Effects and mechanisms of Celastrol on the formation of neutrophil extracellular traps (NETs)
Highlight box
Key findings
• Celastrol could inhibit PMA-induce NET formation in vitro.
What is known and what is new?
• NET formation is considered relate to ROS level.
• Inhibit ROS level may be the mechanism of Celastrol inhibit NET formation.
What is the implication, and what should change now?
• It provides an explanation that why Tripterygium wilfordii can treat rheumatic immune diseases.
Introduction
Tripterygium wilfordii is a traditional medicine with anti-inflammatory effects that has been widely used as a Chinese herbal medicine for a long time. In recent years, in-depth research on Tripterygium wilfordii has demonstrated its immunosuppressive (1), anti-inflammatory, anti-tumor (2), and anti-autophagy (3) effects. At present, Tripterygium wilfordii is widely used in the treatment of rheumatoid arthritis, systemic lupus erythematosus, and other rheumatoid immune diseases; however, its specific mechanism remains unclear. Although Tripterygium wilfordii has been applied in a variety of pharmaceutical preparations and clinical settings, it has exhibited a certain toxic effect in clinical applications. Animal experiments have demonstrated that large doses of Tripterygium wilfordii can cause damage to multiple organs, such as the liver and kidney (4). At the same time, as a Chinese patent medicine, the specific mechanism of action of Tripterygium wilfordii remains unknown, which has led to apprehension among many clinicians due to its side effects and restricted its clinical promotion. Therefore, identifying the active ingredients in Tripterygium wilfordii and exploring its specific and effective mechanism to reduce its toxic side effects are important directions of current research. Previous studies on the efficacy of Tripterygium wilfordii have focused on macrophages, fibroblast synovial cells (5-7), and other cells; however, the effects of Tripterygium wilfordii on neutrophils have rarely been studied.
A special mechanism of neutrophil death, known as NETosis, was discovered in 2004; when neutrophils die, they release their chromatin components to form a network structure [known as neutrophil extracellular traps (NETs)] to fix and inhibit the spread of bacteria, thereby promoting bacterial death (8). These mechanisms play an important role in the immune protection, inflammatory and cancer. In immune protection, neutrophils release NETs selectively in response to large pathogens (9). NETs itself also contain several antimicrobial agents which could kill bacteria (8). In inflammatory, NETs can both promote and reduce it. In NETs formation neutrophils formed aggregates and both released and degraded cytokines (10). In cancer, NETs are present in tumor microenvironment and fuel cancer progression and indicate poor prognosis (11).
However, increasing evidence has shown that the NETs produced by neutrophils are a double-edged sword. If the generated NETs are not promptly removed, they can cause damage to the body from various aspects. The direct damage caused by the NETs is mainly caused by the release of histones and deoxyribonucleic acid (DNA) outside the cell. The antibacterial and bactericidal effects of histones were discovered as early as 1958 (12), and they are also an important component of NETs. At the same time, histones also have a direct killing effect on normal tissues and organs of the body, including by directly killing cells, activating inflammation, activating platelets, protecting extracellular DNA components, as well as various unique roles in different diseases (13). All of these functions make histones an important cause of disease caused by NETs.
Another important component of the NETs network is the chromatin component DNA of neutrophils. Extracellular DNA is a substance with strong immunogenicity that can bind to receptors to activate inflammatory signals, produce inflammatory factors, or induce cell death. In a variety of autoimmune diseases such as systemic lupus erythematosus (14), rheumatoid arthritis (15), vasculitis (16), the level of neutrophil ROS is significantly increased (17), leading to NETs produced in large quantities, which results in the release of excessive inflammatory factors and aggravating the inflammatory response (18). NETs have also been found to be a source of various autoantibodies and are the initiating factors leading to the development of rheumatoid arthritis and systemic lupus erythematosus.
Celastrol, a triterpenoid compound extracted from Tripterygium wilfordii, is considered to be one of its main active components. Although Tripterygium wilfordii does not officially possess a clinical drug application, it has been the subject of numerous clinical studies. A study in 2015 elaborated on its artificial synthesis pathway (19). As an effective ingredient of medicine, Celastrol is easy to extract and has a synthetic basis for medicine production. A previous study of its drug effects found that Tripterygium wilfordii exerts unique effects in tumors (20), metabolic diseases (21), neurodegenerative diseases (22), and inflammation inhibition.
In addition to inhibiting angiogenesis, preventing the blood supply and metastasis of tumor cells (23), and increasing the sensitivity of tumor cells to DNA damage (24), Celastrol is also able to induce tumor cell apoptosis, which promotes the death of tumor cells (25). In neurodegenerative diseases with neuronal damage as the main manifestation, triptolide plays a role in protecting nerve cells. On the one hand, Tripterygium wilfordii directly inhibits neurodegenerative diseases, such as Alzheimer’s disease or Parkinson’s disease, by reducing β-amyloid and α-synuclein (26,27). At the same time, it also reduces the levels of ROS and promotes the production of apoptosis-related cytokines to indirectly protect nerve cells (27). As for inflammation, Tripterygium wilfordii has also been found to inhibit the production of inflammatory factors (28), prevent the migration of inflammatory cells, and reduce the swelling of inflammatory joints (29). Thus, it exerts a certain therapeutic effect on a variety of autoimmune diseases and has been confirmed in animal models of Crohn’s disease (28), rheumatoid arthritis (29), systemic lupus erythematosus (30), and other diseases. Similarly, in the process of inhibiting inflammation, studies have found that Tripterygium wilfordii can protect cells. For example, research on necrotizing colitis has demonstrated that triptolide inhibits the Receptor-interacting protein kinase 3-mixed lineage kinase domain-like protein (RIPK3-MLKL) pathway to prevent cell death due to necroptosis (programmed necrosis) (31), thereby reducing the impact of inflammation.
The mode of neutrophil death release NETs is related to a variety of autoimmune diseases. Moreover, Tripterygium wilfordii is also a therapeutic drug for several autoimmune diseases, and it is closely related to cell death and intracellular ROS levels. Therefore, we speculate that Celastrol can also protect cell viability during the NETs process and treat related autoimmune diseases. Therefore, this study aims to investigate whether Tripterygium wilfordii can inhibit the production of NETs by exerting an anti-inflammatory and immunosuppressive mechanism, and provide a theoretical basis for the further development and utilization of Tripterygium wilfordii. We present the following article in accordance with the MDAR reporting checklist (available at https://atm.amegroups.com/article/view/10.21037/atm-22-5720/rc).
Methods
Drugs and reagents
The following drugs and reagents were used in this study: Celastrol (Dalian Meilun Biological Biotechnology Co., Ltd., China); human neutrophil isolation solution (Tianjin Haoyang Biological Products Technology Co., Ltd., China); PMA (Sigma, Germany); 2',7'-Dichlorofluorescin diacetate (DCFH-DA) (Shanghai Biyuntian Biotechnology Co., Ltd., China); Hochest (Shanghai Biyuntian Biotechnology Co., Ltd., China); SytoxGreen (Thermo Fisher Technology, China); phosphate buffer (Hyclon, USA); and Roswell Park Memorial Institute (RPMI)-1640 medium (Hyclon Company, USA).
Instruments and consumables
The following instruments and consumables were used in this study: ultra-clean workbench (Suzhou Purification Equipment Co., Ltd., China); SpectraMax M5 multifunctional microplate reader (Molecular Devices, USA); desktop high-speed refrigerated centrifuge (Beckman, USA); inverted fluorescence microscope (Olympus, Japan); inverted optical microscope (Olympus); FACSVerse™ flow cytometer (BD Biosciences, USA); Midea refrigerator (Midea Group Co., Ltd., China); disposable human venous blood sample collection container (Jiangsu Kangjie Medical Instrument Co., Ltd., China); disposable venous blood sample collection needle (Shandong Chengwu Medical Products Factory, China); sterile rubber gloves (Liaoning Weihua Pharmaceutical Co., Ltd., China); iodophor disinfectant (Liaoning Weihua Pharmaceutical Co., Ltd.); pipette (Eppendorf, Germany); 15 mL centrifuge tube (Corning, USA); cell counting plate (Jiangsu Shitai Laboratory Equipment Co., Ltd., China); coverslips (Shanghai No.1 Biochemical Pharmaceutical Co., Ltd., China); and 96-well plates (Corning, USA).
Experimental method
Human peripheral blood neutrophil isolation method
All patients who participated in this study signed an informed consent form, and this study was approved by the Ethics Committee of The Third Affiliated Hospital of Southern Medical University (No. 2022-Lunshen-005), and was conducted in accordance with the Declaration of Helsinki (as revised in 2013).
After preparing the disposable venous blood sample collection needles, rubber gloves, disinfectant, and other blood collection equipment, we selected the more vigorous vein in the upper arm of the volunteer and disinfected the blood collection point and its surroundings. The blood was collected in a relatively sterile environment with a lancet into a blood sample collection container containing anticoagulant components, and 10 mL of venous peripheral blood was drawn from each healthy volunteer. The blood collection process was completed with the assistance of nurses from the Department of Rheumatology and Immunology at the Third Affiliated Hospital of Southern Medical University.
Next, we sterilized the blood collection tube and moved it under the ultra-clean table, and added it to a pre-prepared 15 mL centrifuge tube containing a neutrophil separation liquid. We added the blood to the neutrophil separation liquid slowly to stratify them as much as possible. Following centrifugation (500 g for 35 minutes at room temperature), two layers of ring-shaped white cell bands were visible to the naked eye. After extracting the neutrophils in the lower layer, the RPMI-1640 culture medium was used to wash off the excess neutrophil separation solution, and the supernatant was discarded. We then added the RPMI-1640 culture medium to resuspend the cells, added an appropriate amount of red blood cell lysate, and it let stand for 10 minutes at 4 degrees. We then observed the cell pellet after 1,000 revolutions and centrifugation for 5 minutes. If the red blood cells did not appear red, they were considered fully lysed; otherwise, an appropriate amount of red blood cell lysate was added and lysed again until the red blood cells were not visible to the naked eye in the cell pellet after centrifugation. We subsequently discarded the supernatant and washed the cells using the RPMI-1640 culture medium.
Next, we added 1 mL of RPMI-1640 culture medium to resuspend the cells and used a pipette to take 10 µL of the cell suspension and place it in the space between the cell counting plate and the cover glass. We then counted the number of cells in the four large squares on one side of the cytometer under an optical microscope [neutrophil count = (total number of cells in the four large squares/4) × 104 × dilution factor]. Subsequently, the RPMI-1640 culture medium was used to dilute the cells according to the experimental requirements and then set them aside.
Quantitative detection of NETs
The isolated neutrophils were added into 96-well plates (at 100,000 cells/well in equal amounts) and divided into a Control group, a phorbol ester (PMA) group, a PMA + Celastrol (PMA + cel) group, and a Celastrol (cel) group. The corresponding concentration of Celastrol was added in the cel and PMA + cel groups. After 30 minutes of incubation in the cell incubator, PMA (25 nM) was added to the PMA and PMA + cel groups. After a further 3 hours of incubation in the cell incubator, 5 µM SytoxGreen solution was added under “light-off” conditions, and the cells were then placed in the cell incubator and incubated for another 10 minutes. Subsequently, the fluorescence intensity of SytoxGreen was detected at 528 µM using a microplate reader under 485 µM light excitation.
NETs immunofluorescence staining
After grouping the separated neutrophils according to 1.5.2, they were placed in a 37 ℃, 5% CO2 cell incubator. In the cel and PMA + cel groups, the corresponding concentration of Celastrol was added. After 30 minutes of incubation in the cell incubator, PMA (25 nM) was added to the PMA and PMA + cel groups, and the cells were incubated for another 3 hours. After adding Hochest and SytoxGreen in a dark environment, we observe the cell conditions under a fluorescent microscope and selected representative images.
Neutrophil ROS detection
The corresponding concentration of Celastrol was added in the cel and PMA + cel groups. After 30 minutes of incubation in the cell incubator, PMA (25 nM) was added to the PMA and PMA + cel groups. After incubating in the cell incubator for a further 30 minutes, 10 µM DCFH-DA was added under dark conditions and mixed well, and then incubated in the cell incubator for another 30 minutes in the dark. After centrifugation at low speed, the supernatant was removed, washed with phosphate buffered saline (PBS), and centrifuged again, and the flow cytometer and multi-function microplate reader were excited by 485 µM of light. DCFH luminescence was observed at 528 µM to detect the ROS level.
Statistical methods
Data in all graphs were presented as the mean ± standard deviation () and conformed to a normal distribution. SPSS 21.0 statistical software (International Business Machines Corporation, USA) was used for data analysis, and GraphPad Prism 5.0 (GraphPad Software, USA) analytics and graphics software was used for graphing. One-way analysis of variance (ANOVA) was used to compare the means between groups. P<0.05 was considered statistically significant.
Results
Celastrol inhibits the formation of NETs
To determine the optimal non-toxic concentration of Celastrol for neutrophils, we first used a concentration of 5 µM to conduct the experiments. Neutrophils from healthy volunteers were separated and treated with triptolide and then with 25 nM of PMA for 3 hours to induce NETs in the cells. The fluorescence intensity of SytoxGreen (Figure 1), as detected by a multifunctional microplate reader, indirectly reflected the concentration of NETs. The results showed that Celastrol produced significant cytotoxicity (average fluorescence intensity 213.2±75.07) at a concentration of 5 µM. No statistical difference was observed in the treatment effect of the stimulant PMA (25 nM) (average fluorescence intensity: 197.3±25.15) (P=0.9167), indicating that neutrophils are more sensitive to triptorubin. Thus, we used lower concentrations of Celastrol (1.25, 0.625, and 0.3125 µM) for the experiment. The results showed that none of the Celastrol concentrations (1.25, 0.625, and 0.3125 µM) showed cytotoxicity when treating neutrophils. Compared with the PMA (25 nM) + Celastrol (1.25, 0.625, and 0.3125 µM) group and the PMA (25 nM) alone group, SytoxGreen showed a statistically significant reduction in fluorescence at 528 µM under 485 µM light excitation, suggesting that Celastrol can inhibit PMA-induced neutrophils to release NETs.
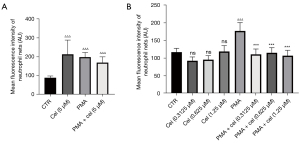
To further determine the effect of Celastrol on PMA-induced neutrophil release of NETs, we used a fluorescence microscope to observe the morphological changes of neutrophils. After applying Hochest to label the nuclei of living cells and SytoxGreen to label the extracellular nucleic acids, the results showed that the release of NETs in the PMA-treated group (under the double-stained Hochest and SytoxGreen co-localization labeling) was higher than that in the control group. The subsequent release of NETs from neutrophils induced by PMA was markedly reduced compared with the PMA treatment group. The release of NETs distinguished under the double staining of the Hochest and SytoxGreen co-localization markers was also notably decreased (Figure 2).
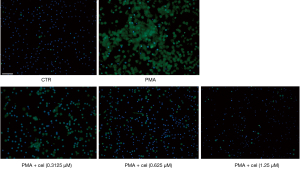
Celastrol inhibits the PMA-induced increase of ROS levels in neutrophils
The experimental results in 2.1 showed that Celastrol had an inhibitory effect on the release of NETs from neutrophils stimulated by PMA, and the increased intracellular ROS level was the promoting factor for the production of NETs. To explore the effect of Celastrol on the intracellular ROS level during the inhibition of NETs formation, we chose the intermediate concentration (0.625 µM). Neutrophils from healthy volunteers were isolated and extracted. After pretreatment with Celastrol, PMA was added to induce neutrophils to produce NETs. After 1 hour, the ROS in the cells were labeled with a DCFH-DA fluorescent dye, and the fluorescence intensity of DCFH was then detected by a multi-functional microplate reader and flow cytometer (Table 1, Figures 3,4). The microplate reader quantitative detection results showed that the fluorescence intensity of the PMA + cel group was significantly lower than that of the PMA alone group, indicating that the level of intracellular ROS was decreased. Interestingly, the treatment group using that received Celastrol alone had lower ROS levels than those in the control group, indicating that Celastrol can inhibit ROS spontaneously produced by neutrophils without stimulation.
Table 1
Group | Control group | Cel (0.625 μM) | PMA | PMA + cel (0.625 μM) |
---|---|---|---|---|
ROS level (RFU) | 188.0±63.56 | 65.67±3.78ΔΔ | 502.7±74.74ΔΔΔ | 326.0±40.63ΔΔ*** |
Note: Data are presented as mean ± standard deviation. Compared with the control group, ΔΔΔ, P<0.001, ΔΔ, P<0.01; compared with PMA group, ***, P<0.001. ROS, reactive oxygen species; Cel, Celastrol; PMA, Phorbol Myristate Acetate; RFU, relative fluorescence unit.
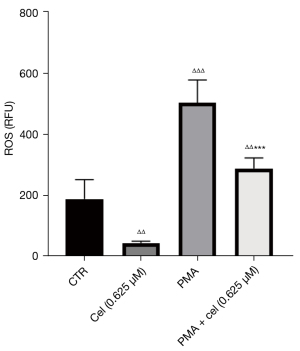
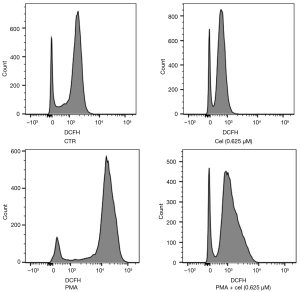
The flow cytometry results showed that the average fluorescence intensity of the PMA + cel group was lower than that of the PMA alone treatment group. Similarly, the average fluorescence of cells in the Celastrol alone treatment group decreased compared with the control group. This is suggested that Celastrol can produce ROS in PMA-stimulated and untreated cells, both of which exert a certain inhibitory effect.
Discussion
In traditional medicine, Tripterygium wilfordii has been used for the treatment of various rheumatoid diseases, such as rheumatoid arthritis, Behcet’s disease, systemic lupus erythematosus, and other diseases. Increasing evidence in the literature demonstrates that Tripterygium wilfordii exhibits an autoimmune arthritis treatment effect in animal experiments (32), and can also treat rheumatoid arthritis in clinical practice. However, its mechanism of action it works is poorly understood, and the specific molecular mechanism is unknown.
Trying to explain the various effects of Tripterygium wilfordii on autoimmune diseases and work out therapeutic drugs for clinical use. Celastrol was found as the active ingredient of Tripterygium wilfordii. With increased research, Tripterygium wilfordii lived up to its expectations and was found to play an important role in a variety of functions, including anti-inflammation, regulation of cell death, and the release of inflammation-related factors.
As a unique substance released by neutrophils, NETs have a potent immunogenic DNA component and other cellular components, such as histones and cellular granules, that are subsequently released (33). DNA binds to DNA receptors such as cyclic GMP-AMP synthase (cGAS) (34) and toll-like receptor 9 (TLR9) both inside and outside cells to activate inflammatory signals to produce inflammatory factors. In sexually-transmitted diseases (35,36), histones play a direct role in various pathogenic processes, including killing cells, activating inflammation, activating platelets, and protecting extracellular DNA components (3), especially in rheumatoid arthritis. Anti-citrullinated protein autoantibodies (ACPAs), which are closely related to NETs in clinical and research studies, are considered to be important signs of rheumatoid arthritis (37). This coincides with the application of Tripterygium wilfordii in traditional medicine, and therefore, there is likely a link between Tripterygium wilfordii and NETs.
In the present study, we first clarified that Celastrol can significantly inhibit the production of NETs. The results showed that the high, medium, and low Celastrol dose groups could significantly inhibit the production of NETs. The immunofluorescence results also demonstrated that after using Celastrol, the NETs produced by PMA stimulation of neutrophils were markedly reduced. Tripterygium wilfordii has different effects on cell death in different diseases. Reports in the literature show that for tumor cells, Tripterygium wilfordii can also increase by inhibiting the nuclear factor kappa-B (NF-κB) pathway. The sensitivity of tumor cells to tumor necrosis factor-α (TNF-α) promotes cell apoptosis (38); however, in various neurodegenerative diseases, heat shock protein 70 production promoted by triptolide achieves the effect of protecting cells (26). Our experiment confirmed that for NETs that are closely related to infection and inflammation, Celastrol also protects neutrophils and prevents cell death. This will, in turn, prevent the release of large numbers of inflammatory factors produced by the death of neutrophils and the exposure of autoantigens carried by neutrophils and provides a new explanation of the mechanism of action of Celastrol in the treatment of various autoimmune diseases.
At present, the specific molecular mechanism of NETs formation is not completely clear. However, the current research suggests that ROS produced by reduced nicotinamide adenine dinucleotide phosphate (NADPH) oxidase is a key factor leading to the rupture of the nuclear membrane and the neutrophil granule membrane, which will eventually lead to cell membrane rupture and NETsrelease (39). Therefore, the importance of ROS generation in the formation and release of NETs is self-evident. Tripterygium wilfordii has been shown to exert a certain inhibitory effect on nerve cell ROS in the treatment of neurodegenerative diseases (27). This effect may be produced by promoting nerve cells to clear senescent mitochondria through autophagy (40).
Celastrol have already find inhibits inflammatory stimuli-induced NETs (41). But different from classic NETs stimulant——PMA, inflammatory stimuli may from NETs in different mechanism.
In the subsequent experiment, we determined the effect of Celastrol on the ROS of neutrophils. The results showed that at a concentration of 0.625 µM, Celastrol down-regulated the intracellular ROS level of neutrophils, which may explain the decrease in NETs formation. At the same time, we also found that Celastrol at the same concentration also inhibited the spontaneous increase in the ROS levels of neutrophils, suggesting that Celastrol may have a certain cytoprotective effect, as elevated ROS levels can activate calcium ion transcription. The signal transduction pathway of neutrophils is an important molecule in the formation of NETs, and thus, we have reason to think that the inhibition of NETs production by Celastrol is related to its inhibition of intracellular ROS levels. In summary, our experimental results provide a certain theoretical basis for Celastrol inhibition of NETs production by neutrophils and provide a theory on the molecular mechanism of Tripterygium wilfordii on neutrophils to guide future research.
Conclusions
Previous studies have demonstrated the close relationship between NETs and inflammation (42). The formation of NETs can induce a series of diseases, including rheumatoid diseases (43), asthma (44), and thrombosis (45). In general, our experimental results confirmed that the main active ingredient of Tripterygium wilfordii inhibits the formation of NETs by down-regulating the levels of ROS in neutrophils. This explains the action of Tripterygium wilfordii in the traditional medical treatment of rheumatic immune diseases, and also provides some guidance for the clinical treatment of NETs-related diseases by Tripterygium wilfordii in the future.
Acknowledgments
Funding: None.
Footnote
Reporting Checklist: The authors have completed the MDAR reporting checklist. Available at https://atm.amegroups.com/article/view/10.21037/atm-22-5720/rc
Data Sharing Statement: Available at https://atm.amegroups.com/article/view/10.21037/atm-22-5720/dss
Conflicts of Interest: All authors have completed the ICMJE uniform disclosure form (available at https://atm.amegroups.com/article/view/10.21037/atm-22-5720/coif). The authors have no conflicts of interest to declare.
Ethical Statement: The authors are accountable for all aspects of the work in ensuring that questions related to the accuracy or integrity of any part of the work are appropriately investigated and resolved. All patients who participated in this study signed an informed consent form, and the study was approved by the Ethics Committee of The Third Affiliated Hospital of Southern Medical University (No. 2022-Lunshen-005), and was conducted in accordance with the Declaration of Helsinki (as revised in 2013).
Open Access Statement: This is an Open Access article distributed in accordance with the Creative Commons Attribution-NonCommercial-NoDerivs 4.0 International License (CC BY-NC-ND 4.0), which permits the non-commercial replication and distribution of the article with the strict proviso that no changes or edits are made and the original work is properly cited (including links to both the formal publication through the relevant DOI and the license). See: https://creativecommons.org/licenses/by-nc-nd/4.0/.
References
- Fan D, Guo Q, Shen J, et al. The Effect of Triptolide in Rheumatoid Arthritis: From Basic Research towards Clinical Translation. Int J Mol Sci 2018;19:376. [Crossref] [PubMed]
- Hou ZY, Tong XP, Peng YB, et al. Broad targeting of triptolide to resistance and sensitization for cancer therapy. Biomed Pharmacother 2018;104:771-80. [Crossref] [PubMed]
- Wei YM, Wang YH, Xue HQ, et al. Triptolide, A Potential Autophagy Modulator. Chin J Integr Med 2019;25:233-40. [Crossref] [PubMed]
- Chen M, Ni Y, Duan H, et al. Mass spectrometry-based metabolic profiling of rat urine associated with general toxicity induced by the multiglycoside of Tripterygium wilfordii Hook. f. Chem Res Toxicol 2008;21:288-94. [Crossref] [PubMed]
- Gong T, Tan T, Zhang P, et al. Palmitic acid-modified bovine serum albumin nanoparticles target scavenger receptor-A on activated macrophages to treat rheumatoid arthritis. Biomaterials 2020;258:120296. [Crossref] [PubMed]
- Jiang X, Chen S, Zhang Q, et al. Celastrol is a novel selective agonist of cannabinoid receptor 2 with anti-inflammatory and anti-fibrotic activity in a mouse model of systemic sclerosis. Phytomedicine 2020;67:153160. [Crossref] [PubMed]
- Li R, Li Y, Zhang J, et al. Targeted delivery of celastrol to renal interstitial myofibroblasts using fibronectin-binding liposomes attenuates renal fibrosis and reduces systemic toxicity. J Control Release 2020;320:32-44. [Crossref] [PubMed]
- Brinkmann V, Reichard U, Goosmann C, et al. Neutrophil extracellular traps kill bacteria. Science 2004;303:1532-5. [Crossref] [PubMed]
- Branzk N, Lubojemska A, Hardison SE, et al. Neutrophils sense microbe size and selectively release neutrophil extracellular traps in response to large pathogens. Nat Immunol 2014;15:1017-25. [Crossref] [PubMed]
- Hahn J, Schauer C, Czegley C, et al. Aggregated neutrophil extracellular traps resolve inflammation by proteolysis of cytokines and chemokines and protection from antiproteases. FASEB J 2019;33:1401-14. [Crossref] [PubMed]
- Demkow U. Neutrophil Extracellular Traps (NETs) in Cancer Invasion, Evasion and Metastasis. Cancers (Basel) 2021;13:4495. [Crossref] [PubMed]
- Hirsch JG. Bactericidal action of histone. J Exp Med 1958;108:925-44. [Crossref] [PubMed]
- Allam R, Kumar SV, Darisipudi MN, et al. Extracellular histones in tissue injury and inflammation. J Mol Med (Berl) 2014;92:465-72. [Crossref] [PubMed]
- Dwivedi N, Radic M. Citrullination of autoantigens implicates NETosis in the induction of autoimmunity. Ann Rheum Dis 2014;73:483-91. [Crossref] [PubMed]
- Schreiber A, Rousselle A, Becker JU, et al. Necroptosis controls NET generation and mediates complement activation, endothelial damage, and autoimmune vasculitis. Proc Natl Acad Sci U S A 2017;114:E9618-25. [Crossref] [PubMed]
- Skopelja-Gardner S, Jones JD, Rigby WFC. "NETtling" the host: Breaking of tolerance in chronic inflammation and chronic infection. J Autoimmun 2018;88:1-10. [Crossref] [PubMed]
- Jorch SK, Kubes P. An emerging role for neutrophil extracellular traps in noninfectious disease. Nat Med 2017;23:279-87. [Crossref] [PubMed]
- Lee KH, Kronbichler A, Park DD, et al. Neutrophil extracellular traps (NETs) in autoimmune diseases: A comprehensive review. Autoimmun Rev 2017;16:1160-73. [Crossref] [PubMed]
- Camelio AM, Johnson TC, Siegel D. Total Synthesis of Celastrol, Development of a Platform to Access Celastroid Natural Products. J Am Chem Soc 2015;137:11864-7. [Crossref] [PubMed]
- Lim HY, Ong PS, Wang L, et al. Celastrol in cancer therapy: Recent developments, challenges and prospects. Cancer Lett 2021;521:252-67. [Crossref] [PubMed]
- Xu S, Feng Y, He W, et al. Celastrol in metabolic diseases: Progress and application prospects. Pharmacol Res 2021;167:105572. [Crossref] [PubMed]
- Liu D, Zhang Q, Luo P, et al. Neuroprotective Effects of Celastrol in Neurodegenerative Diseases-Unscramble Its Major Mechanisms of Action and Targets. Aging Dis 2022;13:815-36. [Crossref] [PubMed]
- He MF, Liu L, Ge W, et al. Antiangiogenic activity of Tripterygium wilfordii and its terpenoids. J Ethnopharmacol 2009;121:61-8. [Crossref] [PubMed]
- Dai Y, DeSano JT, Meng Y, et al. Celastrol potentiates radiotherapy by impairment of DNA damage processing in human prostate cancer. Int J Radiat Oncol Biol Phys 2009;74:1217-25. [Crossref] [PubMed]
- Aqil F, Kausar H, Agrawal AK, et al. Exosomal formulation enhances therapeutic response of celastrol against lung cancer. Exp Mol Pathol 2016;101:12-21. [Crossref] [PubMed]
- Zhao Y, Zhao H, Lobo N, et al. Celastrol enhances cell viability and inhibits amyloid-β production induced by lipopolysaccharide in vitro. J Alzheimers Dis 2014;41:835-44. [Crossref] [PubMed]
- Deng YN, Shi J, Liu J, et al. Celastrol protects human neuroblastoma SH-SY5Y cells from rotenone-induced injury through induction of autophagy. Neurochem Int 2013;63:1-9. [Crossref] [PubMed]
- Pinna GF, Fiorucci M, Reimund JM, et al. Celastrol inhibits pro-inflammatory cytokine secretion in Crohn's disease biopsies. Biochem Biophys Res Commun 2004;322:778-86. [Crossref] [PubMed]
- Cascão R, Vidal B, Raquel H, et al. Effective treatment of rat adjuvant-induced arthritis by celastrol. Autoimmun Rev 2012;11:856-62. [Crossref] [PubMed]
- Jia Z, Xu C, Shen J, et al. The natural compound celastrol inhibits necroptosis and alleviates ulcerative colitis in mice. Int Immunopharmacol 2015;29:552-9. [Crossref] [PubMed]
- Li H, Zhang YY, Huang XY, et al. Beneficial effect of tripterine on systemic lupus erythematosus induced by active chromatin in BALB/c mice. Eur J Pharmacol 2005;512:231-7. [Crossref] [PubMed]
- Venkatesha SH, Yu H, Rajaiah R, et al. Celastrus-derived celastrol suppresses autoimmune arthritis by modulating antigen-induced cellular and humoral effector responses. J Biol Chem 2011;286:15138-46. [Crossref] [PubMed]
- Selders GS, Fetz AE, Radic MZ, et al. An overview of the role of neutrophils in innate immunity, inflammation and host-biomaterial integration. Regen Biomater 2017;4:55-68. [Crossref] [PubMed]
- Chan YK, Gack MU. Viral evasion of intracellular DNA and RNA sensing. Nat Rev Microbiol 2016;14:360-73. [Crossref] [PubMed]
- Villanueva E, Yalavarthi S, Berthier CC, et al. Netting neutrophils induce endothelial damage, infiltrate tissues, and expose immunostimulatory molecules in systemic lupus erythematosus. J Immunol 2011;187:538-52. [Crossref] [PubMed]
- Kegerreis BJ, Catalina MD, Geraci NS, et al. Genomic Identification of Low-Density Granulocytes and Analysis of Their Role in the Pathogenesis of Systemic Lupus Erythematosus. J Immunol 2019;202:3309-17. [Crossref] [PubMed]
- Khandpur R, Carmona-Rivera C, Vivekanandan-Giri A, et al. NETs are a source of citrullinated autoantigens and stimulate inflammatory responses in rheumatoid arthritis. Sci Transl Med 2013;5:178ra40. [Crossref] [PubMed]
- Tozawa K, Sagawa M, Kizaki M. Quinone methide tripterine, celastrol, induces apoptosis in human myeloma cells via NF-κB pathway. Int J Oncol 2011;39:1117-22. [PubMed]
- Fuchs TA, Abed U, Goosmann C, et al. Novel cell death program leads to neutrophil extracellular traps. J Cell Biol 2007;176:231-41. [Crossref] [PubMed]
- Zhang L, Dong Y, Xu X, et al. The role of autophagy in Parkinson's disease. Neural Regen Res 2012;7:141-5. [PubMed]
- Yu Y, Koehn CD, Yue Y, et al. Celastrol inhibits inflammatory stimuli-induced neutrophil extracellular trap formation. Curr Mol Med 2015;15:401-10. [Crossref] [PubMed]
- Castanheira FVS, Kubes P. Neutrophils and NETs in modulating acute and chronic inflammation. Blood 2019;133:2178-85. [Crossref] [PubMed]
- Apel F, Zychlinsky A, Kenny EF. The role of neutrophil extracellular traps in rheumatic diseases. Nat Rev Rheumatol 2018;14:467-75. [Crossref] [PubMed]
- Lachowicz-Scroggins ME, Dunican EM, Charbit AR, et al. Extracellular DNA, Neutrophil Extracellular Traps, and Inflammasome Activation in Severe Asthma. Am J Respir Crit Care Med 2019;199:1076-85. [Crossref] [PubMed]
- Kimball AS, Obi AT, Diaz JA, et al. The Emerging Role of NETs in Venous Thrombosis and Immunothrombosis. Front Immunol 2016;7:236. [Crossref] [PubMed]
(English Language Editor: A. Kassem)