T1 mapping and feature tracking imaging of left ventricular extracellular remodeling in severe aortic stenosis
Introduction
Aortic stenosis (AS) is the most common valvular disease, with a growing prevalence in the aging population (1). In response to the narrowed valve, left ventricular (LV) hypertrophy is initially adaptive to restore wall stress and cardiac function. Ultimately, LV decompensation occurs as symptoms, followed by heart failure and death (2). The transition from adaptation to decompensation is mainly driven by LV remodeling (3,4). The extracellular matrix remodeling, mostly caused by diffuse interstitial fibrosis and/or focal replacement fibrosis, is the critical process of LV remodeling (5). Consequently, researchers are extensively interested in identifying and developing objective markers to detect structural and functional changes in the process of LV extracellular remodeling.
Cardiac magnetic resonance (CMR) imaging allows the comprehensive assessment of LV remodeling with its unlimited windows and excellent myocardial tissue characterization (6). CMR imaging can detect replacement myocardial fibrosis (MF) with late gadolinium enhancement (LGE) and detect diffuse interstitial fibrosis with the T1 mapping technique. T1 mapping-derived extracellular volume (ECV) has been reported as a promising marker for assessing diffuse MF in patients with AS (5,7). Both cellular hypertrophy and extracellular fibrosis can contribute to abnormal myocardial mechanics. Recently, myocardial deformation imaging assessed by echocardiography and/or CMR imaging has gradually become a valuable and noninvasive tool to reflect intrinsic myocardial contractility and functional consequences of related cardiac diseases (8). Although deformation imaging has been used to analyze MF and myocardial remodeling in various cardiac conditions (9-12), it was seldom reported in AS by the CMR-feature tracking (FT) technique. Furthermore, data regarding the utility of combined CMR T1 mapping and FT imaging for assessing the process of LV extracellular remodeling in AS are scarce.
The purpose of this study was to investigate the ability of CMR imaging parameters to identify and assess the changes in the process of LV extracellular remodeling in patients with severe AS using the T1 mapping and FT techniques. We present the following article in accordance with the STROBE (Strengthening the Reporting of Observational Studies in Epidemiology) reporting checklist, and MDAR reporting checklist (available at http://dx.doi.org/10.21037/cdt-20-803).
Methods
Study population
Patients with severe AS were prospectively and consecutively recruited from January 2018 to June 2019 in a single tertiary center. The inclusion criteria for the patient group were as follows (should meet two or more criteria): mean transvalvular pressure gradient (MPG) ≥50 mmHg, peak pressure difference gradient (PPG) ≥70 mmHg, and/or aortic valvular area (AVA) ≤1.0 cm2 as detected using echocardiography following guidelines (13). The exclusion criteria were as follows: moderate or severe concomitant valvular disease, history of myocardial infarction or acute coronary syndrome, contraindications to CMR imaging, and estimated glomerular filtration rate <30 mL/min/1.73 m2. Twenty healthy volunteers who were recruited from the community without cardiovascular disease were selected as controls. This study conformed to the ethical guidelines of the Declaration of Helsinki (as revised in 2013) and was approved by the Guangdong Provincial People’s Hospital, Guangdong Academy of Medical Sciences ethics committee (No. 20170216). Written informed consent was obtained from all participants.
Echocardiography
Transthoracic echocardiography was performed using a GE Vivid 9 (GE Vingmed Ultrasound, Horten, Norway) with an M5S probe (2–4 MHz) following the guidelines of the European Society of Echocardiography (14). Flow velocity across the aortic valve was measured at multiple transducer positions using continuous-wave Doppler. MPG and PPG were calculated with a simplified Bernoulli equation. AVA and AVA index was calculated using the continuity equation.
CMR imaging acquisition
All studies were performed on a 3.0-T magnetic resonance imaging scanner (Ingenia, Philips Medical Systems, Best, the Netherlands) using a standard clinical scan protocol. For cine imaging, a stack of short-axis single-shot balanced standard steady-state free-precession sequence images from the apex to the base were collected along with long-axis planes (two-, three-, and four-chamber views). The cine imaging parameters were as follows: field of view, 230×230 mm2; voxels, 2×2×8 mm3; TR, shortest; TE, shortest; sense factor, 2; minimum inversion time, 105 ms; and flip angle, 45°.
T1 mapping was performed with a modified look-locked inversion-recovery sequence in three short-axis slices, including basal, mid-, and apical ventricular planes. For pre-contrast, a 5s(3s)3s scheme was used. A total dose of 0.2 mmol/kg gadopentetate dimeglumine injection (Consun Pharmaceutical Co., Ltd.) was administered. For post-contrast, a 4s(1s)3s(1s)2s scheme was used approximately 15–17 min after the contrast ejection. The T1 mapping imaging parameters were as follows: field of view, 230×230 mm2; voxels, 2×2×8 mm3; TR, shortest; TE, shortest; sense factor, 2; minimum inversion time, 105 ms; and flip angle, 20°.
LGE imaging was performed using a phase-sensitive inversion-recovery (PSIR) sequence approximately 10–11 min after contrast ejection, covering a stack of short-axis images from the apex to the base along with long-axis planes (two-, three-, and four-chamber views). The PSIR imaging parameters were as follows: field of view, 230×230 mm2; voxels, 2×2×8 mm3; TR, shortest; TE, shortest; and TI, measured at that time.
CMR imaging analysis
LV volume, function, and LGE were analyzed offline using the QMASS software (Version 2.0, Medis, Leiden, the Netherlands). LGE extent was quantified using a signal intensity threshold level of five standard deviations (SDs), and expressed in grams and derived as a percentage of LV. For T1 mapping, myocardial T1 times were measured carefully in a global region of interest (ROI) at the whole mid-ventricular wall by sketching the endocardial and epicardial borders. Meanwhile, an ROI was drawn in the LV cavity to measure the blood pool T1 time (Figure S1). Areas with LGE were not excluded from T1 analysis. T1 mapping was calculated using the MapMarker software (Version 2.0, Medis, Leiden, the Netherlands). The λ and ECV were calculated as follows:
[1]
[2]
LV myocardial strain and strain rate analysis were evaluated by loading cine SSFP images the into the tissue tracking module (QStrain 2.0, Medis, Leiden, the Netherlands) using two-dimensional (2D) FT technique. The LV’s endocardial and epicardial borders were manually sketched in the end-diastolic and end-systolic phases, respectively (Figure S2). Trabeculations were all excluded from the endocardial borders. Global longitudinal strain (GLS) and strain rate were obtained from two-, three-, and four-chamber views for LV. Global circumferential strain (GCS), global radial strain (GRS), and strain rate were obtained from the LV’s basal, middle, and apical levels in the short-axis view.
Patients were divided into subgroups according to preserved LVEF (LVEF ≥50%) or reduced LVEF (LVEF% <50%). The intraobserver and interobserver reproducibility were tested in randomly selected 21 patients and 9 controls for measuring T1-derived and FT-derived parameters by 2 blinded investigators (with 5 and 12 years of CMR imaging experience).
LV myocardial biopsy
Thirteen patients with severe AS underwent myocardial biopsy at aortic valve replacement (AVR) surgery. Two to four biopsy samples from the septum were gathered, stained with hematoxylin-eosin and Masson trichome strain, and used for histopathological examination under a high-power microscope (Microscope BX-51-32F01, Olympus, ×200). The quantification of histologic fibrosis was performed using ImageJ software (http://rsb.info.nih.gov/ij) and expressed as collagen volume fraction (CVF). Five different histological slices were analyzed and averaged to quantify extracellular fibrosis burden.
Statistical analysis
Statistical analyses were conducted using SPSS version 17.0 software (IBM Inc., IL, USA) and MedCalc Statistical Software version 19.0.7 (MedCalc Software bvba, Ostend, Belgium). Normality was checked using the Shapiro-Wilk test. Continuous variables were expressed as mean ± standard deviation. Categorical variables were expressed as numbers and percentages. Groups were compared using the unpaired-sample t-test and chi-square test, as appropriate. The relationship between two continuous variables was assessed using simple linear regression. The strength of correlation was presented as a Pearson correlation coefficient. Univariate and multivariate binary logistic regression by the stepwise backward method was used to test the ability of CMR imaging measures to discriminate patients with preserved LV ejection fraction (LVEF) from healthy controls. The sensitivity, specificity, discrimination threshold, and area under the curve (AUC) were calculated using receiver operating characteristics curve (ROC) analysis. The intraclass correlation coefficient (ICC) and Bland-Altman plots were used to assess inter- and intraobserver reproducibility. A two-sided P value <0.05 was considered significant.
Results
Baseline characteristics
The demographic data of patients with severe AS are shown in Table 1. A total of 49 patients with severe AS (58.3±11 years; 20 male) were included; of these, 43 patients (43/49, 88%) had AVR. The bicuspid was the most common cause of AS (29/49, 59%). Regarding the echocardiographic indices of stenosis of the aortic valve, the MPG, PPG, AVA, and AVA index were 65.5±19.3 mmHg, 106.5±29.1 mmHg, 0.9±0.2 cm2, and 0.5±0.1 cm2/m2, respectively.
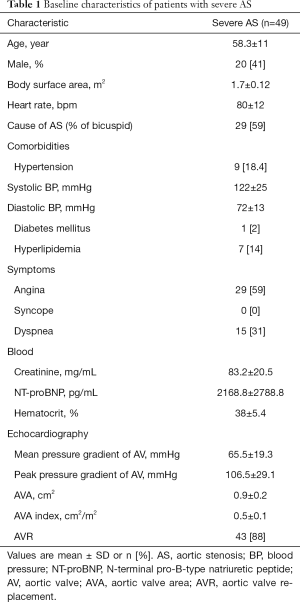
Full table
CMR imaging findings
The CMR imaging findings are summarized in Table 2. An increasing gradient of LV volume (LV end-diastolic index: 82.6±9.1 vs. 100.8±24.1 vs. 146.1±48.6 mL/m2, P<0.05; LV end-systolic index: 30.2±6.7 vs. 39.7±12.2 vs. 98.0±46.2 mL/m2, P<0.05) and hypertrophy (LV mass index: 37.4±7.2 vs. 85.7±24.1 vs. 125.1±38.4 g/m2, P<0.05) indices was observed from the control group (n=20) to the preserved LVEF AS group (n=29) to the reduced LVEF AS group (n=20). However, the LVEF (35.1%±11.1% vs. 61.1%±4.9%, P<0.001) and stroke volume (SV) index (48.2±15.0 vs. 61.2±13.6 mL/m2, P<0.01) of LV systolic function both decreased in patients with reduced LVEF group compared with the preserved LVEF group.
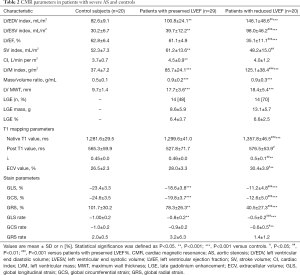
Full table
Regarding the parameters of fibrosis imaging, focal displacement fibrosis measured by LGE was detected in 28 patients (28/49, 57%) with infarct-like and/or non-infarct two patterns. There was no statistically significant difference in focal displacement fibrosis burden between patients with preserved LVEF and reduced LVEF groups (LGE%: 6.4%±3.7% vs. 6.6%±2.5%, P>0.05). For the diffuse fibrosis burden, the native T1 and ECV values estimated by T1 mapping was significantly higher in the reduced LVEF group than in the preserved LVEF group (1,357.8±46.5 vs. 1,299.6±41.0 ms, P<0.001; 30.4%±3.9% vs. 28.0%±3.3%, P<0.05), and higher than that in the healthy controls (1,357.8±46.5 vs. 1,281.6±29.5 ms, P<0.001; 30.4%±3.9% vs. 26.5%±2.3%, P<0.01). However, the native T1 and ECV values were statistically insignificant between patients with preserved LVEF and healthy controls (1,299.6±41.0 vs. 1,281.6±29.5 ms, P>0.05; 28.0%±3.3% vs. 26.5%±2.3%, P>0.05).
Regarding the strain parameters of FT, the values of global longitudinal strain (GLS) (–23.4%±3.3% vs. –18.6%±3.8% vs. –11.2%±4.8%, P<0.05), circumferential strain (GCS) (–24.6%±3.5% vs. –19.8%±3.7% vs. –12.6%±5.0%, P<0.05), and radial strain (GRS) (101.7%±30.2% vs. 78.3%±26.3% vs. 40.5%±27.3%, P<0.05) values showed increasing impairment from the control group to the preserved LVEF AS group to the reduced LVEF AS group.
Analysis of relationships
A significant linear correlation was found between ECV and CVF (r=0.64, P=0.020). However, the correlation between GLS and CVF was insignificant (P=0.538) (Figure 1). Linear correlation analysis between ECV/GLS and global LV structural/functional parameters in patients with severe AS (Figure 2) revealed significant correlations between GLS and LVMi (r=0.072, P=0.006) and between GLS and LVEF (r=−0.83, P<0.001). However, no significant correlations were observed between ECV and LVMi (P=0.172) and between ECV and LVEF (P=0.339).
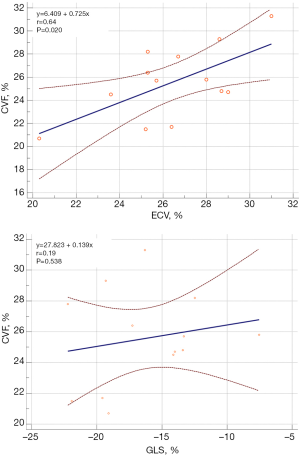
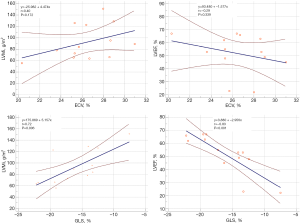
Discrimination of patients with preserved LVEF from controls
GLS yielded the best diagnostic performance as defined by AUC (0.83), followed by GCS (0.82), GLS rate (0.79), post T1 (0.71), and GRS (0.71), in discriminating patients with preserved LVEF from healthy controls. The discriminatory values of other parameters are summarized in Table 3. The GLS [Exp (B): 1.46, 95% confidence interval (CI): 1.16–1.85, P=0.002], ECV [Exp (B): 1.40, 95% CI: 1.02–1.90, P=0.035], and post T1 [Exp (B): 0.98, 95% CI: 0.79–0.99, P=0.042] were the significant parameters in discriminating patients with preserved LVEF from healthy controls. Univariate and multivariate binary logistic regression results are presented in Table 4.
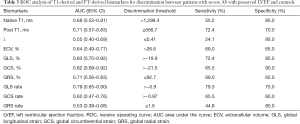
Full table
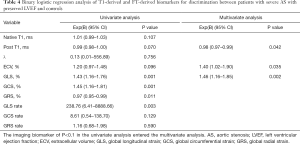
Full table
Intraobserver and interobserver reproducibility
The intraobserver and interobserver reproducibility of T1-derived and global LV strain parameters were calculated and assessed. Excellent intraobserver reproducibility was found for GLS (ICC =0.91; 95% CI: 0.83 to 0.96) and ECV (ICC =0.90; 95% CI: 0.81 to 0.95). Excellent interobserver reproducibility was found for GLS (ICC =0.91; 95% CI: 0.82 to 0.96) and slightly excellent interobserver reproducibility for ECV (ICC =0.86; 95% CI: 0.73 to 0.93). The results of ICCs are summarized in Table 5, and those of Bland-Altman plots are shown in Figure 3 and Figure S3.
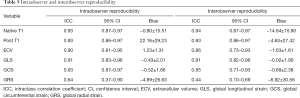
Full table
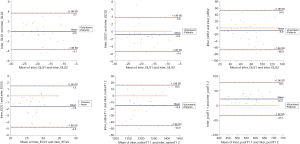
Discussion
The principal findings of the present study were as follows: (I) GLS was the functional marker of extracellular remodeling, which showed significant correlations between GLS and LVEF (r=−0.83, P<0.001). (II) GLS is the functional marker of myocardial mechanics related to disease state before the fibrosis burden intensifies. It showed increasing impairment from the control group to the preserved LVEF AS group to the reduced LVEF AS group (–23.4%±3.3% vs. –18.6%±3.8% vs. –11.2±4.8%, P<0.05), and showed the best diagnostic performance (AUC 0.83) as a significant discriminator between patients with preserved LVEF and controls. (III) ECV was the structural marker of extracellular diffuse fibrosis burden, which showed a significant correlation between ECV and CVF (r=0.64, P=0.020).
Myocardial deformation imaging has been reported as a sensitive modality to evaluate the functional consequences of MF (8,15). The LV function is determined by the sum of contraction and relaxation in the endo-, mid-, and epicardial layers. Therefore, the myocardial dysfunction in the involved myocardial layer can be classified into subendocardial, transmural, and subepicardial myocardial dysfunction (16). During the course of AS, the early stage of MF starts in the subendocardial layers leading to a reduction in longitudinal LV mechanics (17). However, LVEF, which is predominantly determined by mid-MF, can be normal even in the presence of extensive subendocardial fibrosis (3,18). Consistent with previous studies using the echocardiographic technique (19-21), the present study demonstrated a reduction in GLS in patients with AS compared with controls despite a preserved LVEF using the CMR-FT technique. These findings suggested that GLS might better characterize subtle mechanical changes in LV during the remodeling process in patients with AS and that GLS had an earlier diagnostic power than LVEF in this clinical setting. Furthermore, the present study showed that GLS, GCS, and GRS were increasingly impaired from the control group to the preserved LVEF AS group to the reduced LVEF AS groups, which indicated that subtle changes in longitudinal and radial deformation were gradually related to the disease state. Thus, an objective change in myocardial strain is more sensitive than observable symptoms and general functional parameters (e.g., LVEF and SV) in detecting a functional abnormality in AS.
GLS has been validated as a sensitive parameter in quantifying longitudinal LV function, which is more reproducible and less variable than other deformation indices in line with the results of reproducibility in the present study. CMR imaging strain parameters derived from FT could reflect early functional change using routine cine images in less than 10 min (22). As a simple and practical method, GLS acquired from FT is a promising technique in assessing functional consequences in the subclinical stage of severe AS, especially in patients with suboptimal echocardiographic image quality.
T1 mapping–derived ECV has emerged as a novel and promising marker to evaluate diffuse MF due to better histopathological correlation in the studies by Park (23) (r=0.645, P<0.001) and Chin (24) (r=0.70, P=0.016), in line with the findings of the present study (r=0.64, P=0.020). With regards to LV structure and function, no significant correlation of ECV with LVMi and LVEF was found, consistent with the study by Treibel (18), which showed that ECV correlated weakly with LVEF (r2=–0.096, P=0.001) and did not correlate with LVMi (P=0.06). However, a strong correlation of GLS with LV structure (LVMi) and function (LVEF) was found in the present study. Therefore, the ECV obtained using T1 mapping could be an ideal surrogate marker for detecting extracellular diffuse fibrosis burden but not be a sensitive marker for assessing the functional changes in LV extracellular remodeling.
A comparison of the control group with the preserved LVEF AS and reduced LVEF AS groups in the present study showed that GLS was an early marker of myocardial mechanics related to the disease state before the fibrosis burden intensified. When no difference was observed in extracellular fibrosis burden (ECV) between patients with preserved LVEF and controls, global LV strain parameters (e.g., GLS and GCS) had been impaired. This finding suggested that functional changes might be manifested before structural changes. Further studies on myocardial biopsy specimens from more early-stage and asymptomatic patients are needed to verify the findings.
This study had several limitations. First, this was a single-center cohort study with a small sample size. Hence, further larger-scale investigations are needed. Second, although the histopathological analysis (the gold standard for assessing fibrosis) was performed in some patients, the sample size was still small. Third, the present study using combined T1 mapping and FT techniques lacked follow-up and prognostic information. Studies with long-term follow-up are required to validate and expand the findings of this study in the future.
Conclusions
In the process of LV extracellular remodeling in severe AS, ECV is the structural marker of extracellular fibrosis burden, and GLS is the functional marker before the fibrosis burden intensifies. Although large-scale clinical studies are needed, the results suggested that the GLS and ECV must be combined to assess the process of LV extracellular remodeling to optimize clinical strategy and improve patient prognosis.
Acknowledgments
Funding: This study was funded by the National Natural Science Foundation of China (Grant No. 81974262), the Natural Science Foundation of Guangdong Province (Grant No. 2020A1515010650), the Guangdong Provincial People’s Hospital Project Grant (Grant No. 2016dzx01), and the Guangzhou City Science and Technology Planning Project of China (Grant No. 201707010306).
Footnote
Reporting Checklist: The authors have completed the STROBE reporting checklist and MDAR reporting checklist. Available at http://dx.doi.org/10.21037/cdt-20-803
Data Sharing Statement: Available at http://dx.doi.org/10.21037/cdt-20-803
Peer Review File: Available at http://dx.doi.org/10.21037/cdt-20-803
Conflicts of Interest: All authors have completed the ICMJE uniform disclosure form (available at http://dx.doi.org/10.21037/cdt-20-803). The authors have no conflicts of interest to declare.
Ethical Statement: The authors are accountable for all aspects of the study in ensuring that questions related to the accuracy or integrity of any part of the study are appropriately investigated and resolved. The research was conducted in accordance with the Declaration of Helsinki (as revised in 2013), and was approved by the Guangdong Provincial People’s Hospital, Guangdong Academy of Medical Sciences ethics committee (No.: 20170216). Written informed consent was obtained for all participants before CMR imaging.
Open Access Statement: This is an Open Access article distributed in accordance with the Creative Commons Attribution-NonCommercial-NoDerivs 4.0 International License (CC BY-NC-ND 4.0), which permits the non-commercial replication and distribution of the article with the strict proviso that no changes or edits are made and the original work is properly cited (including links to both the formal publication through the relevant DOI and the license). See: https://creativecommons.org/licenses/by-nc-nd/4.0/.
References
- Baumgartner H, Falk V, Bax JJ, et al. 2017 ESC/EACTS Guidelines for the management of valvular heart disease. Eur Heart J 2017;38:2739-91. [Crossref] [PubMed]
- Chin CW, Vassiliou V, Jenkins WS, et al. Markers of left ventricular decompensation in aortic stenosis. Expert Rev Cardiovasc Ther 2014;12:901-12. [Crossref] [PubMed]
- Hein S, Arnon E, Kostin S, et al. Progression from compensated hypertrophy to failure in the pressure-overloaded human heart: structural deterioration and compensatory mechanisms. Circulation 2003;107:984-91. [Crossref] [PubMed]
- Dweck MR, Boon NA, Newby DE. Calcific aortic stenosis: a disease of the valve and the myocardium. J Am Coll Cardiol 2012;60:1854-63. [Crossref] [PubMed]
- Bing R, Cavalcante JL, Everett RJ, et al. Imaging and Impact of Myocardial Fibrosis in Aortic Stenosis. JACC Cardiovasc Imaging 2019;12:283-96. [Crossref] [PubMed]
- Singh A, McCann GP. Cardiac magnetic resonance imaging for the assessment of aortic stenosis. Heart (British Cardiac Society) 2019;105:489-97. [Crossref] [PubMed]
- Kockova R, Kacer P, Pirk J, et al. Native T1 Relaxation Time and Extracellular Volume Fraction as Accurate Markers of Diffuse Myocardial Fibrosis in Heart Valve Disease- Comparison With Targeted Left Ventricular Myocardial Biopsy. Circ J 2016;80:1202-9. [Crossref] [PubMed]
- Claus P, Omar AMS, Pedrizzetti G, et al. Tissue Tracking Technology for Assessing Cardiac Mechanics: Principles, Normal Values, and Clinical Applications. JACC Cardiovasc Imaging 2015;8:1444-60. [Crossref] [PubMed]
- Hwang JW, Kim SM, Park SJ, et al. Assessment of reverse remodeling predicted by myocardial deformation on tissue tracking in patients with severe aortic stenosis: a cardiovascular magnetic resonance imaging study. J Cardiovasc Magn Reson 2017;19:80. [Crossref] [PubMed]
- Almaas VM, Haugaa KH, Strom EH, et al. Noninvasive assessment of myocardial fibrosis in patients with obstructive hypertrophic cardiomyopathy. Heart 2014;100:631-8. [Crossref] [PubMed]
- Cameli M, Mondillo S, Righini FM, et al. Left Ventricular Deformation and Myocardial Fibrosis in Patients With Advanced Heart Failure Requiring Transplantation. J Card Fail 2016;22:901-7. [Crossref] [PubMed]
- Hoffmann R, Altiok E, Friedman Z, et al. Myocardial deformation imaging by two-dimensional speckle-tracking echocardiography in comparison to late gadolinium enhancement cardiac magnetic resonance for analysis of myocardial fibrosis in severe aortic stenosis. Am J Cardiol 2014;114:1083-8. [Crossref] [PubMed]
- Nishimura RA, Otto CM, Bonow RO, et al. 2017 AHA/ACC Focused Update of the 2014 AHA/ACC Guideline for the Management of Patients With Valvular Heart Disease: A Report of the American College of Cardiology/American Heart Association Task Force on Clinical Practice Guidelines. J Am Coll Cardiol 2017;70:252-89. [Crossref] [PubMed]
- Nishimura RA, Otto CM, Bonow RO, et al. 2017 AHA/ACC Focused Update of the 2014 AHA/ACC Guideline for the Management of Patients With Valvular Heart Disease: A Report of the American College of Cardiology/American Heart Association Task Force on Clinical Practice Guidelines. Circulation 2017;135:e1159-95. [Crossref] [PubMed]
- Reisner SA, Lysyansky P, Agmon Y, et al. Global longitudinal strain: a novel index of left ventricular systolic function. J Am Soc Echocardiogr 2004;17:630-3. [Crossref] [PubMed]
- Sengupta PP, Narula J. Reclassifying heart failure: predominantly subendocardial, subepicardial, and transmural. Heart Fail Clin 2008;4:379-82. [Crossref] [PubMed]
- Heymans S, Schroen B, Vermeersch P, et al. Increased cardiac expression of tissue inhibitor of metalloproteinase-1 and tissue inhibitor of metalloproteinase-2 is related to cardiac fibrosis and dysfunction in the chronic pressure-overloaded human heart. Circulation 2005;112:1136-44. [Crossref] [PubMed]
- Treibel TA, Lopez B, Gonzalez A, et al. Reappraising myocardial fibrosis in severe aortic stenosis: an invasive and non-invasive study in 133 patients. Eur Heart J 2018;39:699-709. [Crossref] [PubMed]
- Kusunose K, Goodman A, Parikh R, et al. Incremental prognostic value of left ventricular global longitudinal strain in patients with aortic stenosis and preserved ejection fraction. Circ Cardiovasc Imaging 2014;7:938-45. [Crossref] [PubMed]
- Delgado V, Tops LF, van Bommel RJ, et al. Strain analysis in patients with severe aortic stenosis and preserved left ventricular ejection fraction undergoing surgical valve replacement. Eur Heart J 2009;30:3037-47. [Crossref] [PubMed]
- Nagata Y, Takeuchi M, Wu VC, et al. Prognostic value of LV deformation parameters using 2D and 3D speckle-tracking echocardiography in asymptomatic patients with severe aortic stenosis and preserved LV ejection fraction. JACC Cardiovasc Imaging 2015;8:235-45. [Crossref] [PubMed]
- Pedrizzetti G, Claus P, Kilner PJ, et al. Principles of cardiovascular magnetic resonance feature tracking and echocardiographic speckle tracking for informed clinical use. J Cardiovasc Magn Reson 2016;18:51. [Crossref] [PubMed]
- Park SJ, Cho SW, Kim SM, et al. Assessment of Myocardial Fibrosis Using Multimodality Imaging in Severe Aortic Stenosis: Comparison With Histologic Fibrosis. JACC Cardiovasc Imaging 2019;12:109-19. [Crossref] [PubMed]
- Chin CWL, Everett RJ, Kwiecinski J, et al. Myocardial Fibrosis and Cardiac Decompensation in Aortic Stenosis. JACC Cardiovasc Imaging 2017;10:1320-33. [Crossref] [PubMed]