Abstract
Phlebotomine sand flies are the known vectors of Leishmania parasites. New approaches in vaccination against Leishmania have investigated the possibility of integrating Phlebotomus papatasi salivary proteins to enhance the immune response and protect against the transmission of the infection. The aim of the present study was to screen human immune responses to wild sand fly saliva and evaluate immunogenic salivary proteins. Blood samples were collected from donors in control and sand fly infested areas. Antibodies specific for sand fly antigens in donor plasma were probed using immunoblotting. In addition, recall proliferation capability of peripheral blood mononuclear cells (PBMC) was tested after sand fly salivary homogenates stimulation. The significant immunogenic salivary proteins (SPs) identified by immunoblotting were SP28, SP32, and SP36. A specific proliferative response of PBMC after stimulation with sand fly salivary homogenates was evident in donors that have antibody responses against sand fly salivary proteins. Individuals with antibody recognition to a higher number of salivary proteins (i.e., 3 or more SP bands) showed lower PBMC proliferative responses after in vitro stimulation with salivary gland homogenates (SGH) only in the sand fly infested, leishmaniasis free area. Interestingly, the presence of a humoral immune response to many SP antigens inversely correlates with a strong cell-mediated immune response (CMI). It was also noticed that some other heavily expressed antigens, in sand fly salivary homogenate, lack or have weak humoral immune reactivity in exposed individuals. Therefore, considering these antigens alone as CMI activators, without including the immunodominant humoral immune response proteins, needs future investigation.
Similar content being viewed by others
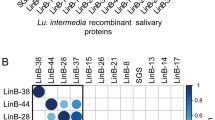
Introduction
Leishmaniasis is a vector-borne parasitic disease with a global distribution that reaches approximately two million new cases reported annually (WHO 2015). Leishmaniasis is transmitted by the bites of female Phlebotomine sand flies (Bates 2007; Lane et al. 1993). The clinical manifestations of leishmaniasis are quite diverse depending on the infecting Leishmania species, the geographic location, and the immune status of the host (Desjeux 1996; Kebaier et al. 2006). There are three primary clinical forms of leishmaniasis depending on the disease manifestation, organ involved, and the geographical distribution which are categorized as visceral leishmaniasis (VL), cutaneous leishmaniasis (CL), and muco-cutaneous leishmaniasis (MCL). The disease ranges from self-healing CL to severe systemic disease and is generally fatal if left untreated as in case of VL (Herwaldt 1999; Reithinger et al. 2007). Leishmania spp. have a digenetic life-cycle, alternating between extra-cellular promastigotes in Phlebotomine sand flies (insect-stage) to obligate intracellular amastigotes within cells of the vertebrate host (mammalian-stage) (Cecilio et al. 2014).
Zoonotic cutaneous leishmaniasis (ZCL) is an endemic disease in Jordan, mostly caused by L. major with a reported prevalence rate at 313/105 persons between year 2004 and 2005 (Mosleh et al. 2008). The disease is primarily maintained by the reservoir host Psammomys obesus and the sand fly vector Phlebotomus papatasi (Janini et al. 1995; Kamhawi et al. 1993). Inhabitants of areas endemic for leishmaniasis exhibit attenuated Leishmania infections and are less likely to contract the disease as compared to newcomers (Andrade et al. 2007). This observation has been attributed to the repeated exposure of individuals to non-infected sand fly bites that is thought to induce a protective immunity against Leishmania infection (Kamhawi 2000). It is important to address that this protection induction was achieved from pre-immunization with salivary proteins of P. papatasi collected from long-term colonized P. papatasi (Valenzuela et al. 2001a, b). On the other hand, natural and frequent exposures in endemic areas to non-infective bites of P. papatasi do not result in similar disease protection. Therefore, it is clear that wild-caught and/or recently colonized P. papatasi immunological behavior is different from that long-term colonized P. papatasi (Ahmed et al. 2010; Ben Hadj Ahmed et al. 2010, 2011).
Sand fly females release approximately 10 to 1000 Leishmania parasites along with saliva during the blood-feeding process (Kimblin et al. 2008; Rogers et al. 2004; Titus and Ribeiro 1988). Sand fly saliva is composed of potent pharmacologically active molecules that act as anticoagulants, anti-platelets, and vasodilators and have immunomodulatory and anti-inflammatory activities (Abdeladhim et al. 2014; Charlab et al. 1999; Kamhawi et al. 2000). Sand fly saliva components are important in facilitating the blood-feeding process (Ribeiro and Francischetti 2003; Rohousova and Volf 2006). For example, P. papatasi secretes adenosine and adenosine monophosphate (AMP) during a blood meal (Ribeiro et al. 1999). Both molecules act as vasodilators and exhibit anti-platelet aggregation properties (Dionisotti et al. 1992). Furthermore, P. papatasi saliva also contains a significant level of endogenous protein phosphatase 1 (PP-1) and PP-2A like activity (Desjeux 2004; Katz et al. 2000). Moreover, an anti-platelet aggregation enzyme known as apyrase (SP36) has also been identified in P. papatasi (Chatelain et al. 1992; Valenzuela et al. 2001b).
Several studies have been conducted to characterize the main components of the saliva and their role in the immune response initiation. It was reported that P. papatasi salivary proteins elicit antibody responses in mice (Rohousova et al. 2005; Valenzuela et al. 2001a, b). These saliva immunogens consisted of 12 major protein bands ranging in size from 10 to 100 kDa (Valenzuela et al. 2001a). It is generally thought that the enhancement of Leishmania infections by sand fly saliva is due to its non-specific immune-modulatory activities. Conversely, the delayed-type hypersensitivity (DTH) elicited by the repeated exposure to sand fly bites or salivary gland homogenates (SGH) inhibits Leishmania infection (Belkaid et al. 1998). Vaccination with SGH, or pre-exposure to uninfected sand fly bites, modulates the cytokine milieu at the site of parasite delivery toward a T-helper 1 (Th1) response that is necessary to generate cell-mediated immune responses against Leishmania parasites. The principle objective in the current work was to screen for saliva proteins from wild-caught P. papatasi that are immunogenic to humans in a natural setting.
Materials and methods
Insects collection and salivary gland homogenates (SGH) preparation
Adult sand flies were collected during the sand flies activity season once weekly from June to September during 2011 and 2012 (Schlein and Muller 2010) (Table 1). In addition, mosquitoes (Culex sp.) were also collected for the purpose of comparison with the collected sand flies salivary proteins. Collections were carried out using CDC light traps (John W. Hock Company, USA) in Alkhaldeih (Mafraq) and Swaymeh (Dead Sea) Jordan; both areas are known to harbor high and comparable abundance of P. papatasi (Kamhawi et al. 1995). Traps were placed near potential resting and breeding sites around houses and near reservoir host burrows with more than 90 % collected from the resting sites. Generally, traps were placed at sunset and collected before the following sunrise. Collected flies were transferred in the trap net bags which were kept humidified in plastic bags and transferred back to the laboratory where the sand flies were removed and identified.
Collected flies were immersed in 1 % detergent soap solution for killing and body hair removal and then washed three times in tap water. Flies were then transferred to container with sterile (1X) phosphate buffered saline (PBS, Lonza, USA) and kept cool on ice until dissected using an Olympus sz61 zoom stereomicroscope. The flies were identified based on specific morphological features according to the keys developed by Lewis (1978).
The spermatheca in female sand flies was used for species identification. Briefly, the terminal abdominal segment was separated and placed in a drop of (1X) PBS on a clean microscope slide and pressed gently with the cover glass to flatten it to facilitate species identification by light microscopy (Motic BA 200 Trinocular Biological Microscope, Australia). After positive identification of female P. papatasi flies which were more than 95 % of trapped sand flies, the salivary glands were extracted on the same day of trapping by pulling the head gently from the body using forceps and needles; the visualized glands were carefully scooped into a storage tube using syringe head (Titus and Ribeiro 1988; Volf et al. 2000). In order to cover all the variations between time and sites of collection (Ramalho-Ortigao et al. 2015; Volf et al. 2000), salivary glands were pooled in groups of 20 in a volume of 20 μL (1X) PBS in 1.5 mL micro-centrifuge tubes and stored at −70 °C. Immediately before use, salivary gland homogenates (SGHs) were prepared by exposing the glands to three freeze-thaw cycles then sonicated (Bransonic 5510 E-MT, Mexico) and centrifuged (10,000 RCF for 2 min) (Abdeladhim et al. 2011). SGH used for peripheral blood mononuclear cells (PBMC) stimulation and proliferation assay were filter sterilized through 0.22 μm ultra-free centrifugal filter units (Millipore).
Blood samples
In the period between July 2012 and December 2012, a total of 114 blood samples from donors living in three different locations, Swaymeh, Amman, and Mafraq, were collected. The selected sites were chosen according to the presence or absence of both sand flies and Leishmania parasites as summarized in the Table 2. It is important to note that the selected areas are in close proximity and traveling for people among these areas is expected. In addition, it was observed that the abundance of trapped P. papatasi was comparable at the two experimental areas (MF and SW). Samples from peripheral blood (9 mL) from the vein of volunteers’ were collected in vacutainer tubes with acid citrate dextrose solution A (ACD-A, Greiner Bio-One, Austria). Blood plasma was separated by centrifugation of blood at 1500 RCF for 10 min at room temperature before being transferred to cryo-tubes and then frozen at −70 °C. The donors were asked to sign a consent form regarding the work conducted and individual information was filled for each donor separately. Data for each donor were collected in a questionnaire that was filled during interview. All personal information has been kept secret. All donors participating in this study were healthy and above 18 years of age.
Peripheral blood mononuclear cells isolation
PBMCs were separated using Ficoll-hypaque density gradient centrifugation. Briefly, fresh blood samples were diluted (after plasma separation) twofold with sterile (1X) PBS and slowly laid over the Lymphocyte Separation Medium (LSM1.077, Lonza, USA). After centrifugation for 30 min at 900 RCF, the mononuclear cell layer was collected into a separate 15 mL tubes and washed by adding Hanks balanced salt solution (HBSS, EuroClone, Italy) before centrifugation for 10 min at 400 RCF. RBCs were subsequently lysed with lysis buffer (Promega, USA) for 5 min on ice. PBMCs were then washed two times with tissue culture medium (RPMI-1640, EuroClone, Italy) by centrifuging for 10 min at 250 RCF. Finally, the pellet was re-suspended in 1 mL complete RPMI-1640 supplemented with 10 % heat inactivated fetal bovine serum (FBS), 2 mM L-glutamine, and 1 % penicillin/ streptomycin (100 U/mL penicillin and 100 μg/mL streptomycin) (all supplements were obtained from EuroClone, Italy). Viable cells were counted using trypan blue (Sigma-Aldrich, Germany) exclusion test by hemocytometer chamber (Marienfeld, Germany) (Chang et al. 2007; Freshney 1987; Gantt et al. 2001).
Cell proliferation
Cell proliferation has been assessed using a non-radioactive 5-bromo-deoxyuridine (BrdU) incorporation assay. For each sample, 12 wells with 1 × 105 PBMC per well were plated in a U-shape 96 well tissue culture plate setup. SGH was added at a concentration of 0.5 salivary gland per mL and phytohemagglutinin (PHA; 10 μg/mL) (Remel, UK) was used as a positive proliferation control (Geraci et al. 2014). For each donor, wells without any stimulation were used as a negative control. Total volumes were adjusted to 200 μL per well and plates were incubated in a 5 % CO2 humidified incubator at 37 °C for 5 days.
At day 4, BrdU was added to the wells then further incubated for 18 h. The proliferation was then measured using BrdU Enzyme Linked Immunosorbent Assay (ELISA) kit (BrdU [colorimetric}, Roche, Germany) according to manufacturer’s instruction. All solutions, buffers, and media used in tissue culturing were sterilized using a 0.22 μm Vacuum filtration unit (JET BIOFIL, USA) and kept at 4 °C for further use. Moreover, cells were treated under sterile conditions in endotoxin free environment and exclusively under ESCO class II Biological Safety Cabinet). Proliferation results were expressed as stimulation index (SI) which represents the mean of antigen-stimulated cultures/mean of un-stimulated cultures in all tested individuals.
SDS-PAGE
Pooled proteins of wild-caught sand fly salivary glands as well as mosquitoes (Culex sp.) salivary proteins were separated using the sodium dodecyl sulfate polyacrylamide gel electrophoresis (SDS-PAGE) technique to characterize the salivary proteins of P. papatasi collected from all selected study areas (Ramalho-Ortigao et al. 2015). Briefly, Tris-glycine gels (12 %) with a thickness of 1 mm were prepared using Modular Mini Vertical Gel and Blotting System (Major Science, Taiwan) according to manufacturer’s protocol.
Before use, salivary glands were subjected to three freeze-thaw cycles then sonicated for 1 min and centrifuged at 8000 RCF for 2 min. An equivalent of 20 glands was used per gel. Furthermore, the glands were lysed in 16 μL 2X SDS sample buffer (0.125 M Tris-Cl, pH 6.8, 4 % SDS, 20 % glycerol, 10 % 2-mercaptoethanol, 0.25 % bromophenol blue), 4 μL 2-mercaptoethanol and 50 μL PBS, and incubated at 85 °C for 10 min. The mixture was then cooled down immediately on ice then centrifuged at 13,000 RCF for 3 min. Finally, the two lanes of the gel were loaded, one with salivary gland lysate and the other with 2.5 μL ladder’s (BlueRAY Pre-stained Protein Ladder markers, GeneDirex, USA). Electrophoresis was carried out at 125 V 40 mA for 100 min using Tris-Glycine running buffer with SDS (0.025 M Tris, 0.192 M Glycine and 1 % SDS).
Staining
Gels were placed in a fixation solution (50 % methanol, 10 % acetic acid) overnight then stained for 4 h in 1 % Coomassie Brilliant Blue R-250 (Sigma-Aldrich, Germany). The gel was de-stained overnight in de-staining solution (5 % methanol, 7.5 % acetic acid) and stored in 7 % acetic acid. The gel image was taken using the doc Universal hood ΙΙ (Bio-Rad, USA).
Immunobloting
To identify the immune reactive salivary proteins recognized by sand fly bitten individuals, western blot analysis of P. papatasi SGH was performed (Barral et al. 2000; Geraci et al. 2014). Briefly, separated proteins were transferred onto a nitrocellulose membrane using the Modular Mini Electro Blot System (Major Science, Taiwan). After protein transfer, the nitrocellulose membrane was blocked for 1 h in 0.1 % Tween/Tris-buffered saline (100 mM Tris HCL, pH 7.5, 0.9 % NaCl) (TBS-T) with 5 % non-fat dried milk. Membranes were then placed in a Mini-protean II multi-screen apparatus (Bio-Rad, USA) to allow the screening of plasma samples. Plasma samples were diluted 1:100 in blocking buffer (2 % non-fat milk-TBS-T) and applied to the blot overnight at 4 °C on a rotating platform. Membranes were then removed from the cast and washed 3 times with TBS-T (15 min for each wash). Goat anti-human immunoglobulin conjugated to alkaline phosphatase (goat anti-human IgG F(ab’)2 Alkaline Phosphatase, AbD Serotec, UK) secondary antibodies were used at a 1:5000 dilution and applied to blots for 1 h at room temperature. Recognized proteins were visualized using the colorimetric substrate BCIP/NBT (BCIP/NBT Color Development Substrate, Promega, USA). All chemicals for SDS-PAGE and western blot assays were obtained from Bio Basic Canada Inc. The blots were scanned using computer scanner for documentation.
Statistical analysis
The proliferation assay results and the comparison between the different areas were performed using two-way analysis of variance (ANOVA) test at 95 % confidence interval for mean. The significant differences among means were tested using Student’s t test. For all tests and comparisons, the difference was considered significant at P < 0.05.
Ethics statement
This study was approved by the ethic committee of the Institutional Review Board (JUST-IRB) at Jordan University of Science and Technology and performed according to Declaration of Helsinki principles. The donors were asked to read and sign a consent form regarding the work conducted and individual information was filled for each donor separately. The consent form informed the participants of any potential risks of the study.
Results
Humoral antibody response
SDS-PAGE of separated SGH of P. papatasi were stained by Coomassie Brilliant Blue which revealed approximately 11 heavily expressed salivary proteins (SP14, SP15, SP28, SP30, SP32, SP36, SP42, SP44, SP46, SP52, and SP64) and one modestly expressed protein, SP12 (Fig. 1). Plasma from 114 donors was tested against PAGE-separated SGH from wild female P. papatasi by western immunoblot. Antibodies of donor plasma displayed differential specificities for some salivary proteins, after transfer to western blot (Fig. 2). There were individuals that had antibody responses to some specific bands (SP28, SP32, SP36, and SP42) while other heavily expressed bands for SGH on SDS-PAGE (SP14, Sp15, SP30, SP44, and SP46) showed no humoral immune reactivity on immunoblotting assay (Table 3). Reactivity to the heavily expressed sand fly salivary protein SP64 was observed in approximately half of donors (54 donor out of 114), from all three areas. Interestingly, the same donor plasma recognized a similarly sized protein in mosquitoes (Culex sp.) SGH (Fig. 3). This cross-species reactivity drives the exclusion of this antigen (SP64) from further use as a vaccine candidate.
Salivary protein profile of Jordanian wild Phlebotomus papatasi. A whole salivary gland protein homogenate isolated from Jordanian wild Phlebotomus papatasi. Salivary gland proteins were separated using 12 % SDS-PAGE gel electrophoresis stained with Coomassie Brilliant Blue R-250. The bands where compared visually with existing salivary protein profile of colonized P. papatasi according to Valenzuela et al. (2001a). (Numbers indicate the position of the molecular weight marker in the same gel)
Immunoblot of reactive Phlebotomus papatasi salivary proteins. Immunoblot reactive bands of donor plasma from the three selected sites with antibody specificity to Phlebotomus papatasi salivary proteins. Lanes were designated by regional serum donor codes: AM, MF, and SW (see Table 2), positive donor designated as (+ve), and negative control designated as (−ve)
Salivary protein profile and immunoblot of Jordanian wild mosquito (Culex sp.). a A whole salivary gland protein homogenate of Jordanian wild P. papatasi and a whole salivary gland protein homogenate of Jordanian wild mosquitoes (Culex sp.) run on the same denaturing 12 % SDS-PAGE gel and stained with Coomassie Brilliant Blue R-250. b Immunoblot reactive bands of donor plasma from the three selected sites with antibody specificity to mosquitoes and P. papatasi salivary proteins run on the same gel. Lanes are designated by regional serum donor codes: AM, MF, and SW (see Table 2)
The immunoblotting results were compared between areas infested with sand flies (Mafraq and Swaymeh) (Table 3). High levels of antibody reactivity for three salivary proteins (SP28, SP32, and SP36) were observed in Mafraq, while in Swaymeh, additional, moderate, antibody reactivity was observed to another salivary protein (SP42). In general, proteins such as SP12, SP30, SP46, and SP52 provoked weak humoral immune responses in individuals living in areas infested with sand flies. Although they heavily expressed in SGH, reactivity to SP14, SP15, and SP44 was not detected in any individuals.
Cell-mediated immune response
Among all experimental groups, and regardless of the crude SGH concentration used to stimulate PBMC, the negative control area had the lowest cell proliferation SI (Fig. 4). In general, individuals from Mafraq, where sand flies are present without reported leishmaniasis cases, had the highest SI when compared to other areas. Finally, cell proliferation SI values of individuals from Swaymeh, an area endemic with L. major, was significantly higher than SI observed in negative control area (Amman) but lower than Mafraq, especially when PBMC stimulated with a lower SGH concentration. It is important to note that samples from Amman that showed a positive antibody response in the immunoblotting assay to SGH (19 samples), as well as samples from Mafraq (5 samples) and Swaymeh area (4 samples) that showed no humoral immune response against SGH, were excluded from SI comparison.
The index of proliferation in all tested individuals at the three selected sites. The stimulation index (SI) of peripheral blood mononuclear cells (PBMC) in response to P. papatasi salivary gland homogenates at a concentration of 2, 1, and 0.5 salivary gland per mL. PBMC were isolated from volunteer donors; bars are designated by regional donor codes: control area (i.e., Amman (AM)) and sand flies infested area (Mafraq (MF) and Swaymeh (SW)). Stimulation index expresses the proliferation mean in antigen-stimulated cultures/proliferation mean in un-stimulated culture. Each value represents the mean value ± S.E.M. Different letters indicate a significant difference between groups (P < 0.05)
We further compared cell proliferation SI in accordance with the frequency of specific antibody reactivity to different salivary P. papatasi proteins in individuals resident in sand fly infested areas (Fig. 5). Individuals with antibody reactivity to many antigen panels in the immunoblot assay exhibited less cell proliferation to SGH in samples collected from Mafraq. This adverse effect was only apparent in samples stimulated with 0.5 and 1 gland per mL (Fig. 5a). Regardless of SGH concentration, PBMCs collected from donors living in Leishmania-endemic area (i.e., SW) had no significant differences in the proliferation index results (Fig. 5b, c). Thus, SW individuals with antibody reactivity to 3 or more SP antigens on immunoblotting assay had similar proliferation index to those individuals with antibody reactivity to two or less bands.
The stimulation index in individuals with low and high antibody reactivity toward different numbers of salivary P. papatasi proteins. The stimulation index (SI) of peripheral blood mononuclear cells (PBMC) in response to P. papatasi salivary gland homogenates at a concentration of 0.5 (a), 1 (b), and 2 (c) salivary gland per mL. PBMC were isolated from volunteer donors from sand flies infested area (Mafraq (MF) and Swaymeh (SW)). The SI results for the sand flies exposed individuals in each area were categorized according to their antibody reactivity toward different numbers of salivary P. papatasi proteins. Each value represents the mean value ± S.E.M. Different letters indicate a significant difference between groups (P < 0.05)
Discussion
The goal of the present study was to identify sand fly salivary proteins that activate cellular and humoral immune responses in volunteers naturally exposed to frequent bites by the wild sand flies (P. papatasi). The analysis of specific circulating antibodies to whole sand fly salivary gland homogenates, as well as the cellular response elicited by the presence of memory lymphocytes, was investigated. Several previous studies suggested that exposure of mice to sand fly bites results in strong cellular and humoral immunity specific to salivary gland proteins (Rohousova and Volf 2006; Silva et al. 2005). Analysis of cellular immunity against sand fly saliva in volunteers exposed to the bites of P. papatasi is limited (Abdeladhim et al. 2011; Geraci et al. 2014; Marzouki et al. 2011). In comparison to other studies that used salivary proteins obtained from colony raised sand flies, the current work specifically assessed the proliferative immune response toward the salivary gland homogenates collected from wild-caught sand flies (i.e., P. papatasi) with the presence of specific antibodies in people naturally exposed to sand fly bites, in leishmania-endemic and leishmania free, sand fly infested areas.
A higher cellular proliferation rate in response to SGH stimulation was identified among donors from sand fly infested areas compared to donors from the negative control area. Cellular proliferation was inversely correlated with an increased antibody response activity in individuals that were resident in sand fly infested areas but free of leishmaniasis (MF). The most interesting finding in the current work is that individuals exhibiting humoral immunity to a higher number of salivary proteins had a lower ability to mount a strong cell proliferative response. It was also remarkable that in the leishmaniasis endemic area (SW), individuals do not exhibit a cell proliferative response regardless of antibody reactivity or SGH concentration. These data may suggest that individuals with a low humoral immune response (possibly early respondents) that reside in sand fly areas free form leishmaniasis have strong cell-mediated immunity responses when their immune cells are exposed to sand fly antigens. Thus, the suggestion of tolerance induction with high SGH stimulation seems as an appealing explanation (Rohousova et al. 2011). Therefore, these findings might explain the high risk incidence of leishmaniasis in endemic areas even with the existence of strong humoral immune responses against sand fly salivary proteins (Barral et al. 2000; Gomes et al. 2002; Rohousova et al. 2005). In addition, the current work suggests a new approach in handling and assessment of immunological sand fly salivary proteins. Although the salivary protein types play several important roles in disease transmission and disease pathogenesis, the emphasis on the number of immunogenic salivary proteins, regardless of the protein types in individuals, need to be considered when evaluating and determining the immunological behavior against SGH exposure.
Immunodominant protein bands that elicit a humoral host immune response could be employed as biomarkers (i.e., SP32) for human exposure to sand fly bites (Marzouki et al. 2015). Moreover, some of proteins with high antibody reactivity can be used to map a sand fly species spectrum in an attempt to identify vector exposure in a certain area. In addition, studies have strongly supported, for example, the possibility of using P. papatasi saliva antibodies as indicators of vector exposure, a potentially useful parameter in forecasting the development of Leishmania major infections (Abdeladhim et al. 2014; Sacks et al. 1995). Clearly, individuals living in sand fly infested areas exhibit increased humoral responses to sand fly saliva compared to individuals residing in uninfested areas. The major humoral immune reactive salivary proteins can be labeled as SP28, SP32, SP36, and SP42 with the SP32 being the immunodominant one followed by SP28 and SP36, somewhat consistent with previous reports (Abdeladhim et al. 2011). Interestingly, the immunodominant antigen was not the same between the two sand fly infested sites. The number of individuals responding to SP42 was 3 donors in MF, while the number of individuals in SW reached 8.
The close proximity of several proteins on immune blots, such as bands present between the 25 and 35 kDa cluster (Fig. 2), make it difficult to distinguish the exact type of the SP identified by humoral immunity. For example, the close location of SP30 and SP32 on the immunoblotting assay might lead to mis-interpretation of the importance and identity of each of these SP antigens (Marzouki et al. 2012). Therefore, previous studies concluded that presence of a protein on a single SDS-PAGE does not always mean one protein but rather suggested several proteins sharing the same band (Geraci et al. 2014; Marzouki et al. 2012). However, the antibody reactivity toward the three close bands (i.e., SP28, SP30 and SP32) that was present in one volunteer (data not shown) was employed as a guide to approximately identify the antibody reactivity to each of these bands in other individuals. Accordingly, the band identified with most antibody reactivity in the current work was labeled as SP32 and not SP30. Despite the above approach in labeling and defining the salivary proteins, data analysis in the current work did not give much weight to each individual band type or size but rather the analysis was completely based on the number of recognized bands in each responder. Therefore, regardless of the type of SP proteins, exposed individuals were divided into low antibody responders (1 or 2 bands) compared with high responders (3 or more bands). Accordingly, PBMC proliferation in response to different SGH concentrations was compared in these two antibody responding groups.
Strikingly, little specificity for SP12, SP30, SP46, and SP52 salivary proteins was observed and no antibody response was reported toward SP14, SP15, and SP44 salivary proteins. Thus, this study also hypothesizes that the weak, or the absence of, humoral immune response for some heavily expressed salivary proteins might point out that these antigens should be further explored for their ability to stimulate cell-mediated immunity. Interestingly, a recent study indicated that the immune response to a single sand fly salivary protein may differ from the response to SGH, and a distinct protein from the same vector can generate a different immune response by skewing to a Th1 or Th2 pattern (Oliveira et al. 2008). For example, SP15 which had no antibody reactivity in the current study was previously demonstrated to activate a cell-mediated immune response and repeatedly reported to enhance protection against Leishmania challenge in murine hosts (Elnaiem et al. 2005; Oliveira et al. 2008; Valenzuela et al. 2001a) and non-human primate models using SP15 from P. duboscqi (Oliveira et al. 2015). Of note is that protection against L. major infection improved in B cell-deficient mice vaccinated with SP15 (Valenzuela et al. 2001a). From this point of view, it was suggested that the protein PpSP15 from P. papatasi as well as P. dubosqui saliva would be a better cell-mediated immune activator and thus a promising candidate for future vaccine development (Elnaiem et al. 2005; Lestinova et al. 2015; Oliveira et al. 2008). Similarly, SP44 did not stimulate any humoral antibody response in the current study although it has been previously demonstrated to induce a strong IL-4 response in mice (Oliveira et al. 2008). As well, this protein was also shown to have an adverse effect on protection in infected mice (Oliveira et al. 2008). In addition, it is well established that most blood-feeding arthropods that are responsible for transmission of many diseases have immunosuppressive proteins in their saliva (Titus et al. 2006). Therefore, some antigens with low or no humoral immunogenic responses reported in the present study such as SP14, SP15, and SP44 might have characteristics worthy of further investigations to clarify their exact immune-modulatory effects.
The differential immune responses toward separate salivary proteins suggest that the immune cells in these recruited individuals respond to one type of immune arm of defense over another in response to these proteins. Thus, proteins such as SP28, SP32, SP36, and SP42 were known to stimulate the antibody response in the majority of exposed donors. The presence of SP36 (apyrase) in sand fly SGH might have caused the suppression of the proliferative responses of PBMC by increasing the hydrolytic reactions to boost AMP levels in dendritic cell cultures (Carregaro et al. 2011). Marzouki et al. (2011) found that all positive sera from naturally exposed donors strongly reacted with a P. papatasi 30-kDa protein band which correspond to SP30 using mass spectrometry. Interestingly, later conformational studies by the same group showed that SP32 salivary protein from P. papatasi is the immunodominant one (Marzouki et al. 2012). Indeed, adenosine has been shown to be present in large quantities in P. papatasi salivary glands (Ribeiro et al. 1999), and it has been reported to enhance the production of IL-10 and decrease the production of IL-12 and IFN-γ (Carregaro et al. 2011) However, even in this adenosine presence, significant differences were identified in the proliferation results in the current study (Figs. 4 and 5).
It is important to emphasize that although the selected sites in Jordan, representing control and experimental regions, are climatically diverse, they are relatively in close geographical proximity. In addition, the ease of movement within these areas might be an important factor that affected the antibody specificity profiles of donors against SGH despite the characteristics of their primary residence sites. This might explain that several individuals in the Amman area, which supposedly had no previous exposure to sand fly salivary proteins, elicited a humoral response against several SGH bands.
The significant appearance of SP64, and to some extent SP52 in several volunteers in all experimental groups, could be attributed to the possibility of volunteers’ exposure to salivary gland proteins of other non-sand fly hematophagous arthropods such as mosquitoes. Antibodies with specificity to potentially conserved protein domains among the different insects (Fig. 3) might explain the arbitrary antibody reactivity appearance in several individuals. This might also suggest common conserved protein in blood sucking insects that could cause cross reactivity in host humoral immune response (Francischetti et al. 2002).
The variations in antibody specificity for different salivary proteins were clearly obtained by naturally exposed individuals to saliva of P. papatasi. The most reactive salivary proteins in endemic areas were SP28, SP32, and SP36 with the SP32 being immunodominant for antibody production. The low PBMC proliferation response that appeared in individuals with high humoral reactivity suggests the need to explore the specific cell-mediated immune responses toward other SGH heavily expressed proteins that were lacking humoral immune response stimulation ability alone without including humoral immunodominant proteins.
References
Abdeladhim M, Ben Ahmed M, Marzouki S, Belhadj Hmida N, Boussoffara T, Belhaj Hamida N, Ben Salah A, Louzir H (2011) Human cellular immune response to the saliva of Phlebotomus papatasi is mediated by IL-10-producing CD8+ T cells and Th1-polarized CD4+ lymphocytes. PLoS Negl Trop Dis 5:e1345. doi:10.1371/journal.pntd.0001345
Abdeladhim M, Kamhawi S, Valenzuela JG (2014) What’s behind a sand fly bite? The profound effect of sand fly saliva on host hemostasis, inflammation and immunity. Infect Genet Evol 28:691–703. doi:10.1016/j.meegid.2014.07.028
Ahmed SB, Kaabi B, Chelbi I, Derbali M, Cherni S, Laouini D, Zhioua E (2010) Lack of protection of pre-immunization with saliva of long-term colonized Phlebotomus papatasi against experimental challenge with Leishmania major and saliva of wild-caught P. papatasi. Am J Trop Med Hyg 83:512–514. doi:10.4269/ajtmh.2010.09-0687
Andrade BB, de Oliveira CI, Brodskyn CI, Barral A, Barral-Netto M (2007) Role of sand fly saliva in human and experimental leishmaniasis: current insights. Scand J Immunol 66:122–127. doi:10.1111/j.1365-3083.2007.01964.x
Barral A, Honda E, Caldas A, Costa J, Vinhas V, Rowton ED, Valenzuela JG, Charlab R, Barral-Netto M, Ribeiro JM (2000) Human immune response to sand fly salivary gland antigens: a useful epidemiological marker? Am J Trop Med Hyg 62:740–745
Bates PA (2007) Transmission of Leishmania metacyclic promastigotes by phlebotomine sand flies. Int J Parasitol 37:1097–1106. doi:10.1016/j.ijpara.2007.04.003
Belkaid Y, Kamhawi S, Modi G, Valenzuela J, Noben-Trauth N, Rowton E, Ribeiro J, Sacks DL (1998) Development of a natural model of cutaneous leishmaniasis: powerful effects of vector saliva and saliva preexposure on the long-term outcome of Leishmania major infection in the mouse ear dermis. J Exp Med 188:1941–1953
Ben Hadj Ahmed S, Chelbi I, Kaabi B, Cherni S, Derbali M, Zhioua E (2010) Differences in the salivary effects of wild-caught versus colonized Phlebotomus papatasi (Diptera: Psychodidae) on the development of zoonotic cutaneous leishmaniasis in BALB/c mice. J Med Entomol 47:74–79
Ben Hadj Ahmed S, Kaabi B, Chelbi I, Cherni S, Derbali M, Laouini D, Zhioua E (2011) Colonization of Phlebotomus papatasi changes the effect of pre-immunization with saliva from lack of protection towards protection against experimental challenge with Leishmania major and saliva. Parasite Vectors 4:126. doi:10.1186/1756-3305-4-126
Carregaro V, Sa-Nunes A, Cunha TM, Grespan R, Oliveira CJ, Lima-Junior DS, Costa DL, Verri WA Jr, Milanezi CM, Pham VM, Brand DD, Valenzuela JG, Silva JS, Ribeiro JM, Cunha FQ (2011) Nucleosides from Phlebotomus papatasi salivary gland ameliorate murine collagen-induced arthritis by impairing dendritic cell functions. J Immunol 187:4347–4359. doi:10.4049/jimmunol.1003404
Cecilio P, Perez-Cabezas B, Santarem N, Maciel J, Rodrigues V, Cordeiro da Silva A (2014) Deception and manipulation: the arms of leishmania, a successful parasite. Front Immunol 5:480. doi:10.3389/fimmu.2014.00480
Chang HK, Thalhofer C, Duerkop BA, Mehling JS, Verma S, Gollob KJ, Almeida R, Wilson ME (2007) Oxidant generation by single infected monocytes after short-term fluorescence labeling of a protozoan parasite. Infect Immun 75:1017–1024. doi:10.1128/iai.00914-06
Charlab R, Valenzuela JG, Rowton ED, Ribeiro JM (1999) Toward an understanding of the biochemical and pharmacological complexity of the saliva of a hematophagous sand fly Lutzomyia longipalpis. Proc Natl Acad Sci U S A 96:15155–15160
Chatelain R, Varkila K, Coffman RL (1992) IL-4 induces a Th2 response in Leishmania major-infected mice. J Immunol 148:1182–1187
Desjeux P (1996) Leishmaniasis. Public health aspects and control. Clin Dermatol 14:417–423
Desjeux P (2004) Leishmaniasis: current situation and new perspectives. Comp Immunol Microbiol Infect Dis 27:305–318. doi:10.1016/j.cimid.2004.03.004
Dionisotti S, Zocchi C, Varani K, Borea PA, Ongini E (1992) Effects of adenosine derivatives on human and rabbit platelet aggregation. Correlation of adenosine receptor affinities and antiaggregatory activity. Naunyn Schmiedebergs Arch Pharmacol 346:673–676
Elnaiem DE, Meneses C, Slotman M, Lanzaro GC (2005) Genetic variation in the sand fly salivary protein, SP-15, a potential vaccine candidate against Leishmania major. Insect Mol Biol 14:145–150
Francischetti IM, Valenzuela JG, Pham VM, Garfield MK, Ribeiro JM (2002) Toward a catalog for the transcripts and proteins (sialome) from the salivary gland of the malaria vector Anopheles gambiae. J Exp Biol 205:2429–2451
Freshney RI (1987) Culture of animal cells : a manual of basic technique, 2nd edn. A.R. Liss, New York
Gantt KR, Goldman TL, McCormick ML, Miller MA, Jeronimo SM, Nascimento ET, Britigan BE, Wilson ME (2001) Oxidative responses of human and murine macrophages during phagocytosis of Leishmania chagasi. J Immunol 167:893–901
Geraci NS, Mukbel RM, Kemp MT, Wadsworth MN, Lesho E, Stayback GM, Champion MM, Bernard MA, Abo-Shehada M, Coutinho-Abreu IV, Ramalho-Ortigao M, Hanafi HA, Fawaz EY, El-Hossary SS, Wortmann G, Hoel DF, McDowell MA (2014) Profiling of human acquired immunity against the salivary proteins of Phlebotomus papatasi reveals clusters of differential immunoreactivity. Am J Trop Med Hyg 90:923–938. doi:10.4269/ajtmh.13-0130
Gomes RB, Brodskyn C, de Oliveira CI, Costa J, Miranda JC, Caldas A, Valenzuela JG, Barral-Netto M, Barral A (2002) Seroconversion against Lutzomyia longipalpis saliva concurrent with the development of anti-Leishmania chagasi delayed-type hypersensitivity. J Infect Dis 186:1530–1534. doi:10.1086/344733
Herwaldt BL (1999) Leishmaniasis. Lancet 354:1191–1199. doi:10.1016/s0140-6736(98)10178-2
Janini R, Saliba E, Kamhawi S (1995) Species composition of sand flies and population dynamics of Phlebotomus papatasi (Diptera: Psychodidae) in the southern Jordan Valley, an endemic focus of cutaneous leishmaniasis. J Med Entomol 32:822–826
Kamhawi S (2000) The biological and immunomodulatory properties of sand fly saliva and its role in the establishment of Leishmania infections. Microbes Infect 2:1765–1773
Kamhawi S, Arbagi A, Adwan S, Rida M (1993) Environmental manipulation in the control of a zoonotic cutaneous leishmaniasis focus. Arch Inst Pasteur Tunis 70:383–390
Kamhawi S, Abdel-Hafez SK, Molyneux DH (1995) A comprehensive account of species composition, distribution and ecology of phlebotomine sandflies in Jordan. Parasite 2:163–172
Kamhawi S, Belkaid Y, Modi G, Rowton E, Sacks D (2000) Protection against cutaneous leishmaniasis resulting from bites of uninfected sand flies. Science 290:1351–1354
Katz O, Waitumbi JN, Zer R, Warburg A (2000) Adenosine, AMP, and protein phosphatase activity in sandfly saliva. Am J Trop Med Hyg 62:145–150
Kebaier C, Uzonna JE, Beverley SM, Scott P (2006) Immunization with persistent attenuated Delta lpg2 Leishmania major parasites requires adjuvant to provide protective immunity in C57BL/6 mice. Infect Immun 74:777–780. doi:10.1128/iai.74.1.777-780.2006
Kimblin N, Peters N, Debrabant A, Secundino N, Egen J, Lawyer P, Fay MP, Kamhawi S, Sacks D (2008) Quantification of the infectious dose of Leishmania major transmitted to the skin by single sand flies. Proc Natl Acad Sci U S A 105:10125–10130. doi:10.1073/pnas.0802331105
Lane RP, Crosskey RW (1993) British museum (natural history). Medical insects and arachnids, 1st edn. Chapman & Hall, London
Lestinova T, Vlkova M, Votypka J, Volf P, Rohousova I (2015) Phlebotomus papatasi exposure cross-protects mice against Leishmania major co-inoculated with Phlebotomus duboscqi salivary gland homogenate. Acta Trop 144:9–18. doi:10.1016/j.actatropica.2015.01.005
Lewis J (1978) The Phlebotomine sanflies (Diptera: Psychodidae) of the oriental region. In: Museum B, Museum NH (eds) Bulletin of the British museum (natural history) entomology, vol 37. The Museum, London, pp 217–343
Marzouki S, Ben Ahmed M, Boussoffara T, Abdeladhim M, Ben Aleya-Bouafif N, Namane A, Hamida NB, Ben Salah A, Louzir H (2011) Characterization of the antibody response to the saliva of Phlebotomus papatasi in people living in endemic areas of cutaneous leishmaniasis. Am J Trop Med Hyg 84:653–661. doi:10.4269/ajtmh.2011.10-0598
Marzouki S, Abdeladhim M, Abdessalem CB, Oliveira F, Ferjani B, Gilmore D, Louzir H, Valenzuela JG, Ben Ahmed M (2012) Salivary antigen SP32 is the immunodominant target of the antibody response to Phlebotomus papatasi bites in humans. PLoS Negl Trop Dis 6:e1911. doi:10.1371/journal.pntd.0001911
Marzouki S, Kammoun-Rebai W, Bettaieb J, Abdeladhim M, Hadj Kacem S, Abdelkader R, Gritli S, Chemkhi J, Aslan H, Kamhawi S, Ben Salah A, Louzir H, Valenzuela JG, Ben Ahmed M (2015) Validation of recombinant salivary protein PpSP32 as a suitable marker of human exposure to Phlebotomus papatasi, the vector of Leishmania major in Tunisia. PLoS Negl Trop Dis 9:e0003991. doi:10.1371/journal.pntd.0003991
Mosleh IM, Geith E, Natsheh L, Abdul-Dayem M, Abotteen N (2008) Cutaneous leishmaniasis in the Jordanian side of the Jordan Valley: severe under-reporting and consequences on public health management. Trop Med Int Health 13:855–860. doi:10.1111/j.1365-3156.2008.02063.x
Oliveira F, Lawyer PG, Kamhawi S, Valenzuela JG (2008) Immunity to distinct sand fly salivary proteins primes the anti-Leishmania immune response towards protection or exacerbation of disease. PLoS Negl Trop Dis 2:e226. doi:10.1371/journal.pntd.0000226
Oliveira F, Rowton E, Aslan H, Gomes R, Castrovinci PA, Alvarenga PH, Abdeladhim M, Teixeira C, Meneses C, Kleeman LT, Guimaraes-Costa AB, Rowland TE, Gilmore D, Doumbia S, Reed SG, Lawyer PG, Andersen JF, Kamhawi S, Valenzuela JG (2015) A sand fly salivary protein vaccine shows efficacy against vector-transmitted cutaneous leishmaniasis in nonhuman primates. Sci Transl Med 7:290ra90. doi:10.1126/scitranslmed.aaa3043
Ramalho-Ortigao M, Coutinho-Abreu IV, Balbino VQ, Figueiredo CA Jr, Mukbel R, Dayem H, Hanafi HA, El-Hossary SS, Fawaz Eel D, Abo-Shehada M, Hoel DF, Stayback G, Wadsworth M, Shoue DA, Abrudan J, Lobo NF, Mahon AR, Emrich SJ, Kamhawi S, Collins FH, McDowell MA (2015) Phlebotomus papatasi SP15: mRNA expression variability and amino acid sequence polymorphisms of field populations. Parasite Vectors 8:298. doi:10.1186/s13071-015-0914-2
Reithinger R, Dujardin JC, Louzir H, Pirmez C, Alexander B, Brooker S (2007) Cutaneous leishmaniasis. Lancet Infect Dis 7:581–596. doi:10.1016/s1473-3099(07)70209-8
Ribeiro JM, Francischetti IM (2003) Role of arthropod saliva in blood feeding: sialome and post-sialome perspectives. Annu Rev Entomol 48:73–88. doi:10.1146/annurev.ento.48.060402.102812
Ribeiro JM, Katz O, Pannell LK, Waitumbi J, Warburg A (1999) Salivary glands of the sand fly Phlebotomus papatasi contain pharmacologically active amounts of adenosine and 5′-AMP. J Exp Biol 202:1551–1559
Rogers ME, Ilg T, Nikolaev AV, Ferguson MA, Bates PA (2004) Transmission of cutaneous leishmaniasis by sand flies is enhanced by regurgitation of fPPG. Nature 430:463–467
Rohousova I, Volf P (2006) Sand fly saliva: effects on host immune response and Leishmania transmission. Folia Parasitol (Praha) 53:161–171
Rohousova I, Ozensoy S, Ozbel Y, Volf P (2005) Detection of species-specific antibody response of humans and mice bitten by sand flies. Parasitology 130:493–499
Rohousova I, Hostomska J, Vlkova M, Kobets T, Lipoldova M, Volf P (2011) The protective effect against Leishmania infection conferred by sand fly bites is limited to short-term exposure. Int J Parasitol 41:481–485. doi:10.1016/j.ijpara.2011.01.003
Sacks DL, Kenney RT, Kreutzer RD, Jaffe CL, Gupta AK, Sharma MC, Sinha SP, Neva FA, Saran R (1995) Indian kala-azar caused by Leishmania tropica. Lancet 345:959–961
Schlein Y, Muller GC (2010) Experimental control of Phlebotomus papatasi by spraying attractive toxic sugar bait (ATSB) on vegetation. Trans R Soc Trop Med Hyg 104:766–771. doi:10.1016/j.trstmh.2010.08.014
Silva F, Gomes R, Prates D, Miranda JC, Andrade B, Barral-Netto M, Barral A (2005) Inflammatory cell infiltration and high antibody production in BALB/c mice caused by natural exposure to Lutzomyia longipalpis bites. Am J Trop Med Hyg 72:94–98
Titus RG, Ribeiro JM (1988) Salivary gland lysates from the sand fly Lutzomyia longipalpis enhance Leishmania infectivity. Science 239:1306–1308
Titus RG, Bishop JV, Mejia JS (2006) The immunomodulatory factors of arthropod saliva and the potential for these factors to serve as vaccine targets to prevent pathogen transmission. Parasite Immunol 28:131–141. doi:10.1111/j.1365-3024.2006.00807.x
Valenzuela JG, Belkaid Y, Garfield MK, Mendez S, Kamhawi S, Rowton ED, Sacks DL, Ribeiro JM (2001a) Toward a defined anti-Leishmania vaccine targeting vector antigens: characterization of a protective salivary protein. J Exp Med 194:331–342
Valenzuela JG, Belkaid Y, Rowton E, Ribeiro JM (2001b) The salivary apyrase of the blood-sucking sand fly Phlebotomus papatasi belongs to the novel Cimex family of apyrases. J Exp Biol 204:229–237
Volf P, Tesarova P, Nohynkova EN (2000) Salivary proteins and glycoproteins in phlebotomine sandflies of various species, sex and age. Med Vet Entomol 14:251–256
WHO (2015) WHO report on global surveillance of epidemic-prone infectious diseases - leishmaniasis. WHO. http://www.who.int/csr/resources/publications/CSR_ISR_2000_1leish/en/. Accessed 15 April 2015
Author information
Authors and Affiliations
Corresponding author
Additional information
Rami M. Mukbel and Rehab H. Khasharmeh contributed equally to this work.
Rights and permissions
About this article
Cite this article
Mukbel, R.M., Khasharmeh, R.H., Hijjawi, N.S. et al. Human immune response to salivary proteins of wild-caught Phlebotomus papatasi . Parasitol Res 115, 3345–3355 (2016). https://doi.org/10.1007/s00436-016-5094-2
Received:
Accepted:
Published:
Issue Date:
DOI: https://doi.org/10.1007/s00436-016-5094-2