KI effects on corrosion inhibition for 1018 steel in acid media using Medicago sativa
- 1Metropolitan Polytechnic University of Hidalgo, Tolcayuca, México
- 2School of Chemical Sciences and Engineering, Autonomous University of Morelos State, Cuernavaca, Morelos, México
- 3Research Center for Engineering and Applied Sciences, Autonomous University of Morelos State, Cuernavaca, Morelos, México
Medicago sativa (M. sativa) extract is a safe and eco-friendly corrosion inhibitor of 1018 steel in acid media. The inhibitor reached a maximum efficiency of 85% by using 500 ppm. In this work, we study the use of KI as an add-on to increase the inhibition efficiency of M. sativa, as well as making the natural inhibitor competitive with the commercial ones. We evaluated the effect of halide ions through the variation of different concentrations of KI and its synergy with the extract of M. sativa as a corrosion inhibitor of carbon steel in 0.5 M sulfuric acid and at different temperatures. The results were obtained through electrochemical techniques such as electrochemical impedance spectroscopy (EIS), potentiodynamic polarization (PDP) curves, and weight loss gravimetric technique. It was found that halide ions increase the inhibition efficiency of M. sativa from 85 to 95% when 5 mM concentration of KI was used. The efficiency of the inhibition increases proportionally with the immersion time but reduces when the temperature increases. The addition of iodide ions (I−) revealed that it exerts a synergistic effect on the inhibition of corrosion with the extract of M. sativa. However, when studying the metal surface using a scanning electron microscope, pitting corrosion was found.
Introduction
Steel is one of the few materials that have significance in human life. During the modern era, it drove architectural, technological, and even medical development, as it is a major constituent in buildings, bridges, vehicles, ships, and trains, among others.
Steel, compared to other materials of its type, has low production costs. The energy required for extracting iron from its ore is about 25% of what is needed for extracting aluminum. Steel is environmentally friendly as it can be recycled. Iron as an element is present in Earth’s crust by 5.6%, representing a secure raw material base (Gan 2011).
Carbon steels are used in a wide range of applications, such as structural components, industrial pipes, and kitchen appliances, and are considered a more economical option than the costly corrosion-resistant alloys. Carbon steels typically contain less than 1.5% carbon along with a low presence of Mn, Si, P, and S. Based on the percentage of carbon, the classification is further divided into three forms: low-carbon steels (<0.25% C), medium-carbon steels (0.25–0.70% C), and high-carbon steels (0.70–1.05% C) (Dwivedi et al., 2017).
The effects caused by corrosion on different metals are of much interest for industries, due to its impact on the economic loss and the failure of security systems, as they are exposed to aggressive media (Revie and Uhlig 2008). It has been looked for different alternatives for corrosion protection as there is preoccupation of having options with no effects on environment and following the standards, in which the use of green inhibitors is one of the most used alternatives.
The use of vegetal species as corrosion inhibitors has been widely used. However, in some cases, they have been reported to possess low efficiencies, and thus, they are not able to reach the standards for a good inhibitor. To increase the inhibitor efficiency, different research studies proved that the use of halide ions along with vegetal extracts as corrosion inhibitors reported higher efficiency. For example, Occimum viridis is used as a corrosion inhibitor for mild steel in sulfuric acid showing a maximum efficiency of 69%, which after adding 0.5 mM of KI showed an increase in the efficiency of 94.5% (Oguzie 2006). Sansevieria trifasciata as a corrosion inhibitor for aluminum in hydrochloric acid shows an initial efficiency of 87%, and by adding 0.5 mM of KI, it reaches a maximum efficiency of 94% (Oguzie 2007). Following this, in 2013, Priya conducted a study on Abelmoschus esculentus seeds and used as a corrosion inhibitor for mild steel in sulfuric acid where an efficiency of 94% was obtained. In this research, she proved different haloid salts such as KCl, KBr, and KI at 0.1 mM obtaining maximum efficiencies of 86, 91.5, and 99%, respectively, where KI is clearly the one that shows a better interaction with the extract and synergic parameter S with values
A synergic effect of halides and quaternary ammonium salts on steel corrosion in sulfuric acid has been observed. The ion efficiency increases in the following order: Cl < Br < I. Pseudohalides also show synergism, while the fluoride ion does not show any synergic activity. Active anions are selectively chemisorbed on the metallic surface and raise the absorbability of the inhibitory cations, which interfere in the corrosion process (Räuchle F. and Díaz M. I. 1990).
Medicago sativa (M. sativa) extract has been previously proved as a corrosion inhibitor for 1018 steel in acid media showing a maximum efficiency of 90% after 8 h, and it maintained until 12 h. After this time, the inhibition efficiency decreases (Rodríguez Torres et al., 2016). The aim of the present work is to evaluate the effect of adding different concentrations of KI when using M. sativa as a corrosion inhibitor for 1018 steel in 0.5 M of H2SO4.
Experimental procedure
Testing metal
Steel samples with a chemical composition w/w%: C 0.14%, Mn 0.90%, Pmax 0.05%, Smax 0.04%, and Fe± 98.87% were used. The samples were encapsulated in a commercial epoxy resin. It is used for corrosion protection covering almost the entire surface, leaving a defined contact area of 1 cm2 for electrochemical tests and 5.52 cm2 for gravimetric tests. Each of the samples was sanded with a carbide silicon paper from 100 to 600 grades to obtain a homogenous surface.
Aggressive solution
Sulfuric acid was used at a reactive grade to prepare 0.5 M of H2SO4 solution.
Inhibitory solution
To prepare the inhibitory solution, 500 ppm of M. sativa extract was used, as it was considered the best concentration as previously reported (Rodríguez Torres et al., 2016). We added different KI concentrations (1–5 mM) to evaluate the effect of halide ions on inhibition efficiency.
Electrochemical evaluation
The electrochemical techniques used for the evaluation of M. sativa with different KI concentrations as a corrosion inhibitor were electrochemical impedance spectroscopy (EIS) and polarization potentiodynamic (PDP) curves. During the measurements, a conventional three-electrode cell was used, with 1018 carbon steel as a working electrode, Ag/AgCl as a reference electrode, and graphite as a counter electrode. PDP measurements were carried out with a speed rate of 1 mV/s at an interval of ±1000 mV from the corrosion potential. The corrosion current density values, icorr, were obtained using Tafel extrapolation. The inhibition efficiency (η) was calculated according to Eq. 1:
where
EIS tests were carried out with a signal amplitude of 10 mV and a frequency interval between 0.05 and 10,000 Hz in an ACM instrument GillAC potentiostat.
Results and discussion
Electrochemical impedance spectroscopy
Figure 1 shows the Nyquist diagrams for the effect of different KI concentrations on 1018 steel corrosion inhibition in acid media using the aerial parts of M. sativa in a 500 ppm concentration. When KI is added, it shows flattened capacitive semicircles with the center in the real axis. This indicates that the corrosion mechanism is controlled due to the charge-transfer resistance (Bentiss et al., 2009; Behpour et al., 2010). By increasing the KI concentration in media, the diameter of the semicircles increases. This indicates an increase in the resistance and the produced efficiency in the interaction between halide ions and the inhibitor.
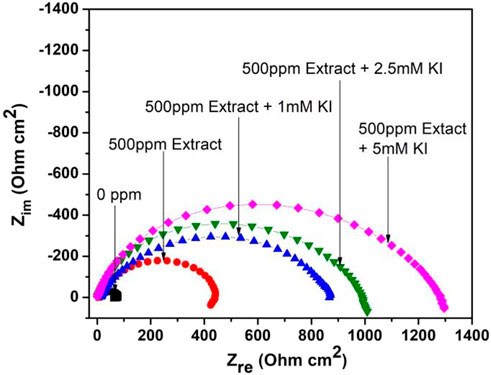
FIGURE 1. Nyquist diagram showing the effect of different concentrations of KI on the corrosion inhibition of 1018 steel in acid media using M. sativa aerial parts with 500 ppm concentration at 25 ± 2°C.
Bode diagrams are shown in Figure 2. In phase diagram Figure 2A, a peak is observed around 100 Hz, which is displaced to lower frequencies as the KI concentration increases and the phase angle increases as the KI concentration increases. In module diagram Figure 2B, the solution resistance at high frequencies and at lower frequencies, the double-layer capacitance, and the charge-transfer resistance are shown, where the module value increases as the KI concentration increases, showing only one slope and as a consequence one time constant.
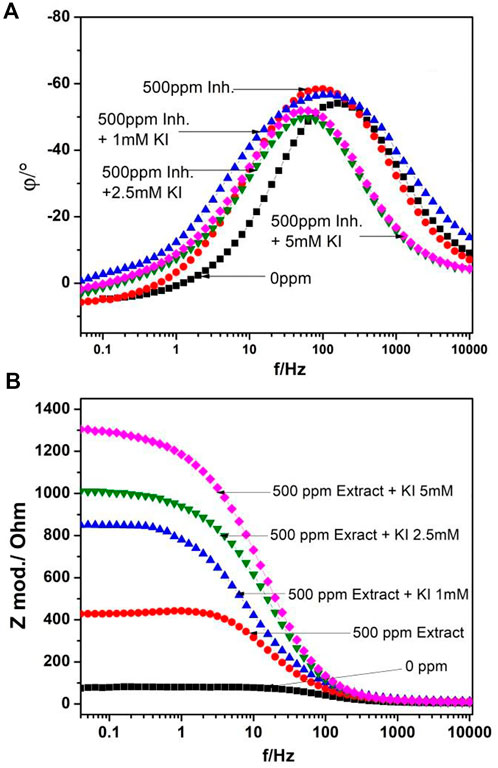
FIGURE 2. Bode diagrams for 1018 steel corrosion in acid media when adding 500 ppm of M. sativa at different KI concentrations (A) phase diagram and (B) module diagram.
Figure 3 shows the equivalent circuit model, where R1 is the solution resistance and R2 is the charge-transfer resistance. The double-layer capacitance value is affected due to imperfections on the surface, such as roughness; thus, this effect is simulated through a constant phase element QPE1 (Bommersbach et al., 2006).
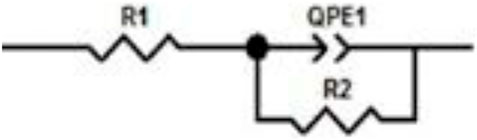
FIGURE 3. Adjusted and modeled data for the equivalent circuit of corrosion inhibition for 1018 steel in acid media with 500 ppm concentration of M. sativa and the addition of 5 mM of KI.
Impedance-associated values
where
The double-layer capacitance value
The obtained parameters for the simulation and efficiency calculus are shown in Table 1, where it is observed that as the KI concentration increases, the charge-transfer resistance increases and at the same time the inhibitor efficiency increases and the values of the double electrochemical layer decrease. This can be caused due to the local dielectric constant and/or due to the increase in thickness in the double electric (Kuznetsov and Andreev, 2014) layer. This suggests that the presence of the extract and halide ions modifies the double-layer electric structure due to the inhibitor molecules that act by adsorption in the metal/solution interphase (Cardozo da Rocha et al., 2010).

TABLE 1. Electrochemical parameters obtained from the model circuit and corrosion inhibition efficiency (IE) for 1018 steel in acid media with and without 500 ppm concentration of M. sativa at different KI concentrations.
Temperature effect on the corrosion inhibition for 1018 steel in acid media at different KI concentration is shown in Figure 4. When different KI concentrations are added, the corrosion mechanism changes showing a depressed semicircle, followed by a second semicircle, which is more evident in 1 mM concentration. However, at high concentrations, it is not evident because the time constant is too low.
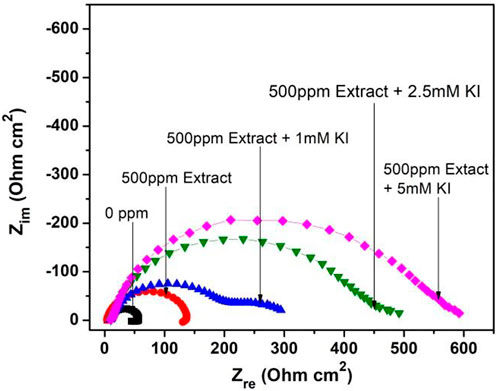
FIGURE 4. Nyquist diagram for 1018 steel corrosion in acid media with and without 500 ppm M. sativa extract and the addition of different KI concentrations at 40°C.
As a result, the corrosion process happens in two stages. The first is metal oxidation (charge-transfer process). The second stage is due to mass transport, as it is the slowest process where metallic ion diffusion could be exhibited from the metallic surface to the solution or the formation of a second corrosion product layer. Similar mechanisms have been observed for steel corrosion when using halide ions (Lagrenée et al., 2002).
Figure 5 shows the effect of temperature when it is increased to 60°C for 1018 steel corrosion with 500 ppm M. sativa extract and different KI concentrations, and a capacitive behavior defining a depressed semicircle at high frequencies and an inductive semicircle at low frequencies are observed. As the KI concentration increases, the semicircle diameter increases, favoring M. sativa inhibition effect.
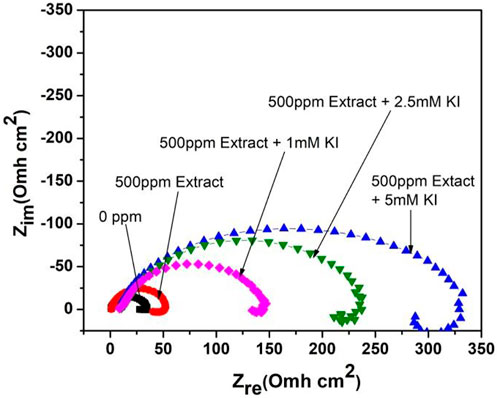
FIGURE 5. Nyquist diagrams for 1018 steel corrosion in acid media with and without 500 ppm of M. sativa and the addition of different KI concentrations at 60°C.
Inhibition efficiency comparisons of 500 ppm M. sativa with different KI concentrations and different temperatures are shown in Figure 6. When the KI concentration increases, the efficiency increases considerably, and as the temperature increases, the efficiency decreases. When KI is added at a concentration of 5 mM, the efficiency values are maintained in the range of 91–95%.
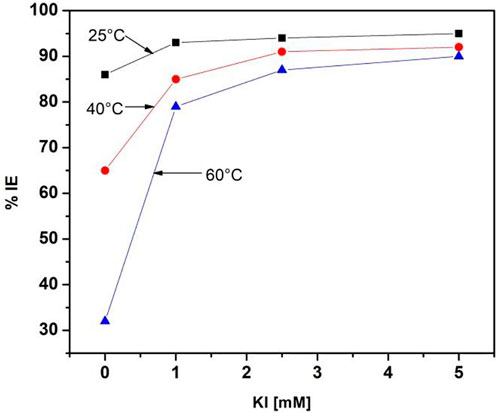
FIGURE 6. M. sativa inhibition efficiency comparison at 500 ppm with different KI concentrations and different temperatures.
Nyquist diagrams at different exposure times for 1018 steel in acid media with 500 ppm of M. sativa and the addition of 5 mM KI are shown in Figure 7. It is observed that the semicircle diameter remains almost constant for impedance values around 1,200 Ωcm2 until 40 h. However, its corrosion mechanism, at an initial time, is controlled by the charge-transfer resistance, with changes in time showing that for low frequencies, a second semicircle changing its mechanism into a two stage is observed; the first leading to metal oxidation (charge-transfer process) and the second stage is the mass-transfer process, thus causing a possible metallic ion diffusion from the metallic surface toward the solution or to the formation of a second layer.
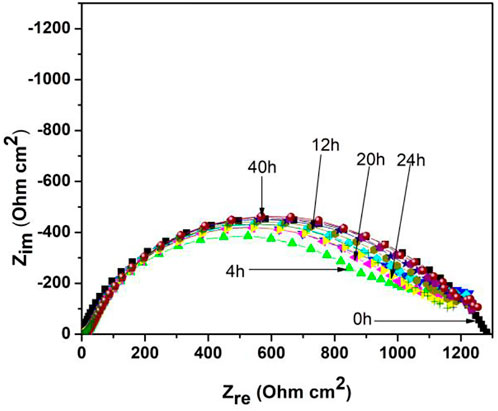
FIGURE 7. Nyquist diagrams at different exposure times of 1018 steel in acid media with 500 ppm of M. sativa and the addition of 5 mM KI at 25 ± 2°C.
This mass-transfer process is a characteristic of adsorption inhibitors due to the adsorption of their molecules onto the metal surface, which limits O2 diffusion on the surface or retains the metallic ions. This provokes a reduction in the corrosion rate (Feliu and Andrade 1991).
Synergistic effect
The inhibition mechanism and the KI-adding effect require knowing the interaction between protective molecules and the metal surface. According to the steel dissolution mechanism in (Chauhan and Gunasekaran, 2007) sulfuric acid (Bockris et al., 1961), steel electrodissolution in an acid solution depends mainly on the adsorbed species as shown as follows:
The hydrogen evolution cathodic reaction is given by the following steps:
With the presence of halide ions (X), the anodic dissolution is given by:
Some aspects such as its polarization ease, high hydrophobicity, and its low electronegativity in comparison with other halides make the iodide ions have good chemisorption onto the metallic surface, favoring a better synergic inhibitor (Chin and Nobe, 1972; MacFarlane and Smedley, 1986; Jeyaprabha et al., 2006).
Another factor that promotes the adsorption of organic molecules is the chemisorption of I− ions which is capable of decreasing the hydrophilicity of metal surfaces. However, this effect is much less significant than the surface charge effect (Kuznetsov 1996).
The inhibitor is adsorbed due to the metallic surface’s charge attraction (Priya et al., 2013), where iodide ions are being chemisorbed. This electrostatic interaction between the inhibitor and halide ions makes a greater surface coverage and therefore an increase in its efficiency (Umoren et al., 2010; Azim et al., 1995).
On the other hand, the cathodic reaction with an inhibitor is given as follows:
The synergism parameter (S) between the inhibitor and the halide ions was calculated using the Aramaki and Hackermann relation (Obot et al., 2010; Oguzie et al., 2004; Umoren and Ebenso 2007):
where
The values at room temperature and 40°C were greater than 1, indicating the existence of a synergic effect that favors metal protection, while at 60°C, the synergic parameter is equal to 1, suggesting that the interaction between inhibitor compounds and halide ions has been lost, but not speeding up the corrosion process in metal.
Potentiodynamic polarization (PDP) curves for the aerial parts of M. sativa extract
In Figure 8, the polarization curves for 1018 steel corrosion in acid media with 500 ppm of M. sativa at different KI concentrations are shown. An evident decrease in the corrosion density is observed, and at the same time, the corrosion potential is displaced to noble potentials when the KI concentration increases, exhibiting metal protection (Ahamad et al., 2010). During the test, when 2.5 and 5 mM of KI were added, a slight passivation around 22 and −86 mV, respectively, is observed.
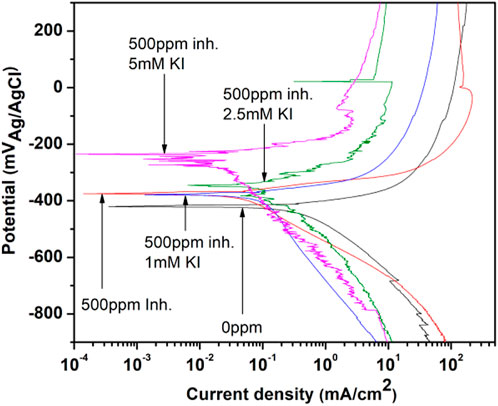
FIGURE 8. Polarization curves for 1018 steel corrosion inhibition with 500 ppm of M. sativa and different KI concentrations.
Electrochemical parameters were obtained from the polarization curves and are shown in Table 3, where Ecorr is the corrosion potential, log icorr is the corrosion current density, βa is the anodic slope, βc is the cathodic slope, and IE is the inhibition efficiency associated to the corrosion current. It is observed that the corrosion potential becomes more positive as the KI concentration increases, indicating that the interaction between the extract and iodide ions acts in the anodic process (metal dissolution) (Yaro et al., 2011).

TABLE 3. Obtained values by Tafel extrapolation for 1018 steel corrosion inhibition with 500 ppm of M. sativa and different KI concentrations at 25 ± 2°C.
However, the cathodic slope values increase. Meanwhile, the anodic slope values vary minimally with the presence of KI, showing that the corrosion process really affects the cathodic process (Chauhan and Gunasekaran, 2007).
The corrosion current density increases initially when adding 1 and 2.5 mM of KI; thus, the efficiency values decrease (Rodríguez Torres et al., 2016). However, by increasing the KI concentration to 5 mM, the current density decreases and the efficiency rises to 97%.
Figure 9 shows the polarization curves for corrosion inhibition of 1018 steel in acid media at 40°C, when 500 ppm of M. sativa and different concentrations of KI are added, where the current density decreases and the corrosion potential is displaced to more negative values when the KI concentration increases. Low passivation around −397 mV for 2.5 and 5 mM KI concentrations is observed.
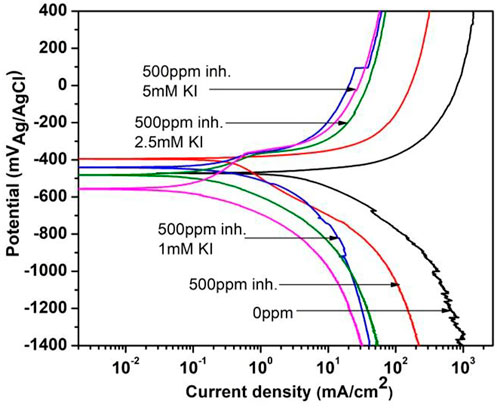
FIGURE 9. Polarization curves for corrosion inhibition of 1018 steel with and without 500 ppm of M. sativa and different KI concentrations at 40°C.
Electrochemical parameters obtained from the polarization curves are shown in Table 4, where it is evident that by adding KI concentration, efficiency values increase. There is a low variation in cathodic and anodic slopes, which indicates that the inhibition process affects both reactions during the corrosion process (Hazwan Hussin and Jain Kassim, 2011).

TABLE 4. Electrochemical parameters obtained from the polarization curves for corrosion inhibition of 1018 steel in acid media with 500 ppm of M. sativa and different KI concentrations at 40°C.
The polarization curves for 1018 steel corrosion with and without 500 ppm of M. sativa and different KI concentrations at 60°C are shown in Figure 10. It is seen that the corrosion current density is displaced to lower values according to the curve with no inhibitor and with no KI and few passivation around −350 mV for curves when KI was added.
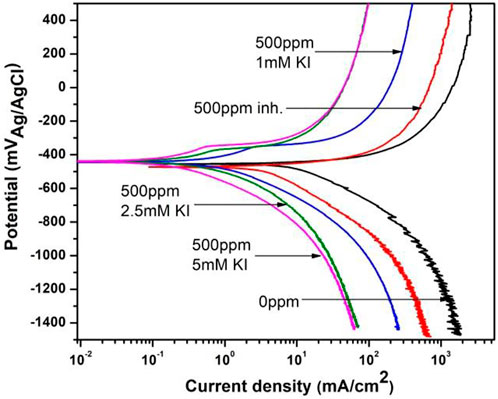
FIGURE 10. Polarization curves for corrosion inhibition of 1018 steel with and without 500 ppm of M. sativa and different KI concentrations at 60°C.
Electrochemical parameters for these polarization curves are shown in Table 5. There are no significant variations in the corrosion potential and anodic and cathodic slopes. However, the corrosion current decreases and the efficiency increases from 21 to 97% as the KI concentration increases.

TABLE 5. Electrochemical parameters obtained from the polarization curves for corrosion inhibition of 1018 steel in acid media with 500 ppm of M. sativa and different KI concentrations at 60°C.
Weight loss gravimetric technique
For gravimetric tests, we selected 8 h M. sativa residence time to compare the inhibitor efficiency with and without KI addition. The obtained values for weight loss of 500 ppm M. sativa are shown in Figure 11A, proving that by increasing the KI concentration, the weight loss decreases. However, when the temperature increases, the weight loss increases too. It is shown in Figure 11B that by adding and increasing the KI concentration, the inhibitor efficiency increases, and then it decreases when the temperature increases. However, at 60°C, when 5 mM of KI is added, the efficiency is acceptable with 85%.
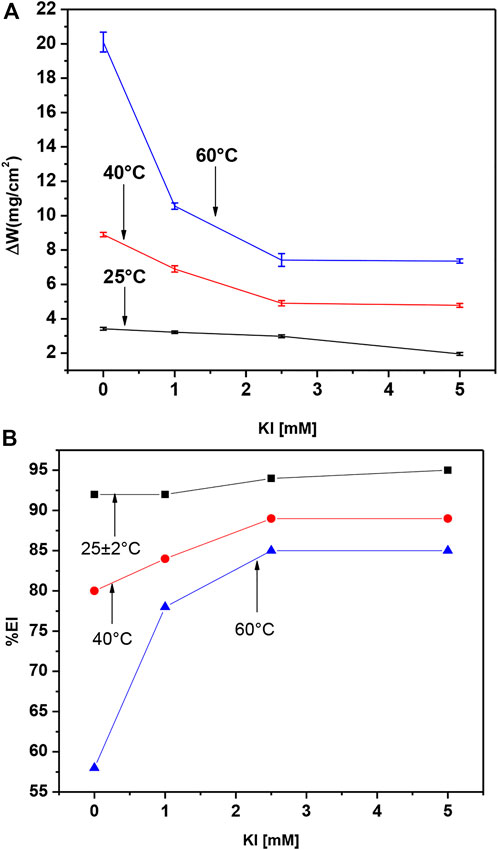
FIGURE 11. Effect of KI concentration and temperature on (A) weight loss and (B) inhibition efficiency for 1018 steel in acid media with 500 ppm of M. sativa.
Adsorption isotherm model analysis
In order to understand the adsorption phenomena of M. sativa and KI molecules on the metal surface, the use of adsorption models are very common (Nwosu et al., 2013; Christov and Popova, 2004; Eddy and Ebenso, 2008). We used different adsorption isotherms to fit the weight loss results. These adsorption isotherms contain Langmuir, Freundlich, Temkin, El-Awady, and Flory–Huggins. From all the employed isotherms, the best fitting and description of the adsorption behavior of M. sativa with different KI concentrations was obtained with the Flory–Huggins adsorption isotherm, which is represented by:
where
Figure 12 shows the dependence of ln (θ/C) vs. ln (1-θ), where a good fitting process can be observed. The adsorption coefficient R2 of M. sativa and KI in the interface Fe/solution is consistent with (Hazazi et al., 2014) the Flory–Huggins adsorption isotherm (Nwosu et al., 2013).
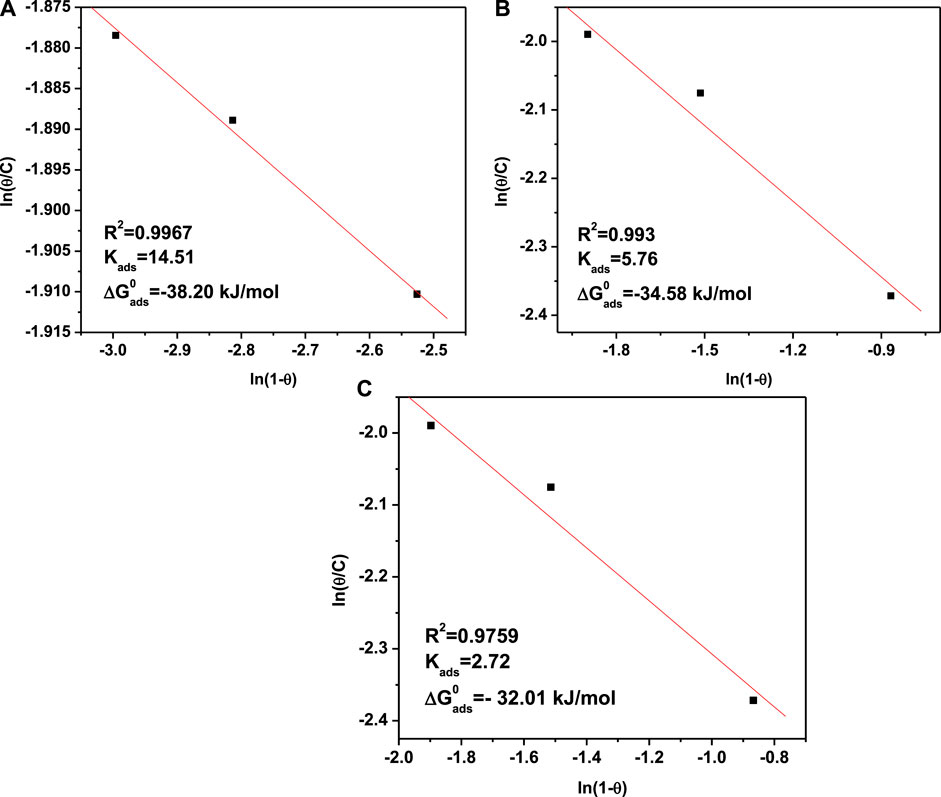
FIGURE 12. Flory–Huggins adsorption isotherms from 1018 carbon steel in 0.5 M H2SO4 with 500 ppm M. sativa and different KI concentrations at (A) 25°C, (B) 40°C, and (C) 60°C.
In order to confirm the interaction between the inhibition concentration and the steel surface, the standard free adsorption energy
where R is the gas constant, 55.5 is the molar concentration of water in the solution, and T is the absolute temperature.
Metallic Surfaces analysis by Scanning Electron Microscope (SEM)
Surface analysis comparative with and with no 500 ppm of M. sativa and 5 mM of KI are shown in Figure 13. In the tests at 25°C ± 2°C (Figure 13A) is evident that when 5 mM of KI is added, metal surface is less damaged and cracking is not present by comparing with the micrograph with no inhibitor and when inhibitor is added. However by increasing the temperature to 40°C (Figure 13B) when KI is added, it can be observed pitting corrosion, this type of corrosion is one of the most dangerous due to metal structure sensitize provoking failures in equipment (Pourbaix, 1970; Groysman, 2010; Sarver and Edwards, 2012). At 60°C (Figure 13C) is shown a less damaged surface with the appearance of some corrosion products, pitting corrosion is not present however corrosion products could be covering the pits generated and hiding them in the micrograph.
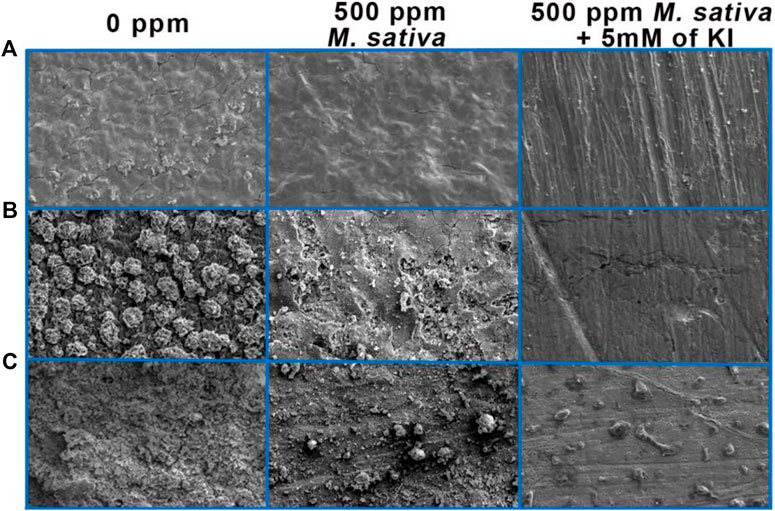
FIGURE 13. Micrographs of 1018 steel after exposure to acid media with and without 500 ppm M. sativa and 5 mM of KI at different temperatures. (A) 25°C ± 2°C, (B) 40°C, and (C) 60°C.
Conclusion
The data of electrochemical measurements indicate that M. sativa with different KI concentrations effectually inhibit the corrosion of 1018 steel in H2SO4 media. Additionally, the inhibition efficiency increases from 85 to 95% by adding 5 mM of KI.
The adsorption of M. sativa and KI at the steel interface is according to Flory–Huggins adsorption. Also, the adsorption of inhibitor molecules on to the steel surface is mainly through physical adsorption.
The inhibition efficiency decreases by raising the temperature. However, even at 60°C, the efficiency remains at 91%.
The addition of iodide ions (I−) revealed that it exerts a synergistic effect on the corrosion inhibition with the extract of M. sativa. However, when temperature increases, the metal surface becomes susceptible to pitting.
Data availability statement
The original contributions presented in the study are included in the article/Supplementary Material. Further inquiries can be directed to the corresponding author.
Author contributions
AR-T and AH performed the laboratory experimental results. AR-T and MV-C performed the chemical analysis. AR-T and JG-G studied the corrosion results.
Conflict of interest
The authors declare that the research was conducted in the absence of any commercial or financial relationships that could be construed as a potential conflict of interest.
Publisher’s note
All claims expressed in this article are solely those of the authors and do not necessarily represent those of their affiliated organizations, or those of the publisher, the editors, and the reviewers. Any product that may be evaluated in this article, or claim that may be made by its manufacturer, is not guaranteed or endorsed by the publisher.
References
Ahamad, I., Prasad, R., and Quraishi, M. A. (2010). Adsorption and inhibitive properties of some new Mannich bases of Isatin derivatives on corrosion of mild steel in acidic media. Corros. Sci. 52 (4), 1472–1481. doi:10.1016/j.corsci.2010.01.015
Aldana Gonzalez, J., Espinoza Vazquez, A., Romero Romo, M., Uruchurtu Chavarin, J., and Palomar Pardave, M. (2015). Electrochemical evaluation of cephalothin as corrosion inhibitor for API 5L X52 steel immersed in an acid medium. Arab. J. Chem. 12, 3244–3253. doi:10.1016/j.arabjc.2015.08.033
Azim, S. S., Muralidharan, S., and Iyer, V. (1995). Studies on the influence of iodide ions on the synergistic inhibition of the corrosion of mild steel in acidic solution. J. Appl. Electrochem 25, 495–500.
Bahremand, F., Shahrabi, T., and Ramezanzadeh, B. (2021). Development of a nanostructured film based on samarium (III)/polydopamine on the steel surface with superior anticorrosion and water-repellency properties. J. Colloid Interface Sci. 582, 342–352. doi:10.1016/j.jcis.2020.08.039
Behpour, M., Ghoreishi, S. M., Mohammadi, N., Soltani, N., and Salavati-Niasari, M. (2010). Investigation of some Schiff base compounds containing disulfide bond as HCl corrosion inhibitors for mild steel. Corros. Sci. 52, 4046–4057. doi:10.1016/j.corsci.2010.08.020
Bentiss, F., Jama, C., Mernari, B., El-Attrari, H., El-Kadi, L., Lebrini, M., et al. (2009). Corrosion control of mild steel using 3, 5-bis(4-methoxyphenyl)-4-amino-1, 2, 4-triazole in normal hydrochloric acid medium. Corros. Sci. 51 (8), 1628–1635. doi:10.1016/j.corsci.2009.04.009
Bockris, J. O’M., Drazic, D., and Despic, A. R. (1961). The electrode kinetics of the deposition and dissolution of iron. Electrochim. Acta 4, 325–361. doi:10.1016/0013-4686(61)80026-1
Bommersbach, P., Alemany-Dumont, C., Millet, J. P., and Normand, B. (2006). Hydrodynamic effect on the behavior of a corrosion inhibitor film: Characterization by electrochemical impedance spectroscopy. Electrochim. Acta 51, 4011–4018.
Cardozo da Rocha, J., da Cunha Ponciano Gomesa, J. A., and D’Eliab, E. (2010). Corrosion inhibition of carbon steel in hydrochloric acid solution by fruit peel aqueous extracts. Corros. Sci. 52, 2341–2348. doi:10.1016/j.corsci.2010.03.033
Chauhan, L. R., and Gunasekaran, G. (2007). Corrosion inhibition of mild steel by plant extract in dilute HCl medium. Corros. Sci. 40, 1143–1161. doi:10.1016/j.corsci.2006.08.012
Chin, R. J., and Nobe, K. (1972). Electrodissolution kinetics of iron in chloride solutions. J. Electrochem. Soc. 119, 1457–1461. doi:10.1149/1.2404023
Christov, M., and Popova, A. (2004). Adsorption characteristics of corrosion inhibitors from corrosion rate measurements. Corros. Sci. 46, 1613–1620. doi:10.1016/j.corsci.2003.10.013
Dwivedi, D., Lepková, K., and Becker, T. (2017). Carbon steel corrosion: A review of key surface properties and characterization methods. RSC Adv. 7, 4580–4610. doi:10.1039/c6ra25094g
Eddy, N. O., and Ebenso, E. E. (2008). Corrosion inhibitive properties and adsorption behaviour of ethanol extract of Piper guinensis as a green corrosion inhibitor for mild steel in H2SO4”. Afr. J. Pure Appl. Chem. 2 (6), 046–054.
Gan, Y. (2011). “Advanced steel and our society: Better steel, better world (opening address and the introduction of the specific proceedings),” in Advanced steels. Editors Y. Weng, H. Dong, and Y. Gan (Berlin, Heidelberg: Springer). doi:10.1007/978-3-642-17665-4_1
Hazazi, O. A., Fawzy, A., and Awad, M. (2014). Synergistic effect of halides on the corrosion inhibition of mild steel in H2SO4 by a triazole derivative: Kinetics and thermodynamic studies. Int. J. Electrochem. Sci. 9, 4086–4103.
Hazwan Hussin, M., and Jain Kassim, M. (2011). The corrosion inhibition and adsorption behavior of Uncaria gambir extract on mild steel in 1 M HCl. Mat. Chem. Phys. 125 (3), 461–468. doi:10.1016/j.matchemphys.2010.10.032
Jeyaprabha, C., Sathiyanarayanan, S., Muralidharan, S., and Venkatachari, G. (2006). Corrosion inhibition of iron in 0.5 molL−1 H2SO4 by halide ions. J. Braz. Chem. Soc. 17, 61–67. doi:10.1590/s0103-50532006000100009
Keleş, H., Keleş, M., Dehri, İ., and Serindağ, O. (2008). Adsorption and inhibitive properties of aminobiphenyl and its schiff base on mild steel corrosion in 0.5 M HCl medium. Colloids Surfaces, A Physicochem. Eng. Aspects 320, 138–145. doi:10.1016/j.colsurfa.2008.01.040
Lagrenée, M., Mernari, B., Bouanis, M., Traisnel, M., and Bentiss, F. (2002). Study of the mechanism and inhibiting efficiency of 3, 5-bis(4-methylthiophenyl)-4H-1, 2, 4-triazole on mild steel corrosion in acidic media. Corros. Sci. 44, 573–588. doi:10.1016/s0010-938x(01)00075-0
MacFarlane, D. R., and Smedley, S. I. (1986). The dissolution mechanism of iron in chloride solutions. J. Electrochem. Soc. 133, 2240–2244. doi:10.1149/1.2108381
Nwosu, F. O., Nnanna, L. A., and Osarolube, O. (2013). The use of eco-friendly leaf as a corrosion inhibitor of mild steel in an acidic environment. Int. J. Mater Chem. 3, 64–68.
Obot, I. B., Obi-Egbedi, N. O., Umoren, S. A., and Ebenso, E. E. (2010). Synergistic and antagonistic effects of anions and Ipomoea invulcrata as green corrosion inhibitor for aluminium dissolution in acidic medium. Int. J. Electrochem. Sci. 5, 994–1007.
Obot, I. B., Umoren, S. A., and Obi-Egbedi, N. O. (2011). Corrosion inhibition and adsorption behaviour for aluminuim by extract of Aningeria robusta in HCl solution: Synergistic effect of iodide ions. J. Mat. Environ. Sci. 2 (1), 60–71.
Oguzie, E. E. (2007). Corrosion inhibition of aluminium in acidic and alkaline media by Sansevieria trifasciata extract. Corros. Sci. 49, 1527–1539. doi:10.1016/j.corsci.2006.08.009
Oguzie, E. E., Okolue, B. N., Ebenso, E. E., Onuoha, G. N., and Onuchukwu, A. I. (2004). Evaluation of the inhibitory effect of methylene blue dye on the corrosion of aluminium in hydrochloric acid. Mat. Chem. Phys. 87, 394–401. doi:10.1016/j.matchemphys.2004.06.003
Oguzie, E. E. (2006). Studies on the inhibitive effect of Occimum viridis extract on the acid corrosion of mild steel. Mater. Chem. Phys. 99, 441–446. doi:10.1016/j.matchemphys.2005.11.018
Pourbaix, M. (1970). Significance of protection potential in pitting and intergranular corrosion. Corrosion 26 (10), 431–438. doi:10.5006/0010-9312-26.10.431
Priya, V. S., Ali Fathima Sabirneeza, A., and Subhashini, S. (2013). Synergistic effect of halides ions on the corrosion inhibition of Abelmoschus esculentus seed extract on mild steel in H2SO4. Asian J. Chem. 25 (13), 7083–7087. doi:10.14233/ajchem.2013.14440
Revie, R. W., and Uhlig, H. H. (2008). Corrosion and corrosion control. An introdruction to corrosion science and engineering”. Canada: Wiley-Interscience.
Rodríguez Torres, A., Valladares Cisneros, M. G., and González Rodríguez, J. G. (2016). Medicago sativa as a green corrosion inhibitor for 1018 carbon steel in 0.5 M H2SO4 solution. Green Chem. Lett. Rev. 9 (3), 143–155. doi:10.1080/17518253.2016.1195017
Sarver, E., and Edwards, M. (2012). Inhibition of copper pitting corrosion in aggressive potable waters. Int. J. Corros. 11, 1–16. doi:10.1155/2012/857823
Singh, A., Ansari, K. R., Chauhan, D. S., Quraishi, M. A., Lgaz, H., and Chung, I. (2020). Comprehensive investigation of steel corrosion inhibition at macro/micro level by ecofriendly green corrosion inhibitor in 15% HCl medium. J. Colloid Interface Sci. 15, 225–236. doi:10.1016/j.jcis.2019.10.040
Tan, B., Lan, W., Zhang, S., Deng, H., Qiang, Y., Fu, A., et al. (2022b). Passiflora edulia Sims leaves Extract as renewable and degradable inhibitor for copper in sulfuric acid solution. Colloids Surfaces A Physicochem. Eng. Aspects 645, 128892. doi:10.1016/j.colsurfa.2022.128892
Tan, B., Zhang, S., Cao, X., Fu, A., Guo, L., Marzouki, R., et al. (2022). Insight into the anti-corrosion performance of two food flavors as eco-friendly and ultra-high performance inhibitors for copper in sulfuric acid medium. J. Colloid Interface Sci. 609, 838–851. doi:10.1016/j.jcis.2021.11.085
Umoren, S. A., and Ebenso, E. E. (2007). The synergistic effect of polyacrylamide and iodide ions on the corrosion inhibition of mild steel in H2SO4. Mat. Chem. Phys. 106, 387–393. doi:10.1016/j.matchemphys.2007.06.018
Umoren, S. A., Solomon, M. M., Udosoro, I. I., and Udoh, A. P. (2010). Synergistic and antagonistic effects between halide ions and carboxymethyl cellulose for the corrosion inhibition of mild steel in sulphuric acid solution. Cellulose 17, 635–648. doi:10.1007/s10570-010-9409-7
Keywords: M. sativa, corrosion inhibition, electrochemical techniques, efficiency, KI
Citation: Rodríguez-Torres A, Valladares-Cisneros MG, Saldaña Heredia A and González-Rodríguez JG (2022) KI effects on corrosion inhibition for 1018 steel in acid media using Medicago sativa. Front. Chem. 10:1032522. doi: 10.3389/fchem.2022.1032522
Received: 30 August 2022; Accepted: 25 October 2022;
Published: 10 November 2022.
Edited by:
Edward Gillan, The University of Iowa, United StatesReviewed by:
Bochuan Tan, Chongqing University of Science and Technology, ChinaBrahim El Ibrahimi, Université Ibn Zohr, Morocco
Copyright © 2022 Rodríguez-Torres, Valladares-Cisneros, Saldaña Heredia and González-Rodríguez. This is an open-access article distributed under the terms of the Creative Commons Attribution License (CC BY). The use, distribution or reproduction in other forums is permitted, provided the original author(s) and the copyright owner(s) are credited and that the original publication in this journal is cited, in accordance with accepted academic practice. No use, distribution or reproduction is permitted which does not comply with these terms.
*Correspondence: A. Rodríguez-Torres, adrodriguez@upmh.edu.mx