Abstract
The generation of magnetic fields of galaxies is usually described by the dynamo mechanism. This process is characterized by the Steenbeck – Krause – Rädler equation, which is the result of averaging the magnetohydrodynamics equations by distances which are associated with the size of turbulent cells in the interstellar medium. This equation is quite difficult to solve both from an analytical and numerical point of view. For galaxies, the no-z approximation is widely used. It describes the magnetic fields in thin discs. For such objects, where it is important to study the vertical structure of the field, it is not very applicable, so it is quite useful to adopt the RZ-model, which takes into account the dependence of the distance from the equatorial plane. During our research we have obtained the critical values of the dynamo number for galaxies with large half-thickness. We have also described typical z-structure for the magnetic field. Moreover, we have demonstrated that it is possible to generate dipolar magnetic fields.
Export citation and abstract BibTeX RIS
1. Introduction
It is now well-known that some spiral galaxies have magnetic fields of several microgauss (Beck et al. 1996). From an observational point of view, their existence is proved by measurements of Faraday rotation of the polarization plane of electromagnetic waves on different radio telescopes. As for the theory, the magnetic field large-scale structures are thought to be generated with the dynamo mechanism. The dynamo is based on joint action of two different effects. The first one is the alpha-effect, which describes the vorticity of interstellar turbulent motions. It is connected with transition of the angular component of the field to the radial one. The second one is differential rotation, which characterizes the nonsolid rotation of the galaxy. It is associated with transition of the radial field component to the angular one. These effects compete with the turbulent diffusion, which tries to destroy the magnetic field. So, the dynamo mechanism is a threshold effect: if the joint intensity of the alphaeffect and differential rotation is higher than the turbulent diffusion, the magnetic field will grow. But if it is less intensive than the dissipative process, the field will decay (Arshakian et al. 2009).
Usually, the magnetic field evolution is described by the Steenbeck – Krause – Rädler equation, which is a result of averaging the magnetohydrodynamics equations. Unfortunately, they are quite difficult to be solved analytically in a general way, and as for the numerical modeling, the process of the solution needs quite high computational resources. Also even if we solve the equations numerically, the results are not very transparent to be analyzed. So, it is quite important to apply some semi-analytical models, which can be useful to describe the magnetic field. As for galaxies, most of the problems are solved utilizing the so-called no-z approximation, which was developed for thin discs (Subramanian & Mestel 1993; Moss 1995; Phillips 2001). It takes into account that the magnetic field nearly lies in the equatorial plane. In addition, the z-dependence of the field is approximated by a cosine law. So, we can assume that some of the z-derivatives can be taken from the solenoidality condition, and another can be changed by algebraic expressions. It gives an opportunity to make some estimates for the magnetic field and to model it numerically employing quite modest computational resources. This model has shown efficiency for most actual problems of galaxy magnetism: it can be taken for problems connected with generation of the field in different objects. For example, it was used to describe the connection between material and magnetic arms (Moss 1998), to study the magnetic field reversals (Moss et al. 2012; Andreasyan et al. 2020) and even for magnetic fields in the outer rings of galaxies (Moss et al. 2016a). Some of the extensions of this model were applied to examine fields with helicity fluxes (Sur et al. 2007). We can also add that it can also be utilized for some objects with similar shape, such as accretion disks (Moss et al. 2016b). So, we can conclude that the no-z approximation can be regarded as the basic model to compare the results of different approaches.
Sadly, the no-z approximation cannot be used for some objects. Firstly, if we study outer rings of galaxies, the vertical length-scales cannot be assumed as a small parameter compared to radial ones. So for such objects, the torus dynamomodel has been developed (Deinzer et al. 1993; Brooke & Moss 1994; Mikhailov 2018). There are some special effects which cannot be obtained in the no-z approximation, but we can add that for some cases, even for main parts of the galaxies, we should describe the vertical structure of the field. For example, we can take the galaxies with large half-thickness of the disc. For galaxies with intensive star formation, it could be important to take into account vertical flows of the medium, which are associated with corresponding regions (Mikhailov 2014).
There have been different approaches to study the vertical structure of the galactic magnetic field. Firstly, direct numerical simulation of the equations can be used (Brandenburg 2015). This gives quite important results, but it would be better to have a simplified model which takes into account z-dependence, which is more clear for analysis and does not require large computational resources. Equations containing z-derivatives of the field were written in the early works connected with galaxy magnetism (Ruzmaikin et al. 1988; Poezd et al. 1993), but the main interest in these works was the radial dependence of the field. Also for thin discs, the approximate solution for the z-dependence was obtained (Soward 1992; Priklonsky et al. 2000). There are some models for the field in halos (Moss & Sokoloff 2008; Ferrière & Terral 2014), which describe the vertical structure of the magnetic field, but they rely on analytical approximations and ideas about the parity of the field. The model which accurately takes into account the z-structure was characterized (Chamandy et al. 2014), but it does not study the r-dependence. Of course, we should mention the models which were applied to describe the observational data (Han et al. 2018). Now there are some works which describe both r- and z-dependence (see, for example, Shukurov et al. 2019), but they expressed the magnetic field as a series expansion. Also it would be interesting to describe the poloidal field utilizing vector potential.
In this work, we construct the RZ-model for the magnetic field of galaxies. We follow the approaches which are similar to the ones employed when constructing the torus dynamo model (Deinzer et al. 1993; Brooke & Moss 1994; Mikhailov 2018). The equations are written for the φ-components of the field and vector potential, and they are solved numerically. We obtain the solutions which describe different parameters of the model and initial conditions.
2. Main Equations
The large-scale magnetic field B of galaxies (and other objects) is described by the Steenbeck – Krause – Rädler equation

where V is the large-scale velocity, η is the turbulent diffusion coefficient and α characterizes the alpha-effect.
For the magnetic field in the axisymmetric case, we can take the model (Deinzer et al. 1993)

where B is the toroidal component of the magnetic field, and A is the part of the vector potential which is connected with the poloidal field.
If we rewrite the Steenbeck – Krause – Rädler equation for every component and take into account that the poloidal component is much smaller than the toroidal one, we will obtain the following equations (Mikhailov 2018)
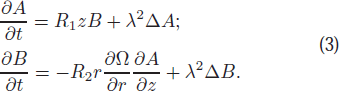
These equations are written in dimensionless form. The distances are measured in the radius of the galaxy, and time is measured in h2/η, where h is the half-thickness of the galaxy. Here are different parameters which describe the dynamo action: R1 describes the alpha-effect, R2 — the differential rotation and λ characterizes the disc thickness.
The magnetic field growth cannot be infinite, so we should take into account saturation of the field. If the magnetic field is measured in units of equipartition, the equations will be the following
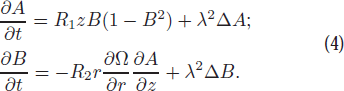
It is also interesting to study the influence of vertical flows in this model. If the velocity of vertical flow is Vz , the equations will be the following
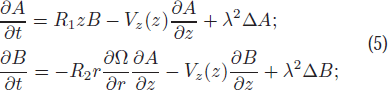
where vertical velocity Vz (z) is described with profile
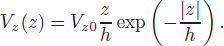
We use the following initial conditions in simulations. There is one type of condition for field A in all cases

and two different types of conditions for field B
- - quadrupole
- - dipole
3. Results
The magnetic field evolution is characterized by the dynamo-number D = R1 R2. We have ascertained that if initial conditions for field B satisfy a quadrupole, the magnetic field grows for D > 15, else it decays. As for the no-z approximation and similar models (Moss et al. 2012, 2016a), the critical value is smaller (Dcr ≈ 7), which can be explained by taking into account some new effects in our model, which are connected with vertical structure of the field. Also, we can ascribe a bit different meaning to the dynamo number in the no-z model and our approximation. In case of large values of D (such as D ≳ 800) it is possible to generate dipole field structure from dipole initial conditions. This field configuration cannot be obtained in the no-z approximation, and such time dependence is impossible, too. The evolution for different values of parameter D is displayed in Figure 1. Results of numerical simulations in the case of a nonlinear model, described by Equations (4), are depicted in Figure 2. The evolution of the magnetic field in the presence of vertical flows is presented in Figure 3. Possible configurations of the vertical structure are shown in Figure 4.
Fig. 1 The magnetic field evolution for different values of the parameter D.
Download figure:
Standard imageFig. 2 The magnetic field evolution in the nonlinear case.
Download figure:
Standard imageFig. 3 The magnetic field evolution in the model with vertical flows for different values of vertical velocity.
Download figure:
Standard imageFig. 4 Vertical structure of the magnetic field; t = 3.0.
Download figure:
Standard image4. Conclusions
We have studied the magnetic field evolution using the RZ-model. The typical field dependencies have been studied. The magnetic field can grow for values of the dynamo number which are larger than one for the no-z approximation. We have demonstrated the possibility of generating dipole magnetic field structures. The influence of vertical flows was also investigated, which cannot be done using the no-z approximation. Some such configurations are not a subject of specific interest in works which take into account the vertical structure of the field, but they demonstrate such effects as time oscillations of the magnetic fields. This is quite similar with the results obtained for a dynamo in a torus (Brooke & Moss 1994; Mikhailov 2018) and now we see that they can take place in the disk, too.
Also, we would like to emphasize that r− and z− components of the field in our model are described utilizing the vector potential, which makes the equations more convenient to solve than the equations which are written for the field components themselves (Shukurov et al. 2019). The described model can be used in different problems connected with galactic discs with large thickness. It gives us an opportunity to examine effects that cannot be described applying simpler approximations. It also can be interesting to study different objects that have similar shape, such as, for example, accretion discs (Moss et al. 2016b).
Acknowledgements
This work was supported by RFBR (Grant No.18-32-00124) and Foundation for the advancement of theoretical physics and mathematics "BASIS" (Grant 18-2-6-277-1).