Abstract
Reduced graphene oxide (rGO) dispersion was obtained by ultrasonication of rGO powder using dodecanethiol (-SH) as an exfoliating agent. Using thiol chemistry, the gold nanoparticles (Au-NPs) were assembled onto the rGO-SH modified glassy carbon electrode (GCE) which showed a strong binding with the surface of the coated electrode. Furthermore, the GCE/rGO-SH/Au-NPs electrode was used to detect mercury (Hg2+) ions in the aqueous solution. When employed as a working electrode, Hg2+ ions get adsorbed on the electrode surface which was later electrochemically oxidized by differential pulse voltammetry (DPV) with the enhanced oxidation current at +0.172 V. Moreover, this sensor platform showed linear response for Hg detection from 1–10 μM in phosphate buffer saline (PBS) solution and the detection limit was found to be 0.2 μM (S/N = 3). The characterization of the Au-NPs and rGO-SH films were studied by Fourier-transform infrared spectroscopy (FT-IR), UV-Visible spectroscopy, transmission electron microscopy (TEM) and field emission scanning electron microscopy (FE-SEM). The application of the prepared sensor was also demonstrated in detecting mercury ions in tap water samples with satisfactory recovery analysis.
Export citation and abstract BibTeX RIS

This is an open access article distributed under the terms of the Creative Commons Attribution Non-Commercial No Derivatives 4.0 License (CC BY-NC-ND, http://creativecommons.org/licenses/by-nc-nd/4.0/), which permits non-commercial reuse, distribution, and reproduction in any medium, provided the original work is not changed in any way and is properly cited. For permission for commercial reuse, please email: oa@electrochem.org.
The Internet of things (IoT) is a unique system of interrelated communication devices (wireless sensor networks) which can able to transfer data without a need of human-to-computer interaction. In the aspect of environmental protection, smart sensors can able to finish the job where human cannot. By employing online sensing devices, we could track the changes in the concentration of pollutants in air or water, radiation and hazardous substances in our environments.1,2
Heavy metals (Pb, As, Hg, Cd etc.) are considered as major pollutants to the land and water pollutions.3,4 Mercury is the dense liquid metal and used in thermometers, light bulbs, dental amalgam, batteries and electrolytic cell.5 The discharge of mercury into the environment may come from the industrial waste, mining waste, power plants and some naturally occurring mercury minerals.6 The allowed level of mercury in drinking water is 1 μg/L.7 If inorganic mercury can be converted into methyl mercury by aerobic oxidation which is the main root for the respiratory failure and immune system in humans.8 So, it is very critical to detect mercury ions in tap and sea water samples. Current techniques such as atomic absorption spectroscopy (AAS),9 fluorescence spectroscopy10 and inductively coupled plasma spectroscopy11 were reported to detect mercury ions. But most of these methods have their own limitations. For example high operating cost, tedious sample preparation and longer analysis time etc.12 It is of great interest to develop simple, fast and selective detection for Hg ions.13 In this context, electrochemical sensors are relatively preferable because they offer several advantages for mercury detection such as simple electrode fabrication procedure, selective, sensitive detection and rapid analysis time and lower-cost techniques.14–17
To prepare electrochemical sensors, noble metal nanoparticles (NPs) such as Au, Pt and Ag based are more attractive nanomaterials because they offer high volume to surface area, pore size, good conductivity and high electro-catalytic activity compared to the bulk metal. Because of their extraordinary properties they have extensively used in batteries,18 solar cells,19 bio-imaging,20 biosensors,21 etc. Among these, Au-NPs have received particular attention in bio-imaging and chemical/biosensors. The detection of mercury ion at the Au-NPs interface is more sensitive and selective because they can form amalgam only with Hg compared to other metal ions.22
Wang et al. developed an electrochemical sensor based on Au-NPs and rGO using thymine 1-carboxylic acid.8 Similarly, Kanchana and coworkers modified a glassy carbon electrode (GCE) using biosynthesized hydroxyapatite from aloevera plant and it acted as the sensing agent for Hg ion.23 Other methods such as Au-NPs/thymine rich oligonucleotide,24 and mercury ion co-ordination with artificial nucleic acid25 were reported for Hg detection. These methods are developed and used at ambient condition using bioactive materials (DNA, biomolecules etc.), so they are relatively expensive, and problem arises with stability, tedious sampling process and complicated reaction mechanisms and also, they needed carriers or binders. But, some of the electrode binding agents will not allow the target metal ion to coordinate with biomolecules.
Graphene is a crystalline allotrope of graphite and it arranged in hexagonally lattices.26,27 Reduced graphene oxide (rGO) possess exceedingly low dispersion in aqueous solution and other organic solvents due to poor solubility.28 The solubility of rGO is increased when some functional groups are introduced into the graphene sheets such as nitrogen and sulfur.29 That dispersability makes the material to be versatile and useful for various applications such as polymer composite, energy related materials, sensors, field-effect transistors and biomedical applications.30
To increase the dispersibility/solubility of rGO in an organic solvent, for the first time, we used dodecanethiol (-SH) as a dispersing agent to disperse rGO sheets by a probe-assisted ultrasonication. The successful dispersion of rGO was verified by field-emission scanning electron microscopy (FE-SEM) and transmission electron microscopy (TEM). Furthermore, the interaction between as-synthesized Au-NPs and Hg2+ was ascertained by UV-Vis spectroscopy.
Next, surface of the GCE was modified with rGO-SH as followed by Au-NPs to study its electrochemical and catalytic properties toward Hg ions in 0.1 M phosphate buffered saline (PBS) solution. We also demonstrated the enhanced oxidation of Hg by differential pulse voltammetry (DPV) in the applied potential range from 0.0 to 0.3 V. The linear range of Hg detection was obtained from 1 to 10 μM. The developed sensor was highly selective to mercury ions, and the other metal ions (Ni2+, Cu2+, Mn2+, Co2+, Zn2+, Pb2+, and Al3+) did not interfere significantly in the electroanalysis. The real applicability of the electrode system is tested in real tap water samples which showed satisfactory recovery values.
Experimental
Chemicals and materials
Reduced graphene oxide (rGO) was purchased from Carbon Solution, USA. Dodecanethiol was purchased from Alfa-Aeser. Mercury (II) nitrate purchased from Acros Organics. Gold chloride trihydrate (HAuCl4.3H2O), ascorbic acid, sodium borohydride, zinc acetate trihydrate, copper sulfate pentahydrate, cobalt acetate, manganese acetate, lead acetate and nickel nitrate were purchased from Sigma-Aldrich, India. Silver nitrate (AgNO3) was received from SRL, India.
All the solutions were prepared by using milli-Q water. The 0.1 M PBS (pH = 7.0) was prepared using potassium monohydrogen phosphate (K2HPO4) and potassium dihydrogen phosphate (KH2PO4) which were used as an electrolyte solution. All the metal salt solutions were prepared at a required concentration by dissolving the corresponding salts in the aqueous solution.
Apparatus
The electrochemical measurements were performed with Biologic science instrument (SP-300), Electrochemical workstation from CH Instruments, USA (Model: CHI-760E) and Klyte 1.2 (Potentiostat, India). GCE (3 mm in diameter) was used as the working electrode, Ag/AgCl (1 M KCl) as the reference electrode and platinum wire as the counter electrode in a three-electrode system. The surface morphology of Au-NPs and rGO-SH were analyzed by field-emission scanning electron microscope (FE-SEM-EDS, FEI-Quanta-200FEG) and FEI-Tecnai F20 transmission electron microscope at accelerating voltage of 200 kV. The absorption spectrum of Au-NPs was characterized by 2000c nanodrop spectrophotometer, Nanodrop technologies, USA.
Functionalization of rGO material
The rGO powder (2 mg) was dispersed in 15 mL dodecanethiol via the probe sonication for 1 hr with the amplitude of 40% with the pulse of 5 sec On and 2 sec OFF. During the ultrasonication process, an ice-bath was used to control the temperature. As obtained rGO/SH dispersion was highly stable up to 4 weeks without any settling effect at room temperature which may due to dodecanethiol having a long aliphatic carbon chain group which strongly attached with rGO sheets (Scheme 1, Step-1).
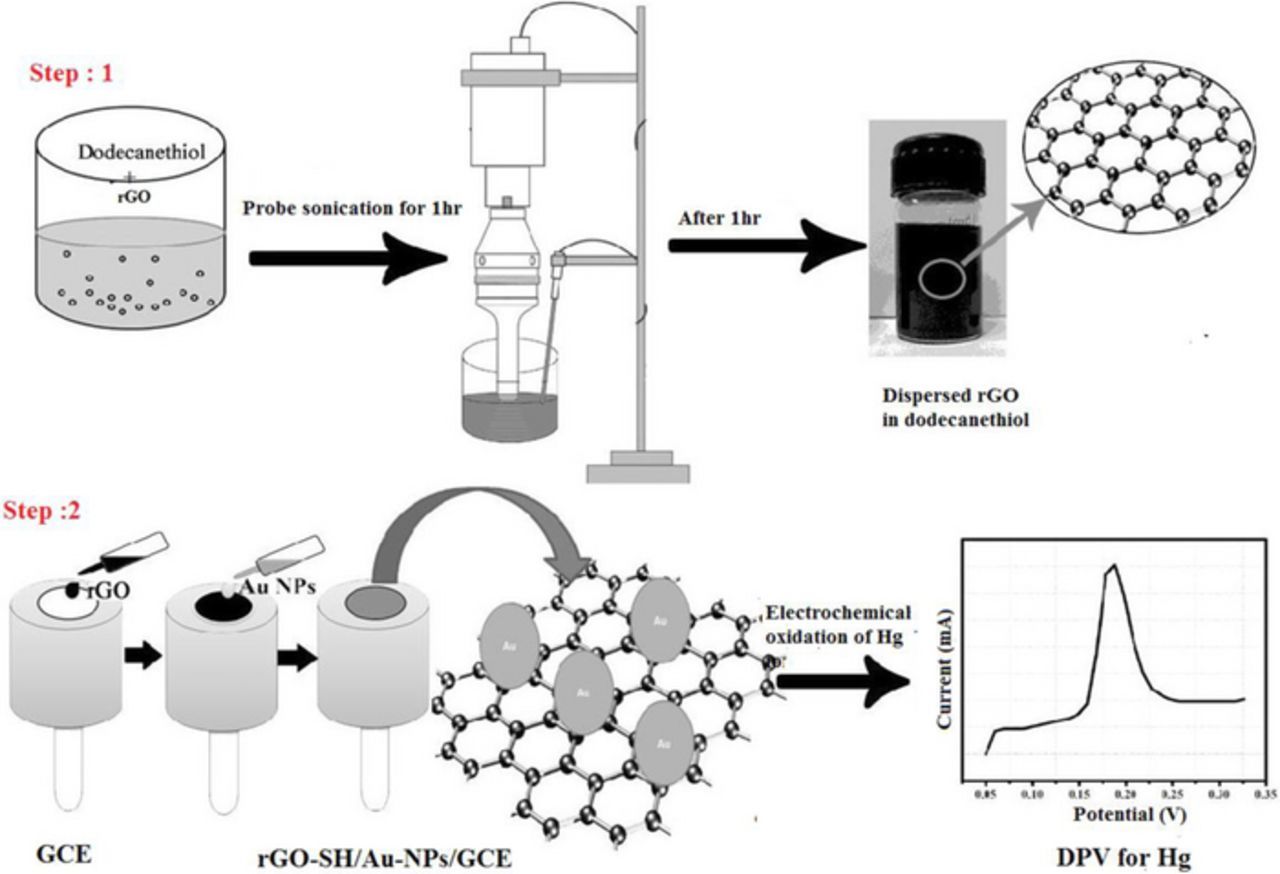
Scheme 1. (Step 1) Preparation of rGO/SH dispersion. (Step 2) Fabrication of electrochemical sensor to detect Hg2+ ions by DPV.
Preparation of gold nanoparticles (Au-NPs)
10 ml aqueous solution containing 1 mM HAuCl4.3H2O and 0.1 M cetyltributylammonium bromide (CTAB) were prepared. Further, 0.2 mM AgNO3 (10 mL) and 2 mM ascorbic acid added until it turns the color from orange to colorless. Finally, 10–100 μL of 1 mM NaBH4 (freshly prepared) added until pinkish violet color appears.31
Hg sensor fabrication
The surface of GCE was cleaned with α-alumina powder (0.05 μm) on a polishing cloth after that washed with double-distilled water until it gives a mirror-like surface. Further, the GCE was activated by potential cycling from −0.5 to 0.5 at a scan rate of 50 mV/s in 0.1 M H2SO4 for 10 cycles. Subsequently, the electrode was tested by recording cyclic voltammograms (CVs) in 5 mM K4[Fe(CN)6] and K3[Fe(CN)6) in 0.1 M KCl solution.32–34
10 μL rGO/SH dispersion was placed on the surface of GCE by drop casting method. After complete drying at 40°C in a hot air-oven, 10 μL of AuNPs solution was coated on the GCE/rGO-SH surface and then it dried again in hot air oven at 40°C for 10 min (Scheme 1, Step 2). Later, the GCE/rGO-SH/Au-NPs was gently washed using distilled water to remove any loosely bounded materials from the electrode surface. After that the GCE/rGO-SH/Au-NPs is dipped in different concentration of Hg2+ solution (Note: Hg(NO3)2 is sparingly soluble in water, so to obtain a clear solution, 3–4 drops of HNO3 was added) for 20 min and then it was scanned between −0.2 and 0.3 V by cyclic voltammetry in 0.1 M PBS (during this potential scan, adsorbed Hg2+ on the electrode surface get reduced to Hg). Later, the electrochemical oxidation of Hg present on the GCE/rGO-SH/Au-NPs was studied by differential pulse voltammetry (DPV). Differential pulse voltammograms (DPVs) were recorded by potential scanning from 0.0 to 0.3 V at a scan rate of 50 mV/s in 0.1 M PBS. The deposited Hg get oxidized to Hg2+ ion and giving the corresponding oxidation peak at 0.172 V (Scheme 1, Step 2).35
Results and Discussion
Characterization of rGO and rGO/-SH/Au-NPs
Fig. 1 shows the photographic images of dodecanethiol (image i) and rGO dispersed in dodecanethiol, (image ii) resulting the black color solution as obtained by probe-sonication. Schematic diagram of the functionalization of rGO-SH with Au-NPs for the detection of Hg ion, is shown in Scheme 1. As it shown in Fig. 1, image (ii), rGO sheets were well dispersed (as it confirmed by SEM and TEM analysis) in dodecanethiol solution up to four weeks without any aggregation.
Figure 1. Photographic images of (i) dodecanethiol and (ii) rGO-SH dispersion.
Next, the formation of Au-NPs was characterized by UV-Vis absorption spectrum. A strong absorption peak appeared at 520 nm indicated the formation of Au-NPs (Fig. 2a). Furthermore, the interaction between Au-NPs and Hg2+ ions was studied by UV-Vis spectroscopy. UV-Vis spectra were recorded before and after addition of 5 μM concentration of Hg2+ into Au-NPs solution (Fig. 2b). The addition of Hg2+ exhibited a peak shift of 10 nm (blue curve). It revealed that Au-NPs had interaction with the Hg2+, so the absorption spectrum was down (blue) shifted. It was also reported that Au-NPs could form amalgam with Hg2+ ions.36
Figure 2. (a) UV-Vis spectrum of the Au-NPs dispersion. (b) UV-Vis spectra of the Au-NPs dispersion before (red curve) and after (blue curve) the addition of 5 μM Hg2+ ions. (c) FT-IR spectra of (red dotted line) dodecanethiol and (solid blue line) rGO-SH film.
FT-IR spectra were recorded for dodecanethiol and –SH functionalized rGO powder (Fig. 2c, red and blue curves). Fig. 2c, red curve shows the absorption peak at (peak i) 745 cm−1 and (peak v) 2900 cm−1 correspond to stretching and bending of –CH3, (iv) peak at 2800 cm−1 is for –CH2 stretching and the peak position37 (peak iii) at 1600 cm−1 corresponds to carbonyl group. Those peaks were observed in dodecanethiol and rGO-SH except (ii) the peak at 1200 cm−1 corresponds to thioketals C=S formed between carbonyl group in rGO and -SH of dodecanethiol. Since this peak was not appeared in dodecanethiol, so it confirmed the covalent interaction between the rGO and dodecanethiol.38 An another peak (vi) was observed in rGO-SH due to rGO material which corresponds to –OH group (Fig. 2c).33,34
The samples for FE-SEM were prepared by drying overnight in the hot air oven and collected the powder samples. TEM sample was prepared by coating on the copper grid and then it was dried at the room temperature. Fig. 3 shows the surface morphologies of (image a) rGO-SH as characterized by the FE-SEM. Further, TEM image which revealed sheets like structures of rGO-SH film, as it confirmed the successful exfoliation of rGO sheets in dodecanethiol (image b).39 Dodecanethiol did increase the rGO sheets interlayer distance after functionalization with basal plane of rGO containing the carbonyl group. It might form thioketals which made the material to be more functional. Next, the Au-NPs were also characterized by TEM. The average particle sizes of Au-NPs were in the range of ∼10 nm (Fig. 3c).
Figure 3. (a) FE-SEM and (b) TEM images of rGO-SH film. (c) TEM image of Au-NPs.
Electrochemical detection of mercury ion
The solution of 5 μM Hg2+ was prepared and characterized by DPV technique at the (a) bare-GCE, (b) GCE/rGO/–SH and (c) GCE/rGO-SH/Au-NPs (Fig. 4). All these modified electrodes were dipped in the standard solution of 5 μM Hg2+ (5 mL) for 20 min after that it was dried in hot air oven for 5 min. Further the electrodes were immersed in the distilled water to remove if any loosely bounded soluble materials present on the electrodes surface. After that all the modified electrodes were used as working electrodes to record DPVs in the potential range from 0.0 to 0.3 V at a scan rate of 100 mV/s using PBS as an electrolyte (pH = 7) (Fig. 4). The oxidation peak was not observed for Hg at 0.172 V on bare GCE (curve i) and GCE/rGO-SH (curve ii). It corroborated that these electrodes were not suitable to attract Hg2+ ions toward the electrodes surface. Interestingly, before immersion of GCE/rGO-SH/Au-NPs in Hg2+ ion, it did not show any oxidation peak. But, after the immersion of GCE/rGO/-SH/Au-NPs in Hg2+ ion, the GCE/rGO/-SH/Au-NPs only showed a strong oxidation peak at 0.172 V (curve iv), it confirmed that this electrode material is suitable for sensing of mercury ions with the combination of rGO-SH/Au-NPs film because of the strong Au-NPs affinity toward the Hg2+ ion (Fig. 4).
Figure 4. DPVs were recorded in 0.1 M PBS (pH 7.0) using (i) bare GCE, (ii) GCE/rGO-SH and (iv) GCE/rGO-SH/Au-NPs after immersion in 5 μM Hg2+. (iii) DPV of GCE/rGO-SH/Au-NPs in 0.1 M PBS (blank) before immersion in 5 μM Hg2+. Scan rate = 100 mV/s.
We also described the schematic presentation of electrode active material synthesis and detecting Hg2+ ion in Fig. 5. The rGO-SH film may acted as a nucleophile as it had the sulfur group which easily interacted with Au-NPs.40 The interaction between rGO-SH/Au-NPs film and Hg2+ ion might have occurred through the amalgamation type reactions. During the potential cycling between 0.3 and −0.2 V, the assembled Hg2+ ions get reduced to Hg0. Later, when DPV was recorded (anodic scan), the attached Hg0 was get oxidized back to Hg2+ ion at 0.172 V.35
Figure 5. Schematic representation for electro-oxidation of Hg at rGO-SH/Au-NPs modified electrode.
Stability of the modified electrode
Figs. 6a and 6b shows CVs of the of (a) GCE/rGO/Au-NPs and (b) GCE/rGO-SH/Au-NPs recorded in 0.1 M KCl solution in the potential range from −0.2 to 0.3 V at a scan rate of 50 mV/s. The stability of the modified electrodes was analyzed by cyclic voltammetry (CV) by comparing the materials of rGO dispersed in dimethylformamide (DMF) and in dodecanethiol. Both dispersions were prepared after probe sonication independently for 1 hr. Two individual GCE's were modified with rGO/DMF and rGO/SH dispersions using 10 μL of each dispersion on the electrode surface. After that, 10 μL of Au-NPs dispersion was placed on the both electrode's surface and dried (in hot air oven for 5 min) it as a second layer. Finally, the CVs were recorded by applying potential between −0.2 and 0.3 V at a scan rate of 50 mV/s for 10 cycles in KCl solution. The GCE/rGO/SH film exhibited enhanced anodic peak currents due to higher loading of Au-NPs compared to the GCE/rGO/DMF film (Figs. 6a and 6b). The reason might be due to presence of –SH group on the rGO sheets; it had strong affinity toward the Ag-NPs. This result confirmed that rGO-SH functionalized film can be utilized for fabrication of Hg2+ sensor.
Figure 6. CVs of (a) GCE/rGO/Au-NPs and (b) GCE/rGO/-SH/Au-NPs recorded in 0.1 M KCl solution in the potential range from −0.2 to 0.3 V at a scan rate of 50 mV/s.
Self-assembly of Au-NPs on rGO/SH film and time variation studies
We also studied the self-assembly behavior of Au-NPs on the rGO-SH film. Fig. 7 shows the time variation studies upon self-assembly of Au-NPs onto the rGO-SH film modified GCE. The oxidation peak increases with the immersion time in Au-NPs dispersion. After the immersion of GCE/rGO-SH in Au-NPs dispersion, the CVs were recorded in the potential range from −0.2 to 0.3 at a scan rate of 50 mV/s. The oxidation peak currents were increased with the immersion time due to the continuous adsorption of Au-NPs on the rGO-SH film modified electrode (Fig. 7). The self-assembling nature of nanoparticles was increased up to 3 hr. However, after 4 hr of immersion time, the oxidation peak current was again started to decrease which indicated the saturation effect of Au-NPs self-assembly on the modified electrode's surface. This study has also proved the existence of strong interaction between rGO-SH film and Au-NPs (Fig. 7).
Figure 7. CVs of self-assembled Au-NPs on rGO-SH modified GCE at different time intervals: (i) 1 hr, (ii) 2 hr, (iii) 3 hr and (iv) 4 hr. Electrolyte: 0.1 M KCl. Scan rate = 50 mV/s.
Calibration plot of Hg2+
The different concentrations of mercury ion solutions were prepared by diluting the stock solution of 1 mM Hg2+ solution. The prepared various Hg2+ concentration solutions were analyzed by DPV technique using the GCE/rGO-SH/Au-NPs. The GCE/rGO-SH/Au-NPs was immersed in different concentration of Hg2+ ion (5 mL) solution using the same procedure as given in Hg sensor fabrication section. The Hg oxidation current was gradually increased from the lower concentration to higher which indicated the high sensitivity of the electrode (Fig. 8a). Fig. 8b shows the calibration curve plotted against Hg2+ ions concentration vs. oxidation peak current in the range from 1–10 μM in 0.1 M PBS (Each data point is the mean value of three measurements recorded using three different GCE/rGO-SH/Au-NPs modified electrodes, Fig. 8b). The linear graph was well fitted with the correlation coefficient (R2) value of 0.972 (Fig. 8b). The detection limit of Hg2+ was found to be 0.2 μM (S/N = 3).21 The reproducibility of the GCE/rGO-SH/Au-NPs sensor was also analyzed by detecting the concentration of 5.0 μM Hg2+ in 0.1 M PBS. The obtained data indicated that the modified electrode possesses an acceptable reproducibility with a relative standard deviation (RSD) of 5.52% for five parallel experiments with different electrodes.
Figure 8. (a) DPVs of Hg oxidation at rGO-SH/Au-NPs modified electrode in 0.1 M PBS. (i) 1 μM, (ii) 3 μM, (iii) 5 μM and (iv) 10 μM Hg2+. (b) Calibration curve obtained for different concentration of Hg vs. oxidation current (Each data point is the mean of three measurements).
Interfering ion studies
The GCE/rGO-SH/AuNPs based sensor was studied with different metal ions (Co2+, Ni2+, Cu2+, Mn2+, Zn2+, Al3+, Pb2+, and Hg2+). The standard solutions of all metal ions (5 μM) were prepared and the analysis procedure was repeated as given in Hg sensor fabrication section. As shown in Fig. 9, the Hg oxidation was only exhibited the higher peak current at 0.172 V. All other metal ions did not show significant oxidation current compared to Hg. From this interference metal ion analysis, it was confirmed that the rGO-SH-AuNPs film is very selectively interacted with Hg2+ ion compared to other metal ions which may be due to the unique combination of rGO-SH and AuNPs (Fig. 9).
Figure 9. Effect of common metal ion interferences analysis at the GCE/rGO/-SH/Au-NPs. Interfering metal ions were: Hg2+, Ni2+, Cu2+, Mn2+, Co2+, Zn2+, Al3+, and Pb2+ (concentration of each metal ion was fixed as 5 μM).
Detecting Hg in tap water samples
The as-prepared GCE/rGO-SH/Au-NPs was utilized as a sensor to measure the known concentration of Hg2+ spiked into the real water samples. The tap water samples (collected from our laboratory) (1 mL) were spiked with 1 and 5 μM concentration of Hg2+ ions and made up to 10 mL solution using distilled water. The DPV was recorded as given in Electrochemical detection of mercury ion section. The Hg recovery was estimated as it was in the range of 94.0–97.5%. We envisage that this GCE/rGO-SH/Au-NPs can be applied to detect Hg2+ ions selectively in the environmental samples (Table I).
Conclusions
The rGO sheets were functionalized with dodecanethiol via probe sonication. The obtained rGO dispersion was stable up to 4 weeks. The well dispersed material had greater interaction with AuNPs due to thiol chemistry. The rGO-SH/Au-NPs coated GCE was prepared and used for sensing of Hg2+ ion with good selectivity. The effect of other metal interference analysis was also carried out, which revealed that this electrode platform is very selective toward Hg2+ ions over other studied metal ions. The linear detection range was found to be from 1 to 10 μM Hg2+. Furthermore, the rGO-SH/Au-NPs materials were characterized using UV-vis, FT-IR, FE-SEM, and TEM. The real applicability of the electrode was also tested by analyzing real water samples spiked with known concentration of Hg2+ and satisfactory recovery of Hg was demonstrated. We believe that this proposed electrochemical method could be applied as a simple and highly sensitive technique to detect mercury ion.
Acknowledgment
We acknowledge the financial support from the Department of Science and Technology (DST)-Science and Engineering Research Based (SERB), Government of India. Award No.: ECR/2016/001446. We also acknowledge the seed grant funding from SRM Institute of Science and Technology. NR acknowledges PhD student fellowship from SRM Institute of Science and Technology.
ORCID
Ashok K. Sundramoorthy 0000-0002-8512-9393