- 1Jiangsu Key Laboratory of Crop Genetics and Physiology/Co-Innovation Center for Modern Production Technology of Grain Crops, Yangzhou University, Yangzhou, China
- 2Joint International Research Laboratory of Agriculture and Agri-Product Safety, Yangzhou University, Yangzhou, China
High-amylose cereal starches provide many health benefits for humans. The inhibition or mutation of starch branching enzyme (SBE) genes is an effective method to develop high-amylose cereal crops. This review summarizes the development of high-amylose cereal crops through the inactivation of one or more SBE isoforms or combination with other genes. This review also reveals the causes of increase in amylose content in high-amylose crops. A series of changes, including amylopectin structure, crystalline structure, thermal properties, and hydrolysis properties, occurs as amylose content increases. The different morphological starch granules nominated as heterogeneous starch granules or differently stained starch granules are detected in high-amylose cereal crops. Detailed studies on four heterogeneous starch granules in high-amylose rice, which is developed by antisense RNA inhibition of SBEI/IIb, indicate that granules with different morphologies possess various molecular structures and physicochemical and functional properties. This variation diversifies their applications in food and non-food industries. However, current knowledge regarding how these heterogeneous starch granules form and why they exhibit regional distribution in endosperm remain largely unknown.
Introduction
High-amylose starches, rich in resistant starch (RS), have been extensively investigated because of their many potential health benefits. RS is a portion of starch that can evade degradation in the upper gastrointestinal tract and function as a substrate for the bacterial fermentation in the large intestine (Nugent, 2005). RS-enriched food can decrease glycemic and insulin responses and reduce the risk of developing type II diabetes mellitus, obesity, and cardiovascular diseases (Granfeldt et al., 1995; Regina et al., 2006; Zhu et al., 2012). The proportion of amylose is positively related to RS content (Sang et al., 2008; Cai et al., 2015; Lin et al., 2016b; Zhou et al., 2016). High-amylose starches exhibit various physicochemical properties, including high gelling strength, excellent film-forming ability, and ease of retrogradation; with these properties, industrial applications, such as adhesive, paper, and biodegradable plastic production, have been developed (Avella et al., 2002). Therefore, considerable research has focused on high-amylose starches.
Starch is composed of approximately 15–25% linear amylose. The remaining portion of starch consists of highly branched and organized amylopectin (Smith et al., 2002). Amylose in the endosperm can only be synthesized by granule bound starch synthase I (GBSSI), which is tightly bound to starch granules to extend α-1,4 linkages of glucose polymers (Jeon et al., 2010). Amylopectin synthesis is mainly attributed to three biosynthetic enzymes, namely, starch synthases (SSs), starch branching enzymes (SBEs), and debranching enzymes (DBEs). SSs and SBEs are responsible for the elongation of glucan chains and the formation of branch points, respectively (Jeon et al., 2010; Tetlow and Emes, 2014). The irregular glucan structure produced by SSs and SBEs is trimmed by DBEs to derive an ordered amylopectin branched chains (Nakamura, 2002). Plastidial phosphorylase (Pho1) also participates in starch synthesis; however, its precise role remains unclear (Yu et al., 2001; Satoh et al., 2008).
The amylose content (AC) in cereal endosperm can be effectively increased by applying two methods, that is, enhancing GBSSI expression or eliminating SBEs, SSIIa, or other enzymes involved in amylopectin synthesis (Umemoto and Terashima, 2002; Itoh et al., 2003; Crofts et al., 2012; Zhou et al., 2016). Once a certain level is reached, AC no longer increases in GBSSI-enhanced lines possibly because of the limited non-reducing ends in amylose and the substrate competition between amylose and amylopectin (Tsai et al., 1970; Smith et al., 2002; Sestili et al., 2012). The promotion of AC in SSIIa nulls varies widely in different species; for instance, the elimination of SSIIa unlikely change AC in rice alk (Umemoto et al., 2004, 2008), moderately increases AC (40–50%) in maize sugary2 (su2) and wheat sgp-1 (Yamamori et al., 2000; Tziotis et al., 2004; Zhang et al., 2004), and yields about 50% AC in barley sex6 (Morell et al., 2003). Compared with the effectiveness of SBE inactivation for high-amylose cereal crops, which have remarkable increase in AC (Li et al., 2008; Carciofi et al., 2012; Zhu et al., 2012; Regina et al., 2015), the enhanced AC in SSIIa nulls is moderate (Yamamori et al., 2000; Tziotis et al., 2004; Zhang et al., 2004). Therefore, AC can be promoted effectively by suppressing or eliminating one or more SBE activities in cereal crops (Table 1).
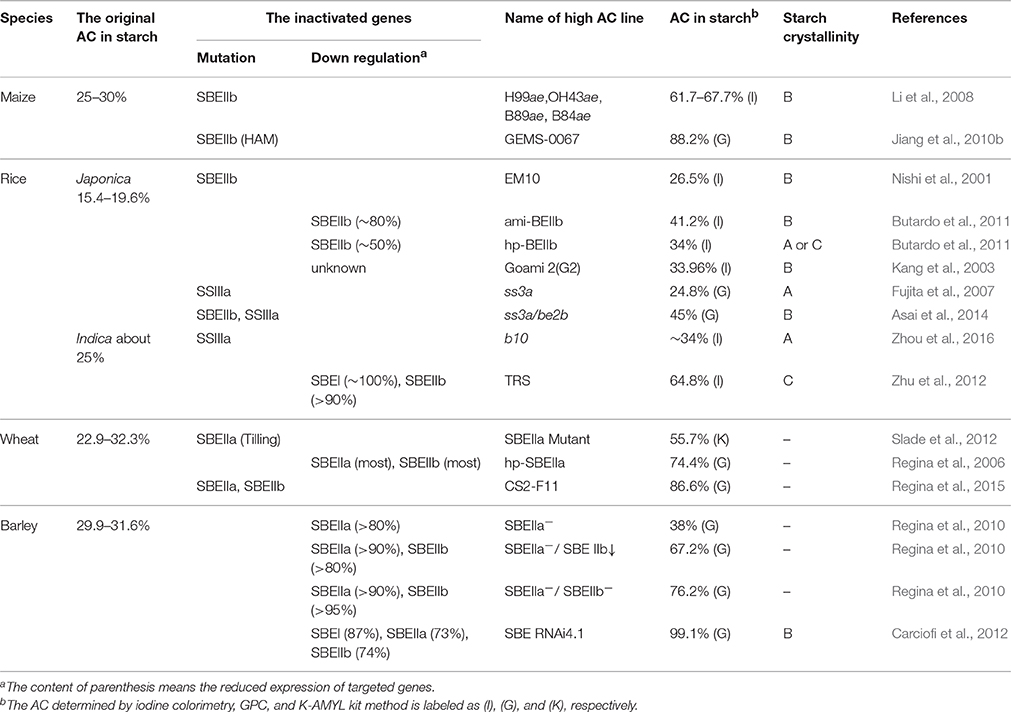
Table 1. The inactivated gene, amylose content in starch, and starch crystallinity of high-amylose cereal crops.
This review summarizes the development of high-amylose cereal crops through the inactivation of SBE(s) and a series of starch changes caused by the increased amylose percentage.
Development of High-Amylose Cereal Crops Through SBE Inactivation
Cereal crops have three SBE classes, namely, SBEI, SBEIIa, and SBEIIb. Biochemical observations indicate that SBEI preferentially branches longer chains, whereas SBEII isoforms have a higher capacity for transferring of short chains (Guan and Preiss, 1993; Takeda et al., 1993; Guan et al., 1997; Nishi et al., 2001; Nakamura, 2002; Nakamura et al., 2010; Tetlow and Emes, 2014). Among these observations, studies on eliminating SBEI activity show no effect on the starch composition and kernel morphology (Blauth et al., 2002; Satoh et al., 2003b; Regina et al., 2004); however, in rice and maize, minor changes in the fine structure of amylopectin have been reported (Fujita et al., 2006; Xia et al., 2011). However, AC resulting from the inactivation of either one of two SBEII isoforms and the combination of two or three SBE isoforms is significantly increased in different species (Table 1).
Amylose Increase Through SBEIIa or SBEIIb Deficiency
In maize and rice, SBEIIb inactivation is required to obtain a high-amylose phenotype, whereas no significant changes including AC and amylopectin fine structure are observed in SBEIIa-defective endosperms (Blauth et al., 2001; Nakamura, 2002; Satoh et al., 2003a). Mutations of SBEIIb in maize and rice are commonly referred to as amylose-extender (ae) mutants. Normal maize occupies about 25–30% AC, whereas the AC in H99ae, OH43ae, B89ae, and B84ae inbred ae-lines has been reported to reach at least 60%, ranging from 61.7 to 67.7% (Li et al., 2008). In rice containing Wxb, which mutates at the 5′ end of intron one, japonica type contains low GBSSI mRNA and protein levels, leading to reduced AC in contrast to indica type, which has a highly expressed Wxa allele (Isshiki et al., 2000). The ae mutants from these two backgrounds have higher AC than their wild-type parents. However, AC increase extent is not more than 15%, which is significantly lower than that in analogous maize mutants, which show at least 35% promotion (Nishi et al., 2001; Shannon et al., 2009). Another ae-like mutant Goami 2 in rice, also known as Suweon 464, which is derived from high-quality temperate japonica rice variety (Iipumbyeo), shows about 2-fold increase in AC (to around 34.0%, see Kang et al., 2003). However, the specific mutation responsible for high-amylose phenotype of Goami 2 remains to be proved.
On the contrary, an almost complete SBEIIb inhibition in wheat and barley causes a minor change in AC, whereas SBEIIa inhibition can evidently increase AC (Regina et al., 2006, 2010). By targeting induced local lesions in genomes (TILLING), wheat SBEIIa mutant increases AC from 23 to 55% (Slade et al., 2012). In barley transgenic lines, by RNA-mediated silencing technology, a high-amylose phenotype (>38%) is observed when SBEIIa expression is reduced by >80% (Regina et al., 2010).
In summary, SBEIIb in rice and maize plays a distinct role in starch synthesis. By contrast, SBEIIa in wheat and barley is more significant than SBEIIb. High-amylose phenotype generation is determined by the difference in SBEIIa and SBEIIb expression levels in cereals. In maize, SBEIIb, which is at least 50 times the abundance of SBEIIa, is the predominant isoform in endosperm (Gao et al., 1997). On the other hand, in rice, the ratio of SBEIIb and SBEIIa is closer to 5:1, which partly elucidates the higher AC promotion in maize ae mutants. Furthermore, SBEIIa is expressed at much higher levels than SBEIIb counterpart in both wheat and barley kernels (Regina et al., 2006, 2010). Therefore, SBEIIa inhibition is more effective for developing high-amylose species in the two crops.
Higher Amylose Increase through Inactivation of Two or More SBEs
Combining reduced SBE isoforms with the base of single SBEIIa or SBEIIb inactivation is an efficient way to acquire high-amylose crops, especially for wheat and barley (Table 1). A transgenic resistant starch rice line (TRS) with 60% AC is developed by simultaneously declining the expressions of SBEIIb and SBEI in an indica rice variety (Te-qing, TQ). The AC of TRS is significantly higher than that of TQ-derived rice line by declining the expression of SBEIIb alone (Wei et al., 2010c; Zhu et al., 2012). In wheat, transformation with a hairpin construct targeting SBEIIa alone not only reduces transcription to <10%, but also leads to an almost complete loss of SBEIIb in the protein levels, which yields an AC of ~75% in transgenic wheat (Regina et al., 2006). Furthermore, a genetic strategy to combine deletion and single nucleotide polymorphism (SNP) generates wheat genotypes with the complete absence of SBEIIa from all three genomes and the absence of SBEIIb from one of the genomes, which elevates the AC to an unprecedented ~85% (Regina et al., 2015). In barley, a combination of SBEIIa and SBEIIb inhibition leads to an amylose increase up to 76.2% at maximum, which is much higher than the suppression by SBEIIa alone (Regina et al., 2010, 2012). In addition, an “amylose-only barley” is derived through concerted repression of SBEI, SBEIIa, and SBEIIb using a chimeric RNAi hairpin (Carciofi et al., 2012). Through this method, the highest known AC-contained lines in rice, wheat, and barley are derived (Table 1).
Higher Amylose Increase through SBEII Isoform Deficiency with Other Genes
By introducing a modifier gene or by coupling with isozyme deficiencies related to starch biosynthesis on the base of inactivation of SBEII, additive effects show a more profound meaning on AC in cereal crops (Wu et al., 2009; Jiang et al., 2010b; Asai et al., 2014).
When an unknown number of high-amylose modifier (HAM) gene is introduced into a homozygous ae-mutant background in maize, AC is further increased (Wu et al., 2009). The commercial maize GEMS-0067, which is the highest known AC-contained line (>85%), is derived from the predigree of [GUAT209:S13 × (OH43ae × H99ae)] (Li et al., 2008; Wu et al., 2009). GUAT209:S13 is a cross-breed serving as HAM gene. Jiang et al. (2010b) found that HAM gene dosage has an additive effect on AC; amylose percentage ranges from 68.9 to 88.2%, when the HAM gene-dosage levels are 0, 16.7, 33.3, 50, 66.7, 83.3, and 100%.
Single SBEII mutation coupled with other high-amylose mutation is an effective method in producing high-amylose starches. SSIIIa deficiency in rice produces 30.7% AC phenotype. However, when this mutation is introduced into SBEIIb-defective background, the AC is further promoted into 45% in ss3a/sbe2b double mutant (Asai et al., 2014).
Factors Affecting Amylose Increase in High-Amylose Cereal Crops
Three factors are proposed to be responsible for amylose enrichment caused by SBE isoform lesions in endosperm starch. First, amylopectin synthesis reduction emphasizes the amylose percentage in these high-amylose lines compared with the normal ones. Second, the increase protein amount of GBSSI or higher GBSSI activity results in an elevated amylose synthesis. Third, the extra-long chains (ELCs) resulting from amylopectin, which can bind iodine to develop a dark-blue color, significantly enriches AC value in high-amylose varieties, (Jane et al., 1999). Factors responsible for the amylose increase are different across various species.
In rice, different researchers have various opinions on amylose enrichment. An increase in amylose is attributed to the first two factors. In rice ss3a/be2b mutant, a significantly higher AGPase activity resulting from SSIIIa and SBEIIb deficiency leads to a high concentration of ADP-glucose. GBSSI yields a higher Km for ADP-glucose than other soluble SS isozymes. Coupled with the fact that the GBSSI amounts in ss3a/sbe2b and sbe2b are significantly higher than those in wild type varieties; these results strongly explain why amylose synthesis is remarkably enriched in the endosperm of sbe2b and ss3a/sbe2b compared with wild-type varieties (Nishi et al., 2001; Asai et al., 2014). In addition, amylopectin synthesis stops at later starch development stage when dehydration begins in sbe2b-related mutants, emphasizing the AC in sbe2b and ss3a/sbe2b (Asai et al., 2014).
For other researchers, the third factor is the main one responsible for amylose increase in rice. The waxy ae double mutant, which eliminates SBEIIb in an amylose-free background, still contains amylose, and AC increase extent is almost similar to that in its counterpart in ae single mutant (Nishi et al., 2001). Thus, amylose increase in the double mutant is not due to the true amylose increase but to modified amylopectin. The maximum wavelength of the absorbance of the starch-iodine complex (λmax) of starch from waxy ae is 32 nm higher than that from the waxy mutant. Although ELC is not observed directly, this result strongly suggests that the AC increase is caused predominantly by the abnormal structure of amylopectin. Except genetic evidence, structural analyses on debranched starch from two SBEIIb down-regulated lines, amiRNA and hpRNA demonstrate directly that AC doubling in these two transgenic lines is not due to an increase in the relative proportion of amylose but to the elevated proportion of ELC, which ranges from degree of polymerization (DP) 120–1,000 (Butardo et al., 2011).
For maize, the amylose increase mainly results from ELC. Although starch contains no amylose, similar to the waxy ae double mutant of rice, the waxy ae mutant of maize displays an apparent AC of 34.5% (Jane et al., 1999). On the other hand, detailed structural analyses have demonstrated that those long chains do not originate from the real amylopectin of high-amylose starches, but from intermediate components (IC), which is a fraction consisting of branched molecules with molecular weights smaller than amylopectin but similar to amylose (Wang et al., 1993; Kasemsuwan et al., 1995) and is capable of escaping 1-butanol precipitation with amylopectin (Jane and Chen, 1992; Li et al., 2008; Peymanpour et al., 2016). When removing amylose from high-amylose starches, a mixture containing both IC and amylopectin is further separated using gel permeation chromatography (GPC) (Li et al., 2008). Peymanpour et al. (2016) divided this mixture according to their distribution of molecular structure into high molecular weight fraction (HMF) and low molecular weight fraction (LMF). HMF is the typical amylopectin, whereas LMF corresponds to the IC fraction, which increases with AC and appears to have substantially more of long chains than HMF. Li et al. (2008) further divided IC fraction according to the blue-values into large-Mw IC and low-Mw IC. Chain-length distribution results indicate that large-Mw IC has a similar branch structure to amylopectin but with smaller molecular-weight. On the other hand, low-Mw IC, which is the main source of fluctuating apparent AC in starch, has longer chains compared with amylopectin and large-Mw IC (Li et al., 2008).
Structure Changes of Amylopectin in High-Amylose Cereal Crops
In those high-amylose lines, reduced or completely lost SBEs actually does not only result in a simple promotion of AC within the granules, but also complicates the starch structure, specifically the amylopectin structure. Amylopectin is mainly composed of short- to mediated-length chains in normal and waxy cultivars. However, starch from SBEs-deficient lines has an abnormal amylopectin structure, which is enriched with long branch-chains but depleted of short ones (Nishi et al., 2001; Yao et al., 2004). For example, in rice ae mutants with the DP ≤ 17, chains with DP 8–12 are remarkably reduced. On the other hand, the long branch-chains with DP > 24, which connect the clusters of amylopectin, are more abundant compared with those in the wild type varieties (Nishi et al., 2001; Asai et al., 2014). Furthermore, through GPC analyses of a series of different AC-contained starches, Lin et al. (2016c) found that the ratio of long amylopectin branch-chains in high-amylose species is positively correlated with the AC.
Effect of different SBEIIb dosages on the amylopectin in rice is widely researched. First, due to the triploid characteristic of endosperm, Nishi et al. (2001) performed reciprocal crosses between null ae mutant (aeaeae) and wild type (AeAeAe) to generate F1 seeds with two different doses of the SBEIIb allele, duplex (AeAeae) and simplex (Aeaeae), which shows 74 and 26% protein levels relative to the wild type, respectively. This result implies that the protein level of SBEIIb appears to increase almost linearly with the increase in the number of dominant Ae alleles; a similar trend is observed in gene dosage effect on SBEIIb activity. The proportion of short branch-chains with DP ≤ 17 is 74% in duplex (AeAeae), 72% in simplex (Aeaeae), and 66% in nulliplex (aeaeae), indicating that DP ≤ 17 increases with the increasing number of Ae allele; however, the increase is not linear. Second, when SBEIIb gene is introduced into SBEIIb-defective mutant, resulting transgenic rice plants show a wide range of SBEIIb activities (Tanaka et al., 2004). As the SBEIIb activity increases, short branch-chains with DP ≤ 13 become more frequent, whereas the number of long branch-chains with DP = 15–30 and DP ≥ 40 decline. Although structural and physicochemical properties caused by different SBEIIb dosages are not remarkably increased with the increase of Ae allele or SBEIIb expression level, the above results reveal that starch composition and molecular structure are entirely dependent on the level of SBEIIb activity. In addition, SBEIIb overexpression results in a severely shrunken phenotype of the kernel, which is caused by the accumulation of excessive branched, water-soluble polysaccharides instead of amylose and amylopectin (Tanaka et al., 2004).
Starch Properties of High-Amylose Cereal Crops
Increased amylose, especially the enriched percentage of amylopectin long branch-chains dramatically affected starch properties including crystalline structure (Cheetham and Tao, 1998; Nishi et al., 2001; Li et al., 2008; Jiang et al., 2010b; Wei et al., 2010b,d; Butardo et al., 2011; Man et al., 2013a; Asai et al., 2014; Cai et al., 2014c; Lin et al., 2016c), thermal properties (Jiang et al., 2010c; Regina et al., 2010; Wei et al., 2011; Man et al., 2014; Pan et al., 2017), and hydrolysis properties (Jiang et al., 2010d; Qin et al., 2011; Man et al., 2012a,b, 2013b,c; Cai J. et al., 2014; Huang et al., 2015; Lin et al., 2016a). These properties determine the quality of starch and the application of high-amylose crops.
Crystalline Structure of High-Amylose Starch
Starch is stored as granules with alternating semi-crystalline and amorphous growth rings. The semicrystalline ring consists of the lamellar structure of alternating crystalline and amorphous regions. Amylopectin branch-chains form double helices and are laterally packed to form crystalline regions (Blazek and Gilbert, 2011). Starch crystallinity has types A and B according to X-ray diffraction pattern. A-type crystallinity is formed by amylopectin with short branch-chains and closed branching points, whereas B-type crystallinity is formed by amylopectin with long branch-chains and distant branching points. Usually, waxy and normal cereal crops contain A-type starch (A-type crystallinity), tuberous crops consist of B-type starch (B-type crystallinity), and some legumes and rhizomes possess C-type starch, which is a mixture of both A- and B-type crystallinities (Cheetham and Tao, 1998; Blazek and Gilbert, 2011).
For high-amylose crops with SBEs inactivation, their amylopectin branch-chain length increases and branching degree decreases with increasing AC, leading to the change of starch crystallinity (Table 1). Most high-amylose crop starches display B-type crystallinity (Nishi et al., 2001; Li et al., 2008; Jiang et al., 2010b; Butardo et al., 2011; Asai et al., 2014; Lin et al., 2016c), which is easily understood through the change in amylopectin structure. However, some high-amylose crops have C-type starch (Cheetham and Tao, 1998; Wei et al., 2010a,d; Butardo et al., 2011; Cai et al., 2014c; Huang et al., 2015; Lin et al., 2016c). Cheetham and Tao (1998) proposed that starch changes from A-type to B-type via C-type as AC increases in maize. The transition occurs at approximately 40% AC, a value capable of maintaining C-type starch. Starch with AC lower than 40% has A-type crystallinity, whereas AC higher than 40% has B-type crystallinity. Cai et al. (2014c) thought that high-amylose maize starch with AC 35.6% and apparent AC 56% contain differently sized granules and large and small granules exhibit A- and B-type crystallinities, respectively. However, how and where these different-sized starch granules are synthesized in maize endosperm remains unclear. High-amylose rice TRS with 60% AC is composed of polygonal, aggregate, elongated, and hollow granules distributed in specific endosperm regions (Wei et al., 2010c; Cai et al., 2014b). Polygonal and elongated granule has A- and B-type crystallinity, respectively. However, the aggregate granule contains both A- and B-type crystallinities within the same granule (Wei et al., 2010a,b; Man et al., 2014). TRS is a good material to investigate the synthesis of different crystallinities in the future.
Thermal Properties of High-Amylose Starch
For the applications of starch in food industry, heating starch in water is necessary. During heating, starch granules absorb water and swell, crystalline structure is destroyed, and birefringence is lost. This process is defined as starch gelatinization and can be detected by hot-stage microscopy and differential scanning calorimetry (Cai et al., 2014a). AC and amylopectin structure in starch granule significantly affect starch gelatinization (Kaur et al., 2007; Wei et al., 2011; Qin et al., 2012; Cai et al., 2014d). Amylose can maintain the integrity of swollen granule and restrain its swelling (Tester and Morrison, 1992). Granule swelling is positively correlated to amylopectin short branch-chains, whereas negatively to amylopectin long branch-chains (Salman et al., 2009; Lin et al., 2016a). In addition, lipid content in starch granule is positively correlated with AC, and lipid-complexed amylose chains also restrict granular swelling (Tester and Morrison, 1992). Therefore, high-amylose starches have lower granule swelling.
For gelatinization temperature, normal starches usually show a clear gelatinization transition peak due to the dissociation of short-chain double-helical crystallites of amylopectin molecules (Jiang et al., 2010c). However, high-amylose starches yield two thermal transition peaks that correspond to the melting of amylopectin double-helical crystallite and amylose-lipid complex (Jiang et al., 2010c; Qin et al., 2012; Man et al., 2014). In high-amylose starch with relatively low AC increase, the amylopectin has longer branch-chains and its double-helical crystallite requires a higher gelatinization temperature than normal starch does; on the other hand, high AC causes the simultaneous formation of an amylose-lipid complex resulting in the second thermal transition peak appearance (Jiang et al., 2010c; Qin et al., 2012; Man et al., 2014). As AC continually increases, the molecular structure of amylopectin is severely disordered; thus, intensity of the first peak gradually becomes weak, whereas the second peak becomes strong (Regina et al., 2010; Man et al., 2014). When AC becomes the dominant component in the starch granules, the first peak disappears and only the second one is preserved (Jiang et al., 2010c; Regina et al., 2010; Man et al., 2014).
Hydrolysis of High-Amylose Starch
Starch hydrolysis by amylase is involved in many biological and industrial processes (Tawil et al., 2011). Usually, amylase hydrolyzes starch in two ways as follows: Amyloglucosidase erodes the outer surface of the granule (exocorrosion), and α-amylase creates channels leading to the granule center and consequently leads to its breakdown from the inside out (endocorrosion) (Li et al., 2004). The susceptibility of starch to amylase is influenced by many factors, such as granule morphology, size, integrity and porosity, AC, and crystalline structure (Blazek and Gilbert, 2010). Generally, large starch granule with low relative surface area is slowly hydrolyzed compared with a small granule (Kim et al., 2008). Surface pores and internal channels of starch granule can increase enzyme diffusion into the interior of granule (Naguleswaran et al., 2011, 2012). B-type starch has higher resistance to amylase than A-type starch; amylose inhibits starch hydrolysis (Jiang et al., 2010d; Man et al., 2013b,c; Lin et al., 2016a,b). Proportion of the elongated and aggregate starch granules in high-amylose maize and rice increases with the increase in AC. Elongated and aggregate granules, consisting of two or many fused sub-granules, have larger size than normal starch, show smooth surface without porosity and internal channel, and exhibit high amylose in the outer layer of the granule (Jiang et al., 2010a,d; Wei et al., 2010c; Cai et al., 2014d). Therefore, B-type crystallinity, large size, and smooth surface increase the resistance of high-amylose starches to amylase hydrolysis, and the outer region of the granule is highly resistant to hydrolysis (Jiang et al., 2010d; Man et al., 2013b,c).
Heterogeneity of Starch Granules in High-Amylose Cereal Crops
Recently, a highly diverse population of differently stained and different morphological starch granules are detected in the endosperm of high-amylose cereal crop (Jiang et al., 2010a; Wei et al., 2010c; Butardo et al., 2011; Wellner et al., 2011; Carciofi et al., 2012; Liu et al., 2013; Cai et al., 2014d; Man et al., 2014). More interestingly, different morphological granules have an obviously regional distribution in mature kernels (Wellner et al., 2011; Liu et al., 2013; Cai et al., 2014b).
Occurrence of Differently Stained Starch Granules
Isolated starch granules from high-amylose maize and rice grains show different colors under normal and polarized light when being stained with iodine (Cai et al., 2014b,d). More interesting, in the kernel of maize ae mutants, a narrow band of sub-aleurone is occupied by blue-staining granules, whereas the central endosperm region is dominated by pink-staining granules. Between the differently stained regions, a transition region with a range of biphasic granules containing blue- and pink-stained colors simultaneously are detected (Liu et al., 2013). This observation indicates that the three differently stained starch granules exhibit a spatial distribution and that the heterogeneity of starch granules increases slightly from the inner to outer layer. Raman microscopy reveals that the pink regions contain starch molecules with reduced levels of branching compared with those blue-stained regions, which are ordered (crystalline) but not radially oriented (Wellner et al., 2011). Thus far, the physicochemical and functional properties of the three differently stained granules have yet to be extensively investigated.
Occurrence of Different Morphological Starch Granules
Recently, several reports state that high-amylose crops have different morphological starch granule distinguished from the normal ones. Normal maize contains angular or spherical granules, whereas in the single mutant ae, a small percentage (~1.7%) of elongated granules is observed. Furthermore, this kind of granules has a positive relationship with AC. The percentage of elongated granules is increased to 32% in GEMS-0067 endosperm with 85% AC (Jiang et al., 2010a). Some aggregate starch granules are also reported in high-amylose maize (Cai et al., 2014d). Wheat and barley grains have large lenticular starch granules and small spherical ones; however, the large granules from their high-amylose counterparts become sickle-shaped (Regina et al., 2006, 2010). In barley amylose-only line, multi-lobed starch granules with elongated, rough, and globular morphology dominates the whole kernel (Carciofi et al., 2012). Rice grain is filled with homogeneous compound granules, which can be easily separated to individual polygonal ones with various angles during starch isolation process. In different source of high-amylose rice, including ae and Goami 2 mutants and SBEIIb-inhibited lines, polygonal granule is mixed with large voluminous, nonangular rounded bodies (Nishi et al., 2001; Kang et al., 2003; Butardo et al., 2011). In high-amylose rice TRS, mature kernel has four types of different morphological starches including polygonal, aggregate, elongated, and hollow granules (Wei et al., 2010c; Cai et al., 2014b).
Even though different morphological starch granules are popularly detected in high-amylose cereals, their physicochemical properties still remain unknown due to the fact that they are always studied as a mixture with the normal ones. Therefore, all the results from high-amylose starch are averaged values. Actually, not only do starch granules with different morphologies have the different structures, but also they contain different physicochemical properties across identical and different species. For example, in wheat and barley, the large granule has higher AC, gelatinization enthalpy and pasting viscosity, and lower amylopectin short branch-chains and swelling power than its small counterpart, which results in different end uses (Salman et al., 2009; Naguleswaran et al., 2011; Li et al., 2013). Small starches are predominantly applied as fat substitute, paper coating, and as carrier material in cosmetics, whereas large starches are used to manufacture biodegradable plastic film, carbonless copy paper, and brewing beer (Lindeboom et al., 2004). Thus, separation and purification of different morphological starch granules are essential for further applications in food and non-food industries.
Research on Purified Heterogeneous Starch Granules from High-Amylose Rice TRS
Contrary to the distribution of different morphological starch granules in the grain of ae maize mutants (Liu et al., 2013), the heterogeneous starch granules with polygonal, aggregate, elongated, and hollow shapes in high-amylose rice TRS grain have obvious regional distribution from the inner region to the outer region. The polygonal starch is located in the innermost region, the aggregate, and elongated starches exist in the center region, and the hollow starch is distributed in the outmost region (Cai et al., 2014b). To investigate their individual structure and physicochemical properties, the four granule types are separated and purified on the basis of their regionally distributed characteristics (Man et al., 2014). GPC analysis shows that from the polygonal to hollow starch granules, their AC displays a gradually elevated trend, from 35.9 to 75.8%. The four granule types are essentially different in relative crystallinity, short-range ordered structure, relative proportions of single helix, double helix, and amorphous conformation; these differences imply that their structures are completely divergent (Man et al., 2014). The different structures of TRS heterogeneous starch granules yield significantly different XRD patterns, thermal properties, and digestion properties. Polygonal starch exhibits A-type crystallinity, aggregate and elongated starches show C-type crystallinity, whereas hollow starch has no crystallinity (Man et al., 2014). Cai et al. (2014b) found that polygonal and elongated starches have the lowest and highest gelatinization temperatures, respectively, while the gelatinization temperature of hollow starch is undetected due to its extremely weak or no birefringence. The in vitro digestion properties of native starches from the four granule types are investigated, and results reveal that polygonal and aggregate starches possess monophasic digestograms, whereas elongated and hollow starches exhibit biphasic digestograms from 0 to 8 h mainly because of their different structural compositions (Huang et al., 2016).
Conclusions and Future Perspectives
In maize and rice, mutating or suppressing SBEIIb is necessary to obtain high-amylose lines. However, the efficiency for an AC increase in rice is much lower than that in maize. When HAM genes are introduced to maize ae mutants and SBEI and SBEIIb are simultaneously inhibited in indica rice, AC is further increased and thus reaches 88.2 and 60%, respectively. In wheat and barley, high-amylose lines with AC (>85%) are derived by simultaneously suppressing SBEIIb and SBEIIa. The enhanced amylose synthesis and the suppressed amylopectin synthesis and the ELC from amylopectin/IC are responsible for AC increase in high-amylose species. Along with the AC increase, fewer longer branch-chains are detected in amylopectin. The significantly altered structure of high-amylose starches causes a series of other important changes, such as crystallinity, thermal properties, and hydrolysis properties.
Different morphological starches and differently stained granules are detected in high-amylose rice and maize. They are also regularly distributed in the grains from the inner to outer regions and occupy different molecular structures. Four heterogeneous granules in high-amylose rice TRS possess different physicochemical and digestion properties, which can be used for further applications in food and non-food industries. Future biochemical experiments should be performed to elucidate how heterogeneous starch granules are formed in one kernel. The different morphological starches are also detected in other high-amylose cereal crops, but whether these heterogeneous starches exhibit regional distribution in kernels and have different physicochemical properties are unknown.
Studies have also yet to identify the causes of regional distribution of heterogeneous starches in kernels. Grain filling originally begins in the core and then spreads to the outer region during endosperm development in rice and maize. Combined with regionally distributed phenotypes in high-amylose grains, various regulated mechanisms may be observed in different regions of grains. For example, white-core mutants, such as flo4, flo5, and rsr1 (Kang et al., 2005; Ryoo et al., 2007; Fu and Xue, 2010), and aberrant-periphery mutants, including flo7 (Zhang et al., 2016), play essential roles in the early stage and late stages of endosperm development in rice, respectively. However, we have yet to reveal how starch synthesis-related enzymes are regulated in grain during endosperm development from the inner parts to the outer parts.
Author Contributions
All authors listed, have made substantial, direct and intellectual contribution to the work, and approve it for publication.
Conflict of Interest Statement
The authors declare that the research was conducted in the absence of any commercial or financial relationships that could be construed as a potential conflict of interest.
Acknowledgments
This work is supported by grants from the National Key Research and Development Program of China (2016YFD0101002), the Natural Science Foundation of Jiangsu Province (BK20160457), the China Postdoctoral Science Foundation (2016590509), the Qing Lan Project of Jiangsu Province, the Talent Project of Yangzhou University, and the Priority Academic Program Development of Jiangsu Higher Education Institutions.
References
Asai, H., Abe, N., Matsushima, R., Crofts, N., Oitome, N. F., Nakamura, Y., et al. (2014). Deficiencies in both starch synthase IIIa and branching enzyme IIb lead to a significant increase in amylose in SSIIa inactive japonica rice seeds. J. Exp. Bot. 65, 5497–5507. doi: 10.1093/jxb/eru310
Avella, M., Errico, M. E., Rimedio, R., and Sadocco, P. (2002). Preparation of biodegradable polyesters/high-amylose-starch composites by reactive blending and their characterization. J. Appl. Polym. Sci. 83, 1432–1442. doi: 10.1002/app.2304
Blauth, S. L., Kim, K., Klucinec, J., Shannon, J. C., Thompson, D., and Guiltinan, M. (2002). Identification of Mutator insertional mutants of starch-branching enzyme 1 (sbe1) in Zea mays L. Plant Mol. Bio. 48, 287–297. doi: 10.1023/A:1013335217744
Blauth, S. L., Yao, Y., Klucinec, J. D., Shannon, J. C., Thompson, D. B., and Guiltinan, M. J. (2001). Identification of mutator insertional mutants of starch-branching enzyme 2a in corn. Plant Physiol. 125, 1396–1405. doi: 10.1104/pp.125.3.1396
Blazek, J., and Gilbert, E. P. (2010). Effect of enzymatic hydrolysis on native starch granule structure. Biomacromolecules 11, 3275–3289. doi: 10.1021/bm101124t
Blazek, J., and Gilbert, E. P. (2011). Application of small-angle X-ray and neutron scattering techniques to the characterisation of starch structure: a review. Carbohydr. Polym. 85, 281–293. doi: 10.1016/j.carbpol.2011.02.041
Butardo, V. M., Fitzgerald, M. A., Bird, A. R., Gidley, M. J., Flanagan, B. M., Larroque, O., et al. (2011). Impact of down-regulation of starch branching enzyme IIb in rice by artificial microRNA- and hairpin RNA-mediated RNA silencing. J. Exp. Bot. 62, 4927–4941. doi: 10.1093/jxb/err188
Cai, C., Cai, J., Zhao, L., and Wei, C. (2014a). In situ gelatinization of starch using hot stage microscopy. Food Sci. Biotechnol. 23, 15–22. doi: 10.1007/s10068-014-0003-x
Cai, C., Huang, J., Zhao, L., Liu, Q., Zhang, C., and Wei, C. (2014b). Heterogeneous structure and spatial distribution in endosperm of high-amylose rice starch granules with different morphologies. J. Agric. Food Chem. 62, 10143–10152. doi: 10.1021/jf502341q
Cai, C., Lin, L., Man, J., Zhao, L., Wang, Z., and Wei, C. (2014c). Different structural properties of high-amylose maize starch fractions varying in granule size. J. Agric. Food Chem. 62, 11711–11721. doi: 10.1021/jf503865e
Cai, C., Zhao, L., Huang, J., Chen, Y., and Wei, C. (2014d). Morphology, structure and gelatinization properties of heterogeneous starch granules from high-amylose maize. Carbohydr. Polym. 102, 606–614. doi: 10.1016/j.carbpol.2013.12.010
Cai, J., Man, J., Huang, J., Liu, Q., Wei, W., and Wei, C. (2015). Relationship between structure and functional properties of normal rice starches with different amylose contents. Carbohydr. Polym. 125, 35–44. doi: 10.1016/j.carbpol.2015.02.067
Cai, J., Yang, Y., Man, J., Huang, J., Wang, Z., Zhang, C., et al. (2014). Structural and functional properties of alkali-treated high-amylose rice starch. Food Chem. 145, 245–253. doi: 10.1016/j.foodchem.2013.08.059
Carciofi, M., Blennow, A., Jensen, S. L., Shaik, S. S., Henriksen, A., Buleon, A., et al. (2012). Concerted suppression of all starch branching enzyme genes in barley produces amylose-only starch granules. BMC Plant Biol. 12:223. doi: 10.1186/1471-2229-12-223
Cheetham, N. W. H., and Tao, L. (1998). Variation in crystalline type with amylose content in maize starch granules: an X-ray powder diffraction study. Carbohydr. Polym. 36, 277–284. doi: 10.1016/S0144-8617(98)00007-1
Crofts, N., Abe, K., Aihara, S., Itoh, R., Nakamura, Y., Itoh, K., et al. (2012). Lack of starch synthase IIIa and high expression of granule-bound starch synthase I synergistically increase the apparent amylose content in rice endosperm. Plant Sci. 193–194, 62–69. doi: 10.1016/j.plantsci.2012.05.006
Fu, F. F., and Xue, H. W. (2010). Coexpression analysis identifies rice starch regulator1, a rice AP2/EREBP family transcription factor, as a novel rice starch biosynthesis regulator. Plant Physiol. 154, 927–938. doi: 10.1104/pp.110.159517
Fujita, N., Yoshida, M., Asakura, N., Ohdan, T., Miyao, A., Hirochika, H., et al. (2006). Function and characterization of starch synthase I using mutants in rice. Plant Physiol. 140, 1070–1084. doi: 10.1104/pp.105.071845
Fujita, N., Yoshida, M., Kondo, T., Saito, K., Utsumi, Y., Tokunaga, T., et al. (2007). Characterization of SSIIIa-deficient mutants of rice: the function of SSIIIa and pleiotropic effects by SSIIIa deficiency in the rice endosperm. Plant Physiol. 144, 2009–2023. doi: 10.1104/pp.107.102533
Gao, M., Fisher, D. K., Kim, K. N., Shannon, J. C., and Guiltinan, M. J. (1997). Independent genetic control of maize starch-branching enzymes IIa and IIb. Plant Physiol. 114, 69–78. doi: 10.1104/pp.114.1.69
Granfeldt, Y., Drews, A., and Björck, I. (1995). Arepas made from high amylose corn flour produce favorably Low glucose and insulin responses in healthy humans. J. Nutr. 125, 459–465.
Guan, H., Li, P., Imparl-Radosevich, J., Preiss, J., and Keeling, P. (1997). Comparing the properties of Escherichia coli branching enzyme and maize branching enzyme. Arch. Biochem. Biophys. 342, 92–98. doi: 10.1006/abbi.1997.0115
Guan, H. P., and Preiss, J. (1993). Differentiation of the properties of the branching isozymes from maize (Zea mays). Plant Physiol. 102, 1269–1273. doi: 10.1104/pp.102.4.1269
Huang, J., Lin, L., Wang, J., Wang, Z., Liu, Q., and Wei, C. (2016). In vitro digestion properties of heterogeneous starch granules from high-amylose rice. Food Hydrocolloids 54, 10–22. doi: 10.1016/j.foodhyd.2015.09.016
Huang, J., Shang, Z., Man, J., Liu, Q., Zhu, C., and Wei, C. (2015). Comparison of molecular structures and functional properties of high-amylose starches from rice transgenic line and commercial maize. Food Hydrocolloids 46, 172–179. doi: 10.1016/j.foodhyd.2014.12.019
Isshiki, M., Nakajima, M., Satoh, H., and Shimamoto, K. (2000). Dull: rice mutants with tissue-specific effects on the splicing of the waxy pre-mRNA. Plant J. 23, 451–460. doi: 10.1046/j.1365-313x.2000.00803.x
Itoh, K., Ozaki, H., Okada, K., Hori, H., Takeda, Y., and Mitsui, T. (2003). Introduction of Wx transgene into rice wx mutants leads to both high- and low-amylose rice. Plant Cell Physiol. 44, 473–480. doi: 10.1093/pcp/pcg068
Jane, J., Chen, Y. Y., Lee, L. F., McPherson, A. E., Wong, K. S., Radosavljevic, M., et al. (1999). Effects of amylopectin branch chain length and amylose content on the gelatinization and pasting properties of starch. Cereal Chem. 76, 629–637. doi: 10.1094/CCHEM.1999.76.5.629
Jane, J. L., and Chen, J. F. (1992). Effect of amylose molecular size and amylopectin branch chain length on paste properties of starch. Cereal Chem. 69, 60–65.
Jeon, J. S., Ryoo, N., Hahn, T. R., Walia, H., and Nakamura, Y. (2010). Starch biosynthesis in cereal endosperm. Plant Physiol. Biochem. 48, 383–392. doi: 10.1016/j.plaphy.2010.03.006
Jiang, H., Campbell, M., Blanco, M., and Jane, J. L. (2010d). Characterization of maize amylose-extender (ae) mutant starches: part II. structures and properties of starch residues remaining after enzymatic hydrolysis at boiling-water temperature. Carbohydr. Polym. 80, 1–12. doi: 10.1016/j.carbpol.2009.10.060
Jiang, H., Horner, H. T., Pepper, T. M., Blanco, M., Campbell, M., and Jane, J. L. (2010a). Formation of elongated starch granules in high-amylose maize. Carbohyd. Polym. 80, 533–538. doi: 10.1016/j.carbpol.2009.12.016
Jiang, H., Jane, J. L., Acevedo, D., Green, A., Shinn, G., Schrenker, D., et al. (2010b). Variations in starch physicochemical properties from a generation-means analysis study using amylomaize V and VII parents. J. Agric. Food Chem. 58, 5633–5639. doi: 10.1021/jf904531d
Jiang, H., Lio, J., Blanco, M., Campbell, M., and Jane, J. L. (2010c). Resistant-starch formation in high-amylose maize starch during kernel development. J. Agric. Food Chem. 58, 8043–8047. doi: 10.1021/jf101056y
Kang, H. G., Park, S., Matsuoka, M., and An, G. (2005). White-core endosperm floury endosperm-4 in rice is generated by knockout mutations in the C4-type pyruvate orthophosphate dikinase gene (OsPPDKB). Plant J. 42, 901–911. doi: 10.1111/j.1365-313X.2005.02423.x
Kang, H. J., Hwang, I. K., Kim, K. S., and Choi, H. C. (2003). Comparative structure and physicochemical properties of Ilpumbyeo, a high-quality japonica rice, and its mutant, Suweon 464. J. Agric. Food Chem. 51, 6598–6603. doi: 10.1021/jf0344946
Kasemsuwan, T., Jane, J., Schnable, P., Stinard, P., and Robertson, D. (1995). Characterization of the dominant mutant amylose-extender (Ae1-5180) maize starch. Cereal Chem. 72, 457–464.
Kaur, L., Singh, J., McCarthy, O. J., and Singh, H. (2007). Physico-chemical, rheological and structural properties of fractionated potato starches. J. Food Eng. 82, 383–394. doi: 10.1016/j.jfoodeng.2007.02.059
Kim, J. C., Kong, B. W., Kim, M. J., and Lee, S. H. (2008). Amylolytic hydrolysis of native starch granules affected by granule surface area. J. Food Sci. 73, C621–C624. doi: 10.1111/j.1750-3841.2008.00944.x
Li, J. H., Vasanthan, T., Hoover, R., and Rossnagel, B. G. (2004). Starch from hull-less barley: V. in-vitro susceptibility of waxy, normal, and high-amylose starches towards hydrolysis by alpha-amylases and amyloglucosidase. Food Chem. 84, 621–632. doi: 10.1016/S0308-8146(03)00287-5
Li, L., Jiang, H., Campbell, M., Blanco, M., and Jane, J. L. (2008). Characterization of maize amylose-extender (ae) mutant starches. Part I: relationship between resistant starch contents and molecular structures. Carbohydr. Polym. 74, 396–404. doi: 10.1016/j.carbpol.2008.03.012
Li, W., Shan, Y., Xiao, X., Luo, Q., Zheng, J., Ouyang, S., et al. (2013). Physicochemical properties of A- and B-starch granules isolated from hard red and soft red winter wheat. J. Agric. Food Chem. 61, 6477–6484. doi: 10.1021/jf400943h
Lin, L., Cai, C., Gibert, R. G., Li, E., Wang, J., and Wei, C. (2016a). Relationships between amylopectin molecular structures and functional properties of different-sized fractions of normal and high-amylose maize starches. Food Hydrocolloids 52, 359–368. doi: 10.1016/j.foodhyd.2015.07.019
Lin, L., Guo, D., Huang, J., Zhang, X., Zhang, L., and Wei, C. (2016b). Molecular structure and enzymatic hydrolysis properties of starches from high-amylose maize inbred lines and their hybrids. Food Hydrocolloids 58, 246–254. doi: 10.1016/j.foodhyd.2016.03.001
Lin, L., Guo, D., Zhao, L., Zhang, X., Wang, J., Zhang, F., et al. (2016c). Comparative structure of starches from high-amylose maize inbred lines and their hybrids. Food Hydrocolloids 52, 19–28. doi: 10.1016/j.foodhyd.2015.06.008
Lindeboom, N., Chang, P. R., and Tyler, R. T. (2004). Analytical biochemical and physicochemical aspects of starch granule size, with emphasis on small granule starch: a review. Starch 56, 89–99. doi: 10.1002/star.200300218
Liu, D., Parker, M. L., Wellner, N., Kirbyb, A. R., Cross, K., Morris, V. J., et al. (2013). Structural variability between starch granules in wild type and in ae high-amylose mutant maize kernels. Carbohydr. Polym. 97, 458–468. doi: 10.1016/j.carbpol.2013.05.013
Man, J., Lin, L., Wang, Z., Wang, Y., Liu, Q., and Wei, C. (2014). Different structures of heterogeneous starch granules from high-amylose rice. J. Agric. Food Chem. 62, 11254–11263. doi: 10.1021/jf503999r
Man, J., Qin, F., Zhu, L., Shi, Y., Gu, M., Liu, Q., et al. (2012a). Ordered structure and thermal property of acid-modified high-amylose rice starch. Food Chem. 134, 2242–2248. doi: 10.1016/j.foodchem.2012.04.100
Man, J., Yang, Y., Huang, J., Zhang, C., Chen, Y., Wang, Y., et al. (2013a). Effect of simultaneous inhibition of starch branching enzymes I and IIb on the crystalline structure of rice starches with different amylose content. J. Agric. Food Chem. 61, 9930–9937. doi: 10.1021/jf4030773
Man, J., Yang, Y., Huang, J., Zhang, C., Zhang, F., Wang, Y., et al. (2013b). Morphology and structural properties of high-amylose rice starch residues hydrolyzed by amyloglucosidase. Food Chem. 138, 2089–2098. doi: 10.1016/j.foodchem.2012.12.009
Man, J., Yang, Y., Zhang, C., Zhang, F., Wang, Y., Liu, Q., et al. (2013c). Morphology and structural characterization of high-amylose rice starch residues hydrolyzed by porcine pancreatic α-amylase. Food Hydrocolloids 31, 195–203. doi: 10.1016/j.foodhyd.2012.11.003
Man, J., Yang, Y., Zhang, C., Zhou, X., Dong, Y., Zhang, F., et al. (2012b). Structural changes of high-amylose rice starch residues following in vitro and in vivo digestion. J. Agric. Food Chem. 60, 9332–9341. doi: 10.1021/jf302966f
Morell, M. K., Kosar-Hashemi, B., Cmiel, M., Samuel, M. S., Chandler, P., Rahman, S., et al. (2003). Barley sex6 mutants lack starch synthase IIa activity and contain a starch with novel properties. Plant J. 34, 173–185. doi: 10.1046/j.1365-313X.2003.01712.x
Naguleswaran, S., Li, J., Vasanthan, T., and Bressler, D. (2011). Distribution of granule channels, protein, and phospholipid in triticale and corn starches as revealed by confocal laser scanning microscopy. Cereal Chem. 88, 87–94. doi: 10.1094/CCHEM-04-10-0062
Naguleswaran, S., Li, J., Vasanthan, T., Bressler, D., and Hoover, R. (2012). Amylolysis of large and small granules of native triticale, wheat and corn starches using a mixture of α-amylase and glucoamylase. Carbohydr. Polym. 88, 864–874. doi: 10.1016/j.carbpol.2012.01.027
Nakamura, Y. (2002). Towards a better understanding of the metabolic system for amylopectin biosynthesis in plants: rice endosperm as a model tissue. Plant Cell Physiol. 43, 718–725. doi: 10.1093/pcp/pcf091
Nakamura, Y., Utsumi, Y., Sawada, T., Aihara, S., Utsumi, C., Yoshida, M., et al. (2010). Characterization of the reactions of starch branching enzymes from rice endosperm. Plant Cell Physiol. 51, 776–794. doi: 10.1093/pcp/pcq035
Nishi, A., Nakamura, Y., Tanaka, N., and Satoh, H. (2001). Biochemical and genetic analysis of the effects of amylose-extender mutation in rice endosperm. Plant Physiol. 127, 459–472. doi: 10.1104/pp.010127
Nugent, A. P. (2005). Health properties of resistant starch. Nutr. Bull. 30, 27–54. doi: 10.1111/j.1467-3010.2005.00481.x
Pan, T., Zhao, L., Lin, L., Wang, J., Liu, Q., and Wei, C. (2017). Changes in kernel morphology and starch properties of high-amylose brown rice during the cooking process. Food Hydrocolloids 66, 227–236. doi: 10.1016/j.foodhyd.2016.11.035
Peymanpour, G., Marcone, M., Ragaee, S., Tetlow, I., Lane, C. C., Seetharaman, K., et al. (2016). On the molecular structure of the amylopectin fraction isolated from “high-amylose” ae maize starches. Int. J. Biol. Macromol. 91, 768–777. doi: 10.1016/j.ijbiomac.2016.06.029
Qin, F., Man, J., Cai, C., Xu, B., Gu, M., Zhu, L., et al. (2012). Physicochemical properties of high-amylose rice starches during kernel development. Carbohydr. Polym. 88, 690–698. doi: 10.1016/j.carbpol.2012.01.013
Qin, F., Man, J., Xu, B., Hu, M., Gu, M., Liu, Q., et al. (2011). Structural properties of hydrolyzed high-amylose rice starch by α-amylase from Bacillus licheniformis. J. Agric. Food Chem. 59, 12667–12673. doi: 10.1021/jf203167f
Regina, A., Berbezy, P., Kosar-Hashemi, B., Li, S., Cmiel, M., Larroque, O., et al. (2015). A genetic strategy generating wheat with very high amylose content. Plant Biotechnol. J. 13, 1276–1286. doi: 10.1111/pbi.12345
Regina, A., Bird, A., Topping, D., Bowden, S., Freeman, J., Barsby, T., et al. (2006). High-amylose wheat generated by RNA interference improves indices of large-bowel health in rats. Proc. Natl. Acad. Sci. U.S.A. 103, 3546–3551. doi: 10.1073/pnas.0510737103
Regina, A., Blazek, J., Gilbert, E., Flanagan, B. M., Gidley, M. J., Cavanagh, C., et al. (2012). Differential effects of genetically distinct mechanisms of elevating amylose on barley starch characteristics. Carbohydr. Polym. 89, 979–991. doi: 10.1016/j.carbpol.2012.04.054
Regina, A., Kosar-Hashemi, B., Ling, S., Li, Z., Rahman, S., and Morell, M. (2010). Control of starch branching in barley defined through differential RNAi suppression of starch branching enzyme IIa and IIb. J. Exp. Bot. 61, 1469–1482. doi: 10.1093/jxb/erq011
Regina, A., Kosar-Hashemi, B., Li, Z., Rampling, L., Cmiel, M., Gianibelli, M. C., et al. (2004). Multiple isoforms of starch branching enzyme-I in wheat: lack of the major SBE-I isoform does not alter starch phenotype. Funct. Plant Biol. 31, 591–601. doi: 10.1071/FP03193
Ryoo, N., Yu, C., Park, C. S., Baik, M. Y., Park, I. M., Cho, M. H., et al. (2007). Knockout of a starch synthase gene OsSSIIIa/Flo5 causes white-core floury endosperm in rice (Oryza sativa L.). Plant Cell Rep. 26, 1083–1095. doi: 10.1007/s00299-007-0309-8
Salman, H., Blazek, J., Lopez-Rubio, A., Gilbert, E. P., Hanley, T., and Copeland, L. (2009). Structure-function relationships in A and B granules from wheat starches of similar amylose content. Carbohydr. Polym. 75, 420–427. doi: 10.1016/j.carbpol.2008.08.001
Sang, Y., Bean, S., Seib, P. A., Pedersen, J., and Shi, Y. C. (2008). Structure and functional properties of sorghum starches differing in amylose content. J. Agric. Food Chem. 56, 6680–6685. doi: 10.1021/jf800577x
Satoh, H., Nishi, A., Fujita, N., Kubo, A., Nakamura, Y., Kawasaki, T., et al. (2003a). Isolation and characterization of starch mutants in rice. J. Appl. Glycosci. 50, 225–230. doi: 10.5458/jag.50.225
Satoh, H., Nishi, A., Yamashita, K., Takemoto, Y., Tanaka, Y., Hosaka, Y., et al. (2003b). Starch-branching enzyme I-deficient mutation specifically affects the structure and properties of starch in rice endosperm. Plant Physiol. 133, 1111–1121. doi: 10.1104/pp.103.021527
Satoh, H., Shibahara, K., Tokunaga, T., Nishi, A., Tasaki, M., Hwang, S. K., et al. (2008). Mutation of the plastidial α-glucan phosphorylase gene in rice affects the synthesis and structure of starch in the endosperm. Plant Cell 20, 1833–1849. doi: 10.1105/tpc.107.054007
Sestili, F., Botticella, E., Proietti, G., Janni, M., D'Ovidio, R., and Lafiandra, D. (2012). Amylose content is not affected by overexpression of the Wx-B1 gene in durum wheat. Plant Breed. 131, 700–706. doi: 10.1111/j.1439-0523.2012.02004.x
Shannon, J. C., Garwood, D. L., and Boyer, C. D. (2009). “Genetics and physiology of starch developmen,” in Starch, Chemistry and Technology, eds J. BeMiller and R. L. Whistler (New York, NY: Academic Press), 23–82.
Slade, A. J., McGuire, C., Loeffler, D., Mullenberg, J., Skinner, W., Fazio, G., et al. (2012). Development of high amylose wheat through TILLING. BMC Plant Biol. 12:69. doi: 10.1186/1471-2229-12-69
Smith, A. M., Zeeman, S. C., and Denyer, K. (2002). “The synthesis of amylose,” in Starch, Advance in Structure and Function, eds T. L. Barsby, A. M. Donald, and P. J. Frazier (Cambridge: Royal Society of Chemistry), 150–163.
Takeda, Y., Guan, H. P., and Preiss, J. (1993). Branching of amylose by the branching isoenzymes of maize endosperm. Carbohydr. Res. 240, 253–263. doi: 10.1016/0008-6215(93)84188-C
Tanaka, N., Fujita, N., Nishi, A., Satoh, H., Hosaka, Y., Ugaki, M., et al. (2004). The structure of starch can be manipulated by changing the expression levels of starch branching enzyme llb in rice endosperm. Plant Biotech. J. 2, 507–516. doi: 10.1111/j.1467-7652.2004.00097.x
Tawil, G., Viksø-Nielsen, A., Rolland-Sabaté, A., Colonna, P., and Buléon, A. (2011). In depth study of a new highly efficient raw starch hydrolyzing a-amylase from Rhizomucor sp. Biomacromolecules 12, 34–42. doi: 10.1021/bm100913z
Tester, R. F., and Morrison, W. R. (1992). Swelling and gelatinization of cereal starches. III. some properties of waxy and normal nonwaxy barley starches. Cereal Chem. 69, 654–658.
Tetlow, I. J., and Emes, M. J. (2014). A review of starch-branching enzymes and their role in amylopectin biosynthesis. IUBMB Life 66, 546–558. doi: 10.1002/iub.1297
Tsai, C. Y., Salamini, F., and Nelson, O. E. (1970). Enzymes of carbohydrate metabolism in the developing endosperm of maize. Plant Physiol. 46, 299–306. doi: 10.1104/pp.46.2.299
Tziotis, A., Seetharaman, K., Wong, K. S., Klucinec, J. D., Jane, J. L., and White, P. J. (2004). Structural properties of starch fractions isolated from normal and mutant corn genotypes using different methods. Cereal Chem. 81, 611–620. doi: 10.1094/CCHEM.2004.81.5.611
Umemoto, T., Aoki, N., Lin, H., Nakamura, Y., Inouchi, N., Sato, Y., et al. (2004). Natural variation in rice starch synthase IIa affects enzyme and starch properties. Funct. Plant Biol. 31, 671–684. doi: 10.1071/FP04009
Umemoto, T., Horibata, T., Aoki, N., Hiratsuka, M., Yano, M., and Inouchi, N. (2008). Effects of variations in starch synthase on starch properties and eating quality of rice. Plant Prod. Sci. 11, 472–480. doi: 10.1626/pps.11.472
Umemoto, T., and Terashima, K. (2002). Activity of granule-bound starch synthase is an important determinant of amylose content in rice endosperm. Funct. Plant Biol. 29, 1121–1124. doi: 10.1071/PP01145
Wang, Y. J., White, P., Pollak, L., and Jane, J. (1993). Amylopectin and intermediate materials in starches from mutant genotypes of the Oh43 inbred line. Cereal Chem. 70, 521–525.
Wei, C., Qin, F., Zhou, W., Chen, Y., Xu, B., Wang, Y., et al. (2010a). Formation of semi-compound C-type starch granule in high-amylose rice developed by antisense RNA inhibition of starch branching enzyme. J. Agric. Food Chem. 58, 11097–11104. doi: 10.1021/jf1024533
Wei, C., Qin, F., Zhou, W., Xu, B., Chen, C., Chen, Y., et al. (2011). Comparison of the crystalline properties and structural changes of starches from high-amylose transgenic rice and its wild type during heating. Food Chem. 128, 645–652. doi: 10.1016/j.foodchem.2011.03.080
Wei, C., Qin, F., Zhou, W., Yu, H., Xu, B., Chen, C., et al. (2010b). Granule structure and distribution of allomorphs in C-type high-amylose rice starch granule modified by antisense RNA inhibition of starch branching enzyme. J. Agric. Food Chem. 58, 11946–11854. doi: 10.1021/jf103412d
Wei, C., Qin, F., Zhu, L., Zhou, W., Chen, Y., Wang, Y., et al. (2010c). Microstructure and ultrastructure of high-amylose rice resistant starch granules modified by antisense RNA inhibition of starch branching enzyme. J. Agric. Food Chem. 58, 1224–1232. doi: 10.1021/jf9031316
Wei, C., Xu, B., Qin, F., Yu, H., Chen, C., Meng, X., et al. (2010d). C-type starch from high-amylose rice resistant starch granules modified by antisense RNA inhibition of starch branching enzyme. J. Agric. Food Chem. 58, 7383–7388. doi: 10.1021/jf100385m
Wellner, N., Georget, D. M. R., Parker, M. L., and Morris, V. J. (2011). In situ Raman microscopy of starch granule structures in wild type and ae mutant maize kernels. Starch 63, 128–138. doi: 10.1002/star.201000107
Wu, Y., Campbell, M., Yang, Y., Lii, Z. W., and Ibrahim, A. M. H. (2009). Genetic analysis of high amylose content in maize (Zea mays L.) using a triploid endosperm model. Euphytica 166, 155–164. doi: 10.1007/s10681-008-9798-y
Xia, H., Yandeau-Nelson, M., Thompson, D. B., and Guiltinan, M. J. (2011). Deficiency of maize starch-branching enzyme i results in altered starch fine structure, decreased digestibility and reduced coleoptile growth during germination. BMC Plant Biol. 11:95. doi: 10.1186/1471-2229-11-95
Yamamori, M., Fujita, S., Hayakawa, K., Matsuki, J., and Yasui, T. (2000). Genetic elimination of a starch granule protein, SGP-1, of wheat generates an altered starch with apparent high amylose. Theor. Appl. Genet. 101, 21–29. doi: 10.1007/s001220051444
Yao, Y., Thompson, D. B., and Guiltinan, M. J. (2004). Maize starch-branching enzyme isoforms and amylopectin structure. In the absence of starch-branching enzyme IIb, the further absence of starch-branching enzyme Ia leads to increased branching. Plant Physiol. 136, 3515–3523. doi: 10.1104/pp.104.043315
Yu, Y., Mu, H. H., Wassermanm, B. P., and Carman, G. M. (2001). Identification of the maize amyloplast stromal 112-kD protein as a plastidic starch phosphorylase. Plant Physiol. 125, 351–359. doi: 10.1104/pp.125.1.351
Zhang, L., Ren, Y., Lu, B., Yang, C., Feng, Z., Liu, Z., et al. (2016). FLOURY ENDOSPERM7 encodes a regulator of starch synthesis and amyloplast development essential for peripheral endosperm development in rice. J. Exp. Bot. 67, 633–647. doi: 10.1093/jxb/erv469
Zhang, X., Colleoni, C., Ratushna, V., Sirghle-Colleoni, M., James, M. G., and Myers, A. M. (2004). Molecular characterization demonstrates that the Zea mays gene sugary 2 codes for the starch synthase isoform SSIIa. Plant Mol. Biol. 54, 865–879. doi: 10.1007/s11103-004-0312-1
Zhou, H., Wang, L., Liu, G., Meng, X., Jing, Y., Shu, X., et al. (2016). Critical roles of soluble starch synthase SSIIIa and granule-bound starch synthase Waxy in synthesizing resistant starch in rice. Proc. Natl. Acad. Sci. U.S.A. 2016, 12844–12849. doi: 10.1073/pnas.1615104113
Keywords: high-amylose cereal crop, starch branching enzyme, starch molecular structure, starch property, heterogeneous starch granule
Citation: Wang J, Hu P, Chen Z, Liu Q and Wei C (2017) Progress in High-Amylose Cereal Crops through Inactivation of Starch Branching Enzymes. Front. Plant Sci. 8:469. doi: 10.3389/fpls.2017.00469
Received: 06 December 2016; Accepted: 17 March 2017;
Published: 04 April 2017.
Edited by:
Chengdao Li, Murdoch University, AustraliaReviewed by:
Steven Graham Ball, Lille University of Science and Technology, FranceAlberto A. Iglesias, National University of the Littoral, Argentina
Daniel Cozzolino, Central Queensland University, Australia
Xiangbai Dong, Institute of Botany (CAS), China
Copyright © 2017 Wang, Hu, Chen, Liu and Wei. This is an open-access article distributed under the terms of the Creative Commons Attribution License (CC BY). The use, distribution or reproduction in other forums is permitted, provided the original author(s) or licensor are credited and that the original publication in this journal is cited, in accordance with accepted academic practice. No use, distribution or reproduction is permitted which does not comply with these terms.
*Correspondence: Cunxu Wei, cxwei@yzu.edu.cn