Abstract
Multiple morphological abnormalities of the flagella (MMAF) is a severe disease of male infertility, while the pathogenetic mechanisms of MMAF are still incompletely understood. Previously, we found that the deficiency of Ccdc38 might be associated with MMAF. To understand the underlying mechanism of this disease, we identified the potential partner of this protein and found that the coiled-coil domain containing 146 (CCDC146) can interact with CCDC38. It is predominantly expressed in the testes, and the knockout of this gene resulted in complete infertility in male mice but not in females. The knockout of Ccdc146 impaired spermiogenesis, mainly due to flagellum and manchette organization defects, finally led to MMAF-like phenotype. Furthermore, we demonstrated that CCDC146 could interact with both CCDC38 and CCDC42. It also interacts with intraflagellar transport (IFT) complexes IFT88 and IFT20. The knockout of this gene led to the decrease of ODF2, IFT88, and IFT20 protein levels, but did not affect CCDC38, CCDC42, or ODF1 expression. Additionally, we predicted and validated the detailed interactions between CCDC146 and CCDC38 or CCDC42, and built the interaction models at the atomic level. Our results suggest that the testis predominantly expressed gene Ccdc146 is essential for sperm flagellum biogenesis and male fertility, and its mutations might be associated with MMAF in some patients.







Similar content being viewed by others
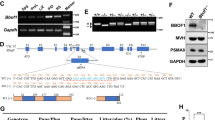
Data availability
All the data supporting the findings of this study are available from the corresponding author upon reasonable request.
References
Hwang YS et al (2020) Reconstitution of prospermatogonial specification in vitro from human induced pluripotent stem cells. Nat Commun 11(1):5656. https://doi.org/10.1038/s41467-020-19350-3
Tüttelmann F, Ruckert C, Röpke A (2018) Disorders of spermatogenesis: perspectives for novel genetic diagnostics after 20 years of unchanged routine. Med Gen 30(1):12–20. https://doi.org/10.1007/s11825-018-0181-7
Tuttelmann F, Ruckert C, Ropke A (2018) Disorders of spermatogenesis: perspectives for novel genetic diagnostics after 20 years of unchanged routine. Med Genet 30(1):12–20. https://doi.org/10.1007/s11825-018-0181-7
Coutton C et al (2015) Teratozoospermia: spotlight on the main genetic actors in the human. Hum Reprod Update 21(4):455–485. https://doi.org/10.1093/humupd/dmv020
Ray PF et al (2017) Genetic abnormalities leading to qualitative defects of sperm morphology or function. Clin Genet 91(2):217–232. https://doi.org/10.1111/cge.12905
Jiao SY, Yang YH, Chen SR (2021) Molecular genetics of infertility: loss-of-function mutations in humans and corresponding knockout/mutated mice. Hum Reprod Update 27(1):154–189. https://doi.org/10.1093/humupd/dmaa034
Fawcett DW (1975) The mammalian spermatozoon. Dev Biol 44(2):394–436. https://doi.org/10.1016/0012-1606(75)90411-x
Russell LD, Russell J, MacGregor GR, Meistrich ML (1991) Linkage of manchette microtubules to the nuclear envelope and observations of the role of the manchette in nuclear shaping during spermiogenesis in rodents. Am J Anat 192(2):97–120. https://doi.org/10.1002/aja.1001920202
Eddy EM, Toshimori K, O’Brien DA (2003) Fibrous sheath of mammalian spermatozoa. Microsc Res Tech 61(1):103–115. https://doi.org/10.1002/jemt.10320
Miyata H, Morohoshi A, Ikawa M (2020) Analysis of the sperm flagellar axoneme using gene-modified mice. Exp Anim 69(4):374–381. https://doi.org/10.1538/expanim.20-0064
Zhao W et al (2018) Outer dense fibers stabilize the axoneme to maintain sperm motility. J Cell Mol Med 22(3):1755–1768. https://doi.org/10.1111/jcmm.13457
Baltz JM, Williams P, Cone RA (1990) Dense fibers protect mammalian sperm against damage. Biol Reprod 43(3):485–491. https://doi.org/10.1095/biolreprod43.3.485
Escalier D, Toure A (2012) Morphological defects of sperm flagellum implicated in human male infertility. Med Sci 28(5):503–511. https://doi.org/10.1051/medsci/2012285015
Touré A et al (2021) The genetic architecture of morphological abnormalities of the sperm tail. Hum Genet 140(1):21–42. https://doi.org/10.1007/s00439-020-02113-x
Wang J et al (2022) Clinical detection, diagnosis and treatment of morphological abnormalities of sperm flagella: a review of literature. Front Genet 13:1034951. https://doi.org/10.3389/fgene.2022.1034951
Wang X et al (2017) Homozygous DNAH1 frameshift mutation causes multiple morphological anomalies of the sperm flagella in Chinese. Clin Genet 91(2):313–321. https://doi.org/10.1111/cge.12857c
Gao Y et al (2021) Novel bi-allelic variants in DNAH2 cause severe asthenoteratozoospermia with multiple morphological abnormalities of the flagella. Reprod Biomed 42(5):963–972. https://doi.org/10.1016/j.rbmo.2021.01.011
Lu S et al (2021) Bi-allelic variants in human WDR63 cause male infertility via abnormal inner dynein arms assembly. Cell Discov 7(1):110. https://doi.org/10.1038/s41421-021-00327-5
Tu C et al (2021) Bi-allelic mutations of DNAH10 cause primary male infertility with asthenoteratozoospermia in humans and mice. Am J Hum Genet 108(8):1466–1477. https://doi.org/10.1016/j.ajhg.2021.06.010
Tu C et al (2019) Identification of DNAH6 mutations in infertile men with multiple morphological abnormalities of the sperm flagella. Sci Rep 9(1):15864. https://doi.org/10.1038/s41598-019-52436-7
Wu H et al (2023) DNALI1 deficiency causes male infertility with severe asthenozoospermia in humans and mice by disrupting the assembly of the flagellar inner dynein arms and fibrous sheath. Cell Death Dis 14(2):127. https://doi.org/10.1038/s41419-023-05653-y
Chen D et al (2021) A novel CCDC39 mutation causes multiple morphological abnormalities of the flagella in a primary ciliary dyskinesia patient. Reprod Biomed 43(5):920–930. https://doi.org/10.1016/j.rbmo.2021.07.005
Xu Y et al (2022) Novel compound heterozygous variants in CCDC40 associated with primary ciliary dyskinesia and multiple morphological abnormalities of the sperm flagella. Pharmacogenomics Pers Med 15:341–350. https://doi.org/10.2147/PGPM.S359821
Cong J et al (2022) Homozygous mutations in CCDC34 cause male infertility with oligoasthenoteratozoospermia in humans and mice. J Med Genet 59(7):710–718. https://doi.org/10.1136/jmedgenet-2021-107919
Sha Y et al (2020) Biallelic mutations of CFAP74 may cause human primary ciliary dyskinesia and MMAF phenotype. J Hum Genet 65(11):961–969. https://doi.org/10.1038/s10038-020-0790-2
Liu S et al (2021) CFAP61 is required for sperm flagellum formation and male fertility in human and mouse. Development. https://doi.org/10.1242/dev.199805
Liu C et al (2021) Deleterious variants in X-linked CFAP47 induce asthenoteratozoospermia and primary male infertility. Am J Hum Genet 108(2):309–323. https://doi.org/10.1016/j.ajhg.2021.01.002
He X et al (2020) Bi-allelic loss-of-function variants in CFAP58 cause flagellar axoneme and mitochondrial sheath defects and asthenoteratozoospermia in humans and mice. Am J Hum Genet 107(3):514–526. https://doi.org/10.1016/j.ajhg.2020.07.010
Tian S et al (2023) Biallelic mutations in CFAP54 cause male infertility with severe MMAF and NOA. J Med Genet 60(8):827–834. https://doi.org/10.1136/jmg-2022-108887
Lu Y et al (2013) The compound heterozygous mutations of CFAP65 cause multiple morphological abnormalities of sperm flagella in infertile men. QJM. https://doi.org/10.1093/qjmed/hcad205
Beurois J et al (2019) CFAP70 mutations lead to male infertility due to severe astheno-teratozoospermia. A case report. Hum Reprod 34(10):2071–2079. https://doi.org/10.1093/humrep/dez166
Martinez G et al (2020) Biallelic variants in MAATS1 encoding CFAP91, a calmodulin-associated and spoke-associated complex protein, cause severe astheno-teratozoospermia and male infertility. J Med Genet 57(10):708–716. https://doi.org/10.1136/jmedgenet-2019-106775
Shen Q et al (2021) Bi-allelic truncating variants in CFAP206 cause male infertility in human and mouse. Hum Genet 140(9):1367–1377. https://doi.org/10.1007/s00439-021-02313-z
Auguste Y et al (2018) Loss of calmodulin- and radial-spoke-associated complex protein CFAP251 leads to immotile spermatozoa lacking mitochondria and infertility in men. Am J Hum Genet 103(3):413–420. https://doi.org/10.1016/j.ajhg.2018.07.013
Tang S et al (2017) Biallelic mutations in CFAP43 and CFAP44 cause male infertility with multiple morphological abnormalities of the sperm flagella. Am J Hum Genet 100(6):854–864. https://doi.org/10.1016/j.ajhg.2017.04.012
Dong FN et al (2018) Absence of CFAP69 causes male infertility due to multiple morphological abnormalities of the flagella in human and mouse. Am J Hum Genet 102(4):636–648. https://doi.org/10.1016/j.ajhg.2018.03.007
Lei C et al (2022) DRC1 deficiency caused primary ciliary dyskinesia and MMAF in a Chinese patient. J Hum Genet 67(4):197–201. https://doi.org/10.1038/s10038-021-00985-z
Tu C et al (2020) Novel mutations in SPEF2 causing different defects between flagella and cilia bridge: the phenotypic link between MMAF and PCD. Hum Genet 139(2):257–271. https://doi.org/10.1007/s00439-020-02110-0
Xu C et al (2022) Homozygous SPAG6 variants can induce nonsyndromic asthenoteratozoospermia with severe MMAF. Reprod Biol Endocrinol 20(1):41. https://doi.org/10.1186/s12958-022-00916-3
Zhu Z-J et al (2022) Novel mutation in ODF2 causes multiple morphological abnormalities of the sperm flagella in an infertile male. Asian J Androl 24(5):463–472. https://doi.org/10.4103/aja202183
Shen Y et al (2019) Loss-of-function mutations in QRICH2 cause male infertility with multiple morphological abnormalities of the sperm flagella. Nat Commun 10(1):433. https://doi.org/10.1038/s41467-018-08182-x
Coutton C et al (2019) Bi-allelic mutations in ARMC2 lead to severe astheno-teratozoospermia due to sperm flagellum malformations in humans and mice. Am J Hum Genet 104(2):331–340. https://doi.org/10.1016/j.ajhg.2018.12.013
Martinez G et al (2018) Whole-exome sequencing identifies mutations in FSIP2 as a recurrent cause of multiple morphological abnormalities of the sperm flagella. Hum Reprod 33(10):1973–1984. https://doi.org/10.1093/humrep/dey264
Liu C et al (2023) Homozygous variants in AKAP3 induce asthenoteratozoospermia and male infertility. J Med Genet 60(2):137–143. https://doi.org/10.1136/jmedgenet-2021-108271
Lv M et al (2020) Homozygous mutations in DZIP1 can induce asthenoteratospermia with severe MMAF. J Med Genet 57(7):445–453. https://doi.org/10.1136/jmedgenet-2019-106479
Liu W et al (2019) Bi-allelic mutations in TTC21A induce asthenoteratospermia in humans and mice. Am J Hum Genet 104(4):738–748. https://doi.org/10.1016/j.ajhg.2019.02.020
Lorès P et al (2019) Mutations in TTC29, encoding an evolutionarily conserved axonemal protein, result in asthenozoospermia and male infertility. Am J Huma Genet 105(6):1148–1167
Ni X et al (2020) A novel homozygous mutation in WDR19 induces disorganization of microtubules in sperm flagella and nonsyndromic asthenoteratospermia. J Reprod Genet 37(6):1431–1439
Lorès P et al (2021) A missense mutation in IFT74, encoding for an essential component for intraflagellar transport of Tubulin, causes asthenozoospermia and male infertility without clinical signs of Bardet-Biedl syndrome. Hum Genet 140(7):1031–1043
Martinez G et al (2023) New mutations in DNHD1 cause multiple morphological abnormalities of the sperm flagella. Int J Mol Sci. https://doi.org/10.3390/ijms24032559
Lorès P et al (2018) Homozygous missense mutation L673P in adenylate kinase 7 (AK7) leads to primary male infertility and multiple morphological anomalies of the flagella but not to primary ciliary dyskinesia. Hum Mol Genet 27(7):1196–1211
Guo T et al (2021) Bi-allelic BRWD1 variants cause male infertility with asthenoteratozoospermia and likely primary ciliary dyskinesia. Hum Genet 140(5):761–773
Ma H et al (2021) Novel frameshift mutation in STK33 is associated with asthenozoospermia and multiple morphological abnormalities of the flagella. Hum Mol Genet 30(21):1977–1984
Liu C et al (2021) Novel mutations in X-linked, USP26-induced asthenoteratozoospermia and male infertility. Cells. https://doi.org/10.3390/cells10071594
Toure A et al (2021) The genetic architecture of morphological abnormalities of the sperm tail. Hum Genet 140(1):21–42. https://doi.org/10.1007/s00439-020-02113-x
Priyanka PP, Yenugu S (2021) Coiled-coil domain-containing (CCDC) proteins: functional roles in general and male reproductive physiology. Reprod Sci 28(10):2725–2734
Kaczmarek K et al (2009) Ccdc33, a predominantly testis-expressed gene, encodes a putative peroxisomal protein. Cytogenet Genome Res 126(3):243–252
Song M-H et al (2012) KP-CoT-23 (CCDC83) is a novel immunogenic cancer/testis antigen in colon cancer. Int J Oncol 41(5):1820–1826
Zhang R et al (2022) CCDC38 is required for sperm flagellum biogenesis and male fertility in mice. Development. https://doi.org/10.1242/dev.200516
Chen J-b et al (2016) Expression characteristics of the Ccdc70 gene in the mouse testis during spermatogenesis. Zhonghua Nan Ke Xue 22(1):12–16
Vandenbrouck Y, Pineau C, Lane L (2020) The functionally unannotated proteome of human male tissues: a shared resource to uncover new protein functions associated with reproductive biology. J Proteome Res 19(12):4782–4794
Khan M et al (2018) The evolutionarily conserved genes: Tex37, Ccdc73, Prss55 and Nxt2 are dispensable for fertility in mice. Sci Reports 8(1):4975
Wang T et al (2018) Ccdc87 is critical for sperm function and male fertility. Biol Reprod 99(4):817–827
Imsland F et al (2012) The Rose-comb mutation in chickens constitutes a structural rearrangement causing both altered comb morphology and defective sperm motility. PLoS Genet 8(6):e1002775
Sarkar S et al (2022) Histone methylation regulates gene expression in the round spermatids to set the RNA payloads of sperm. Reproductive Sci 29(3):857–882. https://doi.org/10.1007/s43032-021-00837-3
Wang M et al (2023) CCDC189 affects sperm flagellum formation by interacting with CABCOCO1. Nat Sci Rev 10(9):nwad181. https://doi.org/10.1093/nsr/nwad181
Burkhard P, Sergei J, Strelkov SV (2001) Coiled coils: a highly versatile protein folding motif. Trends Cell Biol 11(2):82–88. https://doi.org/10.1016/s0962-8924(00)01898-5
Lupas AN, Bassler J, Dunin-Horkawicz S (2017) The structure and topology of alpha-helical coiled coils. Subcell Biochem 82:95–129. https://doi.org/10.1007/978-3-319-49674-0_4
Rose A et al (2005) Coiled-coil protein composition of 22 proteomes–differences and common themes in subcellular infrastructure and traffic control. BMC Evol Biol 5:66. https://doi.org/10.1186/1471-2148-5-66
Truebestein L, Leonard TA (2016) Coiled-coils: the long and short of it. BioEssays 38(9):903–916. https://doi.org/10.1002/bies.201600062
Pasek RC et al (2016) Coiled-coil domain containing 42 (Ccdc42) is necessary for proper sperm development and male fertility in the mouse. Dev Biol 412(2):208–218. https://doi.org/10.1016/j.ydbio.2016.01.042
Zhang R, W B, Liu C, Zhang Z, Wang X, Wang L, Xiao S, Chen Y, Wei H, Jiang H, Gao F, Yuan L, Li W (2022) CCDC38 is required for sperm flagellum biogenesis and male fertility in mice. Development 149(11):200516. https://doi.org/10.1242/dev.200516
Tapia Contreras C, Hoyer-Fender S (2019) CCDC42 localizes to manchette, HTCA and tail and interacts with ODF1 and ODF2 in the formation of the male germ cell cytoskeleton. Front Cell Dev Biol. 7:151. https://doi.org/10.3389/fcell.2019.00151
Firat-Karalar EN, Stearns T (2014) The centriole duplication cycle. Philos Trans R Soc Lond B Biol Sci. https://doi.org/10.1098/rstb.2013.0460
Lehti MS, Sironen A (2016) Formation and function of the manchette and flagellum during spermatogenesis. Reproduction 151(4):R43-54. https://doi.org/10.1530/REP-15-0310
Kierszenbaum AL, Rivkin E, Tres LL (2003) Acroplaxome, an F-actin-keratin-containing plate, anchors the acrosome to the nucleus during shaping of the spermatid head. Mol Biol Cell 14(11):4628–4640. https://doi.org/10.1091/mbc.e03-04-0226
Wei YL, Yang WX (2018) The acroframosome-acroplaxome-manchette axis may function in sperm head shaping and male fertility. Gene 660:28–40. https://doi.org/10.1016/j.gene.2018.03.059
Piperno GFM (1985) Monoclonal antibodies specific for an acetylated form of a-tubulin recognize the antigen in cilia and flagella from a variety of organisms. J Cell Biol 101(6):2085–2094
Symon A, Harley V (2017) SOX9: a genomic view of tissue specific expression and action. Int J Biochem Cell Biol 87:18–22. https://doi.org/10.1016/j.biocel.2017.03.005
Yan Y et al (2020) The HDOCK server for integrated protein-protein docking. Nat Protoc 15(5):1829–1852. https://doi.org/10.1038/s41596-020-0312-x
Pettersen EF et al (2004) UCSF Chimera–a visualization system for exploratory research and analysis. J Comput Chem 25(13):1605–1612. https://doi.org/10.1002/jcc.20084
Gui M et al (2022) SPACA9 is a lumenal protein of human ciliary singlet and doublet microtubules. Proc Natl Acad Sci United States Am 119(41):e2207605119
Cho EH et al (2020) A nonsense variant in NME5 causes human primary ciliary dyskinesia with radial spoke defects. Clin Genet 98(1):64–68. https://doi.org/10.1111/cge.13742
Zhang X et al (2022) Differential requirements of IQUB for the assembly of radial spoke 1 and the motility of mouse cilia and flagella. Cell Rep 41(8):111683. https://doi.org/10.1016/j.celrep.2022.111683
Sapiro R et al (2002) Male infertility, impaired sperm motility, and hydrocephalus in mice deficient in sperm-associated antigen 6. Mol Cell Biol 22(17):6298–6305
Zhang Z et al (2006) Deficiency of SPAG16L causes male infertility associated with impaired sperm motility. Biol Reprod 74(4):751–759
Gaffney EA et al (2011) Mammalian sperm motility: observation and theory. Ann Rev Fluid Mech 43(1):501–528. https://doi.org/10.1146/annurev-fluid-121108-145442
Lehti MS, Sironen A (2017) Formation and function of sperm tail structures in association with sperm motility defects. Biol Reprod 97(4):522–536. https://doi.org/10.1093/biolre/iox096
Sun X et al (2011) The role of actin and myosin during spermatogenesis. Mol Biol Rep 38(6):3993–4001. https://doi.org/10.1007/s11033-010-0517-0
Roy A et al (2007) Absence of tektin 4 causes asthenozoospermia and subfertility in male mice. FASEB J 21(4):1013–1025. https://doi.org/10.1096/fj.06-7035com
Donkor FF et al (2004) Outer dense fibre protein 2 (ODF2) is a self-interacting centrosomal protein with affinity for microtubules. J Cell Sci 117(20):4643–4651. https://doi.org/10.1242/jcs.01303
Young SAM et al (2016) CABYR is essential for fibrous sheath integrity and progressive motility in mouse spermatozoa. J Cell Sci 129(23):4379–4387. https://doi.org/10.1242/jcs.193151
Moretti E et al (2007) Localization of AKAP4 and tubulin proteins in sperm with reduced motility. Asian J Androl 9(5):641–649. https://doi.org/10.1111/j.1745-7262.2007.00267.x
Fang X et al (2021) Hypomorphic and hypermorphic mouse models of Fsip2 indicate its dosage-dependent roles in sperm tail and acrosome formation. Development. https://doi.org/10.1242/dev.199216
Fiedler SE et al (2013) Loss of R2D2 proteins ROPN1 and ROPN1L causes defects in murine sperm motility, phosphorylation, and fibrous sheath integrity. Biol Reprod 88(2):41. https://doi.org/10.1095/biolreprod.112.105262
San Agustin JT, Pazour GJ, Witman GB (2015) Intraflagellar transport is essential for mammalian spermiogenesis but is absent in mature sperm. Mol Biol Cell 26(24):4358–4372
O’Donnell L, O’Bryan MK (2014) Microtubules and spermatogenesis. Semin Cell Dev Biol 30:45–54. https://doi.org/10.1016/j.semcdb.2014.01.003
Kierszenbaum AL, Rivkin E, Tres LL (2011) Cytoskeletal track selection during cargo transport spermatids is relevant to male fertility. Spermatogenesis 1(3):221–230. https://doi.org/10.4161/spmg.1.3.18018
Kumar S et al (2018) MEGA X: molecular evolutionary genetics analysis across computing platforms. Mol Biol Evol 35(6):1547–1549. https://doi.org/10.1093/molbev/msy096
Pond SLK, Frost SDW, Muse SV (2005) HyPhy: hypothesis testing using phylogenies. Bioinformatics 21(5):676–679. https://doi.org/10.1093/bioinformatics/bti079
Muroňová J et al (2023) Lack of CCDC146, a ubiquitous centriole and microtubule-associated protein, leads to non-syndromic male infertility in human and mouse. 2023.02.27.530236
Liu C et al (2016) Autophagy is required for ectoplasmic specialization assembly in sertoli cells. Autophagy 12(5):814–832. https://doi.org/10.1080/15548627.2016.1159377
Mooers BH (2020) Shortcuts for faster image creation. Protein Sci 29(1):268–276. https://doi.org/10.1002/pro.3781
Bailey TL et al (2015) The MEME suite. Nucleic Acids Res 43(1):39–49. https://doi.org/10.1093/nar/gkv416
Gamallat Y et al (2021) Bi-allelic mutation in Fsip1 impairs acrosome vesicle formation and attenuates flagellogenesis in mice. Redox Biol 43:101969. https://doi.org/10.1016/j.redox.2021.101969
Acknowledgements
We thank Jingnan Liang and Pengyan Xia for their help with transmission electron microscopy, Chunli Li for his help with scanning electron microscopy, and Guofeng Zhou for his help with the protein-protein docking experiment. This work was supported by the National Science Fund for Distinguished Young Scholars (81925015) and the National Natural Science Foundation of China (Grant No. 91649202).
Funding
This work was supported by the National Science Fund for Distinguished Young Scholars (Grant No. 81925015) and the National Natural Science Foundation of China (Grant No. 91649202).
Author information
Authors and Affiliations
Contributions
All authors contributed to the study conception and design. YM, BW, and YC performed most of the experimental work of the study, analyzed data, and wrote the manuscript. SM, LW, Tingting Han, XL, and FY performed part of the experiments. WL, JZ, and CL designed the experiments, supervised the whole project, and revised the manuscript. All the authors read and approved the final manuscript.
Corresponding authors
Ethics declarations
Conflict of interest
The authors declare that they have no competing interests.
Ethical approval
All the animal experiments were approved by the Animal Research Panel of the Committee on Research Practice of the University of the Chinese Academy of Sciences (Approval number: IOZ20180012). All of the animal experiments were performed according to approved institutional animal care and use committee (IACUC) protocols (#08–133) of the Institute of Zoology, Chinese Academy of Sciences. All the 8-week male mice were sacrificed by cervical dislocation before testes collection.
Consent to participate
Informed consent was obtained from all individual participants included in the study.
Consent for publication
Not applicable.
Additional information
Publisher's Note
Springer Nature remains neutral with regard to jurisdictional claims in published maps and institutional affiliations.
Supplementary Information
Below is the link to the electronic supplementary material.
Supplementary file1 (MP4 1695 KB)
Supplementary file2 (MP4 1692 KB)
Supplementary file3 (MP4 2611 KB)
Supplementary file5 (AVI 12277 KB)
Supplementary file6 (AVI 12564 KB)
Rights and permissions
Springer Nature or its licensor (e.g. a society or other partner) holds exclusive rights to this article under a publishing agreement with the author(s) or other rightsholder(s); author self-archiving of the accepted manuscript version of this article is solely governed by the terms of such publishing agreement and applicable law.
About this article
Cite this article
Ma, Y., Wu, B., Chen, Y. et al. CCDC146 is required for sperm flagellum biogenesis and male fertility in mice. Cell. Mol. Life Sci. 81, 1 (2024). https://doi.org/10.1007/s00018-023-05025-x
Received:
Revised:
Accepted:
Published:
DOI: https://doi.org/10.1007/s00018-023-05025-x