting them out of cancer cells (Robey et al., 2018; Wang et al., 2020b; Feng et al., 2020; Thomas and Tampe, 2020; Wang et al., 2021), cinobufagin and bufalin may likely have potentials in sensitizing certain conventional chemotherapeutics that are substrates of P-gp. In addition, as all of these bufadienolides possess the same pharmacophore which indicates that they may have similar bioactivities, it is reasonable to predict that other TVAs (in addition to cinobufagin and bufalin) may also impact P-gp and may have synergistic/sensitizing effects in PCa treatment when used by combination (Wu et al., 2020a; Gao et al., 2020; Chen et al., 2021), warranting further exploration. Furthermore, it’s also worth studying whether TVAs impact other members of ABC transporters. Thus, a broader screening and validation is necessary to explore their full potential.
Secondly, TVAs can induce cytochrome P450 3A in the pharmacokinetic (PK) study (Jiang et al., 2012; Dai et al., 2019), suggesting that they may affect other drugs metabolism and requiring a real-time monitor of PK profiles when used by combination.
Thirdly, since TVAs could alleviate cancer-related pain, it is meaningful in trying optimal combinational strategies with certain anticancer drugs (Xu et al., 2019).
Furthermore, there are several bufalin-derived TVAs that show better inhibitory effect in PCa cells than bufalin, including compound 6 (Figure 2), a de-hydroxyl bufalin, showed higher AR binding affinity but lower inhibition on the Na+/K+-ATPase, which may suggest a higher cytotoxic effect to PCa cells but lower toxic effect to heart, warranting further evaluation (Tian et al., 2014).
While several TVAs derivatives also exhibited promising anticancer effects in PCa and other cancer types (Yuan et al., 2014; Meng et al., 2021; Sampath et al., 2022), their application remains to be fully exploited. It’s also noteworthy that except for bufalin, arenobufagin, and cinobufagin, very few studies of bufotalin and resibufogenin in PCa have been reported in the past decade.
5 Toxic and potential adverse effects of TVAs
One of the major challenges in using TVAs is the toxic effect, which may significantly cripple their application potential in PCa. Thus, the toxic effects and the associated mechanism are discussed briefly.
5.1 Cardiac toxic effects via regulating Na+/K+-ATPase
Several TVAs have been confirmed to induce cardiac toxicity. Resibufogenin (0.2 mg/kg, iv) could significantly increase heart burden as indicated by contractile force in rabbit, cat and dog, leading to delayed afterdepolarization and triggered arrhythmias (Xie et al., 2001). These effects were partially mediated by its disturbance of Na+/K+-ATPase which caused calcium (Ca+) overload (Xie et al., 2001). Similarly, in human cardiomyocytes model, bufalin (30–300 nM) showed a biphasic effect on the contractility, which was strengthening contractility, accelerating conduction, and increasing beating rate at the earlier stage, while in the opposite when at the later stage (Li et al., 2020).
5.2 Neuron toxicity due to inhibit voltage-gated potassium channels
TVAs are known to cause neuron toxicity as reported previously (Brubacher et al., 1999; Dasgupta, 2003; Ma et al., 2007). In addition to the inhibition of Na+/K+-ATPase, in rat hippocampal neurons (Wang et al., 2014a), both resibufogenin and cinobufagin could also inhibit outward delayed rectifier potassium current (Hao et al., 2011), which may work together to induce toxicity in neuron system. However, we believe that more studies are needed to reveal the doses or concentrations on inducing human neuron cells related toxicity.
5.3 Drug-drug interactions due to the inhibition of human cytochrome P450 3A4 (CYP3A4)
A study by Li et al. (2009) found that bufalin had an inhibitory toward recombinant human CYP3A4 in vitro, with an IC50 of 14.52 μM, leading to increased elimination half-time, peak plasma level of midazolam (a substrate of CYP3A4) in the rat model. Thus, when being used with combination, adverse effects due to CYP3A4 inhibition of TVAs should be monitored and prevented.
5.4 The narrow therapeutic window
It’s known that in mouse model the median lethal dose (LD50) of bufalin in nude mice is 2.2 mg/kg (Tu et al., 2000), which is pretty close to the doses of achieving therapeutic effects of tumor inhibition (normally not more than 1 mg/kg), suggesting a very narrow therapeutic window, and that the accumulative TVAs may further worsen certain toxic effects. Thus, when being tested in humans, a close monitor of serum concentration is necessary.
6 Discussion and future perspectives
Cancers have become a great burden to modern people due to high prevalence and high cost in treatment and care (Desai et al., 2021; Wells, 2021). Cancer-related deaths rank the second among all deaths caused by different diseases (Siegel et al., 2022). As our major research interest, PCa stands out for three reasons, the most diagnosed cancer in men, the second most cancer deaths in men globally, and extremely high rate of drug resistance (Wade and Kyprianou, 2018). Currently, effective therapeutic strategies for PCa include surgery, cytotoxic chemotherapy agents, AR inhibitors, PARP inhibitors, and radiopharmaceuticals, etc. (Do and Webster, 2021). Unfortunately, the vast majority of PCa patients will develop acquired resistance to most of these therapeutic agents (Moreira-Silva et al., 2022).
Toad venom is a traditional Chinese medicine that has been applied (mostly used by certain extraction/mixture in combination with other drugs) in clinic for hundreds of years in China (Li et al., 2021a). It should be mentioned that all the approved drugs contain the extraction of toad venom but not the isolated active components such as these discussed bufadienolides in this review. For example, while Chansu injection, an approved drug in China for infective diseases, has been evaluated for its potential in cancer treatment, its effectiveness and safety among cancer patients are yet to be proved (Jia et al., 2022). A clinical study, published in 2016 in stage III-IV patients of non-Hodgkin lymphoma, showed that the combination of Chansu injection with EOAP (etoposide, vincristine, cytosine arabinoside and prednisone) failed to improve the therapeutic effect when compared to EOAP group (Niu et al., 2016). Another clinical evaluation showed that while Chansu injection might enhance the treatment effects of certain anticancer agents (Ma et al., 2018), we believe that further broader clinical trials are still needed to validate the efficacy.
It’s assumed that bufadienolides have several advantages over those approved drugs, because 1) bufadienolides are new therapeutic agents with distinct structures, and laboratory studies have suggested that PCa cells are sensitive to them. Thus, it’s likely that PCa cells may not be able to quickly develop resistance. 2) The above mentioned approved drugs are all single-targeted agents, which can be antagonized by adaptation of PCa cells. Bufadienolides are known for multi-targeted compounds, rendering them hard to develop resistance by PCa cells. 3) Reports have shown that P-gp can induce resistance of some PCa drugs including docetaxel (Kato et al., 2015), PARP inhibitor talazoparib (Naito et al., 2021; Teyssonneau et al., 2022), etc. As P-gp is one of bufadienolides’ targets, thus, to reverse or achieve sensitizing effects, it’s reasonable to use combinational regimens, including combination composed with approved drugs in PCa. However, cautions remain since 1) the efficacy and safety of pure isolated bufadienolides in human is unknown; 2) bufadienolides may have intensive drug-drug interactions as they have interactions with cytochrome P450 3A (Jiang et al., 2012; Dai et al., 2019); and 3) it’s unclear of the exact targets.
Though these components have been extensively studied in the past decade, none of them have been approved. The application of TVAs in PCa is still at early stage but is attracting more attentions recently.
6.1 Summary of TVAs in PCa
The above literature review has summarized the application of TVAs in PCa (Table 1; Figure 3). Generally, TVAs could suppress PCa cells proliferation via inducing apoptosis and regulating certain miRNAs and lncRNAs; meanwhile, they also show activity in reducing PCa cells migration and invasion in-vitro and in-vivo through negatively regulating critical players involved in metastasis. It’s known that bufalin targets steroid receptor coactivators SRC-3 and SRC-1 (Wang et al., 2014b), while growing evidence suggests that bufalin is a multi-targeting or multi-functional agent, especially in the treatment of cancers.
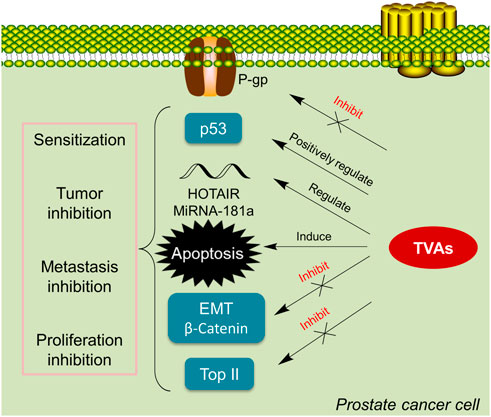
FIGURE 3. TVAs suppress cancer growth via various pathways. TVAs appear to be able to suppress P-gp, activate p53, and regulate critical players in EMT, inhibit Top II and modulate certain mi/lncRNA, leading to PCa cells apoptosis which thereby suppressing cancer progression.
By far, except for bufalin that has been extensively studied, the therapeutic applications of other TVAs in PCa are yet to be fully revealed. While we suspect that since they all share a very similar scaffold, there may be limited differences of the underlying mechanisms, requiring further validations.
It also comes to our notice that TVAs have been approved and/or under active clinical evaluations only in China. Currently, combinational therapies of using some of TVAs are also actively tested in clinical trials, such as the combination of thalidomide with cinobufagin to treat lung cancer cachexia (Xie et al., 2018). Other TVAs-related clinical trial was either conducted more than 10 years ago (Meng et al., 2009), or using formulations made of toad venom extraction rather than isolated single component (Meng et al., 2012; Wu et al., 2020b; Tan et al., 2021). Since both the active components (major and minor) and the associated mechanisms remain largely elusive, thus, these formulations will likely meet many obstacles to be approved in other countries outside China due to different new drugs regulations. More studies using corresponding isolated pure compounds are in urgent need to support their further evaluation in humans.
6.2 Future perspectives
While the anticancer of TVAs in PCa can be confirmed in lab (in-vitro and in-vivo), much more works are needed before they can be eventually applied in patients worldwide. The authors propose that six future directions are worth trying.
(1) Rational design of TVAs derivatives or analogs via the assistance of computer-aided drug design (CADD). These structures of bufadienolides can serve as leading compounds that may undergo structural modification for improved target-binding and anticancer effects. By far, this research area is extremely undeveloped. Very few studies have been published, and most of them are focusing on the modification of hydroxyl groups at different positions (Sampath et al., 2022). Further and varied structural modification at other positions and functional groups are necessary.
(2) In-depth pharmacological/mechanistic study for target(s) identification and verification. While bufadienolides appear to regulating multifaceted signal pathways and targets in PCa, it remains elusive regarding the decisive factor. Proteomics study and gene sequence after TVAs treatment along with the associated pharmacological and validation studies is required.
(3) Following the pharmacological/mechanistic study, toxicological mechanisms, beside their inhibition on Na+/K+-ATPase or other ion channels, are needed. In addition, it is also possible that certain metabolites of TVAs may contribute to toxic effects, requiring further validation.
(4) PK study. The PK study can answer the time-course of the absorption, distribution, metabolism and elimination, as well as toxicity of bufadienolides, which may offer solutions for the doses and frequency of administration in PCa patients. Unfortunately, there is no PK data using isolated TVAs in humans. Recently in 2019, a PK study using bufalin in rats were published (Wei et al., 2019). It is shown that bufalin (10 mg/kg, oral administration) reached the peak serum concentration (14.722 ± 4.681 ng/mL) after only 15 min, which had a half time of 5.7 ± 3.06 h (Wei et al., 2019). Bufalin could quickly undergo metabolism into more than nine different metabolites. This study provided very useful information of using bufalin in rats, which may help to design and develop protocols in monitoring metabolism of TVAs in humans. In addition, these identified metabolites may help to reveal potential pharmacological effects as well as toxic effects in humans.
(5) More in-vivo models validation of bufadienolides in PCa. In addition to in-vitro models, in-vivo models including patient-derived xenograft models are warranted. Furthermore, due to its innate toxic effects, the combinational regimens of low-dose bufadienolides with certain conventional chemotherapeutics will be promising.
(6) Deciphering associated resistance reasons and developing combinational strategy. Drug resistance is a major obstacle in PCa treatment (Do and Webster, 2021; Zhao et al., 2021). While we suspect that PCa cells may not develop resistance to bufadienolides easily, it’s largely unknown when and how, as well as the resistance rate and resistant mechanisms. For the full application and indications, more studies are needed to reveal resistant reasons.
Finally, more clinical trials in PCa are necessary to test the efficacies of TVAs including their pharmaceutical formulations.
7 Conclusion
PCa, due to its high incidence rate and prevalence of drug resistance, is one of the leading threats to men’s health. Chinese traditional medicine toad venom and TVAs have emerged as promising therapeutic agents in PCa, which have been validated by cell- and animal-based models. Further in-depth studies are also clearly needed for the underlying mechanisms, toxicology, and for exploring combinational therapies in PCa.
Author contributions
QY designed and wrote the draft; XZ, FH, and CZ revised this review. QY and CZ finalized the manuscript. All authors read and approved the final manuscript.
Funding
We are grateful to the support from the National Natural Science Foundation of China (Grant number: 82160735), Hainan Normal University 2021 Annual Graduate Innovation and Scientific Research Project (Grant number: hsyx 2021-4) and Hainan Province Clinical Medical Center.
Conflict of interest
The authors declare that the research was conducted in the absence of any commercial or financial relationships that could be construed as a potential conflict of interest.
Publisher’s note
All claims expressed in this article are solely those of the authors and do not necessarily represent those of their affiliated organizations, or those of the publisher, the editors and the reviewers. Any product that may be evaluated in this article, or claim that may be made by its manufacturer, is not guaranteed or endorsed by the publisher.
References
Armstrong, C. M., and Gao, A. C. (2015). Drug resistance in castration resistant prostate cancer: Resistance mechanisms and emerging treatment strategies. Am. J. Clin. Exp. Urol. 3, 64–76.
Baek, S. H., Kim, C., Lee, J. H., Nam, D., Lee, J., Lee, S. G., et al. (2015). Cinobufagin exerts anti-proliferative and pro-apoptotic effects through the modulation ROS-mediated MAPKs signaling pathway. Immunopharm Immunot 37, 265–273. doi:10.3109/08923973.2015.1027916
Bai, Y., Wang, X., Cai, M., Ma, C., Xiang, Y., Hu, W., et al. (2021). Cinobufagin suppresses colorectal cancer growth via STAT3 pathway inhibition. Am. J. Cancer Res. 11, 200–214.
Bick, R. J., Poindexter, B. J., Sweney, R. R., and Dasgupta, A. (2002). Effects of chan su, a traditional Chinese medicine, on the calcium transients of isolated cardiomyocytes: Cardiotoxicity due to more than Na, K-ATPase blocking. Life Sci. 72, 699–709. doi:10.1016/s0024-3205(02)02302-0
Brubacher, J. R., Lachmanen, D., Ravikumar, P. R., and Hoffman, R. S. (1999). Efficacy of digoxin specific Fab fragments (Digibind®) in the treatment of toad venom poisoning. Toxicon 37, 931–942. doi:10.1016/s0041-0101(98)00224-4
Cao, Y., Wu, J., Pan, H., and Wang, L. (2019). Chemical profile and multicomponent quantitative analysis for the quality evaluation of toad venom from different origins. Molecules 24, 3595. doi:10.3390/molecules24193595
Cao, Y., Yu, L., Dai, G., Zhang, S., Zhang, Z., Gao, T., et al. (2017). Cinobufagin induces apoptosis of osteosarcoma cells through inactivation of Notch signaling. Eur. J. Pharmacol. 794, 77–84. doi:10.1016/j.ejphar.2016.11.016
Chen, K. K., and Kovarikova, A. (1967). Pharmacology and toxicology of toad venom. J. Pharm. Sci-Us 56, 1535–1541. doi:10.1002/jps.2600561202
Chen, L., Mai, W., Chen, M., Hu, J., Zhuo, Z., Lei, X., et al. (2017). Arenobufagin inhibits prostate cancer epithelial-mesenchymal transition and metastasis by down-regulating beta-catenin. Pharmacol. Res. 123, 130–142. doi:10.1016/j.phrs.2017.07.009
Chen, X. Y., Wang, J. Q., Yang, Y., Li, J., and Chen, Z. S. (2021). Natural product as substrates of ABC transporters: A review. Recent Pat. Anti-Canc 16, 222–238. doi:10.2174/1574892816666210218220943
Chen, Y. L., Dai, Y. H., Wang, A. D., Zhou, Z. Y., Lei, M., Liu, J., et al. (2020). Two new indole alkaloids from toad venom of Bufo bufo gargarizans. Molecules 25, 4511. doi:10.3390/molecules25194511
Cho, Y. S., and Cho-Chung, Y. S. (2003). Antisense protein kinase A RIalpha acts synergistically with hydroxycamptothecin to inhibit growth and induce apoptosis in human cancer cells: Molecular basis for combinatorial therapy. Clin. Cancer Res. 9, 1171–1178.
Cohen, L., Livney, Y. D., and Assaraf, Y. G. (2021). Targeted nanomedicine modalities for prostate cancer treatment. Drug Resist Update 56, 100762. doi:10.1016/j.drup.2021.100762
Cunha-Filho, G. A., Resck, I. S., Cavalcanti, B. C., Pessoa, C. O., Moraes, M. O., Ferreira, J. R., et al. (2010). Cytotoxic profile of natural and some modified bufadienolides from toad Rhinella schneideri parotoid gland secretion. Toxicon 56, 339–348. doi:10.1016/j.toxicon.2010.03.021
Dai, G., Zheng, D., Guo, W., Yang, J., and Cheng, A. Y. (2018). Cinobufagin induces apoptosis in osteosarcoma cells via the mitochondria-mediated apoptotic pathway. Cell Physiol. Biochem. 46, 1134–1147. doi:10.1159/000488842
Dai, Y. H., Wang, A. D., Chen, Y. L., Xia, M. Y., Shao, X. Y., Liu, D. C., et al. (2018). A new indole alkaloid from the traditional Chinese medicine Chansu. J. Asian Nat. Prod. Res. 20, 581–585. doi:10.1080/10286020.2017.1339697
Dai, Z. R., Ning, J., Sun, G. B., Wang, P., Zhang, F., Ma, H. Y., et al. (2019). Cytochrome P450 3A enzymes are key contributors for hepatic metabolism of bufotalin, a natural constitute in Chinese medicine Chansu. Front. Pharmacol. 10, 52. doi:10.3389/fphar.2019.00052
Dasgupta, A. (2003). Review of abnormal laboratory test results and toxic effects due to use of herbal medicines. Am. J. Clin. Pathol. 120, 127–137. doi:10.1309/p024k7vrddpjctvn
de Leeuw, R., Cao, Q., and Lam, H. M. (2020). Editorial: Response and resistance in castration-resistant prostate cancer. Front. Oncol. 10, 607298. doi:10.3389/fonc.2020.607298
Desai, A., Kuderer, N. M., and Lyman, G. H. (2021). Aligning cancer clinical trials with cancer burden: Need for greater global leadership, resources, and vision. JAMA Oncol. 7, 357–358. doi:10.1001/jamaoncol.2020.7293
Do, P. C., and Webster, R. M. (2021). The prostate cancer drug market. Nat. Rev. Drug Discov. 20, 663–664. doi:10.1038/d41573-021-00111-w
Dong, Q., Liu, L., Yuan, Y., Turdu, G., Mirzaakhmedov, S., Aisa, H. A., et al. (2022). Two new polyamine alkaloids from the Bufo viridis toad venom. Nat. Prod. Res. 2022, 1–5. doi:10.1080/14786419.2022.2086545
Feng, W., Zhang, M., Wu, Z. X., Wang, J. Q., Dong, X. D., Yang, Y., et al. (2020). Erdafitinib antagonizes ABCB1-mediated multidrug resistance in cancer cells. Front. Oncol. 10, 955. doi:10.3389/fonc.2020.00955
Filon, M. J., Gillette, A. A., Yang, B., Khemees, T. A., Skala, M. C., and Jarrard, D. F. (2022). Prostate cancer cells demonstrate unique metabolism and substrate adaptability acutely after androgen deprivation therapy. Prostate 82, 1547–1557. doi:10.1002/pros.24428
Gao, F., Wang, X., Li, Z., Zhou, A., Tiffany-Castiglioni, E., Xie, L., et al. (2017). Identification of anti-tumor components from toad venom. Oncol. Lett. 14, 15–22. doi:10.3892/ol.2017.6160
Gao, H. L., Gupta, P., Cui, Q., Ashar, Y. V., Wu, Z. X., Zeng, L., et al. (2020). Sapitinib reverses anticancer drug resistance in colon cancer cells overexpressing the ABCB1 transporter. Front. Oncol. 10, 574861. doi:10.3389/fonc.2020.574861
Giri, B., Dey, S., and Gomes, A. (2018). Indian toad (Bufo melanostictus, Schneider) skin extract induces apoptosis and shows cytotoxic effect on Ehrlich ascites carcinoma (EAC) cells. J. Drug Deliv. Ther. 8, 303–312. doi:10.22270/jddt.v8i5.1873
Gomella, L. G. (2017). Prostate cancer statistics: Anything you want them to Be. Can. J. Urol. 24, 8603–8604.
Gomes, A., Giri, B., Alam, A., Mukherjee, S., Bhattacharjee, P., and Gomes, A. (2011). Anticancer activity of a low immunogenic protein toxin (BMP1) from Indian toad (Bufo melanostictus, Schneider) skin extract. Toxicon 58, 85–92. doi:10.1016/j.toxicon.2011.05.008
Griffith, T. S., and Kemp, T. J. (2003). The topoisomerase I inhibitor topotecan increases the sensitivity of prostate tumor cells to TRAIL/Apo-2L-induced apoptosis. Cancer Chemoth Pharm. 52, 175–184. doi:10.1007/s00280-003-0656-2
Gu, R., and Zhang, Q. (2021). Effects of low-dose bufalin combined with hydroxycamptothecin on human castration-resistant prostate cancer xenografts in nude mice. Exp. Ther. Med. 22, 1015. doi:10.3892/etm.2021.10447
Hao, S., Bao, Y. M., An, L. J., Cheng, W., Zhao, R. G., Bi, J., et al. (2011). Effects of Resibufogenin and Cinobufagin on voltage-gated potassium channels in primary cultures of rat hippocampal neurons. Toxicol Vitro 25, 1644–1653. doi:10.1016/j.tiv.2011.07.001
Hashimoto, S., Jing, Y., Kawazoe, N., Masuda, Y., Nakajo, S., Yoshida, T., et al. (1997). Bufalin reduces the level of topoisomerase II in human leukemia cells and affects the cytotoxicity of anticancer drugs. Leuk. Res. 21, 875–883. doi:10.1016/s0145-2126(97)00061-1
Hirasaki, Y., Okabe, A., Fukuyo, M., Rahmutulla, B., Mano, Y., Seki, M., et al. (2022). Cinobufagin inhibits proliferation of acute myeloid leukaemia cells by repressing c-Myc pathway-associated genes. Chem-Biol Interact. 360, 109936. doi:10.1016/j.cbi.2022.109936
Hou, R., Liu, X., Yang, H., Deng, S., Cheng, C., Liu, J., et al. (2022). Chemically synthesized cinobufagin suppresses nasopharyngeal carcinoma metastasis by inducing ENKUR to stabilize p53 expression. Cancer Lett. 531, 57–70. doi:10.1016/j.canlet.2022.01.025
Ji, X., Liu, K., Li, Q., Shen, Q., Han, F., Ye, Q., et al. (2022). A mini-review of flavone isomers apigenin and genistein in prostate cancer treatment. Front. Pharmacol. 13, 851589. doi:10.3389/fphar.2022.851589
Jia, J., Li, J., Zheng, Q., and Li, D. (2022). A research update on the antitumor effects of active components of Chinese medicine ChanSu. Front. Oncol. 12, 1014637. doi:10.3389/fonc.2022.1014637
Jiang, B., Cai, F., Gao, S., Meng, L., Liang, F., Dai, X., et al. (2012). Induction of cytochrome P450 3A by shexiang baoxin pill and its main components. Chem-Biol Interact. 195, 105–113. doi:10.1016/j.cbi.2011.12.001
Jo, S., Yang, E., Lee, Y., Jeon, D., and Namkung, W. (2021). Cinobufagin exerts anticancer activity in oral squamous cell carcinoma cells through downregulation of ANO1. Int. J. Mol. Sci. 22, 12037. doi:10.3390/ijms222112037
Kato, T., Mizutani, K., Kameyama, K., Kawakami, K., Fujita, Y., Nakane, K., et al. (2015). Serum exosomal P-glycoprotein is a potential marker to diagnose docetaxel resistance and select a taxoid for patients with prostate cancer. Urol. Oncol-Semin Ori 33, 385.e15–385.e20. doi:10.1016/j.urolonc.2015.04.019
Kesavardhana, S., Malireddi, R., and Kanneganti, T. D. (2020). Caspases in cell death, inflammation, and pyroptosis. Annu. Rev. Immunol. 38, 567–595. doi:10.1146/annurev-immunol-073119-095439
Kim, G. H., Fang, X. Q., Lim, W. J., Park, J., Kang, T. B., Kim, J. H., et al. (2020). Cinobufagin suppresses melanoma cell growth by inhibiting LEF1. Int. J. Mol. Sci. 21, 6706. doi:10.3390/ijms21186706
Lan, Y. L., Lou, J. C., Jiang, X. W., Wang, X., Xing, J. S., Li, S., et al. (2019). A research update on the anticancer effects of bufalin and its derivatives. Oncol. Lett. 17, 3635–3640. doi:10.3892/ol.2019.10062
Li, F. J., Hu, J. H., Ren, X., Zhou, C. M., Liu, Q., and Zhang, Y. Q. (2021). Toad venom: A comprehensive review of chemical constituents, anticancer activities, and mechanisms. Arch. Pharm. 354, e2100060. doi:10.1002/ardp.202100060
Li, H. Y., Xu, W., Zhang, X., Zhang, W. D., and Hu, L. W. (2009). Bufalin inhibits CYP3A4 activity in vitro and in vivo. Acta Pharmacol. Sin. 30, 646–652. doi:10.1038/aps.2009.42
Li, J., Zhang, Z., Deng, H., and Zheng, Z. (2021). Cinobufagin-loaded and folic acid-modified polydopamine nanomedicine combined with photothermal therapy for the treatment of lung cancer. Front. Chem. 9, 637754. doi:10.3389/fchem.2021.637754
Li, M., Wang, X. J., Zhao, Q., Wang, J. X., Xing, H. Y., Zhang, Y. Z., et al. (2020). Bufalin-induced cardiotoxicity: New findings into mechanisms. Chin. J. Nat. Med. 18, 550–560. doi:10.1016/s1875-5364(20)30065-0
Li, X., Chen, C., Dai, Y., Huang, C., Han, Q., Jing, L., et al. (2019). Cinobufagin suppresses colorectal cancer angiogenesis by disrupting the endothelial mammalian target of rapamycin/hypoxia-inducible factor 1α axis. Cancer Sci. 110, 1724–1734. doi:10.1111/cas.13988
Liotti, A., La Civita, E., Cennamo, M., Crocetto, F., Ferro, M., Guadagno, E., et al. (2021). Periprostatic adipose tissue promotes prostate cancer resistance to docetaxel by paracrine IGF-1 upregulation of TUBB2B beta-tubulin isoform. Prostate 81, 407–417. doi:10.1002/pros.24117
Liu, H., Zhang, Z., and Li, Q. (2017). DR5 but not miRNA-181 or miRNA-211 is involved in ER stress-mediated apoptosis induced by palmitate in islet beta cells. Int. J. Clin. Exp. Patho 10, 7692–7698.
Liu, J. S., Deng, L. J., Tian, H. Y., Ruan, Z. X., Cao, H. H., Ye, W. C., et al. (2019). Anti-tumor effects and 3D-quantitative structure-activity relationship analysis of bufadienolides from toad venom. Fitoterapia 134, 362–371. doi:10.1016/j.fitote.2019.03.006
Liu, T., and Huang, Q. (2016). Biodegradable brush-type copolymer modified with targeting peptide as a nanoscopic platform for targeting drug delivery to treat castration-resistant prostate cancer. Int. J. Pharm. 511, 1002–1011. doi:10.1016/j.ijpharm.2016.08.017
Lopez-Lopez, J. M., Sanabria, M. R., and de Prada, S. J. (2008). Ocular toxicity caused by toad venom. Cornea 27, 236–237. doi:10.1097/ico.0b013e31815b8317
Ma, F., Zhang, L., and Wang, H. (2018). Clinical evaluation of the combination of Chansu injection with targeted therapy in NSCLC. Dr. 3, 76–77.
Ma, H. Y., Kou, J. P., Wang, J. R., and Yu, B. Y. (2007). Evaluation of the anti-inflammatory and analgesic activities of Liu-Shen-Wan and its individual fractions. J. Ethnopharmacol. 112, 108–114. doi:10.1016/j.jep.2007.02.008
Madugula, N. K., and Neerati, P. (2020). Influence of toad parotid gland secretion from Indian toad (Bufo melanostictus) in diabetic rats: An experimental evidence of P-glycoprotein inhibition. J. Pharm. Res. Int. 32, 125–135. doi:10.9734/jpri/2020/v32i2030736
Meng, L., Li, S., Kong, Q., Wang, M., Zhang, X., Zhu, X., et al. (2021). Two new 19-hydroxy bufadienolides with cytotoxic activity from the skins of Bufo melanosticus. Nat. Prod. Res. 35, 4894–4900. doi:10.1080/14786419.2020.1741582
Meng, Z., Garrett, C. R., Shen, Y., Liu, L., Yang, P., Huo, Y., et al. (2012). Prospective randomised evaluation of traditional Chinese medicine combined with chemotherapy: A randomised phase II study of wild toad extract plus gemcitabine in patients with advanced pancreatic adenocarcinomas. Brit J. Cancer 107, 411–416. doi:10.1038/bjc.2012.283
Meng, Z., Yang, P., Shen, Y., Bei, W., Zhang, Y., Ge, Y., et al. (2009). Pilot study of huachansu in patients with hepatocellular carcinoma, nonsmall-cell lung cancer, or pancreatic cancer. Cancer-Am Cancer Soc. 115, 5309–5318. doi:10.1002/cncr.24602
Messina, C., Cattrini, C., Soldato, D., Vallome, G., Caffo, O., Castro, E., et al. (2020). BRCA mutations in prostate cancer: Prognostic and predictive implications. J. Oncol. 2020, 1–7. doi:10.1155/2020/4986365
Moreira-Silva, F., Henrique, R., and Jeronimo, C. (2022). From therapy resistance to targeted therapies in prostate cancer. Front. Oncol. 12, 877379. doi:10.3389/fonc.2022.877379
Morel, K. L., Hamid, A. A., Clohessy, J. G., Pandell, N., Ellis, L., and Sweeney, C. J. (2021). NF-κB blockade with oral administration of dimethylaminoparthenolide (DMAPT), delays prostate cancer resistance to androgen receptor (AR) inhibition and inhibits AR variants. Mol. Cancer Res. 19, 1137–1145. doi:10.1158/1541-7786.mcr-21-0099
Morishita, S., Shoji, M., Oguni, Y., Ito, C., Higuchi, M., and Sakanashi, M. (1992). Pharmacological actions of "kyushin," a drug containing toad venom: Cardiotonic and arrhythmogenic effects, and excitatory effect on respiration. Am. J. Chin. Med. 20, 245–256. doi:10.1142/s0192415x92000254
Naito, Y., Kuboki, Y., Ikeda, M., Harano, K., Matsubara, N., Toyoizumi, S., et al. (2021). Safety, pharmacokinetics, and preliminary efficacy of the PARP inhibitor talazoparib in Japanese patients with advanced solid tumors: Phase 1 study. Invest. New Drug 39, 1568–1576. doi:10.1007/s10637-021-01120-7
Neerati, P., and Munigadapa, S. (2022). Novel indole derivative as the first P-glycoprotein inhibitor from the skin of Indian toad <i>(Bufo melanostictus)</i>. Turk J. Pharm. Sci. 19, 63–69. doi:10.4274/tjps.galenos.2021.47417
Niu, J., Wang, J., Zhang, Q., Zou, Z., and Ding, Y. (2021). Cinobufagin-induced DNA damage response activates G2/M checkpoint and apoptosis to cause selective cytotoxicity in cancer cells. Cancer Cell Int. 21, 446. doi:10.1186/s12935-021-02150-0
Niu, T., and Qin, G. (2018). The inhibitory effects of cinobufagin on the in vitro proliferation of human castration-resistant prostate cancer PC3 cells. J. Beijing Univ. Traditional Chin. Med. 41, 1019–1024.
Niu, Y., Shi, J., Wang, G., and Yang, J. (2016). The combination of Chansu injection with EOAP in stage III-IV non-Hodgkin lymphoma. Leuk. Lymphoma 25, 224–227.
Pan, Z., Luo, Y., Xia, Y., Zhang, X., Qin, Y., Liu, W., et al. (2020). Cinobufagin induces cell cycle arrest at the S phase and promotes apoptosis in nasopharyngeal carcinoma cells. Biomed. Pharmacother. 122, 109763. doi:10.1016/j.biopha.2019.109763
Pan, Z., Zhang, X., Yu, P., Chen, X., Lu, P., Li, M., et al. (2019). Cinobufagin induces cell cycle arrest at the G2/M phase and promotes apoptosis in malignant melanoma cells. Front. Oncol. 9, 853. doi:10.3389/fonc.2019.00853
Pastor, N., and Cortes, F. (2003). Bufalin influences the repair of X-ray-induced DNA breaks in Chinese hamster cells. Dna Repair 2, 1353–1360. doi:10.1016/j.dnarep.2003.08.001
Peery, R., Cui, Q., Kyei-Baffour, K., Josephraj, S., Huang, C., Dong, Z., et al. (2022). A novel survivin dimerization inhibitor without a labile hydrazone linker induces spontaneous apoptosis and synergizes with docetaxel in prostate cancer cells. Bioorgan Med. Chem. 65, 116761. doi:10.1016/j.bmc.2022.116761
Peery, R., Kyei-Baffour, K., Dong, Z., Liu, J., de Andrade, H. P., Dai, M., et al. (2020). Synthesis and identification of a novel lead targeting survivin dimerization for proteasome-dependent degradation. J. Med. Chem. 63, 7243–7251. doi:10.1021/acs.jmedchem.0c00475
Peery, R. C., Liu, J. Y., and Zhang, J. T. (2017). Targeting survivin for therapeutic discovery: Past, present, and future promises. Drug Discov. Today 22, 1466–1477. doi:10.1016/j.drudis.2017.05.009
Pei, Y. F., He, Y., Hu, L. Z., Zhou, B., Xu, H. Y., and Liu, X. Q. (2020). The crosstalk between lncRNA-SNHG7/miRNA-181/cbx7 modulates malignant character in lung adenocarcinoma. Am. J. Pathol. 190, 1343–1354. doi:10.1016/j.ajpath.2020.02.011
Qu, T., Gao, H. M., Chen, L. M., Wang, Z. M., Zhang, Q. W., and Cheng, Y. Y. (2012). Content of indole alkaloids and bufadienolides contained in toad medicines. Zhongguo Zhong Yao Za Zhi 37, 3086–3091.
Ren, J., Wang, S., Jin, L., Ma, F., Zhou, D., and Cai, Q. (2019). Cinobufagin inhibits tumor growth by inducing apoptosis through Notch signaling pathways in human cholangiocarcinoma. Transl. Cancer Res. 8, 2461–2469. doi:10.21037/tcr.2019.10.06
Robey, R. W., Pluchino, K. M., Hall, M. D., Fojo, A. T., Bates, S. E., and Gottesman, M. M. (2018). Revisiting the role of ABC transporters in multidrug-resistant cancer. Nat. Rev. Cancer 18, 452–464. doi:10.1038/s41568-018-0005-8
Sampath, V., Horesh, N., Sasi, B., Zannadeh, H., Pogodin, I., Singh, S. V., et al. (2022). Synthesis and biological evaluation of novel bufalin derivatives. Int. J. Mol. Sci. 23, 4007. doi:10.3390/ijms23074007
Schatten, H. (2018). Brief overview of prostate cancer statistics, grading, diagnosis and treatment strategies. Adv. Exp. Med. Biol. 1095, 1–14. doi:10.1007/978-3-319-95693-0_1
Shao, H., Li, B., Li, H., Gao, L., Zhang, C., Sheng, H., et al. (2021). Novel strategies for solubility and bioavailability enhancement of bufadienolides. Molecules 27, 51. doi:10.3390/molecules27010051
Siegel, R. L., Miller, K. D., Fuchs, H. E., and Jemal, A. (2022). Cancer statistics, 2022. Ca-Cancer J. Clin. 72, 7–33. doi:10.3322/caac.21708
Soumoy, L., Ghanem, G. E., Saussez, S., and Journe, F. (2022). Bufalin for an innovative therapeutic approach against cancer. Pharmacol. Res. 184, 106442. doi:10.1016/j.phrs.2022.106442
Tan, X., Liang, X., Xi, J., Guo, S., Meng, M., Chen, X., et al. (2021). Clinical efficacy and safety of huachansu injection combination with platinum-based chemotherapy for advanced non-small cell lung cancer: A systematic review and meta-analysis of randomized controlled trials. Medicine 100, e27161. doi:10.1097/md.0000000000027161
Teyssonneau, D., Thiery-Vuillemin, A., Dariane, C., Barret, E., Beauval, J. B., Brureau, L., et al. (2022). PARP inhibitors as monotherapy in daily practice for advanced prostate cancers. J. Clin. Med. 11, 1734. doi:10.3390/jcm11061734
Thomas, C., and Tampe, R. (2020). Structural and mechanistic principles of ABC transporters. Annu. Rev. Biochem. 89, 605–636. doi:10.1146/annurev-biochem-011520-105201
Tian, H. Y., Yuan, X. F., Jin, L., Li, J., Luo, C., Ye, W. C., et al. (2014). A bufadienolide derived androgen receptor antagonist with inhibitory activities against prostate cancer cells. Chem-Biol Interact. 207, 16–22. doi:10.1016/j.cbi.2013.10.020
Toma, S., Morishita, S., Kuronuma, K., Mishima, Y., Hirai, Y., and Kawakami, M. (1987). Metabolism and pharmacokinetics of cinobufagin. Xenobiotica 17, 1195–1202. doi:10.3109/00498258709167411
Tu, S. P., Zhong, J., Tan, J. H., Jiang, X. H., Qiao, M. M., Wu, Y. X., et al. (2000). Induction of apoptosis by arsenic trioxide and hydroxy camptothecin in gastriccancer cells in vitro. World J. Gastroentero 6, 532–539. doi:10.3748/wjg.v6.i4.532
Wade, C. A., and Kyprianou, N. (2018). Profiling prostate cancer therapeutic resistance. Int. J. Mol. Sci. 19, 904. doi:10.3390/ijms19030904
Wang, C., Liu, Z., Ke, Y., and Wang, F. (2019). Intrinsic FGFR2 and ectopic FGFR1 signaling in the prostate and prostate cancer. Front. Genet. 10, 12. doi:10.3389/fgene.2019.00012
Wang, J., Wang, J. Q., Cai, C. Y., Cui, Q., Yang, Y., Wu, Z. X., et al. (2020). Reversal effect of ALK inhibitor NVP-TAE684 on ABCG2-overexpressing cancer cells. Front. Oncol. 10, 228. doi:10.3389/fonc.2020.00228
Wang, J., Xia, Y., Zuo, Q., and Chen, T. (2018). Molecular mechanisms underlying the antimetastatic activity of bufalin. Mol. Clin. Oncol. 8, 631–636. doi:10.3892/mco.2018.1591
Wang, J. Q., Teng, Q. X., Lei, Z. N., Ji, N., Cui, Q., Fu, H., et al. (2020). Reversal of cancer multidrug resistance (MDR) mediated by ATP-binding cassette transporter G2 (ABCG2) by AZ-628, a RAF kinase inhibitor. Front. Cell Dev. Biol. 8, 601400. doi:10.3389/fcell.2020.601400
Wang, J. Q., Wu, Z. X., Yang, Y., Teng, Q. X., Li, Y. D., Lei, Z. N., et al. (2021). ATP-binding cassette (ABC) transporters in cancer: A review of recent updates. J. Evid-Based Med. 14, 232–256. doi:10.1111/jebm.12434
Wang, P. F., Fang, Y. G., Li, Z. Y., Chen, L. M., Wang, Z. M., Zou, Z. M., et al. (2018). Study on quality control method of toad venom based on characteristic chromatogram and QAMS. Zhongguo Zhong Yao Za Zhi 43, 2863–2871. doi:10.19540/j.cnki.cjcmm.2018.0088
Wang, Y., Lonard, D. M., Yu, Y., Chow, D. C., Palzkill, T. G., Wang, J., et al. (2014). Bufalin is a potent small-molecule inhibitor of the steroid receptor coactivators SRC-3 and SRC-1. Cancer Res. 74, 1506–1517. doi:10.1158/0008-5472.can-13-2939
Wang, Z. J., Sun, L., and Heinbockel, T. (2014). Resibufogenin and cinobufagin activate central neurons through an ouabain-like action. Plos One 9, e113272. doi:10.1371/journal.pone.0113272
Wei, W., Yu, Y., Wang, X., Yang, L., Zhang, H., Ji, H., et al. (2019). Simultaneous determination of bufalin and its nine metabolites in rat plasma for characterization of metabolic profiles and pharmacokinetic study by LC(-)MS/MS. Molecules 24, 1662. doi:10.3390/molecules24091662
Wells, J. M. (2021). Cancer burden, finance, and health-care systems. Lancet Oncol. 22, 13–14. doi:10.1016/s1470-2045(20)30681-1
Wu, J., Zhang, D., Ni, M., Xue, J., Wang, K., Duan, X., et al. (2020). Effectiveness of huachansu injection combined with chemotherapy for treatment of gastric cancer in China: A systematic review and meta-analysis. J. Tradit. Chin. Med. 40, 749–757. doi:10.19852/j.cnki.jtcm.2020.05.004
Wu, Z. X., Yang, Y., Wang, J. Q., Zhou, W. M., Chen, J., Fu, Y. G., et al. (2020). Elevated ABCB1 expression confers acquired resistance to aurora kinase inhibitor GSK-1070916 in cancer cells. Front. Pharmacol. 11, 615824. doi:10.3389/fphar.2020.615824
Xie, J. T., Dey, L., Wu, J. A., Lowell, T. K., and Yuan, C. S. (2001). Cardiac toxicity of resibufogenin: Electrophysiological evidence. Acta Pharmacol. Sin. 22, 289–297.
Xie, M., Chen, X., Qin, S., Bao, Y., Bu, K., and Lu, Y. (2018). Clinical study on thalidomide combined with cinobufagin to treat lung cancer cachexia. J. Cancer Res. Ther. 14, 226–232. doi:10.4103/0973-1482.188436
Xiong, X., Lu, B., Tian, Q., Zhang, H., Wu, M., Guo, H., et al. (2019). Inhibition of autophagy enhances cinobufagininduced apoptosis in gastric cancer. Oncol. Rep. 41, 492–500. doi:10.3892/or.2018.6837
Xu, D., Wang, J., Chen, W., Yang, X., Zhou, J., Ma, H., et al. (2021). Evaluation of analgesic and anti-inflammatory actions of indolealkylamines from toad venom in mice using lipidomics and molecular docking. J. Ethnopharmacol. 269, 113677. doi:10.1016/j.jep.2020.113677
Xu, L. S., Feng, Q. L., Zhang, X. P., Wang, Y. G., and Yao, M. (2019). Study on analgesic effect and mechanism of cinobufagin on rats with bone cancer pain. Zhonghua Yi Xue Za Zhi 99, 1307–1311. doi:10.3760/cma.j.issn.0376-2491.2019.17.007
Yang, A. L., Wu, Q., Hu, Z. D., Wang, S. P., Tao, Y. F., Wang, A. M., et al. (2021). A network pharmacology approach to investigate the anticancer mechanism of cinobufagin against hepatocellular carcinoma via downregulation of EGFR-CDK2 signaling. Toxicol. Appl. Pharm. 431, 115739. doi:10.1016/j.taap.2021.115739
Yang, F., Zhang, Y., Ressler, S. J., Ittmann, M. M., Ayala, G. E., Dang, T. D., et al. (2013). FGFR1 is essential for prostate cancer progression and metastasis. Cancer Res. 73, 3716–3724. doi:10.1158/0008-5472.can-12-3274
Yang, T., Jiang, Y. X., Wu, Y., Lu, D., Huang, R., Wang, L. L., et al. (2021). Resibufogenin suppresses triple-negative breast cancer angiogenesis by blocking VEGFR2-mediated signaling pathway. Front. Pharmacol. 12, 682735. doi:10.3389/fphar.2021.682735
Yang, Y., Ji, N., Cai, C. Y., Wang, J. Q., Lei, Z. N., Teng, Q. X., et al. (2020). Modulating the function of ABCB1: In vitro and in vivo characterization of sitravatinib, a tyrosine kinase inhibitor. Cancer Commun. 40, 285–300. doi:10.1002/cac2.12040
Yuan, X. F., Tian, H. Y., Li, J., Jin, L., Jiang, S. T., Liu, K. W., et al. (2014). Synthesis of bufalin derivatives with inhibitory activity against prostate cancer cells. Nat. Prod. Res. 28, 843–847. doi:10.1080/14786419.2014.881363
Yuan, Z., Shi, X., Qiu, Y., Jia, T., Yuan, X., Zou, Y., et al. (2017). Reversal of P-gp-mediated multidrug resistance in colon cancer by cinobufagin. Oncol. Rep. 37, 1815–1825. doi:10.3892/or.2017.5410
Zhai, X. F., Fang, F. F., Liu, Q., Meng, Y. B., Guo, Y. Y., and Chen, Z. (2013). MiR-181a contributes to bufalin-induced apoptosis in PC-3 prostate cancer cells. Bmc Complem Altern. M. 13, 325. doi:10.1186/1472-6882-13-325
Zhan, Y., Qiu, Y., Wang, H., Wang, Z., Xu, J., Fan, G., et al. (2020). Bufalin reverses multidrug resistance by regulating stemness through the CD133/nuclear factor-κB/MDR1 pathway in colorectal cancer. Cancer Sci. 111, 1619–1630. doi:10.1111/cas.14345
Zhang, C., Chao, F., Wang, S., Han, D., and Chen, G. (2022). Cell-free DNA as a promising diagnostic biomarker in prostate cancer: A systematic review and meta-analysis. J. Oncol. 2022, 1505087. doi:10.1155/2022/1505087
Zhang, C., Ma, K., and Li, W. Y. (2019). <p>Cinobufagin suppresses the characteristics of osteosarcoma cancer cells by inhibiting the IL-6-OPN-STAT3 pathway</p>. Drug Des. Devel Ther. 13, 4075–4090. doi:10.2147/dddt.s224312
Zhang, D. M., Liu, J. S., Deng, L. J., Chen, M. F., Yiu, A., Cao, H. H., et al. (2013). Arenobufagin, a natural bufadienolide from toad venom, induces apoptosis and autophagy in human hepatocellular carcinoma cells through inhibition of PI3K/Akt/mTOR pathway. Carcinogenesis 34, 1331–1342. doi:10.1093/carcin/bgt060
Zhang, D. M., Liu, J. S., Tang, M. K., Yiu, A., Cao, H. H., Jiang, L., et al. 2012). Bufotalin from Venenum Bufonis inhibits growth of multidrug resistant HepG2 cells through G2/M cell cycle arrest and apoptosis. Eur. J. Pharmacol. 692, 19–28. doi:10.1016/j.ejphar.2012.06.045
Zhang, J., Hong, Y., Xie, P., Chen, Y., Jiang, L., Yang, Z., et al. (2020). Spatial lipidomics reveals anticancer mechanisms of bufalin in combination with cinobufagin in tumor-bearing mice. Front. Pharmacol. 11, 593815. doi:10.3389/fphar.2020.593815
Zhang, J. J., Zhou, X. H., Zhou, Y., Wang, Y. G., Qian, B. Z., He, A. N., et al. (2019). Bufalin suppresses the migration and invasion of prostate cancer cells through HOTAIR, the sponge of miR-520b. Acta Pharmacol. Sin. 40, 1228–1236. doi:10.1038/s41401-019-0234-8
Zhang, L., Huang, X., Guo, T., Wang, H., Fan, H., and Fang, L. (2020). Study of cinobufagin as a promising anticancer agent in uveal melanoma through intrinsic apoptosis pathway. Front. Oncol. 10, 325. doi:10.3389/fonc.2020.00325
Zhang, P., Cui, Z., Liu, Y., Wang, D., Liu, N., and Yoshikawa, M. (2005). Quality evaluation of traditional Chinese drug toad venom from different origins through a simultaneous determination of bufogenins and indole alkaloids by HPLC. Chem. Pharm. Bull. 53, 1582–1586. doi:10.1248/cpb.53.1582
Zhang, P. W., Jiang, R. W., Ye, W. C., and Tian, H. Y. (2014). Bufadienolides from venom of Bufo bufo gargarizans. Zhongguo Zhong Yao Za Zhi 39, 841–845.
Zhang, W., Jiang, B., Liu, Y., Xu, L., and Wan, M. (2022). Bufotalin induces ferroptosis in non-small cell lung cancer cells by facilitating the ubiquitination and degradation of GPX4. Free Radic. Bio Med. 180, 75–84. doi:10.1016/j.freeradbiomed.2022.01.009
Zhang, X., Zhao, X., Liu, K., Che, Y., Qiu, X., Qu, Y., et al. (2020). Bufalin: A systematic review of research hotspots and antitumor mechanisms by text mining and bioinformatics. Am. J. Chin. Med. 48, 1633–1650. doi:10.1142/s0192415x20500810
Zhang, Y., Dong, Y., Melkus, M. W., Yin, S., Tang, S. N., Jiang, P., et al. (2018). Role of P53-senescence induction in suppression of LNCaP prostate cancer growth by cardiotonic compound bufalin. Mol. Cancer Ther. 17, 2341–2352. doi:10.1158/1535-7163.mct-17-1296
Zhao, L., Fu, L., Xu, Z., Fan, R., Xu, R., Fu, R., et al. (2019). The anticancer effects of cinobufagin on hepatocellular carcinoma Huh7 cells are associated with activation of the p73 signaling pathway. Mol. Med. Rep. 19, 4119–4128. doi:10.3892/mmr.2019.10108
Zhao, R., Ma, X., Bai, L., Li, X., Mamouni, K., Yang, Y., et al. (2021). Overcoming prostate cancer drug resistance with a novel organosilicon small molecule. Neoplasia 23, 1261–1274. doi:10.1016/j.neo.2021.11.006
Zheng, Y., Deng, L., Cao, H., Xu, N., Zhang, D., Tian, H., et al. (2022). Screening of bufadienolides from toad venom identifies gammabufotalin as a potential anti-inflammatory agent. Planta Med. 88, 43–52. doi:10.1055/a-1248-2626
Keywords: toad venom-derived bufadienolides, therapeutic application, prostate cancers, current status, future directions
Citation: Ye Q, Zhou X, Han F and Zheng C (2023) Toad venom-derived bufadienolides and their therapeutic application in prostate cancers: Current status and future directions. Front. Chem. 11:1137547. doi: 10.3389/fchem.2023.1137547
Received: 04 January 2023; Accepted: 07 March 2023;
Published: 16 March 2023.
Edited by:
Peter Rose, University of Nottingham, United KingdomReviewed by:
Qingbin Cui, University of Toledo College of Medicine and Life Sciences, United StatesJunmin Zhang, Lanzhou University, China
Copyright © 2023 Ye, Zhou, Han and Zheng. This is an open-access article distributed under the terms of the Creative Commons Attribution License (CC BY). The use, distribution or reproduction in other forums is permitted, provided the original author(s) and the copyright owner(s) are credited and that the original publication in this journal is cited, in accordance with accepted academic practice. No use, distribution or reproduction is permitted which does not comply with these terms.
*Correspondence: Caijuan Zheng, caijuan2002@163.com
†These authors have contributed equally to this work