Book contents
- Frontmatter
- Contents
- List of Figures
- List of Tables
- List of Contributors
- Introduction
- PART I ADVANCES IN GPCR PROTEIN RESEARCH
- PART II OLIGOMERIZATION OF GPCRS
- PART III GPCR SIGNALING FEATURES
- 7 G protein functions identified using genetic mouse models
- 8 Kinetics of GPCR, G protein, and effector activation
- 9 RGS-RhoGEFs and other RGS multidomain proteins as effector molecules in GPCR-dependent and GPCR-independent cell signaling
- 10 Adenylyl cyclase isoform-specific signaling of GPCRs
- 11 G protein-independent and β arrestin-dependent GPCR signaling
- 12 Assays to read GPCR modulation and signaling
- PART IV LIGAND PHARMACOLOGY OF GPCRS
- PART V PHYSIOLOGICAL FUNCTIONS AND DRUG TARGETING OF GPCRS
- Index
- References
11 - G protein-independent and β arrestin-dependent GPCR signaling
from PART III - GPCR SIGNALING FEATURES
Published online by Cambridge University Press: 05 June 2012
- Frontmatter
- Contents
- List of Figures
- List of Tables
- List of Contributors
- Introduction
- PART I ADVANCES IN GPCR PROTEIN RESEARCH
- PART II OLIGOMERIZATION OF GPCRS
- PART III GPCR SIGNALING FEATURES
- 7 G protein functions identified using genetic mouse models
- 8 Kinetics of GPCR, G protein, and effector activation
- 9 RGS-RhoGEFs and other RGS multidomain proteins as effector molecules in GPCR-dependent and GPCR-independent cell signaling
- 10 Adenylyl cyclase isoform-specific signaling of GPCRs
- 11 G protein-independent and β arrestin-dependent GPCR signaling
- 12 Assays to read GPCR modulation and signaling
- PART IV LIGAND PHARMACOLOGY OF GPCRS
- PART V PHYSIOLOGICAL FUNCTIONS AND DRUG TARGETING OF GPCRS
- Index
- References
Summary
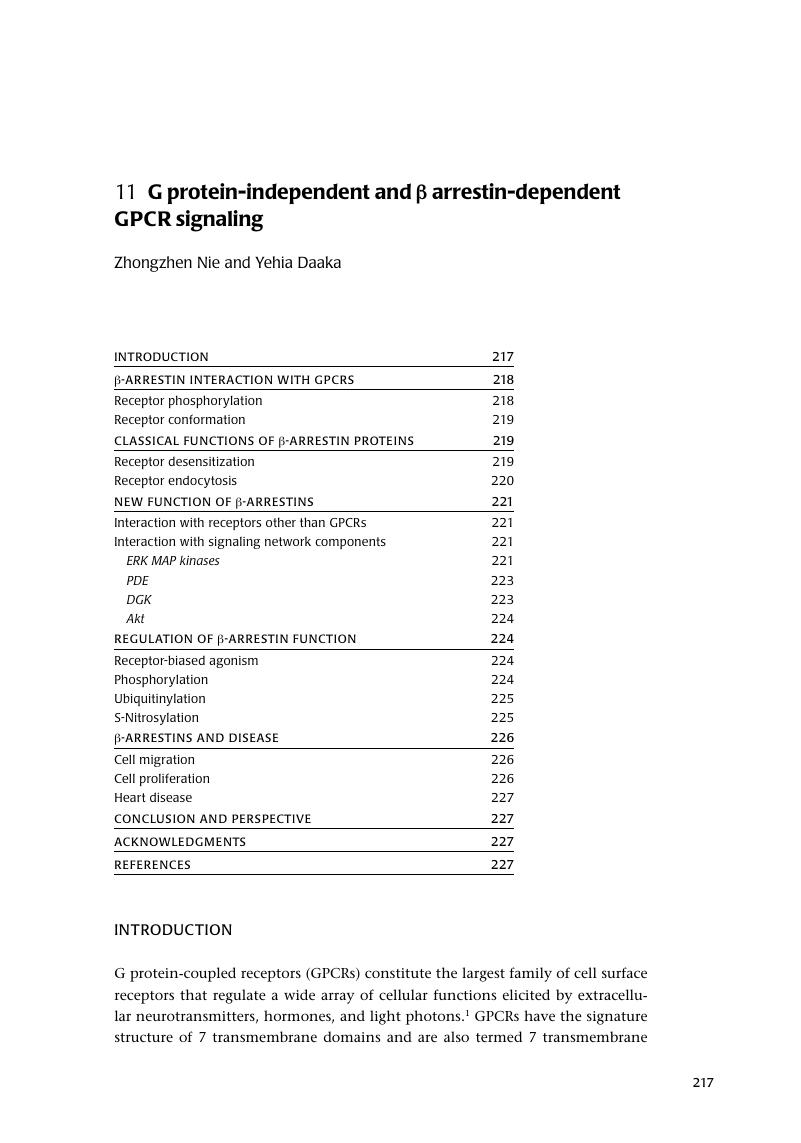
- Type
- Chapter
- Information
- G Protein-Coupled ReceptorsStructure, Signaling, and Physiology, pp. 217 - 230Publisher: Cambridge University PressPrint publication year: 2010