Novel Implications of MicroRNAs, Long Non-coding RNAs and Circular RNAs in Drug Resistance of Esophageal Cancer
- 1Shandong Provincial Key Laboratory of Radiation Oncology, Cancer Research Center, Shandong Cancer Hospital and Institute, Shandong First Medical University and Shandong Academy of Medical Sciences, Jinan, China
- 2Department of Pathology, Shandong Cancer Hospital and Institute, Jinan, China
- 3Department of Radiation Oncology, Shandong Cancer Hospital and Institute, Jinan, China
- 4Department of Endoscopy, Shandong Cancer Hospital and Institute, Jinan, China
Esophageal cancer is the eighth most common malignancy and the sixth leading cause of cancer-related deaths worldwide. Chemotherapy based on platinum drugs, 5-fluorouracil, adriamycin, paclitaxel, gemcitabine, and vinorelbine, as well as targeted treatment and immunotherapy with immune checkpoint inhibitors improved the prognosis in a portion of patients with advanced esophageal cancer. Unfortunately, a number of esophageal cancer patients develop drug resistance, resulting in poor outcomes. Multiple mechanisms contributing to drug resistance of esophageal cancer have been reported. Notably, non-coding RNAs (ncRNAs), including microRNAs (miRNAs), long non-coding RNAs (lncRNAs) and circular RNAs (circRNAs), have been identified to play crucial roles in modulating esophageal cancer drug resistance. In the present review, we highlight the underlying mechanisms how miRNAs, lncRNAs, and circRNAs impact the drug resistance of esophageal cancer. Several miRNAs, lncRNAs, and circRNAs may have potential clinical implications as novel biomarkers and therapeutic targets for esophageal cancer.
Introduction
Esophageal cancer is a complex malignancy and the sixth leading cause of cancer death worldwide. A total of 572,034 esophageal cancer cases were diagnosed worldwide and 508,585 cases dead in 2018 (Bray et al., 2018). There are two major histological subtypes of esophageal cancer, esophageal adenocarcinoma (EAC) and esophageal squamous cell carcinoma (ESCC). EAC is prevalent in Western countries including North America and Western Europe; while ESCC is the major histologic type of esophageal cancer in Eastern Asia and Africa (Bray et al., 2018). In the past 15 years, the 5-year survival rate for patients with esophageal cancer for all stages combined is only 20%. However, patients with metastatic esophageal cancer have a poor prognosis, with the 5-year survival rate of approximately 5% (Bray et al., 2018; Siegel et al., 2020).
Although treatment options are limited for patients with unresectable, locally advanced, or metastatic esophageal cancer, a portion of patients could benefit from the comprehensive treatment of chemotherapy, targeted therapy and immunotherapy with immune checkpoint inhibitors (Eltweri et al., 2019; Kojima et al., 2020; Lopez et al., 2020; Stroes et al., 2020). The commonly used chemotherapeutic agents in clinics include platinum drugs, 5-fluorouracil (5-FU), adriamycin (ADM), paclitaxel (PTX), irinotecan, gemcitabine (GEM), and vinorelbine (Liu S. L. et al., 2015; Wang Y. S. et al., 2016; Tin et al., 2018; van Zweeden et al., 2018; Ni et al., 2020). Molecular targeted therapy drugs include anti-HER2 monoclonal antibodies (trastuzumab and pertuzumab), oral tyrosine kinase inhibitors (TKIs) targeting HER-1/HER-2 (lapatinib), anti-vascular endothelial growth factor receptor 2 (VEGFR-2) antibody (ramucirumab) and anti-EGFR monoclonal antibody (panitumumab) (Press et al., 2017; Shepard et al., 2017; Chakrabarti et al., 2018; Guo et al., 2018; Yamaguchi et al., 2018; De Vita et al., 2019; Hassan et al., 2019; Rogers et al., 2019; Wagner et al., 2019; Stroes et al., 2020). Immune-checkpoint blockade agents are also used, such as anti-programmed cell death protein 1 (PD-1) monoclonal antibodies (nivolumab and pembrolizumab) (Herbst et al., 2019; Shah et al., 2019; Kato et al., 2020; Rogers et al., 2020).
Unfortunately, esophageal cancer cells frequently develop multi-drug resistance (MDR) which seriously impaired the efficacy of drugs and subsequently led to poor prognosis. The underlying complicated mechanisms involved in drug resistance of esophageal cancer have been reported, such as the enhanced DNA damage repair capability, the up-regulated expression of drug efflux transporters to pump out chemo-agents from cells, the accelerated cell growth and autophagy flux, dysregulation of cell cycle, epithelial-mesenchymal transition (EMT), apoptosis inactivation as well as activation of cancer stem cells (CSCs) (Liu D. S. et al., 2015; Cheng et al., 2017; Zhou et al., 2017, 2020; Huang et al., 2018; Qiao et al., 2018; Guo et al., 2019; Lin C. H. et al., 2019).
Non-coding RNAs (ncRNAs) are a class of RNA transcripts without protein-coding ability, such as microRNAs (miRNAs), long non-coding RNAs (lncRNAs), and circular RNAs (circRNAs). Multiple miRNAs, lncRNAs, and circRNAs have been reported to be involved in controlling various cellular functions, such as apoptosis, cell growth, autophagy, EMT, and cell cycle regulation (Wang et al., 2015c; Bhan et al., 2017; Peng et al., 2017; Ren et al., 2017a, b; Rupaimoole and Slack, 2017; Pan et al., 2018; Vo et al., 2019; Chen et al., 2020; Yuan et al., 2020; Zhang et al., 2020a). Among these ncRNAs, several miRNAs, lncRNAs, and circRNAs are dysregulated in esophageal cancer and have been shown to be associated with tumorigenesis, metastasis, prognosis, as well as treatment resistance to radiotherapy and drugs (Song et al., 2014; Wang et al., 2015b; Wen et al., 2016; Zhang E. et al., 2017; Zhang et al., 2018; Sang et al., 2018). So far, there have been few reports of ncRNAs involvement in the resistance to targeted therapy and immunotherapy. Interestingly, serum miRNAs, including miR-1233-5p, miR-6885-5p, miR-4698, and miR-128-2-5p, have been identified to predict the response to nivolumab, a PD-1 inhibitor, in advanced ESCC patients (Sudo et al., 2020). Notably, a number of miRNAs, lncRNAs, and circRNAs have been shown to play crucial roles in esophageal cancer chemoresistance (Jin et al., 2016; Li et al., 2018; Lin K. et al., 2019; Wu et al., 2020; Zou et al., 2020). Considering the importance of ncRNAs in the development of drug resistance of esophageal cancer, we systematically summarized the underlined mechanisms of these miRNAs, lncRNAs, and circRNAs in the current review (Figure 1).
MicroRNAs and Drug Resistance
MicroRNAs are small, endogenous, single-stranded ncRNAs and function as crucial regulators of gene expression at the post-transcriptional level. MiRNAs down-regulate expression levels of target genes through binding to the 3′-untranslated region (3′-UTR) of target mRNA and leading to target mRNA degradation or blocking translation. It has been found that miRNAs can act as oncogenes or tumor suppressors to regulate cell differentiation, proliferation, apoptosis, metabolic reprogramming and angiogenesis (Bracken et al., 2016; Rupaimoole and Slack, 2017). Additionally, miRNAs could be released from esophageal cancer cells via exosomes and affect neighboring or distant cells. The exchange of the genetic information and/or regulation of target gene expression of miRNAs may change biological behaviors of recipient cells (Tanaka Y. et al., 2013; Luo et al., 2019; Gao et al., 2020). Multiple aberrantly expressed miRNAs have been identified in esophageal cancer, especially in the development of drug resistance (Hamano et al., 2011; Wang Y. et al., 2016; Liu et al., 2017; Zhang J. X. et al., 2017). Here, we summarized the roles of miRNAs in the resistance to platinum drugs, 5-FU and other agents in esophageal cancer.
MicroRNAs and Resistance to Platinum Drugs
Platinum drugs are the most commonly used antitumor drugs in clinic. In cells, platinum binds to genomic DNA to form platinum-DNA adducts, resulting in DNA replication and transcription disorders, and subsequently tumor cell death. Multiple platinum drugs have been applied in clinical managements of esophageal cancer, such as cisplatin (DDP, the first-generation platinum agent), carboplatin (the second-generation platinum agent), oxaliplatin, and loplatin (the third-generation platinum agents). However, response rates to platinum drugs are low in some esophageal cancer patients. Several miRNAs have been reported to participate in development of resistance to platinum drugs in esophageal cancer (Table 1).
There are many oncogenic miRNAs promoting the resistance of esophageal cancer to platinum drugs (Table 1). MiR-10b could enhance DDP resistance through silencing peroxisome proliferator-activated receptor-γ (PPARγ) and activating the AKT/mTOR/p70S6K signaling pathway (Wu et al., 2020). MiR-432-3p has been found to promote the resistance to DDP by directly suppressing expression of Kelch-like ECH-associated protein 1 (KEAP1) and stabilizing NF-E2-related factor 2 (NRF2). On the contrary, miR-432-3p knocking-off through the CRISPR/Cas9 technology could reverse DDP resistance of ESCC cells (Akdemir et al., 2017). As the most highly expressed miRNA in DDP-resistant ESCC cells, miR-141 could potentiate the resistance to DDP by directly silencing Yes-associated protein 1 (YAP1) (Imanaka et al., 2011). In addition, the expression of miR-200c was also found to be significantly up-regulated in DDP-resistant esophageal cancer cells compared to their parent cells. Mechanistically, miR-200c increases DDP resistance through modulating activity of the AKT pathway (Hamano et al., 2011). Among esophageal cancer patients, miR-200c levels were markedly correlated with response to chemotherapy. That is, high levels of miR-200c in patient serum were significantly correlated with poor response to neoadjuvant chemotherapy treated with DDP, 5-FU and adriamycin (ACF) (Tanaka K. et al., 2013). Ectopic miR-21 in ESCC cells has been found to promote DDP resistance (Komatsu et al., 2016a). Moreover, the levels of miR-21 and miR-23a in pre-operative plasma of ESCC patients might be used to predict the resistance to pre-operative chemotherapy regimens with DDP plus 5-FU (Komatsu et al., 2016a, b). Consistently, exosome-derived oncogenic miR-21 has also been shown to weaken DDP sensitivity of esophageal cancer cells by silencing programmed cell death 4 (PDCD4) (Yang et al., 2019). Interestingly, miR-27a/b may confer DDP resistance through transforming normal fibroblast into cancer-associated fibroblasts (CAF). Although ectopic miR-27a/b could not significantly impair chemosensitivity of esophageal cancer cells, the supernatant originating from miR-27a/b-transfected CAFs has been shown to promote DDP resistance in esophageal cancer cells, compared with supernatant deriving from normal fibroblast. Moreover, the resistance to DDP could be overcame after adding neutralized antibody against transforming growth factor-β (TGF-β) to the supernatant (Tanaka et al., 2015). MiR-483 and miR-214 were dramatically up-regulated in ESCC tissues compared with those in normal tissues and could confer DDP resistance in ESCC cells (Zhou and Hong, 2013). Oncogenic miR-223 has been found to diminish DNA repair and apoptosis potentials of esophageal cancer cells and increase the resistance to DDP via targeting and down-regulating Poly (ADP-ribose) polymerase 1 (PARP1) (Streppel et al., 2013). MiR-196a and miR-296 could promote DDP resistance via promoting the expression of cell membrane transporter ATP binding cassette subfamily G member 2 (ABCG2) and P-glycoprotein (P-gp) (Hong et al., 2010; Ma et al., 2016). Oncogenic miR-455-3p could increase the subpopulations of CD90+ and CD271+ CSCs/tumor-initiating cells (T-ICs) through activating the Wnt/β-catenin signaling and the TGF-β signaling, which leads to resistance of ESCC cells to DDP (Liu et al., 2017). MiR-193, a highly expressed miRNA in DDP-resistant esophageal cancer cell exosomes (TE-1/DDP/exo), has been shown to promote DDP resistance by targeting transcription factor AP-2 gamma (TFAP2C). Moreover, level of high miR-193 or low TFAP2C could suppress apoptosis and abate cell cycle inhibition (Shi et al., 2020). Most recently, miR-106b-3p, an overexpressed miRNA in ESCC tissues, has also been demonstrated to confer the resistance to DDP by targeting glutamine γ-glutamyltransferase E (TGM3) in esophageal cancer cells (Zhu Y. et al., 2021). In addition, oncogenic miR-141-3p is highly expressed in oxaliplatin-resistant esophageal cancer cells and has been found to enhance resistance by silencing phosphatase and tensin homolog (PTEN), in vitro and in vivo (Jin et al., 2016).
By contrast, several tumor suppressor miRNAs can reverse resistance of esophageal cancer to platinum drugs (Table 1). Ectopic miR-544 and miR-338-5p could overcome DDP resistance of esophageal cancer via targeting and down-regulating oncogene E2F transcription factor 5 (E2F5) and fermitin family homolog 2 (FERMT2) (Lin W. C. et al., 2019; Sun et al., 2019). Similarly, ectopic miR-125a-5p could potentiate the cytotoxic and apoptotic effects of DDP on esophageal cancer cells through modulating the signal transducer and activator of transcription 3 (STAT3) signaling pathway (Zhao et al., 2018). Tumor suppressor miR-218 could reverse DDP resistance and promote apoptosis of esophageal cancer cells by silencing oncogene survivin (Jingjing et al., 2016). Interestingly, miR-218 could inhibit cell proliferation, promote cell apoptosis, induce cell cycle arrested in G0/G1 phase, as well as increase DDP sensitivity of esophageal cancer cells through suppressing phosphorylation of PI3K, AKT, and mTOR (Tian et al., 2015). MiR-214-3p, a highly down-regulated miRNA in ESCC cells, could weaken DDP resistance by targeting and down-regulating both survivin and RNA-binding protein (RBP) CUG-BP1 (Phatak et al., 2016). Tumor suppressor miR-499 have also been found to reverse the DDP resistance of esophageal cancer cells by silencing DNA polymerase β (polβ) (Wang et al., 2015d). Through suppressing the IL-6/STAT3 pathway and drug transporter ABCC10, let-7, and let-7g/i could restore the sensitivity to DDP and oxaliplatin and promote apoptosis of esophageal cancer cells (Sugimura et al., 2012; Wu et al., 2016). Tumor suppressor miR-634 could enhance the cytotoxicity induced by DDP via concurrently targeting multiple genes which were linked with anti-apoptosis, mitochondrial homeostasis, autophagy and antioxidant ability. Specifically, anti-apoptotic genes include APAF1 interacting protein (APIP), baculoviral IAP repeat containing 5 (BIRC5), and E3 ubiquitin protein ligase X-linked inhibitor of apoptosis (XIAP); mitochondrial homeostasis genes involve transcription factor A, mitochondrial (TFAM) and optic atrophy 1 (OPA1); autophagy and antioxidant genes refer to lysosomal-associated membrane protein 2 (LAMP2) and NRF2 (NFE2L2; nuclear factor, erythroid 2-like 2) (Fujiwara et al., 2015). MiR-187 was significantly down-regulated in pre-treatment tumors of EAC patients with worse response to neoadjuvant chemoradiation therapy. Mechanistically, miR-187 could reverse the resistance to DDP and X-ray irradiation in EAC cells by modulating multiple signaling pathways, including the complement component 3 (C3) signaling (Winther et al., 2016). Through silencing Bcl-2, miR-130a-3p could sensitize esophageal cancer cells to DDP (Lindner et al., 2018). By suppressing the PI3K/AKT pathway and expression of MDR-associated proteins MRP1 and P-gp, tumor suppressor miR-145 could sensitize ESCC to DDP and promote DDP-induced apoptosis and cell cycle arrest (Zheng et al., 2019). Most recently, miR-181a-5p, a down-regulated miRNA in DDP-resistant EAC cell line (OE19/DDP), has also been demonstrated to reverse the resistance to DDP in EAC by modulating CBLB. Moreover, ectopic expression of miR-181a-5p could potentiate the in vivo sensitivity to DDP in EAC (Yang S. et al., 2020). Additionally, tumor suppressor miR-153-3p could also potentiate the sensitivity of EC cells to DDP via Nrf-2 (Zuo et al., 2020).
MicroRNAs and 5-Fluorouracil Resistance
5-fluorouracil is a heterocyclic aromatic chemotherapeutic agent which is broadly utilized in esophageal cancer treatments. 5-FU inhibits thymidylate synthase (TS), hampers DNA replication, and subsequently resulting in arrested cell cycle and apoptosis (Longley et al., 2003; Subbarayan et al., 2010). It has been reported that several oncogenic or tumor suppressive miRNAs are involved in 5-FU resistance (Table 2).
Multiple oncogenic miRNAs could promote 5-FU resistance of esophageal cancer cells. Oncogenic miR-141-3p can confer 5-FU resistance by silencing PTEN and the elevated levels of miR-141-3p was associated with TNM stage and differentiation status of ESCC patients (Jin et al., 2016). MiR-221 was overexpressed in 5-FU resistant esophageal cancer cells and EAC tissue and could potentiate 5-FU resistance by directly down-regulating the expression of dickkopf Wnt signaling pathway inhibitor 2 (DKK2) and activating the Wnt/β-catenin-EMT pathways (Wang Y. et al., 2016). In 5-FU resistant esophageal cancer cells, miR-27b-3p and miR-193b-3p have been found to be significantly up-regulated. Ectopic miR-27b-3p and miR-193b-3p could promote 5-FU resistance though silencing expression of their target gene KRAS (Hummel et al., 2014). MiR-296 has been found to contribute to 5-FU resistance in esophageal cancer cells through modulating the expression of P-gp, Bcl-2, Bax, cyclin D1 and P27 (Hong et al., 2010). In addition, oncogenic miR-21, miR-214, and miR-483 could also promote 5-FU resistance of ESCC cells (Zhou and Hong, 2013; Komatsu et al., 2016a). By targeting presenilin-1 (PSEN1), miR-193a-3p could also confer 5-FU resistance of esophageal cancer cells (Meng et al., 2016).
On the contrary, a number of tumor suppressor miRNAs can reverse 5-FU resistance of esophageal cancer cells. MiR-193b was highly expressed in chemosensitive esophageal cancer cells. MiR-193b has been shown to significantly promote the sensitivity to 5-FU in KYSE450 cells by silencing stathmin 1, which leads to activation of the autophagic flux and non-apoptotic cell death (Nyhan et al., 2016). Additionally, tumor suppressor miR-634 have also been found to be involved in development of 5-FU resistance by directly targeting a number of mitochondrial apoptosis pathway genes, such as OPA1, TFAM, LAMP2, APIP, XIAP, BIRC5, and NRF2 (Fujiwara et al., 2015). Several dysregulated miRNAs, including miR-192-5p, miR-378a-3p, miR-194-5p, miR-18a-3p, and miR-125a-5p, have been identified to be down-regulated in 5-FU resistant esophageal cancer cells. Ectopic miR-192-5p, miR-378a-3p, miR-194-5p, miR-18a-3p, and miR-125a-5p could reverse 5-FU resistance through silencing the expression of their target genes thymidylate synthase (TYMS), CBL-B, ABCC3, KRAS, and ERBB2 (Hummel et al., 2014). In ESCC cells treated with 5-FU, miR-145 has been found to obviously enhance apoptosis and expression of Bax, Bcl-2, and caspase3, via down-regulating REV3L (Chen Q. et al., 2019). MiR-29c was down-regulated in tumor tissues and serum samples of ESCC patients and has also been found to reverse 5-FU resistance by silencing F-box only protein 31 (FBXO31) (Li et al., 2019). Tumor suppressor miR-338-5p was down-regulated in 5-FU resistant ESCC cells as well as sera and tumor tissue of ESCC patients. Low miR-338-5p levels in serum was associated with poor response to neoadjuvant chemoradiotherapy based on 5-FU/DDP and worse survival of ESCC patients. Mechanistically, miR-338-5p could restore 5-FU sensitivity of ESCC cells by silencing the gene expression of inhibitor of differentiation 1 (Id-1) in vitro and in vivo (Han et al., 2019).
MicroRNAs and Resistance to Other Drugs
In clinics, adriamycin, vincristine, paclitaxel, gemcitabine, and vinorelbine are also commonly used in esophageal cancer therapy. Multiple miRNAs have been shown to participate in their resistance (Table 3). MiR-27a could confer adriamycin resistance and inhibit the apoptosis induced by adriamycin. It has been found that miR-27a could increase the expression of P-gp and Bcl-2, as well as reduce Bax expression in esophageal cancer cells (Zhang et al., 2010). Besides promoting the resistance to DDP and 5-FU, miR-483 and miR-214 could also potentiate adriamycin resistance and reduce intracellular accumulation of adriamycin in esophageal cancer cells (Zhou and Hong, 2013). MiR-223 has also been found to confer adriamycin resistance through inhibiting PARP levels (Streppel et al., 2013). Oncogenic miR-296 could confer the resistance to adriamycin and vincristine through silencing gene expression controlling apoptosis and cell cycle (Hong et al., 2010). For paclitaxel resistance, it has been reported that the combined miR-133a and miR-133b down-regulation could predict the sensitivity to paclitaxel-based chemotherapy in ESCC patients (Chen et al., 2014). Interestingly, esophageal cancer patients with low expression of miR-214 appeared to show higher sensitivity to the combination regimen of gemcitabine plus vinorelbine, indicating that miR-214 may predict esophageal cancer chemosensitivity (Wang Y. S. et al., 2016). In addition, miR-193a-3p has been found to potentiate the chemoresistance to docetaxel, paclitaxel and vinorelbine in esophageal cancer cells via silencing PSEN1 (Meng et al., 2016).
Long Non-Coding RNAs and Drug Resistance
Long non-coding RNAs are a group of ncRNAs longer than 200 nt without protein-coding capacity. Accumulating evidences showed that lncRNAs play important roles in regulating various cellular processes (Frye et al., 2016; Kopp and Mendell, 2018). For instance, lncRNAs could regulate target gene expression at either the transcriptional level or the post-transcriptional level through interaction with various DNA, RNA or proteins. Abnormally expressed lncRNAs have been identified in almost all cancer types, including esophageal cancer (Li J. et al., 2014; Yang et al., 2014; Tan et al., 2017; Xu et al., 2019) and have been implicated in diagnosis, metastasis, prognosis, radioresistance, and chemoresistance of esophageal cancer (Li W. et al., 2014; Wang et al., 2015a, 2017; Lin et al., 2018; You et al., 2019; Zhang H. et al., 2019; Liu J. et al., 2020). Importantly, several lncRNAs have been found to contribute to development of drug resistance in esophageal cancer.
Long Non-coding RNAs and Resistance to Platinum Drugs
It has been found that multiple lncRNAs were involved in the resistance to platinum drugs in esophageal cancer (Table 4), including oncogenic lncRNAs NSUN2 methylated lncRNA (NMR), colon cancer-associated transcript-1 (CCAT1), taurine up-regulated gene 1 (TUG1), TP73-AS1, prostate cancer associated ncRNA transcript 1 (PCAT-1), AFAP1-AS1, FOXD2-AS1, POU3F3, LINC00337, LINC00152, and tumor suppressive lncRNA tumor suppressor candidate 7 (TUSC7).
Oncogenic lncRNA NMR (namely ENST00000432429.1 in GENCODE v13 or ENST00000432429.5 in Ensembl release 83), highly methylated by methyltransferase NSUN2 which can catalyze cytosine methylation to 5-methylcytosine (m5C) in tRNA and some poly(A) RNAs, has been found to play crucial roles in regulating DDP resistance and metastasis of ESCC cells (Li et al., 2018). LncRNA NMR was evidently up-regulated in ESCC and associated with poor overall survival (OS) of ESCC patients (Li et al., 2018). Functionally, ectopic expression of lncRNA NMR could not only suppress DDP-induced apoptosis, but also promote invasion and migration of ESCC cells. Mechanistically, it has been shown that lncRNA NMR could competitively suppress potential mRNAs m5C levels, such as procollagen-lysine, 2-oxoglutarate 5-dioxygenase 3 (PLOD3), collagen type IV Alpha 5 (COL4A5), laminin beta 1 (LAMB1), and heparan sulfate proteoglycan 2 (HSPG2). Moreover, lncRNA NMR directly bond to chromatin regulator of bromodomain PHD finger transcription factor (BPTF), and regulated the expression of matrix metallopeptidase 3 (MMP3) and matrix metallopeptidase 10 (MMP10) through the ERK1/2 pathway (Li et al., 2018).
Long non-coding RNA CCAT1, which is highly expressed in esophageal cancer, has also been found to confer DDP resistance in ESCC cells through the miR-143/PLK1/BUBR1 signaling axis (Hu M. et al., 2019). Specifically, silencing of CCAT1 could dramatically potentiate miR-143 expression in a negative regulatory manner and inhibit both mRNA and protein expression of Polo-like kinase 1 (PLK1) and BUBR. Moreover, ectopic expression of miR-143 has been shown to suppress the expression of PLK1, BUBR1, and CCAT1. Functionally, silencing of lncRNA CCAT1 and ectopic miR-143 could reverse DDP drug resistance and inhibit ESCC cell proliferation. Inhibition of lncRNA CCAT1 has also been found to enhance sensitivity of ESCC xenografts in nude mice to DDP, indicating that lncRNA CCAT1 may act as a potential regulator of DDP chemoresistance in esophageal cancer (Hu M. et al., 2019).
Long non-coding RNA TUG1 has also been found to be abundantly expressed in TE-1-derived DDP-resistant esophageal cancer cells TE-1/DDP (Zhang Z. et al., 2019). Mechanistically, lncRNA TUG1 could confer DDP resistance of ESCC cells through elevating P-gp expression and inhibiting apoptosis. Conversely, silencing of lncRNA TUG1 reversed DDP resistance of ESCC cells (Zhang Z. et al., 2019). RNA immunoprecipitation and RNA pull-down assays verified that TUG1 could directly bind the protein of nuclear factor (erythroid-derived 2)-like 2 (Nrf2) and increase Nrf2 protein level. Moreover, Nrf2 antibody could relieve DDP resistance mediated by TUG1 overexpression in ESCC cell, indicating an involvement of TUG1/Nrf2 signaling pathway in DDP resistance (Zhang Z. et al., 2019).
Long non-coding RNAs TP73-AS1 also could promote DDP resistance of esophageal cancer cells (Zang et al., 2016). LncRNA PCAT-1 has been shown to accelerate DDP resistance and tumor growth of esophageal cancer cells (Zhen et al., 2018). Additionally, AFAP1-AS1, a dramatically up-regulated lncRNA in esophageal cancer tissues and DDP-resistant esophageal cancer cells, has been found to be positively associated with not only advanced clinical stages and definitive chemoradiotherapy (dCRT) response, but also shorter OS and progression free survival (PFS) (Zhou et al., 2016). Oncogenic lncRNAs FOXD2-AS1, POU3F3, and LINC00337 were revealed to be involved in DDP resistance of esophageal cancer (Liu H. et al., 2020; Tong et al., 2020; Yang C. et al., 2020). Via the miR-195/Akt/mTOR axis, ectopic expression of FOXD2-AS1, an up-regulated lncRNA in ESCC patients and DDP resistant ESCC cells (TE-1/DDP), could contribute to DDP resistance in ESCC (Liu H. et al., 2020). LncRNA POU3F3 could confer DDP resistance of ESCC cells through exosome POU3F3 inducing normal fibroblasts (NFs) to differentiate into CAFs via secreting interleukin 6 (IL-6). In addition, higher expression of plasma exosome POU3F3 has been shown to predict bad complete response and survival of ESCC patients (Tong et al., 2020). By increasing ESCC cell autophagy, exogenous expression of LINC00337 has been demonstrated to potentially promote DDP resistance through TPX2 up-regulation via recruiting E2F4 (Yang C. et al., 2020). Additionally, through interacting with EZH2, oncogenic LINC00152 has been found to increase ZEB1 expression and accelerate EMT and oxaliplatin resistance in esophageal cancer (Zhang et al., 2020b).
On the contrary, tumor suppressor lncRNA may reverse the resistance of cancer cells to platinum drugs. For instance, lncRNA TUSC7 could overcome the resistance to DDP and promote apoptosis of ESCC cells, via inhibiting miR-224 to modulate differentially expressed in squamous cell carcinoma 1 (DESC1)/EGFR/AKT signaling pathway. Overexpression of DESC1 could reverse the resistance to DDP through EGFR/AKT pathway in ESCC EC9706 and KYSE30 cells. Moreover, esophageal cancer patients with lower lncRNA TUSC7 expression had short OS (Chang et al., 2018).
Long Non-coding RNAs and 5-Fluorouracil Resistance
Several oncogenic or tumor suppressive lncRNAs are associated with the resistance to 5-FU in esophageal cancer (Table 5). Oncogenic LINC01419 has been found to promote 5-FU resistance and inhibit apoptosis of ESCC cells (Chen J. L. et al., 2019). LINC01419 could bind to the promoter region of glutathione S-transferase pi 1 (GSTP1) gene, increase DNA methylation levels of the region through recruiting DNA methyltransferase 1 (DNMT1), DNA methyltransferase 3A (DNMT3A) and DNA methyltransferase 3B (DNMT3B) into GSTP1 promoter region and diminish GSTP1 expression in ESCC cells (Chen J. L. et al., 2019). On the contrary, the demethylation of GSTP1 via DNA methyltransferase inhibitor 5-Aza-CdR could weaken 5-FU resistance in LINC01419 overexpressed ESCC cells, demonstrating that LINC01419 functions as a modulator of 5-FU-based chemotherapy sensitivity in ESCC (Chen J. L. et al., 2019). Recently, oncogenic lncRNA HOTAIR has also been found to accelerate 5-FU resistance in esophageal cancer cells by promoting the promoter hypermethylation of methylene tetrahydrofolate reductase (MTHFR) gene. Silencing of HOTAIR could promote the apoptosis induced by 5-FU and alleviate cell proliferation and MTHFR promoter methylation of esophageal cancer cells. Moreover, overexpression of MTHFR has been shown to reverse 5-FU resistance caused by HOTAIR overexpression. Meanwhile, xenografts from HOTAIR-silenced esophageal cancer cells in nude mice also demonstrated the diminished 5-FU resistance, indicating that HOTAIR may represent a novel potential target for conquering 5-FU resistance of esophageal cancer (Zhang et al., 2020c). In addition, oncogenic lncRNA LINC01270 has also been shown to promote the resistance to 5-FU through regulating GSTP1 promoter methylation via recruiting three important DNA methyltransferases, including DNMT3A, DNMT3B, and DNMT1 (Li et al., 2021).
By contrast, tumor suppressor lncRNAs play opposite role in development of 5-FU resistance of esophageal cancer. For instance, through suppressing miR-224 and regulating the ESC1/EGFR/AKT signaling, lncRNA TUSC7 has been demonstrated to conquer 5-FU resistance and increase the apoptosis of ESCC cells. Moreover, exogenous expression of DESC1 could enhance the sensitivity to 5-FU in ESCC cells (Chang et al., 2018). In addition, tumor suppressor lncRNA LINC00261 could also reverse the chemoresistance to 5-FU in human esophageal cancer cells through regulating DNA methylation-dependent expression inhibition of dihydropyrimidine dehydrogenase (DYPD). Exogenous expression of LINC00261 could significantly suppress cell growth and potentiate apoptosis sensitivity to 5-FU in ESCC cells. On the contrary, inhibition of LINC00261 has been shown to promote proliferation and apoptosis resistance of ESCC cells. Moreover, 5-aza-2′-deoxycytidine, a demethylation reagent, could reverse DNA methylation of DYPD promoter and DYPD activity in 5-FU resistant ESCC cells (Lin K. et al., 2019).
Long Non-coding RNAs and Resistance to Other Drugs
Paclitaxel, adriamycin, and gefitinib are also used in esophageal cancer treatments. It has been found that multiple oncogenic lncRNAs participate in the resistance to these anti-cancer agents (Table 6). LncRNA DDX11-AS1, a highly expressed lncRNA in esophageal cancer tissues, has been found to increase paclitaxel resistance of esophageal cancer cells. Through binding to transcription factor TATA-box binding protein-associated factor 1 (TAF1) and up-regulating TAF1 expression, lncRNA DDX11-AS1 could promote the transcription of topoisomerase alpha 2 (TOP2A) and subsequently, increase TOP2A expression levels (Zhang S. et al., 2019). Silencing of lncRNA DDX11-AS1 could potentiate the inhibitory effects of paclitaxel on esophageal cancer xenografts in nude mice and suppress TOP2A expression, suggesting that lncRNA DDX11-AS1 may be a promising potential target for overcoming paclitaxel resistance of esophageal cancer (Zhang S. et al., 2019). LncRNA VLDLR was up-regulated in ESCC tissue and could promote adriamycin resistance of esophageal cancer cells via increasing ABCG2 expression (Chen Y. et al., 2019). LncRNA prostate androgen-regulated transcript 1 (PART1) has been found to confer the resistance to gefitinib, an oral epidermal growth factor receptor tyrosine kinase inhibitor (EGFR-TKI), through regulating miR-129/Bcl-2 pathway in ESCC cells. Interestingly, extracellular lncRNA PART1 could be secreted with exosomes, transferred to the sensitive ESCC cells, and promoted gefitinib resistance of ESCC cells. In addition, high expression of serum lncRNA PART1 in exosome was also associated with unfavorable response to gefitinib in ESCC patients (Kang et al., 2018). Through modulating PI3K-AKT-mTOR signaling, Linc01014 overexpression could also dramatically suppress the apoptosis of esophagus cancer cells and promote gefitinib resistance (Fu et al., 2020).
Circular RNAs and Drug Resistance in Esophageal Cancer
Circular RNAs, a special type of endogenous circular ncRNAs, are generated through the process called back-splicing of linear precursor mRNA (pre-mRNA) transcripts and lack 3′ poly (A) tail and 5′ cap (Chen, 2016). CircRNAs could regulate gene expression through sponge adsorption of miRNA, modification of parental genes, and regulation of transcription and splicing of target genes. Amounting evidence has demonstrated that circRNAs are involved in multiple cellular processes and several malignancies, including esophageal cancer (Hu X. et al., 2019; Kristensen et al., 2019). Interestingly, circRNAs could not only serve as diagnostic and prognosis markers of esophageal cancer, but also participate in drug resistance (Zhang et al., 2020d; Zou et al., 2020). For instance, circRNA_001275, an up-regulated circRNA in DDP-resistant esophageal cancer cells and tissues, has been shown to accelerate cell growth and reduce the apoptosis of DDP-resistant cells. On the contrary, knockdown of circRNA_001275 inhibited the proliferation of DDP-resistant cells. It has been found that circRNA_001275 could contribute to DDP resistance in esophageal cancer through directly binding to and competitively sponging miR-370-3p to up-regulate Wnt family member 7A (Wnt7a) expression (Zou et al., 2020). In addition, via regulating miR-194-5p/JMJD1C axis, oncogenic circ_0006168 has been shown to potentiate Taxol resistance in ESCC (Qu et al., 2021). Recently, tumor suppressive circPSMC3, down-regulated in ESCC tissues and gefitinib-resistant (GR) ESCC cells, its overexpression could conquer gefitinib resistance, increase apoptosis rate and cleaved caspase-3 level in GR ESCC cells through modulating the miR-10a-5p/PTEN axis, which provide a promising therapeutic strategy for overcoming gefitinib resistance in ESCC (Zhu H. et al., 2021).
Conclusion
Accumulating evidences have shown that ncRNAs significantly contribute to drug resistance of esophageal cancer. The corresponding mechanisms of miRNAs, lncRNAs, and circRNAs involved in drug resistance of esophageal cancer are illustrated in Figure 2. Multiple mechanisms including abnormal histone and DNA modifications, genomic amplification/loss and post-transcriptional regulations are involved in the dysregulation of 3 kinds of ncRNAs in esophageal cancer. Personalized therapy according to abnormally expressed miRNAs, lncRNAs, and circRNAs may be a promising way to overcome drug resistance. Silencing of oncogenic ncRNAs using small interfering RNAs (siRNAs) or short hairpin RNAs (shRNAs) have been reported to be effective in restoring the therapeutic sensitivity of esophageal cancer (Hu X. et al., 2019). Alternatively, ectopic expression of tumor suppressor ncRNAs have been demonstrated to be beneficial to conquer therapeutic resistance in esophageal cancer. Locked nucleic acid (LNA) modifications of ncRNAs can enhance in vivo stability and affinity. However, drug safety, immune-related toxicities or other adverse effects remain important issues to be solved for ncRNAs-based therapeutics. After searching the http://clinicaltrials.gov database, we found that there are still no therapeutic clinical trials based on ncRNAs in esophageal cancer currently. Due to the complexities of cancer signaling pathways, the inhibition of a single target signaling or ncRNA may show minor effects. The combination based on modulation of ncRNAs expression and classical chemotherapy, novel targeted therapy or immunotherapy may be a promising choice to treat advanced or metastatic esophageal cancer patients. However, selecting key target ncRNA from numerous candidate ncRNAs for the intervention remains a difficult issue.
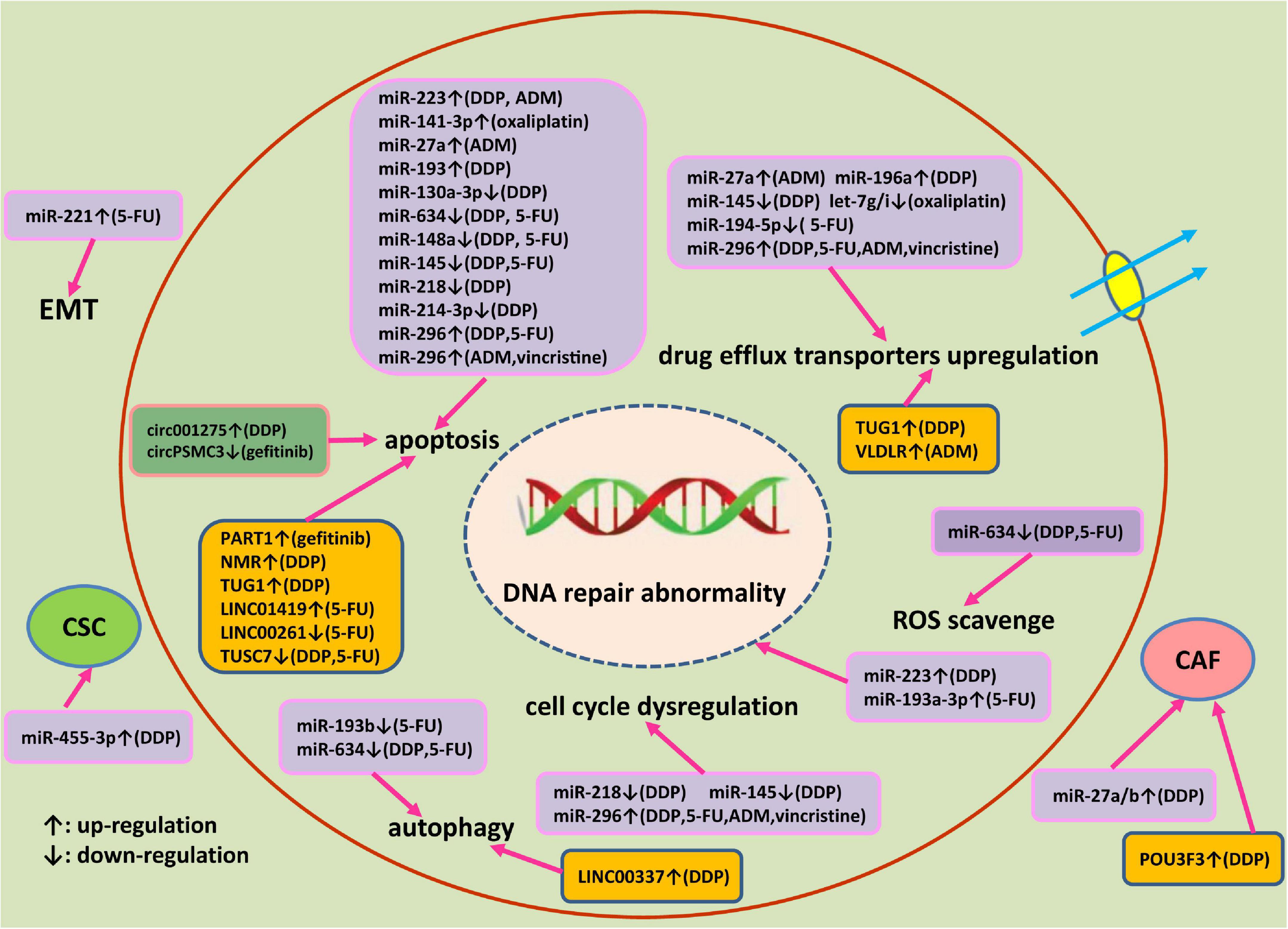
Figure 2. An outlined diagram of miRNAs, lncRNAs, and circRNA involved in the drug resistance of esophageal cancer. Multiple miRNAs, lncRNAs, and circRNA have been found to be linked to the drug resistance of esophageal cancer through altering cell proliferation, apoptosis, DNA damage repair, cell cycle progression, autophagy, cancer stem cell, and epithelial-mesenchymal transition via modulating corresponding target genes and signaling pathway.
Author Contributions
LW and MY conceived the review, acquired data, provided project funding, and drafted the manuscript. MY and ZL reviewed and supervised the manuscript. JS, NZ, YS, and TW undertook the initial research. All authors read and approved the submitted version.
Funding
This work was financially supported by the Natural Science Foundation of Shandong Province (ZR2020MH235), National Natural Science Foundation of China (82173070, 31871306, and 82103291), Program of Science and Technology for the youth innovation team in Universities of Shandong Province (2020KJL001), Taishan Scholars Program of Shandong Province (tsqn20161060), National Key R&D Program of China (2018YFC0114707), and Key R&D program of Shandong Province (2018GSF118047).
Conflict of Interest
The authors declare that the research was conducted in the absence of any commercial or financial relationships that could be construed as a potential conflict of interest.
Publisher’s Note
All claims expressed in this article are solely those of the authors and do not necessarily represent those of their affiliated organizations, or those of the publisher, the editors and the reviewers. Any product that may be evaluated in this article, or claim that may be made by its manufacturer, is not guaranteed or endorsed by the publisher.
References
Akdemir, B., Nakajima, Y., Inazawa, J., and Inoue, J. (2017). miR-432 induces NRF2 stabilization by directly targeting KEAP1. Mol. Cancer Res. 15, 1570–1578. doi: 10.1158/1541-7786.MCR-17-0232
Bhan, A., Soleimani, M., and Mandal, S. S. (2017). Long noncoding RNA and cancer: a new paradigm. Cancer Res. 77, 3965–3981. doi: 10.1158/0008-5472.CAN-16-2634
Bracken, C. P., Scott, H. S., and Goodall, G. J. (2016). A network-biology perspective of microRNA function and dysfunction in cancer. Nat. Rev. Genet. 17, 719–732. doi: 10.1038/nrg.2016.134
Bray, F., Ferlay, J., Soerjomataram, I., Siegel, R. L., Torre, L. A., and Jemal, A. (2018). Global cancer statistics 2018: GLOBOCAN estimates of incidence and mortality worldwide for 36 cancers in 185 countries. CA Cancer J. Clin. 68, 394–424. doi: 10.3322/caac.21492
Chakrabarti, S., Dong, H., Paripati, H. R., Ross, H. J., and Yoon, H. H. (2018). First report of dramatic tumor responses with ramucirumab and paclitaxel after progression on pembrolizumab in two cases of metastatic gastroesophageal adenocarcinoma. Oncologist 23, 840–843. doi: 10.1634/theoncologist.2017-0561
Chang, Z. W., Jia, Y. X., Zhang, W. J., Song, L. J., Gao, M., Li, M. J., et al. (2018). LncRNA-TUSC7/miR-224 affected chemotherapy resistance of esophageal squamous cell carcinoma by competitively regulating DESC1. J. Exp. Clin. Cancer Res. 37:56. doi: 10.1186/s13046-018-0724-4
Chen, G., Peng, J., Zhu, W., Tao, G., Song, Y., Zhou, X., et al. (2014). Combined downregulation of microRNA-133a and microRNA-133b predicts chemosensitivity of patients with esophageal squamous cell carcinoma undergoing paclitaxel-based chemotherapy. Med. Oncol. 31:263. doi: 10.1007/s12032-014-0263-6
Chen, J. L., Lin, Z. X., Qin, Y. S., She, Y. Q., Chen, Y., Chen, C., et al. (2019). Overexpression of long noncoding RNA LINC01419 in esophageal squamous cell carcinoma and its relation to the sensitivity to 5-fluorouracil by mediating GSTP1 methylation. Ther. Adv. Med. Oncol. 11:1758835919838958. doi: 10.1177/1758835919838958
Chen, L. L. (2016). The biogenesis and emerging roles of circular RNAs. Nat. Rev. Mol. Cell Biol. 17, 205–211. doi: 10.1038/nrm.2015.32
Chen, Q., Hou, J., Wu, Z., Zhao, J., and Ma, D. (2019). miR-145 Regulates the sensitivity of esophageal squamous cell carcinoma cells to 5-FU via targeting REV3L. Pathol. Res. Pract. 215:152427. doi: 10.1016/j.prp.2019.04.019
Chen, Y., Liu, L., Li, J., Du, Y., Wang, J., and Liu, J. (2019). Effects of long noncoding RNA (linc-VLDLR) existing in extracellular vesicles on the occurrence and multidrug resistance of esophageal cancer cells. Pathol. Res. Pract. 215, 470–477. doi: 10.1016/j.prp.2018.12.033
Chen, Y., Lu, Y., Ren, Y., Yuan, J., Zhang, N., Kimball, H., et al. (2020). Starvation-induced suppression of DAZAP1 by miR-10b integrates splicing control into TSC2-regulated oncogenic autophagy in esophageal squamous cell carcinoma. Theranostics 10, 4983–4996. doi: 10.7150/thno.43046
Cheng, C. Y., Liu, J. C., Wang, J. J., Li, Y. H., Pan, J., and Zhang, Y. R. (2017). Autophagy inhibition increased the anti-tumor effect of cisplatin on drug-resistant esophageal cancer cells. J. Biol. Regul. Homeost. Agents 31, 645–652.
De Vita, F., Borg, C., Farina, G., Geva, R., Carton, I., Cuku, H., et al. (2019). Ramucirumab and paclitaxel in patients with gastric cancer and prior trastuzumab: subgroup analysis from RAINBOW study. Future Oncol. 15, 2723–2731. doi: 10.2217/fon-2019-0243
Eltweri, A. M., Thomas, A. L., Chung, W. Y., Morgan, B., Thompson, J., Dennison, A. R., et al. (2019). The effect of supplementary omegaven(R) on the clinical outcome of patients with advanced esophagogastric adenocarcinoma receiving palliative epirubicin, oxaliplatin, and capecitabine chemotherapy: a phase II clinical trial. Anticancer Res. 39, 853–861. doi: 10.21873/anticanres.13185
Frye, M., Jaffrey, S. R., Pan, T., Rechavi, G., and Suzuki, T. (2016). RNA modifications: what have we learned and where are we headed? Nat. Rev. Genet. 17, 365–372. doi: 10.1038/nrg.2016.47
Fu, X., Cui, G., Liu, S., and Zhao, S. (2020). Linc01014 regulates gefitinib resistance in oesophagus cancer via EGFR-PI3K-AKT-mTOR signalling pathway. J. Cell Mol. Med. 24, 1670–1675. doi: 10.1111/jcmm.14860
Fujiwara, N., Inoue, J., Kawano, T., Tanimoto, K., Kozaki, K., and Inazawa, J. (2015). miR-634 activates the mitochondrial apoptosis pathway and enhances chemotherapy-induced cytotoxicity. Cancer Res. 75, 3890–3901. doi: 10.1158/0008-5472.CAN-15-0257
Gao, P., Wang, D., Liu, M., Chen, S., Yang, Z., Zhang, J., et al. (2020). DNA methylation-mediated repression of exosomal miR-652-5p expression promotes oesophageal squamous cell carcinoma aggressiveness by targeting PARG and VEGF pathways. PLoS Genet. 16:e1008592. doi: 10.1371/journal.pgen.1008592
Guo, X. F., Li, S. S., Zhu, X. F., Dou, Q. H., and Liu, D. (2018). Lapatinib in combination with paclitaxel plays synergistic antitumor effects on esophageal squamous cancer. Cancer Chemother. Pharmacol. 82, 383–394. doi: 10.1007/s00280-018-3627-3
Guo, Y., Jia, Y., Wang, S., Liu, N., Gao, D., Zhang, L., et al. (2019). Downregulation of MUTYH contributes to cisplatinresistance of esophageal squamous cell carcinoma cells by promoting Twistmediated EMT. Oncol. Rep. 42, 2716–2727. doi: 10.3892/or.2019.7347
Hamano, R., Miyata, H., Yamasaki, M., Kurokawa, Y., Hara, J., Moon, J. H., et al. (2011). Overexpression of miR-200c induces chemoresistance in esophageal cancers mediated through activation of the Akt signaling pathway. Clin. Cancer Res. 17, 3029–3038. doi: 10.1158/1078-0432.CCR-10-2532
Han, L., Cui, D., Li, B., Xu, W. W., Lam, A. K. Y., Chan, K. T., et al. (2019). MicroRNA-338-5p reverses chemoresistance and inhibits invasion of esophageal squamous cell carcinoma cells by targeting Id-1. Cancer Sci. 110, 3677–3688. doi: 10.1111/cas.14220
Hassan, M. S., Williams, F., Awasthi, N., Schwarz, M. A., Schwarz, R. E., Li, J., et al. (2019). Combination effect of lapatinib with foretinib in HER2 and MET co-activated experimental esophageal adenocarcinoma. Sci. Rep. 9:17608. doi: 10.1038/s41598-019-54129-7
Herbst, R. S., Arkenau, H. T., Santana-Davila, R., Calvo, E., Paz-Ares, L., Cassier, P. A., et al. (2019). Ramucirumab plus pembrolizumab in patients with previously treated advanced non-small-cell lung cancer, gastro-oesophageal cancer, or urothelial carcinomas (JVDF): a multicohort, non-randomised, open-label, phase 1a/b trial. Lancet Oncol. 20, 1109–1123. doi: 10.1016/S1470-2045(19)30458-9
Hong, L., Han, Y., Zhang, H., Li, M., Gong, T., Sun, L., et al. (2010). The prognostic and chemotherapeutic value of miR-296 in esophageal squamous cell carcinoma. Ann. Surg. 251, 1056–1063. doi: 10.1097/SLA.0b013e3181dd4ea9
Hu, M., Zhang, Q., Tian, X. H., Wang, J. L., Niu, Y. X., and Li, G. (2019). lncRNA CCAT1 is a biomarker for the proliferation and drug resistance of esophageal cancer via the miR-143/PLK1/BUBR1 axis. Mol. Carcinog. 58, 2207–2217. doi: 10.1002/mc.23109
Hu, X., Wu, D., He, X., Zhao, H., He, Z., Lin, J., et al. (2019). circGSK3beta promotes metastasis in esophageal squamous cell carcinoma by augmenting beta-catenin signaling. Mol. Cancer 18:160. doi: 10.1186/s12943-019-1095-y
Huang, X. P., Li, X., Situ, M. Y., Huang, L. Y., Wang, J. Y., He, T. C., et al. (2018). Entinostat reverses cisplatin resistance in esophageal squamous cell carcinoma via down-regulation of multidrug resistance gene 1. Cancer Lett. 414, 294–300. doi: 10.1016/j.canlet.2017.10.023
Hummel, R., Sie, C., Watson, D. I., Wang, T., Ansar, A., Michael, M. Z., et al. (2014). MicroRNA signatures in chemotherapy resistant esophageal cancer cell lines. World J. Gastroenterol. 20, 14904–14912. doi: 10.3748/wjg.v20.i40.14904
Imanaka, Y., Tsuchiya, S., Sato, F., Shimada, Y., Shimizu, K., and Tsujimoto, G. (2011). MicroRNA-141 confers resistance to cisplatin-induced apoptosis by targeting YAP1 in human esophageal squamous cell carcinoma. J. Hum. Genet. 56, 270–276. doi: 10.1038/jhg.2011.1
Jin, Y. Y., Chen, Q. J., Xu, K., Ren, H. T., Bao, X., Ma, Y. N., et al. (2016). Involvement of microRNA-141-3p in 5-fluorouracil and oxaliplatin chemo-resistance in esophageal cancer cells via regulation of PTEN. Mol. Cell. Biochem. 422, 161–170. doi: 10.1007/s11010-016-2816-9
Jingjing, L., Wangyue, W., Qiaoqiao, X., and Jietong, Y. (2016). MiR-218 increases sensitivity to cisplatin in esophageal cancer cells via targeting survivin expression. Open Med. 11, 31–35. doi: 10.1515/med-2016-0007
Kang, M., Ren, M., Li, Y., Fu, Y., Deng, M., and Li, C. (2018). Exosome-mediated transfer of lncRNA PART1 induces gefitinib resistance in esophageal squamous cell carcinoma via functioning as a competing endogenous RNA. J. Exp. Clin. Cancer Res. 37:171. doi: 10.1186/s13046-018-0845-9
Kato, K., Doki, Y., Ura, T., Hamamoto, Y., Kojima, T., Tsushima, T., et al. (2020). Long-term efficacy and predictive correlates of response to nivolumab in Japanese patients with esophageal cancer. Cancer Sci. 111, 1676–1684. doi: 10.1111/cas.14380
Kojima, T., Shah, M. A., Muro, K., Francois, E., Adenis, A., Hsu, C. H., et al. (2020). Randomized phase III KEYNOTE-181 study of pembrolizumab versus chemotherapy in advanced esophageal cancer. J. Clin. Oncol. 38, 4138–4148. doi: 10.1200/JCO.20.01888
Komatsu, S., Ichikawa, D., Kawaguchi, T., Miyamae, M., Okajima, W., Ohashi, T., et al. (2016a). Circulating miR-21 as an independent predictive biomarker for chemoresistance in esophageal squamous cell carcinoma. Am. J. Cancer Res. 6, 1511–1523.
Komatsu, S., Ichikawa, D., Kawaguchi, T., Takeshita, H., Miyamae, M., Ohashi, T., et al. (2016b). Plasma microRNA profiles: identification of miR-23a as a novel biomarker for chemoresistance in esophageal squamous cell carcinoma. Oncotarget 7, 62034–62048. doi: 10.18632/oncotarget.11500
Kopp, F., and Mendell, J. T. (2018). Functional classification and experimental dissection of long noncoding RNAs. Cell 172, 393–407. doi: 10.1016/j.cell.2018.01.011
Kristensen, L. S., Andersen, M. S., Stagsted, L. V. W., Ebbesen, K. K., Hansen, T. B., and Kjems, J. (2019). The biogenesis, biology and characterization of circular RNAs. Nat. Rev. Genet. 20, 675–691. doi: 10.1038/s41576-019-0158-7
Li, B., Hong, P., Zheng, C. C., Dai, W., Chen, W. Y., Yang, Q. S., et al. (2019). Identification of miR-29c and its target FBXO31 as a key regulatory mechanism in esophageal cancer chemoresistance: functional validation and clinical significance. Theranostics 9, 1599–1613. doi: 10.7150/thno.30372
Li, J., Chen, Z., Tian, L., Zhou, C., He, M. Y., Gao, Y., et al. (2014). LncRNA profile study reveals a three-lncRNA signature associated with the survival of patients with oesophageal squamous cell carcinoma. Gut 63, 1700–1710. doi: 10.1136/gutjnl-2013-305806
Li, N., Zhao, Z., Miao, F., Cai, S., Liu, P., Yu, Y., et al. (2021). Silencing of long non-coding RNA LINC01270 inhibits esophageal cancer progression and enhances chemosensitivity to 5-fluorouracil by mediating GSTP1methylation. Cancer Gene Ther. 28, 471–485. doi: 10.1038/s41417-020-00232-1
Li, W., Zheng, J., Deng, J., You, Y., Wu, H., Li, N., et al. (2014). Increased levels of the long intergenic non-protein coding RNA POU3F3 promote DNA methylation in esophageal squamous cell carcinoma cells. Gastroenterology 146, 1714.e5–1726.e5. doi: 10.1053/j.gastro.2014.03.002
Li, Y., Li, J., Luo, M., Zhou, C., Shi, X., Yang, W., et al. (2018). Novel long noncoding RNA NMR promotes tumor progression via NSUN2 and BPTF in esophageal squamous cell carcinoma. Cancer Lett. 430, 57–66. doi: 10.1016/j.canlet.2018.05.013
Lin, C., Zhang, S., Wang, Y., Wang, Y., Nice, E., Guo, C., et al. (2018). Functional role of a novel long noncoding RNA TTN-AS1 in esophageal squamous cell carcinoma progression and metastasis. Clin. Cancer Res. 24, 486–498. doi: 10.1158/1078-0432.CCR-17-1851
Lin, C. H., Li, H. Y., Liu, Y. P., Kuo, P. F., Wang, W. C., Lin, F. C., et al. (2019). High-CLDN4 ESCC cells harbor stem-like properties and indicate for poor concurrent chemoradiation therapy response in esophageal squamous cell carcinoma. Ther. Adv. Med. Oncol. 11:1758835919875324. doi: 10.1177/1758835919875324
Lin, K., Jiang, H., Zhuang, S. S., Qin, Y. S., Qiu, G. D., She, Y. Q., et al. (2019). Long noncoding RNA LINC00261 induces chemosensitization to 5-fluorouracil by mediating methylation-dependent repression of DPYD in human esophageal cancer. FASEB J. 33, 1972–1988. doi: 10.1096/fj.201800759R
Lin, W. C., Chen, L. H., Hsieh, Y. C., Yang, P. W., Lai, L. C., Chuang, E. Y., et al. (2019). miR-338-5p inhibits cell proliferation, colony formation, migration and cisplatin resistance in esophageal squamous cancer cells by targeting FERMT2. Carcinogenesis 40, 883–892. doi: 10.1093/carcin/bgy189
Lindner, K., Eichelmann, A. K., Matuszcak, C., Hussey, D. J., Haier, J., and Hummel, R. (2018). Complex epigenetic regulation of chemotherapy resistance and biohlogy in esophageal squamous cell carcinoma via MicroRNAs. Int. J. Mol. Sci. 19:499. doi: 10.3390/ijms19020499
Liu, A., Zhu, J., Wu, G., Cao, L., Tan, Z., Zhang, S., et al. (2017). Antagonizing miR-455-3p inhibits chemoresistance and aggressiveness in esophageal squamous cell carcinoma. Mol. Cancer 16:106. doi: 10.1186/s12943-017-0669-9
Liu, D. S., Read, M., Cullinane, C., Azar, W. J., Fennell, C. M., Montgomery, K. G., et al. (2015). APR-246 potently inhibits tumour growth and overcomes chemoresistance in preclinical models of oesophageal adenocarcinoma. Gut 64, 1506–1516. doi: 10.1136/gutjnl-2015-309770
Liu, H., Zhang, J., Luo, X., Zeng, M., Xu, L., Zhang, Q., et al. (2020). Overexpression of the long noncoding RNA FOXD2-AS1 promotes cisplatin resistance in esophageal squamous cell carcinoma through the miR-195/Akt/mTOR axis. Oncol. Res. 28, 65–73. doi: 10.3727/096504019X15656904013079
Liu, J., Liu, Z. X., Wu, Q. N., Lu, Y. X., Wong, C. W., Miao, L., et al. (2020). Long noncoding RNA AGPG regulates PFKFB3-mediated tumor glycolytic reprogramming. Nat. Commun. 11:1507. doi: 10.1038/s41467-020-15112-3
Liu, S. L., Yang, H., Zhang, P., Zhang, L., Zhao, L., Luo, L. L., et al. (2015). Neoadjuvant chemoradiotherapy with cisplatin plus vinorelbine versus cisplatin plus fluorouracil for esophageal squamous cell carcinoma: a matched case-control study. Radiother. Oncol. 116, 262–268. doi: 10.1016/j.radonc.2015.07.020
Longley, D. B., Harkin, D. P., and Johnston, P. G. (2003). 5-fluorouracil: mechanisms of action and clinical strategies. Nat. Rev. Cancer 3, 330–338. doi: 10.1038/nrc1074
Lopez, A., Harada, K., Chen, H. C., Bhutani, M. S., Weston, B., Lee, J. H., et al. (2020). Taxane-based or platinum-based combination chemotherapy given concurrently with radiation followed by surgery resulting in high cure rates in esophageal cancer patients. Medicine 99:e19295. doi: 10.1097/MD.0000000000019295
Luo, A., Zhou, X., Shi, X., Zhao, Y., Men, Y., Chang, X., et al. (2019). Exosome-derived miR-339-5p mediates radiosensitivity by targeting Cdc25A in locally advanced esophageal squamous cell carcinoma. Oncogene 38, 4990–5006. doi: 10.1038/s41388-019-0771-0
Ma, Y., Wang, B., Guo, Y., Zhang, Y., Huang, S., Bao, X., et al. (2016). Inhibition of miR-196a affects esophageal cancer cell growth in vitro. Biomed. Pharmacother. 84, 22–27. doi: 10.1016/j.biopha.2016.09.013
Meng, F., Qian, L., Lv, L., Ding, B., Zhou, G., Cheng, X., et al. (2016). miR-193a-3p regulation of chemoradiation resistance in oesophageal cancer cells via the PSEN1 gene. Gene 579, 139–145. doi: 10.1016/j.gene.2015.12.060
Ni, W., Yu, S., Zhang, W., Xiao, Z., Zhou, Z., Chen, D., et al. (2020). A phase-II/III randomized controlled trial of adjuvant radiotherapy or concurrent chemoradiotherapy after surgery versus surgery alone in patients with stage-IIB/III esophageal squamous cell carcinoma. BMC Cancer 20:130. doi: 10.1186/s12885-020-6592-2
Nyhan, M. J., O’donovan, T. R., Boersma, A. W., Wiemer, E. A., and Mckenna, S. L. (2016). MiR-193b promotes autophagy and non-apoptotic cell death in oesophageal cancer cells. BMC Cancer 16:101. doi: 10.1186/s12885-016-2123-6
Pan, W., Zhang, N., Liu, W., Liu, J., Zhou, L., Liu, Y., et al. (2018). The long noncoding RNA GAS8-AS1 suppresses hepatocarcinogenesis by epigenetically activating the tumor suppressor GAS8. J. Biol. Chem. 293, 17154–17165. doi: 10.1074/jbc.RA118.003055
Peng, W. X., Koirala, P., and Mo, Y. Y. (2017). LncRNA-mediated regulation of cell signaling in cancer. Oncogene 36, 5661–5667. doi: 10.1038/onc.2017.184
Phatak, P., Byrnes, K. A., Mansour, D., Liu, L., Cao, S., Li, R., et al. (2016). Overexpression of miR-214-3p in esophageal squamous cancer cells enhances sensitivity to cisplatin by targeting survivin directly and indirectly through CUG-BP1. Oncogene 35, 2087–2097. doi: 10.1038/onc.2015.271
Press, M. F., Ellis, C. E., Gagnon, R. C., Grob, T. J., Buyse, M., Villalobos, I., et al. (2017). HER2 Status in Advanced or Metastatic Gastric, Esophageal, or Gastroesophageal Adenocarcinoma for Entry to the TRIO-013/LOGiC Trial of Lapatinib. Mol. Cancer Ther. 16, 228–238. doi: 10.1158/1535-7163.MCT-15-0887
Qiao, Y., Zhang, C., Li, A., Wang, D., Luo, Z., Ping, Y., et al. (2018). IL6 derived from cancer-associated fibroblasts promotes chemoresistance via CXCR7 in esophageal squamous cell carcinoma. Oncogene 37, 873–883. doi: 10.1038/onc.2017.387
Qu, F., Wang, L., Wang, C., Yu, L., Zhao, K., and Zhong, H. (2021). Circular RNA circ_0006168 enhances Taxol resistance in esophageal squamous cell carcinoma by regulating miR-194-5p/JMJD1C axis. Cancer Cell Int. 21:273. doi: 10.1186/s12935-021-01984-y
Ren, Y., Chen, Y., Liang, X., Lu, Y., Pan, W., and Yang, M. (2017a). MiRNA-638 promotes autophagy and malignant phenotypes of cancer cells via directly suppressing DACT3. Cancer Lett. 390, 126–136. doi: 10.1016/j.canlet.2017.01.009
Ren, Y., Shang, J., Li, J., Liu, W., Zhang, Z., Yuan, J., et al. (2017b). The long noncoding RNA PCAT-1 links the microRNA miR-215 to oncogene CRKL-mediated signaling in hepatocellular carcinoma. J. Biol. Chem. 292, 17939–17949. doi: 10.1074/jbc.M116.773978
Rogers, J. E., Xiao, L., Amlashi, F. G., Elimova, E., Blum Murphy, M. A., Sanders, E., et al. (2019). Ramucirumab and paclitaxel administered every 2 weeks (mRAINBOW Regimen) in advanced gastroesophageal adenocarcinoma. Oncology 96, 252–258. doi: 10.1159/000496978
Rogers, J. E., Xiao, L., Trail, A., Blum Murphy, M., Palmer, M., and Ajani, J. A. (2020). Nivolumab in combination with irinotecan and 5-fluorouracil (FOLFIRI) for refractory advanced gastroesophageal cancer. Oncology 98, 289–294. doi: 10.1159/000505974
Rupaimoole, R., and Slack, F. J. (2017). MicroRNA therapeutics: towards a new era for the management of cancer and other diseases. Nat. Rev. Drug Discov. 16, 203–222. doi: 10.1038/nrd.2016.246
Sang, M., Meng, L., Sang, Y., Liu, S., Ding, P., Ju, Y., et al. (2018). Circular RNA ciRS-7 accelerates ESCC progression through acting as a miR-876-5p sponge to enhance MAGE-A family expression. Cancer Lett. 426, 37–46. doi: 10.1016/j.canlet.2018.03.049
Shah, M. A., Kojima, T., Hochhauser, D., Enzinger, P., Raimbourg, J., Hollebecque, A., et al. (2019). Efficacy and safety of pembrolizumab for heavily pretreated patients with advanced, metastatic adenocarcinoma or squamous cell carcinoma of the esophagus: the phase 2 KEYNOTE-180 study. JAMA Oncol. 5, 546–550. doi: 10.1001/jamaoncol.2018.5441
Shepard, G., Arrowsmith, E. R., Murphy, P., Barton, J. H. Jr., Peyton, J. D., Mainwaring, M., et al. (2017). A Phase II study with lead-in safety cohort of 5-Fluorouracil, Oxaliplatin, and Lapatinib in combination with radiation therapy as neoadjuvant treatment for patients with localized HER2-positive esophagogastric adenocarcinomas. Oncologist 22, 1152–1198. doi: 10.1634/theoncologist.2017-0186
Shi, S., Huang, X., Ma, X., Zhu, X., and Zhang, Q. (2020). Research of the mechanism on miRNA193 in exosomes promotes cisplatin resistance in esophageal cancer cells. PLoS One 15:e0225290. doi: 10.1371/journal.pone.0225290
Siegel, R. L., Miller, K. D., and Jemal, A. (2020). Cancer statistics, 2020. CA Cancer J. Clin. 70, 7–30. doi: 10.3322/caac.21590
Song, Y., Li, L., Ou, Y., Gao, Z., Li, E., Li, X., et al. (2014). Identification of genomic alterations in oesophageal squamous cell cancer. Nature 509, 91–95. doi: 10.1038/nature13176
Streppel, M. M., Pai, S., Campbell, N. R., Hu, C., Yabuuchi, S., Canto, M. I., et al. (2013). MicroRNA 223 is upregulated in the multistep progression of Barrett’s esophagus and modulates sensitivity to chemotherapy by targeting PARP1. Clin. Cancer Res. 19, 4067–4078. doi: 10.1158/1078-0432.CCR-13-0601
Stroes, C. I., Schokker, S., Creemers, A., Molenaar, R. J., Hulshof, M., Van Der Woude, S. O., et al. (2020). Phase II feasibility and biomarker study of neoadjuvant trastuzumab and pertuzumab with chemoradiotherapy for resectable human epidermal growth factor receptor 2-positive esophageal adenocarcinoma: TRAP Study. J. Clin. Oncol. 38, 462–471. doi: 10.1200/JCO.19.01814
Subbarayan, P. R., Sarkar, M., Nelson, G., Benitez, E., Singhal, S., and Ardalan, B. (2010). Chronic exposure of colorectal cancer cells in culture to fluoropyrimidine analogs induces thymidylate synthase and suppresses p53. A molecular explanation for the mechanism of 5-FU resistance. Anticancer Res. 30, 1149–1156.
Sudo, K., Kato, K., Matsuzaki, J., Takizawa, S., Aoki, Y., Shoji, H., et al. (2020). Identification of serum microRNAs predicting the response of esophageal squamous-cell carcinoma to nivolumab. Jpn. J. Clin. Oncol. 50, 114–121. doi: 10.1093/jjco/hyz146
Sugimura, K., Miyata, H., Tanaka, K., Hamano, R., Takahashi, T., Kurokawa, Y., et al. (2012). Let-7 expression is a significant determinant of response to chemotherapy through the regulation of IL-6/STAT3 pathway in esophageal squamous cell carcinoma. Clin. Cancer Res. 18, 5144–5153. doi: 10.1158/1078-0432.CCR-12-0701
Sun, F., Zhang, C., Ma, D., and Wang, K. (2019). MicroRNA-544 inhibits esophageal squamous cell carcinoma cell proliferation and enhances sensitivity to cisplatin by repressing E2F transcription factor 5. Oncol. Lett. 18, 4203–4209. doi: 10.3892/ol.2019.10749
Tan, D. S. W., Chong, F. T., Leong, H. S., Toh, S. Y., Lau, D. P., Kwang, X. L., et al. (2017). Long noncoding RNA EGFR-AS1 mediates epidermal growth factor receptor addiction and modulates treatment response in squamous cell carcinoma. Nat. Med. 23, 1167–1175. doi: 10.1038/nm.4401
Tanaka, K., Miyata, H., Sugimura, K., Fukuda, S., Kanemura, T., Yamashita, K., et al. (2015). miR-27 is associated with chemoresistance in esophageal cancer through transformation of normal fibroblasts to cancer-associated fibroblasts. Carcinogenesis 36, 894–903. doi: 10.1093/carcin/bgv067
Tanaka, K., Miyata, H., Yamasaki, M., Sugimura, K., Takahashi, T., Kurokawa, Y., et al. (2013). Circulating miR-200c levels significantly predict response to chemotherapy and prognosis of patients undergoing neoadjuvant chemotherapy for esophageal cancer. Ann. Surg. Oncol. 20, (Suppl. 3), S607–S615. doi: 10.1245/s10434-013-3093-4
Tanaka, Y., Kamohara, H., Kinoshita, K., Kurashige, J., Ishimoto, T., Iwatsuki, M., et al. (2013). Clinical impact of serum exosomal microRNA-21 as a clinical biomarker in human esophageal squamous cell carcinoma. Cancer 119, 1159–1167. doi: 10.1002/cncr.27895
Tian, H., Hou, L., Xiong, Y. M., Huang, J. X., She, Y. J., Bi, X. B., et al. (2015). miR-218 suppresses tumor growth and enhances the chemosensitivity of esophageal squamous cell carcinoma to cisplatin. Oncol. Rep. 33, 981–989. doi: 10.3892/or.2014.3657
Tin, A. W., Smith, E., Hepworth, R., Walker, J., Wilson, D., and Wadd, N. (2018). Perioperative ECX chemotherapy in older adults with gastroesophageal adenocarcinoma. J. Geriatr. Oncol. 9, 569–574. doi: 10.1016/j.jgo.2018.05.007
Tong, Y., Yang, L., Yu, C., Zhu, W., Zhou, X., Xiong, Y., et al. (2020). Tumor-secreted exosomal lncRNA POU3F3 promotes cisplatin resistance in ESCC by inducing fibroblast differentiation into CAFs. Mol. Ther. Oncolytics 18, 1–13. doi: 10.1016/j.omto.2020.05.014
van Zweeden, A. A., Van Groeningen, C. J., Honeywell, R. J., Giovannetti, E., Ruijter, R., Smorenburg, C. H., et al. (2018). Randomized phase 2 study of gemcitabine and cisplatin with or without vitamin supplementation in patients with advanced esophagogastric cancer. Cancer Chemother. Pharmacol. 82, 39–48. doi: 10.1007/s00280-018-3588-6
Vo, J. N., Cieslik, M., Zhang, Y., Shukla, S., Xiao, L., Zhang, Y., et al. (2019). The landscape of circular RNA in cancer. Cell 176, 869.e13–881.e13. doi: 10.1016/j.cell.2018.12.021
Wagner, A. D., Grabsch, H. I., Mauer, M., Marreaud, S., Caballero, C., Thuss-Patience, P., et al. (2019). EORTC-1203-GITCG - the “INNOVATION”-trial: effect of chemotherapy alone versus chemotherapy plus trastuzumab, versus chemotherapy plus trastuzumab plus pertuzumab, in the perioperative treatment of HER2 positive, gastric and gastroesophageal junction adenocarcinoma on pathologic response rate: a randomized phase II-intergroup trial of the EORTC-Gastrointestinal Tract Cancer Group, Korean Cancer Study Group and Dutch Upper GI-Cancer group. BMC Cancer 19:494. doi: 10.1186/s12885-019-5675-4
Wang, W., He, X., Zheng, Z., Ma, X., Hu, X., Wu, D., et al. (2017). Serum HOTAIR as a novel diagnostic biomarker for esophageal squamous cell carcinoma. Mol. Cancer 16:75. doi: 10.1186/s12943-017-0643-6
Wang, X., Ren, Y., Yang, X., Xiong, X., Han, S., Ge, Y., et al. (2015c). miR-190a inhibits epithelial-mesenchymal transition of hepatoma cells via targeting the long non-coding RNA treRNA. FEBS Lett. 589, 4079–4087. doi: 10.1016/j.febslet.2015.11.024
Wang, X., Ren, Y., Wang, Z., Xiong, X., Han, S., Pan, W., et al. (2015b). Down-regulation of 5S rRNA by miR-150 and miR-383 enhances c-Myc-rpL11 interaction and inhibits proliferation of esophageal squamous carcinoma cells. FEBS Lett. 589, 3989–3997. doi: 10.1016/j.febslet.2015.11.012
Wang, Y., Feng, J., Zang, W., Du, Y., Chen, X., Sun, Q., et al. (2015d). MiR-499 enhances the cisplatin sensitivity of esophageal carcinoma cell lines by targeting DNA Polymerase beta. Cell Physiol. Biochem. 36, 1587–1596. doi: 10.1159/000430321
Wang, X., Li, M., Wang, Z., Han, S., Tang, X., Ge, Y., et al. (2015a). Silencing of long noncoding RNA MALAT1 by miR-101 and miR-217 inhibits proliferation, migration, and invasion of esophageal squamous cell carcinoma cells. J. Biol. Chem. 290, 3925–3935. doi: 10.1074/jbc.M114.596866
Wang, Y., Zhao, Y., Herbst, A., Kalinski, T., Qin, J., Wang, X., et al. (2016). miR-221 mediates chemoresistance of esophageal adenocarcinoma by direct targeting of DKK2 expression. Ann. Surg. 264, 804–814. doi: 10.1097/SLA.0000000000001928
Wang, Y. S., Tian, J., Han, Y., Han, S. M., and Shi, S. B. (2016). Gemcitabine plus vinorelbine as second-line therapy in patients with metastatic esophageal cancer previously treated with platinum-based chemotherapy. Oncol. Res. 24, 129–135. doi: 10.3727/096504016X14618564639213
Wen, J., Luo, K., Liu, H., Liu, S., Lin, G., Hu, Y., et al. (2016). MiRNA expression analysis of pretreatment biopsies predicts the pathological response of esophageal squamous cell carcinomas to neoadjuvant chemoradiotherapy. Ann. Surg. 263, 942–948. doi: 10.1097/SLA.0000000000001489
Winther, M., Knudsen, S., Dahlgaard, J., Jensen, T., Hansen, A., Jensen, P. B., et al. (2016). Clinical impact of a novel MicroRNA chemo-sensitivity predictor in gastrooesophageal cancer. PLoS One 11:e0148070. doi: 10.1371/journal.pone.0148070
Wu, K., Hu, Y., Yan, K., Qi, Y., Zhang, C., Zhu, D., et al. (2020). microRNA-10b confers cisplatin resistance by activating AKT/mTOR/P70S6K signaling via targeting PPARgamma in esophageal cancer. J. Cell. Physiol. 235, 1247–1258. doi: 10.1002/jcp.29040
Wu, K., Yang, Y., Zhao, J., and Zhao, S. (2016). BAG3-mediated miRNA let-7g and let-7i inhibit proliferation and enhance apoptosis of human esophageal carcinoma cells by targeting the drug transporter ABCC10. Cancer Lett. 371, 125–133. doi: 10.1016/j.canlet.2015.11.031
Xu, Y., Li, Y., Jin, J., Han, G., Sun, C., Pizzi, M. P., et al. (2019). LncRNA PVT1 up-regulation is a poor prognosticator and serves as a therapeutic target in esophageal adenocarcinoma. Mol. Cancer 18:141. doi: 10.1186/s12943-019-1064-5
Yamaguchi, K., Fujitani, K., Nagashima, F., Omuro, Y., Machida, N., Nishina, T., et al. (2018). Ramucirumab for the treatment of metastatic gastric or gastroesophageal junction adenocarcinoma following disease progression on first-line platinum- or fluoropyrimidine-containing combination therapy in Japanese patients: a phase 2, open-label study. Gastr. Cancer 21, 1041–1049. doi: 10.1007/s10120-018-0811-4
Yang, C., Shen, S., Zheng, X., Ye, K., Ge, H., Sun, Y., et al. (2020). Long non-coding RNA LINC00337 induces autophagy and chemoresistance to cisplatin in esophageal squamous cell carcinoma cells via upregulation of TPX2 by recruiting E2F4. FASEB J. 34, 6055–6069. doi: 10.1096/fj.201900731RR
Yang, S., Wang, P., Wang, S., Cong, A., Zhang, Q., Shen, W., et al. (2020). miRNA-181a-5p enhances the sensitivity of cells to cisplatin in esophageal adenocarcinoma by targeting CBLB. Cancer Manag. Res. 12, 4981–4990. doi: 10.2147/CMAR.S251264
Yang, X., Song, J. H., Cheng, Y., Wu, W., Bhagat, T., Yu, Y., et al. (2014). Long non-coding RNA HNF1A-AS1 regulates proliferation and migration in oesophageal adenocarcinoma cells. Gut 63, 881–890. doi: 10.1136/gutjnl-2013-305266
Yang, Y. C., Liu, G. J., Yuan, D. F., Li, C. Q., Xue, M., and Chen, L. J. (2019). Influence of exosome-derived miR-21on chemotherapy resistance of esophageal cancer. Eur. Rev. Med. Pharmacol. Sci. 23, 1513–1519. doi: 10.26355/eurrev_201902_17109
You, B. H., Yoon, J. H., Kang, H., Lee, E. K., Lee, S. K., and Nam, J. W. (2019). HERES, a lncRNA that regulates canonical and noncanonical Wnt signaling pathways via interaction with EZH2. Proc. Natl. Acad. Sci. U.S.A. 116, 24620–24629. doi: 10.1073/pnas.1912126116
Yuan, J., Song, Y., Pan, W., Li, Y., Xu, Y., Xie, M., et al. (2020). LncRNA SLC26A4-AS1 suppresses the MRN complex-mediated DNA repair signaling and thyroid cancer metastasis by destabilizing DDX5. Oncogene 39, 6664–6676. doi: 10.1038/s41388-020-01460-3
Zang, W., Wang, T., Wang, Y., Chen, X., Du, Y., Sun, Q., et al. (2016). Knockdown of long non-coding RNA TP73-AS1 inhibits cell proliferation and induces apoptosis in esophageal squamous cell carcinoma. Oncotarget 7, 19960–19974. doi: 10.18632/oncotarget.6963
Zhang, E., Han, L., Yin, D., He, X., Hong, L., Si, X., et al. (2017). H3K27 acetylation activated-long non-coding RNA CCAT1 affects cell proliferation and migration by regulating SPRY4 and HOXB13 expression in esophageal squamous cell carcinoma. Nucleic Acids Res. 45, 3086–3101. doi: 10.1093/nar/gkw1247
Zhang, H., Hua, Y., Jiang, Z., Yue, J., Shi, M., Zhen, X., et al. (2019). Cancer-associated fibroblast-promoted LncRNA DNM3OS confers radioresistance by regulating DNA damage response in esophageal squamous cell carcinoma. Clin. Cancer Res. 25, 1989–2000. doi: 10.1158/1078-0432.CCR-18-0773
Zhang, H., Li, M., Han, Y., Hong, L., Gong, T., Sun, L., et al. (2010). Down-regulation of miR-27a might reverse multidrug resistance of esophageal squamous cell carcinoma. Dig. Dis. Sci. 55, 2545–2551. doi: 10.1007/s10620-009-1051-6
Zhang, J. X., Chen, Z. H., Xu, Y., Chen, J. W., Weng, H. W., Yun, M., et al. (2017). Downregulation of MicroRNA-644a promotes esophageal squamous cell carcinoma aggressiveness and stem cell-like phenotype via dysregulation of PITX2. Clin. Cancer Res. 23, 298–310. doi: 10.1158/1078-0432.CCR-16-0414
Zhang, N., Song, Y., Xu, Y., Liu, J., Shen, Y., Zhou, L., et al. (2020a). MED13L integrates Mediator-regulated epigenetic control into lung cancer radiosensitivity. Theranostics 10, 9378–9394. doi: 10.7150/thno.48247
Zhang, S., Jiang, H., Xu, Z., Jiang, Y., She, Y., Huang, X., et al. (2019). The resistance of esophageal cancer cells to paclitaxel can be reduced by the knockdown of long noncoding RNA DDX11-AS1 through TAF1/TOP2A inhibition. Am. J. Cancer Res. 9, 2233–2248.
Zhang, S., Liao, W., Wu, Q., Huang, X., Pan, Z., Chen, W., et al. (2020b). LINC00152 upregulates ZEB1 expression and enhances epithelial-mesenchymal transition and oxaliplatin resistance in esophageal cancer by interacting with EZH2. Cancer Cell Int. 20:569. doi: 10.1186/s12935-020-01620-1
Zhang, S., Zheng, F., Zhang, L., Huang, Z., Huang, X., Pan, Z., et al. (2020c). LncRNA HOTAIR-mediated MTHFR methylation inhibits 5-fluorouracil sensitivity in esophageal cancer cells. J. Exp. Clin. Cancer Res. 39:131. doi: 10.1186/s13046-020-01610-1
Zhang, X., Lu, N., Wang, L., Wang, Y., Li, M., Zhou, Y., et al. (2020d). Circular RNAs and esophageal cancer. Cancer Cell Int. 20:362. doi: 10.1186/s12935-020-01451-0
Zhang, X. D., Huang, G. W., Xie, Y. H., He, J. Z., Guo, J. C., Xu, X. E., et al. (2018). The interaction of lncRNA EZR-AS1 with SMYD3 maintains overexpression of EZR in ESCC cells. Nucleic Acids Res. 46, 1793–1809. doi: 10.1093/nar/gkx1259
Zhang, Z., Xiong, R., Li, C., Xu, M., and Guo, M. (2019). LncRNA TUG1 promotes cisplatin resistance in esophageal squamous cell carcinoma cells by regulating Nrf2. Acta Biochim. Biophys. Sin. 51, 826–833. doi: 10.1093/abbs/gmz069
Zhao, Y., Ma, K., Yang, S., Zhang, X., Wang, F., Zhang, X., et al. (2018). MicroRNA-125a-5p enhances the sensitivity of esophageal squamous cell carcinoma cells to cisplatin by suppressing the activation of the STAT3 signaling pathway. Int. J. Oncol. 53, 644–658. doi: 10.3892/ijo.2018.4409
Zhen, Q., Gao, L. N., Wang, R. F., Chu, W. W., Zhang, Y. X., Zhao, X. J., et al. (2018). LncRNA PCAT-1 promotes tumour growth and chemoresistance of oesophageal cancer to cisplatin. Cell Biochem. Funct. 36, 27–33. doi: 10.1002/cbf.3314
Zheng, T. L., Li, D. P., He, Z. F., and Zhao, S. (2019). miR-145 sensitizes esophageal squamous cell carcinoma to cisplatin through directly inhibiting PI3K/AKT signaling pathway. Cancer Cell Int. 19:250. doi: 10.1186/s12935-019-0943-6
Zhou, J., Wu, Z., Wong, G., Pectasides, E., Nagaraja, A., Stachler, M., et al. (2017). CDK4/6 or MAPK blockade enhances efficacy of EGFR inhibition in oesophageal squamous cell carcinoma. Nat. Commun. 8:13897. doi: 10.1038/ncomms13897
Zhou, T., Fu, H., Dong, B., Dai, L., Yang, Y., Yan, W., et al. (2020). HOXB7 mediates cisplatin resistance in esophageal squamous cell carcinoma through involvement of DNA damage repair. Thorac. Cancer 11, 3071–3085. doi: 10.1111/1759-7714.13142
Zhou, X. L., Wang, W. W., Zhu, W. G., Yu, C. H., Tao, G. Z., Wu, Q. Q., et al. (2016). High expression of long non-coding RNA AFAP1-AS1 predicts chemoradioresistance and poor prognosis in patients with esophageal squamous cell carcinoma treated with definitive chemoradiotherapy. Mol. Carcinog. 55, 2095–2105. doi: 10.1002/mc.22454
Zhou, Y., and Hong, L. (2013). Prediction value of miR-483 and miR-214 in prognosis and multidrug resistance of esophageal squamous cell carcinoma. Genet. Test Mol. Biomarkers 17, 470–474. doi: 10.1089/gtmb.2012.0518
Zhu, H., Du, F., and Cao, C. (2021). Restoration of circPSMC3 sensitizes gefitinib-resistant esophageal squamous cell carcinoma cells to gefitinib by regulating miR-10a-5p/PTEN axis. Cell Biol. Int. 45, 107–116. doi: 10.1002/cbin.11473
Zhu, Y., Zhang, Y., Li, X., Su, Y., Wang, N., Chen, M., et al. (2021). Downregulation of miR-106b-3p increases sensitivity to cisplatin in esophageal cancer cells by targeting TGM3. Mol. Med. Rep. 23:471. doi: 10.3892/mmr.2021.12110
Zou, F. W., Yang, S. Z., Li, W. Y., Liu, C. Y., Liu, X. H., Hu, C. H., et al. (2020). circRNA_001275 upregulates Wnt7a expression by competitively sponging miR-370-3p to promote cisplatin resistance in esophageal cancer. Int. J. Oncol. 57, 151–160. doi: 10.3892/ijo.2020.5050
Keywords: esophageal cancer, drug resistance, microRNA, long non-coding RNA, circular RNA
Citation: Wei L, Sun J, Zhang N, Shen Y, Wang T, Li Z and Yang M (2021) Novel Implications of MicroRNAs, Long Non-coding RNAs and Circular RNAs in Drug Resistance of Esophageal Cancer. Front. Cell Dev. Biol. 9:764313. doi: 10.3389/fcell.2021.764313
Received: 25 August 2021; Accepted: 12 October 2021;
Published: 22 November 2021.
Edited by:
Junming Yue, The University of Tennessee, Knoxville, United StatesReviewed by:
Daozhi Xu, Shenyang Medical College, ChinaRicardo Marques, University of Coimbra, Portugal
Copyright © 2021 Wei, Sun, Zhang, Shen, Wang, Li and Yang. This is an open-access article distributed under the terms of the Creative Commons Attribution License (CC BY). The use, distribution or reproduction in other forums is permitted, provided the original author(s) and the copyright owner(s) are credited and that the original publication in this journal is cited, in accordance with accepted academic practice. No use, distribution or reproduction is permitted which does not comply with these terms.
*Correspondence: Zengjun Li, lizengjun6@126.com; Ming Yang, aaryoung@yeah.net
†Lead contact