7.0T cardiac magnetic resonance imaging of right ventricular function in rats with high-altitude deacclimatization
Highlight box
Key findings
• Partial recovery of right ventricular function in plateau acclimatized rats after returning to plain for 4 weeks, However, the right ventricular systolic function and strain did not recover completely.
What is known and what is new?
• In our previous study, we found that the structure and function of the right ventricle in rats changed after 12 weeks of exposure to high-altitude environment.
• Recently, we observed that the right ventricular systolic function and strain did not recover completely after 4 weeks of returning to the plain, which is a new discovery.
What is the implication, and what should change now?
• This implicated that the damage of high-altitude hypoxia on cardiac function is reversible, and high-altitude deacclimatization cannot be achieved in a short time.
Introduction
When people who live in the plain area enter a plateau environment with high-altitude for a period of time, a series of functional and structural changes occur to the body to adapt to the plateau environment. When they return to the plain, the adaptability to the plateau hypoxia environment is gradually eliminated, and functional, metabolic, and even structural changes appear to re-adapt to the plain environment. This is known as high-altitude deacclimatization (HADA) (1-5). Epidemiological studies show that about 50–80% of high-altitude immigrants and natives will suffer from high-altitude deacclimatization syndrome (HADAS) after returning to the plain, and this is characterized by dizziness, palpitation, drowsiness, fatigue, chest tightness, arrhythmia, and other clinical manifestations (1,3). These symptoms can persist for several years, and some people may even have to return to the plateau (6,7). The severity and incidence of HADAS are positively correlated with altitude. HADAS has serious impacts on the health, quality of life, and working ability of people at high altitude. Therefore, efforts to understand this chronic altitude sickness, constructing border areas to protect the health of people returning to the plain from high-altitude, reducing the acclimatization time, and alleviating symptoms, are crucial in achieving national health.
Cardiac magnetic resonance (CMR) technology has been widely used in the diagnosis of clinical cardiovascular diseases (8). Cardiac film sequence can collect real-time and dynamic data of cardiac function, and accurately evaluate changes in cardiac function. CMR tissue tracking (CMR-TT) is a major focus in magnetic resonance imaging (MRI) cardiac function research. Compared with ejection fraction (EF) which is commonly used in clinics, CMR-TT can detect and evaluate cardiac function damage earlier, and is also a sensitive index for the diagnosis of many kinds of heart diseases (9,10). 7.0T CMR cine sequence can not only accurately evaluate cardiac function parameters, but also have better tissue contrast and superior display effect for local fine structures (11-13). It has been widely used in animal imaging experiments (14,15).
The study of HADA is still in its infancy. Since the pathophysiological mechanism of HADA occurs on the basis of high-altitude acclimatization, these events must be studied together rather than as isolated events. Our previous investigation (16) demonstrated that a high-altitude hypobaric hypoxia environment exerts different effects on the structure, function, and strain of the right ventricle of rat hearts. This current study used 7.0T CMR cinematography and CMR-TT technique to further investigate the recovery of right ventricular function after returning to a plain oxygen-enriched environment for a period of time. This imaging evidence will provide a theoretical basis for the study of cardiac changes in HADA. We present the following article in accordance with the ARRIVE reporting checklist (available at https://atm.amegroups.com/article/view/10.21037/atm-22-5991/rc).
Methods
Experimental animals
A total of 30 6-week-old, male, specific pathogen-free (SPF) Sprague Dawley (SD) rats were purchased from Chengdu Dossy Experimental Animals Co., Ltd. (Chengdu, China). Rats were randomly divided into 3 groups (n=10 per group) and kept at different altitudes. Rats in the plain group were housed in Chengdu, Sichuan, at an altitude of 360 m for 12 weeks. Rats in the plateau group were maintained in Yushu, Qinghai, at an altitude of 3,850 m for 12 weeks. Rats in the HADA group were kept in the plateau for 12 weeks, followed by 4 weeks in the plain. All rats were housed individually in a laboratory environment at 18–26 ℃ and 45–70% humidity, with free access to food and water. This study was conducted according to the guidelines for Nursing and Use of Experimental Animals (17). The study was approved by the Ethics Committee of Qinghai Provincial People’s Hospital (approval No. 2022-26) and conducted in accordance with relevant provisions of ethical principles such as animal protection and animal welfare and the Drug Inspection and Testing in Qinghai Province Institutional Guidelines for the Care and Use of Animals. A protocol was prepared before the study without registration.
CMR imaging
All scans were performed on a 7.0T Bruker Biospec 70/30 MRI scanner (Bruker, Germany) equipped with radiofrequency birdcage coils of 72 mm inner diameter (I.D.) and surface array receiving coils of 56 mm I.D. The rats were placed in a sealed glass container and anesthetized with 2–3% lurane premixed in oxygen on a MatrxTM VIP 3000 small animal anesthesia machine (Midmark Co., USA). The chest of the rat was positioned in the center of the coil. The electrocardiograph (ECG) electrode was inserted into the forelimb and the right hindlimb, and the respiratory sensor was placed on the abdomen. After the respiratory and ECG signals were stable, the chest three-plane positioning sequence was scanned, and the standard left ventricular short axis position was determined after scanning the four-chamber view of the reference image. The Cine-Flash-flc imaging data were obtained from the short-axis plane from the apex to the bottom of the heart. The images of the continuous short axis, two-chamber and four-chamber heart of the left and right ventricles were obtained by imaging 8 layers of rat heart from apical to fundus. The following imaging parameters were set: echo time 2.5 ms, repetition time 8 ms, flip angle 20°, slice thickness 1.5 mm, visual field range (FOV) 50 mm × 50 mm, matrix size 256×256, and excitation times (NEX) = 4.
Data acquisition
The CMR-TT imaging data were processed using the cvi42 software (Circle Cardiovascular Imaging Inc., Canada) by a radiologist with substantial CMR experience. The software automatically identified the end-diastolic and end-systolic phases of the heart, and on this basis, the endo- and epicardial borders at end-systole and end-diastole were carefully drawn out. The cvi42 SD short model was used to calculate the left and right ventricle functional parameters including the end-diastolic volume (EDV), end-systolic volume (ESV), stroke volume (SV), and EF. The cvi42 Tissue Tracking model was used to calculate the left and right ventricular myocardial strain parameters including the peak global longitudinal strain (GLS), global radial strain (GRS), and global circumferential strain (GCS).
Histopathology
After the CMR data acquisition was completed, the rats were sacrificed by potassium chloride injection, and the heart tissue was collected, washed, fixed in 10% formaldehyde, and embedded in paraffin. Paraffin slices were prepared, dewaxed, stained with hematoxylin-eosin (HE), and analyzed under light microscopy.
Blood tests
Blood samples were collected from the caudal vein, and the levels of red blood cells (RBC), hemoglobin (HGB), and hematocrit (HCT) were measured.
Statistical analysis
The SPSS software (version 23.0) was used for data analysis. All results are presented as mean and standard deviation (SD). The normality test and variance homogeneity test were performed. One way analysis of variance (ANOVA) was used to compare the data among the three groups, and independent sample t-test was used to compare the data between the two groups. A P value less than 0.05 was considered statistically significant.
Results
Hematological parameters
Moving to a high-altitude can cause hyperventilation and tachycardia to cope with the low pressure and hypoxia environment (18). Residents living in high-altitude regions usually have elevated levels of RBC, HGB, and HCT to compensate for the low partial pressure of oxygen (19,20). In this study, the levels of RBC, HGB, and HCT in the blood of rats exposed to a high-altitude environment for 12 weeks were significantly increased, indicating that these rats acquired a greater capacity for blood oxygen transport to adapt to the high-altitude hypobaric and hypoxic environment. Interestingly, in the HADA group, after returning from the plateau to the plain for 4 weeks, the blood oxygen transport capacity returned to normal levels (P<0.001; Table 1; Figure 1A-1C).
Table 1
Index | Plain group | Plateau group | HADA group | F value | P value |
---|---|---|---|---|---|
RBC count, 1012/L | 8.81±0.48^ | 9.66±0.45 | 8.63±0.31^ | 17.26 | 0.000 |
HGB, g/L | 161.40±6.13^ | 174.70±7.09 | 163.90±4.43^ | 13.95 | 0.000 |
HCT, % | 47.67±2.09^ | 53.60±2.37 | 48.56±1.75^ | 23.42 | 0.000 |
The data are presented as mean ± standard deviation. ^, P<0.001, relative to plateau group. RBC, red blood cell; HGB, hemoglobin; HCT, hematocrit; HADA, high-altitude deacclimatization.
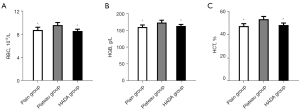
Right ventricular function
Since right ventricular end-diastolic volume (RVEDV), right ventricular end-systolic volume (RVESV), right ventricular stroke volume (RVSV), and right ventricular ejection fraction (RVEF) are important parameters of cardiac function, these were assessed using CMR (Figure 2A,2B). After 12 weeks of continuous exposure to high-altitude, the plateau rats had significantly higher RVEDV, RVESV, and RVSV values compared to the plain rats (P<0.05). The RVEF value also increased, however, this did not reach statistical significance (P>0.05). These data suggested that the right ventricular pumping function of the plateau rats was compensated so as to adapt to the hypoxic environment. Four weeks after returning to the plain, the RVEDV and RVSV values of the HADA group basically returned to that observed in plain rats. Interestingly, the RVESV value of the HADA group was higher than that of the plain and plateau group, while the RVSV and RVEF values were lower than that in the plain and plateau groups. These results suggested that the right ventricular systolic function did not recover completely, and the ventricular systolic function was irreversible impaired (Figure 3A-3C; Table 2).
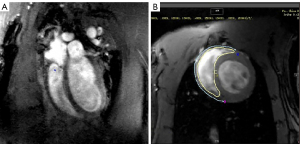
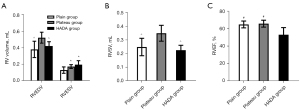
Table 2
Index | Plain group | Plateau group | HADA group | F value | P value |
---|---|---|---|---|---|
RVEDV, mL | 0.38±0.09^ | 0.53±0.06 | 0.43±0.05^ | 9.54 | 0.001 |
RVESV, mL | 0.13±0.04 | 0.18±0.02* | 0.20±0.05* | 7.74 | 0.002 |
RVSV, mL | 0.25±0.06^ | 0.35±0.06 | 0.23±0.04^ | 14.85 | 0.000 |
RVEF, % | 65.45±3.93# | 66.35±3.97# | 53.65±8.16 | 15.41 | 0.000 |
RVGCS, % | −20.42±3.55^ | −16.07±3.22 | −20.29±3.12^ | 5.61 | 0.009 |
RVGLS, % | −18.75±5.62 | −12.81±2.30* | −12.75±4.88* | 5.88 | 0.008 |
RVGRS, % | 49.47±7.54^ | 34.97±8.92 | 45.99±10.87^ | 6.74 | 0.004 |
The data are presented as mean ± standard deviation. *, P<0.05, relative to the plain group; ^, P<0.05, relative to plateau group; #, P<0.05, relative to HADA group. RVEDV, right ventricular end-diastolic volume; RVESV, right ventricular end-systolic volume; RVSV, right ventricular stroke volume; RVEF, right ventricular ejection fraction; RVGCS, right ventricular global circumferential strain; RVGLS, right ventricular global longitudinal strain; RVGRS, right ventricular global radial strain; HADA, high-altitude deacclimatization.
Right ventricular strain
Myocardial strain is an important index used to evaluate myocardial dysfunction in patients with heart failure, of which GLS is a reliable index to reflect cardiac systolic function (21). CMR-TT imaging showed that the RVGCS, RVGRS, and RVGLS values of the rats in the plateau group were lower than that in the plain group (Figure 4A,4B). In the HADA group, 4 weeks after returning to the plain from the plateau, the RVGCS and RVGRS values basically returned to levels that were comparable to the plain rats. It is interesting that the RVGLS value of the HADA group was significantly lower than that of the plain group. A study has found that when healthy people in the plain reached an altitude of 5,050 m, their RVGLS decreased significantly (22). All these data showed that the hypoxic environment can cause overall strain and damage to the right ventricle, and this cannot be fully reversed even after returning to the plain (Figure 5A-5C; Table 2).
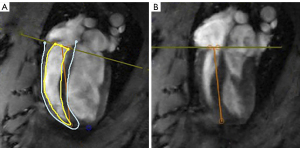
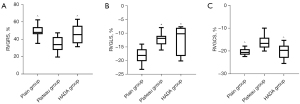
Pathology of the myocardial tissue
HE staining was performed to assess the histology of the ventricular myocardium. The right ventricular myocardium of rats in the plain group was normal, as were the tissue of the muscle strips and the morphology and interstitium of the cardiomyocytes. Furthermore, the nucleus was clear (Figure 6A). There was slight muscle strip separation, myocardial steatosis in rats in the plateau group (Figure 6B,6C). These pathological changes detected in the right ventricular myocardium in plateau rats were consistent with damage of right ventricular function. In the HADA rats, at 4 weeks after returning to the plain, there were no obvious abnormal changes to the histology of the heart, except for a small amount of steatosis (Figure 6D), indicating that some pathological changes in the myocardial tissue and interstitium had recovered.
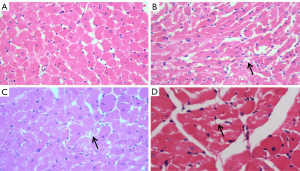
Discussion
Analysis of the hematological parameters
High-altitude acclimatization is the physiological stress response of the body to protect tissues and cells from injury after entering the high-altitude environment. In this study, the levels of RBC, HGB, and HCT in the blood of rats increased significantly after exposure to high altitude for 12 weeks, indicating that the rats increased the capacity of blood oxygen transport to meet the oxygen demand of tissues under the hypoxic environment. Studies have shown that when individuals enter the hypoxic environment, the stress response regulates the cardiovascular system through nerves, body fluids, and the endocrine system, resulting in an increase in heart rate, pulmonary artery pressure, and peripheral blood erythrocytes, thereby increasing the oxygen-carrying capacity of RBCs to meet the oxygen demand of tissues (23-25). Moreover, hypoxia can induce increased erythropoietin (EPO) synthesis mediated by hypoxia-inducible factor (HIF), which then stimulates the production of RBCs and increases the levels of HGB and HCT (26). This series of physiological changes are compensatory changes.
Four weeks after returning to the plain environment, the values of RBC, HGB, and HCT in HADA rats decreased to levels that were slightly lower than observed in the plain rats, indicating that the blood oxygen transport ability of these rats had returned to normal levels after re-adaptation and regulation of hypoxia/reoxygenation (H/R). It should be noted that the physiological processes related to HADA are time dependent. Indeed, the adaptive changes and acquired hypoxia tolerance do not reverse immediately after returning to the plain area, but gradually recover over time (3). The results of previous multicenter, randomized, controlled studies showed that the severity and duration of HADAS were positively correlated with altitude, the period of high-altitude acclimatization, and labor intensity at high altitude (6). It was the best choice to enter the plateau through ladder adaptation training. If the stepwise adaptation to low altitude areas could be selected for deacclimatization, the deacclimatization time and symptoms could be reduced. (7). However, a previous study has shown that after returning to the plain, the indexes of blood pressure, heart rate, and hemorheology of the migrants at high altitude were lower than those of healthy people in the plain, which is similar to the phenomenon of “overcorrection” (27). In this current study, similar interesting results were observed. It may be that this is part of the overall changes in the process of body re-adaptation, which may be related to hypoxia/ reoxygenation injury.
The effects of high-altitude hypoxia on right ventricular function and strain
Increased numbers of RBCs can help to improve the oxygen-carrying capacity and oxygen transport capacity of blood at high altitude, which compensates for the effect of hypobaric hypoxia on tissues. However, the increased erythropoiesis caused by hypoxia is a double-edged sword. Excessive increases in RBC, HGB, and HCT can lead to increased blood viscosity, increased blood flow resistance, decreased blood flow velocity, and increased cardiac afterload, which can result in a vicious circle of reduced cardiac output and contradictory hypoxia, eventually leading to high-altitude heart disease (HAHD) (28). Hypoxic pulmonary hypertension and thickening of the pulmonary arterioles are the central links and fundamental characteristics of the pathogenesis of HAHD. Long-term hypoxia stimulation can lead to pulmonary artery hyperplasia and pulmonary vascular remodeling (29,30). Pulmonary vascular remodeling can lead to an irreversible increase in pulmonary artery pressure and right ventricular afterload, resulting in right ventricular hypertrophy and decreased function (31,32). In this current study, compared with plain rats, plateau rats at high altitude had significantly increased RVEDV, RVESV and RVSV, slightly elevated RVEF, and significantly decrease, RVGLS, RVGCS, and RVGRS after 12 weeks, indicating that in order to adapt to high-altitude hypoxia, the right ventricular pumping function increased compensatively, and the right ventricle was damaged due to increased output and afterload. At the same time, it also indicated that the myocardial strain changed in the early stages of heart disease, since myocardial strain is more sensitive than EF in the detection of cardiac function.
Recovery of right ventricular function and strain in rats with HADA
Myocardial strain is the interaction between the systolic and diastolic myocardium. Yan et al. demonstrated that cardiac fibroblasts express α-smooth muscle actin (SMA) in a hypoxic environment, transforms to myofibroblasts and induces myocardial fibrosis (33). Studies have shown that the effects of high-altitude hypobaric and hypoxic environments on the myocardium are reversible, and the cardiac function and structure may be recovered to a certain extent, for example, hypertrophic myocardial fibers can gradually become smaller (34). Previous studies on HADA mainly focused on the changes of clinical symptoms, myocardial zymogram, hematological indicators and their mechanisms. There were no similar studies in right ventricular function caused by HADA at present. Our study found that compared with plateau rats, the RVEDV of HADA rats decreased. Interestingly, RVESV increased, resulting in the decrease of RVSV and RVEF, which indicated that after the transition from hypoxia to normothermia, arterial oxygen saturation increased, the body no longer needed more blood to transport oxygen to various systems and organs, the heart preload decreased, the diastolic function of the right ventricle was improved, and the compliance was increased. However, the contractile function was not restored nor improved. The RVEDV and RVSV of HADA rats basically returned to the plain state, indicating that the rats gradually adapted to the plain oxygen-rich environment, and the right ventricle was pumping according to the body’s demand. However, the RVESV of HADA rats was significantly higher than that of plain rats, and the RVEF was significantly lower than that of plain rats, indicating that the right ventricular contractile function did not fully recover when the rats returned to the plain for 4 weeks, resulting in decreased EF (7).
Pulmonary hypertension caused by long-term hypoxia, elevated right ventricular afterload, increase in right ventricular dilatation and filling pressure, right ventricular hypertrophy, and fibrosis can lead to decreased compliance, resulting in a significant decrease in right ventricular strain. In this study, 4 weeks after returning to the plain normoxic environment, the RVGCS and RVGRS of HADA rats increased significantly, but RVGLS did not recover, indicating that there was partial recovery of myocardial strain in plateau rats after a period of adaptation to the normoxic environment.
Analysis of the myocardial pathological results
Previous studies on HADAS have focused on human beings. However, since it is difficult to obtain relevant pathological data, there are few reports on the pathological characteristics of HADAS. In this study, histological examination showed pathological changes in the right ventricular myocardium at high altitude, including mild muscle strip separation, cardiomyocyte steatosis. HADAS occurs at low altitudes after subjects return from high-altitude, and its occurrence and development involve damage caused by H/R (35). Oxidative stress mediated by H/R can induce the release of inflammatory mediators and activate many signal pathways, resulting in apoptosis and tissue damage (36-40). Several studies have reported that H/R causes injury and inflammatory response to the nervous system and myocardium, and increases myocardial enzyme levels (40-45). Patients with HADAS showed inflammatory reaction and myocardial injury on the third day after returning to the plain, with the elevated levels of inflammatory mediators [tumor necrosis factor (TNF)-α and interleukin (IL)-17A] decreasing over time (3,7), indicating that the inflammatory response induced by H/R was involved in the occurrence of HADAS and decreased over time.
Conclusions
In summary, under the stimulation of a hypoxic environment for a long time, the right ventricular function of rats changed to adapt to the high-altitude hypoxia environment. However, 4 weeks after the rats were removed from the high-altitude hypoxia environment and entered the plain normoxic environment, the physiological changes caused by hypoxia could not be reversed immediately due to various body regulatory processes. Furthermore, the right ventricular function could not be fully recovered.
Advantages and limitations
Based on the true replication of high-altitude hypobaric and hypoxic environment, we observed the changes in cardiac structure and function of rats under hypobaric and hypoxic environments and obtained some relevant pathological data. This study more accurately demonstrates the pathophysiological characteristics of heart disease in rats under high-altitude environments, and provides an experimental and theoretical basis for the study of high-altitude diseases in the future. There were some limitations to this study, including the small sample size and short exposure time at high altitude. Furthermore, the evolution of right ventricular function and pathophysiology in rats could not be studied in stages. Future studies may include a larger sample size and longer exposure times.
Acknowledgments
Funding: This study was supported by the National Natural Science Foundation of China (Nos. 81930046, 81771800, to Fabao Gao), the Qinghai Provincial Health Committee Guiding Program Project (No. 2020-wjzdx-04, to Yanqiu Sun), the Qinghai Provincial Department of Science and Technology Basic Research Project (No. 2021-ZJ-732, to Yanqiu Sun), and the Qinghai province “Kunlun Elite High-end Innovative and Entrepreneurial Talents” Program To Cultivate Leading Talents (Project No. Youth Talent Word (2021) No. 13, to Yanqiu Sun).
Footnote
Reporting Checklist: The authors have completed the ARRIVE reporting checklist. Available at https://atm.amegroups.com/article/view/10.21037/atm-22-5991/rc
Data Sharing Statement: Available at https://atm.amegroups.com/article/view/10.21037/atm-22-5991/dss
Conflicts of Interest: All authors have completed the ICMJE uniform disclosure form (available at https://atm.amegroups.com/article/view/10.21037/atm-22-5991/coif). YS reports that this study was supported by the Qinghai Provincial Health Committee Guiding Program Project (No. 2020-wjzdx-04), the Qinghai Provincial Department of Science and Technology Basic Research Project (No. 2021-ZJ-732), and the Qinghai province “Kunlun Elite High-end Innovative and Entrepreneurial Talents” Program To Cultivate Leading Talents (Project No. Youth Talent Word (2021) No. 13). FG reports that this study was supported by the National Natural Science Foundation of China (Nos. 81930046, 81771800). The other authors have no conflicts of interest to declare.
Ethical Statement: The authors are accountable for all aspects of the work in ensuring that questions related to the accuracy or integrity of any part of the work are appropriately investigated and resolved. The study was approved by the Ethics Committee of Qinghai Provincial People’s Hospital (approval No. 2022-26) and conducted in accordance with relevant provisions of ethical principles such as animal protection and animal welfare and the Drug Inspection and Testing in Qinghai Province Institutional Guidelines for the Care and Use of Animals.
Open Access Statement: This is an Open Access article distributed in accordance with the Creative Commons Attribution-NonCommercial-NoDerivs 4.0 International License (CC BY-NC-ND 4.0), which permits the non-commercial replication and distribution of the article with the strict proviso that no changes or edits are made and the original work is properly cited (including links to both the formal publication through the relevant DOI and the license). See: https://creativecommons.org/licenses/by-nc-nd/4.0/.
References
- Gao Y. Military medicine at high altitude. Chongqing: Chongqing Press; 2005:237-8.
- Dempsey JA, Powell FL, Bisgard GE, et al. Role of chemoreception in cardiorespiratory acclimatization to, and deacclimatization from, hypoxia. J Appl Physiol (1985) 2014;116:858-66. [Crossref] [PubMed]
- He B, Wang J, Qian G, et al. Analysis of high-altitude de-acclimatization syndrome after exposure to high altitudes: a cluster-randomized controlled trial. PLoS One 2013;8:e62072. [Crossref] [PubMed]
- Ryan BJ, Wachsmuth NB, Schmidt WF, et al. AltitudeOmics: rapid hemoglobin mass alterations with early acclimatization to and de-acclimatization from 5260 m in healthy humans. PLoS One 2014;9:e108788. [Crossref] [PubMed]
- Zhou Q, Yang S, Luo Y, et al. A randomly-controlled study on the cardiac function at the early stage of return to the plains after short-term exposure to high altitude. PLoS One 2012;7:e31097. [Crossref] [PubMed]
- Zhou QQ, Yang SY, Yuan ZC, et al. A research in diagnostic criteria of high altitude de-adaptation for plateau migrants returning to the plains: a multicenter, randomized controlled trial. Medical Journal of Chinese People’s Liberation Army 2012;37:146-55.
- Zhang HY, Zhang YX, Yang ZX, et al. High altitude acclimatization and de-acclimatization. Chinese Journal of Applied Physiology 2012;28:94-6.
- Chang KF, Lin G, Huang PC, et al. Left Ventricular Function and Myocardial Triglyceride Content on 3T Cardiac MR Predict Major Cardiovascular Adverse Events and Readmission in Patients Hospitalized with Acute Heart Failure. J Clin Med 2020;9:169. [Crossref] [PubMed]
- Hu L, Sun A, Guo C, et al. Assessment of global and regional strain left ventricular in patients with preserved ejection fraction after Fontan operation using a tissue tracking technique. Int J Cardiovasc Imaging 2019;35:153-60. [Crossref] [PubMed]
- Duo G, Liu T, Dai X. Cardiac magnetic resonance tissue tracking technique to evaluate the diagnostic value of coronary heart disease and myocardial infarction. Magnetic Resonance Imaging 2018;9:346-53.
- Yang B. Applied research and medical prospect of ultra-high field magnetic resonance imaging of human body. Miscellaneous Records of Spectroscopy 2015;32:707-14.
- Arts T, Siero JCW, Biessels GJ, et al. Automated Assessment of Cerebral Arterial Perforator Function on 7T MRI. J Magn Reson Imaging 2021;53:234-41. [Crossref] [PubMed]
- Qu L, Zhang Y, Wang S, et al. Synthesized 7T MRI from 3T MRI via deep learning in spatial and wavelet domains. Med Image Anal 2020;62:101663. [Crossref] [PubMed]
- Somma F, Berritto D, Iacobellis F, et al. 7T μMRI of mesenteric venous ischemia in a rat model: timing of the appearance of findings. Magn Reson Imaging 2013;31:408-13. [Crossref] [PubMed]
- Wang L, Zhu J, Zhu T, et al. 7 T cardiac magnetic resonance two-dimensional tissue tracking technique to evaluate the cardiac function of tree shrew. Journal of Sichuan University: medical Edition 2019; 050:478-82.
- Sun Y, Zhang C, Tian D, et al. Application of 7.0 T ultra-high-field MRI in evaluating the structure and function of the right ventricle of the heart in rats under a chronic hypoxic environment at high altitude. Ann Transl Med 2021;9:1585. [Crossref] [PubMed]
- Carbone L. Pain management standards in the eighth edition of the Guide for the Care and Use of Laboratory Animals. J Am Assoc Lab Anim Sci 2012;51:322-8.
- Grocott M, Montgomery H, Vercueil A. High-altitude physiology and pathophysiology: implications and relevance for intensive care medicine. Crit Care 2007;11:203. [Crossref] [PubMed]
- León-Velarde F, Gamboa A, Chuquiza JA, et al. Hematological parameters in high altitude residents living at 4,355, 4,660, and 5,500 meters above sea level. High Alt Med Biol 2000;1:97-104. [Crossref] [PubMed]
- Windsor JS, Rodway GW. Heights and haematology: the story of haemoglobin at altitude. Postgrad Med J 2007;83:148-51. [Crossref] [PubMed]
- Marwick TH, Shah SJ, Thomas JD. Myocardial Strain in the Assessment of Patients With Heart Failure: A Review. JAMA Cardiol 2019;4:287-94. [Crossref] [PubMed]
- Stembridge M, Ainslie PN, Hughes MG, et al. Ventricular structure, function, and mechanics at high altitude: chronic remodeling in Sherpa vs. short-term lowlander adaptation. J Appl Physiol 1985;2014:334-43. [Crossref] [PubMed]
- Azad P, Villafuerte FC, Bermudez D, et al. Protective role of estrogen against excessive erythrocytosis in Monge’s disease. Exp Mol Med 2021;53:125-35. [Crossref] [PubMed]
- Hansen AB, Moralez G, Amin SB, et al. Global REACH 2018: the adaptive phenotype to life with chronic mountain sickness and polycythaemia. J Physiol 2021;599:4021-44. [Crossref] [PubMed]
- Oberholzer L, Lundby C, Stauffer E, et al. Reevaluation of excessive erythrocytosis in diagnosing chronic mountain sickness in men from the world's highest city. Blood 2020;136:1884-8. [Crossref] [PubMed]
- Haase VH. Regulation of erythropoiesis by hypoxia-inducible factors. Blood Rev 2013;27:41-53. [Crossref] [PubMed]
- Zhang X, He F, Kang X, et al. Changes of RBC-SOD and GSH-PX after living at high altitude and returning to the plain. Chinese Journal of Internal Medicine 1994;448.
- Nima Y, De Y, Shi J, et al. Analysis of echocardiographic indicators of high altitude heart disease in Tibetans at different altitudes in Tibet. Chinese Journal of Medical Ultrasound 2019;16:306-10. (Electronic Edition).
- Reichenberger F, Kohstall MG, Seeger T, et al. Effect of sildenafil on hypoxia-induced changes in pulmonary circulation and right ventricular function. Respir Physiol Neurobiol 2007;159:196-201. [Crossref] [PubMed]
- Sylvester JT, Shimoda LA, Aaronson PI, et al. Hypoxic pulmonary vasoconstriction. Physiol Rev 2012;92:367-520. [Crossref] [PubMed]
- Xu XQ, Jing ZC. High-altitude pulmonary hypertension. Eur Respir Rev 2009;18:13-7. [Crossref] [PubMed]
- Penaloza D, Arias-Stella J. The heart and pulmonary circulation at high altitudes: healthy highlanders and chronic mountain sickness. Circulation 2007;115:1132-46. [Crossref] [PubMed]
- Yan J, Zhai Y, Zhang H, et al. Effects of hypoxia on phenotype of cardiac fibroblasts in adult rats. Chinese Journal of Aerospace Medicine 2005;16:117-20.
- Zhang Y, Zhou ZN. Beneficial effects of intermittent hypobaric hypoxia on the body. Zhongguo Ying Yong Sheng Li Xue Za Zhi 2012;28:504-9.
- He B, Li H, Hu M, et al. Association between Serum Interleukin-17A Level and High-Altitude Deacclimatization Syndrome. Mediators Inflamm 2016;2016:1732352. [Crossref] [PubMed]
- Nistri S, Boccalini G, Bencini A, et al. A new low molecular weight, MnII-containing scavenger of superoxide anion protects cardiac muscle cells from hypoxia/reoxygenation injury. Free Radic Res 2015;49:67-77. [Crossref] [PubMed]
- Li C, Jackson RM. Reactive species mechanisms of cellular hypoxia-reoxygenation injury. Am J Physiol Cell Physiol 2002;282:C227-41. [Crossref] [PubMed]
- Liu XJ, Tan Y, Geng YQ, et al. Proximal tubule toll-like receptor 4 expression linked to inflammation and apoptosis following hypoxia/reoxygenation injury. Am J Nephrol 2014;39:337-47. [Crossref] [PubMed]
- Inoue D, Numasaki M, Watanabe M, et al. IL-17A promotes the growth of airway epithelial cells through ERK-dependent signaling pathway. Biochem Biophys Res Commun 2006;347:852-8. [Crossref] [PubMed]
- Ban K, Kozar RA. Protective role of p70S6K in intestinal ischemia/reperfusion injury in mice. PLoS One 2012;7:e41584. [Crossref] [PubMed]
- Ngoh GA, Facundo HT, Hamid T, et al. Unique hexosaminidase reduces metabolic survival signal and sensitizes cardiac myocytes to hypoxia/reoxygenation injury. Circ Res 2009;104:41-9. [Crossref] [PubMed]
- Katayama K, Sato K, Matsuo H, et al. Effect of intermittent hypoxia on oxygen uptake during submaximal exercise in endurance athletes. Eur J Appl Physiol 2004;92:75-83. [Crossref] [PubMed]
- Kambara T, Ohashi K, Shibata R, et al. CTRP9 protein protects against myocardial injury following ischemia-reperfusion through AMP-activated protein kinase (AMPK)-dependent mechanism. J Biol Chem 2012;287:18965-73. [Crossref] [PubMed]
- Ma X, Liu H, Foyil SR, et al. Impaired autophagosome clearance contributes to cardiomyocyte death in ischemia/reperfusion injury. Circulation 2012;125:3170-81. [Crossref] [PubMed]
- Zhao MH, Jiang ZT, Liu T, et al. Flavonoids in Juglans regia L. leaves and evaluation of in vitro antioxidant activity via intracellular and chemical methods. ScientificWorldJournal 2014;2014:303878. [Crossref] [PubMed]