ABSTRACT
High spatial resolution images at 12.5 μm of the nuclei of five nearby Seyfert galaxies—I Zwicky 1, NGC 1320, NGC 2992, M81, and NGC 7479—have been obtained with the 10 m Keck Telescope. The angular size limits indicate that under typical conditions the Keck Telescope shows an unresolved nucleus for these active galactic nuclei. In all cases, the lower limit to the infrared surface brightness is above 3 × 1012 L⊙ kpc−2; this argues that nuclear starbursts do not contribute significantly to the infrared luminosities in these nuclei.
Export citation and abstract BibTeX RIS
1. INTRODUCTION
There is much evidence (e.g., Krolik 1999) that the nuclei (i.e., the central sources) of Seyfert galaxies are powered by nonthermal processes. In the mid‐infrared, however, thermal radiation from heated dust contributes an additional emission component. This mid‐infrared radiation, at characteristic temperatures of a few hundred degrees kelvin, is thought to emerge from a disklike structure that is heated by the inner accretion disk (Sanders et al. 1989) or is in the form of a dusty torus (Antonucci 1993). The geometry of the central, presumably mainly nonthermal source is in debate, however, as is how much of the surrounding dust is heated by the central source and how much is heated by starbursts that are located throughout the nuclear region and thus form an extended cloud. It is difficult to resolve the nuclear heated dust component in direct observations using currently available telescopes, because the angular extent of the region containing the emitting dust is small. High spatial resolution observations in the mid‐infrared with 10 m class telescopes offer the possibility of resolving an extended starburst nucleus to distinguish this from more centrally concentrated emission arising from a dust‐heated central source or a nonthermal central source.
In Soifer et al. (2003, henceforth Paper 1) high spatial resolution observations made at the Keck I Telescope at 12.5 μm of three well known Seyfert galaxies—NGC 1275, NGC 4151, and NGC 7469—were presented, yielding observational upper limits on the nuclear size on the order of 0 1 or projected linear limits on the order of tens of parsecs. Furthermore, the observations of the Seyfert galaxy NGC 7469, when deconvolved, resolve a linear structure that is (< 13) × 26 pc. The orientation of the infrared emission, when compared to CO and radio observations, supports the conjecture that the mid‐infrared emission traces a disk.
The diffraction‐limited full width at half‐maximum (FWHM) of the 10 m Keck Telescope—the largest aperture telescope presently available—is 0 31 at 12.5 μm. The intrinsic seeing at the mid‐infrared wavelengths at the telescope site is often comparable to or better than this. Thus, mid‐infrared observations without adaptive optics or other special optical techniques often achieve a resolution of ≲0
3, which is important for investigating many problems. The size limits presented in Paper 1 were considered typical of what could be achieved using the Keck telescopes directly at mid‐infrared wavelengths.
In this paper we present observations of the nuclei of five more Seyfert galaxies with infrared‐bright nuclei that were observed with the 10 m Keck Telescope in the same program presented in Paper 1. These five galaxies are less well known than the three previously studied. The observations are given here to show angular size limits that can be achieved under typical conditions with the Keck Telescope. The observations were designed and optimized to probe the pointlike source, and they are not optimized to delineate low surface brightness, extended emission. We take the Hubble constant to be 75 km s−1 Mpc−1.
2. SAMPLE
The sample was chosen to consist of Seyfert galaxies which were known to be bright at mid‐infrared wavelengths and were available at the time of observation. The characteristics of the five Seyfert galaxies, taken from the literature, are shown in Table 1. It is seen that, with the exception of NGC 7479, the galaxies in the present sample are significantly fainter in the mid‐infrared than those of Paper 1. The galaxy M81 is much closer, but its far‐infrared luminosity is by far the smallest in either sample.
![]() |
3. OBSERVATIONS
The observations, as described in Paper 1, were made using the Keck Long Wavelength Spectrometer (LWS; Jones & Puetter 1993) in the imaging mode at 12.5 μm (Δλ = 1.16 μm) at the f/25 forward Cassegrain focus of the Keck I 10 m Telescope. The LWS Si:As array has 128 × 128 pixels. The scale is 0 08 pixel−1, giving a field of ∼ 10'' × 10''. A log of the observations is given in Table 2. The nights were clear and apparently photometric, with low humidity and with "seeing" stable and less than 0
5 FWHM. High‐quality photometry was not attempted; bright stars HR 8775 and/or HR 1457 were observed just one time each night to establish an approximate photometric scale.
![]() |
In the mid‐infrared, there were no infrared bright stars in the field of the object Seyfert galaxies from which to estimate profiles of the point‐source functions (PSFs). Each measurement of the object size was therefore accompanied by an observation of one of the five bright stars, identified as the PSF star in Table 2, that was near the object and of comparable infrared brightness. The observations were made by interleaving, at least five times, imaging of the Seyfert galaxy with observations of the PSF star. Observations involved nodding the telescope to two adjacent positions in the sky. At each nod position, the image was "chopped" by oscillating the secondary mirror with an amplitude of 5'' at a frequency of ∼5 Hz. For these observations, a chop‐nod scheme was utilized in which the throw of the secondary chopper was equal to the amplitude of the (telescope) nod. In order to monitor the steadiness of the "seeing," several subimages of 27 s duration each (for the PSF for NGC 2992, the duration was 13.5 s) were taken before the telescope was switched from the object to the PSF star. The number of subimages per comparison of the galaxy and of the PSF star, as well as the number of comparisons between the galaxy and the PSF star, are indicated in Table 2. The telescope was guided during the observations of the objects using a visual light guider on stars near the galaxies.
4. RESULTS AND FULL WIDTH AT HALF‐MAXIMUM ANALYSIS
To first order, the image in the mid‐infrared of each of the Seyfert galaxies is a pointlike nucleus, the same as that of the PSF star. In the analysis, each subimage of the object and of the PSF star produced by chopping at one nod position was considered independently, except in the case of M81, for which second differences were necessary to extract the signal from the background. The FWHM of both the Seyfert nucleus and the PSF star, and the relevant statistics, are given in Table 3. The photometry in a 1'' diameter beam, based on the one measurement of a photometric standard, is included in Table 4; the IRAS flux densities are repeated in Table 4 for convenience.
![]() |
![]() |
The same formalism and notation that was applied to the images in Paper 1 was applied to the five new Seyfert galaxies. An estimate of the intrinsic source size of the object was obtained by assuming the sizes add in quadrature,
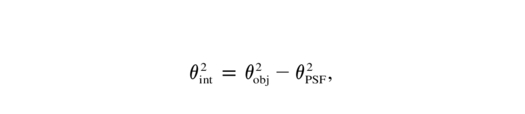
where θ int is the intrinsic source size of the object, θ PSF is the observed size of the PSF star, and θ obj is the observed object size, all expressed as FWHM. An estimate of the uncertainty can be obtained from δ θ 2int, the dispersion of θ 2int in the population of pairs. We will take the quantity θ UL = [〈 θ 2int〉 + δ θ 2int]1/2 as the estimate of the upper limit on the source size. It is seen that in September the upper limits on the angular size of the intrinsic source, as deduced from the population standard deviation in the FWHM, were ∼0 15, while in December the limits were significantly larger, ∼0
35.
5. RESULTS AND ANALYSIS OF IMAGE SELECTION
Although the fluctuations in the FWHM had a standard deviation that, applying rigorously the definition of limiting size given in Paper 1, yielded a limiting size as large as 0 35 in December, it was possible to select, by examining the FWHM of the PSF star only and not considering the galaxy FWHM in the selection, a period of time when the atmospheric turbulence was stable and relatively small during the observation of M81. For this single observation of M81, the FWHMs of the PSF star prior and subsequent to the observation of M81 were 0
33 and 0
34, while the measured FWHM second difference size of M81 was 0
36. These measurements imply an intrinsic FWHM for the nucleus of M81 of 0
12 or 2 pc, which we take as the limit on the intrinsic size. If the size of M81 in this measurement were due to unmonitored seeing variation, the limit on the size would be even smaller. The peak signal‐to‐noise ratio (S/N) of the single selected object image was ∼10, sufficient to yield a statistically significant FWHM angular size.
The "best" selected images of M81 and of three other Seyfert galaxies, where a selection of the "best" images was warranted, were deconvolved against the images of their PSF stars, thus potentially gaining a significant factor in angular resolution (see Bock et al. 2000; Paper 1). The resulting S/Ns were too low, however, to warrant refining the limits below those stated above or in Table 3.
6. DISCUSSION
From Table 3 it is seen that the limits on the angular sizes of these galaxies in the mid‐infrared is in the tenths of arcseconds range, corresponding to projected distances of tens of parsecs. No galaxy in the sample was resolved, and none showed any structure.
For M81, the closest but least luminous Seyfert nucleus in this sample, this observed limit can be compared to the size of a putative infrared cloud of grains heated by a central source. If the far‐infrared luminosity is assumed to be the bolometric luminosity, the standard relation between luminosity, distance from heating source, and temperature gives blackbody grains at a temperature of 295 K, the temperature yielding the maximum 12.5 μm luminosity, which comes to equilibrium 0.035 pc from the central source. If the grains are made of silicates, this distance, calculated using the emissivity given by Draine & Lee (1984), is increased by a factor of about 5. Parenthetically, it may be remarked that Grossan et al. (2001), from a comparison between their photometry and that of Rieke & Lebofsky (1978), observe a 25 yr variability in M81, which implies a size limit on the source of 8 pc. The crude photometry obtained here for M81 agrees with that made by Grossan et al. The size limits given in Table 3 for the other, more luminous—and more distant—Seyfert galaxies are also all significantly larger than the expected equilibrium distances.
Table 4 includes formal lower limits on the infrared surface brightness of the Seyfert nuclei, if it is assumed that the entire infrared luminosity is contained in a source of the size given as the upper limit in Table 3. The far‐infrared luminosity, which is assumed to correspond to the bolometric luminosity, was calculated by reducing the IRAS far‐infrared luminosity given in Table 1 in the ratio of the flux density measured at 12.5 μm to the flux density measured by IRAS at 12 μm. This procedure is clearly wrong but is consistent with the accuracy of the photometry. These surface brightnesses are at the very highest end of those seen in compact starburst systems (Soifer et al. 2001) and are more suggestive of active galactic nuclei power sources (Paper 1; Evans et al. 2003). These lower limits to the infrared surface brightnesses thus suggest that nuclear starbursts contribute a minor fraction to the infrared luminosities in these nuclei.
We thank the staff of the Keck Observatory for their assistance in making these observations possible. The W. M. Keck Observatory is operated as a scientific partnership between the California Institute of Technology, the University of California, and NASA. It was made possible by the generous financial support of the W. M. Keck Foundation. We extend special thanks to those of Hawaiian ancestry on whose sacred mountain we are privileged to be guests. Without their generous hospitality, none of the observations presented herein would have been possible. B. T. S. and E. E. were supported by grants from the NSF and NASA. B. T. S. and L. A. are supported by the Spitzer Science Center at Caltech. Spitzer is carried out at the Jet Propulsion Laboratory (JPL), which is operated by Caltech under an agreement with NASA. This research has made use of the NASA/IPAC Extragalactic Database, which is operated by JPL, California Institute of Technology, under contract with NASA.