This article has an erratum available at: http://dx.doi.org/10.21037/jss-2022-01 the article has been update on 2022-08-15 at here.
Evaluation of K-wireless robotic and navigation assisted pedicle screw placement in adult degenerative spinal surgery: learning curve and technical notes
Introduction
While pedicle screw constructs are a commonly used surgical implant for spinal internal fixation, associated complication rates can be significant (1,2). There are multiple indications for the use of pedicle screw constructs to including treatment of scoliosis and deformity correction, spondylolisthesis, spinal fractures, and tumor reconstruction (3). Despite the widespread utilization of pedicle screws, significant complications with implant misplacement continue to be reported (2).
Historically, freehand pedicle screw placement without image guidance has been associated with relatively high rates of inaccuracy, and fluoroscopic imaging guidance was introduced to assist in screw insertion (1). The first robotic-assisted system for adult spine surgery received FDA clearance in 2004, which reported an average error of less than 2 mm in 98.3% of implanted pedicle screws (4). Newer robotic systems with integrated surgical navigation have shown significantly better accuracy, faster time per screw placement, less fluoroscopy/radiation time, and shorter hospital stays when compared to free hand technique (5,6).
Despite the advantages to robotic navigated assistance (RNA), there are still disadvantages including additional training, potentially longer operative times, more personnel required in the operating room (OR) (1,7,8). While fluoroscopy has been reported to offer significant improvements, there are risks that remain, such as nerve injury, vascular injury, and radiation exposure to surgical staff.
Understanding the learning curve and the limitations of this new technology is crucial for successful implementation. RNA in the OR can address many concerns associated with freehand technique. To address the growing concerns with adaptation of RNA systems, the purpose of this study was to record and evaluate a single surgeon’s learning curve of k-wireless robotic assisted pedicle screw placement with integrated navigation in adult patients undergoing posterior spine fusion surgery for lumbar degenerative conditions. In doing so, an understanding of screw accuracy, reasons for intraoperative conversion to either K-wire or freehand techniques, radiation exposure, and time expenditure is gained.
We present the following article in accordance with the STROBE reporting checklist (available at http://dx.doi.org/10.21037/jss-20-687).
Methods
A retrospective review of prospectively collected data was conducted on 65 consecutive adult patients who underwent posterior spinal fusion from June 2019 to July 2020. The study consisted of one orthopaedic spine surgeon, who used a RNA system (Mazor X Stealth Edition, Medtronic, Dublin, Ireland) (Figure 1). Ethical approval was obtained Institutional Review Board at the Hospital for Special Surgery (IRB#2019-1402). The participants did not need to give informed consent in addition to routine informed consent procedures. The study was conducted in accordance with the Declaration of Helsinki (as revised in 2013).
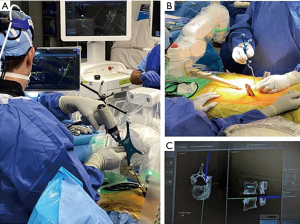
There was no funding allocated specifically for this study.
Computed tomography (CT) scans were performed pre-operatively, then loaded into the robotic planning software. Planning was performed pre-operatively to determine optimal screw positioning into the pedicles (Figure 2). All patients underwent posterior approach through either midline skin and fascial incisions, separate Wiltse skin and fascial incisions, or midline skin and separate fascial Wiltse incisions. Following pedicle screw placement, all patients underwent an intraoperative scan with a three-dimensional mobile C-arm (Ziehm imaging GmBH, Nuremberg, Germany). Screw position was checked intraoperatively on each 3D fluoroscopic imaging by the attending surgeon.
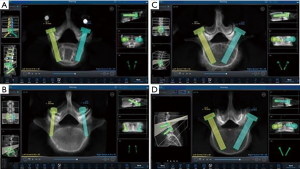
Patient demographics including age, sex, diagnosis, weight, height, and body mass index (BMI) were recorded. Collected data was used to evaluate the surgeon’s learning curve. Cases in which the robotic system was converted intraoperatively and conversion to either Kirschner wire (K-wire) (KW) or freehand (FH) techniques were recorded and studied. Additionally, radiation exposure, accuracy and trajectory of screw placement to the planned pedicle was recorded.
During each procedure, registration time was recorded when the robotic mount was attached to the reference posterior superior iliac spine (PSIS) or spinous process reference clamp, and ended when confirmation of fluoroscopic imaging colocalized with the software planning template. During this step, all required surgical instrumentation was registered and verified. Registration was usually performed once, however, in cases with longer constructs an additional registration was required if all planned operative motion segments were not completely visualized in a single fluoroscopic frame.
Screw insertion time was defined to begin when the robotic arm was positioned at a single planned pedicle and to end when the robotic arm was retracted following screw insertion. Following each procedure, the robotic software log was obtained by research staff and all commands and time intervals were reviewed. Procedure time was recorded from the hospital electronic medical record and measured from skin incision to end of closure. Lastly, the time between the first and last screw placed was recorded.
Imaging and radiation
Procedure radiation dosage (mGy) and exposure time (minutes) were captured at two time points: C-arm images were taken intraoperatively before and after the procedure, as well as 3d fluoroscopic imaging, which was taken once intraoperatively after the procedure either before or after the second C-arm image was taken. This was done in order to assess and confirm screw positioning as well. Note that, due to the multiple time points of radiographic images taken the patient and surgical staff were more likely exposed to radiation. Post-operative 3D fluoroscopic imaging was evaluated using both the axial and sagittal planes. Axial planes were measured to assess screw placement in the medial-lateral plane, meanwhile the sagittal plane was utilized to determine cranial-caudal placement. Deviation from the pre-operative plan was then determined by measuring the difference (mm) in both the axial and sagittal plane. Some patients underwent a combined anterior and posterior approach, or a combined lateral and posterior approach.
After each procedure, patients are scheduled for a routine standard of care post-operative appointment with the attending spine surgeon around 2 weeks, 8 weeks, 6 months and a year from surgery. During these visits, patients will underwent routine X-rays and CT scans to make sure hardware is intact and if there is no lucency of screws.
Pedicle screw evaluation
Screw placements with pre-operatively planned trajectories were measured and compared to 3d fluoroscopic imaging. Distances from pre-operative planning template were measured on a planning template via Mazor software, specifically the anterorposterior (AP) and lateral (LT) planes. Pedicle screw accuracy was classified as described by Gertzbein and Robbins system (GRS) – screws were classified and categorized into four different groups based on a clinical positioning grade: category A, fully contained within pedicle; category B, breach <2 mm; category C, breach between 2–4 mm; category D, breach >4 mm (Table 1) (3,9). Critical breaches were defined as being greater than 2 mm. If breaches were detected, the direction was recorded, as evidence of inaccuracy and trajectory issues with RNA.
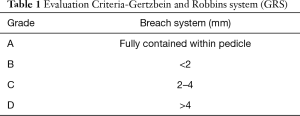
Full table
In addition to critical breach analysis, screw trajectory for all screws was assessed. Planning for each case was performed via preoperative planning to see orientation of pedicle screw (Figure 2A). Between cortical trajectories were defined as positioned within the mid axis of the screw contained entirely within the pedicle. This is due to anatomical landmarks and different morphologies of pedicle, such as narrowing of the pedicle. Cortical screw trajectory was defined as a screw with pars-pedicular fixation in a medial to lateral and caudal to cranial orientation (Figure 2B). As seen in Figure 2C, screws that were not at the cranial instrumented level may have had a slightly more medial starting point to avoid soft tissue pressure on the robotic cannula. Figure 2D shows pedicle screw trajectory in routine fashion in a patient with spinal instability due to isthmic spondylolisthesis.
Clinical outcome evaluation
During each scheduled post-operative visit, including their preoperative visit, patients fill out their patient-reported outcome measures (PROMs), which are questionnaires that will assess patient’s health and quality of life. PROMs include Modified Oswestry Low Back Disability Questionaire (ODI), Scoliosis Research Society (SRS-22r), and the Numeric Rating Scale (NRS) for back and leg pain.
Conversion from robotic to KW or FH techniques
Reasons and potential risk factors for screw mal-positioning or concern about robotic-navigation screw placement were recorded, and cases where conversion from RNA occurred were recorded. These included: medialization pressure of soft tissue on the cannula, hypoplastic pedicles, suboptimal morphology of the screw starting point with high skive potential, spinal instability, trajectory interference with reference pin placement, and mechanical robotic arm interference.
Statistical analysis
Mean values and standard deviations for all parameters were calculated. Significant findings were found through statistical probability (P values) for screws implanted using RNA. A learning curve was created by plotting robotically placed screws, time per screw, average screw time per case, mean cumulative registration time per case, and incidence/reason for skiving off trajectory. A two-tail t-test was performed on all levels that had the same trajectory as preoperative plan, breaches per level, converted screws per level, and for clinical outcome evalutaions. Additionally, trajectories that differed from preoperative plan, average measurement of screw trajectories, trajectories that were the same as preoperative plan and screws without ziehm imaging were recorded. Statistical significance was defined as P value less than 0.05.
Results
Sixty-five consecutive adult patients with a mean age of 59.7±13.4 years underwent posterior spine fusion surgery for lumbar degenerative conditions using RNA over a 13-month period. Baseline characteristics such as patient demographics, procedure information, radiology data, and learning curve data are listed in Table 2.
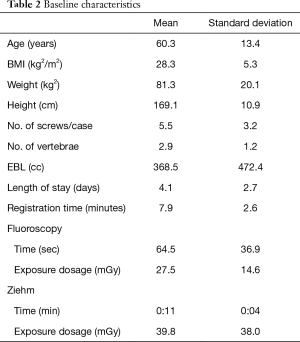
Full table
Screw placement and learning curve
A total of 364 instrumented pedicles in 65 adult patients (59.7±13.4 years) were planned robotically, 311 (85.4%) of which were placed robotically. Seventeen screws (4.7%) were converted to k-wire, 21 (5.8%) converted to freehand, and 15 (4.1%) were planned freehand. Reasons for conversion included morphology of starting point causing skive (n=18), soft tissue pressure on the drill guide causing displacement from the pre-operative plan (n=9), hypoplastic pedicles causing skive (n=6), obstructive reference pin placement (n=2), and mechanical robotic arm issues (n=1).
All left side of surgeries and 92.9% of all right sided surgeries were performed by the attending surgeon. Surgical assistants (physician assistants, residents or fellows) performed the remaining right sided surgeries, and no significance was found between attending surgeon and surgical assistant (P=0.095).
Time per screw, mean time per screw for each case (Figure 3) and average registration time per case significantly decreased with surgeon experience (Figure 4). Registration per case was 7.4±2.4 minutes. A learning curve for registration of the RNA by the surgical team was demonstrated (Figure 4). Compared to the first 10 cases, the mean cumulative registration time improved in the last 10 cases (9.1±2.0 vs. 6.1±2.5 minutes, P=0.006).
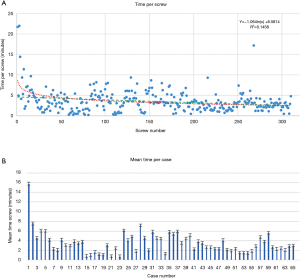
Screw position analysis
Of the 311 robotically placed pedicle screws, three dimensional fluoroscopic imaging showed 291 (93.5%) to be GRS Grade A in the axial plane (fully contained within the pedicle) and 281 (90.4%) were GRS Grade A in the sagittal plane. 3D fluoroscopic imaging screw positioning was used to confirm mean deviation from preoperative plan in all planes were within 1.0±0.7 mm. Screw accuracy was evaluated by a research assistant and resident with oversight from the attending spine surgeon. Screw accuracy was determined to be significant between the first to last few cases, indicating screws were placed more accurately over time with the RNA series. The average displacement from the preoperative plan decreased within the axial and sagittal planes as cases proceeded. Within the axial plane, the first ten cases had an average displacement of 0.8±0.7 mm from preoperative plan, which was not significantly less than when compared to the last ten cases that reported an average of 1.0±0.8 mm. In the sagittal plane when comparing the first and last ten cases, average screw accuracy changed from 0.7±0.5 to 0.9±0.6 mm difference from the preoperative plan and was not statistically significant.
The directionality of deviation of screw position from preoperative plan was determined to be medial in 143 (46.0%) screws with average displacement of 1.2±0.9 mm and 170 (54.0%) screws were placed lateral with average displacement of 1.2±1.0 mm. In the sagittal plane, 117 (37.9%) screws were placed cranial to plan with an average difference of 0.6±0.5 mm, and 193 (62.1%) screws were placed caudal to plan with an average difference of 1.0±0.6 mm. A total of 10 screws axially (3.2%) and 13 (4.2%) screws sagittal had no measurable difference in screw placement between the plan and postoperative 3D fluoroscopic images (Table 3). Additionally, 10 (3.2%) screws had missing axial images, and 17 (5.5%) screws had missing sagittal images (Table 3). There were no adverse clinical or intraoperative sequelae related to pedicle screw placement.
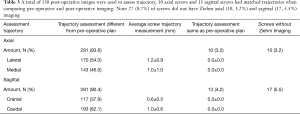
Full table
Breach
A total of 17 (5.5%) critical breaches were identified intraoperatively in 11 out of 65 patients (16.9%).Nine (2.9%) of the critical breaches due to soft tissue pressure onto drill guide from midline incisions, causing displacement from the planned trajectory and resulted in skive. All breaches were identified during surgery and all screws were repositioned by either FH or KW technique. All patients were asymptomatic after surgery and zero patients were taken back for revision surgery in this cohort. The critical breaches that were found and were reported as lateral breaches. As intraoperative breaching was found, the attending surgeon would use intraoperative C-arm and intraoperative 3D fluoroscopy. When breaching was confirmed through intraoperative imaging, the surgeon corrected breaching intraoperatively. The surgeon would remove the RNA placed screw and re-position the screw by either FH or KW technique. After the screw was placed, another 3D fluoroscopy image was taken to confirm satisfactory position (GRS Grade A).
An additional two patients exhibited a total of 6 (1.9%) critical breaches (>2−4 mm) due to hypoplastic pedicles. There was a total of 3 (0.9%) screws which were unplanned lateral breaches, in one patient. Due to morphology and spinal instability from isthmic spondylolisthesis skive occurred with breach of one screw (0.3%) in one patient. All screws were identifies on 3D fluoroscopy during surgery and re-positioned.
Clinical outcome evaluation
Clinical outcomes were recorded during each preoperative and postoperative visit, where patients filled out their patient-reported outcome measures (PROMs): Modified Oswestry Low Back Disability Questionaire (ODI), Scoliosis Research Society (SRS-22r), and the Numeric Rating Scale (NRS) for back pain and leg pain.
All postoperative visit scores were compared to final preoperative visit scores, and on average scores decreased throughout each visit. ODI scores decreased by 27.8% form 41.3±16.9 (P=0.005), NRS leg pain decreased by 39.1% from 6.4±2.7 to 2.6±2.5 (P=0.044) and NRS back pain scores decreased by 70.4% from 7.1±2.4 to 5.0±2.7 (P=0.049). SRS-22r on average did show a decrease of scores over time and did not show statistical significance.
Screws converted to other technique
Of the 311 instrumented pedicles that were planned robotically (85.4%), the reasons for an exclusion were based on surgeon decision, robotic malfunction before surgical case, and a cervical case done robotically with 10 (2.9%) screws. Cervical case was templated robotically and ultimately instrumented freehand after visualization of the proposed robotic trajectory as a proof of concept. From the 311 planned instrumented pedicles, a total of 36 (11.6%) of screws were determined intraoperatively to be unsuitable for RNA technique and were therefore converted to either KW technique (n=17) or FH technique (n=21).
The most common reason for conversion of RNA to another technique was the morphology of the pedicle screw starting point resulting in risk of intra-operative skive which was determined to be the case in 18 of 36 (50.0%) screws (Table 4). A profile of RNA conversions to FH or KW was recorded (Table 5). Intraoperative 3-D fluoroscopic imaging identified breached screws, which required the attending surgeon to convert to freehand.
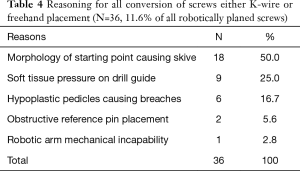
Full table
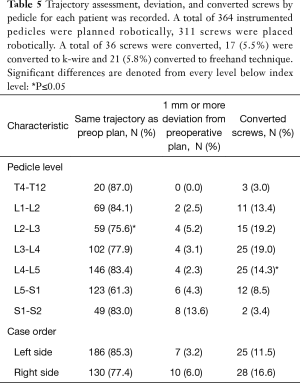
Full table
Screws placed within the same trajectory as the preoperative plan were found to statistically significant were between L1−L2 and L2−L3 (84.1% and 75.6%, P=0.004). Additionally, significance was found within converted screws between L3−L4 and L4−L5 (19.0% vs. 14.3%, P=0.04) (Table 5). Lastly, there was no significant difference between case orders between left-sided matched trajectories, deviation from preoperative plan, or converted screws over the right side of cases (Table 5).
Discussion
The learning curve for RNA demonstrated improvements within the first several cases. Previous studies concluded that there was almost no learning curve and no significant difference between screw placement accuracy and time per screw between first cases and more experience cases (9). Our data suggests that improvements were made throughout the learning curve in time per screw and accuracy of screw placement (avoiding skive). Throughout this study, statistically significant improvements were found in pedicle screws that had the same screw trajectory and position as the preoperative plan. In addition to that, registration time between the first to last ten cases and speed of workflow was found to be significant as the study proceeded. This improved efficiency was likely due to improved surgeon and OR staff familiarity with equipment and workflow.
Studies have compared manual versus robotic assistance in other fields of surgery such as knee and hip, which reported robotic assistance have improved outcomes (10). Total hip arthroplasty (THA) reported a decrease in time for instrumentation set up, bone registration, and was not associated with increased operative time (10). When comparing robotic THA’s learning curve to our learning curve, 12 out of 50 hip surgery cases were in the learning phase, meanwhile, our findings show 14 out of 65 cases were within this phase (10). Furthermore, knee arthroplasty has reported stacked error results and implant position differences between Manual Total Knee Arthroplasty (MTKA) to compare to Robotic Arm Total Knee Arthroplasty (RATKA). Overall, MTKA positional error was greater than RATKA and accuracy to plan improved with RATK (11).
When comparing RNA to FH pedicle screw placement in spine surgery, studies have reported FH screw misplacements that have involved implants impinging or deforming the walls of major vascular structures, such as the aorta, common iliac vein, and internal iliac vein. The aorta is the highest risk of surgery in during surgery utilizing FH technique in the thoracic and thoracolumbar spine (12). Additionally, FH misplacement rates range from 3−55% in the thoracic spine and 5−41% in the lumbar spine (12). Freehand studies reported a 12.1% breach rate, 1.1% revision rate, and a 2.3% rate of neurological complications (13,14). When comparing these results, our data show a lower breach rate of 5.5% all of which were without adverse clinical sequelae. No patients in the study group were taken back to the OR for robotic screw malposition and no neurological complications were observed. Furthermore, previous studies have reported FH placed screws tend to perforate the cortex medially, whereas screws planned with CT and navigation perforate more often laterally (15). A correlation between medially malpositioned screws and neurological complications have been proposed, as lateral cortex violation is likely less related to neurological complications (15).
Understanding the potential complications before implementation of RNA during surgery provides multiple learning points. Prior studies have reported the detrimental effects that intraoperative motion of the spine has on robotic systems because navigation accuracy cannot be maintained (16). Motion of the robotic arm due to soft tissue pressure on the drill guide can impact screw trajectory/displacement from the preoperative plan. This may result in skive with associated increased surgical time and the possible need for conversion. Additionally, spinal instability due to pathology such as isthmic spondylolisthesis might result in motion at the docking point of the surgical cannula and increase the risk of skive and motion within the robotic apparatus. This was encountered in our series and required conversion to KW in 2 (0.6%) of all screws.
Open midline skin incisions that reported soft tissue pressure on to the cannula and drill guide, made up 4.5% of critical breaches, 0.9% of lateral breaching, and 25% of all converted screws from RNA. One method for avoiding this issue is by maintaining sufficient soft tissue retraction to allow proper seating of the cannula onto the pedicle without undesired pressure (17,18). In our study, screw removal and FH re-instrumentation was reported in 1.9% of all robotically placed screws. This was determined to be necessary after 3D fluoroscopic imaging was taken following the initial instrumentation. Furthermore, trajectory changes made intra-operatively that differed from the starting point were reported in 10.9% robotically placed screws and second drill passes were done in 0.6% of all robotically placed screws (Table 6). As such, the RNA technique may be best combined with an advanced intraoperative imaging modality such as 3d fluoroscopy or intraoperative CT to allow precise post-instrumentation confirmation scan before surgical closure.
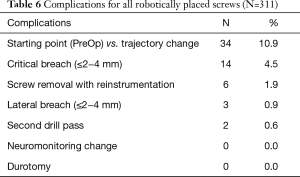
Full table
In our series, while the surgeon was placing the pedicle screw, haptic feedback is received through the drill-guide, tap, and screw insertion steps of the procedure and combined with real-time visual feedback from the surgical navigation monitor. Either the direct haptic feedback or the navigation monitor may alert the surgical team to a potential issue with screw positioning. Loss of accuracy may occur due to motion within the robotic system by soft tissue pressure, bumping of the robotic arm which may require a second registration procedure, which occurred in 2.6% of all cases. Additionally, trying to correct a suboptimal trajectory through the robotic arm guide may result in pressure on the arm which can trigger encoders inside of the robotic arm to automatically shift the robotic system. This mechanism is designed to alert the surgical team to a loss of accuracy and such that a re-registration can be performed.
The majority of screws were placed uneventfully in 94.5% of robotically planned screws without any breach or significant deviation from the preoperative plan. In addition, it was found that the rate of the screws converted to manual placement decreased after the first 30 patients. This result is consistent with some previous reports (19). Pechlivanis et al. evaluated the accuracy of percutaneous pedicle screw placement in the lumbar spine using this same robotic system. As analyzed by postoperative CT scans, of 133 total pedicle screws placed, 91.7% screws were placed exactly within the pedicle (20).
Screw conversion was due to skiving in 18 of 36 (50%) of cases that were converted to FH or KW technique and 17 of 311 screws (5.5%) of all robotically planned screws (Figure 5). Skive can result from an extreme angle of the bone surface at starting point to the planned screw trajectory, unfavorable morphology of the bone surface with large osteophytes or irregularities, or a planned trajectory down the long-axis of a cortical surface such as a hypoplastic pedicle. Therefore, skiving off of the desired trajectory may be minimized by thoughtful pre-operative planning, and using a high-speed burr to flatten the starting point surface to a more favorable morphology, and firmly docking the inner drill guide cannula with an anti-skive pin (21,22). This was seen in our study as skiving occurred less towards the end of the learning curve as surgeon’s gained experience. The first half of our cases reported eight series with incidence of skive, 16 out of 49 (32.7%) screws reporting skive. The second half of our series reported three cases, 4 out of 20 (20%) screws reporting skive.
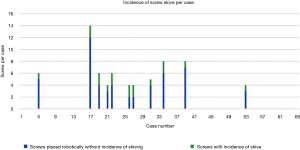
A systematic review summarized the most common causes of robotic failure from 12 studies—including registration failure, soft tissue hindrance, and drill guide skiving (10,23). Half of our converted screws were due to skive of the drill guide. To prevent skiving, a burr was utilized to create a landing spot for the drill guide to enable proper seating onto the pedicle. The anti-skive pin position can be utilized to dock the inner cannula and can be checked by direct visualization on the navigation monitor by positioning the drill onto the starting point before drilling.
Literature reviews have reported 22 studies utilizing GRS and accuracy ranged from 85−100% with RNA (23). The physical connection between the patient and the robot must be as rigid as possible to prevent any additional movements to ensure accuracy of screw trajectory. This relies on appropriate bone density at the site of the reference pin in the PSIS and that the pin is not levered on or moved during the process of attachment to the robotic apparatus. Prior studies reported reduced screw accuracy due to instability with the shanz pin, reference pin, spinous process clamp, and skiving of the drill on bony surfaces with RNA (17,24). During the setup process of robotic instrumentation, components must be properly tightened to reduce motion of the robotic arm base during pedicle screw placement. If these precautions are not taken, mal-positioning of the robotic arm may result.
Reoperation rates secondary to pedicle screw misplacement were estimated from nationally available data (23). Schröder et al. reported a study with 94 patients, none of the pedicle screws required intraoperative repositioning, and conversion to an open procedure was never required (18). There were no implant-related revisions or complications, which were assessed clinically rather than on postoperative radiological studies, to avoid excessive radiation exposure (18). Kantelhardt et al. reported the rate of revision surgery for screw malposition was significantly higher in the freehand pool than in the robotic guided pool. However, the navigated technique tended toward more revisions in which many of the malpositioned screws were detected intraoperatively and immediately revised (5). Therefore, no significant difference was observed in postoperative revision rates between the navigated group and the robotic guided group (5). Similarly, to Schröder and Kantelhardt’s intraoperative pedicle screw positioning, our study detected malpositioned screws intraoperatively and immediately revised and confirmed by 3D fluoroscopy.
In addition to that, Schröder et al. reported average skin-to-skin time of surgery to be 161±50 minutes (2.6±0.8 hours) (18). Bai et al. compared a learning curve between two surgeons for computer-assisted navigation (CAN) group and conventional intraoperative image intensifier (CIII) group and only considered the cases after 6 months of computer-assisted navigation (CAN) was implemented. There was a significant (P=0.026) decrease in operative time for the CAN group of surgeon A, 106.9 versus 121.3 minutes for the conventional intraoperative image intensifier (CIII group), averaging 14.4 minutes less per case in the CAN group (25). A more significant (P=0.003) decrease in operative time for the CAN group was seen with surgeon B’s cohort; 158.0 for CAN patients versus 189.8 minutes (25). In our study, we recorded the total of 65 surgical cases skin to skin surgery time to be 306±42 min (5.1±0.7 hours). Surgical cases compromised of 16 (24.6%) done via the anterior-posterior approach, 27 (41.5%) with the lateral-posterior approach, and 22 (33.8%) with only a posterior approach. RNA skin start time and skin closing were recorded in all cases, with an average time of 200±6 min (2.1±0.1 hours), which is a 65.4% decrease in total time (P=0.04).
The possibility also exists that the robotic arm may not be able to reach the planned trajectory and can result in conversion to FH or KW. For example, the placement of a reference pin in the PSIS or the iliac crest itself may interfere with the ipsilateral L5 or S1 screw trajectory. Obstructive reference pin placement with a planned trajectory can lead to prolonged surgical time and inability to place screws with RNA. Additionally, certain robotic arm movements can prevent the planned placement of screws, as it occurred in 0.3% of all RNA screws, and obstructive reference pin placement occurred in 0.6% of all robotically placed screws. Planned trajectories could not be reached at the intended pedicle, consequently conversion from RNA was necessary.
Transcortical screws were planned for hypoplastic pedicles to keep screws within the pedicle and on the path of the cortex. Hypoplastic pedicles often deviated from the intended trajectory when a screw was planned down the long axis of the pedicle cortex. An “in-out-in” trajectory may be planned or performed in this setting to avoid skive (21). All cases recorded in the learning curve compared pre-operative planning and post-operative screw positioning. Techniques were developed to adapt to hypoplastic pedicles later in our series, by using different techniques.
This study has several limitations, one limitation is that surgeries were performed by a single fellowship-trained attending spine surgeon, who has 10+ years in practice and recorded a learning curve experience. The attending spine surgeon performed all the left side of surgeries, and the majority of the right side of surgeries were performed by surgical assistants, such as residents, fellows, physician assistants, under direct supervision and guidance of the attending surgeon. We did not find any difference in time or accuracy between the attending surgeon and surgical assistant. Studies have found that the usability of robotics during surgery with trainees in practice has a relatively quick learning curve (26). Additionally, as surgical technologists became familiar with the robotic setup and instrumentation, there were improvements with workflow and surgical time. In our series, 29.8% of all cases had a surgical technologist without previous intraoperative robotic experience, which may have added additional time to the procedures. Lack of a control group and was therefore compared to published historical controls. However, the data reported here is important to identify risk factors for untoward events such as screw breach, skive, technical issues with the software, and re-registration such that these events can be avoided and surgical delays may be avoided.
This study involved the co-localization of preoperative CT with intraoperative fluoroscopic imaging to navigation software. Screws were removed and re-instrumented after 3d fluoroscopic analysis intraoperatively in 1.9% of RNA screws. Factors that increased surgical time included re-registration due to pressure on robotic arm, motion within the robotic mount to patient connection, and cases earlier in the learning curve. One major barrier to adoption of RNA is surgeon concern about added time to the procedure. The data presented here suggests that even in the earlier cases in the series, the additional time expenditure for registration (9.1±2.0 minutes) and instrumentation was nominal. Risk factors for suboptimal screw position by RNA included spinal instability (isthmic spondylolisthesis), suboptimal morphology of the screw starting point, and hypoplastic pedicles. Later in the series, these risk factors were recognized on pre-operative templating, accounted for, and avoided.
Conclusions
A learning curve for robotic k-wireless screw placement showed improvement in screw times within the first several cases. Risk factors for suboptimal robotic screw positioning or necessity for conversion to non-robotic technique included soft tissue pressure on the drill guide impacting planned trajectory, hypoplastic pedicles, suboptimal morphology of the screw starting point, and spinal instability. Understanding the learning curve and situations where the robotic technique may be suboptimal can help guide the surgeon safe and effectively for adoption, as well as further refine these technologies.
Acknowledgments
Funding: None.
Footnote
Reporting Checklist: The authors have completed the STROBE reporting checklist. Available at http://dx.doi.org/10.21037/jss-20-687
Data Sharing Statement: Available at http://dx.doi.org/10.21037/jss-20-687
Peer Review File: Available at http://dx.doi.org/10.21037/jss-20-687
Conflicts of Interest: All authors have completed the ICMJE uniform disclosure form (available at http://dx.doi.org/10.21037/jss-20-687). DRL reports research support from Medtronic, during the conduct of the study; royalties from K2M- Stryker, royalties from NuVasive, ownership interest from Woven Orthopedic Technologies, ownership interest from Vestia Ventures MiRus Investment LLC, investment interest from ISPH II, LLC, outside the submitted work. RFW reports personal fees from Medtronic, outside the submitted work, and fee for Service Consultant to OrthoPediatric Company Spine Division. RFM is on the editorial board Spine Deformity Journal, editorial board of The Journal of Children’s Orthopaedics, and a consultant reviewer for the Journal of Pediatric Orthopaedics. The other authors have no conflicts of interests to declare.
Ethical Statement: The authors are accountable for all aspects of the work in ensuring that questions related to the accuracy or integrity of any part of the work are appropriately investigated and resolved. Ethical approval was obtained Institutional Review Board at the Hospital for Special Surgery (IRB#2019-1402). The participants did not need to give informed consent before taking part as this was a retrospective review of prospectively collected data. The study was conducted in accordance with the Declaration of Helsinki (as revised in 2013).
Open Access Statement: This is an Open Access article distributed in accordance with the Creative Commons Attribution-NonCommercial-NoDerivs 4.0 International License (CC BY-NC-ND 4.0), which permits the non-commercial replication and distribution of the article with the strict proviso that no changes or edits are made and the original work is properly cited (including links to both the formal publication through the relevant DOI and the license). See: https://creativecommons.org/licenses/by-nc-nd/4.0/.
References
- D'souza M, Gendreau J, Feng A, et al. Robotic-Assisted Spine Surgery: History, Efficacy, Cost, And Future Trends. Robot Surg 2019;6:9-23. [PubMed]
- Jutte PC, Castelein RM. Complications of pedicle screws in lumbar and lumbosacral fusions in 105 consecutive primary operations. Eur Spine J 2002;11:594-8. [Crossref] [PubMed]
- Lieberman IH, Hardenbrook MA, Wang JC, et al. Assessment of Pedicle Screw Placement Accuracy, Procedure Time, and Radiation Exposure Using a Miniature Robotic Guidance System. J Spinal Disord Tech 2012;25:241-8. [Crossref] [PubMed]
- Devito DP, Kaplan L, Dietl R, et al. Clinical Acceptance and Accuracy Assessment of Spinal Implants Guided With SpineAssist Surgical Robot. Spine 2010;35:2109-15. [Crossref] [PubMed]
- Kantelhardt SR, Martinez R, Baerwinkel S, et al. Perioperative course and accuracy of screw positioning in conventional, open robotic-guided and percutaneous robotic-guided, pedicle screw placement. Eur Spine J 2011;20:860-8. [Crossref] [PubMed]
- Khan A, Meyers JE, Siasios I, et al. Next-Generation Robotic Spine Surgery: First Report on Feasibility, Safety, and Learning Curve. Oper Neurosurg (Hagerstown) 2019;17:61-9. [Crossref] [PubMed]
- Staub BN, Sadrameli SS. The use of robotics in minimally invasive spine surgery. J Spine Surg 2019;5:S31-40. [Crossref] [PubMed]
- Yu L, Chen X, Margalit A, Peng H, et al. Robot-assisted vs freehand pedicle screw fixation in spine surgery - a systematic review and a meta-analysis of comparative studies. Int J Med Robot 2018;14:e1892 [Crossref] [PubMed]
- Kam JKT, Gan C, Dimou S, et al. Learning Curve for Robot-Assisted Percutaneous Pedicle Screw Placement in Thoracolumbar Surgery. Asian Spine J 2019;13:920-7. [Crossref] [PubMed]
- Perdomo-Pantoja A, Ishida W, Zygourakis C, et al. Accuracy of Current Techniques for Placement of Pedicle Screws in the Spine: A Comprehensive Systematic Review and Meta-Analysis of 51,161 Screws. World Neurosurg 2019;126:664-678.e3. [Crossref] [PubMed]
- Hampp EL, Chughtai M, Scholl LY, et al. Robotic-Arm Assisted Total Knee Arthroplasty Demonstrated Greater Accuracy to Plan Compared to Manual Technique. J Knee Surg 2019;32:239-50. [Crossref] [PubMed]
- Parker SL, Amin AG, Santiago-Dieppa D, et al. Incidence and Clinical Significance of Vascular Encroachment Resulting From Freehand Placement of Pedicle Screws in the Thoracic and Lumbar Spine. Spine (Phila Pa 1976) 2014;39:683-7. [Crossref] [PubMed]
- Mason A, Paulsen R, Babuska JM, et al. The accuracy of pedicle screw placement using intraoperative image guidance systems. J Neurosurg Spine 2014;20:196-203. [Crossref] [PubMed]
- Verma R, Krishan S, Haendlmayer K, et al. Functional outcome of computer-assisted spinal pedicle screw placement: a systematic review and meta-analysis of 23 studies including 5,992 pedicle screws. Eur Spine J 2010;19:370-5. [Crossref] [PubMed]
- Gelalis ID, Paschos NK, Pakos EE, et al. Accuracy of pedicle screw placement: a systematic review of prospective in vivo studies comparing free hand, fluoroscopy guidance and navigation techniques. Eur Spine J 2012;21:247-55. [Crossref] [PubMed]
- Rahmathulla G, Nottmeier EW, Pirris SM, et al. Intraoperative image-guided spinal navigation: technical pitfalls and their avoidance. Neurosurg Focus 2014;36:E3 [Crossref] [PubMed]
- Ahmed AK, Zygourakis CC, Kalb S, et al. First spine surgery utilizing real-time image-guided robotic assistance. Comput Assist Surg (Abingdon) 2019;24:13-7. [Crossref] [PubMed]
- Schröder M, Staartjes V. Revisions for screw malposition and clinical outcomes after robot-guided lumbar fusion for spondylolisthesis. Neurosurgical Focus 2017;42:E12 [Crossref] [PubMed]
- Hu X, Lieberman IH. What is the learning curve for robotic-assisted pedicle screw placement in spine surgery? Clin Orthop Relat Res 2014;472:1839-44. [Crossref] [PubMed]
- Pechlivanis I, Kiriyanthan G, Engelhardt M, et al. K. Percutaneous placement of pedicle screws in the lumbar spine using a bone mounted miniature robotic system: first experiences and accuracy of screw placement. Spine (Phila Pa 1976) 2009;34:392-8. [Crossref] [PubMed]
- Tsai TH, Tzou RD, Su YF, et al. Pedicle screw placement accuracy of bone-mounted miniature robot system. Medicine (Baltimore) 2017;96:e5835 [Crossref] [PubMed]
- Hu X, Ohnmeiss DD, Lieberman IH, et al. Robotic-assisted pedicle screw placement: lessons learned from the first 102 patients. Eur Spine J 2013;22:661-6. [Crossref] [PubMed]
- Joseph JR, Smith BW, Liu X, et al. Current applications of robotics in spine surgery: a systematic review of the literature. Neurosurg Focus 2017;42:E2 [Crossref] [PubMed]
- Overley SC, Cho SK, Mehta AI, et al. Navigation and Robotics in Spinal Surgery: Where Are We Now? Neurosurgery 2017;80:S86-99. [Crossref] [PubMed]
- Bai Y, Zhnag Y, Chen Z, et al. Learning curve of computer-assisted navigation system in spine surgery. Chinese Medical Journal 2010;123:2989-94. [PubMed]
- Xiang L, Zhou Y, Wang H, et al. Significance of Preoperative Planning Simulator for Junior Surgeons’ Training of Pedicle Screw Insertion. J Spinal Disord Tech 2015;28:E25-9. [Crossref] [PubMed]