Abstract
Climate change mitigation policies have usually considered forest-based actions as cheap and fast options to reduce CO2 concentration in the atmosphere and slow down global warming. Most economic analyses, however, have ignored the effects of these actions on land surface albedo and the resulting effect on energy balance and temperature. This study estimates the marginal cost of forest mitigation associated with both carbon sequestration and albedo change, by introducing regional and forest-specific albedo information in a global dynamic forestry model. Our analysis indicates that traditional forest sequestration policies have underestimated the costs of climate mitigation, driving forest-based actions in regions where subsequent changes in albedo are significant. To reduce this inefficiency, this paper proposes a novel approach where both carbon sequestration and albedo effect are incorporated into pricing. Our results suggest that, under the same carbon price path, the integrative policy provides greater net global mitigation in absolute terms and per hectare of forest, and thus it is more efficient and less intrusive than the traditional policy.
Export citation and abstract BibTeX RIS

Original content from this work may be used under the terms of the Creative Commons Attribution 3.0 licence. Any further distribution of this work must maintain attribution to the author(s) and the title of the work, journal citation and DOI.
1. Introduction
Mitigating climate change requires action in all sectors of the economy. The forestry sector has a significant potential to contribute to climate change mitigation by decreasing greenhouse gases in the atmosphere and reducing the increase in global average temperature. Numerous studies have suggested that forest-based actions, such as slowing down deforestation rate, reforestation, changing forest management, and lengthening rotations, are low cost climate change mitigation options (Plantinga et al 1999, Sohngen and Mendelsohn 2003, Kindermann et al 2008). More recently, the studies reviewed by the IPCC AR5 estimate that the forestry sector could capture from 0.2–13.8 GtCO2eq/yr at carbon prices up to 100 USD/tCO2eq (Smith et al 2014). Finally, we note that the RCP 4.5 scenarios suggest that a widespread afforestation, with global forested land area increasing by 25% over the century, is necessary in order to reducing land-use carbon emissions from land use change to near zero (Thomson et al 2010, 2011, Jones et al 2012, Davies-Barnard et al 2015).
However, changing forest management practices also affects the surface energy budget and thus feedback to climate by regulating the biophysical properties such as albedo and roughness length. Several studies have shown that the reduction in albedo due to reforestation could increase the solar radiative energy absorbed by Earth's surface and thus has potential to offset much of the carbon storage benefit provided by forests, especially in boreal forests (Betts 2000, Gibbard et al 2005, Bala et al 2007, Bonan 2008, Kirschbaum et al 2011). The magnitude and sign of albedo change from land conversion to forests, however, depend on many factors, such as snow extent and pre-conversion land cover type, which vary from region to region. Forests also have higher transpiration rate, which further cools down the surface, and can affect hydrological cycles by regulating cloud cover and precipitation (Pielke et al 2007, Mahmood et al 2013).
Given widespread interest among policy makers to use forests to offset world's emissions, it is important to assess the net benefits of forest management on climate mitigation and the extent to which albedo alters the costs of forest mitigation. How much do changes in surface albedo affect the cost of traditional forest mitigation policy? Is there a policy approach to include albedo in forest-based mitigation strategies? A dynamic and global assessment is critical for answering these questions because it captures both the significant variation of carbon benefits and albedo changes across different regions, forest species and age structures, and the interactions across regions through the timber market.
This study aims to assess the costs of using forests for climate change mitigation, considering the effects from carbon sequestration, albedo change converted in carbon equivalent (C-eq), and timber production, in a dynamic and integrated global analysis framework. We incorporate the albedo information of dominant mature forests and cleared land (cropland or bare land) for each world region from the satellite observations in the dynamic optimization model of global forests GTM (Sohngen et al 1999).
A dynamic analysis is crucial for two reasons. First, carbon prices are expected to rise over time (Nordhaus 2010) and forestry actions will become cost effective and be implemented at different times as prices rise. This also means that the effects of changes in albedo will be valued differently over time. Including albedo in the pricing system will alter the time path of adoption of forestry practices, and will have differential effects by region. Second, albedo varies with forest stand age, for example, mature forests usually absorb more energy than young forest, increasing the warming effect (Bright et al 2015, Mykleby et al 2017). Given that forest management for timber and carbon depends on decisions that are based on stand age, it is important to account for the influence of albedo dynamics on forest management strategies. For instance, traditional economic studies that ignore albedo effects suggest that carbon payments increase rotation ages (van Kooten et al 1995 and Sohngen and Mendelsohn 2003), but rotation ages are found to be reduced when albedo is included in accounting (Thompson et al 2009, Sjølie et al 2013, Lutz and Howarth 2014, Lutz et al 2016).
The market interactions across regions through market changes in timber supply and prices need to be accounted for, when measuring costs. Therefore, a global model like the Global Timber Model (GTM) is necessary for our analysis. For instance, meeting stringent policy solutions such as a 2 °C temperature limitation will require significant global effort, likely including forest carbon sequestration, but shifts in land use can have implications for timber prices and land prices beyond the region in which forest mitigation increases (Favero et al 2017).
The regional disaggregation of GTM allows us to take into account the regional differences in carbon sequestration potential and regional changes in albedo from land conversion to forests. Economic studies have suggested that the costs of carbon sequestration, ignoring albedo, vary substantially from region to region depending on land values, forest productivity, and other factors (Sohngen and Mendelsohn 2003, Richards and Stokes 2004). Studies examining albedo have similarly shown that albedo differs across the globe (Betts 2000, Gibbard et al 2005, Bala et al 2007, Bonan 2008, Kirschbaum et al 2011). For example, larger areas of boreal and temperate forests are likely to have much lower surface albedo due to the snow masking effects of canopy than cropland or bare land, resulting in a warming effect. In addition, forest-based mitigation activities resulting in changes in tree species can alter albedo and result in significant climate impacts (Bright 2015, Bright et al 2016). These effects on the marginal costs of forest mitigation can be reconciled with a modeling approach that accounts for many species, and the market mediated responses.
To estimate the effect of changes in albedo on the marginal costs of forest mitigation, we simulate two scenarios in GTM using five carbon price paths from the DICE model (Nordahus 2014). A traditional forest sequestration policy, where only forest carbon sequestration is valued at the carbon price (Traditional Policy), is simulated, and then a contrasting novel policy approach, where both carbon sequestration and changes in albedo are valued at the carbon price (Integrative Policy). These two policy scenarios are then compared with a No Policy scenario where no climate mitigation policy exists. Changes in forest carbon sequestration and albedo converted in carbon equivalent across different carbon price paths are then used to build forest mitigation supply functions under each scenario (figure 1).
Figure 1. Conceptual diagram of our analysis.
Download figure:
Standard image High-resolution image2. Data and methods
2.1. Global timber model
We use the GTM to simulate the optimal management of global forestland for climate change mitigation with albedo. GTM is a global model that combines the spatially detailed data on current forest stocks with an economic model that weighs optimal forest management alternatives (Sohngen et al 1999, 2001, Sohngen and Mendelsohn 2003). It is a dynamic intertemporal optimization model that manages forests by maximizing the net present value (NPV) of consumer and producer surplus in timber markets. The model contains 200 forest types (i) in 16 regions that interacts through the global timber market. By maximizing the NPV, the model optimizes the age of harvesting timber (a) and the intensity of regenerating and managing forests. It is an optimal control problem given the aggregate demand function, starting stock, costs, and growth functions of forest stocks (see section 1 of the supplementary material for details, available online: stacks.iop.org/ERL/13/125002/mmedia).
GTM relies on forward-looking behavior and solves all time periods at the same time; this means that when land owners make decisions today about forest management, they do so by considering the implications of their actions today on forests in the future with complete information. This assumption is consistent with those made in the DICE model, and it allows us to adopt the carbon prices from the DICE model for the policy analysis (Nordhaus 2014).
2.2. Carbon accounting
In GTM carbon storage is counted in three main pools: aboveground forest carbon, forest products and soil carbon.
Aboveground carbon (), accounts for the carbon in all tree components as well as carbon in the forest understory and sitting on the forest floor at each time t. It is a function of the stock of land (
), the growth function (
), and the management intensity (
) of age a and i forest type:

where δ is the conversion factor that converts forest biomass into carbon, it is forest and regional-specific.
Carbon in forest products () is estimated by tracking forest products over time and it is calculated as follows:

where is the timber volume and
is the harvested area in each age class a at any time t.
is the factor to convert market biomass into carbon permanently stored in products. We here assume
to be 0.30 (Winjum et al 1998).
Soil carbon () is measured as the stock of carbon in forest soils of type i in time t. In this analysis, we capture the marginal change in carbon value associated with land use changes. When land use change occurs, we track net carbon gains or losses over time as follows:
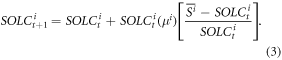
The value of the steady state level of carbon in forest soils, it is unique to each region and timber type i. The parameter
is the growth rate for soil carbon. The same equation is used when land converts from forest to agriculture but with the reverse initial carbon and steady state numbers (Daigneault et al 2012, Favero et al 2017). Harvesting does not affect soil carbon if the land remains in forests but causes a decline in soil carbon if there is a change in land use (Houghton and Goodale 2004).
2.3. Albedo change and radiative forcing at the surface
To include the albedo effect in the forestry model, we first collect and analyze albedo data for each political region and each dominant land cover type, then calculate the radiative forcing (in W m−2) caused by albedo change from land conversion, and finally convert the forcing to C emissions-equivalent (figure 1).
We obtained the latest version of the global daily albedo products (MCD43A3, version 006) from 2010–2016 at 500 m resolution derived from the Moderate Resolution Imaging Spectroradiometer (MODIS) (Schaaf et al 2002). To identify the land cover types, we summarized the dominant land cover types (figure 2) in each climatic region during 2010–2013, based on the Köppen–Geiger climate map and the annual MODIS IGBP land cover classes at 500 m resolution (Kottek et al 2006). For each dominant forest type over each region, we also generated the histograms of tree cover percentage from the MODIS Vegetation Continuous Field (VCF) data and identified the upper ¼ quantile. A forest pixel was assumed as mature forest if its tree cover is higher than 75% of all the forest pixels across a particular region.
Figure 2. Global vegetation type—regional aggregation.
Download figure:
Standard image High-resolution imageWe here used the total shortwave white-sky albedo from MODIS during 2010–2016 to summarize the mean annual albedo for mature forests and cleared land (cropland or bare land) over each political region. We first calculated the 7-year average albedo value for each Julian day of the year for each land cover type. The mean annual albedo of each land cover type is then calculated as an average of daily albedo weighted by daily incoming shortwave radiation. We used the incoming solar radiation product, the surface Energy Balanced and Filled data at 1 × 1° from 01/2010 to 08/2016, from NASA CERES (the Clouds and the Earth's Radiant Energy System) science team, to calculate the 7-year average surface clear-sky shortwave solar radiation for each study region. (Table 2 in the supplementary material provides a detailed summary of albedo statistics for each political region.)
We estimated the resulting shortwave radiative forcing () at the surface caused by changes in albedo values from cleared land to mature forest:

where represents the absorbed surface radiative forcing;
and
are the weighted mean albedo of the cropland (or bare land) and the mature dominant forest type respectively and they are both region and forest specific; K represents the average clear-sky shortwave solar radiation from 2010 to 2016.
2.4. Equivalent carbon change due to changes in land cover albedo and forest maturity
The relationship between changes in radiative forcing induced by changes in albedo () and CO2-equivalent emissions is well known in the literature (see for instance Betts 2000, Joos et al 2013, Caiazzo et al 2014, Mykelby et al 2017). First,
is converted into change in global atmospheric carbon concentration (
) through the logarithmic relation:

where is the global atmospheric CO2 concentration change (in parts per million, ppm) that would result from the radiative forcing
is the surface area of the Earth, and
is the current global mean atmospheric CO2 concentration over the course of the 20th century. The value 5.35 represents a coefficient used to quantify the warming potential of atmospheric CO2. The calculations are done for each region and forest type by determining the change in terrestrial carbon stock that is equivalent to a change in surface albedo resulting from a transition from cleared land to mature forestland. The equivalent increase in carbon stock are considered emissions because transition to forest lowers surface albedo and exerts positive radiative forcing, just like releases of carbon from timber harvest (Zhao and Jackson 2014).
The concentration is then converted into an equivalent carbon emission per unit of hectare subject to albedo change following the method described in Caiazzo et al (2014) and Myklby et al (2017). That is,
corresponds to the C-equivalent emissions that will be released if one hectare of cleared land (cropland or bare land) would be converted in a hectare of mature forest.

where and
are the molecular weights of carbon and air respectively,
is the airborne emission fraction of CO2 for a time horizon of 100 years, Aa is the reference area subject to albedo expressed in hectare and
is the mass of Earth's atmosphere.
Since albedo varies with forest age, we need an expression that describes the albedo 'decay' due to forest growth and the corresponding effect. Yearly changes in albedo are based on the assumption that as forests grow from cleared land, albedo decreases and reaches a minimum when the forest reaches its maturity; we further assume that the resulting increase in the corresponding C-equivalent emissions follows an exponential function (Bright et al 2012, 2015, Cherubini et al 2012). Carbon-equivalent emissions change over forest age (a) is calculated by using the following function:

where parameters η, m and n are typical growth parameters and they include information about forest maturity. (Supplementary figure 5 shows the regional changes in albedo C-eq per hectare (tC-eq/ha) as a function of forest age.)
Finally, to determine total increase in C-eq albedo for a given timber type i, we sum over the hectares in each age class as follows:

Considering the uncertainties in the assumption on the age-dependency of albedo, we further tested the sensitivity of the results to the assumed forest maturity ages and corresponding decay functions for each region and forest type. In particular, in section 4.1 of the supplementary material, we tested two scenarios where forest reaches its maturity 50 years before and 50 years later than the base case.
2.5. Forest mitigation policy scenarios
The GTM captures mitigation potential of the forestry sector through a global carbon incentive that values forest climate change mitigation contribution in terms of net C-equivalent sequestration as follow:
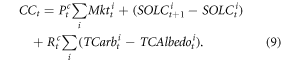
The first part of equation (9) is the carbon transferred to long-lived wood products () from each forest i valued at the carbon price
The change in soil carbon (
) when land switches between forests and agriculture is also valued at the carbon price (Daigneault et al 2012, Favero et al 2017).
The second term of equation (9) is the annual rent scheme whereby the total carbon stocks in forests (
) net of C-eq albedo (
) are rented during the time period that the carbon is stored following Sohngen and Mendelsohn (2003) and Thompson et al (2009). The rental value for carbon is:

where r is the interest rate. This equation accounts for potential price increases in carbon that occur as carbon accumulates in the atmosphere.
To estimate the forest mitigation supply functions (with and without albedo), we run multiple simulations using GTM under five exgoneous carbon price paths () from DICE-2013R (Nordahus 2014), following the approach outlined in Sohngen and Mendelsohn (2003). Carbon price paths start in 20104
at prices ranging from 14 to 57 USD/tC, and reaching 264 to 540 USD/tC in 2100. The starting price, the price path, and the final price depend on assumptions in the DICE-2013R model about the damage function or policy implementation. Section 2 of the supplementary material describes in detail the assumptions behind each carbon price path from DICE-2013R.
We first simulate a No Policy (Reference) scenario where no climate mitigation policy exists and forest owners only receive revenue from timber market. Under this scenario therefore there are no carbon payments for forest mitigation
Then, we simulate a Traditional Policy forest mitigation policy where forest owners are compensated by annual rents for providing annual carbon storage services and, at harvest, they are paid the carbon price
for carbon stored permanently in wood products and they receive revenue from timber market. Under this policy scenario albedo is not considered
Under the Integrative Policy scenario, forest carbon sequestration net of albedo converted in C-equivalent is rewarded following the rent scheme and at harvest, landowners are paid the carbon price for carbon stored permanently in wood products and receive revenue from timber market.
3. Results
3.1. Applications to specific regions
We first focus on three regions and one of their dominant forest types (Brazilian Tropical Evergreen forest, Northern Europe Temperate Evergreen forest, and Canadian Boreal Softwood forest) to illustrate the effect of the alternative policy regimes on NPV of an heactare of forestland and the optimal rotation age.
Table 1 column a shows the changes in radiative forcing of mature forestland with respect to cleared land in the three regions and forest types. In column b radiative forcing is converted in tC-eq per heactare (tC-eq/ha) following equations (4)–(7) presented above. Column c shows the estimated aboveground forest sequestration of each mature forest and in column d we measure the net mitigation of each mature forest in terms of C-eq/ha.
Table 1. Regional estimates of (a) changes in radiative forcing albedo from cropland to mature forestland; (b) albedo carbon equivalent values; (c) aboveground forest C sequestration and (d) net carbon sequestration for regional mature forests.
(a) W m−2 difference forest-bareland ![]() |
(b) Albedo change in C-eq (tC/ha) ![]() |
(c) Aboveground forest sequestration (tC/ha) ![]() |
(d = c − b) Net C-eq sequestration mature forest (tC/ha) | |
---|---|---|---|---|
Brazil tropical evergreen | 10 | 59 | 150 | 91 |
Northern EU temperate evergreen | 28 | 161 | 192 | 31 |
Canada boreal softwood | 22 | 129 | 76 | (53) |
Results presented in supplementary table 3 show that under the No Policy scenario, the optimal rotation year is 26 years for Brazil, 46 for Northern Europe and 57 for Canada, the merchantable biomass yield is 18 m3/ha, 75 m3/ha and 29 m3/ha and the corresponding NPV of the stand is 633 $/ha, 684 $/ha and 171 $/ha respectively.
Under the policy that values only carbon sequestration and ignores albedo (Traditional Policy), forest owners have the incentive to hold timber longer than in the No Policy scenario and forestland increases its value in all regions. That is, lengthening rotations increases carbon storage by increasing the size of trees per hectare. The optimal rotation age increases to 29–31 years (Brazil), 48–51 years (Northern EU) and 59–62 years (Canada) with an increase in the NPV of the stand of 984–3,979 $/ha, 199–820 $/ha and 33–135 $/ha depending on the carbon price.
Finally, when albedo is included in the valuation of forest-based actions (Integrative Policy), the optimal rotation is shorter and NPV is reduced with respect to the Traditional Policy scenario. With albedo, the optimal rotation falls under the Integrative Policy scenario compared to the Traditional Policy scenario because the albedo penalty increases as the forest ages, reducing the value of maintaining forest cover for additional years.
In the case of Canadian Boreal forest, the NPV of the albedo effect is bigger than the NPV of carbon sequestration, suggesting that mitigation with this forest type would not provide economic, or atmospheric, benefits. Similar results have been found in Lutz et al (2016) for some locations in New Hampshire. In the case of the other two species, the albedo effect detracts from carbon value, but for those species there are overall carbon benefits associated with increasing forest cover. In addition to showing the optimal rotation age, these results also illustrate that policies aimed at slowing deforestation in Brazil would still be effective because net sequestration is positive, albeit less effective than suggested by studies that do not include albedo.
3.2. Global changes in albedo due to forest-based mitigation actions
A climate policy (like a carbon price) that values forest carbon sequestration (Traditional Policy) creates the incentive to reduce deforestation, convert land to forest (afforest), to grow forest more quickly (increase management intensity), and to extend the rotation of forests (possibly indefinitely). These actions not only increase the amount of carbon sequester in forest but also affect land albedo through both land cover change (avoided deforestation and afforestation) and land management change (changes in management intensity and forest rotation). In order to measure the global implications of changes in albedo on the climate mitigation potential of forests, we simulate the Traditional Policy under the carbon price paths and then measure ex-post the resulting carbon-equivalent changes in surface albedo.
Figure 3(a) shows the changes of global forest carbon stock in three different pools under the Traditional Policy scenario relative to the No Policy scenario. The conversion of more land to forest, the increase in management intensity and the more extended forest rotation produce an increase in the stock of carbon sequestered above and below ground (soil) with respect to the No Policy scenario. Timber supply also increases in the long-run adding extra market carbon.
Figure 3. Changes in carbon stock in the three carbon pools and changes in albedo carbon-equivalent in Gt C-eq (a) under Traditional Policy scenario relative to the No Policy scenario; and (b) under the Integrative Policy scenario relative to the No Policy scenario. Notes: changes in C-eq albedo under the Traditional Policy scenario relative to the No Policy scenario are calculated offline 'back of the envelope'. In the Integrative Policy scenario, changes in C-eq are endogenous.
Download figure:
Standard image High-resolution imageBy 2100 under an assumed carbon price of 540 USD, global forest will increase the stock of carbon by 298 GtC-eq relative to the No Policy scenario, sequestering approximately 3.3 GtC-eq/yr for 2010–2100. However, all the forest-activities driven by the Traditional Policy indirectly change surface albedo, resulting in a warming effect, with respect to the No Policy scenario. Once the albedo is converted in carbon equivalent and included in the calculation (Traditional Policy Net), the net climate mitigation potential of forests under a carbon price of 540 USD is reduced by 46%, falling to 153 GtC-eq (about 1.7 GtC-eq/yr). This suggests that a traditional policy that values only the carbon sequestration benefits of forest without considering albedo underestimates the costs of a forest mitigation by about 50%, and thus is an inefficient policy.
3.3. Integrative policy
In order to avoid the implementation of counterproductive climate change mitigation (like the Traditional Policy), we propose a novel approach where both carbon sequestration and albedo are incorporated into pricing (Integrative Policy). Does the proposed Integrative Policy fully address the albedo issue in an effective way? Figure 3(b) answers this question. Under the Integrative Policy scenario, global average carbon benefits of forest actions are greater than the corresponding negative climate impacts of changes in surface albedo, therefore there is still the incentive to reduce deforestation, increase forestland and extend forest rotations relative to the No Policy scenario. However, to minimize the effects of albedo, the policy re-allocates forests mitigation activities in regions where changes in surface albedo are smaller, reduces forest rotation and decreases global land conversion to forest relative to the Traditional policy. These changes produce lower forest carbon storage as well as lower changes in C-eq albedo relative to the Traditional Policy scenario. However, under the same carbon price path, when albedo is included in the policy, the Integrative Policy provides greater net mitigation than the Traditional Policy (net).
3.4. Forest global mitigation supply functions
Our results suggest that for all the carbon prices tested a policy that ignores the albedo consequences of forest-based activities underestimates marginal costs (figure 4(a)). For carbon prices below 150 USD, the forestry sector delivers 2–104 Gt of carbon in forested ecosystems, but when albedo effects are included, net mitigation potential is less than 29 GtC-eq. For carbon prices of 150–400 USD/tC, the mitigation potential is 57%–84% lower than what is estimated without albedo. Finally, for carbon prices higher than 400 USD/tC the mitigation potential is 50%–55% lower when the albedo is included in the estimates.
Figure 4. (a) Changes in forest climate mitigation potential in C-eq for different carbon prices under the Traditional Policy regime (light blue), the Traditional Policy (net) which accounts ex post for albedo (dark blue) and the Integrative Policy regime (red) which prices both forest sequestration and albedo relative to the No Policy scenario; (b) net mitigation per changes in forestland (in million hectares) under the Traditional Policy and the Integrative Policy (net) relative to the No Policy scenario.
Download figure:
Standard image High-resolution imageSecond, by comparing the net mitigation of the Traditional Policy with the net mitigation of the Integrative Policy, results suggest that the Integrative Policy is cheaper, or more efficient, than a policy that does not include albedo in the pricing formula. For instance, to attain net mitigation of around 100 GtC-eq will cost 20%–26% more if the pricing formula ignores albedo. That is, the Integrative Policy is always more effective than the Traditional Policy.
Furthermore, at the global level, the Integrative Policy requires less land to be converted to forestland for the same level of net mitigation, and thus is less intrusive than the Traditional Policy. For instance, to achieve a net mitigation of 100 GtC-eq, it will require about 750 million hectares (Mha) more if the pricing formula ignores albedo (figure 4(b)).
3.5. Regional supply functions
Forest mitigation costs were found to vary significantly across regions under the Traditional Policy and Integrative Policy scenarios (figure 5). In temperate and boreal regions, North America and Russia have significantly higher marginal costs of net mitigation, and albedo increases the costs (figures 5(a) and (b)). Estimates for Europe are about the same with or without consideration of albedo (figure 5(c)). While costs are higher when albedo is included in the carbon pricing regime, the Integrative Policy still encourages mitigation in Canada and Russia. For instance, in Russia the Integrative Policy affects forest management by encouraging the conversion of older forests to young forests. Under the Integrative Policy, the amount of forestland in Russia is almost unchanged relative to the Traditional Policy, but forests are converted from old natural forest to young managed forests. This important interaction between albedo, carbon sequestration and forest age arises because the albedo penalty increases as forests age, thus reducing the value of climate mitigation in mature forests. This result can have important policy implications since the Integrative policy might not guarantee the preservation of natural forests. In section 4.2 of the supplementary material we tested how our results will be affected under a scenario where the world natural unmanaged forests are fixed to current values. Our results suggest that under this constrained scenario, the Integrative policy delivers almost the same amount of climate mitigation as the Traditional Policy (net). However, the Integrative Policy is still a preferable policy because it is less intrusive than the Traditional Policy in terms of hectares used for forest mitigation (supplementary figure 9).
Figure 5. Cumulative C-eq sequestration functions in selected regions (a) North America; (b) Russia; (c) Europe; (d) Central and South America; (e) South East Asia and (f) Africa.
Download figure:
Standard image High-resolution imageIn tropical regions, the effects of albedo on marginal costs in South East Asia and Central and South America are relatively modest (figures 5(d)–(e)), however, albedo has important implications for marginal costs in Africa (figure 5(f)). Because native soil surfaces are fairly light in Africa, conversion of land to forests, or reduced deforestation, both have strong effects on albedo, making most actions in Africa counterproductive for net mitigation. Africa differs because cleared land has a very high albedo with respect to the same land cover types in other tropical regions (Fuller and Ottke 2002). Therefore, the variation of albedo with respect to mature forestland in this region is particularly high. Moreover, the incoming solar radiation of Africa is slightly higher than other tropical regions.
In order to measure to what extent Africa is skewing the global mitigation supply functions of forest, in section 4.3 of the supplementary material we simulate the same scenarios excluding forest-based mitigation actions in Africa. Our results indicate that even if Africa is excluded the Traditional Policy still underestimates the forest mitigation potential because forest-based actions are implemented in other regions in which changes in albedo are significant with respect to the carbon sequestration benefits (supplementary figure 10). Moreover, the Integrative policy is still more efficient than the Traditional Policy (net) even if not as substantial as in the scenario with Africa and less intrusive than the Traditional Policy in terms of hectares used for forest mitigation actions (supplementary figure 11).
4. Discussion and policy implications
The importance of albedo implications of land use change and land management change on climate change mitigation is widely acknowledged in the scientific community (Betts 2000, Bright et al 2015, Davies-Barnard et al 2015). There is also an emerging body of economic studies assessing the trade-off between forest albedo and forest carbon sequestration; however, these studies are limited to individual stands, single species or specific regions (Thompson et al 2009, Sjølie et al 2013, Lutz and Howarth 2014, Lutz et al 2016, Mattheis and Valsta 2016).
This study contributes to the literature by providing the first global economic analysis of forests climate mitigation potential that takes account of the combined effects of timber production, carbon sequestration, and surface albedo regulation. By introducing regional and forest-specific albedo information from MODIS satellite observations in the global dynamic forestry model GTM, we compare a novel approach that values both carbon sequestration and changes in albedo (Integrative Policy) in terms of their effects on net forest climate mitigation potential, with a traditional forest sequestration program (Traditional Policy).
Our results under the Traditional Policy scenario are in the range of estimates from previous studies: under a carbon price path starting at 57 USD/tC in 2010 and reaching 540 USD/tC in 2100, global forest sequestration program could capture about 3.3 GtC-eq/yr (Smith et al 2014). Previous studies, however, have not included the albedo effect of forest actions and thus underestimated the mitigation costs.
This study suggests that by ignoring changes in albedo due to changes in land cover and forest management produces an inefficient allocation of resources (land) and higher mitigation costs. By incorporating both carbon sequestration and albedo into pricing, the Integrative Policy reduces some of the inefficiency of the Traditional Policy by shifting mitigation towards regions and activities that are less susceptible to the warming effect of changes surface albedo. Our additional simulations under alternative assumptions on forest maturity age, decay functions and land constrains further indicated that the Integrative Policy has resulted to be a more efficient mitigation than the Traditional Policy (see section 4 of the supplementary material for details).
An alternative policy approach to address this issue is to ignore forest-based mitigation activities in the areas where the associated changes in albedo are large. For instance, Griscom et al (2017) excluded all land-based mitigation actions in boreal areas. However, this approach does not explore cost-effective mitigation solutions in boreal regions, and also ignores the possible albedo implications of forest-based actions in other regions (e.g. Africa). In fact, forest mitigation costs were found to vary significantly across world regions. Under the Integrative Policy scenario, mitigation becomes much more expensive in Canada, Russia and Africa while it remains almost unchanged in the other areas. Additionally, due to the strength of the albedo effect in boreal regions, an integrative policy that includes albedo would potentially encourage land managers to convert old growth forests to younger, more heavily managed forests. However, the policy we simulate here does not consider the environmental benefits and ecosystem services that mature forest supplies, and thus highlights the need for policy makers to consider these benefits when designing incentive systems.
Although this study represents an improvement compared to the other studies assessing only the economics of carbon sequestration in the world forests, there are other factors of forest that need to be integrated to provide a complete description of the climate and economic dynamics of forests for climate change mitigation. Among biogeophysical mechanisms, our study did not consider possible changes in evapotranspiration and the interactions with albedo. This aspect will be particularly important for tropical forests where evaporative cooling effect is most pronounced (Anderson et al 2011). For instance, in Africa the inclusion of evapotranspiration could offset all or a fraction of the extra solar absorption incurred by lower albedo. Results for South America would be very different too because of the Amazon rainforest.
Some other important aspects need further study. For example, this study did not include wood demand for energy production in the forestry model. Under climate mitigation scenarios, demand for woody biomass is predicted to expand, in particular in temperate forests (Favero et al 2017). This in turn will affect the surface albedo, and influence the effective carbon price in the Integrative Policy. Moreover, our results suggest that efficient global policies would do less climate mitigation in the forestry sector, and more climate mitigation in other sectors (e.g. energy) compared to the studies by Sohngen and Mendelsohn (2003), Tavoni et al (2007), and Favero et al (2017). Future research should thus link the forestry model with albedo to an integrated assessment model to assess the implications of pricing albedo on the mitigation potential of other sectors. This study uses the offline 'back of the envelope' type calculation for albedo, and an Integrated Earth System Model (iESM) with a fully coupled ESM and human systems components is an important next step to better capture the albedo dynamics for a more consistent and accurate analysis.
Finally, climate change effects on the growth of forests, changes in dieback rate and changes in biomes could alter the results (Winckler et al 2017). Some studies suggest that climate change will increase forest stocks in many regions (e.g. Tian et al 2016, Favero et al 2018), but the effects of these changes on carbon sequestration policies is unknown. Other studies have indicated that warmer conditions favor tropical forests to expand into where temperate forests are now and temperate forests to expand into where boreal forests currently are (Mendelsohn et al 2016). This replacement may result in changes in carbon uptake and albedo affecting both the location and management of forest-based mitigation strategies. Future research should integrate climate change effects into the assessment of the optimal use of forested lands for climate change mitigation.
Author contributions
AF developed the research idea. AF and BS developed the methodological framework. YJ and YH worked on the albedo analysis and surface albedo-induced radiative forcing. AF performed the GTM model simulations. AF analyzed the results and wrote the first draft of the main manuscript. All authors edited the draft to produce the final version of the manuscript.
Acknowledgments
We thank three anonymous reviewers for their constructive comments.
Footnotes
- 4
2010 is the initial year of the DICE model simulations and the base year for the forest inventory in the GTM.