From Understory to Canopy: In situ Behavior of Neotropical Forest Katydids in Response to Bat Echolocation Calls
- 1Department of Biological Sciences, Dartmouth College, Hanover, NH, United States
- 2Bioacoustics Research Program, Cornell Lab of Ornithology, Ithaca, NY, United States
- 3Smithsonian Tropical Research Institute, Balboa, Panama
Predator-prey interactions take place in complex environments, and research on the sensory ecology of predator-detection relies on understanding when, where, and how prey experience and respond to predator cues. Bats are significant nocturnal predators, and insects have evolved diverse strategies for avoiding predation by bats. While it is well-known that insects exhibit anti-bat strategies, from avoidance flight to reduced acoustic signaling, the specific conditions that elicit some of these behaviors are less well-known. To illuminate how insects respond to bats in nature, we studied how calling behavior changed when katydids experienced echolocation calls in a Neotropical forest. The diverse Neotropical bat community includes species that eavesdrop on prey sounds, such as the songs produced by male katydids. Previous research has shown that some katydid species respond to echolocation calls by reducing acoustic signaling. To capture the interactions of bats and katydids, we placed acoustic monitors at heights of 8, 16, and 24 meters above ground in 10 locations in the forest on Barro Colorado Island, Panama and recorded continuously for 24 h at each location. We randomly selected 250 recordings with echolocation calls and compared the acoustic spectrum of the forest before a bat arrived, when a bat was present, and after the bat was no longer detectable. We tested whether the response to bat calls changes with height, the family of bat producing the calls, the duration of the echolocation sequence, call amplitude, and call peak frequency. Bats appeared on ~50% of nighttime recordings, but echolocation calls that could have been produced by eavesdropping bats were rare (<4% of calls). Insect response to bats was nuanced and context-dependent. Despite the rarity of truly dangerous predator cues, echolocation decreased insect sound at several frequencies and heights. Insect response was not uniform, and in many cases echolocation calls had little effect on insect activity, perhaps reflecting the fact that echolocation calls were an inconsistent cue for the presence of eavesdropping bats. These nuanced responses raise interesting questions about predator detection in noise and provide valuable context for laboratory investigations on the sensory ecology of how individual prey species respond to predator cues.
Introduction
Detecting and avoiding predators is a fundamental selective pressure on sensory systems and behavior (Endler and Basolo, 1998; Fullard, 1998; Lacalli, 2001), but predator detection and avoidance is often studied in laboratory contexts that cannot capture the full complexity of natural environments (Calisi and Bentley, 2009). Therefore, to understand the evolution and maintenance of anti-predator behaviors, it is important to investigate prey responses both in the lab and the field. Quantifying how prey detect and respond to predators in natural environments is often logistically challenging because these interactions occur in habitats that are structurally complex and contain many interacting species (Belwood and Morris, 1987; Williams et al., 2004; Staller et al., 2005). In addition, the behavior, physiology and responses of organisms are affected by variables such as weather, light level, and reproductive stage (Thompson, 1978; Christian and Tracy, 1981; Berger, 1991; Culler et al., 2015). Without information on the natural range of behaviors and responses, scenarios used in laboratory investigations might represent atypical cases or extreme conditions. Understanding the range of natural predator stimuli and prey responses is foundational to choosing appropriate experimental stimuli and testing paradigms for more controlled studies of behavior, physiology, and sensory system evolution.
Bat-insect interactions provide an excellent opportunity for studying predator-prey interactions due to the almost exclusive use of sound for both predator and prey detection (Conner and Corcoran, 2012; Yager, 2012; ter Hofstede and Ratcliffe, 2016). Bats produce high-frequency echolocation calls to orient in their environment and locate insect prey while in flight. The intense predation pressure exerted on insects by bats (Kalka et al., 2008; Williams-Guillén et al., 2008; Boyles et al., 2011) has selected for the evolution of ultrasound sensitive ears and ultrasound-triggered defensive behavior in at least six orders of insects (Yack and Dawson, 2008). The combination of lab and field studies on insect ultrasonic hearing (Roeder and Treat, 1957; Yager and Spangler, 1995; Schul and Sheridan, 2006; Yager and Svenson, 2008), anti-bat behavior (Rydell et al., 1997; Yager et al., 2000; Rosen et al., 2009; Römer et al., 2010; Barber and Kawahara, 2013) and predator-prey interactions (Miller and Olesen, 1979; Ghose et al., 2009; Corcoran and Conner, 2012) have revealed enormous variation in insect defenses against bats. This variation suggests multiple outcomes of arms races between bats and insects depending on the natural history of the predator and prey species involved.
Orthopterans, the insect group that includes crickets and katydids, are especially well-suited for studying predator-prey dynamics because both bats and prey produce and respond to acoustic signals (Nolen and Hoy, 1986; Faure and Hoy, 2000; ter Hofstede and Fullard, 2008; Jones et al., 2011). Male crickets and katydids produce songs to attract females. Bats prey on orthopterans in many environments, but in Neotropical forests, bat predation is particularly intense, especially for insects that produce sound (Belwood and Morris, 1987; Belwood, 1988a,b; ter Hofstede et al., 2017). The Neotropical bat community is one of the most diverse in the world, with species feeding on everything from fruit and nectar, to insects, vertebrates and even blood (Kalko et al., 1996; Bernard, 2002). The majority of insectivorous bat species in Neotropical communities are aerial insectivores, catching insect prey in flight (Denzinger et al., 2018). However, there are also a number of bat species in the family Phyllostomidae that eavesdrop on the communication signals and incidental sounds produced by insects to detect and locate prey and then glean them off vegetation (Tuttle et al., 1985; Belwood, 1988a,b; Falk et al., 2015). These gleaning bats present a particularly serious threat for orthopterans that attract mates using acoustic signals.
Orthopterans, however, are not defenseless. Many can hear bats and modify their behavior in response (Moiseff et al., 1978; ter Hofstede and Fullard, 2008). Crickets and katydids in flight will veer away from the echolocation calls of a bat (Moiseff et al., 1978; Nolen and Hoy, 1984; Libersat and Hoy, 1991; Schulze and Schul, 2001). In Neotropical forest katydids, many species have very low signal repetition rates and produce very little total sound (<5 s/night in some species) (Belwood, 1988a, 1990; Symes et al., 2016). Calling cessation provides a particularly useful lens for assessing prey responses to predator cues because the reaction of the insect and the dynamics of the predator-prey interaction can be captured using acoustic recording alone. Laboratory investigations of Neotropical katydid responses to the echolocation calls of gleaning bats have yielded mixed results, with some katydid species reducing call production while others persist (ter Hofstede et al., 2010). The variable laboratory results suggest that responses in the field may vary also, potentially in ways that shed light on why individual katydid species respond in the way that they do.
By placing acoustic monitors in a Neotropical forest, we used the passage of bats as a natural experiment to test whether katydid call cessation is a common response to predator cues in nature, and to assess the conditions under which it occurs. This in situ design allows us to infer how insects respond when they are experiencing a natural rate and pattern of bat exposure. In addition, recording in the forest captures the behavior of insects when they have a natural range of hiding locations, vegetation density, exposure to conspecific and heterospecifics cues, and spatial information provided by bat echolocation calls. To test how katydids respond to predator cues, we quantified the acoustic profile of the forest before, during and after bat echolocation sequences recorded on acoustic monitors.
In addition to the overall response of katydids to bat calls, we investigated specific parameters that might influence whether katydids respond to bat calls. First, we tested whether katydid responses to bat echolocation calls differ by height in the forest. For example, katydids might show greater responses to bat calls at intermediate heights where vegetation is expected to be less dense than in the understory and canopy (Marten and Marler, 1977). Second, we tested whether katydid responses differ depending on the family of bat or the peak frequency of the echolocation calls. Some bat species produce quasi-constant frequency calls (e.g., Emballonuridae) whereas others use downward frequency modulated sweeps (Phyllostomidae, Vespertilionidae). The peak frequency of echolocation calls varies across species in taxa that produce each type of echolocation call (Fenton et al., 1999; Jung et al., 2007; Surlykke and Kalko, 2008; Zamora-Gutierrez et al., 2016). If katydids are capable of differentiating among bat calls, the strongest insect response is predicted to occur in response to echolocation calls of the Phyllostomidae, the bat family that is known to contain eavesdropping predators. Because phyllostomids produce relatively high frequency calls, katydids might also be more responsive to higher than lower frequency calls. Third, we considered two parameters that provide information about the risk of an individual bat: amplitude, which provides information about proximity of the predator, and the duration of the detected bat pass, which might indicate a bat hunting in the area.
Materials and Methods
Study Site
To quantify the acoustic interactions of Neotropical bats and katydids, we generated audio recordings on Barro Colorado Island, Panama (BCI). The forest on BCI is primarily secondary growth tropical lowland rainforest (Ziegler and Leigh, 2002). We selected 10 sites that represent the mix of closed canopy and small gaps present on BCI and placed audio recorders at three heights in each of these sites.
Recording Insect and Bat Activity in the Forest
We used two towers and eight canopy emergent trees as recording sites (Figure S1). At each site, to sample as much of the height distribution as possible, we placed recording equipment at three heights (8, 16, and 24 m ± ca. 1 m) relative to the base of the tree or tower. In towers, the equipment was secured directly to the structure with the microphones pointed horizontally away from the tower. In trees, we climbed the tree, placed a pulley on a branch above 24 m in height and used a distance-calibrated rope to raise and lower recording equipment in protective cases that held the microphone in a horizontal position. At each site, we recorded continuously for 24 h. Recordings were made using acoustic monitors with ultrasound sensitive microphones (D500X, Pettersson Elektronik AB, Sweden). The trigger sensitivity was set very low so that the recorders were triggered continuously. The recorder created a file each time it was done saving the previous file, generating approximately one 20 s.wav file per minute for 24 h. After each of the 10 sites had been sampled, we repeated the sampling, resulting in a second 24-h recording from each site approximately 2 weeks after the first.
Measuring Frequency Structure of Katydid Community
To characterize the signals of the BCI katydid community, we captured male katydids, identified them, and recorded the sounds they produced. Katydids were collected by searching vegetation in the forest and catching individuals that flew to lights. Katydids were identified to species using several published resources (Nickle, 1992; Naskrecki, 2000; Cigliano et al., 2018). Male katydids were recorded in buildings on BCI with large screen windows so that they experienced ambient temperature, humidity, light and sounds of the forest but were protected from rain and predation during recording.
Male katydids were placed individually in cylindrical mesh cages that do not interfere with sound transmission across the frequencies of interest. A microphone (CM16, Avisoft Bioacoustics) responsive to frequencies between 3 and 100 kHz was positioned 30 cm from and pointing at the cage. Microphones were connected to a high-speed data acquisition board (UltraSoundGate 416, Avisoft Bioacoustics), which was connected by USB to a laptop computer running RECORDER software (Avisoft Bioacoustics). Recordings were triggered by the sound produced by katydids, with a pre-trigger time of at least 1 s to record the entire call.
To determine the typical frequencies produced by katydids on BCI, we analyzed one call per individual for three individuals per species for 34 katydid species (see Table S1 for species). High quality recordings were filtered with the inverse of the microphone frequency response to obtain natural relative amplitudes for each frequency. These recordings were high pass filtered at 7 kHz, a frequency that was lower than the lowest frequency in any of the calls. We then used the spec function of the R seewave package to extract the spectral distribution for each recording (Sueur et al., 2008) and took the median of these spectral distributions to represent the frequency distribution of the community.
Quantifying Bat Presence
To determine how often katydids are exposed to bat echolocation calls, we randomly selected five recordings from each site from each height and each sampling event. The recordings were randomly selected during the nighttime hours of 18:00 to 06:00 with an equal number of recordings drawn from each site and height. This selection process resulted in a sample of 300 recordings that were then scored for whether bat echolocation calls were present or absent. Sound files were opened in SASLab Pro sound analysis software (Avisoft Bioacoustics, Germany) and spectrograms were visually screened at 1 s durations. To identify echolocation signals, we searched the literature for publications describing echolocation signals for the 76 bat species documented on BCI (Denzinger et al., 2018). Signals in recordings that matched the shapes, durations, repetition rates and frequency ranges of documented bat calls were classified as bat echolocation calls. Signals that fell outside the range of one of these parameters were not classified as bat echolocation calls.
Quantifying Katydid Responses to Bat Passes
Our goal was to test whether katydid calling patterns change when bats are present. The katydid chorus consists of a few calls of individuals near the microphone that can be identified to species as well as the calls of more distant individuals that cannot be identified to species because the calls are quieter and are degraded by passage through, and reflection by, the vegetation. Therefore, instead of testing whether individual katydids stopped singing when bats were present, we tested whether the acoustic profile of the forest was changed by the passage of bats, and specifically whether the range of frequencies typical for katydid acoustic signals decreased in amplitude, reflecting calling cessation by some individuals. To our knowledge, this is a novel analysis approach and one that may be broadly applicable in a variety of organisms.
We identified focal recordings that contained echolocation calls indicating a bat pass. We obtained five focal recordings from each height at each site for each sampling interval for a total of 300 possible recordings (5 focal recordings × 3 heights × 10 sites × 2 sampling intervals). Each recording was obtained by first selecting a random number, finding the corresponding recording, and then progressing through the recordings in sequence until we arrived at a recording that contained echolocation calls. Because power to one recorder failed in the middle of recording and some sites did not have enough recordings with sufficient time before the first bat call to collect a complete set of 5 recordings, the extracted sample was 250 recordings that contained echolocation calls. For each focal recording, we identified bats to family, genus or species and obtained the time for the first and last detectable echolocation call on the spectrogram as well as the time, amplitude, and dominant frequency of the highest amplitude echolocation call. We used a similar screening process to select control recordings that did not contain bat calls, allowing us to conduct parallel analyses on these recordings.
To assess changes in acoustic profile, we compared the energy spectrum before the arrival of the bat against the energy spectrum when the bat was present (Figure 1). From each focal recording, we compared two windows of time, a “before bat” window and a “during bat” window, each lasting 3 s. The before bat window spanned from 5 to 2 s before the first detectible echolocation call on the recording. The during bat window centered on the loudest echolocation call and included a window of 1.5 s on either side of this time. These same time windows were applied to the control recordings to assess whether amplitude differed between two time points when bats were not present. To determine how quickly the insect community recovered, we identified a third window of 3 s that spanned from 2 to 5 s after the last detectible echolocation call. Using the R packages seewave and tuneR, we extracted these windows of time from the full recording and used the meanspec function to find the mean spectral energy present in each frequency bin from 0 to 150 kHz (256 bins, each 0.59 kHz) (Sueur et al., 2008; Ligges et al., 2018).
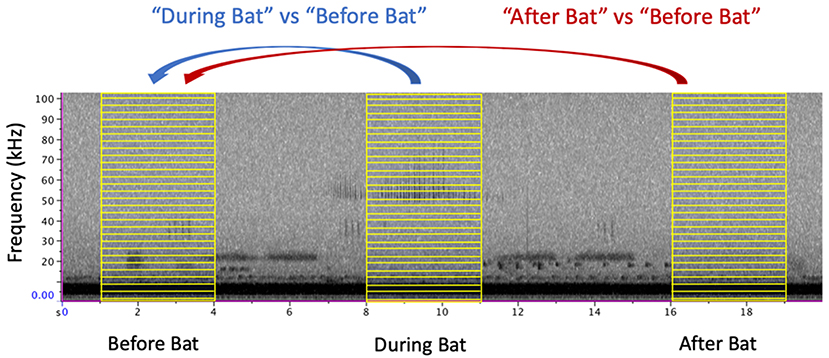
Figure 1. Sample windows were comprised of a 3 s window of sound. The “Before Bat” window ended 3 s before the first detectible echolocation call and the “After Bat” window began 3 s after the last detectible echolocation call. The “During Bat” window was centered on the highest amplitude echolocation call. For clarity of illustration, only a subset of the frequency bins are shown (yellow boxes).
Sound amplitude is typically reported using the decibel scale, which reflects the logarithmic nature of amplitude perception in humans and at least some other animals (Brumm, 2013). On the decibel scale, the amount of sound energy that is needed to increase amplitude by 6 dB is not equal to the amount by which sound energy must decrease to result in a 6 dB amplitude decrease. The nature of this measurement makes numeric means and standard deviations an inaccurate representation of amplitude. Consequently, statistics on decibel level were performed using non-parametric tests.
To analyze spectral changes associated with bat passes, we used paired Wilcoxon rank sum tests, a non-parametric analog of a paired t-test. We tested for significant changes at 5 kHz intervals from 10 to 30 kHz, frequencies representative of katydid calls. Analyzing calls at 5 kHz intervals allowed us to test whether insects that called with high peak frequencies had a different response to echolocation calls than insects that called with a lower peak frequency. The use of 5 kHz bins also allowed us to isolate the bin that contained the low harmonic of emballonurid bat echolocation calls. Analyzing the data by frequency increased the number of tests that we performed, increasing the probability of finding significant differences, but also decreased the power of the tests, which decreased the probability of detecting differences. Wilcoxon rank sum tests were used to compare the two time samples within a recording for the following comparisons: before vs. during bat, before vs. after bat, and the control analysis that compared two times in recordings that did not contain bat echolocation calls. Non-parametric Spearman's rank correlations were used to test for correlations between the strength of insect response to bats and three acoustic characteristics of the echolocation calls: duration of the bat pass (time from the first to the last call detectable on the spectrogram), amplitude (measured as peak-to-peak voltage from the oscillogram), and peak frequency (frequency with the most energy, as measured from the power spectrum).
Due to the combination of tests that include both independent and partial overlap of samples and also recordings that presumably include both katydid species that produce narrowband calls at specific frequencies and those that produce broadband calls across all frequencies, we do not use corrected alpha values for multiple tests. We report p-values and interpret these values using an alpha value of 0.05, but note that readers should consider the possibility of non-independence between tests at adjacent frequencies and pooled samples compared to tests by height and bat family.
Results
Frequency Structure of the Katydid Community
The katydid community of Barro Colorado Island produces calls that occupy a range of frequencies (Figure 2). Most of the sound energy occurs between 10 and 30 kHz, although some species produce frequencies of up to 80 kHz (ter Hofstede et al., 2010; Montealegre, 2012).
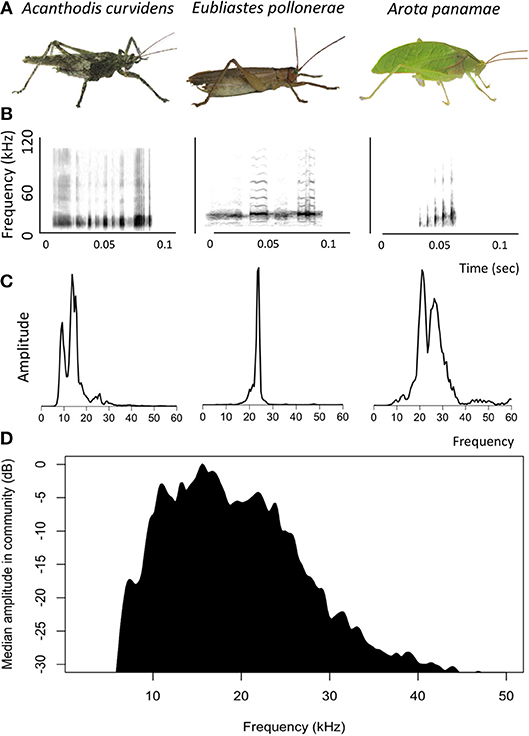
Figure 2. Acoustic signals of katydids recorded on Barro Colorado Island, Panama. (A) Photographs of three katydid species. (B) Spectrograms of the calls of three katydid species. (C) Power spectra of the calls of three katydid species. (D) Median power spectrum of 34 katydid species common on Barro Colorado Island, Panama (three individuals per species).
Presence of Bats
In ambient recordings, bats were pervasive, appearing in 46% of randomly selected recordings between 18:00 and 06:00 (51% of recordings at 8 m, 45% at 16 m, and 43% at 24 m). The probability of bats appearing on the recording did not differ by height ( = 1.39, p = 0.50).
Of the 250 recordings used to measure changes in frequencies typical of katydid calls, we were able to assign approximately 92% of echolocation recordings to bat family (Figure 3). In order of abundance, families included Emballonuridae (N = 130), Vespertilionidae (N = 70), Mormoopidae (N = 37), Phyllostomidae (N = 7), and Molossidae (N = 1). Within Vespertilionidae, we were able to identify all 70 recordings as belonging to bats in the genera Myotis or Rhogeesa. Within Emballonuridae, we identified calls of Saccopteryx bilineata (N = 53), Saccopteryx leptura (N = 16), Centronycteris centralis (N = 36), Cyttarops alecto (N = 16), and Peropteryx macrotis (N = 1). Within Mormoopidae, we were also able to identify calls of three bat species: Pteronotus gymnonotus (N = 22), Pteronotus parnellii (N = 14), and Pteronotus personatus (N = 1). Peak frequency of the bat echolocation calls ranged from 19.7 to 78.1 kHz, with a median of 46.6 kHz (25th/75th quantile spread: 42.9–53.5 kHz).
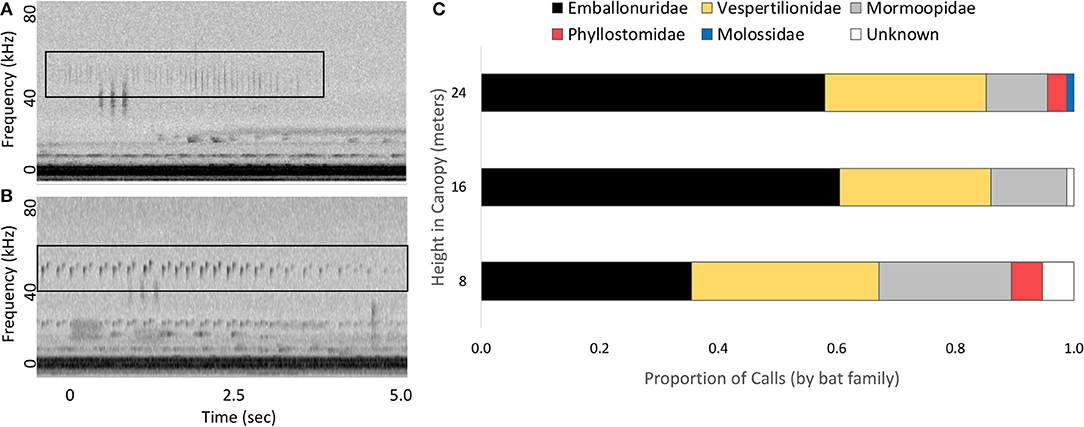
Figure 3. Echolocation calls of bats recorded on Barro Colorado Island, Panama. Example echolocation calls from two bat families (A: Phyllostomidae, B: Emballonuridae, echolocation calls indicated with black boxes) and the proportion of echolocation calls at each height produced by each family of bats (C).
Change in Frequency Structure When Bats Pass
To test whether there is a change in acoustic activity when echolocation calls appear on recordings, we compared the amount of energy at focal frequencies for: (1) two time bins of 3 s in a recording that did not contain a bat pass, (2) the time bin before and the time bin during a bat pass, and (3) the time bin before and the time bin after a bat pass. In randomly selected recordings that do not contain bat echolocation calls, there were no significant differences in the amount of energy at frequencies between 10 and 30 kHz for the two time selections (Wilcoxon paired rank sum tests, p > 0.05; Figure 4A). In recordings with bat echolocation calls, sound levels decrease in a subset of the frequency bands produced by katydids during the bat pass (Figure 4B). The decrease was significant at 10 kHz (V = 18,309, p = 0.011) and 30 kHz (V = 18,128, p = 0.017). The increase in amplitude at ~20 kHz is due to the lower harmonic of bat echolocation calls, primarily those produced by the Emballonuridae, which were abundant in our sample. For comparisons of time bins before and after bat echolocation calls, there was a significant decrease in energy at 30 kHz (V = 13,213, p = 0.008) and a trend toward decreasing amplitude at 10 kHz (V = 12391, p = 0.068).
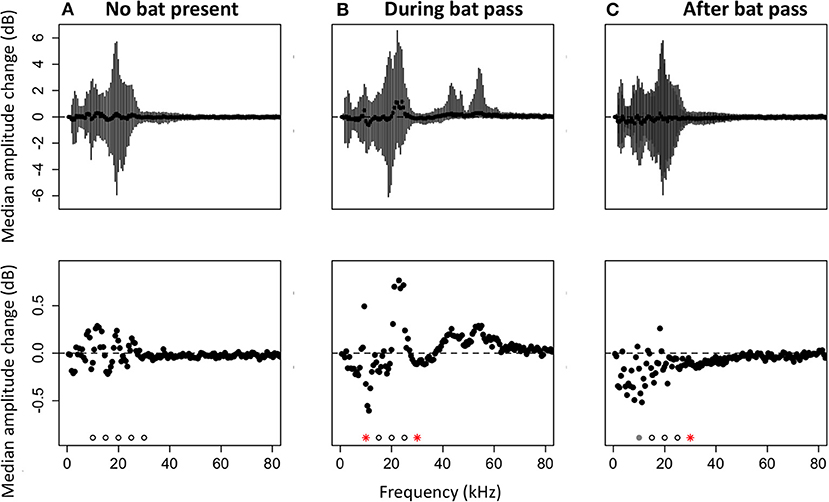
Figure 4. Median changes in amplitude by frequency. Negative values indicate less energy in the second time bin. (A) Median amplitude change between two time bins in a recording without echolocation calls (N = 246 recordings). (B) Median amplitude change comparing time bins before and during an echolocation sequence of a bat (N = 250 recordings). (C) Median amplitude change comparing time bins before and after an echolocation sequence of a bat (N = 210 recordings). Top panels: medians with error bars representing the 25th to the 75th percentile. Bottom panels: same data as top panels, but scaled in to show just the median values. Significance was tested at 5 kHz intervals from 10 to 30 kHz. Significant differences are shown with red asterisks (Wilcoxon paired ranks sums tests, p < 0.05), marginally significant differences are shown with gray circles (0.05 < p < 0.1), and tests that were not significant are shown with open circles.
The strength of the acoustic response to bats also differed with the height of the recorder in the forest and the family of bat (Figures 5, 6). We tested for significant decreases in energy at 10, 15, 20, 25, and 30 kHz at each of the three heights of the recorders. When comparing time windows before and during bat echolocation calls, there was a significant decrease in amplitude at 30 kHz at 24 m in the canopy (V = 2,478, p = 0.015, N = 88), there were no significant changes in amplitude at any frequency at an intermediate height of 16 m (N = 86), and there was a significant decrease in energy at 10 kHz at 8 m (V = 1858, p = 0.021, N = 76; Figure 5). When comparing time bins before and after a bat pass, there were significant decreases in energy at all frequencies except 15 kHz at a height of 24 m (10 kHz: V = 1,757, p = 0.040; 20 kHz: V = 1,747, p = 0.045; 25 kHz: V = 1,859, p = 0.011; 30 kHz: V = 1,815, p = 0.020; N = 75), there were no significant changes in amplitude at any frequency at an intermediate height of 16 m (N = 67), and there were significant decreases in amplitude at 8 m for 25 kHz (V = 1,419, p = 0.040) and 30 kHz (V = 1,464, p = 0.021) and a trend toward decreasing amplitude at 20 kHz (V = 1,419, p = 0.095).
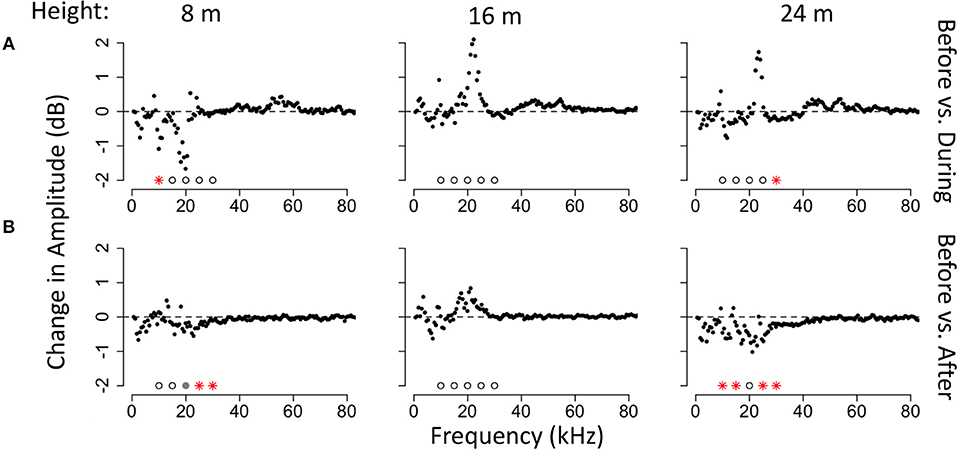
Figure 5. Median changes in amplitude by frequency and height of the recorder. (A) Median amplitude change comparing time bins before and during a bat echolocation sequence. (B) Median amplitude change comparing time bins before and after a bat echolocation sequence. Significance was tested at 5 kHz intervals from 10 to 30 kHz. Significant differences are shown with red asterisks (Wilcoxon paired ranks sums tests, p < 0.05), marginally significant differences are shown with gray circles (0.05 < p < 0.1), and tests that were not significant are shown with open circles.
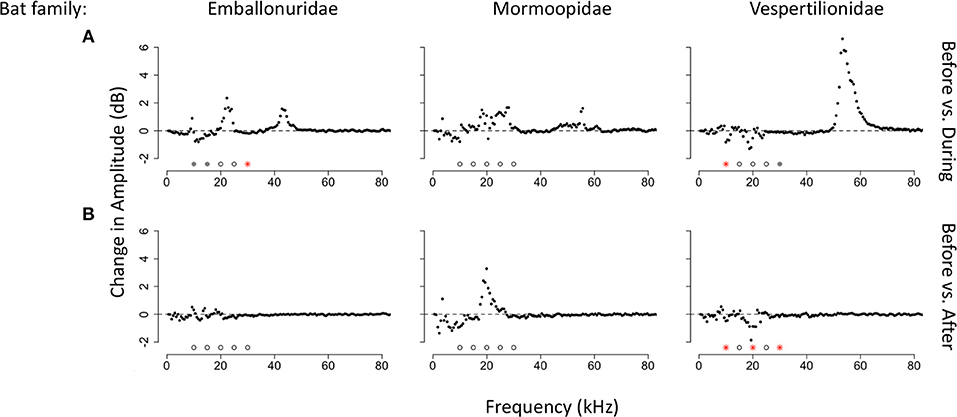
Figure 6. Median changes in amplitude by frequency and bat family. (A) Median amplitude change comparing time bins before and during a bat echolocation sequence. (B) Median amplitude change comparing time bins before and after a bat echolocation sequence. Significance was tested at 5 kHz intervals from 10 to 30 kHz. Significant differences are shown with red asterisks (Wilcoxon paired ranks sums tests, p < 0.05), marginally significant differences are shown with gray circles (0.05 < p < 0.1), and tests that were not significant are shown with open circles.
We also tested for changes in amplitude for the three families of bats with the largest sample sizes in our recordings. When comparing time windows before and during bat echolocation calls, calls of bats from the family Emballonuridae significantly decreased the amplitude of the 30 kHz band (V = 5,747, p < 0.001, N = 130; Figure 6A) with a trend toward reducing insect sound at 10 kHz (V = 4924, p = 0.061) and 15 kHz (V = 4,941, p = 0.056), whereas there were no significant differences at any frequency when comparing time bins before and after emballonurid bat passes. Calls of bats in the family Mormoopidae did not result in detectible decreases in amplitude at any frequency either during (N = 37; Figure 6B) or after echolocation sequences (N = 33). Calls produced by bats of the family Vespertilionidae resulted in a significant decrease at 10 kHz (V = 1,566, p = 0.016, N = 69; Figure 6C) and showed a trend toward reducing sound at 30 kHz (V = 1,460, p = 0.066) during bat passes, and caused significant decreases in amplitude at 10 kHz (V = 1,226, p = 0.011), 20 kHz (V = 1,147, p = 0.044) and 30 kHz (V = 1161, p = 0.035) after bat passes.
Amplitude of the echolocation calls and duration of the bat pass both affected the strength of insect response at some frequencies (Table 1). Louder echolocation calls led to a greater amplitude decrease at 10, 20, and 25 kHz, whereas longer echolocation call sequences led to a greater decrease in amplitude at 20 kHz. When the peak frequency of echolocation calls was higher, there was a greater response at 25 kHz.
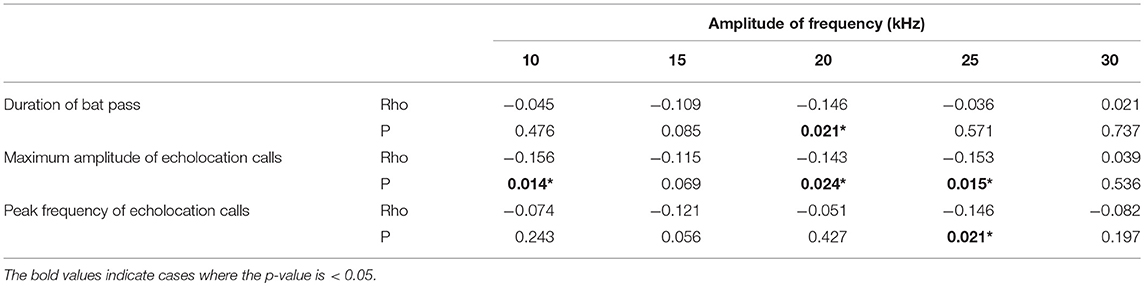
Table 1. The relationship between acoustic characteristics of bat echolocation calls and the change in amplitude of ambient sound by frequency (Spearman rank correlation coefficients and p-values).
Discussion
Bats are common and diverse in Neotropical forests (Denzinger et al., 2018) and the insect response to bat echolocation calls is variable and context-dependent (Figures 4–6). While it is tempting to infer that katydids consistently stop calling when they hear bats, the observed behavior is far more nuanced. A striking feature of the acoustic environment is the sheer prevalence of echolocation calls. Between 18:00 and 06:00, nearly half of the 20 s recordings contained bat echolocation calls, meaning that insects are exposed to this cue repeatedly throughout the night. Of these echolocation calls, less than 4% are produced by bats in the family Phyllostomidae, the family that is known to contain eavesdropping bats that hunt katydids by the sounds that they make (Jones et al., 2014; Falk et al., 2015). Even within the phyllostomids, many species are not known to engage in eavesdropping behavior and instead feed on fruit or flying insects (Fleming, 1991; Teixeira et al., 2009; Weinbeer et al., 2013). Consequently, extremely few of the echolocation calls that are experienced by katydids come from predators that are a threat to these insects when they are singing. The variable results observed in this study might be a reflection of the rare enemy effect, where the strength of selection for defensive adaptations is weak due to the rarity of the predator (Dawkins and Krebs, 1979). In addition, the modest response of insects may reflect reliance on a passive defense (low baseline calling or calling from dense vegetation) rather than an active defense (reduced calling in response to echolocation calls) (Belwood and Morris, 1987). Many of the insect species that produce many calls are relatively small in size, while larger insects that may be more desirable prey for bats produce fewer calls (Symes et al. in prep).
If most of the echolocation calls heard in the forest are not a threat, it raises the question of why any katydid would reduce signaling in response to echolocation calls, potentially reducing its opportunity to attract mates (Sih et al., 1990; Magnhagen, 1991; Candolin, 1998). Phyllostomid bats produce highly directional calls with relatively low amplitude (Brinkløv et al., 2011; Surlykke et al., 2013). Consequently, although phyllostomid echolocation calls are infrequently detected on recorders, it does not necessarily mean that eavesdropping predators are rare in the environment. Using mist nets baited with singing katydids, Belwood captured an average of 1.85 eavesdropping bats per hour (Belwood, 1988a). Many eavesdropping bats will perch and produce low amplitude echolocation while waiting for prey sounds (Surlykke et al., 2013), meaning that even if eavesdropping species are relatively rare on passive recordings, insects that produce sound may attract these predators and experience dangerous echolocation calls more often, driving the evolution of behavioral responses to echolocation calls even when they are produced by other types of bats. A second, non-mutually exclusive, possibility is that even if echolocation calls are an inconsistent indication of risk, the cost of calling reduction is low enough to make call cessation either a favorable behavior or nearly cost-neutral (Wolf et al., 2007). If insects are long-lived and not highly mobile, this lost window of mate signaling may have negligible effects on mate attraction relative to the potential cost of predation. Many Neotropical katydids also tremulate, producing vibrational signals in the substrate to attract females and they might adjust their reliance on acoustic or vibrational signaling depending on predation risk (Römer et al., 2010).
There were differences in the response of the katydid community to bat echolocation calls by height in the forest. Echolocation calls decreased insect sound at 10 kHz low in the canopy (8 m) and at 30 kHz high in the canopy (24 m), but there were no differences in amplitude at the intermediate height (16 m). This result goes against our predictions based on the idea that katydids would be at greater risk of gleaning bats in areas of the forest with less dense vegetation. We do not, however, know the absolute amplitudes of sounds at the different heights in the canopy, and it is possible that the difference in insect response by frequency and height might reflect where different insect calls are more prevalent or which bat species are hunting in a given habitat. If the amplitude of the insects does not change, this could mean that insects do not respond, or that no insects with that frequency are present at that height. Although we had only a few recordings of phyllostomid bat calls and therefore we cannot quantify vertical distribution of this family, it is interesting to note that these bats were only recorded by the lowest and highest recorder, but not by the intermediate height recorder, in our random sample. Within eavesdropping bats, different bat species are known to target different acoustic characteristics of prey calls (Falk et al., 2015), and different bat species are found at different heights in the forest (Bonaccorso, 1979; Bernard, 2001) suggesting that the risk of different call types may co-vary with where particular species of bats hunt.
There were also differences in the response of the katydid community by bat family. We recorded too few echolocation sequences of phyllostomid bats to assess changes in amplitude to this group. Bats in the family Vespertilionidae triggered more significant decreases in insect sound than bats in the families Emballonuridae and Mormoopidae. Vespertilionids are aerial insectivores, but they produce downward frequency-modulated sweeps similar to phyllostomids. The acoustic similarity between the calls of phyllostomids and vespertilionids might be one reason why they elicit greater amplitude reductions in sound than quasi-constant frequency calls typical of the emballonurids. Some of the insect responses to emballonurids, however, might also be masked by the presence of lower frequency harmonics (~20 kHz) in the echolocation calls of these bats. Insect response at these frequencies is particularly apparent when examining the acoustic profile of the forest 3 s after the bat has left the area. In this before and after comparison, it is possible to see that sound is reduced at 20–25 kHz after the bat calls are no longer detected, suggesting that insect response at these frequencies is masked by the harmonic of the bat echolocation call during the bat pass.
The acoustic characteristics of the echolocation calls had strong effects on the responses of insects. Specifically, higher amplitude echolocation calls were associated with stronger insect response across a range of frequencies (Table 1), suggesting that cues related to predator proximity, more than predator species, drive insect responses to bat cues. TN-1, or the T-cell, is a well-studied auditory interneuron in katydids that is broadly tuned to high frequency sound and is thought to function in bat detection in certain contexts (Faure and Hoy, 2000; Schul and Schulze, 2001). It adapts (i.e., stops responding to stimuli) in response to highly repetitive or continuous acoustic stimuli (Schul and Sheridan, 2006; Abernethy et al., 2008; Schul et al., 2012), like the backdrop of crickets in the forest. In the adapted state, however, it will respond to rarer stimuli that differ in frequency from the repetitive stimulus (Schul and Sheridan, 2006; Schul et al., 2012). In this way, it can encode the occasional high-frequency calls of echolocating bats in noisy environments (Römer et al., 2008). A single neuron cannot provide information about changes in the frequencies of the echolocation call it is encoding, which would be necessary for katydids to distinguish between types of bat echolocation calls. TN-1, however, is not the only auditory interneuron providing information to the brain in katydids. Numerous ascending auditory interneurons with varying frequency tuning have been identified in katydids, although these neurons have only been studied in a few katydid species (Stumpner and Nowotny, 2014). Therefore, there is the potential for katydids to gain information about frequency modulation in bat calls and use this information to assess risk of predation by gleaning bats.
It is often difficult to observe how prey respond to predator cues in natural contexts, but it is critical to understand the context in which interactions take place (Apfelbach et al., 2005; Calisi and Bentley, 2009). It has been known for many years that katydids respond to echolocation calls but examining the dynamics in the natural habitat and partitioning responses by height in the forest and bat family provides a more detailed look at the complex interactions in this system. The acoustic characteristics of the call produced by an insect species may interact strongly with how that species responds to bats. Future research that tests for linkages between call characteristics and response to echolocation calls will provide insight about strategies for avoiding and mitigating predation. Additional insight will come from examining how individual insect species respond to playbacks of bats to understand how insect behavior differs with body size, mating strategy, and position in the canopy.
One of the most notable findings in this research is the prevalence of echolocation calls in the nighttime soundscape. Nearly 50% of nighttime recordings contain bat echolocation calls, most produced by bat species that do not eavesdrop on the sounds produced by insects. While detecting and responding to echolocation calls could help insects increase survival, interrupting calling in response to a false danger cue could also reduce opportunities to attract mates and compete with rivals, reducing fitness. The unreliability of echolocation calls as an indicator of predator presence raises important questions about the evolution of anti-predator strategies in noisy environments, particularly when different predator and prey species arrive at diverse solutions (McElreath and Strimling, 2006).
Data Availability Statement
Sound files and extracted data will be archived on Dryad after acceptance.
Author Contributions
HtH and LS conceived and designed the study. L-OH selected the trees and LS and SM climbed them. LS and SM organized the database and L-OH and HtH selected and screened the recordings. LS wrote the analysis code and HtH identified the bats and verified the code. LS wrote the framework of the manuscript with substantial text contributed by HtH and RP. All authors read, revised, and approved the manuscript before submission.
Funding
This study was funded by Dartmouth College HtH, the Neukom Institute LS and the Smithsonian Tropical Research Institute RP.
Conflict of Interest Statement
The authors declare that the research was conducted in the absence of any commercial or financial relationships that could be construed as a potential conflict of interest.
Acknowledgments
The authors would like to acknowledge the staff of the Smithsonian Tropical Research Institute for logistical assistance with particular thanks to Melissa Caño and Oris Acevedo. Nicole Kleinas provided valuable assistance in the field.
Supplementary Material
The Supplementary Material for this article can be found online at: https://www.frontiersin.org/articles/10.3389/fevo.2018.00227/full#supplementary-material
References
Abernethy, K., Abt, G., Reyer, H.-U., Acquistapace, P., Aquiloni, L., Hazlett, B. A., et al. (2008). The fish eye view: are cichlids conspicuous? Evolution 20, 223–231. doi: 10.1111/j.1558-5646.2007.00019.x
Apfelbach, R., Blanchard, C. D., Blanchard, R. J., Hayes, R. A., and McGregor, I. S. (2005). The effects of predator odors in mammalian prey species: a review of field and laboratory studies. Neurosci. Biobehav. Rev. 29, 1123–1144. doi: 10.1016/J.NEUBIOREV.2005.05.005
Barber, J. R., and Kawahara, A. Y. (2013). Hawkmoths produce anti-bat ultrasound. Biol. Lett. 9, 20130161. doi: 10.1098/RSBL.2013.0161
Belwood, J. (1988a). Influence of Bat predation on Calling Behavior in Neotropical Forest Katydids (Insecta: Orthoptera: Tettigoniidae). Ph.D. Dissertation, University of Florida, Gainsville, FL.
Belwood, J. (1990). “Anti-predator defences and ecology of Neotropical forest katydids, especially the Pseudophyllinae,” in The Tettigoniidae: Biology, Systematics and Evolution, eds P. Nachtigall and P. Moore (New York, NY: Springer-Verlag), 8–26.
Belwood, J. J. (1988b). Foraging behavior, prey selection, and echolocation in phyllostomine bats (Phyllostomidae). Anim. sonar 156, 601–605. doi: 10.1007/978-1-4684-7493-0_61
Belwood, J. J., and Morris, G. K. (1987). Bat predation and its influence on calling behavior in neotropical katydids. Science 238, 64–67
Berger, J. (1991). Pregnancy incentives, predation constraints and habitat shifts: experimental and field evidence for wild bighorn sheep. Anim. Behav. 41, 61–77. doi: 10.1016/S0003-3472(05)80503-2
Bernard, E. (2001). Vertical stratification of bat communities in primary forests of Central Amazon, Brazil. J. Trop. Ecol. 17, 115–126. doi: 10.1017/S0266467401001079
Bernard, E. (2002). Diet, activity and reproduction of bat species (Mammalia, Chiroptera) in Central Amazonia, Brazil. Rev. Bras. Zool. 19, 173–188. doi: 10.1590/S0101-81752002000100016
Bonaccorso, F. J. (1979). Foraging and reproductive ecology in a panamanian bat community. Bull. Florida State Mus. Biol. Sci. 24, 359–408.
Boyles, J. G., Cryan, P. M., McCracken, G. F., and Kunz, T. H. (2011). Economic importance of bats in agriculture. Science 332, 41–42. doi: 10.1126/science.1201366
Brinkløv, S., Jakobsen, L., Ratcliffe, J. M., Kalko, E. K. V., and Surlykke, A. (2011). Echolocation call intensity and directionality in flying short-tailed fruit bats, Carollia perspicillata (Phyllostomidae). J. Acoust. Soc. Am. 129, 427–435. doi: 10.1121/1.3519396
Calisi, R. M., and Bentley, G. E. (2009). Lab and field experiments: are they the same animal? Horm. Behav. 56, 1–10. doi: 10.1016/J.YHBEH.2009.02.010
Candolin, U. (1998). Reproduction under predation risk and the trade-off between current and future reproduction in the threespine stickleback. Proc. R. Soc. B Biol. Sci. 265, 1171–1175. doi: 10.1098/rspb.1998.0415
Christian, K. A., and Tracy, C. R. (1981). The effect of the thermal environment on the ability of hatchling galapagos land iguanas to avoid predation during dispersal. Oecologia 49, 218–223. doi: 10.1007/BF00349191
Cigliano, M. M., Braun, H., Eades, D. C., and Otte, D. (2018). Orthoptera Species File. Version 5.0/5.0. Avaliable online at: http//Orthoptera.SpeciesFile.org
Conner, W. E., and Corcoran, A. J. (2012). Sound strategies: the 65-million-year-old battle between bats and insects. Annu. Rev. Entomol. 57, 21–39. doi: 10.1146/annurev-ento-121510-133537
Corcoran, A. J., and Conner, W. E. (2012). Sonar jamming in the field: effectiveness and behavior of a unique prey defense. J. Exp. Biol. 215, 4278–4287. doi: 10.1242/jeb.076943
Culler, L. E., Ayres, M. P., and Virginia, R. A. (2015). In a warmer arctic, mosquitoes avoid increased mortality from predators by growing faster. Proc. Biol. Sci. 282:20151549. doi: 10.1098/rspb.2015.1549
Dawkins, R., and Krebs, J. R. (1979). Arms races between and within species. Proc. R. Soc. London. Ser. B Biol. Sci. 205, 489–511. doi: 10.1098/RSPB.1979.0081
Denzinger, A., Tschapka, M., and Schnitzler, H. (2018). The role of echolocation strategies for niche differentiation in bats. Can. J. Zool. 96, 171–181. doi: 10.1139/cjz-2017-0161
Endler, J. A., and Basolo, A. L. (1998). Sensory ecology, receiver biases and sexual selection. Trends Ecol. Evol. 13, 415–420.
Falk, J. J., ter Hofstede, H. M., Jones, P. L., Dixon, M. M., Faure, P. A., Kalko, E. K., et al. (2015). Sensory-based niche partitioning in a multiple predator–multiple prey community. Proc. R. Soc. London B Biol. Sci. 282:20150520. doi: 10.1098/rspb.2015.0520
Faure, P. A., and Hoy, R. R. (2000). The sounds of silence: cessation of singing and song pausing are ultrasound-induced acoustic startle behaviors in the katydid Neoconocephalus ensiger (Orthoptera; Tettigoniidae). J. Comp. Physiol. A 186, 129–142. doi: 10.1007/s003590050013
Fenton, M. B., Rydell, J., Vonhof, M. J., Eklöf, J., and Lancaster, W. C. (1999). Constant-frequency and frequency-modulated components in the echolocation calls of three species of small bats (Emballonuridae, Thyropteridae, and Vespertilionidae). Can. J. Zool. 77, 1891–1900. doi: 10.1139/z99-168
Fleming, T. H. (1991). The relationship between body size, diet, and habitat use in frugivorous bats, genus Carollia (Phyllostomidae). J. Mammal. 72, 493–501. doi: 10.2307/1382132
Fullard, J. H. (1998). The sensory coevolution of moths and bats. Springer Handb. Audit. Res. 10, 279–326. doi: 10.1007/978-1-4612-0585-2_8
Ghose, K., Triblehorn, J. D., Bohn, K., Yager, D. D., and Moss, C. F. (2009). Behavioral responses of big brown bats to dives by praying mantises. J. Exp. Biol. 212, 693–703. doi: 10.1242/jeb.019380
Jones, P. L., Page, R. A., Hartbauer, M., and Siemers, B. M. (2011). Behavioral evidence for eavesdropping on prey song in two palearctic sibling bat species. Behav. Ecol. Sociobiol. 65, 333–340. doi: 10.1007/s00265-010-1050-9
Jones, P. L., Ryan, M. J., and Page, R. A. (2014). Population and seasonal variation in response to prey calls by an eavesdropping bat. Behav. Ecol. Sociobiol. 68, 605–615. doi: 10.1007/s00265-013-1675-6
Jung, K., Kalko, E. K. V., and von Helversen, O. (2007). Echolocation calls in Central american emballonurid bats: signal design and call frequency alternation. J. Zool. 272, 125–137. doi: 10.1111/j.1469-7998.2006.00250.x
Kalka, M. B., Smith, A. R., and Kalko, E. K. (2008). Bats limit arthropods in tropical forest. Science 320:71. doi: 10.1126/science.1153352
Kalko, E. K., Handley, C. O., and Handley, D. (1996). “Organization, diversity, and long-term dynamics of a Neotropical bat community,” in Long-Term Studies of Vertebrate Communities, eds M. L. Cody and J. A. Smallwodd (San Diego, CL: Academic Press), 503–553.
Lacalli, T. C. (2001). New perspectives on the evolution of protochordate sensory and locomotory systems, and the origin of brains and heads. Philos. Trans. R. Soc. Lond. B Biol. Sci. 356, 1565–1572. doi: 10.1098/rstb.2001.0974
Libersat, F., and Hoy, R. R. (1991). Ultrasonic startle behavior in bushcrickets (Orthoptera; Tettigoniidae). J. Comp. Physiol. A 169, 507–514.
Ligges, U., Krey, S., Mersmann, O., and Schnackenberg, S. (2018). tuneR: Analysis of Music and Speech.
Magnhagen, C. (1991). Predation risk as a cost of reproduction. Trends Ecol. Evol. 6, 183–186. doi: 10.1016/0169-5347(91)90210-O
Marten, K., and Marler, P. (1977). Sound transmission and its significance for animal vocalization. Behav. Ecol. Sociobiol. 2, 271–290. doi: 10.1007/BF00299740
McElreath, R., and Strimling, P. (2006). How noisy information and individual asymmetries can make ‘personality’ an adaptation: a simple model. Anim. Behav. 72, 1135–1139. doi: 10.1016/J.ANBEHAV.2006.04.001
Miller, L. A., and Olesen, J. (1979). Avoidance behavior in green lacewings. J. Comp. Physiol. 131, 113–120. doi: 10.1007/BF00619071
Moiseff, A., Pollack, G. S., and Hoy, R. R. (1978). Steering responses of flying crickets to sound and ultrasound: Mate attraction and predator avoidance. Proc. Natl. Acad. Sci. U.S.A. 75, 4052–4056. doi: 10.1073/PNAS.75.8.4052
Montealegre, Z. and F. (2012). Reverse stridulatory wing motion produces highly resonant calls in a neotropical katydid (Orthoptera: Tettigoniidae: Pseudophyllinae). J. Insect Physiol. 58, 116–124. doi: 10.1016/J.JINSPHYS.2011.10.006
Naskrecki, P. (2000). Katydids of Costa Rica Vol. 1: systematics and bioacoustics of the cone-head katydids. Philadelphia, PA: Orthopterists' Society at the Academy of Natural Sciences of Philadelphia, Department of Entomology.
Nickle, D. A. (1992). Katydids of Panama (Orthoptera: Tettigoniidae), eds. D. Quintero and A. Aiello (Oxford: Oxford Science Publications).
Nolen, T. G., and Hoy, R. R. (1984). Initiation of behavior by single neurons: the role of behavioral context. Science 226, 992–994. doi: 10.1126/SCIENCE.6505681
Nolen, T. G., and Hoy, R. R. (1986). Phonotaxis in flying crickets. J. Comp. Physiol. A 159, 423–439.
Roeder, K. D., and Treat, A. E. (1957). Ultrasonic reception by the tympanic organ of noctuid moths. J. Exp. Zool. 134, 127–157. doi: 10.1002/jez.1401340107
Römer, H., Lang, A., and Hartbauer, M. (2008). No correlation of body size and high-frequency hearing sensitivity in neotropical phaneropterine katydids. J. Orthoptera Res. 17, 343–346. doi: 10.1665/1082-6467-17.2.343
Römer, H., Lang, A., and Hartbauer, M. (2010). The signaller's dilemma: a cost-benefit analysis of public and private communication. PLoS ONE 5:e13325. doi: 10.1371/journal.pone.0013325
Rosen, M. J., Levin, E. C., and Hoy, R. R. (2009). The cost of assuming the life history of a host: acoustic startle in the parasitoid fly Ormia ochracea. J. Exp. Biol. 212, 4056–4064. doi: 10.1242/jeb.033183
Rydell, J., Skals, N., Surlykke, A., and Svensson, M. (1997). Hearing and bat defence in geometrid winter moths. Proc. Biol. Sci. 264, 83–88. doi: 10.1098/rspb.1997.0012
Schul, J., Mayo, A. M., and Triblehorn, J. D. (2012). Auditory change detection by a single neuron in an insect. J. Comp. Physiol. A 198, 695–704. doi: 10.1007/s00359-012-0740-3
Schul, J., and Schulze, W. (2001). Phonotaxis during walking and flight: are differences in selectivity due to predation pressure? Naturwissenschaften 88, 438–442. doi: 10.1007/s001140100262
Schul, J., and Sheridan, R. A. (2006). Auditory stream segregation in an insect. Neuroscience 138, 1–4. doi: 10.1016/j.neuroscience.2005.11.023
Schulze, W., and Schul, J. (2001). Ultrasound avoidance behaviour in the bushcricket Tettigonia viridissima (Orthoptera: Tettigoniidae). J. Exp. Biol. 204, 733–740.
Sih, A., Krupa, J., and Travers, S. (1990). An experimental study on the effects of predation risk and feeding regime on the mating behavior of the water strider. Am. Nat. 135, 284. doi: 10.1086/285044
Staller, E. L., Carroll, J. P., Thornton, R. P., Palmer, W. E., and Sisson, D. C. (2005). Identifying predators at northern bobwhite nests. J. Wildl. Manage. 69, 124–132. doi: 10.2193/0022-541X(2005)069<0124:IPANBN>2.0.CO;2
Stumpner, A., and Nowotny, M. (2014). “Neural Processing in the Bush-Cricket Auditory Pathway,” in Insect Hearing and Acoustic Communication. Animal Signals and Communication, Vol 1, eds B. Hedwig (Heidelberg:Springer), 143–166. doi: 10.1007/978-3-642-40462-7_9
Sueur, J., Aubin, T., and Simonis, C. (2008). Equipment review: seewave, a free modular tool for sound analysis and synthesis. Bioacoustics 18, 213–226. doi: 10.1080/09524622.2008.9753600
Surlykke, A., Jakobsen, L., Kalko, E. K., and Page, R. A. (2013). Echolocation intensity and directionality of perching and flying fringe-lipped bats, Trachops cirrhosus (Phyllostomidae). Front. Physiol. 4:143. doi: 10.3389/fphys.2013.00143
Surlykke, A., and Kalko, E. K. (2008). Echolocating bats cry out loud to detect their prey. PLoS ONE 3:e2036. doi: 10.1371/journal.pone.0002036
Symes, L. B., Page, R. A., and ter Hofstede, H. M. (2016). Effects of acoustic environment on male calling activity and timing in neotropical forest katydids. Behav. Ecol. Sociobiol. 70, 1485–1495. doi: 10.1007/s00265-016-2157-4
Teixeira, R. C., Corrêa, C. E., and Fischer, E. (2009). Frugivory by Artibeus jamaicensis (Phyllostomidae) bats in the pantanal, Brazil. Stud. Neotrop. Fauna Environ. 44, 7–15. doi: 10.1080/01650520802692283
ter Hofstede, H., Voigt-Heucke, S., Lang, A., Römer, H., Page, R., Faure, P., et al. (2017). Revisiting adaptations of neotropical katydids (Orthoptera: Tettigoniidae) to gleaning bat predation. Neotrop. Biodivers. 3, 41–49. doi: 10.1080/23766808.2016.1272314
ter Hofstede, H. M., and Fullard, J. H. (2008). The neuroethology of song cessation in response to gleaning bat calls in two species of katydids, Neoconocephalus ensiger and Amblycorypha oblongifolia. J. Exp. Biol. 211, 2431–2441. doi: 10.1242/jeb.017285
ter Hofstede, H. M., Kalko, E. K. V., and Fullard, J. H. (2010). Auditory-based defence against gleaning bats in neotropical katydids (Orthoptera: Tettigoniidae). J. Comp. Physiol. A 196, 349–358. doi: 10.1007/s00359-010-0518-4
ter Hofstede, H. M., and Ratcliffe, J. M. (2016). Evolutionary escalation: the bat–moth arms race. J. Exp. Biol. 219, 1589–1602. doi: 10.1242/jeb.086686
Thompson, D. J. (1978). Towards a realistic predator-prey model: the effect of temperature on the functional response and life history of larvae of the damselfly, Ischnura elegans. J. Anim. Ecol. 47, 757. doi: 10.2307/3669
Tuttle, M. D., Ryan, M. J., and Belwood, J. J. (1985). Acoustical resource partitioning by two species of phyllostomid bats (Trachops cirrhosus and Tonatia sylvicola). Anim. Behav. 33, 1369–1371. doi: 10.1016/S0003-3472(85)80204-9
Weinbeer, M., Kalko, E. K. V., and Jung, K. (2013). Behavioral flexibility of the trawling long-legged bat, Macrophyllum macrophyllum (Phyllostomidae). Front. Physiol. 4:342. doi: 10.3389/fphys.2013.00342
Williams, T. M., Estes, J. A., Doak, D. F., and Springer, A. M. (2004). Killer appetites: assessing the role of predators in ecological communities. Ecology 85, 3373–3384. doi: 10.1890/03-0696
Williams-Guillén, K., Perfecto, I., and Vandermeer, J. (2008). Bats limit insects in a neotropical agroforestry system. Science 320:70. doi: 10.1126/science.1152944
Wolf, M., van Doorn, G. S., Leimar, O., and Weissing, F. J. (2007). Life-history trade-offs favour the evolution of animal personalities. Nature 447, 581–584. doi: 10.1038/nature05835
Yack, J. E., and Dawson, J. W. (2008). “Insect ears,” in The Senses: A Comprehensive Reference, Vol. 3, Audition, eds. P. Dallos and D. Oertel (Oxford: Elsevier), 35–53.
Yager, D. D. (2012). Predator detection and evasion by flying insects. Curr. Opin. Neurobiol. 22, 201–207. doi: 10.1016/J.CONB.2011.12.011
Yager, D. D., Cook, A. P., Pearson, D. L., and Spangler, H. G. (2000). A comparative study of ultrasound-triggered behaviour in tiger beetles (Cicindelidae). J. Zool. 251, 355–368. doi: 10.1111/j.1469-7998.2000.tb01086.x
Yager, D. D., and Spangler, H. G. (1995). Characterization of auditory afferents in the tiger beetle,Cicindela marutha Dow. J. Comp. Physiol. A 176, 587–599. doi: 10.1007/BF01021579
Yager, D. D., and Svenson, G. J. (2008). Patterns of praying mantis auditory system evolution based on morphological, molecular, neurophysiological, and behavioural data. Biol. J. Linn. Soc. 94, 541–568. doi: 10.1111/j.1095-8312.2008.00996.x.
Zamora-Gutierrez, V., Lopez-Gonzalez, C., MacSwiney Gonzalez, M. C., Fenton, B., Jones, G., Kalko, E. K. V., et al. (2016). Acoustic identification of Mexican bats based on taxonomic and ecological constraints on call design. Methods Ecol. Evol. 7, 1082–1091. doi: 10.1111/2041-210X.12556
Keywords: canopy, predator-prey interaction, eavesdropping, gleaning bats, Tettigoniidae, sensory ecology
Citation: Symes LB, Martinson SJ, Hoeger L-O, Page RA and ter Hofstede HM (2018) From Understory to Canopy: In situ Behavior of Neotropical Forest Katydids in Response to Bat Echolocation Calls. Front. Ecol. Evol. 6:227. doi: 10.3389/fevo.2018.00227
Received: 12 September 2018; Accepted: 06 December 2018;
Published: 19 December 2018.
Edited by:
Ann Valerie Hedrick, University of California, Davis, United StatesReviewed by:
Richard Anthony Peters, La Trobe University, AustraliaEunice Jingmei Tan, Yale-NUS College, Singapore
Copyright © 2018 Symes, Martinson, Hoeger, Page and ter Hofstede. This is an open-access article distributed under the terms of the Creative Commons Attribution License (CC BY). The use, distribution or reproduction in other forums is permitted, provided the original author(s) and the copyright owner(s) are credited and that the original publication in this journal is cited, in accordance with accepted academic practice. No use, distribution or reproduction is permitted which does not comply with these terms.
*Correspondence: Laurel B. Symes, laurel.b.symes@dartmouth.edu