Abstract
Geothermal energy has been widely proposed as a potential renewable energy to replace traditional fossil fuel energy. Hot dry rock (HDR) reservoir which contains abundant geothermal energy widely distributes in China. The Gonghe Basin in Northwest China is chosen to develop the Chinese first HDR field operation project. HDR is a low-permeability, high temperature and hard granite without fluid. Developing HDR requires water cyclically flowing between injection and production wells to extract heat energy. Hydraulic fracturing, as a key reservoir stimulation technology, can create the path of fluid cyclically flowing. However, few studies have investigated hydraulic induced artificial fractures in HDR geothermal formations. This paper investigated HDR geothermal reservoir stimulation characteristics and fracture patterns during hydraulic fracturing. Reservoir stimulation was conducted with a true triaxial hydraulic fracturing apparatus which could establish a real HDR formation environment in the laboratory. The factors affecting breakdown pressure and fracture creation were investigated via experiments and numerical simulations. This study could be used to evaluate and design reservoir stimulation in field HDR geothermal operation.

















Similar content being viewed by others
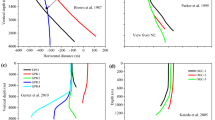
References
AB SK (2010) Data report for the safety assessment SR-Site. Swedish Nuclear Fuel and Waste Management Co., Swedish
Al-Busaidi A, Hazzard JF, Young RP (2005) Distinct element modeling of hydraulically fractured Lac du Bonnet granite. J Geophys Res-Sol Ea. https://doi.org/10.1029/2004jb003297
Al-Shayea N, Khan K, Abduljauwad S (2000) Effects of confining pressure and temperature on mixed-mode (I–II) fracture toughness of a limestone rock. J Int J Rock Mechan Mining Sci 37:629–643
Alm O, Jaktlund L-L, Shaoquan KJ (1985) The influence of microcrack density on the elastic and fracture mechanical properties of Stripa granite. Phys Earth Planet in 40:161–179
Barbier E (2002) Geothermal Energy Technology and Current Status: an Overview. Renew Sust Energ Rev 6:3–65
Baujard C et al (2017) Hydrothermal characterization of wells GRT-1 and GRT-2 in Rittershoffen, France: Implications on the understanding of natural flow systems in the Rhine. Graben Geothermics 65:255–268
Bayer P, Attard G, Blum P, Menberg K (2019) The geothermal potential of cities. Renew Sustain Energy Rev 106:17–30
Bennour Z et al (2015) Crack extension in hydraulic fracturing of shale cores using viscous oil, water, and liquid carbon dioxide. Rock Mech Rock Eng 48:1463–1473
Bertani R (2012) Geothermal power generation in the world 2005–2010 update report. Geothermics 41:1–29
Bing H, Mian C, Zhimeng L, Yonghui W, Ce D (2014) Propagation area evaluation of hydraulic fracture networks in shale gas reservoirs. Petrol Explor Dev+ 41:833–838
Bohloli B, de Pater CJ (2006) Experimental study on hydraulic fracturing of soft rocks: influence of fluid rheology and confining stress. J Petrol Sci Eng 53:1–12. https://doi.org/10.1016/j.petrol.2006.01.009
Brantut N, Heap MJ, Meredith PG, Baud P (2013) Time-dependent cracking and brittle creep in crustal rocks: a review. J Struct Geol 52:17–43. https://doi.org/10.1016/j.jsg.2013.03.007
Cai M (2010) Practical estimates of tensile strength and hoek-brown strength parameter m(i) of brittle rocks. Rock Mech Rock Eng 43:167–184. https://doi.org/10.1007/s00603-009-0053-1
Chitrala Y, Moreno C, Sondergeld C, Rai C (2013) An experimental investigation into hydraulic fracture propagation under different applied stresses in tight sands using acoustic emissions. J Petrol Sci Eng 108:151–161. https://doi.org/10.1016/j.petrol.2013.01.002
Cho N, Martin D, Sego DC (2007) A clumped particle model for rock. Int J Rock Mechan Mining Sci 44:997–1010
Cook B, Lee M, DiGiovanni A, Bronowski D, Perkins E, Williams J (2004) Discrete element modeling applied to laboratory simulation of near-wellbore mechanics J. Int J Geomech 4:19–27
de Lemos JASV (1987) A distinct element model for dynamic analysis of jointed rock with application to dam foundations and fault motion. University Microfilms
Dwivedi RD, Goel RK, Prasad VVR, Sinha A (2008) Thermo-mechanical properties of Indian and other granites. Int J Rock Mech Min Sci 45:303–315. https://doi.org/10.1016/j.ijrmms.2007.05.008
Fallahzadeh S, Hossain M, James Cornwell A, Rasouli V (2017) Near wellbore hydraulic fracture propagation from perforations in tight rocks: the roles of fracturing fluid viscosity and injection rate. Energies. https://doi.org/10.3390/en10030359
Fan T-g, Zhang G-q (2014a) Laboratory investigation of hydraulic fracture networks in formations with continuous orthogonal fractures. Energy 74:164–173. https://doi.org/10.1016/j.energy.2014.05.037
Faoro I, Vinciguerra S, Marone C, Elsworth D, Schubnel A (2013) Linking permeability to crack density evolution in thermally stressed rocks under cyclic loading. Geophys Res Lett 40:2590–2595
Frash LP, Gutierrez M, Hampton J (2014) True-triaxial apparatus for simulation of hydraulically fractured multi-borehole hot dry rock reservoirs. Int J Rock Mech Min Sci 70:496–506. https://doi.org/10.1016/j.ijrmms.2014.05.017
Frash LP, Gutierrez M, Hampton J, Hood J (2015) Laboratory simulation of binary and triple well EGS in large granite blocks using AE events for drilling guidance. Geothermics 55:1–15
Fu W, Ames BC, Bunger AP, Savitski A (2016) Impact of partially cemented and non-persistent natural fractures on hydraulic fracture propagation. Rock Mech Rock Eng 49:4519–4526
Funatsu T, Kuruppu M, Matsui K (2014) Effects of temperature and confining pressure on mixed-mode (I- II) and mode II fracture toughness of Kimachi sandstone. Int J Rock Mechan Mining Sci 67:1–8. https://doi.org/10.1016/j.ijrmms.2013.12.009
Griffiths L, Heap M, Baud P, Schmittbuhl J (2017) Quantification of microcrack characteristics and implications for stiffness and strength of granite. Int J Rock Mech Min Sci 100:138–150
Griffiths L, Lengline O, Heap MJ, Baud P, Schmittbuhl J (2018) Thermal cracking in westerly granite monitored using direct wave velocity. Coda Wave Interferometry, and Acoustic Emissions J Geophys Res-Sol Ea 123:2246–2261. https://doi.org/10.1002/2017jb015191
Guo F, Morgenstern N, Scott J (1993) Interpretation of hydraulic fracturing breakdown pressure. Int J Rock Mech Min 30:617–626
Haimson B, Fairhurst C (1967) Initiation and extension of hydraulic fractures in rocks. Soc Petrol Eng J 7:310–318
Han Z, Zhou J, Zhang L (2018) Influence of grain size heterogeneity and in-situ stress on the hydraulic fracturing process by PFC2D modelling. Energies 11:1413
Hanano M (2004) Contribution of fractures to formation and production of geothermal resources. Renew Sustain Energy Rev 8:223–236
Hazzard JF, Young RP, Oates SJ (2002) Numerical modeling of seismicity induced by fluid injection in a fractured reservoir. In: Proceedings of the 5th North American rock mechanics symposium mining and tunnel innovation and opportunity, Toronto. Citeseer, pp 1023–1030
He J, Lin C, Li X, Wan X (2016) Experimental investigation of crack extension patterns in hydraulic fracturing with shale sandstone and granite cores. Energies. https://doi.org/10.3390/en9121018
Höekmark H, Faelth B, Wallroth T (2006) THM couplings in rock. Overview of results of importance to the SR-Can safety assessment. Swedish Nuclear Fuel and Waste Management Co., Swedish
Hofmann H, Babadagli T, Zimmermann G (2014) Numerical simulation of complex fracture network development by hydraulic fracturing in naturally fractured ultratight formations. J Energ Resour-Asme. https://doi.org/10.1115/1.4028690
Hökmark H, Lönnqvist M, Fälth B (2010) THM-issues in repository rock. Thermal, mechanical, thermo-mechanical and hydro-mechanical evolution of the rock at the Forsmark and Laxemar sites. Swedish Nuclear Fuel and Waste Management Co
Homand-Etienne F, Houpert R (1989) Thermally induced microcracking in granites: characterization and analysis. Int J Rock Mech Min 26:125–134
Hossain MM, Rahman MK, Rahman SS (2000) Hydraulic fracture initiation and propagation: roles of wellbore trajectory, perforation and stress regimes. J Petrol Sci Eng 27:129–149. https://doi.org/10.1016/S0920-4105(00)00056-5
Howard GC, Fast C (1957) Optimum fluid characteristics for fracture extension. In: Drilling and production practice, New York, vol API-57–261. American Petroleum Institute, p 10
Hubbert MK, Willis DG (1957) Mechanics of Hydraulic Fracturing. Petroleum Transactions AIME 210:153–163
Ishida T, Chen Q, Mizuta Y, Roegiers J-C (2004) Influence of fluid viscosity on the hydraulic fracturing mechanism. J Energ Resour-Asme 126:190–200
Ivars DM, Pierce ME, Darcel C, Reyes-Montes J, Potyondy DO, Young RP, Cundall PA (2011) The synthetic rock mass approach for jointed rock mass modelling. Int J Rock Mechan Mining Sci 48:219–244
Jansen D, Carlson S, Young R, Hutchins D (1993) Ultrasonic imaging and acoustic emission monitoring of thermally induced microcracks in Lac du Bonnet granite . J Geophys Res Solid Earth 98:22231–22243
Jeffrey RG, Chen ZR, Zhang X, Bunger AP, Mills KW (2015) Measurement and analysis of full-scale hydraulic fracture initiation and reorientation. Rock Mech Rock Eng 48:2497–2512. https://doi.org/10.1007/s00603-015-0846-3
Jin X, Shah SN, Roegiers J-C, Hou B (2013) Breakdown pressure determination-a fracture mechanics approach. In: SPE Annual Technical Conference and Exhibition. Society of Petroleum Engineers
Kreith F, Black WZ (1980) Basic heat transfer. Harper & Row New York
Kresse O, Weng X, Chuprakov D, Prioul R, Cohen C (2013) Effect of flow rate and viscosity on complex fracture development in UFM model. In: ISRM International Conference for Effective and Sustainable Hydraulic Fracturing. International Society for Rock Mechanics and Rock Engineering
Kumari WGP, Ranjith PG, Perera MBA, Chen BK, Abdulagatov IM (2017a) Temperature-dependent mechanical behaviour of Australian Strathbogie granite with different cooling treatments. Eng Geol 229:31–44. https://doi.org/10.1016/j.enggeo.2017.09.012
Kumari WGP et al (2017b) Mechanical behaviour of Australian Strathbogie granite under in-situ stress and temperature conditions: an application to geothermal energy extraction. Geothermics 65:44–59. https://doi.org/10.1016/j.geothermics.2016.07.002
Lei Z et al (2019) Exploratory research into the enhanced geothermal system power generation project: the Qiabuqia geothermal field Northwest China. Renew Energ 139:52–70
Lemos J, Lorig L (1990) Hydromechanical modelling of jointed rock masses using the distinct element method. In: International conference on mechanics of jointed and faulted rock. pp 605–612
Li L, Xia Y, Huang B, Zhang L, Li M, Li A (2016) The behaviour of fracture growth in sedimentary rocks: a numerical study based on hydraulic fracturing processes. Energies. https://doi.org/10.3390/en9030169
Lian ZH, Yu H, Lin TJ, Guo JH (2015) A study on casing deformation failure during multi-stage hydraulic fracturing for the stimulated reservoir volume of horizontal shale wells. J Nat Gas Sci Eng 23:538–546. https://doi.org/10.1016/j.jngse.2015.02.028
Lin C, He JM, Li X, Wan XL, Zheng B (2017) An experimental investigation into the effects of the anisotropy of shale on hydraulic fracture propagation. Rock Mech Rock Eng 50:543–554. https://doi.org/10.1007/s00603-016-1136-4
Lu S-M (2018) A global review of enhanced geothermal system (EGS). Renew Sust Energ Rev 81:2902–2921
Lund JW, Boyd TL (2016) Direct utilization of geothermal energy 2015 worldwide review. Geothermics 60:66–93
Ma Y, Zhang Y, Huang Y, Zhang Y, Hu Z (2019) Experimental study on flow and heat transfer characteristics of water flowing through a rock fracture induced by hydraulic fracturing for an enhanced geothermal system. Appl Therm Eng 154:433–441
Ma X, Zoback MD (2017) Laboratory experiments simulating poroelastic stress changes associated with depletion and injection in low-porosity sedimentary rocks . J Geophys Res Solid Earth 122:2478–2503. https://doi.org/10.1002/2016jb013668
Magraner T, Montero A, Quilis S (2010) Comparison between design and actual energy performance of a HVAC-ground coupled heat pump system in cooling and heating operation. Energ Buildings 42:1394–1401
Moya D, Aldás C, Kaparaju P (2018) Geothermal energy: Power plant technology and direct heat applications. Renew Sustain Energy Rev 94:889–901
Nagel NB, Gil I, Sanchez-Nagel M, Damjanac B (2011) Simulating hydraulic fracturing in real fractured rocks-overcjavascript: Iterm () oming the limits of pseudo3D models. In: SPE Hydraulic Fracturing Technology Conference. Society of Petroleum Engineers
Nasehi MJ, Mortazavi A (2013) Effects of in-situ stress regime and intact rock strength parameters on the hydraulic fracturing. J Petrol Sci Eng 108:211–221
Nasseri MHB, Schubnel A, Young RP (2007) Coupled evolutions of fracture toughness and elastic wave velocities at high crack density in thermally treated Westerly granite. Int J Rock Mech Min Sci 44:601–616. https://doi.org/10.1016/j.ijrmms.2006.09.008
Olasolo P, Juárez M, Morales M, Liarte I (2016) Enhanced geothermal systems (EGS): a review. Renewable Sustainable Energy Rev 56:133–144
Olson J (2008) Multi-fracture propagation modeling: Applications to hydraulic fracturing in shales and tight gas sands. In: The 42nd US rock mechanics symposium (USRMS). American Rock Mechanics Association,
Papanastasiou P (1999) The effective fracture toughness in hydraulic fracturing. Int J Fracture 96:127–147. https://doi.org/10.1023/A:1018676212444
Perkins T, Kern LR (1961) Widths of hydraulic fractures. J Petrol Technol 13:937–949
Potluri N, Zhu D, Hill AD (2005) The effect of natural fractures on hydraulic fracture propagation. In: SPE European formation damage conference, 2005. Society of Petroleum Engineers
Rutqvist J, Tsang C-F (2008) Review of SKB's Work on Coupled THM Processes within SR-Can. External review contribution in support of SKI's and SSI's review of SR-Can. Swedish Nuclear Power Inspectorate, Swedish
Shao S (2015) Coupled Thermo-Hydro-Mechanical (THM) Behaviour of Rock Relevant to the Geothermal Industry. Monash University
Shimizu H, Murata S, Ishida T (2011) The distinct element analysis for hydraulic fracturing in hard rock considering fluid viscosity and particle size distribution. J Int J Rock Mechan Mining Sci 48:712–727
Solberg P, Lockner D, Pure JBJ, Geophysics A (1977) Shear and tension hydraulic fractures in low permeability rocks pure. Appl Geophys 115:191–198
Song I, Suh M, Won KS, Haimson B (2001) A laboratory study of hydraulic fracturing breakdown pressure in tablerock sandstone J. Geosci J 5:263–271
Thallak S, Rothenburg L, Dusseault M (1991) Simulation of multiple hydraulic fractures in a discrete element system. Paper presented at The 32nd US Symposium on Rock Mechanics (USRMS)
Tomac I, Gutierrez M (2017) Coupled hydro-thermo-mechanical modeling of hydraulic fracturing in quasi-brittle rocks using BPM-DEM . J Rock Mechan Geotech Eng 9:92–104
Tomac I, Sauter M (2018) A review on challenges in the assessment of geomechanical rock performance for deep geothermal reservoir development. Renew Sustain Energy Rev 82:3972–3980
Wang T, Zhou WB, Chen JH, Xiao X, Li Y, Zhao XY (2014) Simulation of hydraulic fracturing using particle flow method and application in a coal mine. Int J Coal Geol 121:1–13. https://doi.org/10.1016/j.coal.2013.10.012
Wang Y, Li X, He J, Zhao Z, Zheng B (2016) Investigation of fracturing network propagation in random naturally fractured and laminated block experiments. Energies. https://doi.org/10.3390/en9080588
Wanne T, Young R (2008) Bonded-particle modeling of thermally fractured granite. Int J Rock Mech Min Sci 45:789–799
Warpinski NR, Schmidt RA, Northrop DA (1982) In-situ stresses: the predominant influence on hydraulic fracture containment J. J Petrol Technol 34:653–664
Wong TF, Brace W (1979) Thermal expansion of rocks: some measurements at high pressure. Tectonophysics 57:95–117
Xie LM, Min KB (2017) Hydraulic fracturing initiation and propagation in deep inclined open hole for Enhanced Geothermal System. Geothermics 70:351–366. https://doi.org/10.1016/j.geothermics.2017.07.007
Xu T, Zhou GL, Heap MJ, Zhu WC, Chen CF, Baud P (2017) The influence of temperature on time-dependent deformation and failure in granite: a mesoscale modeling approach. Rock Mech Rock Eng 50:2345–2364. https://doi.org/10.1007/s00603-017-1228-9
Yin T, Zhang S, Li X, Bai L (2018a) A numerical estimate method of dynamic fracture initiation toughness of rock under high-temperature. Eng Fract Mech 204:87–102
Yoon JS, Zang A, Stephansson O (2014) Numerical investigation on optimized stimulation of intact and naturally fractured deep geothermal reservoirs using hydro-mechanical coupled discrete particles joints model. Geothermics 52:165–184
Yoon JS, Zimmermann G, Zang A (2015) Numerical investigation on stress shadowing in fluid injection-induced fracture propagation in naturally fractured geothermal reservoirs. Rock Mech Rock Eng 48:1439–1454
Zarrouk SJ, Moon H (2014) Efficiency of geothermal power plants: a worldwide review. Geothermics 51:142–153
Zhang WQ, Sun Q, Hao SQ, Geng JS, Lv C (2016) Experimental study on the variation of physical and mechanical properties of rock after high-temperature treatment. Appl Therm Eng 98:1297–1304. https://doi.org/10.1016/j.applthermaleng.2016.01.010
Zhang YS, Zhang JC, Yuan B, Yin SX (2018) In-situ stresses controlling hydraulic fracture propagation and fracture breakdown pressure. J Petrol Sci Eng 164:164–173. https://doi.org/10.1016/j.petrol.2018.01.050
Zhao Z (2016) Thermal influence on mechanical properties of granite: a microcracking perspective. Rock Eng Rock Mechan Str Rock Masses 49:747–762
Zhou J, Chen M, Jin Y, Zhang GQ (2008) Analysis of fracture propagation behavior and fracture geometry using a tri-axial fracturing system in naturally fractured reservoirs. Int J Rock Mechan Mining Sci 45:1143–1152
Zhou J, Zhang L, Braun A, Han Z (2016) Numerical modeling and investigation of fluid-driven fracture propagation in reservoirs based on a modified fluid-mechanically coupled model in two-dimensional particle flow code. Energies 9:699
Zhou J, Zhang L, Braun A, Han Z (2017a) Investigation of processes of interaction between hydraulic and natural fractures by PFC modeling comparing against laboratory experiments and analytical models. Energies. https://doi.org/10.3390/en10071001
Zhou J, Zhang L, Braun A, Han Z (2017b) Investigation of processes of interaction between hydraulic and natural fractures by PFC modeling comparing against laboratory experiments and analytical models. Energies 10:1001
Zhou J, Zhang L, Pan Z, Han Z (2016b) Numerical investigation of fluid-driven near-borehole fracture propagation in laminated reservoir rock using PFC2D. J Natural Gas Sci Eng 36:719–733
Zhou J, Zhang L, Pan Z, Han Z (2016c) Numerical investigation of fluid-driven near-borehole fracture propagation in laminated reservoir rock using PFC2D. J Nat Gas Sci Eng 36:719–733
Zoback M, Rummel F, Jung R, Raleigh C (1977) Laboratory hydraulic fracturing experiments in intact and pre-fractured rock. Int J Rock Mechan Mining Sci Geomechan 14:49–58
Acknowledgements
This research was funded by the National Key Research and Development Program of China (NO. 2018YFB1501803), the National Natural Science Foundation of China (No.41772238).
Author information
Authors and Affiliations
Corresponding author
Additional information
Publisher's Note
Springer Nature remains neutral with regard to jurisdictional claims in published maps and institutional affiliations.
Appendix
Appendix
The Figure Appendix Figs.
12,
13,
14,
15 and
16 could help readers understand this study better. Figure 12 shows the field sampling and processed granite samples. Figure 13 shows the sealing measures at the contact between the rock sample and water inlet. Figure 14 a shows a fractured rock sample, the red fluid flows out along the induced fractures, Fig. 13 b shows a cut rock sample. Figure 15 shows the Brazilian tensile strength results of the granite at different temperatures. Figure 16 shows the relationship of stress and aperture of the granite in Gonghe Basin.
Figure 12 a shows the field sampling in Gonghe Basin, the granite outcrop (Fig. 1a) is processed into 300 × 300 × 300 mm cubes (Fig. 1b) for laboratorial hydraulic fracturing experiment.
Figure 13 a shows the water outlet hole in the flat jack. Figure 2b shows the rubber ring and the Poly Tetra Fluoroethylene (PTFE) on the injection tube head.
Figure 14 a shows a rock sample after hydraulic fracturing. Figure 14 b shows that a rock sample was cut into two parts.
Figure 15 shows the Brazilian tensile strength results of the granite at different temperatures. With the increase of temperature, the Brazilian tensile strength almost linearly decreases.
The validation of Eq. (2) for Gonghe site was shown in Fig. 16, which is the relationship of granite stress and aperture, the constant of – 0.15 is obtained by fitting the experimental results, then \({e}_{0}\) and \({e}_{\mathrm{inf}}\) is got, the value of \({e}_{0}\) is about 30 μm, the value of \({e}_{\mathrm{inf}}\) is about 5 μm, more detail about the relationship of stress-vs-aperture functions could be seen in our previous study (Ma et al. 2019). More validations and applications about this equation in hydraulic fracturing numerical simulation can be referred in previous researches (AB 2010; Höekmark et al. 2006; Hökmark et al. 2010; Liu et al. 2004; Rutqvist and Tsang 2008).
Rights and permissions
About this article
Cite this article
Cheng, Y., Zhang, Y., Yu, Z. et al. Investigation on Reservoir Stimulation Characteristics in Hot Dry Rock Geothermal Formations of China During Hydraulic Fracturing. Rock Mech Rock Eng 54, 3817–3845 (2021). https://doi.org/10.1007/s00603-021-02506-y
Received:
Accepted:
Published:
Issue Date:
DOI: https://doi.org/10.1007/s00603-021-02506-y