The expression and clinical significance of miRNA-183 in cerebral ischemia-reperfusion injury patients with cerebral small vessel disease
Introduction
In recent years, there has been a significant increase in the prevalence of cerebral small vessel disease (CSVD), with cerebral ischemia-reperfusion injury (CIRI) being a major physiological process that occurs during disease onset and subsequent treatment (1). CSVD patients may choose artery thrombolysis or mechanical thrombectomy as treatment in the early stage of the disease. However, regardless of the type of treatment, the rate of vascular recanalization after cerebral ischemia can be increased. If blood perfusion is restored at this time, the patient will face the possibility of increased tissue damage, resulting in cerebral edema, cerebral hemorrhage and neuron injury, leading to CIRI (2).
The first recognition of ischemia-reperfusion injury came from cardiopulmonary bypass cardiac surgery. In the 1950s and 1960s, cardiac surgeons started open-heart surgery with cardiopulmonary bypass and found that some patients had myocardial injury after surgery, and the presence of ischemia-reperfusion injury was gradually discovered (3). Subsequently, other human organs have been found to be involved in ischemia-reperfusion injury after blood oxygen recovery by ischemic treatment. Among these, cerebral infarction has been discovered to also be complicated by ischemia-reperfusion injury after thrombolysis, and has been studied deeply by neuroscientists (4). CIRI is associated with microvascular dysfunction and inflammation caused by microvascular dysfunction. When cerebral ischemia reperfusion, cerebral vascular endothelial cells, platelets, and immune cells release a significant amount of promoting inflammatory cell factors, including interleukin-6 (IL-6), IL-8, IL-1β, tumor necrosis factor-α (TNF-α), and multiple inflammatory cells, this induces an inflammatory reaction, and a variety of pathologic and physiological factors combine to result in CIRI (5).
MicroRNA (miRNA) biological function is the important theoretical basis informing the formation of the endogenous competitive RNA molecule network. Many studies have found that the miRNA can affect cell growth, organ function, and regulation of apparent function and that cancer cells can substantially disrupt many physiological and pathological processes. Furthermore, miRNA concentration or miRNA sequence changes can affect normal cell activity. Brain tissue contains more miRNA types and higher expression levels of miRNA than other organs, and there are seven brain tissue–specific miRNAs in mice and human brains. Among these, miR-183 belongs to a member of the miR-183 gene cluster and has highly conserved characteristics in different species, which can be traced back to 600 million years ago (6). Abnormal expression of miR-183 in non-sensory organs, especially in some malignant tumors, can significantly aggravate the progression of cancer. The level of miR-183 in human tissues and body fluids shows a stable trend of increase and decrease, and it can be used as an independent or auxiliary biomarker for the diagnosis, recurrence, and prognosis monitoring of certain diseases. Currently, the expression and function of miR-183 have been studied in many fields, including in sensory organs, the immune system, lymphatic vessels and bones, cell proliferation and migration after nerve injury, etc., but few studies have examined the function of miR-183 in CIRI (7).
Imaging technology has enabled the relatively clear presentation of ischemic brain tissue, microcirculation disorder, blood-brain barrier (BBB) destruction, vasogenic brain edema, cerebral hemorrhage, and other reperfusion injury lesions. The presence of hemorrhagic foci after CIRI has been acknowledged as a clinically important problem. Therefore, it is essential to find a simple and reliable evaluation technique for determining the perfusion of ischemic foci and the state of reperfusion injury (8). Of the imaging methods available, emission computed tomography (ECT) is time-consuming, requires the use of radioactive tracers in imaging, and provides only a low spatial resolution of the image. Another method, CT perfusion, needs to be applied several times, and repeated use of CTP can increase the X-ray radiation dose, causing poor diagnosis of small hemorrhagic foci under the tentorium and near the skull base. On the other hand, magnetic resonance imaging (MRI) has multiple imaging parameters with an abundant amount of different imaging sequences being available at the same time. Thus, MRI has great potential value for the comprehensive detection of CIRI and has already demonstrated relatively clear imaging for this purpose (9). Additionally, the resolution of craniocerebral MRI is much higher than that of CT, can be used to image any plane, and can show the grey and white matter of the brain more clearly without bone artifact. Furthermore, MRI has a strong diagnostic ability for diseases of sella, inner auditory canal, and posterior fossa, and is far superior in showing structural lesions of the skull base (10). Therefore, our study aimed to analyze the cranial MRI findings and serum miRNA-183 in CIRI patients with CSVD, in order to provide predictive indicators for the prevention of CIRI patients with cerebral vascular disease.
We present the following article in accordance with the MDAR reporting checklist (available at http://dx.doi.org/10.21037/atm-20-5335).
Methods
General information
The CIRI group consisted of 138 CSVD patients with concurrent CIRI aged 60–75 years old who had been admitted to Second Affiliated Hospital, University of South China from May 2018 to May 2019, most of whom came from Hengyang, Hunan Province. This group included 70 males and 68 females, and the average age was 65.12±6.35. The control group, which consisted of healthy volunteers with no abnormalities on MRI, totaled 60 cases, including 31 males and 29 females, with an average age of 65.21±6.17. The patients’ general information was recorded, and there was no statistically significant differences between the groups according to the general data records (P<0.05). The study was conducted in accordance with the Declaration of Helsinki (as revised in 2013) and was approved by the Medical Ethics Committee of University of South China (4304079008946). Written informed consent was obtained from all patients.
Inclusion criteria and exclusion criteria
The inclusion criteria were the following: patients in the CIRI group needed to meet the diagnostic criteria as delineated by the Chinese expert consensus on the diagnosis and treatment of cerebral vascular disease (10); CIRI cases needed to be treated with thrombolytic therapy, with no abnormal communication being observed; patients needed to sign informed consent. Patients were excluded if they had a MRI lesion diameter over 20 mm; a history of intracranial arterial stenosis or intracranial hemorrhage; cerebral hemorrhage; a cerebral embolism type that was cardiogenic; blood disease; tumors; or severe liver, kidney, heart, or lung damage.
Serum miRNA-183 expression detection by quantitative real-time polymerase chain (RT-qPCR)
Early in the morning, 4 mL of blood was drawn from patients who had an empty stomach. Samples were centrifuged at 2,500 r/min, centrifuged for 10 min, and then serum was collected from the centrifugal tube. Serum total RNA was extracted with a whole blood RNA extraction kit (AM1836, Thermo Fisher, USA). Total RNA was reverse transcribed into cDNA, using DNA fluorescent SYBRGreenIto test the expression level of miRNA-183, with U6 as the reference gene, in 40 cycles at 60 °C for 10 min, at 95 °C and 72 °C for 30 s, and at 95 °C for 5 min. Experiments were repeated three times, using 2−ΔΔCT expression of miR-183 for calculations. The primer sequences are shown in Table 1.
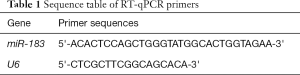
Full table
Enzyme-linked immunosorbent assay (ELISA) methods
The whole blood of each group of patients was collected with heparin for anticoagulation treatment, mixed and placed on ice, centrifuged at 4 °C at about 1,000–2,000 g for 10 minutes, and the light yellow supernatant was taken to obtain plasma. Using an ELISA kit (Beyotime Biotechnology Co., Ltd., China), the levels of TNF-α, IL-18, IL-1β and IL-6 in the supernatant were determined according to the instructions. Add samples or standards of different concentrations to the corresponding wells at 100 µL/well, seal the reaction wells with sealing film, incubate at room temperature for 120 minutes, wash the plate 5 times; add 100 µL/well of biotinylated antibody, and seal with sealing film Hold the reaction well, incubate at room temperature for 60 minutes, wash the plate 5 times; add horseradish peroxidase labeled Streptavidin 100 µL/well. Seal the reaction wells with a sealing film, and incubate for 20 minutes at room temperature in the dark. After incubation, wash the plate 5 times; add 100 µL/well of the developer TMB solution, seal the reaction wells with a sealing film (white), and incubate at room temperature away from light for 20 minute. Add 50 µL/well of stop solution, and measure the absorbance value at 450 nm after mixing.
Cerebral MRI
A Philips Achieva MRI detector (3.0T) was used for MRI imaging, the baseline was the orbitomeatal line (OM line), and the brain stem, cerebellar hemisphere, basal ganglia, lateral ventricular body, parietal cortex, and subcortical layers were scanned, ranging from the intracarotid artery position to the upper part of the corpus callosum artery. The maximum density projection treatment was applied to the patient. The front and back positions were rotated to the left and around. The degree of rotation was 360°, and an image frame was acquired at 19° intervals.
Statistical analysis
Epidata3.10 and SPSS22.0 software were used to analyze the levels of miRNA-183, TNF-α, IL-6, IL-8, and IL-1β in the CIRI group and control group. The variables are expressed by
Results
Craniocerebral MRI examination
As shown in Figure 1, MRI monitoring revealed that patients in the CIRI group had multiple microbleeds outside the infarct in the brain and in the infarct area. The veins in the infarct area were significantly reduced or had disappeared on the contralateral side, and the veins had increased and thickened to varying degrees. T1W1 appeared as a moderately high signal in the low signal area, and T2W1 appeared as a low signal in the high signal area.
The changes of miR-183 levels in peripheral venous blood in each group
Peripheral blood samples were collected from 138 patients with CIRI and 60 healthy volunteers, and the expression of miR-183 in serum was detected by RT-qPCR. As shown in Figure 2, compared with the control group, the expression level of miR-183 in the peripheral blood of patients with CIRI was significantly higher (P<0.01).
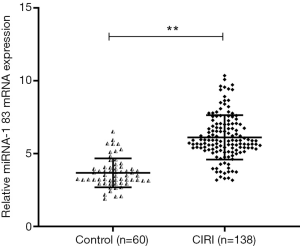
The expression of TNF-α, IL-6, IL-8, and IL-1β in serum was upregulated
We used ELISA to detect the expression of inflammatory factors in serum. Compared with the control group, the serum levels of TNF-α, IL-6, IL-8, and IL-1β in the CIRI group were significantly higher (P<0.05) (Figure 3).
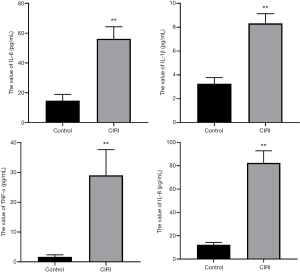
Pearson’s correlation analysis
Figure 4 shows the results of Pearson’s correlation analysis. The level of serum miRNA-183 in the CIRI group was positively correlated with the increase of imaging lesions (r=0.758, P<0.0001). R2=0.57 indicates that the regression relationship can explain the 57% variation of the dependent variable.
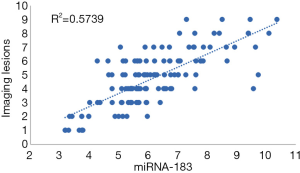
Discussion
Cerebral vascular disease has the characteristics of high morbidity, mortality, and recurrence rate, and most frequently occurs in its ischemic form. Reperfusion of blood flow and restoration of blood oxygen supply are still the main principles of current clinical treatment (11). Although this kind of treatment can achieve occlusive cerebral vascular recanalization, it tends to further or irreversibly aggravate the pathological damage of ischemic tissue and worsen the clinical symptoms, which is known as CIRI. Current studies suggest that CIRI injury is closely related to excitatory amino acid toxicity, intracellular Ca2 overload, excessive generation of free radicals, and leukocyte-induced inflammatory response and cell apoptosis (12). However, the cytokines-induced by free radicals are also closely associated with CIRI. Free radical-induced adhesion molecules also play an important role in the development of reperfusion injury. Lipid, protein, and nucleic acid peroxidation are also caused by free radicals, which indirectly damage the membrane structure and causes protein degradation, destroying the nucleic acid backbone. This causes hyaluronic acid hydrolysis polymerization, cell disintegration, and mitochondrial degeneration, leading to irreversible changes in cells and eventual death. Free radicals and other messengers can stimulate ischemic cells to secrete inflammatory cytokines and chemokines, thus inducing the aggregation and infiltration of white blood cells in the cerebral ischemia site, which will aggravate the inflammatory response in the damaged site and form a vicious inflammatory response cycle (13). Patients are often not aware of the poor condition of their own disease, so the diagnosis of CIRI must rely on brain imaging diagnosis and differentiation. MRI is critical for the diagnosis of the vascular state of brain tissue after reperfusion of the brain in CVSD (14). In the present study, patients in the CIRI group had multiple microbleeds outside the infarct in the brain and in the infarct area. The veins in the infarct area were significantly reduced or disappeared from the contralateral side, and the veins increased and thickened to varying degrees. T1W1 appeared as a moderately high signal in the low signal area, and T2W1 appeared as a low signal in the high signal area, which suggests that MRI could detect early abnormal perfusion in the local brain tissue. MRI is widely used in brain diagnosis, and it is sensitive to the detection of cerebral apoplexy with microbleeds and arterial thrombosis. It can clearly display cerebral venules and sensitive detection of microbleeds. It has potential application value in the diagnosis and evaluation of CIRI (7).
Increasing attention has been paid to the application of miRNA in clinical practice. The brain tissue is rich in miRNA, with more types and higher expression levels of miRNA than in other organs. The expression of miRNA in the brain is closely regulated, and the expression of miRNA has fixed sequences and regions during the development of the brain (15). When the body is geomagnetic, miR-183 exists in sense organs, and when cancer, neurological disorders, or autoimmune disorders occur, miR-183 has a higher expression in the non-sensory organs. In etiology research and target therapy, the use miR-183 and some associated factors has gradually increased for the diagnosis, prevention and treatment of CIRI (16). miR-183 also exhibits specific expression in the human and mouse cerebral cortex, affecting the development of the cerebral cortex and regulating the differentiation of nerve cells. For example, after dorsal root ganglion injury in adult rats, the expression of miR-183 was significantly decreased, and its distribution was closely related to stress granule (SG) protein, suggesting that the regulation of chronic neuralgia by miR-183 could be realized through pain-related genes (17). Yuan et al. (18) found that miR-183-5p increased in the hippocampus under chronic and persistent mild mental stress, indicating that the increased miR-183-5p level was associated with stress-induced depression. Meanwhile, miR-183 was demonstrated to interfere with glucose and lipid metabolism, and its abnormal expression has been related to metabolic diseases like obesity and diabetes, and it has been shown to induce cerebrovascular diseases, such as atherosclerosis (19). In this study, RT-qPCR detection found that, compared with the control group, the level of miR-183 in peripheral blood of patients with CIRI was significantly higher than that of the control group. Meanwhile, the level of serum miR-183 in the CIRI group was positively correlated with the increase of imaging lesions. These results confirm that miR-183 is involved in the occurrence and development of ischemia-reperfusion. MiR-183 can inhibit the activation of microglia and the expression of inflammatory factors through NF-κ-signaling pathway (20). Down regulation of miR-451 can promote angiogenesis by targeting macrophage migration inhibitory factor in hypoxic human umbilical vein endothelial cells, indicating that miR-451 is a potential candidate for the treatment of cerebral ischemia injury (21). SNHG1 targets miR-199a and up regulates SIRT1 expression, thus alleviating cerebral ischemia/reperfusion injury through AMPK pathway activation (22).
The inflammatory response also participates in the injury stage of cerebral ischemia-reperfusion. The aggregation and infiltration of neutrophils are particularly critical in the inflammatory response stage. The inflammatory response generates a release of various cytokines activated by vascular endothelial cells, platelets, and immune cells. During the progression of ischemia-reperfusion, inflammatory cells produce various cytokines, such as TNF-α, IL-1β, IL-6, IL-8, and others (23). In this study, we found that TNF-α, IL-6, IL-8, and IL-1β levels of CIRI patients increased compared with the those of control group, which confirmed the mechanism of vascular endothelial cell inflammatory injury in CIRI patients. We also revealed that the occurrence of CIRI was closely correlated to the expression levels of inflammatory cytokines, and that IL-1β could regulate the immune response produced in macrophages, T cells, B cells and other cells. This in turn increases the release of cell adhesion molecule-1, leading to improved adhesion of white blood cells and aggravating the inflammatory response and the nerve damage. TNF-α can stimulate the production of macrophages, resulting in an increased inflammatory response in the body and a more severe condition. The inflammatory cytokines produced by macrophages need the assistance of IL-1β, and both inflammatory cytokines are highly expressed and aggravate local inflammation. IL-8 is a cytokine that plays a chemotactic role in the body’s immune response and stress response, and the aggregation of inflammatory cells in the infarct area is intensified by the combined action of the above inflammatory factors (24). Studies have found that anti-IL-8 antibody can intervene in tissue damage caused by neutrophils and reduce the infarct area, suggesting that anti-IL-8 antibody may be a key measure in preventing tissue damage and inflammatory response. Zhou et al. (25) found scalp acupuncture can make the inflammatory cytokines TNF-α and IL-1β levels drop, and that IL-1β obvious inhibited leukocyte infiltration after cerebral ischemia reperfusion. Scalp acupuncture can protect the brain tissue injured by CIRI, accelerate the recovery of neural function after cerebral ischemia, and rebuild structural damage in nerve tissue. Deng tested TNF-α and IL-6 in a model and sham operation group by establishing a large CIRI model, and found that the levels of TNF-α and IL-6 in the model group were higher than those in the sham operation group. After the treatment with an alcohol extract of Radix Morindae Officinalis, the expressions of TNF-α and IL-6 were significantly reduced, which had a significantly protective effect on CIRI in rats. At present, the role and mechanism of miR-183 in CIRI has not been deeply studied. In addition to the inflammatory response, miR-183 is closely related to CIRI. Thus to clarify the diversity of expression of miR-183 in CSVD CIRI, a multi-direction study was carried out on miR-183 to provide a new research basis for the prevention and treatment of CIRI.
Conclusions
CIRI injury in CSVD patients is a complex process with multiple factors, one of these being miR-183, which plays a role in the occurrence, development, and prognosis of this condition. miR-183, TNF-α, IL-1β, IL-6, IL-8, and other indicators can be easily obtained to screen for CIRI in CSVD patients. In combination with brain imaging examination, the correlation between each indicator and CIRI in CSVD patients can be determined. Further study on miR-183 may likely yield new approaches and methods for the prevention and treatment of CSVD.
Acknowledgments
Funding: None.
Footnote
Reporting Checklist: The authors have completed the MDAR reporting checklist. Available at http://dx.doi.org/10.21037/atm-20-5335
Data Sharing Statement: Available at http://dx.doi.org/10.21037/atm-20-5335
Conflicts of Interest: All authors have completed the ICMJE uniform disclosure form (available at http://dx.doi.org/10.21037/atm-20-5335). The authors have no conflicts of interest to declare.
Ethical Statement: The authors are accountable for all aspects of the work in ensuring that questions related to the accuracy or integrity of any part of the work are appropriately investigated and resolved. The study was conducted in accordance with the Declaration of Helsinki (as revised in 2013) and was approved by the Medical Ethics Committee of University of South China (4304079008946). Written informed consent was obtained from all patients.
Open Access Statement: This is an Open Access article distributed in accordance with the Creative Commons Attribution-NonCommercial-NoDerivs 4.0 International License (CC BY-NC-ND 4.0), which permits the non-commercial replication and distribution of the article with the strict proviso that no changes or edits are made and the original work is properly cited (including links to both the formal publication through the relevant DOI and the license). See: https://creativecommons.org/licenses/by-nc-nd/4.0/.
References
- Memet O, Zhang L, Shen J. Serological biomarkers for acute mesenteric ischemia. Ann Transl Med 2019;7:394. [Crossref] [PubMed]
- Ravindran S, Kurian GA. Addressing the alterations in cerebral ischemia-reperfusion injury on the brain mitochondrial activity: A possible link to cognitive decline. Biochem Biophys Res Commun 2019;518:100-6. [Crossref] [PubMed]
- Wang M, Niu A, Gong Z, et al. PEG-amino acid-przewaquinone a conjugations: Synthesis, physicochemical properties and protective effect in a rat model of brain ischemia-reperfusion. Bioorg Med Chem Lett 2020;30:126780. [Crossref] [PubMed]
- Hausburg MA, Banton KL, Roman PE, et al. Effects of propofol on ischemia-reperfusion and traumatic brain injury. J Crit Care 2020;56:281-7. [Crossref] [PubMed]
- Qu XY, Zhang YM, Tao LN, et al. XingNaoJing injections protect against cerebral ischemia/reperfusion injury and alleviate blood-brain barrier disruption in rats, through an underlying mechanism of NLRP3 inflammasomes suppression. Chin J Nat Med 2019;17:498-505. [PubMed]
- Luo P, Fu X, Chang M, et al. Cerebral ischemia-reperfusion causes a down regulation of HCN1 expression via enhancing the nuclear NRSF-HDAC4 gathering that contributes to neuron damage. Brain Res Bull 2020;156:50-7. [Crossref] [PubMed]
- Yang S, Krug SM, Heitmann J, et al. Analgesic drug delivery via recombinant tissue plasminogen activator and microRNA-183-triggered opening of the blood-nerve barrier. Biomaterials 2016;82:20-33. [Crossref] [PubMed]
- Li HY, Li P, Yang HG, et al. Investigation and comparison of the protective activities of three functional proteins-lactoferrin, α-lactalbumin, and β-lactoglobulin-in cerebral ischemia reperfusion injury. J Dairy Sci 2020;103:4895-906. [Crossref] [PubMed]
- Abdel-Rahman RF, Alqasoumi SI, Ogaly HA, et al. Propolis ameliorates cerebral injury in focal cerebral ischemia/reperfusion (I/R) rat model via upregulation of TGF-β1. Saudi Pharm J 2020;28:116-26. [Crossref] [PubMed]
- Expert consensus on diagnosis and treatment of cerebrovascular disease. Chinese Journal for Clinicians 2014;42:84-7+90.
- Xu F, Ma R, Zhang G, et al. Estrogen and propofol combination therapy inhibits endoplasmic reticulum stress and remarkably attenuates cerebral ischemia-reperfusion injury and OGD injury in hippocampus. Biomed Pharmacother 2018;108:1596-606. [Crossref] [PubMed]
- Singaravelu R, Ahmed N, Quan C, et al. A conserved miRNA-183 cluster regulates the innate antiviral response. J Biol Chem 2019;294:19785-94. [Crossref] [PubMed]
- Lin CC, Tsai MC, Lee CT, et al. Antidepressant treatment increased serum miR-183 and miR-212 levels in patients with major depressive disorder. Psychiatry Res 2018;270:232-7. [Crossref] [PubMed]
- Thiel J, Alter C, Luppus S, et al. MicroRNA-183 and microRNA-96 are associated with autoimmune responses by regulating T cell activation. J Autoimmun 2019;96:94-103. [Crossref] [PubMed]
- Zhou M. Experimental study on the expression of microRNA in hippocampus of unforeseeable chronic mild stress rat model. Southwest Medical University Luzhou Medical College, 2014.
- Mozaffari Godarzi S, Valizade Gorji A, et al. Antioxidant effect of p-coumaric acid on interleukin 1-β and tumor necrosis factor-α in rats with renal ischemic reperfusion. Nefrologia 2020;40:311-9. [Crossref] [PubMed]
- Jin YC, Kim CW, Kim YM, et al. Cryptotanshinone, a lipophilic compound of Salvia miltiorrriza root, inhibits TNF-alpha-induced expression of adhesion molecules in HUVEC and attenuates rat myocardial ischemia/reperfusion injury in vivo. Eur J Pharmacol 2009;614:91-7. [Crossref] [PubMed]
- Yuan LJ, Zhao L, Ling CY, et al. Effects of Propolis Flavonoids on Cerebral IL-1β,IL-6 and TNF-α in Rats Experienced Focal Cerebral Ischemia/Reperfusion. Chinese Journal of Comparative Medicine 2008;(08):11-3+88.
- Amantea D, Certo M, Russo R, et al. Early reperfusion injury is associated to MMP2 and IL-1beta elevation in cortical neurons of rats subjected to middle cerebral artery occlusion. Neuroscience 2014;277:755-63. [Crossref] [PubMed]
- Xiang B, Zhong P, Fang L, et al. miR-183 inhibits microglia activation and expression of inflammatory factors in rats with cerebral ischemia reperfusion via NF-kappaB signaling pathway. Exp Ther Med 2019;18:2540-6. [PubMed]
- Li Q, Li Y, Zhang D, et al. Downregulation of microRNA451 improves cell migration, invasion and tube formation in hypoxiatreated HUVECs by targeting MIF. Mol Med Rep 2019;20:1167-77. [PubMed]
- Yin WL, Yin WG, Huang BS, et al. LncRNA SNHG12 inhibits miR-199a to upregulate SIRT1 to attenuate cerebral ischemia/reperfusion injury through activating AMPK signaling pathway. Neurosci Lett 2019;690:188-95. [Crossref] [PubMed]
- Di Paola R, Genovese T, Impellizzeri D, et al. The renal injury and inflammation caused by ischemia-reperfusion are reduced by genetic inhibition of TNF-αR1: a comparison with infliximab treatment. Eur J Pharmacol 2013;700:134-46. [Crossref] [PubMed]
- Wei L, Li J, Han Z, et al. Silencing of lncRNA MALAT1 Prevents Inflammatory Injury after Lung Transplant Ischemia-Reperfusion by Downregulation of IL-8 via p300. Mol Ther Nucleic Acids 2019;18:285-97. [Crossref] [PubMed]
- Zhou L, Zhang HX, Liu LG, et al. Effect of scalp-acupuncture on plasma and cerebral TNF-alpha and IL-1beta contents in acute cerebral ischemia/reperfusion injury rats. Zhen Ci Yan Jiu 2008;33:173-8. [PubMed]
(English Language Editor: J. Gray)