Biomechanical evaluation of two modified intramedullary fixation system for treating unstable femoral neck fractures: A finite element analysis
- Department of Orthopedics, Hong Hui Hospital, Xi’an Jiaotong University, Xi’an, Shaanxi, China
Purpose: The existing implants for fixation of femoral neck fractures have poor biomechanical stability, so the failure rate is high. We designed two modified intramedullary implants for treating unstable femoral neck fractures (UFNFs). We tried to improve the biomechanical stability of fixation by shortening the moment and reducing stress concentration. Each modified intramedullary implant was compared with cannulated screws (CSs) through finite element analysis (FEA).
Methods: Five different models were included: three cannulated screws (CSs, Model 1) in an inverted triangle configuration, the dynamic hip screw with an anti-rotation screw (DHS + AS, Model 2), the femoral neck system (FNS, Model 3), the modified intramedullary femoral neck system (IFNS, Model 4), and the modified intramedullary interlocking system (IIS, Model 5). Three-dimensional (3D) models of femur and implants were constructed by using 3D modelling software. Three load cases were simulated to assess the maximal displacement of models and fracture surface. The maximal stress at the bone and implants was also evaluated.
Results: FEA data showed that Model 5 had the best performance in terms of maximum displacement while Model 1 had the worst performance for this index under axial load of 2100 N. With respect to Maximum stress, Model 4 had the best performance while Model 2 had the worst performance under axial load. The general trends under bending and torsion load were consistent with that under axial load. Our data demonstrated that the two modified intramedullary implants exhibited the best biomechanical stability, followed by FNS and DHS + AS, and then three cannulated screws in axial, bending, and torsion load cases.
Conclusion: The two modified intramedullary designs showed the best biomechanical performance among the five implants included in this study. Therefore, this might provide some new options for trauma surgeons to deal with unstable femoral neck fractures.
Introduction
Femoral neck fractures in young patients are usually caused by high-energy injuries, such as car accidents, falling height, etc. The treatment principle for such fractures is anatomical reduction and rigid internal fixation. Unstable femoral neck fractures, such as Pauwels type-III, bear larger shear stress, which is more prone to bone non-union and necrosis of femoral head than type I and type II (Knobe et al., 2018; Knobe et al., 2019). It is reported that the failure rate of fixation for UFNFs can be as high as 15–40% (Parker, 2000; Slobogean et al., 2015). The stability of implants is one of the important measures to ensure successful bone healing and avoid postoperative complications (Ragnarsson and Kärrholm, 1991; Ragnarsson and Kärrholm, 1992; Palm et al., 2009). As for biomechanical characteristics, important parameters such as stress and displacement are considered to affect the realization of good biomechanical stability. It is a challenge for trauma surgeons to improve biomechanical stability of existing implants. In recent years, seeking more effective implants to treat UFNFs has become a research hotspot in this field.
At present, the fixation methods of femoral neck fractures mainly focus on several extramedullary fixation devices, such as cannulated screws, the dynamic hip screw with an anti-rotation screw, and femoral neck system. The fixation method of multiple CSs is less traumatic and easy to insert (Blomfeldt et al., 2005; Lee et al., 2007). Yet, the use of CSs is prone to femoral neck shortening, screw withdrawal, cutting out, etc (Parker, 2009). Dynamic hip screw with an anti-rotation screw can play a better role in anti-rotation and anti-shearing than CSs, but it brings greater trauma (Schwartsmann et al., 2018). Other scholars inserted an medial plate to improve stability and shear resistance (Ye et al., 2017; Zhuang et al., 2018). However, the insertion of the medial auxiliary plate will inevitably lead to greater trauma and damage the blood supply of the femoral head. FNS has been used to fix femoral neck fractures in recent years, but its efficacy remains to be further observed (Stoffel et al., 2017). In short, the above implants are extramedullary fixation devices. They have a long moment, which is easy to cause stress concentration and eventually lead to fixation failure. As far as we know, although intramedullary implants are superior to extramedullary implants in biomechanics, there are few studies about intramedullary implants for treating UFNFs.
In view of this, the authors designed two modified types of intramedullary implants, specifically for the treatment of UFNFs. As shown in Figure 1, the proximal part of the two intramedullary implants are designed with two screws. Two neck screws pass through the fracture line at a specific angle. This design can meet the requirements of fracture anti-compression and anti-rotation. When the femoral head bears the stress in different directions, the stress can be transmitted to the main nail with a short moment and distributed along the femoral bone marrow cavity.
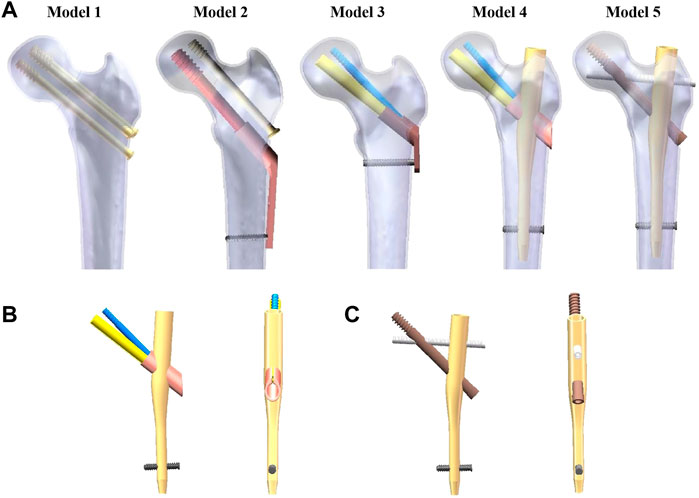
FIGURE 1. Five different implants after assembly of the finite element model. (A) Fixation of five different implants in the UFNF. (B) Schematic diagram of the modified intramedullary femoral neck system. (C) Schematic diagram of the modified intramedullary interlocking system. UFNF stands for unstable femoral neck fracture.
The biomechanical research of orthopedics adopts many methods, such as animal test (in vivo), physical modeling, cadaver model (in vitro), and computational simulation (in silico) through finite element analysis. Each research method has its own characteristics, including advantages and disadvantages (Hu and Wang, 2017). FEA is a numerical analysis method to simulate the real physical system by using mathematical approximation. This method can provide biomechanical evaluation and prognosis for various diseases and injury types, implant fixation and surgical techniques by tailoring finite element settings, such as material properties and boundary conditions (Leonardo-Diaz et al., 2020). It can not only make detailed quantitative estimation of displacement, but also quantitative estimation of load distribution in simulated surgical implants and surrounding bones. FEA has received extensive acceptance in the field of orthopedic research (Jitprapaikulsarn et al., 2021; Chantarapanich and Riansuwan, 2022). In this study, we designed two modified intramedullary implants for treating UFNFs. Each modified intramedullary implant was compared with cannulated screws through finite element analysis. The maximum stress and displacement on the bone and implants were evaluated. Three load cases were considered in the analyses which were axial, bending, and torsion loads. This study aimed to provide some new options for patients with UFNFs.
Materials and methods
Reconstruction of bone through 3D modelling and virtual surgery
The ethics committee of the Xi’an Hong Hui hospital approved this study (No.202202002). We recruited a 26-year-old healthy male volunteer with no history of hip joint or systemic diseases. The participant provided written informed consent to participate in this study. Computed tomography (CT) data images were collected to reconstruct 3D model of cancellous and cortical bones of femur via Mimics software (Materialise, Leuven, Belgium). CT examination uses X-ray to scan the body structure of the examiner, which will cause minimal radiation damage to healthy individuals, but usually will not cause serious damage. The CT (SOMATOM Definition AS1; Siemens, thickness, 0.6 mm; resolution, 512 × 512 pixels) scanning voltage and current were set in the range of 70–140 kV and 30–800 mA, respectively, until clear images were gotten. Then, CT images with the axial slice thickness of 1.5 mm were captured. We used Hounsfield Unit (HU) value (representing bone density threshold value) to distinguish cortical bones. The HU values for cancellous bones were defined as of less than 700, while that of cortical bones was more than 700 (Abdul Wahab et al., 2020). After that, a femoral neck fracture model with Pauwels angle of 70° was established. Computer-aided design (CAD) software (SolidWorks software, Dassault Systemes SolidWorks Corp., USA) was used to build five configurations of implants for UFNFs. The above fixation models were converted into the stereolithography (STL) format and exported to the 3-Matic software (Materialise, Leuven, Belgium), whereby the implants were inserted into the femur bone. Five different constructions were included: three CSs in an inverted triangle configuration (Model 1), DHS + AS (Model 2), FNS (Model 3), the modified IFNS (Model 4), and the modified IIS (Model 5). Figure 1A shows the five fixation models constructed in our study. Figures 1B, C show the two modified intramedullary devices. The dimensions of the implant designs were provided by the relevant manufacturer. For Model 4 and 5, the diameter and length of the main intramedullary nail is 10 mm × 170 mm. The diameter and length of two neck screws and one sleeve are 10 mm × 65 mm, 6.4 mm × 70 mm, and 14 mm × 35 mm for Model 4 while the diameter and length of two neck screws are 10 mm × 95 mm, 6.4 mm × 80 mm for Model 5, respectively. In Model 4, the included angle of the thick neck screw and the main nail is 130° while the included angle of the two neck screws is 7.5°, respectively. In Model 5, the included angle of the thick neck screw and the main nail is also 130° while the included angle of the two neck screws is 60°, respectively.
Finite element setup
All three models had homogeneous and linear isotropic material features and were meshed with tetrahedral elements. In order to ensure the reliability of these models, a convergence study was conducted (Machado et al., 2014; McCartney et al., 2018). With maximum Degree of Freedom, field variables, such as strain energy and displacement, were within 5% for both types of elements and there was no maximum stress point. As shown in Table 1, with respect to material properties of the bones, the Young's modulus was defined as 16,800 MPa for cortical bones, 840 MPa for cancellous bones, and 110,000 MPa for implants while Poisson's ratio was 0.3 for cortical bones and Titanium alloy, and 0.2 for cancellous bones according to the literature (Li et al., 2018a). Table 2 showed the numbers of elements and nodes of five different fixation models. The implants were assigned with titanium material properties. All contact status was defined as frictional contacts, including the contact between fracture fragments and the contact between bone surface and implants. The friction coefficient of 0.4 was determined according to previous literature (Viceconti et al., 2000). As shown in Figure 2, three load conditions were simulated, including axial, bending, and torsion loads. In order to prevent rigid body movement during the analysis, the distal femur was fixed in all directions. In the axial load case, a 2,100 N load was acted axially on the femoral head representing the axial load compression (Zhang et al., 2011; Li et al., 2019). The load was exerted at the top of the femoral head. For the boundary of the torsion load case, a 15 Nm torsion load was acted on the surface of femoral head along the axis of the femoral neck and this represented the maximum load applied to the femoral head during normal human gait (Li et al., 2018b). As shown in Figure 2, in the bending load case, both femoral head and shaft of the femur were fixed in all degrees of freedom and a 175 N load was acted laterally on the femur from the front to simulate the four-point load bending (Li et al., 2018a). Marc Mentat (MSC Software, Santa Ana, CA) software was used for all finite element analysis and the analysis was solved by an implicit solver.
Parameters for analysis
The maximal displacement of models and fracture surface was assessed under axial, bending and torsion loads. The maximal stress of implants and bone for five models were also evaluated. All values were compared with the value of Model 1. Model 1 (three CSs in an inverted triangle configuration) was considered to be the control group as this implant had the most extensive clinical application over the past years and was considered to have good biomechanical stability (Kauffman et al., 1999; Haidukewych et al., 2004). The corresponding variation rates were calculated by the following formula: VR =(V1 − Vn)/V1×100%, where VR = variation rate, Vn = value for Model 2, 3, 4, or 5, and V1 = value for Model 1.
Results
Maximal displacement of five models
Maximal displacement of five models under axial, bending, and torsion load was shown in Figure 3. In order of the largest to smallest displacements under axial load of 2100 N, the five different fixation models were rated as follows: Model 1-3-2-5-4. For bending load of 175 N, it was rated as follows: Model 1-3-2-4-5. For torsion load of 15 Nm, it was rated as follows: Model 1-2-3-4-5. The maximum displacement of UFNFs fixed by the two modified intramedullary devices was smaller than that of CSs. The maximum displacement reduction of Model 4 and 5 relative to Model 1 reached 26.6% and 23.7% under axial load, 47.9% and 52.8% under bending load, 24.7% and 25.3% under torsion load, respectively.
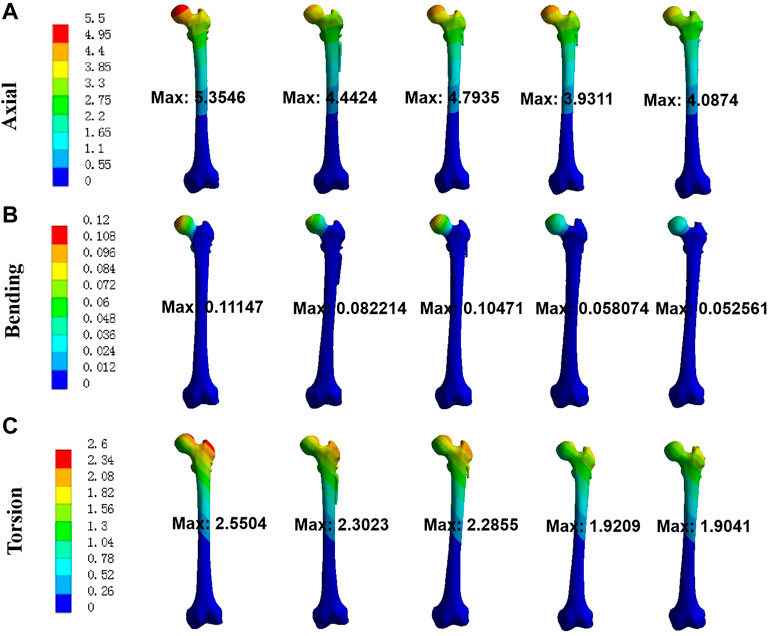
FIGURE 3. Maximal displacement of five models for three different loads. (A) axial; (B) bending; (C) torsion.
Maximal displacement of fracture surface for these models
The trends of maximum displacement of fracture surface were consistent with that of maximum displacement of models (Figure 4). The maximum displacement of fracture surface from Model 1 to 5 was 4.27 mm, 3.66 mm, 3.88 mm, 3.15 mm, and 3.11 mm under axial load, respectively. Compared with Model 1, the maximum displacement reduction of fracture surface for Model 4 and 5 was 26.3% and 27.2% under axial load. Moreover, under bending load, the maximum displacement of fracture surface was 0.029 mm, 0.017 mm, 0.019 mm, 0.011 mm, and 0.011 mm for Model 1 to 5 while under torsion load it was 2.14 mm, 1.94 mm, 1.92 mm, 1.59 mm, and 1.57 mm, respectively. The maximum displacement reduction of fracture surface for Model 4 and 5 relative to Model 1 was 61.9% and 61.1% under bending load while 26.0% and 26.7% under torsion load. The above results showed that the modified intramedullary implant had better biomechanical stability than CSs for treating UFNFs.
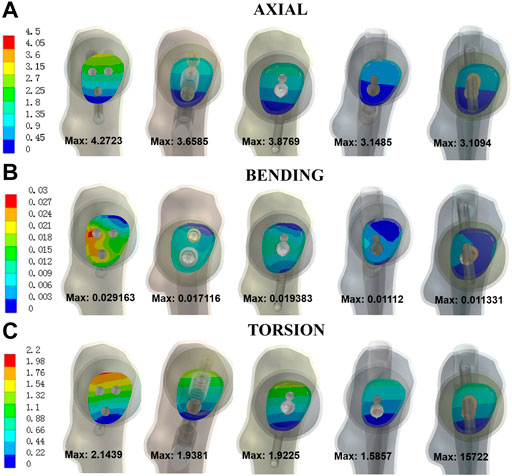
FIGURE 4. Maximal displacement of fracture surface for five models under three different loads. (A) axial; (B) bending; (C) torsion.
Stress at implants
Stress at implants was shown in Figure 5 for five different models. In order of the highest to lowest maximal stress under axial load, the five different fixation models were rated as follows: Model 2-1-3-5-4. For bending load, it was rated as follows: Model 3-2-1-4-5. For torsion load, it was rated as follows: Model 4-3-1-2-5. Except Model 4 under torsion load, the maximum stress of each modified intramedullary fixation implant is less than that of CSs, respectively. The maximum stress reduction of Model 4 and 5 relative to Model 1 was 51.8% and 44.5% under axial load while 16.2% and 31.8% under bending load, respectively. Besides, compared with Model 1, the maximum stress reduction of Model 5 was 38.6% under torsion load.
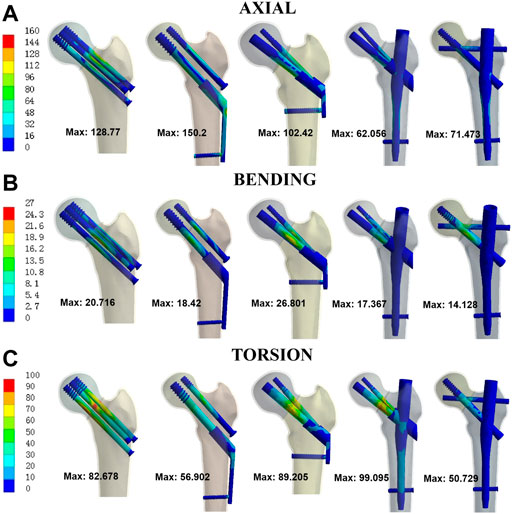
FIGURE 5. Von Mises stress at implants for three different loads. (A) axial; (B) bending; (C) torsion.
Stress at bone
As shown in Figure 6, the trends of stress at bone were consistent with that of stress at implants for UFNFs. The maximum stress at bone from Model 1 to 5 was 54.620 MPa, 107.49 MPa, 54.467 MPa, 43.195 MPa, and 26.547 MPa under axial load (19.609 MPa, 10.499 MPa, 10.532 MPa, 7.8989 MPa, and 7.7388 MPa under bending load; 32.220 MPa, 16.685 MPa, 15.405 MPa, 22.974 MPa, and 15.854 MPa under torsion load). Compared with Model 1, the maximum stress reduction acted on bone for Model 4 and 5 was 20.9% and 51.4% under axial load (59.7% and 60.5% under bending load; 28.7% and 50.8% under torsion load). The above results indicated that each modified intramedullary implant had more uniform stress distribution than CSs for treating UFNFs, respectively.
Discussion
Although the age range of hip replacement is wider than before, internal fixation is still the first choice for most young patients with femoral neck fractures. Weak blood supply and unstable fixation of the femoral head easily lead to non-union and necrosis of the femoral head after operation (Parker, 2000; Slobogean et al., 2015). Currently, surgeons focus on the extramedullary fixation for treating UFNFs, such as CSs, DHS, and FNS (Blomfeldt et al., 2005; Lee et al., 2007; Stoffel et al., 2017; Schwartsmann et al., 2018). Yet, there is no special intramedullary fixation device for the treatment of femoral neck fractures.
Several scholars have tried some modified techniques to fix UFNFs. Satish et al. used four CSs to fix unstable femoral neck fractures, in order to increase the whole stability (Satish et al., 2013). However, in clinical work it is difficult to arrange four CSs reasonably in cross section. Other scholars used three CSs and a medial auxiliary plate to fix such fractures (Ye et al., 2017; Zhuang et al., 2018). Although the stability of fixation has been significantly improved, this fixation method will increase trauma and even damage the blood supply of the femoral head in clinical work. FNS has been used clinically for several years, and some studies have shown that it has good biomechanical properties, and its performance is better than that of three CSs and DHS (Stoffel et al., 2017). Yet, FNS is an extramedullary fixation with a long level arm.
In view of this, some scholars explored whether intramedullary fixation of UFNFs can avoid the disadvantages of extramedullary fixation of such fractures. Guo et al. used intramedullary nails with cannulated screw fixation for the treatment of UFNFs (Guo et al., 2019). Their study showed that intramedullary fixation with cannulated screws had advantages for treating complicated femoral neck fractures in young and middle-aged patients. They used a combination of femoral reconstruction nails and cannulated screws in their study. However, it is difficult to insert the third or even fourth cannulated screw after the two cervical screws of the reconstruction nail have been inserted, especially when there is no robot assisted precise positioning or the patient’s femoral neck is thin. Röderer et al. investigated primary stability of the proximal femoral nailing anti-rotation (PFNA) for the indication of unstable medial femoral neck fractures (Röderer et al., 2011). They found PFNA achieved primary stability comparable to the dynamic hip screw blade. It also indicates that intramedullary fixation may be an option for the treatment of UFNFs. Yet, there is only one spiral blade at the femoral neck, and its anti-rotation ability is limited. Rupprecht et al. reported that compared with CSs and DHS, InterTan nail was more powerful for treating Pauwels type III femoral neck fractures (Rupprecht et al., 2011). Wang et al. conducted a biomechanical comparison of FNS, InterTan nail and three CSs for the treatment of Pauwels III femoral neck fractures (Wang et al., 2022). Based on their results, InterTan nail showed the highest axial stiffness and anteroposterior (AP) bending stiffness, followed by FNS, and then three CSs. However, the neck screws of InterTan nail are designed for parallel contact arrangement of two screws, which cannot achieve the most effective spatial distribution and fixation of the femoral neck section. In view of this, our team has tried to design two modified intramedullary fixation implants to better fix UFNFs.
Finite element analysis is a computer simulation method, which has been widely used to study implants for unstable femoral neck fractures (Peng et al., 2020; Zeng et al., 2020; Wang et al., 2022). It could evaluate the biomechanical properties of different implants by testing the stress and displacement data. We conducted finite element analysis of three CSs, DHS + AS, FNS, the modified IFNS, and the modified IIS for fixation of Pauwels type III femoral neck fractures. Our data demonstrated that the two modified intramedullary implants displayed the best biomechanical properties, followed by DHS + AS, FNS, and then three CSs under axial, bending, and torsion load. This is mainly because the two modified intramedullary implants have a short moment, which could provide enough mechanical strength and transfer the stress on the femoral head to the femoral shaft well. Besides, the intramedullary fixation is a central fixation, which makes the stress distribution of the internal fixation device uniform. There are two screws in the femoral head, which are distributed at a specific angle, so as to increase the anti-rotation effects. Both DHS and FNS are eccentric design, with long moment. In this way, the implants will bear relatively large compression and bending stress. Three CSs transfer torque through the interaction between the screws and the cancellous bones based on the “three-point support principle” (Wang et al., 2022). It should be emphasized that our modified IFNS is similar to classical FNS in design, but the difference is that we shorten the overall moment. This design is similar to the combination of an intramedullary nail and FNS. The finite element results also showed that the maximum displacement of the modified IFNS is less than that of FNS, and the same is true for the maximum stress of implants.
There are still some limitations to be further explored in this study. Firstly, in this research the cortical and cancellous bones were set isotropic, linear, and homogenous characteristics. In real-life, these bones own inhomogeneous characteristics (Couteau et al., 2001; Wong et al., 2021). To avoid excessive time consumption in the process of FEA modelling and considering that the current computer resources cannot be simulated using inhomogeneous models, thus isotropic and homogeneous characteristics were adopted. Secondly, three different loading conditions were simulated separately in our research. In reality, all three conditions might occur simultaneously while patients perform activities. It is another limitation of this research due to the limitation of computer resources at present and time consuming to simulate complex conditions. Further research needs to be carried out to simulate the abovementioned conditions which might provide some new insights to the understanding of biomechanical properties of patients treated by our modified implants. Thirdly, this research did not carry out model validation, which definitely is a common limitation of similar simulation studies. Yet, instead of accurate values of response, our team aimed to compare biomechanical properties and evaluate tendency on the basis of the same femur under the same loading and boundary conditions. As such, the lack of model validation might be justified to some extent. Many other scholars also applied the same method to simulate bones and implants with acceptable outcomes (Peng et al., 2020; Zeng et al., 2020; Wang et al., 2022). We will add the cadaver experiment in the further research, and finally apply the two modified implants to patients with unstable femoral neck fractures. Fourthly, when the two modified implants are used in clinical practice, the use of conventional size of proximal femoral rod may cause some new complications during operation, which needs further research.
Conclusion
The two modified intramedullary designs possessed better biomechanical performance as compared to CSs in the axial, bending, and torsion load cases. Therefore, this might provide some new options for trauma surgeons to deal with unstable femoral neck fractures.
Data availability statement
The original contributions presented in the study are included in the article/supplementary material, further inquiries can be directed to the corresponding authors.
Ethics statement
The ethics committee of the Xi'an Hong Hui hospital approved this study (No.202202002). The participant provided written informed consent to participate in this study. Written informed consent was obtained from the individual for the publication of any potentially identifiable images or data included in this article.
Author contributions
YL and TM designed the study. QH, CZ, HB, QW, ZL, and KZ searched relevant clinical data, analyzed and interpreted the data. QH wrote the manuscript. YL and TM contributed most in the revision of this manuscript. All authors approved the final version of the manuscript.
Funding
This study was supported by the National Natural Science Foundation of Shaanxi Province (2022JQ-757), the Clinical Application-oriented Medical Innovation Foundation from National Clinical Research Center for Orthopedics, Sports Medicine & Rehabilitation and Jiangsu China-Israel Industrial Technical Research Institute Foundation (2021-NCRC-CXJJ-PY-11), and the Medical research project of Xi’an Science and Technology Bureau (22YXYJ0020). The funding source has no role in study design, conduction, data collection or statistical analysis.
Conflict of interest
The authors declare that the research was conducted in the absence of any commercial or financial relationships that could be construed as a potential conflict of interest.
Publisher’s note
All claims expressed in this article are solely those of the authors and do not necessarily represent those of their affiliated organizations, or those of the publisher, the editors and the reviewers. Any product that may be evaluated in this article, or claim that may be made by its manufacturer, is not guaranteed or endorsed by the publisher.
References
Abdul Wahab, A. H., Wui, N. B., Abdul Kadir, M. R., and Ramlee, M. H. (2020). Biomechanical evaluation of three different configurations of external fixators for treating distal third tibia fracture: Finite element analysis in axial, bending and torsion load. Comput. Biol. Med. 127, 104062. doi:10.1016/j.compbiomed.2020.104062
Blomfeldt, R., Törnkvist, H., Ponzer, S., Söderqvist, A., and Tidermark, J. (2005). Internal fixation versus hemiarthroplasty for displaced fractures of the femoral neck in elderly patients with severe cognitive impairment. J. Bone. Jt. Surg. Br. 87, 523–529. doi:10.1302/0301-620x.87b4.15764
Chantarapanich, N., and Riansuwan, K. (2022). Biomechanical performance of short and long cephalomedullary nail constructs for stabilizing different levels of subtrochanteric fracture. Injury 53, 323–333. doi:10.1016/j.injury.2021.11.064
Couteau, B., Mansat, P., Estivalèzes, E., Darmana, R., Mansat, M., and Egan, J. (2001). Finite element analysis of the mechanical behavior of a scapula implanted with a glenoid prosthesis. Clin. Biomech. (Bristol, Avon) 16 (7), 566–575. doi:10.1016/s0268-0033(01)00029-8
Guo, J., Dong, W., Yin, B., Jin, L., Lin, Z., Hou, Z., et al. (2019). Intramedullary nails with cannulated screw fixation for the treatment of unstable femoral neck fractures. J. Int. Med. Res. 47 (2), 557–568. doi:10.1177/0300060518816185
Haidukewych, G. J., Rothwell, W. S., Jacofsky, D. J., Torchia, M. E., and Berry, D. J. (2004). Operative treatment of femoral neck fractures in patients between the ages of fifteen and fifty years. J. Bone. Jt. Surg. Am. 86-A, 1711–1716. doi:10.2106/00004623-200408000-00015
Hu, X. J., and Wang, H. (2017). Biomechanical assessment and 3D finite element analysis of the treatment of tibial fractures using minimally invasive percutaneous plates. Exp. Ther. Med. 14, 1692–1698. doi:10.3892/etm.2017.4629
Jitprapaikulsarn, S., Chantarapanich, N., Gromprasit, A., Mahaisavariya, C., and Patamamongkonchai, C. (2021). Single lag screw and reverse distal femur locking compression plate for concurrent cervicotrochanteric and shaft fractures of the femur: Biomechanical study validated with a clinical series. Eur. J. Orthop. Surg. Traumatol. 31, 1179–1192. doi:10.1007/s00590-020-02868-z
Kauffman, J. I., Simon, J. A., Kummer, F. J., Pearlman, C. J., Zuckerman, J. D., and Koval, K. J. (1999). Internal fixation of femoral neck fractures with posterior comminution: A biomechanical study. J. Orthop. Trauma. 13, 155–159. doi:10.1097/00005131-199903000-00001
Knobe, M., Altgassen, S., Maier, K. J., Gradl-Dietsch, G., Kaczmarek, C., Nebelung, S., et al. (2018). Screw-blade fixation systems in Pauwels three femoral neck fractures: A biomechanical evaluation. Int. Orthop. 42 (2), 409–418. doi:10.1007/s00264-017-3587-y
Knobe, M., Bettag, S., Kammerlander, C., Altgassen, S., Maier, K. J., Nebelung, S., et al. (2019). Is bone-cement augmentation of screw-anchor fixation systems superior in unstable femoral neck fractures? A biomechanical cadaveric study. Injury 50 (2), 292–300. doi:10.1016/j.injury.2018.10.038
Lee, Y. S., Huang, C. R., and Liao, W. Y. (2007). Surgical treatment of undisplaced femoral neck fractures in the elderly. Int. Orthop. 31, 677–682. doi:10.1007/s00264-006-0243-3
Leonardo-Diaz, R., Alonso-Rasgado, T., Jimenez-Cruz, D., Bailey, C. G., and Talwalkar, S. (2020). Performance evaluation of surgical techniques for treatment of scapholunate instability in a type II wrist. Int. J. Numer. Methods. Biomed. Eng. 36, e3278. doi:10.1002/cnm.3278
Li, J., Wang, M., Li, L., Zhang, H., Hao, M., Li, C., et al. (2018a). Finite element analysis of different configurations of fully threaded cannulated screw in the treatment of unstable femoral neck fractures. J. Orthop. Surg. Res. 13 (1), 272. doi:10.1186/s13018-018-0970-3
Li, J., Yin, P., Zhang, L., Chen, H., and Tang, P. (2019). Medial anatomical buttress plate in treating displaced femoral neck fracture A finite element analysis. Injury 50 (11), 1895–1900. doi:10.1016/j.injury.2019.08.024
Li, J., Zhao, X., Hu, X., Tao, C., and Ji, R. (2018b). A theoretical analysis and finite element simulation of fixator-bone system stiffness on healing progression. J. Appl. Biomater. Funct. Mater. 16, 115–125. doi:10.1177/2280800017750357
Machado, M. M., Fernandes, P. R., Zymbal, V., and Baptista, F. (2014). Human proximal femur bone adaptation to variations in hip geometry. Bone 67, 193–199. doi:10.1016/j.bone.2014.07.001
McCartney, W., MacDonald, B., Ober, C. A., Lostado-Lorza, R., and Gómez, F. S. (2018). Pelvic modelling and the comparison between plate position for double pelvic osteotomy using artificial cancellous bone and finite element analysis. Bmc. Vet. Res. 14 (1), 100. doi:10.1186/s12917-018-1416-1
Palm, H., Gosvig, K., Krasheninnikoff, M., Jacobsen, S., and Gebuhr, P. (2009). A new measurement for posterior tilt predicts reoperation in undisplaced femoral neck fractures: 113 consecutive patients treated by internal fixation and followed for 1 year. Acta. Orthop. 80 (3), 303–307. doi:10.3109/17453670902967281
Parker, M. J. (2009). Results of internal fixation of Pauwels type-3 vertical femoral neck fractures. J. Bone. Jt. Surg. Am. 91, 490–491.
Parker, M. J. (2000). The management of intracapsular fractures of the proximal femur. J. Bone. Jt. Surg. Br. 82 (7), 937–941. doi:10.1302/0301-620x.82b7.0820937
Peng, M. J., Xu, H., Chen, H. Y., Lin, Z., Li, X., Shen, C., et al. (2020). Biomechanical analysis for five fixation techniques of pauwels-III fracture by finite element modeling. Comput. Methods. Programs. Biomed. 193, 105491. doi:10.1016/j.cmpb.2020.105491
Ragnarsson, J. I., and Kärrholm, J. (1992). Factors influencing postoperative movement in displaced femoral neck fractures: Evaluation by conventional radiography and stereoradiography. J. Orthop. Trauma. 6 (2), 152–158. doi:10.1097/00005131-199206000-00004
Ragnarsson, J. I., and Kärrholm, J. (1991). Stability of femoral neck fracture. Roentgen stereophotogrammetry of 29 hook-pinned fractures. Acta. Orthop. Scand. 62 (3), 201–207. doi:10.3109/17453679108993593
Röderer, G., Moll, S., Gebhard, F., Claes, L., and Krischak, G. (2011). Side Plate fixation vs. Intramedullary nailing in an unstable medial femoral neck fracture model: A comparative biomechanical study. Clin. Biomech. 26, 141–146. doi:10.1016/j.clinbiomech.2010.09.020
Rupprecht, M., Grossterlinden, L., Ruecker, A. H., de Oliveira, A. N., Sellenschloh, K., Nüchtern, J., et al. (2011). A comparative biomechanical analysis of fixation devices for unstable femoral neck fractures: The intertan versus cannulated screws or a dynamic hip screw. J. Trauma. 71 (3), 625–634. doi:10.1097/TA.0b013e31820e86e6
Satish, B. R., Ranganadham, A. V., Ramalingam, K., and Tripathy, S. K. (2013). Four quadrant parallel peripheral screw fixation for displaced femoral neck fractures in elderly patients. Indian. J. Orthop. 47, 174–181. doi:10.4103/0019-5413.108912
Schwartsmann, C. R., Lammerhirt, H. M., Spinelli, L. F., and Ungaretti Neto, A. D. S. (2018). Treatment of displaced femoral neck fractures in young patients with DHS and its association to osteonecrosis. Rev. Bras. Ortop. 53, 82–87. doi:10.1016/j.rboe.2017.03.003
Slobogean, G. P., Sprague, S. A., Scott, T., and Bhandari, M. (2015). Complications following young femoral neck fractures. Injury 46 (3), 484–491. doi:10.1016/j.injury.2014.10.010
Stoffel, K., Zderic, I., Gras, F., Sommer, C., Eberli, U., Mueller, D., et al. (2017). Biomechanical evaluation of the femoral neck system in unstable Pauwels III femoral neck fractures: A comparison with the dynamic hip screw and cannulated screws. J. Orthop. Trauma. 31 (3), 131–137. doi:10.1097/BOT.0000000000000739
Viceconti, M., Muccini, R., Bernakiewicz, M., Baleani, M., and Cristofolini, L. (2000). Large-sliding contact elements accurately predict levels of bone implant micromotion relevant to osseointegration. J. Biomech. 33 (12), 1611–1618. doi:10.1016/s0021-9290(00)00140-8
Wang, Z., Yang, Y., Feng, G., Guo, H., Chen, Z., Chen, Y., et al. (2022). Biomechanical comparison of the femoral neck system versus InterTan nail and three cannulated screws for unstable Pauwels type III femoral neck fracture. Biomed. Eng. Online. 10 (1), 34. doi:10.1186/s12938-022-01006-6
Wong, D. W. C., Chen, T. L. W., Peng, Y., Lam, W. K., Wang, Y., Ni, M., et al. (2021). An instrument for methodological quality assessment of single-subject finite element analysis used in computational orthopaedics. Med. Nov. Technol. And. Devices 11, 100067. doi:10.1016/j.medntd.2021.100067
Ye, Y., Chen, K., Tian, K., Li, W., Mauffrey, C., and Hak, D. J. (2017). Medial buttress plate augmentation of cannulated screw fixation in vertically unstable femoral neck fractures: Surgical technique and preliminary results. Injury 48, 2189–2193. doi:10.1016/j.injury.2017.08.017
Zeng, W., Liu, Y., and Hou, X. (2020). Biomechanical evaluation of internal fixation implants for femoral neck fractures: A comparative finite element analysis. Comput. Methods. Programs. Biomed. 196, 105714. doi:10.1016/j.cmpb.2020.105714
Zhang, Y., Tian, L., Yan, Y., Sang, H., Ma, Z., Jie, Q., et al. (2011). Biomechanical evaluation of the expansive cannulated screw for fixation of femoral neck fractures. Injury 42 (11), 1372–1376. doi:10.1016/j.injury.2011.07.004
Keywords: biomechanical, intramedullary, femoral neck fracture, axial, bending, torsion, finite element model
Citation: Huang Q, Zhang C, Bai H, Wang Q, Li Z, Lu Y and Ma T (2023) Biomechanical evaluation of two modified intramedullary fixation system for treating unstable femoral neck fractures: A finite element analysis. Front. Bioeng. Biotechnol. 11:1116976. doi: 10.3389/fbioe.2023.1116976
Received: 06 December 2022; Accepted: 10 February 2023;
Published: 21 February 2023.
Edited by:
Eiji Tanaka, Tokushima University, JapanReviewed by:
Duo Wai-Chi Wong, Hong Kong Polytechnic University, Hong Kong SAR, ChinaAnderson Freitas, Hospital Regional do Gama, Brazil
Copyright © 2023 Huang, Zhang, Bai, Wang, Li, Lu and Ma. This is an open-access article distributed under the terms of the Creative Commons Attribution License (CC BY). The use, distribution or reproduction in other forums is permitted, provided the original author(s) and the copyright owner(s) are credited and that the original publication in this journal is cited, in accordance with accepted academic practice. No use, distribution or reproduction is permitted which does not comply with these terms.
*Correspondence: Yao Lu, drluyao@163.com; Teng Ma, gukemateng@126.com
†These authors have contributed equally to this work and share first authorship