Abstract
Keywords
Portulaca oleracea WBC Oxidant and antioxidant levels Lung inflammation Asthmatic rats
Introduction
Portulaca oleracea (P.oleracea) commonly known as “purslane”, is an annual plant with yellow flowers and smooth, reddish, mostly prostrate stems and alternate leaves, which belongs to the Portulacaceae family and grows in the Middle East, India, Europe, Africa, North America, Australia, and Asia (1). P. oleracea contains potassium, magnesium, calcium, omega-3 fatty acid, alpha-linolenic acid, gamma-linolenic acid, alpha-tocopherol and ascorbic acid (2). Several therapeutic effects such as bronchodilatory (3), muscle relaxant (4), antioxidant (5), anti-tussive (6) and anti-inflammatory (7) activities were reported for this plant. Consistently, our previous studies demonstrated antioxidant, anti-inflammatory and immunomodulatory effects for P. oleracea in ovalbumin (OVA)-sensitized rats (8, 9). Aqueous extract of P. oleracea has been demonstrated to inhibit intracellular reactive oxygen species (ROS) production and NF-κB activation in vascular endothelial cells (10).
Asthma is a chronic inflammatory disease that is defined as reversible airway obstruction, bronchial hyperresponsiveness and increased airway inflammation, inflammatory cells infiltration, and mucus production (11, 12). Inflammatory cells such as T cells, mast cells, basophils, macrophages, and eosinophils are involved in the inflammatory processes underlying asthma (13). Concerning these cells, increased number of eosinophils is a characteristic feature of asthma (14). In this regard, several studies indicated that total WBC and eosinophil counts were enhanced in asthmatic animals and patients (15, 16). Exposure to allergens and respiratory infections causes migration of inflammatory cells and production of inflammatory mediators (17). Increased levels of inflammatory mediators such as ROS, histamine and products of arachidonic acid metabolism, lead to airway remodeling and pathological features such as mucus hypersecretion, smooth muscle hyperplasia, sub-epithelial fibrosis, thickening of the airway walls, infiltration of inflammatory cells, increased smooth muscle mass, vascular congestion and airway epithelial shedding (18). Structural changes in the airways induce irreversible airflow obstruction and airway hyperresponsiveness in asthma (19).
Therefore, the present study aimed to evaluate the effect of hydro-ethanolic extract of P. oleracea on total and differential WBC, the levels of nitric oxide (NO), malondialdehyde (MDA), catalase (CAT), superoxide dismutase (SOD) and thiol in asthmatic rats BALF and study pathological features of their lungs.
Experimental
Plant collection and extraction
P. oleracea was collected from Sabzevar city, Khorasan Razavi province, Iran, in July 2017. A voucher sample was kept at the herbarium of the School of Pharmacy, Mashhad University of Medical Sciences (Herbarium No. 240-1615-12). The leaves of P. oleracea were grounded to powder (100 g), mixed with 70% ethanol at a ratio of 1:10 (powder: ethanol) and left for 3 days at 37 °C with occasional shaking and stirring. The mixture was then filtered and the resulting liquid was concentrated under reduced pressure at 45 °C in an Eyela rotary evaporator (Heidolph, Germany). The yield of extraction was 17.5% (9).
Animals
Experiments were performed using Wistar rats (200 ± 20 g) purchased from the animal house of School of Medicine, Mashhad University of Medical Sciences, Mashhad, Iran. The animals were kept in cages receiving clean filtered air (Maximiser, Thoren Caging System Inc., Hazleton, PA, U.S.A.) under standard conditions at 22 ± 2 oC with regular 12 hr/ 12 hr light/dark cycles. They also had free access to food and water ad libitum during the experimental period.
Animals sensitization
A rat model of asthma was induced by three i.p. injections of 1 mg/kg of OVA, along with 0.9% sterile saline containing 100 mg Al(OH)3 as an adjuvant on days 1, 2 and 3 of the experiment. On days 6, 9, 12, 15, 18 and 21 of the experiment, animals were challenged with 1% OVA aerosol produced by a DeVilbiss PulmoSonic nebulizer (DeVilbiss Health Care Ltd., Feltham, U.K.) in a whole-body inhalation exposure chamber of 0.8 m3 for 20 min/day (16).
Experimental groups
Animals were randomly divided into six groups (n = 8 in each group) including: 1) Control group (group C) which was given intra-peritoneal (i.p.) and inhaled normal saline; 2) Asthmatic group (group A) which was sensitized with OVA; 3) Asthmatic group treated with dexamethasone 1.25 μg/mL (group A+D); and 4-6) Asthmatic groups treated with P. oleracea extract 1, 2, and 4 mg/mL (groups A+PO 1, 2, and 4 mg/mL, respectively). P. oleracea extract and dexamethasone were added to animals’ drinking water during the 21-day sensitization period. Each animal consumed almost 40 ml water per day and the volume of consumption was not significantly different among different groups; thus, dexamethasone-treated rats received 50 μg/day and PO-treated rats received 40, 80 or 160 mg/day of the extract for 21 days.
Collection of bronchoalveolar lavage fluid (BALF)
All rats were sacrificed on day 22 by ketamine (50 mg/kg, i.p.), their chest was opened, and the trachea and lungs were removed. The left lung was lavaged five times with 1 mL saline (a total of 5 mL). Then, BALF was centrifuged at 2500 g at 4 °C for 10 min and supernatants were collected and stored at -80 °C until analysis. Total and differential WBC counts as well as oxidant/anti-oxidant biomarkers levels were then assessed in the BALF.
Measurement of total and differential WBC count
To count total leukocytes, 1 mL of BALF was stained with Turk’s solution (containing 1 mL glacial acetic acid, 1 mL gentian violet solution 1% and 100 ml pure water) and total WBC was determined in duplicate using a hemocytometer (in a Burker chamber). The rest of BALF was centrifuged at 2500 g at 4 °C for 10 min. For differential WBC count, a smear was prepared from the cell pellet in BALF and stained with Wright-Giemsa. After staining, differential counts were determined in accordance with standard morphologic protocols under a light microscope by counting a total of 100 cells/slide. Then, the percentage of each leukocyte was calculated (20).
Measurement of oxidant and antioxidant levels
Total stable oxidation products of NO metabolism (NO2-/NO3-) in BALF supernatant was evaluated using the Griess reagent containing sulfanilamide (SULF) and N-(1-Naphthyl) ethylenediamine dihydrochloride (NEDD). The frozen BALF was thawed at 25˚ C, and deproteinized using zinc sulfate (Sigma, America). The liquefied BALF was then centrifuged at 12000 g for 10 min. Next, 300 μL of the clear supernatant was mixed with Griess reagent in water, in a test tube. For reduction of nitrate to nitrite, 300 μL saturated solutions of vanadium (III) chloride (VCl3; Sigma, USA) in 1 M HCl was added to the mixture and incubated for 2 hr at 30 °C, in the dark. Then, the absorbance of samples was assessed at 540 nm against a blank containing the same concentrations of ingredients but no biological sample. Linear regression was used to determine NO concentration using a standard curve plotted for NaNO2. The final results were expressed as μmol (20).
Moreover, MDA levels, as an index of lipid peroxidation, was measured. MDA reacts with thiobarbituric acid (TBA) as a thiobarbituric acid reactive substance (TBARS) to produce a red complex with the maximum absorbance at 535 nm. For MDA measurement, 2 mL of TBA/trichloroacetic acid (TCA)/HCl was added to 1 mL of BALF supernatant and the mixture was heated in a water bath for 40 min. Then, the mixture was centrifuged at 1000 g for 10 min. Finally, the absorbance was measured at 535 nm (20).
A colorimetric assay involving production of superoxide by pyrogallol autoxidation and prevention of diminution of the tetrazolium dye, MTT (3-(4,5-dimethylthiazol-2-yl)2,5-diphenyltetrazolium bromide) to formazan by SOD, was used and measured at 570 nm (20). One unit of SOD activity was defined as the quantity of enzyme required for prevention of MTT reduction rate by 50%.
Catalase (CAT) activity was assessed based on the rate constant k (dimension: S-1, k) of hydrogen peroxide decomposition. The reduction in absorbance at 240 nm per minute and the rate constant of the enzyme were determined. Activities were defined as k (rate constant) per liter (20).
Total thiol concentration was measured using DTNB reagent which reacts with thiol moieties to produce a yellow complex with the maximum absorbance at 412 nm. Briefly, 1 mL tris-ethylenediaminetetraacetic acid (Tris-EDTA) buffer (pH 8.6) was added to 50 μL serum supernatant in 1-mL cuvettes and sample absorbance was read at 412 nm against Tris-EDTA buffer alone (A1). Then, 20 μL DTNB reagents (10 mmol/ L in methanol) was added to the mixture and after 15 min (at room temperature), the sample absorbance was read again (A2). The absorbance of DTNB reagent alone was also read as blank (B). Total thiol concentration (mmol/L) was calculated using the following equation (20):
Total thiol concentration (mmol/L) = (A2–A1–B)×1.07/0.05×13.6.
Pathological evaluations
After sacrificing the animals, lungs were removed and placed into buffered formalin 10% (Merck, Darmstadt, Germany). Seven days later, tissues were dried using Auto Technicon apparatus and cleared by passage of tissues through 70–100% ethanol and xylol and paraffin blocks were prepared. The specimens were cut into 4-μm slices and stained with hematoxylin and eosin (H&E). The tissues were then evaluated under a light microscope. In this study, we focused on the following pathological changes in the lungs of asthmatic and treated groups: interstitial inflammation, interstitial fibrosis, bleeding, emphysema and epithelial damage. Scoring of pathological changes was performed as previously explained: 0) No pathologic changes; 1) Patchy changes; and 2) severe changes (in most parts of the lung) (21).
Statistical Analysis
The data of total and differential WBC count, oxidant/antioxidant biomarkers levels and lung pathological studies were expressed as mean ± SEM. The comparisons of the data obtained from P. oleracea-treated groups, asthma group and control group were made using one way analysis of variance (ANOVA) with Tukey-Kramer’s post-test. Significance was accepted at p < 0.05.
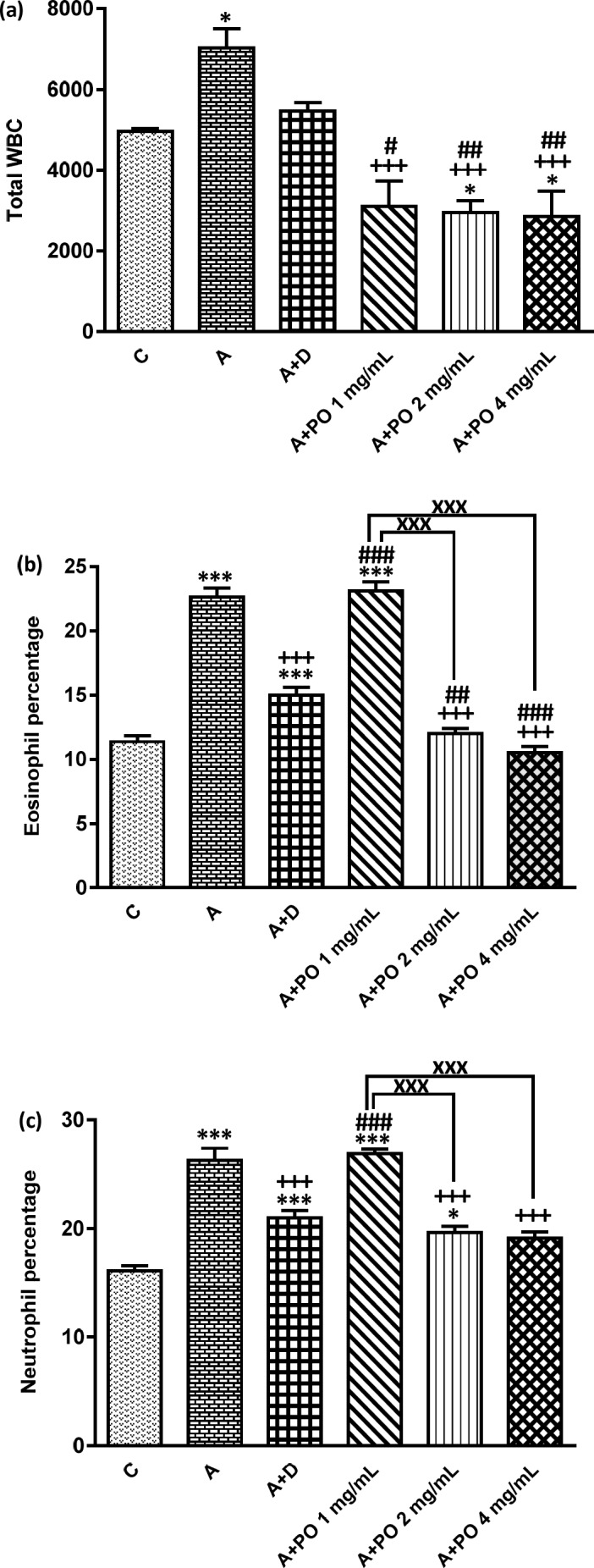
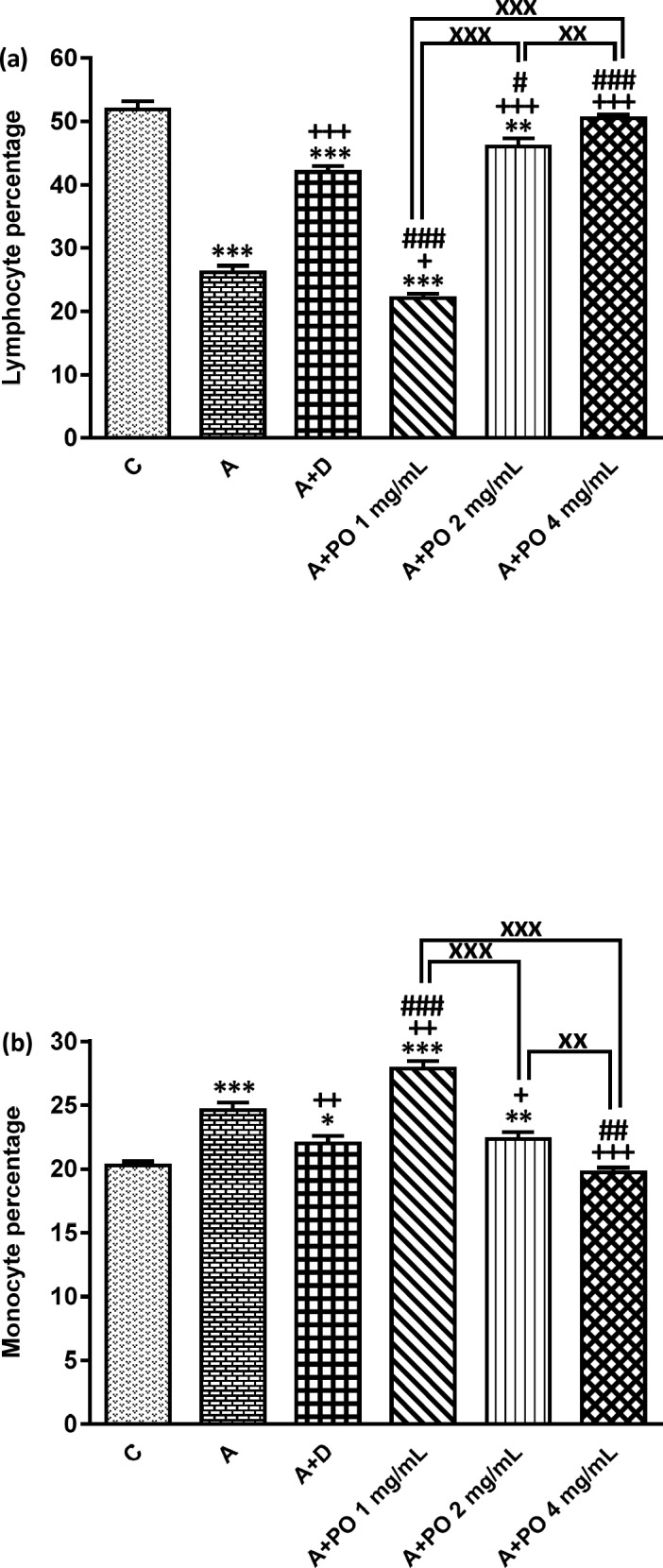
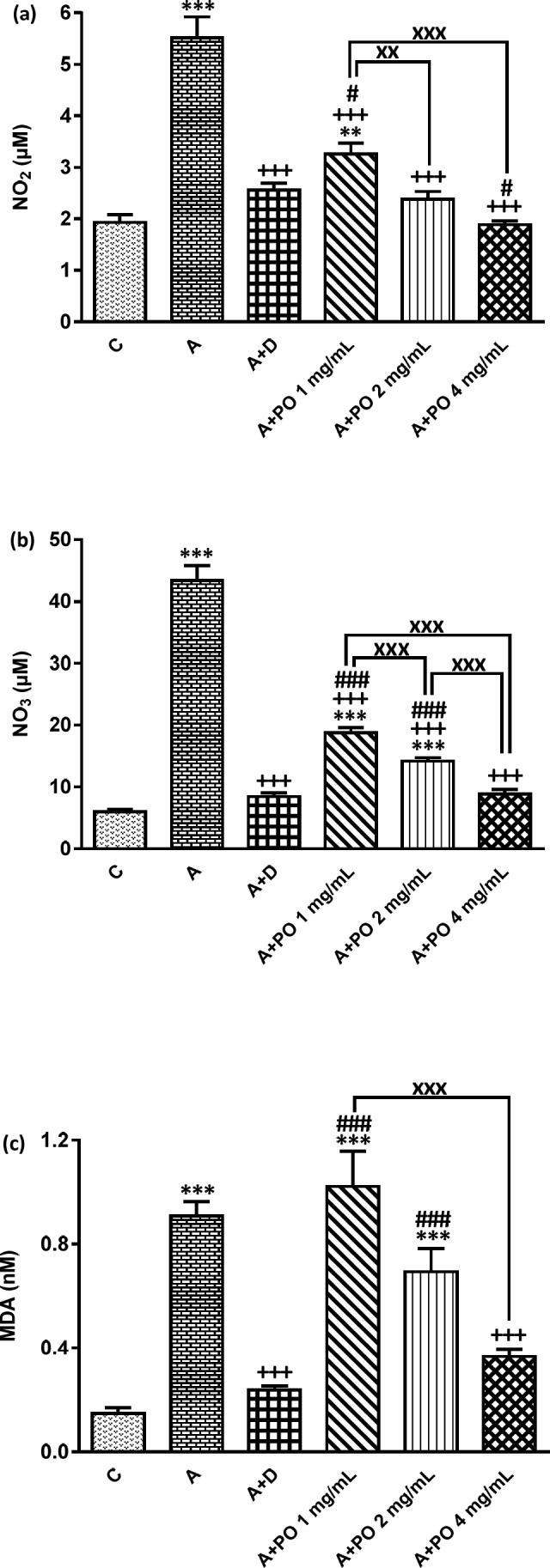
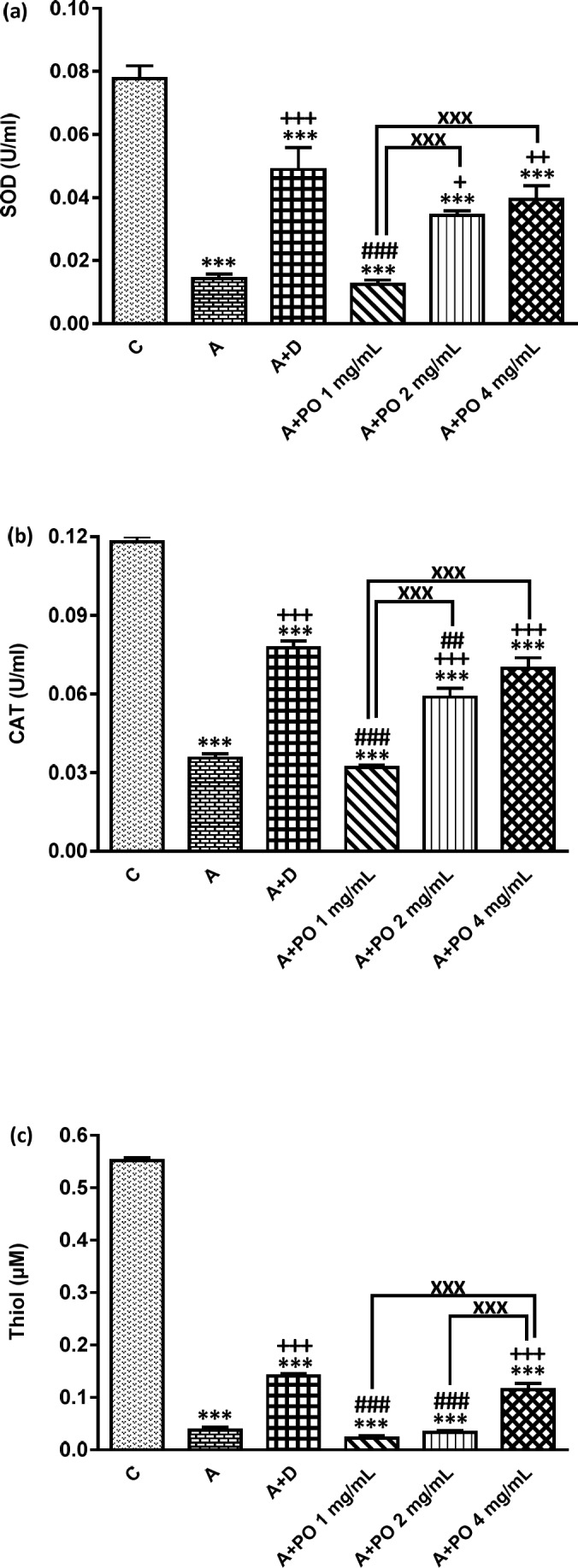
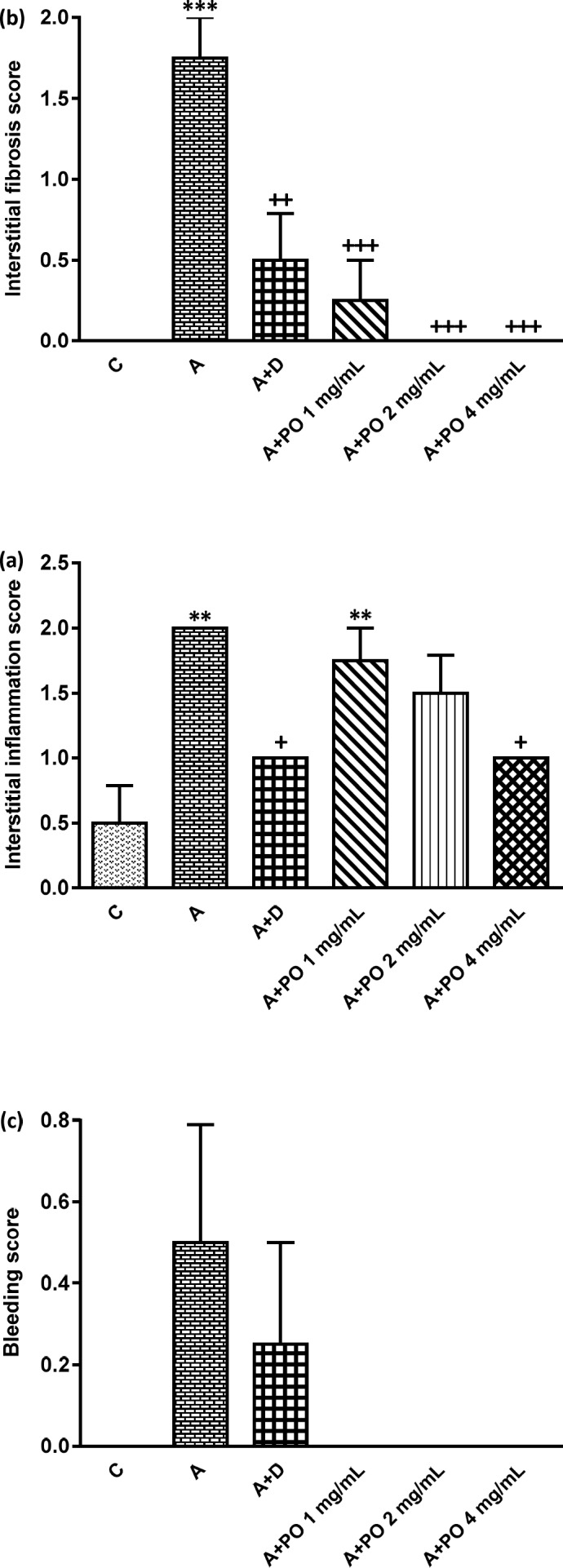
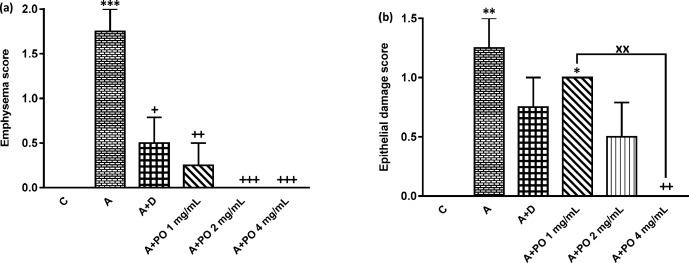
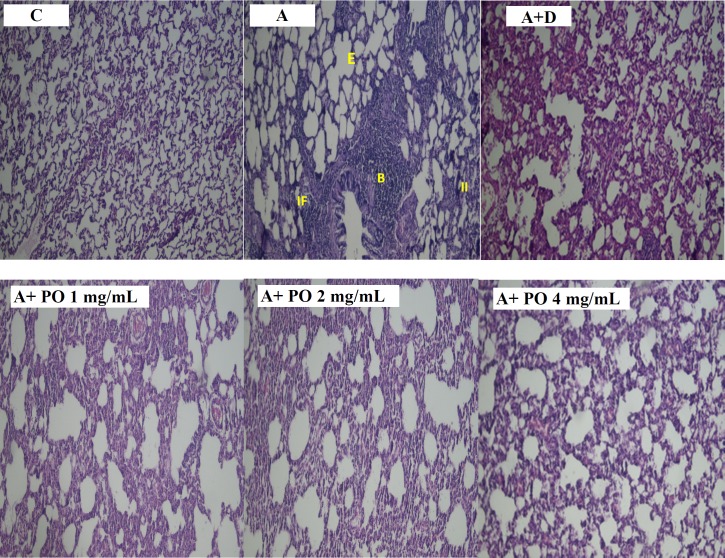
Results
Total and differential WBC count
In asthmatic animals, total WBC count and the percentages of monocytes, eosinophils and neutrophils in the BALF were significantly higher than those of the control group but lymphocyte percentage was lower compared to the control group (p < 0.05 for total WBC and p < 0.001 for other cases; Figures 1 and 2).
Total WBC counts in asthmatic animals treated with all concentrations of P. oleracea as well as the percentages of monocytes, eosinophils, neutrophils and lymphocytes in groups treated with the two higher concentrations of P. oleracea were significantly improved compared to those of untreated asthma group (p < 0.05 to p < 0.001; Figures 1 and 2).
Dexamethasone treatment also significantly reduced the percentages of monocytes, eosinophils and neutrophils but increased lymphocytes percentage compared to asthma group (p < 0.01 to p < 0.001; Figures 1 and 2).
However, the percentage of eosinophils in animals treated with 1 mg/mL of P. oleracea, the percentages of monocytes, neutrophils and lymphocytes in animals treated with the two lower concentrations of P. oleracea as well as total WBC count in groups treated with the two higher concentrations of P. oleracea, were significantly different from those of the control group (p < 0.05 to p < 0.001; Figures 1 and 2).
All differential WBC counts in asthmatic animals treated with dexamethasone were also significantly different from those of the control group (p < 0.05 to p < 0.001; Figures 1 and 2).
Oxidant and antioxidant biomarkers
The BALF levels of NO2, NO3 and MDA were significantly increased while SOD, CAT and thiol levels decreased in asthmatic animals compared to the control group (p < 0.001 for all cases; Figures 3 and 4).
NO2 and NO3 levels in asthmatic animals treated with all concentrations of P. oleracea, SOD and CAT levels in groups treated with the two higher concentrations and MDA and thiol levels in the group treated with the highest concentration of P. oleracea, were significantly improved compared to those of untreated asthma group (p < 0.05 to p < 0.001; Figures 3 and 4).
Dexamethasone treatment also significantly reduced NO2, NO3 and MDA but increased SOD, CAT and thiol levels compared to asthma group (p < 0.001 for all cases; Figures 3 and
4).
SOD, CAT and thiol levels in groups treated with all concentrations of P. oleracea, NO3 and MDA levels in groups treated with the two lower concentrations and NO2 level in the group treated with the lowest concentration, were significantly different from those of the control group (p < 0.01 to p < 0.001; Figures 3 and 4).
The levels of all antioxidant biomarkers in dexamethasone-treated asthmatic animals were also significantly different from those of the control group (p<0.001 for all cases; Figures 3 and 4).
Lung pathological studies
The scores of all pathological changes except for bleeding, in asthma group were significantly increased compared to the control group (p < 0.01 to p < 0.001; Figures 5 and 6).
Interstitial fibrosis and emphysema in asthma groups treated with all concentrations of P. oleracea, and interstitial inflammation and epithelial damage in the group treated with the highest concentration, were significantly reduced compared to untreated asthma group (p < 0.05 to p < 0.001; Figures 5 and 6).
Dexamethasone treatment also significantly reduced all pathological insults except for bleeding and epithelial damage compared to asthma group (p < 0.05 to p < 0.01; Figures 5 and 6).
Interstitial inflammation and epithelial damage scores in group treated with the lowest concentration of P. oleracea were significantly different from those of the control group (p<0.05 to p<0.01; Figures 5 and 6).
Overall, there was no significant difference in all pathological changes between the control and dexamethasone-treated group. A specimen photograph of lung histology in each studied group was presented in Figure 7.
Comparison of the effects of the extract and dexamethasone
The effects of the lowest concentration of P. oleracea on all differential WBC counts were significantly less marked than those of dexamethasone (p < 0.001 for all cases; Figures 1 and 2).
However, the effect of treatment with all concentrations of P. oleracea on total WBC, that of the two higher concentrations of P. oleracea on eosinophils and lymphocytes percentages, as well as the influence of the highest concentration of P. oleracea on monocytes percentage, were significantly higher than those of dexamethasone (p<0.05 to p<0.001; Figures 1 and 2).
The effect of the lowest concentration of P. oleracea on NO2 and SOD levels and its two lower concentrations on NO3, MDA, thiol and CAT levels were significantly less pronounced than those of dexamethasone (p < 0.05 to p < 0.001; Figures 3 and 4). However, the effects of the highest concentration of P. oleracea on NO2 level were significantly more marked than that of dexamethasone (p < 0.05; Figure 3).
There was no significant difference in all pathological scores among dexamethasone-treated group and groups treated with three concentrations of P. oleracea.
Comparison of the effects of different concentrations of the extract
The effects of two higher concentrations of P. oleracea (2 and 4 mg/mL) on monocytes, eosinophils, neutrophils and lymphocytes percentages were significantly more marked than the lowest concentration (1 mg/mL), (p < 0.001 for all cases; Figures 1 and 2). In addition, there was a significant difference between the effects of high and medium concentrations of P. oleracea on monocytes and lymphocytes percentages (p < 0.01 for both cases; Figures 1 and 2).
The effects of two higher concentrations of P. oleracea (2 and 4 mg/mL) on all oxidant and antioxidant biomarkers levels except for the effect of the highest concentration (4 mg/mL) on MDA and thiol levels, were significantly more marked compared to the lowest concentration (1 mg/mL), (p < 0.01 to p < 0.001; Figures 3 and 4). The effect of P. oleracea 4 mg/mL on NO3 and thiol levels was also significantly more marked compared to P. oleracea 2 mg/mL (p < 0.001 for both cases; Figures 3 and 4).
Furthermore, P. oleracea 4 mg/mL had significantly more marked effects on epithelial damage compared to P. oleracea 1 mg/mL (p < 0.01; Figure 4).
Discussion
P. oleracea has been used as an edible plant, and a traditional medicine for alleviating a wide spectrum of diseases such as gastrointestinal problems, respiratory diseases, hepatitis, interstitial cystitis, sleep disorders, fevers and headaches (22, 23). Also, anti-inflammatory and anti-bacterial properties of P. oleracea have been reported (7, 24). This plant has also shown anti-oxidant properties in a model of vitamin A deficiency which was suggested to be due to the presence of active constituents such as oleracein A, oleracein B and oleracein E (25). In an in-vitro study, P. oleracea increased cell survival and reduced IL-6 and TNF-α levels, the latter being known as a key pro-inflammatory mediator (26). Furthermore, in a recent study, oleracone, a novel alkaloid isolated from P. oleracea significantly reduced NO production, in-vitro (27).
In the present study, effects of P. oleracea extract on total and differential WBC count and levels of oxidant (NO2, NO3 and MDA) and antioxidant (CAT, SOD and thiol) biomarkers in BALF as well as lung pathological features were investigated in asthmatic rats.
Based on our results, BALF of asthmatic animals had increased total WBC, eosinophils, neutrophils, monocytes counts but the lymphocytes percentage was reduced compared to non-asthmatic (control) animals. These results confirmed the induction of an experimental model of asthma which was also reported by previous studies (16, 20). Increased numbers of eosinophils, a prominent feature of asthma, have been observed in asthmatic patients and correlate with the severity of asthma which was also seen in the present study (28). It has been found that activated eosinophils play an important role in the inflammatory events involved in allergic asthma and airway hyperresponsiveness (29). Findings of this study showed that treatment of asthmatic animals with the extract of P. oleracea resulted in a significant reduction in total WBC, neutrophils, eosinophils and monocytes counts while it increased lymphocyte percentages. Consistently, a previous study on the effect of the hydro-ethanolic extract of P. oleracea on total and differential WBC count in sensitized rats reported comparable results (9).
In the current experiment, NO3, NO2 and MDA levels as indicators of oxidative stress were significantly increased but SOD, CAT and thiol levels decreased in asthmatic animals compared to the control group. Similarly, previous evidence also indicated an imbalance in oxidant/antioxidant enzymes balance towards oxidative conditions in sensitized animals (9, 20). Oxidative stress plays an important role in asthmatic airway inflammation, and airway responsiveness (30), increased airway inflammation is induced by activation of diverse pro-inflammatory cells including macrophages, neutrophils and eosinophils (31). Treatment with the extract of P. oleracea significantly reduced the levels of NO2, NO3 and MDA while enhanced SOD, CAT and thiol levels compared to the asthmatic group. On the other hand, this condition was reversed in another study using hydro-ethanolic extract of P. oleracea in OVA-sensitized rats (9), which was also observed in the present experiment. However, in our previous study (9), the effects of hydro-ethanolic extract of P. oleracea and linolenic acid on total and differential WBC counts in the blood as well as serum oxidant/antioxidant biomarkers levels in a rat model of asthma were shown which reflected the influence of the plant and it constituent linolenic acid on systemic inflammation and oxidative stress. However, in the present study, total and differential WBC count, as well as oxidant/antioxidant biomarkers levels in BALF of sensitized animals were assessed which reflected inflammatory and oxidant changes in the lung of sensitized animals rather than systemic changes as reported in our previous paper. In addition, the effect of the extract on lung pathological changes was also examined. Therefore, in this study, the effect of the extract of P. oleracea on lung pathological parameters were shown in asthmatic animals.
Our results also showed increased pathological insults including interstitial inflammation, interstitial fibrosis, bleeding, epithelial damage and emphysema, in the lung tissues of asthmatic animals. Previous studies reported the same pathological damages in asthmatic rats (21, 32), which confirms the induction of asthma in sensitized animals. Treatment of asthmatic animals with various concentrations of P. oleracea extract resulted in significant protection against most asthma-related lung pathological damages. A previous study showed that ethanol extract of P. oleracea attenuated alveolar and interstitial edema, neutrophil infiltration, and hemorrhage in lung tissues of a mouse model of hypoxia-induced pulmonary edema (33), which supports the results of the present study.
The effects of the two higher concentrations of the extract on most of the measured parameters were more marked than its lowest concentration. The effect of the highest concentration of P. oleracea extract on some of the measured parameters was also more pronounced than the medium concentration. Overall, we observed dose-dependent protective effects of P. oleracea against inflammation and oxidative state.
Therefore, the results of the present study along with the above-mentioned studies suggest a potential therapeutic effect for P. oleracea against asthma as it decreases lung inflammation and oxidative stress. However, further studies are needed to evaluate the effect of the plant and its constituents in animal models of asthma and asthmatic patients.
Conclusion
Our results indicated the preventive effect of the extract of P. oleracea on total and differential WBC counts, levels of NO2, NO3, MDA, SOD, CAT and thiol in BALF and lung pathological insults in asthmatic rats which introduce this herb as a prophylactic remedy against asthma.
Acknowledgements
References
-
1.
Rashed A, Afifi F, Disi A. Simple evaluation of the wound healing activity of a crude extract of Portulaca oleracea L(growing in Jordan) in Mus musculus JVI-1. J. Ethnopharmacol. 2003;88:131-6. [PubMed ID: 12963132].
-
2.
Uddin MK, Juraimi AS, Hossain MS, Nahar MAU, Ali ME, Rahman M. Purslane weed (Portulaca oleracea): a prospective plant source of nutrition, omega-3 fatty acid, and antioxidant attributes. Sci. World J. 2014;2014:1-6.
-
3.
Malek F, Boskabady M, Borushaki M, Tohidi M. Bronchodilatory effect of Portulaca oleracea in airways of asthmatic patients. J. Ethnopharmacol. 2004;93:57-62. [PubMed ID: 15182905].
-
4.
Boskabady MH, Boroushaki M, Aslani MR. Relaxant effect of Portulaca oleraceae on guinea pig tracheal chains and its possible mechanism (s) of action. Med. Hypotheses. Res. 2004;1:139-47.
-
5.
Dkhil MA, Moniem AEA, Al-Quraishy S, Saleh RA. Antioxidant effect of purslane (Portulaca oleracea) and its mechanism of action. J. Med. Plants Res. 2011;5:1589-93.
-
6.
Boroushaki MT, Boskabady MH, Malek F. Antitussive effect of Portulaca oleracea L in guinea pigs. Iran. J. Pharm. Res. 2010;1:187-90.
-
7.
Boskabady MH, Kaveh M, Shakeri F, Mohammadian Roshan N, Rezaee R. Alpha-linolenic acid ameliorates bronchial asthma features in ovalbumin-sensitized rats. J. Pharm. Pharmacol. 2019;71:1089-99. [PubMed ID: 30993723].
-
8.
Kaveh M, Eidi A, Neamati A, Boskabady MH. Modulation of lung inflammation and immune markers in asthmatic rats treated by Portulaca oleracea. Avicenna J. Phytomed. 2017;1:1-11.
-
9.
Kaveh M, Eidi A, Nemati A, Boskabady MH. The Extract of Portulaca oleracea and its Constituent, Alpha Linolenic Acid Affects Serum Oxidant Levels and Inflammatory Cells in Sensitized Rats. Iran. J. Allergy Asthma Immunol. 2017;16:256-70. [PubMed ID: 28732439].
-
10.
Lee AS, Kim JS, Lee YJ, Kang DG, Lee HS. Anti-TNF-α activity of Portulaca oleracea in vascular endothelial cells. Int. J. Mol. Sci. 2012;13:5628-44. [PubMed ID: 22754320].
-
11.
Ishmael FT. The inflammatory response in the pathogenesis of asthma. J. Am. Osteopath. Assoc. 2011;111:7-11.
-
12.
James AL, Bai TR, Mauad T, Abramson MJ, Dolhnikoff M, McKay KO, Maxwell PS, Elliot JG, Green FH. Airway smooth muscle thickness in asthma is related to severity but not duration of asthma. Eur. Respir. J. 2009;34:1040-5. [PubMed ID: 19282340].
-
13.
Bradley BL, Azzawi M, Jacobson M, Assoufi B, Collins JV, Irani AM, Schwartz LB, Durham SR, Jeffery PK, Kay AB. Eosinophils, T-lymphocytes, mast cells, neutrophils, and macrophages in bronchial biopsy specimens from atopic subjects with asthma: comparison with biopsy specimens from atopic subjects without asthma and normal control subjects and relationship to bronchial hyperresponsiveness. J. Allergy Clin. Immunol. 1991;88:661-74. [PubMed ID: 1918731].
-
14.
Lacoste JY, Bousquet J, Chanez P, Van Vyve T, Simony-Lafontaine J, Lequeu N, Vic P, Enander I, Godard P, Michel FB. Eosinophilic and neutrophilic inflammation in asthma, chronic bronchitis, and chronic obstructive pulmonary disease. J. Allergy Clin. Immunol. 1993;92:537-48. [PubMed ID: 8409114].
-
15.
Luksza A, Jones D. Comparison of whole-blood eosinophil counts in extrinsic asthmatics with acute and chronic asthma. Br. Med. J. Clin Res Ed;(1982) 285:1229-31.
-
16.
Shakeri F, Soukhtanloo M, Boskabady MH. The effect of hydro-ethanolic extract of Curcuma longa rhizome and curcumin on total and differential WBC and serum oxidant, antioxidant biomarkers in rat model of asthma. Iran. J. Basic Med. Sci. 2017;20:155-65. [PubMed ID: 28293392].
-
17.
Barnes PJ. Pathogenesis of asthma: a review. J. R. Soc. Med. 1983;76:580-6. [PubMed ID: 6308253].
-
18.
Barrios RJ, Kheradmand F, Batts LK, Corry DB. Asthma: pathology and pathophysiology. Arch. Pathol. Lab Med. 2006;130:447-51. [PubMed ID: 16594736].
-
19.
Shifren A, Witt C, Christie C, Castro M. Mechanisms of remodeling in asthmatic airways. J. Allergy. 2012;2012:1-12.
-
20.
Shakeri F, Boskabady MH. Anti‐inflammatory, antioxidant, and immunomodulatory effects of curcumin in ovalbumin‐sensitized rat. Biofactors. 2017;42:567-76.
-
21.
Shakeri F, Mohamadian Roshan N, Kaveh M, Eftekhar N, Boskabady MH. Curcumin affects tracheal responsiveness and lung pathology in asthmatic rats. Pharmacol. Rep. 2018;70:981-7.
-
22.
Ibn Sina H. al-Qanun fi al-Tibb. IS aD, editor. 2005;2:417-8.
-
23.
Razi M. Al-Hawi fi›l-tibb (Comprehensive book of medicine). Hyderabad: Osmania Oriental Publications Bureau. 1968;20:548-53.
-
24.
Banerjee G, Mukherjee A. Antibacterial activity of a common weed, Portviaca oleracea L. Geobios. 2003;30:143-4.
-
25.
Arruda SF, Siqueira EM, Souza EM. Malanga (Xanthosoma sagittifolium) and purslane (Portulaca oleracea) leaves reduce oxidative stress in vitamin A-deficient rats. Ann. Nutr Metab. 2004;48:288-95. [PubMed ID: 15452401].
-
26.
Xiao F, Lu F, Xu L. Effect of different parts of Portulace oleracea on the levels of TNF-alpha and IL-6 in the supernatant of cultured adipose cell. Zhongguo Zhong Yao Za Zhi. 2005;30:1763-6. [PubMed ID: 16468375].
-
27.
Meng Y, Ying Z, Xiang Z, Hao D, Zhang W, Zheng Y, Gao Y, Ying X. The anti‐inflammation and pharmacokinetics of a novel alkaloid from Portulaca oleracea L. J Pharm. Pharmacol. 2016;68:397-405. [PubMed ID: 26888212].
-
28.
Khoury P, Grayson PC, Klion AD. Eosinophils in vasculitis: characteristics and roles in pathogenesis. Nat. Rev. Rheumatol. 2014;10:474-83. [PubMed ID: 25003763].
-
29.
Trivedi S, Lloyd C. Eosinophils in the pathogenesis of allergic airways disease. Cell Mol. Life Sci. 2007:64 1269-89.
-
30.
Sackesen C, Ercan H, Dizdar E, Soyer O, Gumus P, Tosun BN, Büyüktuncer Z, Karabulut E, Besler T, Kalayci O. A comprehensive evaluation of the enzymatic and nonenzymatic antioxidant systems in childhood asthma. J. Allergy Clin. Immunol. 2008;122:78-85. [PubMed ID: 18485467].
-
31.
Rahman I, Morrison D, Donaldson K, MacNee W. Systemic oxidative stress in asthma, COPD, and smokers. Am. J. Respir. Crit. Care Med. 1996;154:1055-60. [PubMed ID: 8887607].
-
32.
Shakeri F, Roshan NM, Boskabady MH. Hydro-ethanolic extract of Curcuma longa affects tracheal responsiveness and lung pathology in ovalbumin-sensitized rats. Int. J. Vitam. Nutr. Res. 2019;1:1-10.
-
33.
Yue T, Xiaosa W, Ruirui Q, Wencai S, Hailiang X, Min L. The Effects of Portulaca oleracea on Hypoxia-Induced Pulmonary Edema in Mice. High Alt. Med. Biol. 2015;16:43-51. [PubMed ID: 25761168].